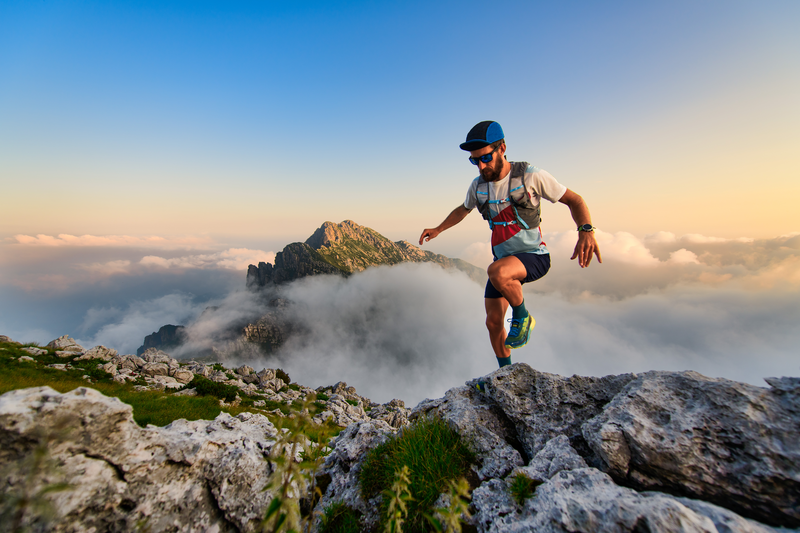
94% of researchers rate our articles as excellent or good
Learn more about the work of our research integrity team to safeguard the quality of each article we publish.
Find out more
ORIGINAL RESEARCH article
Front. Cardiovasc. Med. , 02 April 2024
Sec. Cardiac Rhythmology
Volume 11 - 2024 | https://doi.org/10.3389/fcvm.2024.1362903
This article is part of the Research Topic Atrial Fibrillation: Selection of Management Strategy and Evaluation of Outcomes View all 39 articles
Background: Obesity is a risk factor for atrial fibrillation (AF). Data regarding left atrial (LA) remodeling in obese patients are scarce. Whether obesity favors AF recurrence after catheter ablation (CA) is still controversial. We assessed the distribution of epicardial atrial fat on computed tomography (CT), LA bipolar voltage, low-voltage zone (LVZ) extent, and the outcome of voltage-guided ablation of persistent AF in obese and non-obese patients.
Methods: A total of 139 patients with persistent AF undergoing a first voltage-guided ablation were enrolled and divided into two groups: 74 were non-obese and 65 were obese. Epicardial adipose tissue (EAT) was assessed on a CT scanner. LA endocardial voltage maps were obtained using a 3D mapping system in sinus rhythm. LVZ was defined as a bipolar peak-to-peak voltage amplitude <0.5 mV.
Results: LA volume, voltage, and EAT amount were similar in the two groups. LVZ was less frequent in obese patients [12 (18.8%) vs. 26 (35.1%), p = 0.05], particularly on the anterior wall. The posterior and lateral EATs were correlated with posterior and lateral LVZ extent, respectively, in obese patients. After 36 months of follow-up, the AF-free survival rate was similar. Lateral EAT [odds ratio (OR) 1.21, 95% confidence interval (CI) 1–1.4, p = 0.04] and P-wave duration (OR 1.03, 95% CI 1–1.05, p = 0.03), but not body mass index (BMI), were predictors of AF recurrence after CA.
Conclusion: In obese patients, LVZ was less marked than in non-obese patients with similar LA volumes, voltage, and EAT amounts. In obese patients, posterior and lateral EATs were correlated with posterior and lateral LVZ extents. Obese patients had a similar and favorable 36-month outcome after AF ablation. BMI was not predictive of AF recurrence.
Obesity is associated with an increased cardiovascular mortality and morbidity (1). Several studies have demonstrated a strong association between obesity and atrial fibrillation (AF) occurrence (2). For every 5 kg/m2 increase in body mass index (BMI), the risk of AF could increase from 10% to 30% (3). Obesity has been identified as an independent risk factor for AF (2). A stable weight loss ≥10% in obese patients with AF is associated with a decrease in both AF recurrence and burden, and an improvement in AF symptom severity (4, 5). Recently, epicardial adipose tissue (EAT) extent has been associated with AF incidence and severity (6, 7). The Framingham Heart Study indicated that higher pericardial fat volume was associated with a nearly 40% higher odds of prevalent AF (6). Batal et al. reported that patients with persistent AF had a significantly thicker posterior EAT associated with increased AF burden independent of age, BMI, or left atrial (LA) area (8). Finally, Wong et al. showed that the patients with an extensive EAT had early recurrence after AF catheter ablation (CA) (7).
This EAT, located between the visceral pericardium and epicardium surface, is considered a metabolically active tissue. In a model of adult rat, human epicardial, but not subcutaneous adipose, tissue secretome could induce atrial fibrosis, a local effect mediated by activin A, an adipo-fibrokine (9). In addition, subepicardial fatty infiltration was also observed in patients with AF with abundant EAT on histological sections. Haemers et al. observed that these fatty infiltrates could progressively become fibrosis affecting subepicardial areas of the atrial myocardium (10). Low-voltage zones (LVZ) recorded with LA three-dimensional (3D) mapping systems are a powerful predictor of recurrence after AF ablation (11). In a model of obese sheep, LA enlargement, conduction abnormalities, and fractionated electrograms were associated with a reduced posterior LA endocardial voltage (12). In obese humans, data on electrophysiological remodeling are scarce. Whether obese patients display more EAT and associated LVZ is still discussed. AF recurrence after catheter ablation in obese patients also remains debated.
The purpose of our study was to evaluate the regional epicardial atrial fat distribution and assess the extent of LVZ and LA regional bipolar voltage amplitude in a cohort of obese patients with persistent AF compared to a cohort of non-obese patients. The 36-month outcome of LA voltage-guided substrate ablation in addition to pulmonary vein isolation (PVI) and predictors of AF recurrence after CA were also assessed.
This prospective observational study enrolled 139 consecutive patients presenting for a first persistent AF catheter ablation in our institution. Patients without LA voltage maps obtained in sinus rhythm (SR) and those with structural heart disease were excluded (Figure 1).
Figure 1. Flow chart of the study. Among 305 patients admitted for initial PAF ablation between November 2017 and December 2020, 139 (45.6%) patients met the inclusion criteria. 115 patients (37.7%) had no LA voltage mapping in sinus rhythm and 51 (16.7%) were excluded from analysis due to structural heart disease. VHD, valvular heart disease; HCM, hypertrophic cardiomyopathy; ICM, ischemic cardiomyopathy; DCM, dilated cardiomyopathy; PAF, persistent atrial fibrillation.
Structural heart disease was defined as a previous diagnosis of ischemic heart disease, valve dysfunction (≥moderate), or primary myocardial structural disease including dilated cardiomyopathy and hypertrophic cardiomyopathy. Obesity was defined as BMI ≥30 kg/m2. The cohort was divided into two groups: non-obese [with a BMI <30 kg/m2 (n = 74)] and obese [with a BMI ≥30 kg/m2 (n = 65)]. Patient demographics and baseline clinical characteristics were collected. The study protocol was approved by the institutional review board of Strasbourg University (CE-2023-113). All patients have given their written informed consent for the ablation and participation in this study. Our obese patients were aware of weight loss to improve the results of AF ablation. Therefore, we would advise them to change their lifestyle (diet and exercise) for weight loss before and after AF ablation.
Images were acquired using a 320-row CT scanner (Aquilion ONE Genesis; Canon Medical Systems). Image analysis was performed on a dedicated workstation (AW4.6 workstation; GE Healthcare). All CT scanners were reconstructed in three dedicated cardiac planes, centered on a line (using body imaging planes) from the cardiac apex to the middle of the mitral valve.
EAT was defined as the adipose tissue between the surface of the myocardium and the epicardium. EAT thickness was measured by the same radiologist in millimeters, using a manual 2D caliper, by measuring in the area of maximum thickness the distance between every LA wall and anatomical landmarks, such as the esophagus, for the posterior wall (Figure 2).
Figure 2. EAT measurements were obtained using a cardiac CT scanner. All CT scanners were reconstructed in three dedicated cardiac planes, centered on a line (using body imaging planes) from the cardiac apex to the middle of the mitral valve: short axis plane: perpendicular to true long axis at the level of mid left ventricle; horizontal long axis plane (four-chamber view): horizontal plane perpendicular to short axis; vertical long axis plane (two-chamber view): vertical plane orthogonal to short axis. EAT was defined as the adipose tissue between the surface of the myocardium and the epicardium. EAT thicknesses were measured by the same radiologist in mm, by manual 2D caliper (small yellow line), as the distance between every LA wall and anatomical landmarks: EAT thickness between anterior LA wall and ascending aorta (A); EAT thickness between posterior LA wall and descending thoracic aorta (B); EAT thickness between posterior LA wall and right pulmonary hila (C); EAT thickness between lateral LA wall and visceral pleura of left lung (D); EAT thickness between posterior LA wall and esophagus (E); EAT thickness between periauricular lateral LA wall and mitral root (F).
Patients were efficiently anticoagulated for more than 3 weeks. The antiarrhythmic drugs (AADs) were interrupted for ≥5 half-lives before catheter ablation. Thus, amiodarone was discontinued 3 weeks before the procedure. Electrophysiological studies and catheter ablation procedures were performed under general anesthesia using a 3D electroanatomical mapping (3D-EAM) system (CARTO 3, Biosense Webster, Diamond Bar, CA, USA, or EnSite Velocity, Abbott, St Paul, MN, USA) and a deflectable decapolar circular mapping catheter (Lasso catheter of variable diameter size (15–25 mm), interelectrode spacing 6 mm (Biosense Webster, Diamond Bar, CA, USA, or a spiral multipolar pulmonary vein catheter, Afocus II, diameter 20 mm, electrode spacing 5 mm, Abbott, St Paul, MN, USA). A transesophageal echocardiography was performed at the beginning of the procedure, to both exclude any thrombi in the left atrial appendage (LAA) and guide the transseptal puncture.
LA endocardial voltage mapping was performed in SR before radiofrequency ablation (Figures 3A,B). Endocardial contact during point acquisition was validated by a stable contact signal for >2 beats. All points recorded in SR were analyzed to exclude mechanically induced premature beats. Any area with an abnormal voltage resulting from inadequate contact between the circular catheter and LA tissue was reanalyzed with a 4-mm irrigated contact-force ablation catheter (ThermoCool® SmartTouch®; Biosense Webster, Diamond Bar, CA, USA, or Tacticath®; Abbott, St Paul, MN, USA). The LA was divided into six anatomical regions: posterior; anterior; septal; lateral; LAA; and inferior. The roof was part of the anterior region as previously described (13). The bipolar voltage amplitude was recorded for every point and within each individual region. The median LA and regional bipolar voltage amplitude measurements were calculated. Left atrial intracavitary volume (LAIV) excluding LAA was obtained for each patient after LA anatomic reconstruction and was expressed in milliliters. The left atrial intracavitary volume index (LAIVI) corresponded to the LAIV indexed to the body surface (expressed in ml m2). LVZ was defined as sites of >3 adjacent low-voltage points with a bipolar peak-to-peak voltage amplitude of <0.5 mV (14) and covering >5% of the LA surface area (LVZ surface/LA surface >5% without LAA orifice, the pulmonary venous antral region, and mitral valve). This threshold value corresponds to the lowest degree of atrial fibrosis detected using LGE-MRI (15). LVZ extent was categorized as stage I (no or discrete LVZ, ≤5%), II (mild, >5% to ≤20%), III (moderate, >20% to ≤35%), and IV (severe, >35%) according to the UTAH fibrosis classification (15). The surface (cm2) of each atrial region and of the LVZ within each atrial region was measured using the 3D-EAM software.
Figure 3. Left atrial bipolar voltage map obtained in sinus rhythm with Lasso™ NAV from Biosense Webster with visualization of low-voltage zones at the anterior wall and septum (A) and the same map with ablation lesions colored in red (B).
Irrigated radiofrequency energy was applied point by point with a target temperature of 43°C, infusion rate of 17 mL/mn via the irrigated ablation catheter, and maximal power limit of 35 W (30–35 W for the anterior wall and 20–25 W for the posterior wall) using the Biosense Webster ablation index by targeting 450–500 and 350–400 for anterior and posterior segments, respectively. For the Abbott's procedure, the lesion size index (LSI) was used by targeting 5.5 and 4.5 for the anterior and posterior walls, respectively. These same target values were applied to all patients without differentiating between the two groups. PVI was widely encircled in all patients with point-by-point applications using a 4-mm irrigated contact-force ablation catheter (ThermoCool® SmartTouch®; Biosense Webster, Diamond Bar, CA, USA, or Tacticath®; Abbott, St Paul, MN, USA) to create the contiguous lesions. For stage I patients, only circumferential PVI was performed, whereas an additional LVZ-guided substrate ablation was carried out for stage II–IV patients. Linear ablation across LVZs was performed when the LVZ ablation area could be considered a critical isthmus site for potential macro-reentrant tachycardia. Thus, when identifying large LVZs close to the roof or in the anterior LA, linear lesions were created to connect the LVZ ablation area to anatomical obstacles to prevent roof-dependent or peri-mitral atrial flutter. Linear lesions were also performed to isolate large LVZs from the rest of the healthy atrium, such as a posterior box by a roofline and an infero-posterior line. Atrial burst pacing to refractoriness was performed. Inducible atrial tachycardias (AT) were ablated with AT termination without reducibility. No additional ablation was performed in cases of induced AF.
After the catheter procedure, AADs were continued in all patients for at least 3 months to prevent early arrhythmia recurrences. Patients were reviewed by their cardiologist at 3 months and then every 6 months until the 42nd month. At each visit, 12-lead electrocardiogram (ECG) and routine 24-h ECG Holter monitoring were recorded to document the recurrence of atrial arrhythmias (AAs), which was defined as the presence of any documented atrial fibrillation, atrial flutter, and atrial tachycardia lasting over 30 s. The blanking period corresponded to arrhythmic episodes occurring within the first 3 months and was not included in the evaluation of final success rates. AADs were gradually discontinued at 3–6 months after ablation in the absence of AA recurrence at the physician's discretion.
Categorical variables were presented as absolute values and percentages. A statistical analysis of the categorical variables between the two groups was performed using a chi-square test or Fisher's exact test. The Shapiro–Wilk test was used to determine the Gaussian distribution for each quantitative variable. Continuous variables were expressed as means with standard deviations (SD) for normally distributed data. The variables with a non-normally distribution were presented as medians and interquartile ranges (25th–75th interquartile range). The differences between the continuous variables were analyzed for statistical significance using the Student’s t-test or the Wilcoxon test, depending on data distribution.
The relationships between continuous variables were analyzed using Pearson’s correlation coefficients for parametric data. Kaplan–Meier survival curves were used to analyze freedom from AA recurrence after a single AF catheter procedure between obese patients and non-obese patients using the log-rank test with Bonferroni correction. Binominal logistic regression was applied to calculate the odds ratio (OR) and 95% confidence interval (CI) of independent variables associated with AA recurrence. Variables selected for testing in the multivariate analysis were those with p < 0.1 in the univariate analysis. All statistical analyses were performed using SPSS statistical software, version 23.0 (IBM Corp.). A two-tailed p-value <0.05 was considered statistically significant.
Demographic and clinical characteristics of the patients are shown in Table 1. The patients were mainly men (69.8%). Compared to non-obese patients, obese patients were significantly younger [62 (56–69) vs. 66.5 (62–72) years, p < 0.01] with more cardiovascular risk factors: hypertension [49 (75.38%) vs. 37 (50%), p < 0.01]; diabetes mellitus [20 (30.77%) vs. 6 (8.11%), p < 0.01]; obstructive sleep apnea (OSA) [26 (40%) vs. 16 (21.62%), p < 0.03]. Left ventricular ejection fraction was normal in most patients. There was no significant difference in drug treatments between the two groups. Paroxysmal AF history was less frequent in the obese group [15 (23.08%) vs. 34 (45.95%), p < 0.01]. Indexed to body surface or not, LA intracavitary volume was similar in the two groups [64.7 ml/m2 (57.2–73.4) vs. 60.5 ml/m2 (51.6–68.6), p = 0.07]. At the beginning of the procedure, 23/65 (35.4%) obese patients and 21/77 (28.4%) non-obese patients were in AF (p = 0.48).
No differences could be seen between the obese and non-obese groups regarding EAT thickness in the left atrial posterior wall (LA-descending thoracic aorta, LA-esophagus, LA-right pulmonary hilum) nor in the anterior, lateral, or inferior walls (Table 2).
Of the procedures, 73 (52.5%) were performed with the Afocus II. The 66 (47.5%) remaining procedures were performed with the Lasso™ NAV. No difference was found in the use of the type of mapping catheter between the obese and non-obese groups [Abbott: 36 (55%) vs. 37 (50%), p = 0.64 and Biosense Webster: 29 (45%) vs. 37 (50%), p = 0.64].
The number of mapping points per patient was similar in the two groups [998 (673–1,662) vs. 1,031 (566–2,003), p = 0.83] (Table 3). The global LA bipolar voltage was not significantly different in the obese group compared to the non-obese group [2.2 (1.4–2.6) mV vs. 1.8 (1.2–2.7) mV, p = 0.29] (Table 3). When comparing LA bipolar voltage amplitudes according to atrial regions, there was also no difference in bipolar voltage amplitude in the anterior [1.7 (1.1–2.6) mV vs. 1.6 (1–2.4) mV, p = 0.25], septal [1.6 (1–2.3) mV vs. 1.4 (0.8–2.2) mV, p = 0.29], posterior [2.3 (1.3–3.2) mV vs. 1.9 (1.1–2.8) mV, p = 0.22], inferior [2 (1.2–2.7 mV) vs. 1.8 (1.1–2.6 mV), p = 0.34], or lateral [2.3 (1.3–3.4 mV) vs. 2.1 (1.2–2.8 mV), p = 0.29] regions nor the LAA [2.9 (1.8–3.9 mV) vs. 2.6 (1.7–3.5, mV p = 0.13] between the obese and non-obese patients.
Table 3. Median regional distribution of bipolar voltage amplitudes of the non-obese and obese groups.
LVZs were observed in 27.3% of patients in the whole cohort. LVZs were less frequent in the obese group compared to the non-obese group [12% (18.8%) vs. 26% (35.1%), p = 0.05] (Table 4).
When analyzing LVZs according to the atrial region, anterior LVZs were less frequently seen in the obese group [13% (20.3%) vs. 28% (37.8%), p = 0.04]. In the other atrial regions, no significant difference in LVZs could be observed between the two groups. LA surface indexed-LVZ extents were not significantly different between the two groups [8.2% (3.4%–22.6%) vs. 14% (5.5%–33%), p = 0.17]. LA surface indexed-LVZ extents according to regional atrial surface were also not significantly different, even if the obese group presented less extent, particularly in the anterior, septal, inferior, and lateral regions [18.3% (9.2%–32%) vs. 29.8% (13.9%–52.3%), p = 0.29; 11.4% (8.4%–33.1%) vs. 18.9% (14%–41.4%), p = 0.18; 9.8% (6.2%–14.5%) vs. 12.3% (7.7%–27.2%), p = 0.84; 10.2% (9.3%–35.7%) vs. 26.4% (15.6%–68.9%), p = 0.74, respectively].
To analyze the relationship between EAT thickness and LVZ extent indexed to regional atrial surface, the Pearson correlation coefficients were calculated, as shown in Table 5. No significant correlation was found in non-obese patients. In obese patients, the EAT thickness of LA-esophagus and the indexed posterior LVZ extent showed a positive Pearson correlation coefficient of 0.45 (p = 0.0001) (Table 5). LA-mitral isthmus was also modestly correlated to indexed lateral LVZ extent (Pearson coefficient 0.26, p = 0.04). There was no significant correlation between LVZ extent and EAT in the other regions.
Table 5. Correlation between periatrial fat thicknesses values and percentage of regional LVZ extent in the non-obese patients (A) and in the obese patients (B).
All PVs were successfully isolated during CA. PVI alone was performed in 88 (63.3%) patients of the overall cohort, while the remaining 51 (36.7%) patients had an additional LVZ-guided substrate ablation. Compared to non-obese patients, PVI alone was more frequently performed in obese patients [47 (72.3%) vs. 41 (55.4%), p = 0.06] but the difference was not significant. CTI ablation before or during the procedure was performed in the same proportion in the two groups [13 (17.6%) vs. 13 (20%), p = 0.88]. Total RF duration and fluoroscopy time were also similar (31.9 ± 10.5 min vs. 32.1 ± 11.8 min, p = 0.76; 25.1 ± 11.2 min vs. 24.9 ± 9.4 min, p = 0.66) (Supplementary Table S1).
After a follow-up of 38.1 months (range 34.8–41.4), AAs recurred in 37/138 (26.8%) of the overall cohort after CA. The Kaplan–Meier survival curves are shown in Figure 4. There was no significant difference in AA-free survival rate after CA in the obese and non-obese groups (Log-rank test, p = 0.544). The percentage of patients remaining free from AF/AT 12 months after CA was 93% ± 3% in the obese group and 86% ± 4% in the non-obese group (Figure 4). At 24 months after ablation, 80% ± 6% of the obese group and 75% ± 5% of the non-obese group remained free from AF/AT. Finally, 77% ± 6% of the obese group and 64% ± 7% of the non-obese group remained free from AF/AT 36 months after CA.
Figure 4. Kaplan–Meier survival curves showing the cumulative AAS recurrence-free survival rates in the obese and non-obese patients after a single procedure.
Among patients without AA recurrence at 12 months, AADs were discontinued in 74.4% of the whole cohort: 68.3% in the obese group and 80% in the non-obese group (p = 0.2).
Finally, we found no differences in AA-free survival rate after one procedure between obese patients who received PVI alone and those with additional LVZ ablation (log-rank test, p = 0.712). The percentage of obese patients remaining free of AF/AT after 24 months was 77% ± 8% of those who received PVI alone and 76% ± 13% of those who received additional LVZ ablation (Supplementary Figure S1). For non-obese patients, no differences in AA-free survival rate were observed between PVI alone and additional LVZ ablation (log-rank test, p = 0.548). The percentage of non-obese patients remaining free of AF/AT after 24 months was 68% ± 8% of those who received PVI alone and 75% ± 9% of those who received additional LVZ ablation (Supplementary Figure S2).
During follow-up, we did not observe differences in body weight [102 (94–112) kg vs. 100 (91–112) kg, p = 0.15] and BMI [33 (31–37) kg/m2 vs. 32 (31–36) kg/m2, p = 0.2] in the obese group before and 1 year after AF ablation. An initial weight loss of ≥10% resulted in only 7.7% of obese patients with persistent AF. Of these obese patients, 30% had even gained excess weight at the 1-year follow-up.
To evaluate the predictors of AA recurrence after a single CA procedure, univariate and multivariate analyses were carried out in both the obese and the whole cohorts.
In the obese population, P-wave duration (PWD), LA indexed intracavitary volume, and LA-mitral isthmus were chosen as variables (p < 0.1) for a multivariate analysis, but none of them was a significant predictor of AF recurrence (Table 6A).
Table 6. Univariate and multivariate analyses for prediction of AAs recurrence after catheter ablation in the obese cohort (A) and in the whole cohort (B).
In the whole cohort, obstructive sleep apnea, treatment by aldosterone receptor antagonist, PWD, EAT LA-esophagus and EAT LA-mitral isthmus were selected as variables (p < 0.10) for a multivariate analysis. PWD (OR1.03, 95% CI 1–1.05, p = 0.03) and EAT LA-mitral isthmus (OR 1.21, 95% CI 1–1.4, p = 0.04) were significant predictors of AF recurrence after CA (Table 6B).
As shown in previous studies (16, 17), we reported an increased occurrence of arterial hypertension, diabetes, and obstructive sleep apnea in the obese cohort, all known to be predisposing factors for AF. We also observed fewer cases with a history of paroxysmal AF in the obese group compared to the non-obese group. These data are consistent with other studies (16, 18) observing that AF became more persistent and less paroxysmal with increasing BMI. The underlying mechanisms are not fully understood and are probably multifactorial, suggesting that cardiovascular risk factors may promote the rapid progression from paroxysmal to persistent AF in obese patients through LA electrical remodeling or left ventricular diastolic dysfunction.
EAT has gained attention in the last few years and could be the link between obesity and the onset of AF. Thanassoulis et al. showed that the extent of epicardial fat was associated with AF occurrence whereas intrathoracic and abdominal fat were not (6). Batal et al. also evidenced that posterior EAT was associated with AF burden (8).
In addition, Wong et al. observed that patients with extensive EAT had early AF recurrence after the ablation procedure (7). Finally, a meta-analysis also underlined an association between increased EAT and AF recurrence in patients undergoing AF ablation (19). As evidenced in previous studies, EAT measured on the lateral wall was also an independent predictor of AF recurrence in our study.
Even if obesity is closely associated with AF occurrence and persistent AF, BMI might not be directly linked to EAT extent. Moreover, the amount of fat is probably not the best way of evaluating EAT. As demonstrated by Venteclef et al., EAT is a reservoir of adipokines acting as pro-inflammatory actors (9). Its metabolic activity measurement could be more relevant but more difficult to highlight. Wang et al. showed that the increased inflammatory activity of LA-EAT was positively correlated with AF (20). In addition, Ciuffo et al. found that the quality of the left periatrial adipose tissue, assessed by CT attenuation, was an independent predictor of AF recurrence after ablation (21).
The quality of LA-EAT, rather than its quantity, may also play an important role in the gender disparity of LA remodeling between LA-EAT and LA-LVZ. All these findings remain to be confirmed and prompt further investigation in future studies.
Matos et al. recently showed for the first time a weak correlation between EAT measured with computed tomography (CT) and LA fibrosis measured with cardiac magnetic resonance. EAT was more strongly associated with AF recurrence than LA fibrosis. This finding suggested that LA fibrosis is not the main mechanism linking EAT and AF (22).
EAT might play a role in AF onset and progression through other mechanisms, such as shortening the potential duration of action (23). In addition, large amounts of ganglionated plexi and cholinergic and adrenergic nerves are mostly embedded in the EAT. An autonomic nervous system (ANS) dysfunction could change the endocrine properties of the epicardial fat through a secretory activity enhancement and adipogenesis (24).
EAT could play an important role in cardiomyocyte function by channel dysfunctions (25) and in atrial inflammation via interleukin-6 elevation (26) inducing atrial electrical remodeling by downregulating cardiac connexins. Recently, NLRP3-inflammasome has been demonstrated to be a key driver of obesity-induced AF (27).
These mechanisms could play an important role in the rapid progression from paroxysmal to persistent AF in obese patients. Moreover, time to treatment was also shorter in the obese cohort, probably because of the persistent behavior of AF. All these elements listed above could explain both the younger age of this cohort and the absence of severe LA dilatation with thus less LVZ in obese group. Indeed, today we know that the presence of LVZ was associated both with age (13, 28) and LA enlargement (13, 29).
Overall, obesity-induced FA could be initiated by EAT-mediated reversible electrophysiological remodeling via electrical and molecular mechanisms as in our younger obese patients.
Indeed, Middeldorp et al. demonstrated that weight loss and management of risk factors may reverse the natural progression of AF. A weight loss of ≥10% resulted in 88% of patients with persistent AF reversal to paroxysmal or no AF, with 45% of patients no longer requiring ablation (30).
In addition, Mahajan et al. demonstrated that weight loss in an obese ovine model was associated with structural and electrophysiological reverse remodeling (31).
Moreover, Schram-Serban et al. recently demonstrated that the percentages of low-voltage potentials were similar between obese and non-obese patients within the majority of atrial locations (posterior LA, inferior LA, mitral isthmus, LAA, right atrium) except the Bachmann bundle using high-resolution epicardial mapping during SR. This mapping was performed during open chest cardiac surgery after sternotomy before connecting the patient to cardiopulmonary bypass circulation (32). These findings are consistent with our data except for the age of the cohort being the same in their study.
Interestingly, BMI was not a predictive factor of AF recurrence after CA. If obesity is clearly identified as a risk factor of AF onset, the impact of BMI on AF ablation outcome is still under debate with contrasting results (16, 33, 34).
Despite fewer LVZs in the obese group, we observed that the EAT amounts on the posterior and lateral walls were significantly correlated with posterior and lateral LVZ extent. The LA surface indexed-LVZ extent was the only one to be increased on the posterior wall compared to the non-obese group, although this was not significant.
Previous studies demonstrated that the presence of EAT was associated with lower bipolar voltage and atrial electrogram fractionation (12, 35). Mahajan et al. even showed that EAT infiltrated the posterior LA in the obese group with reduced endocardial voltage and conduction abnormalities in this region (12). All EAT measurements in their study were consistently greater in the obese group. We could not see such differences in our cohort. When analyzing their population, there were only 16 obese patients and 10 non-obese patients. In the non-obese group, 80% of patients displayed paroxysmal AF, known to be associated with less LA remodeling. Obesity was also defined as a BMI > 27 kg/m2. These elements may explain such differences between the two groups. In our cohort, EAT measurements were not significantly different among obese and non-obese patients, explaining the moderate substrate remodeling in our obese group. We could therefore postulate that the EAT-mediated fibrotic irreversible remodeling may occur later with increasing age in obese patients.
Several studies showed that obesity had a negative impact on AF ablation outcomes (17, 33). In our study, we could not find any difference after one single voltage-guided ablation, which had never been evaluated in an obese cohort. A recent study showed that obesity does not adversely affect the outcome of ablation until BMI exceeds 35 kg/m2 (17). Only patients with morbid obesity (BMI ≥ 35 kg/m2) have a higher recurrence rate of atrial arrhythmias during follow-up in persistent AF compared to other groups. In our obese patients, the median BMI was 32.7 kg/m2, which could explain the absence of difference in the outcome after CA.
Sivasambu et al. evidenced that persistent AF ablation outcome in patients undergoing PVI alone with cryoballoon was not affected by BMI. Freedom from AF recurrence at 1 year was around 45%–50% after PVI alone (36). We reported a better outcome in the obese group after a single LVZ-guided ablation in addition to PVI with 68% of patients off drugs at 1 year. Our study is among the first to evaluate the outcome of LVZ-guided ablation in obese persistent AF patients.
The sole predictive factor of recurrence after CA in our cohort was PWD. Several studies have also reported this association (37): a prolonged PWD attesting to an intra- and inter-atrial abnormal conduction as well the duration and direction of atrial depolarization. Dąbrowska-Kugacka et al. also observed that PWD was highly correlated with atrial electroanatomical delay assessed by tissue Doppler echocardiography (38). Some authors reported that a prolonged PWD was correlated with left atrial enlargement (39). A significant association between LVZ and prolonged PWD was also seen in patients with both paroxysmal and persistent AF (40, 41).
Whatever the chosen strategy of persistent AF ablation, electrophysiologists should not be discouraged from proposing an early persistent AF ablation in obese patients, which is associated with weight loss and physical activity, especially if the BMI is <35 kg/m2.
This was a single-center observational study with a limited number of patients. During 3D mapping, although a great number of points were collected, high-density catheters could have been used for a more rapid and better resolution, particularly for LVZ assessment. The study started using a circular catheter. To have homogeneous data, the same type of catheter was used for the whole study. In addition, the discontinuation of AADs could not be obtained in the whole cohort because the follow-up was conducted by the patients' cardiologists, who were probably less motivated to stop AADs in obese patients. Further studies are needed to assess the long-term follow-up after AF ablation in obese patients.
Obese patients with persistent AF display less electrophysiological substrate remodeling with similar LA volumes, bipolar voltage, and a similar amount of EAT compared to non-obese patients. In obese patients, EAT measured on the posterior and lateral LA wall were correlated with posterior and lateral LVZ extents. Unlike BMI, the EAT extent on the lateral wall and PWD were independent predictors of AF recurrence. Obese patients have a similar and favorable 36-month outcome after one single voltage-guided substrate persistent AF ablation. An early strategy of rhythm control using AF ablation should be proposed in obese patients to improve the cardiovascular prognosis. At the same time, weight loss and management of risk factors are absolutely necessary to reverse the natural progression of AF to avoid EAT-mediated fibrotic irreversible remodeling.
The original contributions presented in the study are included in the article/Supplementary Material, further inquiries can be directed to the corresponding author.
The study protocol was approved by the institutional review board of Strasbourg University (CE-2023-113). All patients have given their written informed consent for participation in this study.
HM: Conceptualization, Methodology, Supervision, Validation, Writing – original draft, Writing – review & editing. SF: Data curation, Writing – original draft. AL: Data curation, Writing – original draft. JH: Data curation, Writing – original draft. RR: Data curation, Writing – original draft. MK: Data curation, Writing – original draft. TC: Data curation, Writing – original draft. AS: Data curation, Writing – original draft. PO: Supervision, Writing – original draft. OM: Supervision, Writing – original draft. LJ: Supervision, Validation, Writing – review & editing, Writing – original draft.
The authors declare that financial support was received for the research, authorship, and/or publication of this article.
This work was supported by GERCA (Groupe pour l’Enseignement, la Prévention et la Recherche Cardiologique en Alsace).
The authors declare that the research was conducted in the absence of any commercial or financial relationships that could be construed as a potential conflict of interest.
All claims expressed in this article are solely those of the authors and do not necessarily represent those of their affiliated organizations, or those of the publisher, the editors and the reviewers. Any product that may be evaluated in this article, or claim that may be made by its manufacturer, is not guaranteed or endorsed by the publisher.
All the authors take responsibility for all aspects of the reliability and freedom from bias of the data presented and their discussed interpretation.
The Supplementary Material for this article can be found online at: https://www.frontiersin.org/articles/10.3389/fcvm.2024.1362903/full#supplementary-material
1. Murphy NF, MacIntyre K, Stewart S, Hart CL, Hole D, McMurray JJV. Long-term cardiovascular consequences of obesity: 20-year follow-up of more than 15 000 middle-aged men and women (the Renfrew–Paisley study). Eur Heart J. (2006) 27(1):96–106. doi: 10.1093/eurheartj/ehi506
2. Wang TJ, Parise H, Levy D, D’Agostino RB, Wolf PA, Vasan RS, et al. Obesity and the risk of new-onset atrial fibrillation. JAMA. (2004) 292(20):2471–7. doi: 10.1001/jama.292.20.2471
3. Wong CX, Sullivan T, Sun MT, Mahajan R, Pathak RK, Middeldorp M, et al. Obesity and the risk of incident, post-operative, and post-ablation atrial fibrillation: a meta-analysis of 626,603 individuals in 51 studies. JACC Clin Electrophysiol. (2015) 1(3):139–52. doi: 10.1016/j.jacep.2015.04.004
4. Pathak RK, Middeldorp ME, Meredith M, Mehta AB, Mahajan R, Wong CX, et al. Long-term effect of goal-directed weight management in an atrial fibrillation cohort: a long-term follow-up study (LEGACY). J Am Coll Cardiol. (2015) 65(20):2159–69. doi: 10.1016/j.jacc.2015.03.002
5. Aldaas OM, Lupercio F, Han FT, Hoffmayer KS, Krummen D, Ho G, et al. Meta-analysis of effect of modest (≥10%) weight loss in management of overweight and obese patients with atrial fibrillation. Am J Cardiol. (2019) 124(10):1568–74. doi: 10.1016/j.amjcard.2019.08.009
6. Thanassoulis G, Massaro JM, O’Donnell CJ, Hoffmann U, Levy D, Ellinor PT, et al. Pericardial fat is associated with prevalent atrial fibrillation: the Framingham Heart Study. Circ Arrhythm Electrophysiol. (2010) 3(4):345–50. doi: 10.1161/CIRCEP.109.912055
7. Wong CX, Abed HS, Molaee P, Nelson AJ, Brooks AG, Sharma G, et al. Pericardial fat is associated with atrial fibrillation severity and ablation outcome. J Am Coll Cardiol. (2011) 57(17):1745–51. doi: 10.1016/j.jacc.2010.11.045
8. Batal O, Schoenhagen P, Shao M, Ayyad AE, Van Wagoner DR, Halliburton SS, et al. Left atrial epicardial adiposity and atrial fibrillation. Circ Arrhythm Electrophysiol. (2010) 3(3):230–6. doi: 10.1161/CIRCEP.110.957241
9. Venteclef N, Guglielmi V, Balse E, Gaborit B, Cotillard A, Atassi F, et al. Human epicardial adipose tissue induces fibrosis of the atrial myocardium through the secretion of adipo-fibrokines. Eur Heart J. (2015) 36(13):795–805. doi: 10.1093/eurheartj/eht099
10. Haemers P, Hamdi H, Guedj K, Suffee N, Farahmand P, Popovic N, et al. Atrial fibrillation is associated with the fibrotic remodelling of adipose tissue in the subepicardium of human and sheep atria. Eur Heart J. (2017) 38(1):53–61. doi: 10.1093/eurheartj/ehv625
11. Verma A, Wazni OM, Marrouche NF, Martin DO, Kilicaslan F, Minor S, et al. Pre-existent left atrial scarring in patients undergoing pulmonary vein antrum isolation: an independent predictor of procedural failure. J Am Coll Cardiol. (2005) 45(2):285–92. doi: 10.1016/j.jacc.2004.10.035
12. Mahajan R, Lau DH, Brooks AG, Shipp NJ, Manavis J, Wood JPM, et al. Electrophysiological, electroanatomical, and structural remodeling of the atria as consequences of sustained obesity. J Am Coll Cardiol. (2015) 66(1):1–11. doi: 10.1016/j.jacc.2015.04.058
13. Huo Y, Gaspar T, Pohl M, Sitzy J, Richter U, Neudeck S, et al. Prevalence and predictors of low voltage zones in the left atrium in patients with atrial fibrillation. Europace. (2018) 20(6):956–62. doi: 10.1093/europace/eux082
14. Oakes RS, Badger TJ, Kholmovski EG, Akoum N, Burgon NS, Fish EN, et al. Detection and quantification of left atrial structural remodeling with delayed-enhancement magnetic resonance imaging in patients with atrial fibrillation. Circulation. (2009) 119(13):1758–67. doi: 10.1161/CIRCULATIONAHA.108.811877
15. Mahnkopf C, Badger TJ, Burgon NS, Daccarett M, Haslam TS, Badger CT, et al. Evaluation of the left atrial substrate in patients with lone atrial fibrillation using delayed-enhanced MRI: implications for disease progression and response to catheter ablation. Heart Rhythm. (2010) 7(10):1475–81. doi: 10.1016/j.hrthm.2010.06.030
16. Winkle RA, Mead RH, Engel G, Kong MH, Fleming W, Salcedo J, et al. Impact of obesity on atrial fibrillation ablation: patient characteristics, long-term outcomes, and complications. Heart Rhythm. (2017) 14(6):819–27. doi: 10.1016/j.hrthm.2017.02.023
17. Providência R, Adragão P, de Asmundis C, Chun J, Chierchia G, Defaye P, et al. Impact of body mass index on the outcomes of catheter ablation of atrial fibrillation: a European observational multicenter study. J Am Heart Assoc. (2019) 8(20):e012253. doi: 10.1161/JAHA.119.012253
18. Dublin S, French B, Glazer NL, Wiggins KL, Lumley T, Psaty BM, et al. Risk of new-onset atrial fibrillation in relation to body mass index. Arch Intern Med. (2006) 166(21):2322–8. doi: 10.1001/archinte.166.21.2322
19. Sepehri Shamloo A, Dagres N, Dinov B, Sommer P, Husser-Bollmann D, Bollmann A, et al. Is epicardial fat tissue associated with atrial fibrillation recurrence after ablation? A systematic review and meta-analysis. Int J Cardiol Heart Vasc. (2019) 22:132–8. doi: 10.1016/j.ijcha.2019.01.003
20. Wang B, Xu YD, Shao S, Zhai LS, Qian B, Zhang FF, et al. Association between inflammation activity of left atrial epicardial adipose tissue measured by 18F-FDG PET/CT and atrial fibrillation. Zhonghua Xin Xue Guan Bing Za Zhi. (2021) 49(12):1213–9. doi: 10.3760/cma.j.cn112148-20211026-00913
21. Ciuffo L, Nguyen H, Marques MD, Aronis KN, Sivasambu B, de Vasconcelos HD, et al. Periatrial fat quality predicts atrial fibrillation ablation outcome. Circ Cardiovasc Imaging. (2019) 12(6):e008764. doi: 10.1161/CIRCIMAGING.118.008764
22. Matos D, Ferreira AM, Freitas P, Rodrigues G, Carmo J, Costa F, et al. The relationship between epicardial fat and atrial fibrillation cannot be fully explained by left atrial fibrosis. Arq Bras Cardiol. (2022) 118(4):737–42. doi: 10.36660/abc.20201083
23. Martinez-Mateu L, Saiz J, Aromolaran AS. Differential modulation of Ik and ICa,L channels in high-fat diet-induced obese guinea pig atria. Front Physiol. (2019) 10:1212. doi: 10.3389/fphys.2019.01212
24. Stavrakis S, Po S. Ganglionated plexi ablation: physiology and clinical applications. Arrhythmia Electrophysiol Rev. (2017) 6(4):186–90. doi: 10.15420/aer2017.26.1
25. Sato S, Suzuki J, Hirose M, Yamada M, Zenimaru Y, Nakaya T, et al. Cardiac overexpression of perilipin 2 induces atrial steatosis, connexin 43 remodeling, and atrial fibrillation in aged mice. Am J Physiol Endocrinol Metab. (2019) 317(6):E1193–204. doi: 10.1152/ajpendo.00227.2019
26. Lazzerini PE, Laghi-Pasini F, Acampa M, Srivastava U, Bertolozzi I, Giabbani B, et al. Systemic inflammation rapidly induces reversible atrial electrical remodeling: the role of interleukin-6-mediated changes in connexin expression. J Am Heart Assoc. (2019) 8(16):e011006. doi: 10.1161/JAHA.118.011006
27. Scott L Jr, Fender AC, Saljic A, Li L, Chen X, Wang X, et al. NLRP3 Inflammasome is a key driver of obesity-induced atrial arrhythmias. Cardiovasc Res. (2021) 117(7):1746–59. doi: 10.1093/cvr/cvab024
28. Blandino A, Bianchi F, Grossi S, Biondi-Zoccai G, Conte MR, Gaido L, et al. Left atrial substrate modification targeting low-voltage areas for catheter ablation of atrial fibrillation: a systematic review and meta-analysis. Pacing Clin Electrophysiol. (2017) 40(2):199–212. doi: 10.1111/pace.13015
29. Masuda M, Fujita M, Iida O, Okamoto S, Ishihara T, Nanto K, et al. Influence of underlying substrate on atrial tachyarrhythmias after pulmonary vein isolation. Heart Rhythm. (2016) 13(4):870–8. doi: 10.1016/j.hrthm.2015.12.029
30. Middeldorp ME, Pathak RK, Meredith M, Mehta AB, Elliott AD, Mahajan R, et al. PREVEntion and regReSsive effect of Weight-Loss and Risk Factor Modification on atrial fibrillation: the REVERSE-AF Study. Europace. (2018) 20(12):1929–35. doi: 10.1093/europace/euy117
31. Mahajan R, Lau DH, Brooks AG, Shipp NJ, Wood JPM, Manavis J, et al. Atrial fibrillation and obesity: reverse remodeling of atrial substrate with weight reduction. JACC Clin Electrophysiol. (2021) 7(5):630–41. doi: 10.1016/j.jacep.2020.11.015
32. Schram-Serban C, Van Schie MS, Knops P, Kik C, Bogers AJJC, de Groot NMS. Low-voltage potentials contribute to postoperative atrial fibrillation development in obese patients. Heart Rhythm. (2022) 19(5):710–8. doi: 10.1016/j.hrthm.2022.01.027
33. Chilukuri K, Dalal D, Gadrey S, Marine JE, Macpherson E, Henrikson CA, et al. A prospective study evaluating the role of obesity and obstructive sleep apnea for outcomes after catheter ablation of atrial fibrillation. J Cardiovasc Electrophysiol. (2010) 21(5):521–5. doi: 10.1111/j.1540-8167.2009.01653.x
34. Cha YM, Friedman PA, Asirvatham SJ, Shen W-K, Munger TM, Rea RF, et al. Catheter ablation for atrial fibrillation in patients with obesity. Circulation. (2008) 117(20):2583–90. doi: 10.1161/CIRCULATIONAHA.107.716712
35. Zghaib T, Ipek EG, Zahid S, Balouch MA, Misra S, Ashikaga H, et al. Association of left atrial epicardial adipose tissue with electrogram bipolar voltage and fractionation: electrophysiologic substrates for atrial fibrillation. Heart Rhythm. (2016) 13(12):2333–9. doi: 10.1016/j.hrthm.2016.08.030
36. Sivasambu B, Balouch MA, Zghaib T, Bajwa RJ, Chrispin J, Berger RD, et al. Increased rates of atrial fibrillation recurrence following pulmonary vein isolation in overweight and obese patients. J Cardiovasc Electrophysiol. (2018) 29(2):239–45. doi: 10.1111/jce.13388
37. Pranata R, Yonas E, Vania R. Prolonged P-wave duration in sinus rhythm pre-ablation is associated with atrial fibrillation recurrence after pulmonary vein isolation—a systematic review and meta-analysis. Ann Noninvasive Electrocardiol. (2019) 24(5):e12653. doi: 10.1111/anec.12653
38. Dąbrowska-Kugacka A, Lewicka-Nowak E, Ruciński P, Zagożdżon P, Raczak G, Kutarski A. Relationship between P-wave duration and atrial electromechanical delay assessed by tissue Doppler echocardiography. Pacing Clin Electrophysiol. (2011) 34(1):23–31. doi: 10.1111/j.1540-8159.2010.02939.x
39. Andlauer R, Seemann G, Baron L, Dössel O, Kohl P, Platonov P, et al. Influence of left atrial size on P-wave morphology: differential effects of dilation and hypertrophy. Europace. (2018) 20(3):iii36–44. doi: 10.1093/europace/euy231
40. Chen Q, Mohanty S, Trivedi C, Gianni C, Della Rocca DG, Canpolat U, et al. Association between prolonged P wave duration and left atrial scarring in patients with paroxysmal atrial fibrillation. J Cardiovasc Electrophysiol. (2019) 30(10):1811–8. doi: 10.1111/jce.14070
41. Jadidi A, Müller-Edenborn B, Chen J, Keyl C, Weber R, Allgeier J, et al. The duration of the amplified sinus-P-wave identifies presence of left atrial low voltage substrate and predicts outcome after pulmonary vein isolation in patients with persistent atrial fibrillation. JACC Clin Electrophysiol. (2018) 4(4):531–43. doi: 10.1016/j.jacep.2017.12.001
Keywords: atrial fibrillation, catheter ablation, obesity, low-voltage zones, bipolar voltage map, atrial remodeling, epicardial adipose tissue
Citation: Marzak H, Fitouchi S, Labani A, Hammann J, Ringele R, Kanso M, Cardi T, Schatz A, Ohlmann P, Morel O and Jesel L (2024) Left atrial remodeling and voltage-guided ablation outcome in obese patients with persistent atrial fibrillation. Front. Cardiovasc. Med. 11:1362903. doi: 10.3389/fcvm.2024.1362903
Received: 29 December 2023; Accepted: 11 March 2024;
Published: 2 April 2024.
Edited by:
Teresa Strisciuglio, University of Naples Federico II, ItalyReviewed by:
Anuradha Kalyanasundaram, University of North Texas Health Science Center, United States© 2024 Marzak, Fitouchi, Labani, Hammann, Ringele, Kanso, Cardi, Schatz, Ohlmann, Morel and Jesel. This is an open-access article distributed under the terms of the Creative Commons Attribution License (CC BY). The use, distribution or reproduction in other forums is permitted, provided the original author(s) and the copyright owner(s) are credited and that the original publication in this journal is cited, in accordance with accepted academic practice. No use, distribution or reproduction is permitted which does not comply with these terms.
*Correspondence: Halim Marzak aGFsaW0ubWFyemFrQGNocnUtc3RyYXNib3VyZy5mcg==
Disclaimer: All claims expressed in this article are solely those of the authors and do not necessarily represent those of their affiliated organizations, or those of the publisher, the editors and the reviewers. Any product that may be evaluated in this article or claim that may be made by its manufacturer is not guaranteed or endorsed by the publisher.
Research integrity at Frontiers
Learn more about the work of our research integrity team to safeguard the quality of each article we publish.