- Department of Cardiology, The Third People’s Hospital of Chengdu, Affiliated Hospital of Southwest Jiaotong University, Chengdu Cardiovascular Disease Research Institute, Chengdu, Sichuan, China
Background: A novel non-contact system for remote parameter testing and reprogramming offers an alternative method for assessing device parameters during cardiac implantable electronic devices (CIEDs) implantation without the need for physical contact with the manufacturer's clinical service technician. The safety and feasibility of using this system in CIEDs implantation procedures remains to be determined.
Objective: Evaluate the safety and feasibility of remote parameter testing in CIEDs implantation procedures.
Methods: A single center, randomized, open-label, non-inferiority trial (ChiCTR2200057587) was conducted to compare the two approaches for interrogating CIEDs during implantation procedures: routine interrogation performed by on-site technicians or remote interrogation performed by technicians using the 5G-Cloud Technology Platform. Patients aged ≥18 years and elected to receive CIEDs were eligible for inclusion. The primary endpoint was the completion rate of the parameter test. Safety and efficiency were evaluated in all randomly assigned participants.
Results: A total of 480 patients were finally enrolled and were randomly assigned to routine group (n = 240) or remote group (n = 240). The primary endpoint was achieved by 100% in both groups (P = 0.0060 for noninferiority). The parameters of sensing, threshold, and impedance regarding the right atrium, right ventricle, and left ventricle had no statistical significance between the two groups (P > 0.05). Procedure time, parameter testing time, and both duration and dose of x-ray irradiation were not significantly different between the two groups (P > 0.05). Shut-open door frequency was significantly higher in the routine group than the remote group [6.00 (4.00, 8.00) vs. 0, P < 0.0001]. Notably, no clinical or technical complications were observed in the remote group.
Conclusions: Remote parameter testing is safe and feasible across various devices implantation procedures. The utilization of remote parameter testing and reprogramming could represent an innovative approach to improve healthcare accessibility and unlock the full potential of secondary centers in managing CIEDs.
The Registration Identification: ChiCTR2200057587.
Introduction
Cardiac implantable electronic devices (CIEDs) are the most effective means of treating and diagnosing several types of arrhythmias and heart failure (1). To ensure the safety and efficacy, clinicians must evaluate the parameters of the device during the procedure. During routine CIEDs implantation procedures, parameter testing and reprogramming are performed by the manufacturer's clinical service technician in the catheterization laboratory while physically present with the patient. This process inevitably exposes the technician to x-ray radiation, which can potentially cause harmful health effects such as carcinogenesis, gene mutation, and cataracts. Due to the outbreak of the COVID-19 pandemic, the manufacturer's clinical service technicians were banned from entering the catheterization laboratory and ward as a precautionary measure to reduce the potential risk of cross-infection. Meanwhile, the rates of CIEDs implantations declined significantly, especially during the early pandemic wave (2–6). Resolving these issues of parameter testing and programming is absolutely imperative.
A novel real-time system for remote parameter testing and reprogramming, based on China Telecom's 5G-Cloud Technology Platform (5G-CTP), offers an alternative method for assessing device parameters during CIEDs implantation without the need for physical contact with the manufacturer's clinical service technician (7). Recently, the use of this novel noncontact system during CIEDs implantation procedures has been reported in a small, non-randomized study against the background of the global COVID-19 pandemic (8). The significance of this system in clinical practice warrants further investigation. Therefore, the aim of this study was to evaluate the feasibility and safety of using this system in CIEDs implantation procedures through a randomized controlled trial.
Methods
Study design
This single center, randomized, open-label study (ChiCTR2200057587) was conducted at the Third People's Hospital of Chengdu (Sichuan Province, China) comparing two approaches for interrogating and reprogramming CIEDs during implantation procedures: routine interrogation and reprogramming performed by on-site technicians (Routine group) or remote interrogation and reprogramming performed by technicians using the 5G-Cloud Technology Platform (Remote group). The study was approved by the institutional review board committee (CSY-2022-S-11) and adhered to Helsinki Declaration guidelines.
The indications for CIEDs implantation were evaluated based on temporal guidelines. Patients were eligible for inclusion if: they were ≥18 years, elected to receive CIEDs manufactured by Abbott (Chicago, Illinois, USA), and provided written informed consent. A total of 480 patients between April 2022 and May 2023 were enrolled and were randomly assigned to either the Routine or Remote group in a 1:1 ratio.
Remote interrogation and reprogramming solution
The 5G-CTP consists of three primary components. First, an off-site device with 5G cloud follow-up software (China Telecom Corporation Limited Shanghai Branch, Shanghai, China). Second, a Merlin Patient Care System Programmer Model 3650 (St. Jude Medical Inc., Minnesota, USA), which is externally connected to the 5G data transmit module (China Telecom Corporation Limited Shanghai Branch, Shanghai, China) in the catheterization laboratory by USB cables. Both surface electrocardiograph and intracardiac signals are displayed on the programmer. Third, a remote service system, which is deployed on cloud servers (China Telecom Cloud, China). The 5G-CTP is a research tool that enables technicians to remotely test and reprogram CIEDs in real-time without the need for physical presence with the patient through an internet connection or mobile wireless network (Figure 1).
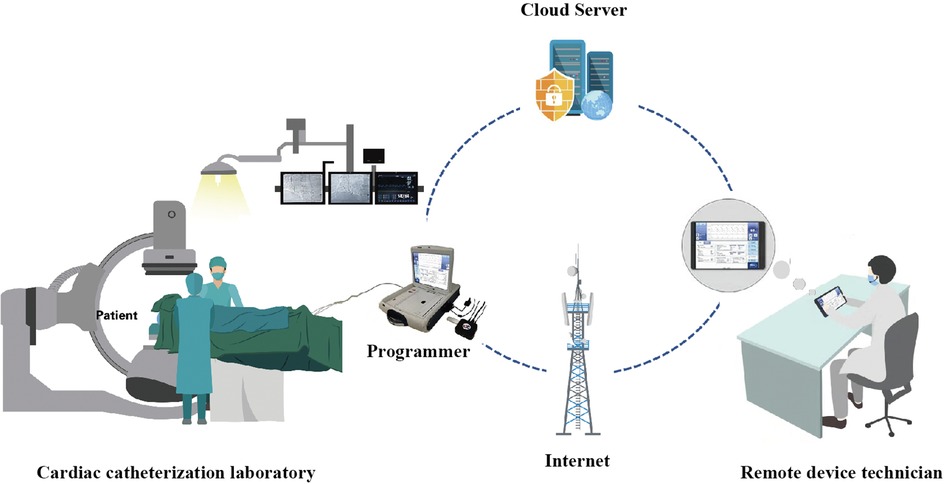
Figure 1. An overview of the utilization of the 5G-cloud technology platform for remote parameter testing. The 5G-cloud technology platform for remote parameter testing, consisting of a 5G remote support terminal connected to the programmer externally, a PAD equipped with a 5G-cloud follow-up application, and a cloud-based remote service system. The 5G remote support terminal connects directly to the 5G-cloud follow-up application via the internet. No network or software is required for the on-site programmer. Remote control of the on-site programmer can be realized by simply connecting to the 5G remote support terminal and using simulated mouse and keyboard information. No direct data interact between the computer and the on-site programmer. This system enables clinical device technicians to provide real-time remote parameter testing and reprogramming for cardiac implantable electronic devices in primary medical and healthcare institutions lacking device specialists.
The 5G-CTP has implemented specific security procedures to ensure patient safety and cybersecurity. Firstly, an authorized technician must undergo a two-step verification process to log into the 5G-cloud follow-up application. Step 1 requires logging into a designated account with a password, while Step 2 involves using an access password for second verification to establish a remote connection for the designated device. Secondly, it employs a 2048-bit Rivest-Shamir-Adleman asymmetric key exchange, autonomous peer-to-peer data transfer protocol, and anti-cracking measures, building on the advanced encryption standard encryption mechanism to safeguard communication and patient security. Thirdly, the servers are deployed in server rooms equipped with multilayer firewalls, customized antivirus scanning, vulnerability scanning, and intrusion detection to ensure data security. Fourthly, the entire remote operation process is saved through screen recording generation, which allows auditors to review the logs later on. Fifthly, in the event of a communication interruption between the on-site programmer and the remote technician's device, the CIEDs will revert back to the original settings. Furthermore, real-time video and audio communication between technicians and onsite medical staff enhances the security of remote parameter testing and programming. Before commencing the research, all enrolled technicians underwent specialized training for this protocol.
Data collection
The data on patient's sociodemographic characteristics, medical history, smoking status, laboratory examination, medical information, and procedural information were obtained from the electronic medical records. Medical history data included a history of conditions such as coronary artery disease, stroke, atrial fibrillation, diabetes mellitus, hypertension, dyslipidemia, chronic obstructive pulmonary disease, and chronic renal dysfunction.
The primary hypothesis was that remote interrogation and reprogramming solution is non-inferior to routine methods in achieving freedom from primary endpoints, with a 5% noninferiority margin. Following indices related to CIEDs implantation procedures were gathered: (1) the completion rate of the parameter test; (2) pacing threshold; (3) sensing amplitude; (4) lead impedance; (5) the proportions of the above parameters in the recommended range; (6) duration of intraoperative testing; (7) duration of operation; (8) duration of x-ray irradiation that device specialists received during the testing process; (9) number of door openings related to parameter testing. Pacing parameters were recommended as follows (sensing amplitude/threshold/impedance): right atrium lead: >2.00 mV/<1.50 V/300–1,500 Ω; right ventricular lead: >5.00 mV/<1.00 V/300–1,500 Ω; left ventricular lead: >5.00 mV/<2.00 V/300–1,500 Ω.
Statistical analyses
It was estimated that 121 patients per study group are needed to support the primary hypothesis with a power of 80% and α level of 0.05. Continuous data were expressed as either mean ± standard deviation (SD) or median [interquartile range (IQR)] for normally or skewed distributed variables, respectively. Statistical analysis between groups employed either Student's t-test or Mann–Whitney U-test. Normality of distribution was determined via Kolmogorov–Smirnov and Shapiro–Wilk tests. Categorical variables were presented as numbers (percentages) and compared using the chi-squared test or Fisher's exact test, as appropriate. A two-tailed P value < 0.05 was considered significant. All statistical analyses were conducted using SPSS software (version 26.0, SPSS, Inc., Chicago, IL, USA) and Prism version 8.0 (GraphPad software, San Diego, CA, USA).
Results
A total of 480 patients were finally enrolled and were randomly assigned to routine group (n = 240) or remote group (n = 240). Baseline characteristics of the individuals stratified by randomization group are shown in Table 1. The primary endpoint was achieved by 100% in both groups (P = 0.0060 for noninferiority). The parameters of sensing, threshold, and impedance regarding the right atrium, right ventricle, and left ventricle had no statistical significance between the two groups (Table 2, all P > 0.05). The parameter values of the two groups were all within the recommended ranges.
Shut-open door frequency was significantly higher in the routine group than the remote group [6.00 (4.00, 8.00) vs. 0, P < 0.0001]. Procedure time, parameter testing time, and both duration and dose of x-ray irradiation were not significantly different between the two groups (Table 3, P > 0.05). Notably, no clinical or technical complications were observed in the remote group. These results indicate that remote parameter testing is a safe method that does not compromise the efficiency of parameter testing during CIEDs implantation procedures.
Discussion
In this randomized, open-label trial, we found that utilizing remote parameter testing with 5G-CTP during CIEDs implantation procedures is feasible and safe across diverse clinical devices. This system holds the potential to decrease personnel contact, lower the risk of infections like COVID-19, and improve healthcare accessibility.
The sudden outbreak of the COVID-19 pandemic had a profound impact on clinical practice worldwide. The COVID-19 pandemic led to significant declines in the rates of CIEDs implantations during its initial phase. The decrease amounted to 56.50% in northeastern Spain (5), above 40% in England (9), 39.38% in Poland (10), 48% in northwestern Greece (11), 28% in the Veneto region of Italy (12), and 22.90% in Germany (3). The rates of CIEDs implantations also decreased significantly in China ranging from 15.04% to 61.49% between January and May 2020 (2). Possible reasons for the decline in the number of CIEDs implantations in the early stages are as follows: (1) The lack of experience in managing COVID-19 has led to a significant strain on healthcare resources as a result of the sudden surge in cases. Consequently, elective procedures and noncritical visits may be postponed or canceled to conserve hospital resources. (2) Governments have enacted comprehensive public health measures, such as lockdowns and stay-at-home orders, resulting in a reduction in regular medical consultations and subsequently a decline in CIEDs implantations. (3) Many patients, apprehensive about contracting the virus, may choose to forgo seeking medical services, even for severe symptoms. This fear of infection likely contributes to the decrease in CIEDs implantations. (4) To reduce the risk of cross-infection, numerous medical institutions have prohibited the access of manufacturer's clinical service technicians to the catheterization laboratory and ward.
During the COVID-19 pandemic, the utilization of telemedicine has been encouraged in order to minimize unnecessary exposure and its adoption has significantly increased (13). Remote parameter testing, facilitated by 5G-CTP, provides an alternative approach for evaluating device parameters during the implantation of CIEDs, without the need for physical contact with the manufacturer's clinical service technician. Stauning et al. found that the number of airborne colonies was strongly correlated with shut-open door frequency and the number of people present in the operating room (14). During a routine CIED implantation procedure, the manufacturer's clinical service technician is not consistently present in the catheterization laboratory but instead enters when parameter testing and reprogramming are necessary. This practice may lead to more frequent door openings, potentially raising the risk of intraoperative airborne colonies and microbial air contamination, thereby increasing the likelihood of pocket infections. Our study findings demonstrate that implementing this innovative non-contact strategy successfully decreases personnel contact, thereby minimizing the potential risks of cross-infection. This approach maintains the safety and efficacy of remote parameter testing, which is comparable to the standard method. Importantly, utilizing remote parameter testing guarantees the successful completion of CIEDs implantation procedures without compromising patient care. Additionally, clinical service technicians can now circumvent unnecessary exposure to x-ray.
A significant proportion of patients with CIEDs benefit from remote monitoring, which serves as an effective tool for managing cardiac rhythm off-site. According to current guidelines, remote monitoring is highly recommended (class I) for routine use in CIEDs patients (1, 15). However, there is a substantial underutilization of remote monitoring due to various patient-related and system-related challenges. In the context of the pandemic, remote monitoring has been strongly recommended in most circumstances to reduce nonurgent clinic visits (13). The 5G-CTP utilized in the present study not only encompasses real-time remote parameters testing but also incorporates the functionality for remote reprogramming. Therefore, the principles of remote parameter testing can be cautiously extended to the management of CIEDs follow-up, transcending geographic, social, and cultural barriers. This extension is crucial to ensure the continuity of care for CIEDs patients.
Recently, one of our previous studies found that the overall compliance with in-office visits in a single region of China was only 60.60%, highlighting a substantial need for improvement (16). Furthermore, remote monitoring remains significantly underutilized in China due to the absence of reimbursement and logistical support necessary for the effective implementation of closed-loop management. In fact, less than 10% of patients with permanent pacemakers are currently enrolled in remote monitoring services for their devices (17, 18). The security and feasibility of remote reprogramming based on 5G-CTP have been confirmed in our previous series of studies (16, 19–21). Moreover, Mariani et al. demonstrated that virtual visits were equally feasible and effective as in-person consultations, achieving high patient satisfaction among clinical electrophysiology patients amid the COVID-19 pandemic (22). Thus, we propose that remote follow-up based on 5G-CTP may be a novel service model to enhance the management of CIEDs follow-up in patients residing in underserved remote areas (7). Establishing a timely and stable connection between major regional medical centers and surrounding secondary hospitals is of great clinical significance in improving the capacity of secondary medical centers and alleviating the burden on major centers in the implantation and follow-up management of CIEDs. Utilizing 5G-CTP for remote parameter testing and reprogramming may be recommended to unlock the complete capabilities of secondary centers in the management of CIEDs.
Limitations
This study has some limitations. First, this is a single center, randomized, open-label study. The limitations in generalizability and sample size must be considered when interpreting our findings. Second, our study was limited by the small number of patients implemented with ICD or CRT devices. The CIEDs employed in this study primarily consist of standard pacemakers, representing a notable deviation from the cohort in Western countries. A multi-center, multi-population clinical study is necessary to assess the feasibility of remote parameter testing in CIEDs implantation. Third, we did not conduct follow-up, it remains uncertain whether this non-contact method has the potential to positively impact the clinical prognosis of patients with CIEDs, particularly in terms of pocket infection. Forth, the 5G-CTP is currently compatible only with Abbott (St. Jude) devices. Further exploration to extend this service model to other brands of CIED would yield significant clinical implications. Although certain limitations have been observed, to the best of our knowledge, this is the first randomized controlled trial to validate the safety and efficacy of remote parameter testing in CIEDs implantation.
Conclusions
Utilizing 5G-CTP for remote parameter testing is safe and feasible across various devices implantation procedures. The principles of remote parameter testing can also be cautiously extended to the management of CIEDs follow-up, offering an innovative approach to enhancing healthcare accessibility and unlock the full potential of secondary centers in managing CIEDs.
Data availability statement
The raw data supporting the conclusions of this article will be made available by the authors, without undue reservation.
Ethics statement
The studies involving humans were approved by the study was approved by the institutional review board committee of the Third People's Hospital of Chengdu (CSY-2022-S-11). The studies were conducted in accordance with the local legislation and institutional requirements. The participants provided their written informed consent to participate in this study.
Author contributions
SX: Data curation, Funding acquisition, Investigation, Writing – original draft. SQ: Data curation, Investigation, Methodology, Writing – original draft. LT: Investigation, Methodology, Writing – original draft. YLo: Data curation, Investigation, Methodology, Writing – original draft. YLu: Data curation, Writing – original draft. QF: Data curation, Writing – original draft. XP: Data curation, Writing – original draft. MJ: Data curation, Writing – original draft. FX: Writing – review & editing. JL: Data curation, Writing – original draft. YZ: Methodology, Writing – original draft. ZZ: Supervision, Validation, Visualization, Writing – review & editing. HL: Supervision, Validation, Visualization, Writing – review & editing. LC: Conceptualization, Funding acquisition, Supervision, Validation, Writing – review & editing.
Funding
The author(s) declare financial support was received for the research, authorship, and/or publication of this article.
This study was supported by the grant from the National Natural Science Foundation of China (31600942), the Scientific Research Project of The Third People's Hospital of Chengdu (CSY-YN-01-2023-041), the Science and Technology Department of Sichuan, China (2021YJ0215, 2023YFS0298), and Chengdu High-level Key Clinical Specialty Construction Project.
Conflict of interest
The authors declare that the research was conducted in the absence of any commercial or financial relationships that could be construed as a potential conflict of interest.
Publisher's note
All claims expressed in this article are solely those of the authors and do not necessarily represent those of their affiliated organizations, or those of the publisher, the editors and the reviewers. Any product that may be evaluated in this article, or claim that may be made by its manufacturer, is not guaranteed or endorsed by the publisher.
References
1. Slotwiner D, Varma N, Akar JG, Annas G, Beardsall M, Fogel RI, et al. HRS expert consensus statement on remote interrogation and monitoring for cardiovascular implantable electronic devices. Heart Rhythm. (2015) 12:e69–100. doi: 10.1016/j.hrthm.2015.05.008
2. Cheng CD, Zhao S, Jiang J, Lin N, Li P, Ning XH, et al. Impact of the COVID-19 pandemic on cardiac implantable electronic device implantation in China: insights from 2 years of changing pandemic conditions. Front Public Health. (2022) 10:1031241. doi: 10.3389/fpubh.2022.1031241
3. Schwab JO, Wiese J, Hauser T. The influence of the 2020 COVID-19 pandemic on the implantation rates of cardiac implantable electronic devices in Germany: changes between 2020 Q1–Q3 and 2019 Q1–Q3. Eur Heart J Qual Care Clin Outcomes. (2022) 8:104–12. doi: 10.1093/ehjqcco/qcab091
4. Tovia-Brodie O, Rav Acha M, Belhassen B, Gasperetti A, Schiavone M, Forleo GB, et al. Implantation of cardiac electronic devices in active COVID-19 patients: results from an international survey. Heart Rhythm. (2022) 19:206–16. doi: 10.1016/j.hrthm.2021.10.020
5. Arbelo E, Angera I, Trucco E, Rivas-Gándara N, Guerra JM, Bisbal F, et al. Reduction in new cardiac electronic device implantations in catalonia during COVID-19. Europace. (2021) 23:456–63. doi: 10.1093/europace/euab011
6. Scott M, Baykaner T, Bunch TJ, Piccini JP, Russo AM, Tzou WS, et al. Contemporary trends in cardiac electrophysiology procedures in the United States, and impact of a global pandemic. Heart Rhythm O2. (2023) 4:193–9. doi: 10.1016/j.hroo.2022.12.005
7. Tong L, Long Y, Xiong S, Li J, Huang W, Liu H, et al. Application of postoperative remote follow-up of CIED based on the 5G cloud technology support platform in areas with underdeveloped medical resources. Front Cardiovasc Med. (2022) 9:894345. doi: 10.3389/fcvm.2022.894345
8. Zhang H, Gao H, Liu X, Mu X, Shi X. A novel non-contact remote interrogate system based on 5G telecommunication technique during cardiac implantable electrical devices implantation against the background of the global COVID-19 pandemic. Chin Med J (Engl). (2023) 136:623–5. doi: 10.1097/CM9.0000000000002069
9. Leyva F, Zegard A, Okafor O, Stegemann B, Ludman P, Qiu T. Cardiac operations and interventions during the COVID-19 pandemic: a nationwide perspective. Europace. (2021) 23:928–36. doi: 10.1093/europace/euab013
10. Filipecki A, Orszulak M, Tajstra M, Kowalski O, Skrzypek M, Kalarus Z, et al. Cardiac implantable electronic devices procedures and their recipients characteristic during COVID-19 pandemic: 3.8 million population analysis. Cardiol J. (2022) 29:27–32. doi: 10.5603/CJ.a2021.0170
11. Bechlioulis A, Sfairopoulos D, Korantzopoulos P. Impact of COVID-19 pandemic on cardiac electronic device implantations in northwestern Greece. Am J Cardiovasc Dis. (2021) 11:489–93. PMID: 34548948
12. Migliore F, Zorzi A, Gregori D, Del Monte A, Falzone PV, Verlato R, et al. Urgent pacemaker implantation rates in the veneto region of Italy after the COVID-19 outbreak. Circ Arrhythm Electrophysiol. (2020) 13:e008722. doi: 10.1161/CIRCEP.120.008722
13. Lakkireddy DR, Chung MK, Gopinathannair R, Patton KK, Gluckman TJ, Turagam M, et al. Guidance for cardiac electrophysiology during the COVID-19 pandemic from the heart rhythm society COVID-19 task force; electrophysiology section of the American college of cardiology; and the electrocardiography and arrhythmias committee of the council on clinical cardiology, American heart association. Circulation. (2020) 141:e823–31. doi: 10.1161/CIRCULATIONAHA.120.047063
14. Stauning MT, Bediako-Bowan A, Andersen LP, Opintan JA, Labi AK, Kurtzhals JAL, et al. Traffic flow and microbial air contamination in operating rooms at a major teaching hospital in Ghana. J Hosp Infect. (2018) 99:263–70. doi: 10.1016/j.jhin.2017.12.010
15. Ferrick AM, Raj SR, Deneke T, Kojodjojo P, Lopez-Cabanillas N, Abe H, et al. 2023 HRS/EHRA/APHRS/LAHRS expert consensus statement on practical management of the remote device clinic. J Arrhythm. (2023) 39:250–302. doi: 10.1002/joa3.12851
16. Tong L, Xiong S, Hou J, Li J, Qin S, Zhang Y, et al. Cloud follow-up in patients with cardiovascular implantable electronic devices: a single-region study in China. Front Cardiovasc Med. (2022) 9:864398. doi: 10.3389/fcvm.2022.864398
17. CSPE, Chinese expert consensus statement on remote interrogation and monitoring for cardiovascular implantable electronic devices. Chin J Cardiac Arrhyth. (2019) 3:187–96.
18. Chen KPZS. Remote follow-up of cardiovascular implantable electronic devices in post-epidemic era. Chin J Cardiac Arrhyth. (2021) 2:93–6.
19. Xiong S, Li J, Tong L, Hou J, Yang S, Qi L, et al. Realtime remote programming in patients carrying cardiac implantable electronic devices requiring emergent reprogramming. Front Cardiovasc Med. (2022) 9:871425. doi: 10.3389/fcvm.2022.871425
20. Chen X, Liu HX, Tong L, Yang SQ, Qi LY, Xiong SQ, et al. Clinical use of real-time remote programming in pacemakers during the COVID-19 pandemic: a case report. Pacing Clin Electrophysiol. (2022) 45:815–7. doi: 10.1111/pace.14461
21. Long Y, Xiong S, Tong L, Li J, Luo Y, Huang W, et al. The real-time remote testing and programming of cardiac implantable electronic devices: a case series report. Front Cardiovasc Med. (2022) 9:1010409. doi: 10.3389/fcvm.2022.1010409
Keywords: cardiac implantable electronic devices, remote testing, remote interrogation, remote reprogramming, implantation, COVID-19
Citation: Xiong S, Qin S, Tong L, Long Y, Luo Y, Feng Q, Peng X, Jiang M, Xiong F, Li J, Zhang Y, Zhang Z, Liu H and Cai L (2024) The clinical use of remote parameter testing during cardiac implantable electronic devices implantation procedures: a single center, randomized, open-label, non-inferiority trial. Front. Cardiovasc. Med. 11:1364940. doi: 10.3389/fcvm.2024.1364940
Received: 3 January 2024; Accepted: 5 March 2024;
Published: 22 March 2024.
Edited by:
Constantinos Bakogiannis, Aristotle University of Thessaloniki, GreeceReviewed by:
Carlo Lavalle, Sapienza University of Rome, ItalyGermanas Marinskis, Vilnius University, Lithuania
© 2024 Xiong, Qin, Tong, Long, Luo, Feng, Peng, Jiang, Xiong, Li, Zhang, Zhang, Liu and Cai. This is an open-access article distributed under the terms of the Creative Commons Attribution License (CC BY). The use, distribution or reproduction in other forums is permitted, provided the original author(s) and the copyright owner(s) are credited and that the original publication in this journal is cited, in accordance with accepted academic practice. No use, distribution or reproduction is permitted which does not comply with these terms.
*Correspondence: Zhen Zhang zhangzhen@swjtu.edu.cn Hanxiong Liu lhanx@126.com Lin Cai clin63@hotmail.com
†These authors have contributed equally to this work and share first authorship
‡These authors have contributed equally to this work and share senior authorship