- 1Department of Internal Medicine, S.Elia Hospital, Caltanissetta, Italy
- 2Cardiovascular and Interventional Department, S.Elia Hospital, Caltanissetta, Italy
- 3Institute of Public Health and Clinical Medicine, Umea University, Umea, Sweden
Risk stratification of cardiovascular death and treatment strategies in patients with heart failure (HF), the optimal timing for valve replacement, and the selection of patients for implantable cardioverter defibrillators are based on an echocardiographic calculation of left ventricular ejection fraction (LVEF) in most guidelines. As a marker of systolic function, LVEF has important limitations being affected by loading conditions and cavity geometry, as well as image quality, thus impacting inter- and intra-observer measurement variability. LVEF is a product of shortening of the three components of myocardial fibres: longitudinal, circumferential, and oblique. It is therefore a marker of global ejection performance based on cavity volume changes, rather than directly reflecting myocardial contractile function, hence may be normal even when myofibril's systolic function is impaired. Sub-endocardial longitudinal fibers are the most sensitive layers to ischemia, so when dysfunctional, the circumferential fibers may compensate for it and maintain the overall LVEF. Likewise, in patients with HF, LVEF is used to stratify subgroups, an approach that has prognostic implications but without a direct relationship. HF is a dynamic disease that may worsen or improve over time according to the underlying pathology. Such dynamicity impacts LVEF and its use to guide treatment. The same applies to changes in LVEF following interventional procedures. In this review, we analyze the clinical, pathophysiological, and technical limitations of LVEF across a wide range of cardiovascular pathologies.
1 Introduction
The assessment of left ventricular (LV) systolic function has important implications for diagnosis, follow-up, treatment, and prediction of clinical outcomes of patients with heart disease. Over the past five decades, left ventricular ejection fraction (LVEF), expressed as the stroke volume (SV) relative to end-diastolic volume (EDV), has been extensively used for quantifying LV systolic function, mostly because of the simplicity of its measurement. Clinical trials evaluating the effectiveness of different treatment strategies in HF patients also used LVEF as a threshold to categorize patients likely to benefit from therapy. It proved to have good prognostic value in HF patients, in the risk stratification of valve heart disease, and in the selection of those eligible for implantable cardioverter defibrillators (1–6). LVEF may be calculated using several cardiac imaging techniques including two-dimensional (2D) and three-dimensional echocardiography (3D), cardiac magnetic resonance (CMR), computed tomography scan (CT), and gated single-photon emission computed tomography (SPECT) myocardial perfusion imaging. However, 2D echocardiography is the most commonly used modality due to its simplicity, low cost, and widespread accessibility in clinical practices.
LVEF is widely accepted as a measure of systolic function to guide the management of several pathological conditions and to track the response to therapy. However, it has important limitations, including poor correlation with symptoms and outcomes of HF patients, being dependent on loading conditions, ventricular geometry, and image quality, that may impact inter- and intra-observer variability (7–12).
This review discusses the available evidence for clinical, pathophysiological, and technical limitations of LVEF as a measure of LV systolic function in various clinical conditions.
2 Clinical limitations of LVEF in HF
The limitations of LVEF as a marker of systolic LV function are particularly evident in HF patients, even in the preclinical stage. In addition to symptoms and signs, the diagnosis of HF largely relies on LVEF as a measure of cavity function, often obtained by 2D echocardiography in clinics. Most HF clinical trials have shown that the treatment benefits are mainly evident in patients with reduced LVEF (2, 13). Current guidelines recognize distinct HF patient phenotypes on the basis of LVEF: reduced LV systolic function (LVEF ≤ 40%, HFrEF), mildly reduced LV systolic function (HFmrEF), and preserved systolic function (LVEF ≥ 50%, HFpEF) (2, 14). However, there are some variations in HF classification and LVEF grading among different clinical practice guidelines (Table 1) (20–22).
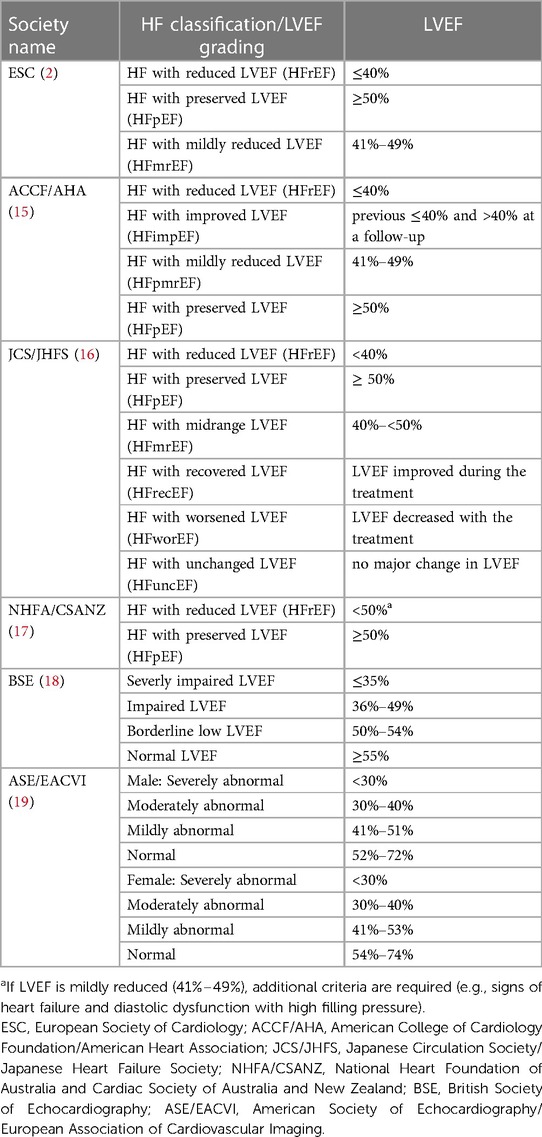
Table 1. HF classifications according to LVEF and LVEF grading in international cardiac societies guidelines.
Based on the above classification, evidence-based therapy has proved effective in patients with HFrEF, with uncertain results in those with HFpEF being limited to certain subgroups, thus underlying the phenotypic heterogeneity of these patients (23–28). Such outcomes could be related to the fundamental differences in the pathophysiology of different groups. HFpEF patients are more often older women, likely to have cardiac and non-cardiac comorbidities, such as diabetes, hypertension, obesity, chronic obstructive pulmonary disease, and ischemic heart disease with respect to patients with HFrEF (29, 30). Patients with HFmrEF, which account for up to 20% of all HF patients, are intermediate between HFrEF and HFpEF. They include a heterogeneous population mostly with HFrEF whose LVEF partially improved with therapies and a smaller proportion with HFpEF whose LVEF declined. Patients in this range represent a transitional phenotype that may further progress either toward improvement or deterioration of LVEF (31–34).
HF, in general, is a dynamic syndrome that may progress or improve over time according to the changes in the underlying pathophysiological processes. Patients with HFrEF (LV EF <40%) may show an improvement or even normalization of LV EF, with an absolute increase of EF ≥10%, which may occur spontaneously or as a result of a good response to therapy (35). However, this change in LVEF does not necessarily mean full recovery of systolic LV function. Indeed, discontinuation of optimal therapies results in the worsening of HF (36). Over one-half of HFrEF patients, who had a myocardial infarction, as well as those with non-ischemic HErEF, demonstrate an improvement in LVEF following the resolution of myocardial stunning in the areas with residual viable myocardium or remission of the underlying pathology, such as myocarditis, peri-partum, Takotsubo, and tachycardia-related cardiomyopathies (37–39). These patients with LVEF >50% represent a different HF phenotype with recovered LVEF (HFrecEF), which from a single echocardiogram may be misdiagnosed as having HFpEF (35, 40). Evidence has shown that approximately 70% of patients with symptomatic HFpEF have recovered from low LVEF (41). Although this group has a better biomarker profile and event-free survival compared to HFrEF and HFpEF, the high rate of HF hospitalization suggests underlying function instability and high risk of HF (39) despite the fact that the normalized LVEF cannot distinguish between resolution of the underlying myocardial pathology or improvement with persistence of subclinical myocardial dysfunction. Also, despite the normalization of LVEF, in most patients, the LV systolic function remains impaired when studied by global longitudinal strain (GLS) echocardiography (42).
Accurate stratification of risk is important for the appropriate management of patients with HF (2). LVEF has been shown as a powerful predictor of fatal and non-fatal cardiovascular outcomes. Nevertheless, this is true only when the systolic function is below 45%, whereas the prognostic capability reduces significantly in patients with LVEF exceeding this threshold (43–45). Some echocardiographic models for risk prediction have been developed to identify the most significant predictors of mortality in HF patients (46, 47). While measures of LV and left atrial volume overload, as well as LV diastolic function, are independent predictors of mortality, LVEF does not significantly contribute to the risk prediction of mortality. Large studies of patients hospitalized with EF have shown similar mortality rates across the LVEF spectrum (48, 49). Also, epidemiological data show a U-shaped relationship between mortality and LVEF in patients with LVEF ≥ 65% (so-called supra-normal LV function) in whom mortality rates are similar to HFrEF (50, 51). These findings imply that categorizing systolic heart failure solely on ejection fraction may not consistently yield accurate results. GLS has been shown as a stronger predictor of cardiovascular outcomes than LVEF although it is influenced by age, sex, and loading conditions (52).
Biomarkers such as N-terminal pro-B type natriuretic peptide (NT-proBNP) have been increasingly used for prognosis and monitoring of HF therapy (53). Studies have shown that in HF patients, LVEF has no significant relationship with the prognostic value of NT-proBNP (54–56). Although overall levels of NT-proBNP are higher in patients with HFrEF compared with HFpEF, a given level of NT-proBNP is related to the same risk of death across all categories of HF, independently of LVEF. This highlights the importance of NT-proBNP in prognosis equations, irrespective of LVEF. Likewise, differences in LVEF have been shown to account very poorly for the explanation of the variation in HF-related protein biomarkers associated with inflammation, cellular proliferation, and metabolism, with a large proportion related to the underlying cause of HF, whether ischemic or non-ischemic (57–61).
2.1 Pathophysiological limitations of LVEF in HF
As mentioned above, LVEF is not an early marker of myocardial dysfunction and may be normal even when LV function is impaired. Several studies have shown significant impairment of myocardial function, assessed by myocardial strain and expressed as the percentage change in myocardial length measured longitudinally (GLS), circumferentially, or radially, despite preserved EF (28, 52). Such discordance is explained by changes in LV geometry which can compensate for large variations in LV function, thus maintaining overall LVEF. Accordingly, in the presence of LV dysfunction and reduced SV, LVEF may be normal in patients with LV hypertrophy and small LV cavities. In addition, LVEF does not provide pathophysiological distinctions between systolic and diastolic dysfunction. Indeed, abnormalities in diastolic function and LV filling are common in HFrEF and contribute to prognosis, while subclinical systolic impairment that can be detected by GLS is common in patients with HFpEF (62–64).
Regardless of the imaging modality used for measurement, LVEF depends on the conditions affecting SV and EDV, such as LV load conditions, myocardial contractility, and cavity dyssynchrony (7, 65). Cardiomyocytes have a limited longitudinal shortening and radial thickening during contraction of approximately 15%, which cannot account for the normal LVEF >50% (66). Experimental studies have shown that a normal LVEF is contributed to by the complex muscular structure of the LV, organized in interconnected layers with oblique spiral myofibrillar arrangement around the ventricles (67, 68). From the apex to the base, the sub-epicardial fibers are longitudinal and clockwise oriented; the sub-endocardial longitudinal and counter-clockwise fibers, and the middle layer formed by circumferential fibers which represent the thickest layer, approximately 60% of LV wall thickness (69–71). During systole, the clockwise shortening of longitudinal epicardial fibers and the counter-clockwise shortening of longitudinal sub-endocardial fibers (LV twisting) displace the LV base toward the apex, while the shortening of circumferential fibers causes LV mid-wall thickening, reducing LV cavity size (69, 72, 73). Thus, LVEF is the result of longitudinal and circumferential LV shortening.
Heart diseases have different effects on LV myocardial fiber architecture. Sub-endocardial longitudinal fibers are most susceptible to ischemia and their impairment may precede the reduction of mid-wall myocardium function, resulting in subclinical LV dysfunction that does not always affect LVEF although may be detected by GLS (Figure 1) (27, 74). Mathematical models have provided evidence that LV longitudinal shortening has a limited effect on LVEF. In contrast, the relationship between LVEF and circumferential shortening is quadratic as the short axis area is a function of the square of the radius. Hence, circumferential fibers shortening in the mid-wall has a predominant effect on LVEF with a 1 cm increase in thickness, exaggerating LVEF by approximately 13% (66, 75). Accordingly, mid-wall shortening may compensate for the impairment of longitudinal function. Moreover, the relationship of the short axis area with the square radius explains why a thick LV, as in hypertensive patients, needs less fiber shortening to produce the same LVEF compared to a thin ventricle (76–78).
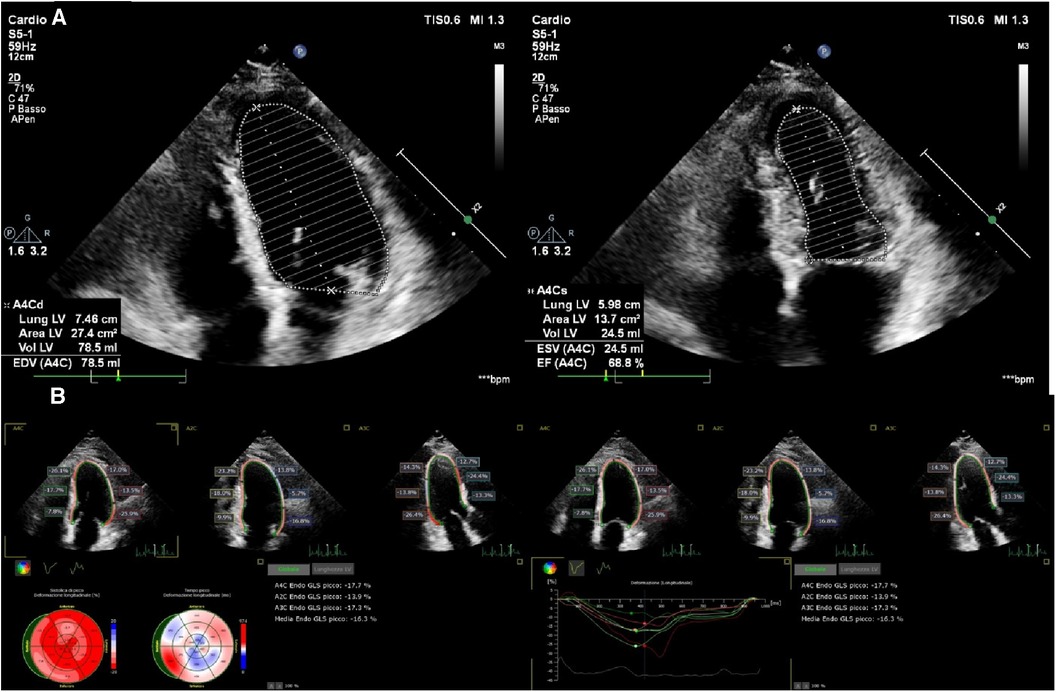
Figure 1. Preserved LVEF (68%) (A) and impaired longitudinal systolic function (GLS −16%) (B) in a 68-year-old man, 4 months after non-ST elevation myocardial infarction (NSTEMI).
3 Limitations of LVEF in heart valve disease
Risk stratification and timely diagnosis have critical importance in the treatment strategies of patients with valve lesions in order to improve clinical outcomes and prevent post-operative LV dysfunction. Current guidelines assign a primary role to LVEF as an independent determinant of long-term outcomes and in the early detection of LV damage in order to define the optimal timing for surgical management. However, LVEF limitation in detecting early myocardial dysfunction in asymptomatic patients has not been fully taken into account.
3.1 Mitral regurgitation (MR)
LVEF is influenced by loading conditions in primary and degenerative MR (intrinsic organic valve diseases, such as mitral valve prolapse or flail leaflets). Surgical valve repair or replacement is effective in relieving symptoms and preventing further progression of LV disease. However, the clinical outcome after mitral valve surgery depends largely on pre-operative LV status. Evidence exists supporting the likelihood of post-operative normalization of LV function with early valve intervention before deterioration of LV function (79, 80). Thus, optimal timing for surgery is crucial for optimum management of patients with degenerative MR.
During systole, a proportion of the LV volume is directed to the left atrium which dilates in order to accommodate the volume overload that eventually returns to the LV during the succeeding diastole. As a consequence, LV dilates and remodels, including myocardial thinning or eccentric LV hypertrophy. Volume expansion of the LV increases preload which in turn increases SV (Frank-Starling mechanism) as a result of the large end-diastolic volume, thus avoiding a relevant increase in filling pressure (81). As long as LV remodeling allows the maintenance of normal SV, LVEF remains preserved or even supernormal for a long time, erroneously suggesting a good ventricular function, although myocardial damage has already developed (82). The increase in LV volume leads to increased wall stress which is directly proportional to LV cavity radius and inversely proportional to wall thickness, according to the Laplace law. In addition, contrary to the widely accepted concept of chronic MR as a low impedance leak into the left atrium, facilitating LV emptying, the LV afterload has been shown to be normal in compensated MR and tends to increase when LV dysfunction develops (81, 83). Indeed, the regurgitant orifice is a fraction of the anatomical mitral orifice area, thus contributing to the magnitude of MR impedance.
Over time, chronic volume overload and normal or increased afterload induce myofibrillar degeneration and the development of irreversible fibrosis which results in LV contractile dysfunction, as has been shown by longitudinal and circumferential strain (84–87). Even in these conditions, LVEF may be ≥60%, hiding the latent LV dysfunction, and can affect long-term survival (Figure 2) (88, 89). Indeed, it has been shown that in patients with MR and preserved global systolic function, the earliest impairment of contractility is heterogeneous and located in the LV septum, with a compensatory increase in myocardial contractile function, providing an explanation for the preserved LVEF (90).
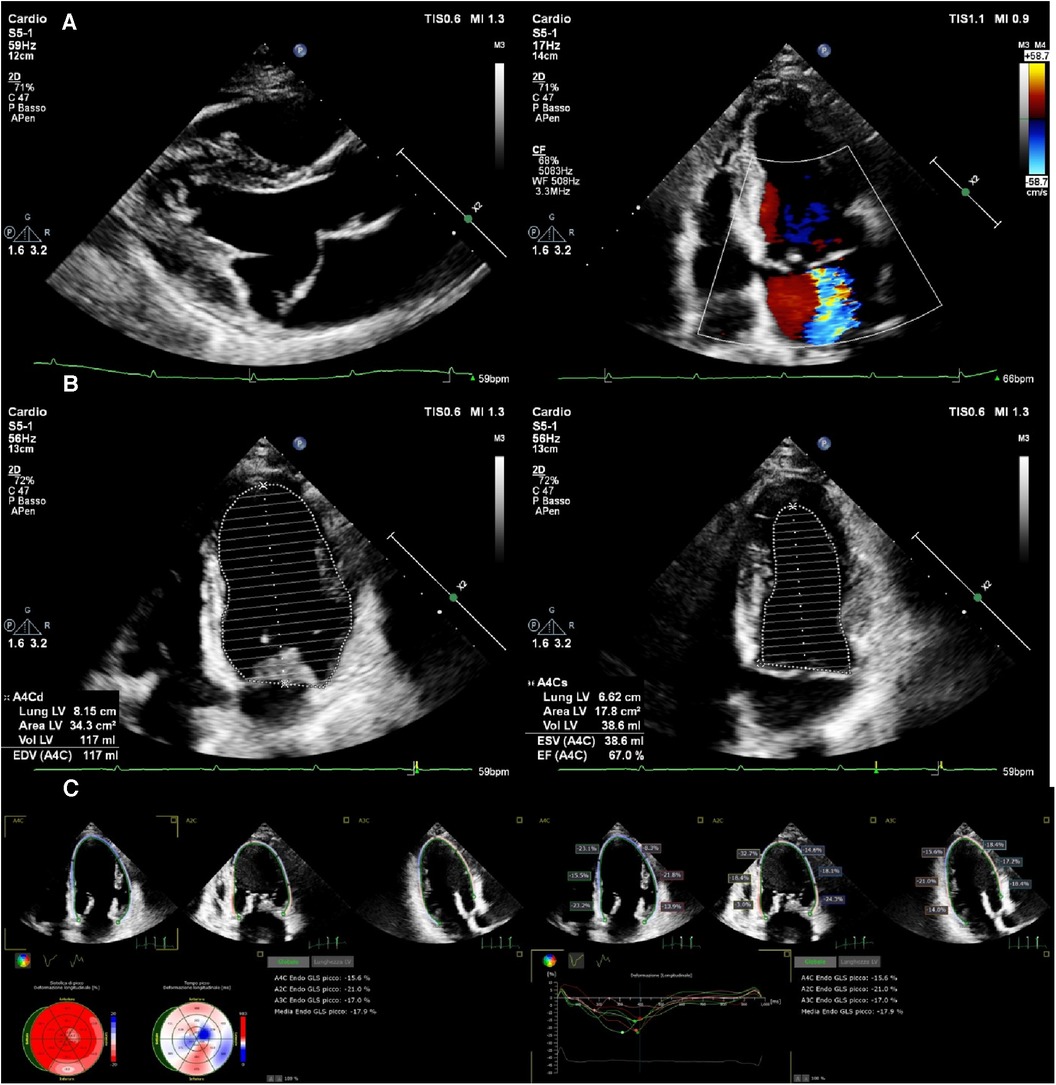
Figure 2. An asymptomatic 54-year-old woman with severe mitral regurgitation arising from anterior leaflet prolapse (A) preserved LVEF (67%) (B) and slightly reduced longitudinal systolic function GLS (−17.9) (C).
Current guidelines recommend surgery in symptomatic MR patients. In the presence of early signs of LV systolic dysfunction, defined as LVEF ≤60% in addition to LV end-systolic diameter (LVESD) ≥ 40 mm, left atrial volume ≥60 ml/m2, atrial fibrillation, or systolic pulmonary arterial pressure >50 mmHg, intervention is indicated regardless of symptoms (Class I recommendation). In asymptomatic patients with preserved LV function (LVEF >60% and LVESD <40 mm), surgery is recommended if they have atrial fibrillation or pulmonary artery hypertension (Class IIa recommendation) (5, 91). In the absence of these criteria, asymptomatic patients undergo watchful waiting or active surveillance, characterized by medical management and careful follow-up of clinical and hemodynamic conditions.
The optimal management strategy for asymptomatic patients with severe MR and preserved LVEF is controversial. Because LV dysfunction based on LVEF is often underestimated in patients with severe MR, surgical decisions can be delayed. Several studies have shown that early surgical correction is associated with significant long-term survival benefits and reduced development of HF and post-operative LV dysfunction, compared to a more conservative treatment strategy (92–94). Also, although a small 5%–10% post-operative decrease in LVEF is common after mitral repair due to changes in LV volumes following withdrawal of the proportion of LV ejection contributing to regurgitation, greater LVEF reductions have been associated with irreversible myocardial dysfunction and poor prognosis. Approximately one-fifth of asymptomatic patients without Class I or IIa indications for surgery can develop severe early post-operative LV dysfunction (LVEF <50%) despite pre-operative LVEF >60% (95). Hence, watchful waiting based on LVEF follow-up may be inadequate for the early identification of impaired myocardial function due to increased fibrosis which can compromise the outcome of surgical MR repair.
3.2 Aortic stenosis (AS)
Calcific AS is a chronic disease characterized by a prolonged latent period where patients are relatively asymptomatic. However, when symptoms develop, they reflect LV disease and progression is usually very rapid. This is highly dependent on the response of the myocardium to the progressive reduction in the aortic valve area. The increased afterload with AS causes increased LV wall stress leading to concentric hypertrophy. This is a compensatory mechanism that results in the normalization of LV wall stress and maintenance of LVEF within the normal range (96–98). Although initially beneficial, when the hypertrophic response can no longer match the excess afterload, the LVEF begins to decline (afterload mismatch) and LV hypertrophy progresses to fibrosis. With the aging of the population, AS is increasingly becoming a syndrome that includes hypertension and arterial disease that impair LV function independently of valvar stenosis. This phenotype is characterized by hypertrophic small LV cavities, paradoxical low-flow low-gradient AS, and eventually HFpEF (99). Only surgical or transcatheter aortic valve replacement (TAVR) can prevent disease progression and poor clinical outcomes (100). Currently, expanding indications of early TAVR in asymptomatic, younger, and low-risk patients are being evaluated (101).
Current indications for AVR are based on the presence of symptoms, aortic valve gradient, and reduced LVEF (5, 91). In asymptomatic patients, AVR is recommended in LVEF <50% (Class I), with exertional symptoms (Class I), LVEF <55% (Class IIa), and LVEF >55% in addition to one of the following conditions: severe aortic stenosis (mean pressure gradient ≥60 mmHg), severe valve calcification, and markedly elevated brain natriuretic peptide (BNP).
Away from these conditions, guidelines suggest a watchful waiting strategy, delaying AVR until the onset of AS symptoms or LV systolic dysfunction (102). This strategy is based on the reported relatively low risk of sudden death in such patients, compared to the risk of surgery (103). However, a longstanding pressure overload in severe AS can cause further impairment of myocardial function, resulting in irreversible damage and development of HF, as explained above (104).
Although adaptive LV hypertrophy maintains LVEF within a normal range until end-stage disease, early subtle myocardial dysfunction may be present in AS patients (105–108). Therefore, the 50% LVEF cut-off for referral for AVR is too low because it could represent late presentation in the course of the disease. To support this view, clinical studies and meta-analyses have shown that survival in patients with AS and no or minimal symptoms at diagnosis is poor when LVEF is <60% (109–112). Moreover, in asymptomatic AS patients with LVEF >60%, early AVR has been shown to be associated with reduced all-cause mortality, acute myocardial infarction, stroke, and hospitalization for HF, compared with patients treated with conservative management and AVR deferred after symptoms onset (113–117). To avoid the latter, alternative parameters, such as GLS, may be more useful to identify early myocardial function changes and the optimal timing for AVR (118, 119).
3.3 Aortic regurgitation (AR)
Chronic AR causes LV volume overload and significant LV afterload (systolic wall stress) due to high stroke volume (120, 121). LV systolic function is maintained through chamber enlargement followed by eccentric hypertrophy. This pathophysiology allows for maintaining normal end-diastolic wall stress, hence normal filling pressures. In general, patients usually tolerate AR and remain compensated and asymptomatic for a long time.
Eventually, the progressive LV dilatation and the increase in LV filling pressure lead to increased systolic wall stress. This afterload mismatch and limited preload reserve of an enlarged ventricle impair cavity performance further, causing symptoms of HF with subsequently impacted survival in the absence of surgical correction (122–124). The development of symptoms also indicates irreversible LV damage caused by myocardial fibrosis, which may impede recovery of LV function after AVR (125–129). Thus, accurate detection of subclinical LV dysfunction before the development of symptoms and a reduction in LVEF should identify patients who need early surgical intervention.
Accordingly, guidelines recommend surgery, even in the absence of symptoms, for patients with a significantly dilated LV, which reflects the severity of volume overload (LVESD >50 mm or >2.0 mm/m2) even in the presence of preserved LVEF which overestimates the real EF (5, 91). Also, studies have shown that more than 80% of deaths in asymptomatic patients occur before reaching the guideline-recommended threshold for surgical intervention (130, 131). Hence, as in other settings of subclinical dysfunction, LVEF is not a sensitive marker of early LV systolic dysfunction compared with longitudinal and circumferential LV strains in identifying patients with impaired LV function, who require AVR, despite preserved LVEF (132–134).
4 Limitations of LVEF in the selection of patients for implantable cardioverter defibrillator (ICD)
Patients with recent myocardial infarction (MI) and LV dysfunction, as well as those with non-ischemic LV systolic dysfunction, are at high risk of sudden cardiac death (SCD), frequently due to ventricular arrhythmias (135, 136). ICD implantation has proved to be effective in reducing the occurrence of SCD in these patients (137–141). The current risk stratification strategy for SCD primary prevention is largely based on the measurement of LVEF function. An LVEF ≤35%, despite optimal medical therapy, is the currently recommended threshold for Class I ICD implantation for the primary prevention of SCD and all-cause mortality in symptomatic patients with HF of ischemic origin, and Class IIa for patients with HF of non-ischemic origin (2). The strongest evidence for benefits from ICD implantation according to LVEF <35% has been observed only in patients with ischemic HF, which resulted in long-term reduction of all-cause mortality (141).
Although LVEF is the most powerful non-invasive assessor, compared to electrocardiographic techniques, for identifying HF patients who are at risk of SCD, it has limited sensitivity (142). Population-based studies have shown that the majority of SCD cases occur in individuals who had no known heart disease or had heart disease with normal or only moderately impaired LVEF function (143–146).
In addition, over the last two decades, there has been a decline in the risk of SCD, especially in patients with non-ischemic HF, attributable to an improvement in medical therapy (147–150). Clinical trials using sacubitril-valsartan (ARNI) and sodium-glucose co-transporter 2 inhibitors (SGLT2) have demonstrated a consistent reduction in ventricular arrhythmias and SCD due to their anti-arrhythmic properties in addition to their ability to improve LVEF, thus reducing the eligibility for ICD implantation based on LVEF ≤35% (151–154).
Patients with ischemic HF are at high risk of mortality early after MI. However, early measurement of LVEF does not take into account the spontaneous improvement of LVEF following the recovery of myocardial stunning occurring in more than half of post-MI patients (37). Hence, a month after MI, there may be no longer an indication for ICD implantation because of the rise in LVEF >35%. However, approximately two-thirds of SCD occurs in patients with LVEF >35% (155, 156).
Thus, LVEF alone is inadequate as a marker of the underlying myocardial damage predisposing to SCD because it has no causal relationship with the mechanisms of arrhythmia (146). LV dysfunction and remodeling both in ischemic and non-ischemic HF result in myocardial fibrosis (157, 158). Even in the absence of contractile impairment, fibrosis induces electrophysiological heterogeneity which promotes the development of ventricular arrhythmias (159, 160).
More accurate strategies to identify patients at risk include the quantification of myocardial fibrosis using mechanical dispersion at strain imaging and cardiac magnetic resonance imaging with late gadolinium enhancement (CMR-LGE) (157, 161–164).
5 Technical limitations
Most therapeutic decisions in current guidelines are based on 2-dimensional (2D) echocardiographic measurement of LVEF which uses the method of disk summation (modified Simpson's rule) to calculate LV volumes in two imaging planes (19). As an index derived from volumes, LVEF is based on the geometric assumption of an ellipsoid ventricular shape to estimate a three-dimensional volume from a two-dimensional image. This may reduce the measurement reliability when the ventricle has a non-geometric shape as occurs in patients with ischemic heart disease (165).
Poor image quality due to body shape and limited acoustic window may impair the delineation of the endocardial border and tracing of the LV cavity, leading to a foreshortened ventricle. Since echocardiography is operator-dependent, measurement inconsistencies result in large intra- and inter-observer variability, particularly in patients with reduced LVEF, which can be clinically relevant in the follow-up of LV function (4, 12). As a general rule, up to 10% variation between examinations in the same patient cannot be considered a change in LV function (9, 166, 167). Moreover, 2D measurement of LVEF shows beat-to-beat variability up to 6% and can vary with blood pressure, heart rate, and inotropic state (168).
The geometric problems of 2D echocardiography may be overcome by three-dimensional (3D) echocardiography which is less influenced by irregular geometry. However, artifacts and reduced spatial and axial resolution may limit its accuracy in estimating LVEF (169, 170). Contrast-enhanced 2D and 3D echocardiography allow accurate measurement of LV dimensions and volumes in patients with suboptimal imaging (171). CMR provides heart imaging in multiple planes with excellent endocardial definition, making it the reference standard for LVEF among non-invasive techniques. However, it is expensive, time-consuming, and not widely available in clinical practices. CT scan and SPECT are also accurate in LVEF measurement but have the disadvantage of needing contrast and radiation exposure (8).
6 Conclusions
Although LVEF is a widely accepted method for assessing LV function in most guidelines, it is an artificial measure with no comparable physiological measurement. It only describes the volumetric alterations of LV and the relationship between LV filling and ejection, which can be unrelated to myocardial systolic function. Echocardiographic assessment of LVEF plays a key role in the diagnostic and prognostic evaluation of HF patients. However, because of its sensitivity to loading conditions, EF reflects cavity systolic performance as a whole, rather than myocardial contractile function. The impact of such limitation significantly affects the right time for decision-making for valve replacement and ICD implantation, particularly in asymptomatic patients. The need for early detection of subclinical changes in systolic function and myocardial performance implies the use of other parameters beyond LVEF including echocardiographic GLS and CMR. However, their routine and inclusion in guidelines require prospective randomized studies.
Author contributions
FV: Conceptualization, Writing – original draft. GL: Writing – original draft. MH: Supervision, Writing – review & editing.
Funding
The author(s) declare that no financial support was received for the research, authorship, and/or publication of this article.
Conflict of interest
The authors declare that the research was conducted in the absence of any commercial or financial relationships that could be construed as a potential conflict of interest.
The author(s) declared that they were an editorial board member of Frontiers, at the time of submission. This had no impact on the peer review process and the final decision.
Publisher's note
All claims expressed in this article are solely those of the authors and do not necessarily represent those of their affiliated organizations, or those of the publisher, the editors and the reviewers. Any product that may be evaluated in this article, or claim that may be made by its manufacturer, is not guaranteed or endorsed by the publisher.
References
1. Rossignol P, Hernandez AF, Solomon SD, Zannad F. Heart failure drug treatment. Lancet. (2019) 393:1034–44. doi: 10.1016/S0140-6736(18)31808-7
2. McDonagh TA, Metra M, Adamo M, Gardner RS, Baumbach A, Böhm M, et al. ESC Guidelines for the diagnosis and treatment of acute and chronic heart failure: developed by the task force for the diagnosis and treatment of acute and chronic heart failure of the European Society of Cardiology (ESC) with the special contribution of the Heart Failure Association (HFA) of the ESC. Eur Heart J. (2021) 2021(42):3599–726. doi: 10.1093/eurheartj/ehab368
3. Burns RJ, Gibbons RJ, Yi Q, Roberts RS, Miller TD, Schaer GL, et al. The relationships of left ventricular ejection fraction, end-systolic volume index and infarct size to six-month mortality after hospital discharge following myocardial infarction treated by thrombolysis. J Am Coll Cardiol. (2002) 39:30–6. doi: 10.1016/S0735-1097(01)01711-9
4. Lenell J, Lindahl B, Karlsson P, Batra G, Erlinge D, Jernberg T, et al. Reliability of estimating left ventricular ejection fraction in clinical routine: a validation study of the SWEDEHEART registry. Clin Res Cardiol. (2023) 112:68–74. doi: 10.1007/s00392-022-02031-0
5. Vahanian A, Beyersdorf F, Praz F, Milojevic M, Baldus S, Bauersachs J, et al. 2021 ESC/EACTS guidelines for the management of valvular heart disease: developed by the task force for the management of valvular heart disease of the European Society of Cardiology (ESC) and the European Association for Cardio-Thoracic Surgery (EACTS). Eur Heart J. (2021) 43:561–632. doi: 10.1093/eurheartj/ehab395
6. Abdelhamid M, Rosano G, Metra M, Adamopoulos S, Böhm M, Chioncel O, et al. Prevention of sudden death in heart failure with reduced ejection fraction: do we still need an implantable cardioverter-defibrillator for primary prevention? Eur J Heart Fail. (2022) 24:1460–6. doi: 10.1002/ejhf.2594
7. Cameli M, Mondillo S, Solari M, Righini FM, Andrei V, Contaldi C, et al. Echocardiographic assessment of left ventricular systolic function: from ejection fraction to torsion. Heart Fail Rev. (2016) 21:77–94. doi: 10.1007/s10741-015-9521-8
8. Marwick TH. Ejection fraction pros and cons: JACC state-of-the-art review. J Am Coll Cardiol. (2018) 72:2360. doi: 10.1016/j.jacc.2018.08.2162
9. Klaeboe LG, Edvardsen T. Echocardiographic assessment of left ventricular systolic function. J Echocardiogr. (2019) 17:10–6. doi: 10.1007/s12574-018-0405-5
10. Konstam MA, Abboud FM. Ejection fraction: misunderstood and overrated (changing the paradigm in categorizing heart failure). Circulation. (2017) 135:717–9. doi: 10.1161/CIRCULATIONAHA.116.025795
11. Severino P, Maestrini V, Mariani MV, Birtolo LI, Scarpati R, Mancone M, et al. Structural and myocardial dysfunction in heart failure beyond ejection fraction. Heart Fail Rev. (2020) 25:9–17. doi: 10.1007/s10741-019-09828-8
12. Cikes M, Solomon SD. Beyond ejection fraction: an integrative approach for assessment of cardiac structure and function in heart failure. Eur Heart J. (2016) 37:1642–50. doi: 10.1093/eurheartj/ehv510
13. Yancy CW, Jessup M, Bozkurt B, Butler J, Casey DE, Colvin MM, et al. 2017 ACC/AHA/HFSA focused update of the 2013 ACCF/AHA guideline for the management of heart failure. J Am Coll Cardiol. (2017) 70:776–803. doi: 10.1016/j.jacc.2017.04.025
14. Bozkurt B, Coats AJS, Tsutsui H, et al. Universal definition and classification of heart failure a report of the Heart Failure Society of America, Heart Failure Association of the European Society of Cardiology, Japanese Heart Failure Society and Writing Committee of the Universal Definition of Heart Failure. J Card Fail. (2021) 27:387–413. doi: 10.1016/j.cardfail.2021.01.022
15. Heidenreich PA, Bozkurt B, Aguilar D, Allen LA, Byun JJ, Colvin MM, et al. 2022 AHA/ACC/HFSA guideline for the management of heart failure: a report of the American college of cardiology/American heart association joint committee on clinical practice guidelines. Circulation. (2022) 145:e895. doi: 10.1161/CIR.0000000000001063
16. Tsutsui H, Ide T, Ito H, Kihara Y, Kinugawa K, Kinugawa S, et al. JCS/JHFS 2021 guideline focused update on diagnosis and treatment of acute and chronic heart failure. Circ J. (2021) 85:2252–91. doi: 10.1253/circj.CJ-21-0431
17. Atherton JJ, Sindone A, De Pasquale CG, Driscoll A, MacDonald PS, Hopper I, et al. National heart foundation of Australia and cardiac society of Australia and New Zealand: guidelines for the prevention, detection, and management of heart failure in Australia 2018. Heart Lung Circ. (2018) 27:1123–208. doi: 10.1016/j.hlc.2018.06.1042
18. Harkness A, Ring L, Augustine DX, Oxborough D, Robinson S, Sharma V. Normal reference intervals for cardiac dimensions and function for use in echocardiographic practice: a guideline from the British society of echocardiography. Echo Res Pract. (2020) 7:G1–G18. doi: 10.1530/ERP-19-0053
19. Lang RM, Badano LP, Mor-Avi V, Afilalo J, Armstrong AE, Ernande L, et al. Recommendations for cardiac chamber quantification by echocardiography in adults: an update from the American Society of Echocardiography and the European Association of Cardiovascular Imaging. J Am Soc Echocardiogr. (2015) 28:1–39.e14. doi: 10.1016/j.echo.2014.10.003
20. Hudson S, Pettit S. What is ‘normal’ left ventricular ejection fraction? Heart. (2020) 106:1445–6. doi: 10.1136/heartjnl-2020-317604
21. Tsao CW, Lyass A, Larson MG, Cheng S, Lam CSP, Aragam JR, et al. Prognosis of adults with borderline left ventricular ejection fraction. JACC Heart Fail. (2016) 4:502–10. doi: 10.1016/j.jchf.2016.03.003
22. Fonarow GC, Hsu JJ. Left ventricular ejection fraction: what is “normal”? JACC Heart Fail. (2016) 4:511–3. doi: 10.1016/j.jchf.2016.03.021
23. Bozkurt B, Ezekowitz J. Substance and substrate: lVEF and sex subgroup analyses of PARAGON-HF and PARADIGM-HF trials. Circulation. (2020) 141:362–6. doi: 10.1161/CIRCULATIONAHA.120.045008
24. Zheng SL, Chan FT, Nabeebaccus AA, Shah AM, McDonagh T, Okonko DO, et al. Drug treatment effects on outcomes in heart failure with preserved ejection fraction: a systematic review and meta-analysis. Heart. (2018) 104:407–15. doi: 10.1136/heartjnl-2017-311652
25. Lam CSP, Solomon SD. The middle child in heart failure: heart failure with mid-range ejection fraction (40-50%). Eur J Heart Fail. (2014) 16:1049–55. doi: 10.1002/ejhf.159
26. Solomon SD, McMurray JJV, Anand IS, Ge J, Lam CSP, Maggioni AP, et al. Angiotensin–neprilysin inhibition in heart failure with preserved ejection fraction. N Engl J Med. (2019) 381:1609–20. doi: 10.1056/NEJMoa1908655
27. Rosch S, Kresoja K-P, Besler C, Fengler K, Schöber AR, Roeder MV, et al. Characteristics of heart failure with preserved ejection fraction across the range of left ventricular ejection fraction. Circulation. (2022) 146:506–18. doi: 10.1161/CIRCULATIONAHA.122.059280
28. Palazzuoli A, Correale M, Iacoviello M, Gronda E. Does the measurement of ejection fraction still make sense in the HFpEF framework? What recent trials suggest. J Clin Med. (2023) 12:693. doi: 10.3390/jcm12020693
29. Zakeri R, Cowie MR. Heart failure with preserved ejection fraction: controversies, challenges and future directions. Heart. (2018) 104:377–84. doi: 10.1136/heartjnl-2016-310790
30. Mentz RJ, Kelly JP, von Lueder TG, Voors AA, Lam CSP, Cowie MR, et al. Noncardiac comorbidities in heart failure with reduced versus preserved ejection fraction. J Am Coll Cardiol. (2014) 64:2281–93. doi: 10.1016/j.jacc.2014.08.036
31. Ibrahim NE, Song Y, Cannon CP, Doros G, Russo P, Ponirakis A, et al. Heart failure with mid-range ejection fraction: characterization of patients from the PINNACLE registry. ESC Heart Fail. (2019) 6:784–92. doi: 10.1002/ehf2.12455
32. Rastogi A, Novak E, Platts AE, Mann DL. Epidemiology, pathophysiology and clinical outcomes for heart failure patients with a mid-range ejection fraction. Eur J Heart Fail. (2017) 19:1597–605. doi: 10.1002/ejhf.879
33. Dunlay SM, Roger VL, Weston SA, Jiang R, Redfield MM. Longitudinal changes in ejection fraction in heart failure patients with preserved and reduced ejection fraction. Circ Heart Fail. (2012) 5:720–6. doi: 10.1161/CIRCHEARTFAILURE.111.966366
34. Packer M. Heart failure with a mid-range ejection fraction: a disorder that a psychiatrist would love. JACC Heart Fail. (2017) 5:805–7. doi: 10.1016/j.jchf.2017.08.012
35. Wilcox JE, Fang JC, Margulies KB, Mann DL. Heart failure with recovered left ventricular ejection fraction JACC scientific expert panel. J Am Coll Cardiol. (2020) 76:719–34. doi: 10.1016/j.jacc.2020.05.075
36. Halliday BP, Wassall R, Lota AS, Khalique Z, Gregson J, Newsome S, et al. Withdrawal of pharmacological treatment for heart failure in patients with recovered dilated cardiomyopathy (TRED-HF): an open-label, pilot, randomised trial. Lancet. (2019) 393:61–73. doi: 10.1016/S0140-6736(18)32484-X
37. Solomon SD, Glynn RJ, Greaves S, Ajani U, Rouleau J-L, Menapace F, et al. Recovery of ventricular function after myocardial infarction in the reperfusion era: the healing and early afterload reducing therapy study. Ann Intern Med. (2001) 134:451–8. doi: 10.7326/0003-4819-134-6-200103200-00009
38. Sjöblom J, Muhrbeck J, Witt N, Alam M, Frykman-Kull V. Evolution of left ventricular ejection fraction after acute myocardial infarction: implications for implantable cardioverter-defibrillator eligibility. Circulation. (2014) 130:743–8. doi: 10.1161/CIRCULATIONAHA.114.009924
39. Tanabe K, Sakamoto T. Heart failure with recovered ejection fraction. J Echocardiogr. (2019) 17:5–9. doi: 10.1007/s12574-018-0396-2
40. Basuray A, French B, Ky B, et al. Heart failure with recovered ejection fraction: clinical description, biomarkers, and outcomes. Circulation. (2014) 129:2380–7. doi: 10.1161/CIRCULATIONAHA.113.006855
41. Punnoose LRMD, Givertz MMMD, Lewis EFMDMPH, Pratibhu PMBAMPH, Stevenson LWMD, Desai ASMDMPH. Heart failure with recovered ejection fraction: a distinct clinical entity. J Card Fail. (2011) 17:527–32. doi: 10.1016/j.cardfail.2011.03.005
42. Merken J, Brunner-La Rocca H-P, Weerts J, Verdonschot J, Hazebroek M, Schummers G, et al. Heart failure with recovered ejection fraction. J Am Coll Cardiol. (2018) 72:1557–8. doi: 10.1016/j.jacc.2018.06.070
43. Solomon SD, Anavekar N, Pfeffer MA, Skali H, McMurray JJV, Swedberg K, et al. Influence of ejection fraction on cardiovascular outcomes in a broad spectrum of heart failure patients. Circulation. (2005) 112:3738–44. doi: 10.1161/CIRCULATIONAHA.105.561423
44. Heidenreich P. What is a normal left ventricular ejection fraction? Circulation. (2023) 148:750–2. doi: 10.1161/CIRCULATIONAHA.123.065791
45. Aimo A, Januzzi JL, Vergaro G, Petersen C, Pasanisi EM, Molinaro S, et al. Left ventricular ejection fraction for risk stratification in chronic systolic heart failure. Int J Cardiol. (2018) 273:136–40. doi: 10.1016/j.ijcard.2018.07.117
46. Carluccio E, Dini FL, Biagioli P, Lauciello R, Simioniuc A, Zuchi C, et al. The ‘Echo heart failure score': an echocardiographic risk prediction score of mortality in systolic heart failure. Eur J Heart Fail. (2013) 15:868–76. doi: 10.1093/eurjhf/hft038
47. Parikh RV, Go AS, Bhatt AS, Tan TC, Allen AR, Feng KY, et al. Developing clinical risk prediction models for worsening heart failure events and death by left ventricular ejection fraction. J Am Heart Assoc. (2023) 12:e029736-e. doi: 10.1161/JAHA.122.029736
48. Shah KS, Xu H, Matsouaka RA, Bhatt DL, Heidenreich PA, Hernandez AF, et al. Heart failure with preserved, borderline, and reduced ejection fraction; 5-year outcomes. J Am Coll Cardiol. (2017) 70:2476–86. doi: 10.1016/j.jacc.2017.08.074
49. Bhatia RS, Tu JV, Lee DS, Austin PC, Fang J, Haouzi A, et al. Outcome of heart failure with preserved ejection fraction in a population-based study. N Engl J Med. (2006) 355:260–9. doi: 10.1056/NEJMoa051530
50. Wehner GJ, Jing L, Haggerty CM, Suever JD, Leader JB, Hartzel DN, et al. Routinely reported ejection fraction and mortality in clinical practice: where does the nadir of risk lie? Eur Heart J. (2020) 41:1249–57. doi: 10.1093/eurheartj/ehz550
51. Ono R, Falcão LM. Supra-normal left ventricular function. Am J Cardiol. (2023) 207:84–92. doi: 10.1016/j.amjcard.2023.08.169
52. Potter E, Marwick TH. Assessment of left ventricular function by echocardiography: the case for routinely adding global longitudinal strain to ejection fraction. JACC Cardiovasc Imaging. (2018) 11:260–74. doi: 10.1016/j.jcmg.2017.11.017
53. Bosseau C, Galli E, Donal E. Prognostic value of BNP in heart failure with preserved or reduced ejection fraction. Heart. (2015) 101:1855–6. doi: 10.1136/heartjnl-2015-308515
54. van Veldhuisen DJ, Linssen GCM, Jaarsma TRN, van Gilst WH, Hoes AW, Tijssen JGP, et al. B-type natriuretic peptide and prognosis in heart failure patients with preserved and reduced ejection fraction. J Am Coll Cardiol. (2013) 61:1498–506. doi: 10.1016/j.jacc.2012.12.044
55. Lam CSP, Gamble GD, Ling LH, Sim D, Leong KTG, Yeo PSD, et al. Mortality associated with heart failure with preserved vs. Reduced ejection fraction in a prospective international multi-ethnic cohort study. Eur Heart J. (2018) 39:1770–80. doi: 10.1093/eurheartj/ehy005
56. Bilchick KC, Stafford P, Laja O, Elumogo C, Bediako P, Tolbert N, et al. Relationship of ejection fraction and natriuretic peptide trajectories in heart failure with baseline reduced and mid-range ejection fraction. The Am Heart J. (2022) 243:1–10. doi: 10.1016/j.ahj.2021.08.015
57. Tromp J, Westenbrink BD, Ouwerkerk W, van Veldhuisen DJ, Samani NJ, Ponikowski P, et al. Identifying pathophysiological mechanisms in heart failure with reduced versus preserved ejection fraction. J Am Coll Cardiol. (2018) 72:1081–90. doi: 10.1016/j.jacc.2018.06.050
58. Hage C, Michaëlsson E, Linde C, Donal E, Daubert J-C, Gan L-M, et al. Inflammatory biomarkers predict heart failure severity and prognosis in patients with heart failure with preserved ejection fraction: a holistic proteomic approach. Circ Cardiovasc Genet. (2017) 10:e001633-e. doi: 10.1161/CIRCGENETICS.116.001633
59. Takvorian KS, Wang D, Courchesne P, Vasan RS, Benjamin EJ, Cheng S, et al. The association of protein biomarkers with incident heart failure with preserved and reduced ejection fraction. Circ Heart Fail. (2023) 16:e009446-e. doi: 10.1161/CIRCHEARTFAILURE.121.009446
60. Michelhaugh SA, Camacho A, Ibrahim NE, Gaggin H, D'Alessandro D, Coglianese E, et al. Proteomic signatures during treatment in different stages of heart failure. Circ Heart Fail. (2020) 13:e006794-e. doi: 10.1161/CIRCHEARTFAILURE.119.006794
61. Adamo L, Yu J, Rocha-Resende C, Javaheri A, Head RD, Mann DL. Proteomic signatures of heart failure in relation to left ventricular ejection fraction. J Am Coll Cardiol. (2020) 76:1982–94. doi: 10.1016/j.jacc.2020.08.061
62. Yip GW-K, Zhang Q, Xie J-M, Liang Y-J, Liu Y-M, Yan B, et al. Resting global and regional left ventricular contractility in patients with heart failure and normal ejection fraction: insights from speckle-tracking echocardiography. Heart. (2011) 97:287–94. doi: 10.1136/hrt.2010.205815
63. Daubert MA. Diastolic function in heart failure with reduced ejection fraction: the overlooked prognosticator? JACC Heart Fail. (2019) 7:818–20. doi: 10.1016/j.jchf.2019.06.005
64. Benfari G, Miller WL, Antoine C, Rossi A, Lin G, Oh JK, et al. Diastolic determinants of excess mortality in heart failure with reduced ejection fraction. JACC: Heart Fail. (2019) 7:808–17. doi: 10.1016/j.jchf.2019.04.024
65. Halliday BP, Senior R, Pennell DJ. Assessing left ventricular systolic function: from ejection fraction to strain analysis. Eur Heart J. (2021) 42:789–97. doi: 10.1093/eurheartj/ehaa587
66. Triposkiadis F, Giamouzis G, Boudoulas KD, Karagiannis G, Skoularigis J, Boudoulas H, et al. Left ventricular geometry as a major determinant of left ventricular ejection fraction: physiological considerations and clinical implications: left ventricular geometry determines ejection fraction. Eur J Heart Fail. (2018) 20:436–44. doi: 10.1002/ejhf.1055
67. Sallin EA. Fiber orientation and ejection fraction in the human left ventricle. Biophys J. (1969) 9:954–64. doi: 10.1016/S0006-3495(69)86429-5
68. Buckberg GD, Nanda NC, Nguyen C, Kocica MJ. What is the heart? Anatomy, function, pathophysiology, and misconceptions. J Cardiovasc Dev Dis. (2018) 5:33. doi: 10.3390/jcdd5020033
69. Greenbaum RA, Ho SY, Gibson DG, Becker AE, Anderson RH. Left ventricular fibre architecture in man. Br Heart J. (1981) 45:248–63. doi: 10.1136/hrt.45.3.248
70. Ho SY. Anatomy and myoarchitecture of the left ventricular wall in normal and in disease. Eur J Echocardiogr. (2009) 10:iii3–7. doi: 10.1093/ejechocard/jen243
71. Anderson RH, Ho SY, Redmann K, Sanchez-Quintana D, Lunkenheimer PP. The anatomical arrangement of the myocardial cells making up the ventricular mass. Eur J Cardiothorac Surg. (2005) 28:517–25. doi: 10.1016/j.ejcts.2005.06.043
72. Sengupta PP, Korinek J, Belohlavek M, Narula J, Vannan MA, Jahangir A, et al. Left ventricular structure and function: basic science for cardiac imaging. J Am Coll Cardiol. (2006) 48:1988–2001. doi: 10.1016/j.jacc.2006.08.030
73. Sengupta PP, Tajik AJ, Chandrasekaran K, Khandheria BK. Twist mechanics of the left ventricle: principles and application. JACC Cardiovasc Imaging. (2008) 1:366–76. doi: 10.1016/j.jcmg.2008.02.006
74. Kraigher-Krainer E, Shah AM, Gupta DK, Santos A, Claggett B, Pieske B, et al. Impaired systolic function by strain imaging in heart failure with preserved ejection fraction. J Am Coll Cardiol. (2014) 63:447–56. doi: 10.1016/j.jacc.2013.09.052
75. Stokke TM, Hasselberg NE, Smedsrud MK, Sarvari SI, Haugaa KH, Smiseth OA, et al. Geometry as a confounder when assessing ventricular systolic function: comparison between ejection fraction and strain. J Am Coll Cardiol. (2017) 70:942–54. doi: 10.1016/j.jacc.2017.06.046
76. Szelényi Z, Fazakas Á, Szénási G, Tegze N, Fekete B, Molvarec A, et al. The mechanism of reduced longitudinal left ventricular systolic function in hypertensive patients with normal ejection fraction. J Hypertens. (2015) 33:1962–9. doi: 10.1097/HJH.0000000000000624
77. Rodrigues JCL, Rohan S, Dastidar AG, Trickey A, Szantho G, Ratcliffe LEK, et al. The relationship between left ventricular wall thickness, myocardial shortening, and ejection fraction in hypertensive heart disease: insights from cardiac magnetic resonance imaging. J Clin Hypertens. (2016) 18:1119–27. doi: 10.1111/jch.12849
78. Aurigemma GP, Silver KH, Priest MA, Gaasch WH. Geometric changes allow normal ejection fraction despite depressed myocardial shortening in hypertensive left ventricular hypertrophy. J Am Coll Cardiol. (1995) 26:195–202. doi: 10.1016/0735-1097(95)00153-Q
79. Kitai T, Okada Y, Shomura Y, Tani T, Kaji S, Kita T, et al. Timing of valve repair for severe degenerative mitral regurgitation and long-term left ventricular function. J Thorac Cardiovasc Surg. (2014) 148:1978–82. doi: 10.1016/j.jtcvs.2014.01.041
80. Tribouilloy C, Rusinaru D, Grigioni F, Michelena HI, Vanoverschelde J-L, Avierinos J-F, et al. Long-term mortality associated with left ventricular dysfunction in mitral regurgitation due to flail leaflets: a multicenter analysis. Circ Cardiovasc Imaging. (2014) 7:363–70. doi: 10.1161/CIRCIMAGING.113.001251
81. Gaasch WH, Meyer TE. Left ventricular response to mitral regurgitation: implications for management. Circulation. (2008) 118:2298–303. doi: 10.1161/CIRCULATIONAHA.107.755942
82. Galli E, Lancellotti P, Sengupta PP, Donal E. LV Mechanics in mitral and aortic valve diseases: value of functional assessment beyond ejection fraction. JACC Cardiovas Imaging. (2014) 7:1151–66. doi: 10.1016/j.jcmg.2014.07.015
83. Gaasch WH, Shah SP, Labib SB, Meyer TE. Impedance to retrograde and forward flow in chronic mitral regurgitation and the physiology of a double outlet ventricle. Heart. (2017) 103:581–5. doi: 10.1136/heartjnl-2016-309747
84. Kitkungvan D, Nabi F, Kim RJ, Bonow RO, Khan MA, Xu J, et al. Myocardial fibrosis in patients with primary mitral regurgitation with and without prolapse. J Am Coll Cardiol. (2018) 72:823–34. doi: 10.1016/j.jacc.2018.06.048
85. Constant Dit, Beaufils A-L, Huttin O, Jobbe-Duval A, Senage T, Filippetti L, Piriou N, et al. Replacement myocardial fibrosis in patients with mitral valve prolapse relation to mitral regurgitation, ventricular remodeling, and arrhythmia. Circulation. (2021) 143:1763–74. doi: 10.1161/CIRCULATIONAHA.120.050214
86. Lisi M, Cameli M, Mandoli GE, Pastore MC, Righini FM, D'Ascenzi F, et al. Detection of myocardial fibrosis by speckle-tracking echocardiography: from prediction to clinical applications. Heart Fail Rev. (2022) 27:1857–67. doi: 10.1007/s10741-022-10214-0
87. Ahmed MI, Gladden J, Litovsky SH, Lloyd SG, Gupta H, Inusah S, et al. Increased oxidative stress and cardiomyocyte myofibrillar degeneration in patients with chronic isolated mitral regurgitation and ejection fraction >60. J Am Coll Cardiol. (2010) 55:671–9. doi: 10.1016/j.jacc.2009.08.074
88. Edwards NC, Moody WE, Yuan M, Weale P, Neal D, Townend JN, et al. Quantification of left ventricular interstitial fibrosis in asymptomatic chronic primary degenerative mitral regurgitation. Circ Cardiovasc Imaging. (2014) 7:946–53. doi: 10.1161/CIRCIMAGING.114.002397
89. Teraguchi I, Hozumi T, Takemoto K, Ota S, Kashiwagi M, Shimamura K, et al. Assessment of decreased left ventricular longitudinal deformation in asymptomatic patients with organic mitral regurgitation and preserved ejection fraction using tissue-tracking mitral annular displacement by speckle-tracking echocardiography. Echocardiography. (2019) 36:678–86. doi: 10.1111/echo.14290
90. Maniar HS, Brady BD, Lee U, Cupps BP, Kar J, Wallace K, et al. Early left ventricular regional contractile impairment in chronic mitral regurgitation occurs in a consistent, heterogeneous pattern. J Thorac Cardiovasc Surg. (2014) 148:1694–9. doi: 10.1016/j.jtcvs.2014.05.088
91. Otto CM, Nishimura RA, Bonow RO, Carabello BA, Erwin JP, Gentile F, et al. 2020 ACC/AHA guideline for the management of patients with valvular heart disease: a report of the American College of Cardiology/American Heart Association joint committee on clinical practice guidelines. Circulation. (2021) 143:e72–e227. doi: 10.1161/CIR.0000000000000923
92. Suri RM, Vanoverschelde J-L, Grigioni F, Schaff HV, Tribouilloy C, Avierinos J-F, et al. Association between early surgical intervention vs watchful waiting and outcomes for mitral regurgitation due to flail mitral valve leaflets. JAMA. (2013) 310:609–16. doi: 10.1001/jama.2013.8643
93. Kang D-H, Jeong Hoon KIM, Ji Hye RIM, Kim M-J, Yun S-C, Song J-M, et al. Comparison of early surgery versus conventional treatment in asymptomatic severe mitral regurgitation. Circulation. (2009) 119:797–804. doi: 10.1161/CIRCULATIONAHA.108.802314
94. Montant P, Chenot F, Robert A, Vancraeynest D, Pasquet A, Gerber B, et al. Long-term survival in asymptomatic patients with severe degenerative mitral regurgitation: a propensity score–based comparison between an early surgical strategy and a conservative treatment approach. J Thorac Cardiovasc Surg. (2009) 138:1339–48. doi: 10.1016/j.jtcvs.2009.03.046
95. Quintana E, Suri RM, Thalji NM, Daly RC, Dearani JA, Burkhart HM, et al. Left ventricular dysfunction after mitral valve repair—the fallacy of “normal” preoperative myocardial function. J Thorac Cardiovasc Surg. (2014) 148:2752–62. doi: 10.1016/j.jtcvs.2014.07.029
96. Calin A, Mateescu AD, Popescu AC, Bing R, Dweck MR, Popescu BA. Role of advanced left ventricular imaging in adults with aortic stenosis. Heart. (2020) 106:962–9. doi: 10.1136/heartjnl-2019-315211
97. Carabello BAP, Paulus WJP. Aortic stenosis. Lancet. (2009) 373:956–66. doi: 10.1016/S0140-6736(09)60211-7
98. Dweck MR, Boon NA, Newby DE. Calcific aortic stenosis: a disease of the valve and the myocardium. J Am Coll Cardiol. (2012) 60:1854–63. doi: 10.1016/j.jacc.2012.02.093
99. Pibarot P, Dumesnil JG. Paradoxical low-flow, low-gradient aortic stenosis: new evidence, more questions. Circulation. (2013) 128:1729–32. doi: 10.1161/CIRCULATIONAHA.113.005718
100. Lindman BR, Dweck MR, Lancellotti P, Généreux P, Piérard LA, O'Gara PT, et al. Management of asymptomatic severe aortic stenosis: evolving concepts in timing of valve replacement. JACC Cardiovasc Imaging. (2020) 13:481–93. doi: 10.1016/j.jcmg.2019.01.036
101. Patel KP, Baumbach A. Future of transcatheter aortic valve implantation: where do we go from here? Heart. (2023) 109:564–71. doi: 10.1136/heartjnl-2022-321575
102. Généreux P, Stone GW, O'Gara PT, Marquis-Gravel G, Redfors B, Giustino G, et al. Natural history, diagnostic approaches, and therapeutic strategies for patients with asymptomatic severe aortic stenosis. J Am Coll Cardiol. (2016) 67:2263–88. doi: 10.1016/j.jacc.2016.02.057
103. Román JAS, Vilacosta I, Antunes MJ, Iung B, Lopez J, Schäfers H-J. The ‘wait for symptoms’ strategy in asymptomatic severe aortic stenosis. Heart. (2020) 106:1792–7. doi: 10.1136/heartjnl-2020-317323
104. Everett RJ, Tastet L, Clavel M-A, Chin CWL, Capoulade R, Vassiliou VS, et al. Progression of hypertrophy and myocardial fibrosis in aortic stenosis: a multicenter cardiac magnetic resonance study. Circ Cardiovasc Imaging. (2018) 11:e007451-e. doi: 10.1161/CIRCIMAGING.117.007451
105. Magne J, Cosyns B, Popescu BA, Carstensen HG, Dahl J, Desai MY, et al. Distribution and prognostic significance of left ventricular global longitudinal strain in asymptomatic significant aortic stenosis: an individual participant data meta-analysis. JACC Cardiovascular Imaging. (2019) 12:84–92. doi: 10.1016/j.jcmg.2018.11.005
106. Vollema EM, Sugimoto T, Shen M, Tastet L, Ng ACT, Abou R, et al. Association of left ventricular global longitudinal strain with asymptomatic severe aortic stenosis: natural course and prognostic value. JAMA Cardiology. (2018) 3:839–47. doi: 10.1001/jamacardio.2018.2288
107. Ng ACT, Prihadi EA, Antoni ML, Bertini M, Ewe SH, Ajmone Marsan N, et al. Left ventricular global longitudinal strain is predictive of all-cause mortality independent of aortic stenosis severity and ejection fraction. Eur Heart J Cardiovasc Imaging. (2018) 19:859–67. doi: 10.1093/ehjci/jex189
108. Anastasiou V, Daios S, Bazmpani M-A, Moysidis DV, Zegkos T, Karamitsos T, et al. Shifting from left ventricular ejection fraction to strain imaging in aortic stenosis. Diagnostics. (2023) 13:1756. doi: 10.3390/diagnostics13101756
109. Marcoff L, Gillam LD. Aortic stenosis: risk stratification and timing of surgery. Curr Cardiol Rep. (2023) 25:89–95. doi: 10.1007/s11886-022-01835-w
110. Bohbot Y, de Meester de Ravenstein C, Chadha G, Rusinaru D, Belkhir K, Trouillet C, et al. Relationship between left ventricular ejection fraction and mortality in asymptomatic and minimally symptomatic patients with severe aortic stenosis. JACC Cardiovasc Imaging. (2019) 12:38–48. doi: 10.1016/j.jcmg.2018.07.029
111. Taniguchi T, Ando K, Kanamori N, Murata K, Kadota K, Sasa T, et al. Prognostic impact of left ventricular ejection fraction in patients with severe aortic stenosis. JACC Cardiovasc Interventions. (2018) 11:145–57. doi: 10.1016/j.jcin.2017.08.036
112. Ito S, Pislaru C, Miranda WR, Nkomo VT, Connolly HM, Pislaru SV, et al. Left ventricular contractility and wall stress in patients with aortic stenosis with preserved or reduced ejection fraction. JACC Cardiovasc Imaging. (2020) 13:357–69. doi: 10.1016/j.jcmg.2019.01.009
113. Perry AS, Li S. Optimal threshold of left ventricular ejection fraction for aortic valve replacement in asymptomatic severe aortic stenosis: a systematic review and meta-analysis. J Am Heart Assoc. (2021) 10:e020252-e. doi: 10.1161/JAHA.120.020252
114. Banovic M, Putnik S, Penicka M, Doros G, Deja MA, Kockova R, et al. Aortic valve replacement versus conservative treatment in asymptomatic severe aortic stenosis: the AVATAR trial. Circulation. (2022) 145:648–58. doi: 10.1161/CIRCULATIONAHA.121.057639
115. Kang D-H, Park S-J, Lee S-A, Lee S, Kim D-H, Kim H-K, et al. Early surgery or conservative care for asymptomatic aortic stenosis. N Engl J Med. (2019) 382:111–9. doi: 10.1056/NEJMoa1912846
116. Tsampasian V, Grafton-Clarke C, Gracia Ramos AE, Asimakopoulos G, Garg P, Prasad S, et al. Management of asymptomatic severe aortic stenosis: a systematic review and meta-analysis. Open Heart. (2022) 9:e001982. doi: 10.1136/openhrt-2022-001982
117. Taniguchi T, Morimoto T, Takeji Y, Kato T, Kimura T. Contemporary issues in severe aortic stenosis: review of current and future strategies from the contemporary outcomes after surgery and medical treatment in patients with severe aortic stenosis registry. Heart. (2020) 106:802–9. doi: 10.1136/heartjnl-2019-315672
118. Dahou A, Bartko PE, Capoulade R, Clavel M-A, Mundigler G, Grondin SL, et al. Usefulness of global left ventricular longitudinal strain for risk stratification in low ejection fraction, low-gradient aortic stenosis: results from the multicenter true or Pseudo-severe aortic stenosis study. Circ Cardiovasc Imaging. (2015) 8:e002117-e. doi: 10.1161/CIRCIMAGING.114.002117
119. Carstensen HG, Larsen LH, Hassager C, Kofoed KF, Jensen JS, Mogelvang R. Basal longitudinal strain predicts future aortic valve replacement in asymptomatic patients with aortic stenosis. Eur Heart J Cardiovasc Imaging. (2016) 17:283–92. doi: 10.1093/ehjci/jev143
120. Wisenbaugh T, Spann JF, Carabello BA. Differences in myocardial performance and load between patients with similar amounts of chronic aortic versus chronic mitral regurgitation. J Am Coll Cardiol. (1984) 3:916–23. doi: 10.1016/S0735-1097(84)80349-6
121. Carabello BA. Aortic regurgitation: a lesion with similarities to both aortic stenosis and mitral regurgitation. Circulation. (1990) 82:1051–3. doi: 10.1161/01.CIR.82.3.1051
122. Ricci DR. Afterload mismatch and preload reserve in chronic aortic regurgitation. Circulation. (1982) 66:826–34. doi: 10.1161/01.CIR.66.4.826
123. Dujardin KS, Enriquez-Sarano M, Schaff HV, Bailey KR, Seward JB, Tajik AJ. Mortality and morbidity of aortic regurgitation in clinical practice: a long-term follow-up study. Circulation. (1999) 99:1851–7. doi: 10.1161/01.CIR.99.14.1851
124. Enriquez-Sarano M, Tajik AJ. Aortic regurgitation. N Engl J Med. (2004) 351:1539–46. doi: 10.1056/NEJMcp030912
125. Azevedo CF, Nigri M, Higuchi ML, Pomerantzeff PM, Spina GS, Sampaio RO, et al. Prognostic significance of myocardial fibrosis quantification by histopathology and magnetic resonance imaging in patients with severe aortic valve disease. J Am Coll Cardiol. (2010) 56:278–87. doi: 10.1016/j.jacc.2009.12.074
126. Donaldson RM, Florio R, Rickards AF, Bennett JG, Yacoub M, Ross DN, et al. Irreversible morphological changes contributing to depressed cardiac function after surgery for chronic aortic regurgitation. Br Heart J. (1982) 48:589–97. doi: 10.1136/hrt.48.6.589
127. Borer JS, Truter S, Herrold EM, Falcone DJ, Pena M, Carter JN, et al. Myocardial fibrosis in chronic aortic regurgitation: molecular and cellular responses to volume overload. Circulation. (2002) 105:1837–42. doi: 10.1161/01.CIR.0000014419.71706.85
128. Petersen J, Iqbal S, Gedeon N, Kloth B, Pecha S, Yildirim Y, et al. Valvular cardiomyopathy in aortic valve regurgitation correlates with myocardial fibrosis. J Clin Med. (2023) 12:2915. doi: 10.3390/jcm12082915
129. Bonow RO, Picone AL, McIntosh CL, Jones M, Rosing DR, Maron BJ, et al. Survival and functional results after valve replacement for aortic regurgitation from 1976 to 1983: impact of preoperative left ventricular function. Circulation. (1985) 72:1244–56. doi: 10.1161/01.CIR.72.6.1244
130. Alashi A, Mentias A, Abdallah A, Feng K, Gillinov AM, Rodriguez LL, et al. Incremental prognostic utility of left ventricular global longitudinal strain in asymptomatic patients with significant chronic aortic regurgitation and preserved left ventricular ejection fraction. JACC Cardiovasc Imaging. (2018) 11:673–82. doi: 10.1016/j.jcmg.2017.02.016
131. Mentias A, Feng K, Alashi A, Rodriguez LL, Gillinov AM, Johnston DR, et al. Long-Term outcomes in patients with aortic regurgitation and preserved left ventricular ejection fraction. J Am Coll Cardiol. (2016) 68:2144–53. doi: 10.1016/j.jacc.2016.08.045
132. Nahar N, Haque T, Kabiruzzaman M, Khan MAM, Choudhury SR, Malik F-T-N. Assessment of subclinical left ventricular systolic dysfunction in patients with asymptomatic severe aortic stenosis with preserved left ventricular systolic function by three-dimensional speckle tracking echocardiography. Echocardiography. (2023) 40:952–7. doi: 10.1111/echo.15662
133. Yurdakul S, Tayyareci Y, Yildirimturk O, Behramoglu F, Colakoglu Z, Memic K, et al. Progressive subclinical left ventricular systolic dysfunction in severe aortic regurgitation patients with normal ejection fraction: a 24 months follow-up velocity vector imaging study. Echocardiography. (2011) 28:886–91. doi: 10.1111/j.1540-8175.2011.01455.x
134. Ewe SH, Haeck MLA, Ng ACT, Witkowski TG, Auger D, Leong DP, et al. Detection of subtle left ventricular systolic dysfunction in patients with significant aortic regurgitation and preserved left ventricular ejection fraction: speckle tracking echocardiographic analysis. Eur Heart J Cardiovasc Imaging. (2015) 16:992–9.25733208
135. Solomon SD, Zelenkofske S, McMurray JJV, Finn PV, Velazquez E, Ertl G, et al. Sudden death in patients with myocardial infarction and left ventricular dysfunction, heart failure, or both. N Engl J Med. (2005) 352:2581–8. doi: 10.1056/NEJMoa043938
136. McMurray JJV. Systolic heart failure. N Engl J Med. (2010) 362:228–38. doi: 10.1056/NEJMcp0909392
137. Theuns D, Smith T, Hunink M, Bardy GH, Jordaens L. Effectiveness of prophylactic implantation of cardioverter-defibrillators without cardiac resynchronization therapy in patients with ischaemic or non-ischaemic heart disease: a systematic review and meta-analysis. Europace. (2010) 12:1564–70. doi: 10.1093/europace/euq329
138. Narins CR, Aktas MK, Chen AY, McNitt S, Ling FS, Younis A, et al. Arrhythmic and mortality outcomes among ischemic versus non-ischemic cardiomyopathy patients receiving primary implantable cardioverter-defibrillator therapy. JACC Clin Electrophysiol. (2022) 8:1–11. doi: 10.1016/j.jacep.2021.06.020
139. Steinbeck G, Andresen D, Seidl K, Brachmann J, Hoffmann E, Wojciechowski D, et al. Defibrillator implantation early after myocardial infarction. N Engl J Med. (2009) 361:1427–36. doi: 10.1056/NEJMoa0901889
140. Beggs SAS, Jhund PS, Jackson CE, McMurray JJV, Gardner RS. Non-ischaemic cardiomyopathy, sudden death and implantable defibrillators: a review and meta-analysis. Heart. (2018) 104:144–50. doi: 10.1136/heartjnl-2016-310850
141. Køber L, Thune JJ, Nielsen JC, Haarbo J, Videbæk L, Korup E, et al. Defibrillator implantation in patients with nonischemic systolic heart failure. N Engl J Med. (2016) 375:1221–30. doi: 10.1056/NEJMoa1608029
142. Goldberger JJ, Cain ME, Stevenson WG, Zipes DP, Hohnloser SH, Kadish AH, et al. American Heart Association/American College of Cardiology Foundation/heart rhythm society scientific statement on noninvasive risk stratification techniques for identifying patients at risk for sudden cardiac death: a scientific statement from the American Heart Association Council on clinical cardiology committee on electrocardiography and arrhythmias and council on epidemiology and prevention. J Am Coll Cardiol. (2008) 52:1179–99. doi: 10.1016/j.jacc.2008.05.003
143. Gorgels APM, Gijsbers C, de Vreede-Swagemakers J, Lousberg A, Wellens HJJ. Out-of-hospital cardiac arrest-the relevance of heart failure. The Maastricht circulatory arrest registry. Eur Heart J. (2003) 24:1204–9. doi: 10.1016/S0195-668X(03)00191-X
144. Stecker EC, Vickers C, Waltz J, Socoteanu C, John BT, Mariani R, et al. Population-based analysis of sudden cardiac death with and without left ventricular systolic dysfunction. J Am Coll Cardiol. (2006) 47:1161–6. doi: 10.1016/j.jacc.2005.11.045
145. Narayanan K, Reinier K, Uy-Evanado A, Teodorescu C, Chugh H, Marijon E, et al. Frequency and determinants of implantable cardioverter defibrillator deployment among primary prevention candidates with subsequent sudden cardiac arrest in the community. Circulation. (2013) 128:1733–8. doi: 10.1161/CIRCULATIONAHA.113.002539
146. Wellens HJJ, Schwartz PJ, Lindemans FW, Buxton AE, Goldberger JJ, Hohnloser SH, et al. Risk stratification for sudden cardiac death: current status and challenges for the future. Eur Heart J. (2014) 35:1642–51. doi: 10.1093/eurheartj/ehu176
147. Shen L, Jhund PS, Petrie MC, Claggett BL, Barlera S, Cleland JGF, et al. Declining risk of sudden death in heart failure. N Engl J Med. (2017) 377:41–51. doi: 10.1056/NEJMoa1609758
148. Disertori M, Masè M, Rigoni M, Nollo G, Ravelli F. Declining clinical benefit of ICD in heart failure patients: temporal trend of mortality outcomes from randomized controlled trials. J Cardiol. (2020) 75:148–54. doi: 10.1016/j.jjcc.2019.06.001
149. McMurray JJV. The ICD in heart failure — time for a rethink? N Engl J Med. (2016) 375:1283–4. doi: 10.1056/NEJMe1609826
150. Chatterjee NA, Levy WC. Implantable cardioverter-defibrillator therapy in the contemporary era of heart failure therapeutics. JACC Clinical Electrophysiology. (2022) 8:1031–3. doi: 10.1016/j.jacep.2022.06.016
151. Felker GM, Butler J, Ibrahim NE, Pina IL, Maisel A, Bapat D, et al. Implantable cardioverter-defibrillator eligibility after initiation of sacubitril/valsartan in chronic heart failure: insights from PROVE-HF. Circulation. (2021) 144:180–2. doi: 10.1161/CIRCULATIONAHA.121.054034
152. Curtain JP, Docherty KF, Jhund PS, Petrie MC, Inzucchi SE, Køber L, et al. Effect of dapagliflozin on ventricular arrhythmias, resuscitated cardiac arrest, or sudden death in DAPA-HF. Eur Heart J. (2021) 42:3727–38. doi: 10.1093/eurheartj/ehab560
153. Valentim Gonçalves A, Pereira-da-Silva T, Galrinho A, Rio P, Moura Branco L, Soares R, et al. Antiarrhythmic effect of sacubitril-valsartan: cause or consequence of clinical improvement? J Clin Med. (2019) 8:869. doi: 10.3390/jcm8060869
154. Wong B, McDonald K, Keane D. Time to rechallenge primary prevention ICD guidelines. ESC Heart Fai. (2022) 9:3694–7. doi: 10.1002/ehf2.14113
155. Mäkikallio TH, Barthel P, Schneider R, Bauer A, Tapanainen JM, Tulppo MP, et al. Prediction of sudden cardiac death after acute myocardial infarction: role of Holter monitoring in the modern treatment era. Eur Heart J. (2005) 26:762–9. doi: 10.1093/eurheartj/ehi188
156. Dagres N, Hindricks G. Risk stratification after myocardial infarction: is left ventricular ejection fraction enough to prevent sudden cardiac death? Eur Heart J. (2013) 34:1964–71. doi: 10.1093/eurheartj/eht109
157. Pour-Ghaz I, Heckle M, Ifedili I, Kayali S, Nance C, Kabra R, et al. Beyond ejection fraction: novel clinical approaches towards sudden cardiac death risk stratification in patients with dilated cardiomyopathy. Curr Cardiol Rev. (2022) 18:e040821195265-e. doi: 10.2174/1573403X17666210804125939
158. Aro AL, Reinier K, Phan D, Teodorescu C, Uy-Evanado A, Nichols GA, et al. Left-ventricular geometry and risk of sudden cardiac arrest in patients with preserved or moderately reduced left-ventricular ejection fraction. Europace. (2017) 19:1146–52. doi: 10.1093/europace/euw126
159. Kanagasundram A, John RM, Stevenson WG. Sustained monomorphic ventricular tachycardia in nonischemic heart disease: arrhythmia-substrate correlations that inform the approach to ablation. Circ Arrhythm Electrophysiol. (2019) 12:e007312-e. doi: 10.1161/CIRCEP.119.007312
160. Wu KC, Calkins H. Powerlessness of a number: why left ventricular ejection fraction matters less for sudden cardiac death risk assessment. Circ Cardiovasc Imaging. (2016) 9. doi: 10.1161/CIRCIMAGING.116.005519
161. Kosiuk J, Dinov B, Bollmann A, Koutalas E, Mussigbrodt A, Sommer P, et al. Association between ventricular arrhythmias and myocardial mechanical dispersion assessed by strain analysis in patients with nonischemic cardiomyopathy. Clin Res Cardiol. (2015) 104:1072–7. doi: 10.1007/s00392-015-0875-7
162. Abou R, Prihadi EA, Goedemans L, van der Geest R, El Mahdiui M, Schalij MJ, et al. Left ventricular mechanical dispersion in ischaemic cardiomyopathy: association with myocardial scar burden and prognostic implications. Eur Heart J Cardiovasc Imaging. (2020) 21:1227–34. doi: 10.1093/ehjci/jeaa187
163. Pontone G, Guaricci AI, Andreini D, Solbiati A, Guglielmo M, Mushtaq S, et al. Prognostic benefit of cardiac magnetic resonance over transthoracic echocardiography for the assessment of ischemic and nonischemic dilated cardiomyopathy patients referred for the evaluation of primary prevention implantable cardioverter-defibrillator therapy. Circulation Cardiovascular Imaging. (2016) 9. doi: 10.1161/CIRCIMAGING.115.004956
164. Rizzello V. Selection of patients eligible for implantable cardioverter defibrillator: beyond left ventricular ejection fraction. Eur Heart J. (2022) 24:I139–I42. doi: 10.1093/eurheartjsupp/suac087
165. Kim W-JC, Beqiri A, Lewandowski AJ, Puyol-Antón E, Markham DC, King AP, et al. Beyond simpson’s rule: accounting for orientation and ellipticity assumptions. Ultrasound Med Biol. (2022) 48:2476–85. doi: 10.1016/j.ultrasmedbio.2022.07.013
166. Thavendiranathan P, Grant AD, Negishi T, Plana JC, Popovic ZB, Marwick TH. Reproducibility of echocardiographic techniques for sequential assessment of left ventricular ejection fraction and volumes: application to patients undergoing cancer chemotherapy. J Am Coll Cardiol. (2013) 61:77–84. doi: 10.1016/j.jacc.2012.09.035
167. Baron T, Berglund L, Hedin E-M, Flachskampf FA. Test–retest reliability of new and conventional echocardiographic parameters of left ventricular systolic function. Clin Res Cardiol. (2019) 108:355–65. doi: 10.1007/s00392-018-1363-7
168. Wood PW, Choy JB, Nanda NC, Becher H. Left ventricular ejection fraction and volumes: it Depends on the imaging method. Echocardiography. (2014) 31:87–100. doi: 10.1111/echo.12331
169. Lang RM, Badano LP, Tsang W, Adams DH, Agricola E, Buck T, et al. EAE/ASE recommendations for image acquisition and display using three-dimensional echocardiography. Eur Heart J Cardiovasc Imaging. (2012) 13:1–46. doi: 10.1093/ehjci/jer316
170. Dorosz JL, Lezotte DC, Weitzenkamp DA, Allen LA, Salcedo EE. Performance of 3-dimensional echocardiography in measuring left ventricular volumes and ejection fraction: a systematic review and meta-analysis. J Am Coll Cardiol. (2012) 59:1799–808. doi: 10.1016/j.jacc.2012.01.037
Keywords: left ventricular ejection fraction, echocardiography, mitral regurgitation, aortic stenosis, aortic regurgitation, implantable cardioverter defibrillator
Citation: Vancheri F, Longo G and Henein MY (2024) Left ventricular ejection fraction: clinical, pathophysiological, and technical limitations. Front. Cardiovasc. Med. 11:1340708. doi: 10.3389/fcvm.2024.1340708
Received: 18 November 2023; Accepted: 12 January 2024;
Published: 7 February 2024.
Edited by:
Spyridon Katsanos, University General Hospital Attikon, GreeceReviewed by:
Ythan H. Goldberg, Lenox Hill Hospital, United StatesErberto Carluccio, Heart Failure Unit, Italy
© 2024 Vancheri, Longo and Henein. This is an open-access article distributed under the terms of the Creative Commons Attribution License (CC BY). The use, distribution or reproduction in other forums is permitted, provided the original author(s) and the copyright owner(s) are credited and that the original publication in this journal is cited, in accordance with accepted academic practice. No use, distribution or reproduction is permitted which does not comply with these terms.
*Correspondence: Federico Vancheri ZmVkZXJpY28udmFuY2hlcmlAZ21haWwuY29t