- 1Department of Ultrasound, The First College of Clinical Medical Science, China Three Gorges University and Yichang Central People’s Hospital, Yichang, China
- 2Department of Critical Care Medicine, The First College of Clinical Medical Science, China Three Gorges University and Yichang Central People’s Hospital, Yichang, China
Background: Right ventricular systolic dysfunction (RVSD) in patients with sepsis is an area of growing interest, but its prognostic significance remains unclear and additional tools are needed to improve our understanding. Right ventricular free wall strain (RV-FWS) is a relatively new parameter to assess RV function. This study aimed to investigate the potential correlation between impaired RV-FWS and prognostic outcomes in patients with sepsis.
Methods: We prospectively assessed right ventricular function in patients with sepsis within the initial 24 h of their hospital admission. RV-FWS, right ventricular global strain (RV-GS), fractional area change (FAC), and tricuspid annular plane systolic excursion (TAPSE) were examined. RVSD was defined as impaired RV-FWS. Moreover, the association between RVSD and 30-day mortality rate was assessed.
Results: This study included 89 patients. Among them, 27 (30.3%) succumbed to their illness within 30 days. The nonsurviving patients demonstrated significantly lower absolute RV-FWS (−19.7% ± 2.4% vs. −21.1% ± 2.1%, P = 0.008) and RV-GS (−17.7% ± 1.2% vs. −18.4% ± 1.4%, P = 0.032) values than the surviving patients. However, TAPSE and FAC values were not significantly different between the two groups. The optimal cutoff values for RV-FWS, RV-GS, FAC, and TAPSE were −19.0%, −17.9%, 36.5%, and 1.55 cm, respectively. Kaplan–Meier survival curves revealed that patients with impaired RV-FWS and RV-GS demonstrated lower 30-day survival rates, and the predictive performance of RV-FWS (hazard ratio [HR]: 3.97, 95% confidence interval [CI]: 1.85–8.51, P < 0.001) was slightly higher than FAC and TAPSE. However, multivariable Cox regression analysis revealed no association between impaired RV-FWS and mortality outcomes (HR: 1.85, 95% CI: 0.56–6.14, P = 0.316).
Conclusions: Impaired RV-FWS is not associated with short-term mortality outcomes, and RV strain imaging is of limited value in assessing the prognosis of sepsis.
1 Introduction
Left ventricular (LV) dysfunction is a prevalent manifestation of sepsis and is significantly correlated with an adverse prognosis (1–3). However, the function of the right ventricle (RV) has been relatively understudied. Several factors such as inflammatory cytokine activation, hypoxia/hypercapnia-induced vasoconstriction, pressure/volume overload, and myocardial ischemia can lead to RV systolic dysfunction (RVSD) in patients with sepsis (4–6). Precise assessment of RVSD is important for preventing severe right heart failure (7, 8).
At present, an internationally accepted definition of RVSD remains elusive (9, 10). Conventional echocardiography is commonly used to assess RV function; however, it may underestimate the extent of myocardial injury (11, 12). Strain imaging can accurately quantify global and segmental myocardial function by assessing the displacement of myocardial tissue. Cardiac magnetic resonance (CMR) is unaffected by acoustic windows and has a high signal-to-noise ratio, establishing it as the standard for non-invasive assessment of ventricular function (13). Within this field, techniques such as strain-encoded MR and feature tracking imaging can measure RV strain and play a role in the diagnostic classification and risk stratification of patients with heart failure (14–16). However, CMR is incompatible with metal objects such as pacemakers and infusion pumps, and patients with septic shock may have difficulties with transport and respiratory coordination.
RV-FWS, as measured by echocardiography, has been proposed to serve as a dependable indicator of RV function (17, 18); it is relatively easy to perform and has high temporal resolution. In addition, compared with traditional parameters, RV-FWS is less affected by imaging angles and demonstrates reduced reliance on LV contraction (19). However, the prognostic predictive value of RV-FWS remains inconclusive. A study conducted by Orde et al. (20) revealed that 72% of patients with sepsis demonstrated a reduction in RV-FWS, which was associated with an increased mortality rate. Conversely, Lanspa et al. (21) proposed that RV-FWS detected RVSD, but its prognostic value is limited in the context of sepsis. The debate remains ongoing. Our study aimed to explore the performance of RV function parameters (RV-FWS, RV-GS, TAPSE, and FAC) in predicting mortality outcomes in patients with sepsis and investigate the association between RVSD defined by RV-FWS and 30-day mortality.
2 Materials and methods
2.1 Study population
We prospectively enrolled adult patients with sepsis who had received treatment at the Yichang Central People's Hospital from May 2019 to April 2023. Sepsis is a life-threatening organ dysfunction caused by a dysregulated host response to infection. Septic shock is sepsis with persisting hypotension requiring vasopressors to maintain a mean arterial pressure of ≥65 mmHg and a serum lactate level of >2 mmol/L despite adequate volume resuscitation (22). The exclusion criteria of this study were as follows: patients with severe valvular disease or previous valve surgery; those with acute coronary syndrome (<1 month); those with persistent or permanent atrial fibrillation; those with other etiologies causing RV remodeling, such as RV cardiomyopathy and pulmonary arterial hypertension; and those with suboptimal ultrasound image quality or missing data. The hospital's institutional ethics committee approved this study (Approval No: PJ-KY2020-27). Patients or their legal guardians provided informed consent.
2.2 Echocardiography
Transthoracic echocardiography (TTE) (GE, Vivid E9, Horten Norway) measurements were completed within the first 24 h after admission. Conventional echocardiographic parameters were assessed according to the guidelines set by the American Society of Echocardiography (ASE) (23). Tricuspid annular plane systolic excursion (TAPSE) was measured at the junction of the lateral tricuspid leaflet and RV-free wall using the M-mode. The areas of RV systolic and diastolic were assessed in the RV-focused apical 4-chamber view. Fractional area change (FAC) was calculated using the following formula: [(end-diastolic area − end-systolic area)/end-diastolic area] × 100%. Inferior vena cava (IVC) diameter and its collapse with respiration were measured in the sagittal view. The ASE guidelines considered an IVC diameter of >2.1 cm and/or a collapse rate of <50% as positive findings. The right atrial pressure was estimated as 8 mmHg with one positive indicator, 15 mmHg with two positive indicators, and 3 mmHg with no positive indicators (23).
Dynamic images capturing a minimum of three consecutive cardiac cycles were saved (in DICOM format) in the RV-focused apical 4-chamber view. Strain analysis was conducted using the EchoPac workstation (GE, version 204, Horten Norway). A region of interest was established following the selection of the tricuspid annulus and apical plane, and the tracing curve was manually fine-tuned to achieve optimal alignment. The software algorithm automatically computed the RV-GS, RV-FWS, and strain values for each myocardial segment (Figure 1). To minimize information bias, data collection was performed by two experienced sonographers who were blinded to the clinical data.
2.3 Clinical data
In addition to vital demographic information, we collected data on patients' temperature; heart rate; respiratory rate; site of infection upon admission; and biochemical parameters, such as myocardial injury markers and lactate levels. We sought the expertise of the attending physician to assess the patient's renal injury and conduct a sequential organ failure assessment (SOFA). Furthermore, we documented the mean arterial pressure and mechanical ventilation utilization during TTE. Norepinephrine was the primary vasopressor medication. Our primary clinical outcome was 30-day mortality.
2.4 Statistical analysis
Continuous variables following a normal distribution are expressed as mean ± standard deviation, whereas those with a non-normal distribution are represented as median (interquartile range). Categorical variables are presented as percentages. Independent sample t-test, Mann–Whitney U-test, chi-square (χ2) test, or Fisher's exact test was used to analyze disparities between the groups. Receiver operating characteristic (ROC) curve analysis was performed for parameters related to RV function. Cutoff values were determined based on the maximum value of Youden's index, and the data were subsequently converted into binary variables. The constructed Kaplan–Meier survival curves and Log-Rank test were used to analyze the disparities among various groups. Univariate and multivariate Cox regression models were used to assess the association between variables and 30-day mortality. The same and different operators randomly analyzed 10 patients; the analyses were spaced at least 1 week apart to assess the intraoperator and interoperator reproducibility of strain measurements. Repeatability was quantified using the intraclass correlation coefficient (ICC). A P-value of <0.05 was considered statistically significant. The Statistical Package for the Social Sciences software version 26 (IBM Corp., Armonk, NY, USA) was used for all statistical analyses.
3 Results
3.1 General characteristics
In total, 127 patients were hospitalized due to sepsis conditions. Of these, 38 (30%) were excluded, predominantly due to substandard ultrasound image quality (n = 16) (Figure 2). Finally, 89 patients (median age: 63.0 ± 11.6 years) were enrolled in the study (Figure 2), including 59.6% males. Of the enrolled patients, 54 (61%) were diagnosed with septic shock and required vasopressor support, 27 (30%) patients died within 30 days of admission (Table 1). Mechanical ventilation was administered to 44 (49%) patients. Acute renal insufficiency was observed in 17 (19%) patients, and renal replacement therapy was initiated in 5 (6%) patients. One (1%) patient developed infective endocarditis. Furthermore, while 9 patients were transferred to other hospitals (Figure 2), subsequent surveys revealed a 30-day mortality rate of approximately 33% for these patients, which is consistent with our findings, mitigating selection bias to some extent.
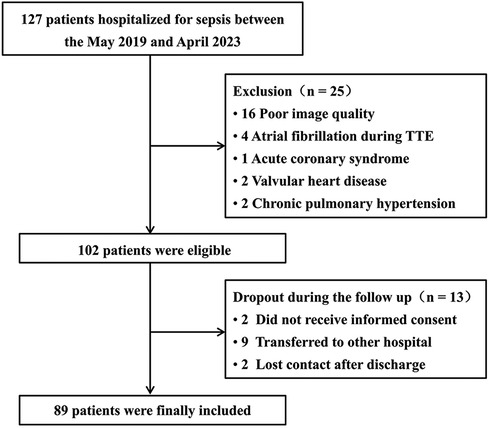
Figure 2. Flow diagram showing the initial selection of the cohort and exclusion of patients. TTE, transthoracic echocardiography.
Table 1 outlines patient characteristics based on survival outcomes. Nonsurvivors were significantly older and presented higher SOFA scores and increased markers of myocardial damage as well as a greater incidence of acute kidney injury (AKI) than survivors. Vasopressor medication administration (77.8% vs. 53.2%, P = 0.029) and mechanical ventilation (66.7% vs. 41.9%, P = 0.032) were more prevalent among nonsurvivors than survivors. Furthermore, nonsurvivors demonstrated lower absolute values of RV-FWS (−21.1% ± 2.1% vs. −19.7% ± 2.4%, P = 0.008) and RV-GS (−18.4% ± 1.4% vs. −17.7% ± 1.2%, P = 0.032) than survivors. TAPSE (1.79 ± 0.27 cm vs. 1.67 ± 0.25 cm, P = 0.052) and FAC (36.6% ± 2.9% vs. 35.4% ± 3.2%, P = 0.092) demonstrated no significant differences between the two groups (Table 1).
3.2 Prognostic value of different ultrasonographic parameters
The ROC analysis included RV-FWS, RV-GS, FAC, and TAPSE and revealed optimal cutoff values of −19.0%, −17.9%, 36.5%, and 1.55 cm, respectively. Of these factors, RV-FWS demonstrated the largest area under the curve (AUC: 0.68, P = 0.008) (Figure 3). The Kaplan–Meier survival analysis revealed that patients with impaired RV-FWS and RV-GS demonstrated a reduced survival rate compared with those with preserved RV-FWS and RV-GS. Neither FAC (P = 0.062) nor TAPSE (P = 0.056) significantly differentiated patients with different outcomes (Figure 4). However, in the multivariate analyses, neither of the two strain parameters, RV-FWS and RV-GS, was found to be associated with increased mortality (Table 2).
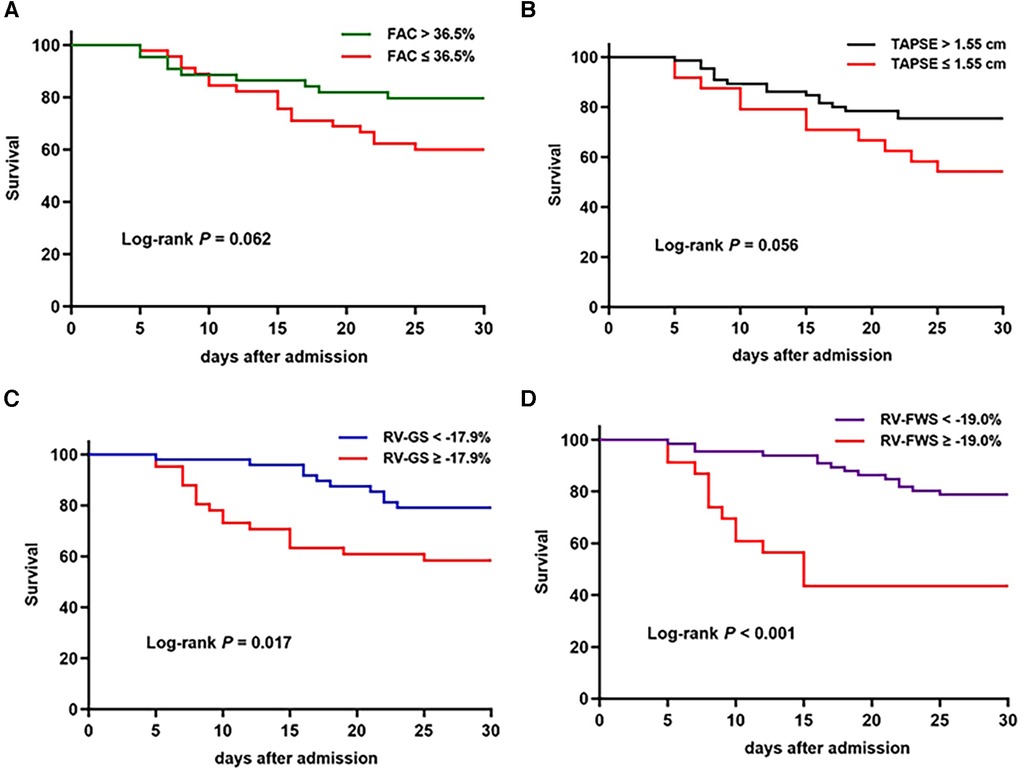
Figure 4. Kaplan–Meier survival curves based on FAC (A), TAPSE (B), RV-GS (C), and RV-FWS (D) cutoff values.
3.3 RVSD and mortality outcome
RVSD was defined by RV-FWS of ≥−19.0%. In the present study, 23 (25.8%) patients with RVSD had a higher mortality rate (56.5% vs. 21.2%, P = 0.002). Furthermore, individuals with RVSD demonstrated an increased prevalence of AKI (34.8% vs. 13.6%, P = 0.026) and mechanical ventilation (73.9% vs. 40.9%, P = 0.006), elevated lactate levels and SOFA scores, as well as a reduced left ventricular ejection fraction (LVEF) (Table 3). However, RVSD did not appear as an independent mortality predictor after adjusting for variables such as age, mechanical ventilation, AKI, myocardial damage markers, and SOFA score in the Cox regression analysis [hazard ratio (HR): 1.85, 95% confidence interval (CI): 0.56–6.14, P = 0.316] (Table 2). In addition, 13 (14.6%) patients demonstrated isolated RVSD (LVEF >50%), and this subgroup of patients demonstrated a significant association with the 30-day mortality outcome (HR: 3.46, 95% CI: 1.22–9.82, P = 0.020). The incidence rate of biventricular dysfunction was approximately 11% (n = 10). However, no significant relationship was observed between biventricular dysfunction and mortality outcome (Table 4).
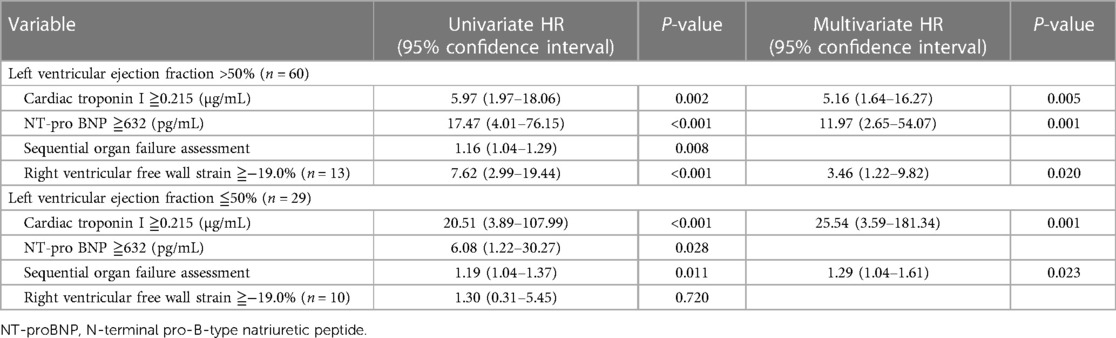
Table 4. Univariate and multivariate Cox analyses of variables associated with 30-day mortality according to LVEF subgroups.
RV-FWLS demonstrated high intraobserver and interobserver reproducibility, with ICC values of 0.89 (95% CI: 0.72–0.96) and 0.85 (95% CI: 0.61–0.94), respectively (Table 5).
4 Discussion
This observational study on sepsis revealed the following results: (1) RVSD defined by RV-FWS was present in 26% of the study patients, and their mortality rate was over double that of patients without RVSD; (2) nonsurvivors had significantly lower absolute values of RV-FWS than the survivors, and RV-FWS may provide added value in identifying RVSD; (3) in multivariate analyses, RV-FWS did not demonstrate an independent association with increased mortality; (4) isolated RVSD (with normal LVEF) may be correlated with prognosis. Further research with a larger sample size is warranted to validate these findings.
RVSD is frequently observed in patients with sepsis (20, 21, 24, 25). Vallabhajosyula et al. applied TAPSE and FAC as indices to quantify RVSD, revealing a 55% prevalence in patients with sepsis (26), a figure apparently exceeding the 26% incidence observed in our cohort (Table 3). This variation could be associated with different RVSD definitions and TTE evaluation timing. We defined RVSD using an RV-FWS threshold of −19%, which may have potentially biased the cohort toward individuals with pronounced RV impairment and possibly neglected subjects with incipient or progressive dysfunction. Recent research has revealed that RV-FWS may serve as a reliable indicator of RV systolic function (27, 28). Although RV-free wall motion occurs primarily in the transverse direction (29), strain analysis enables tracking of myocardial displacement from multiple orientations and is less affected by imaging angle limitations (30). Furthermore, the interventricular septum provides an anatomical basis for the interdependence of biventricular functional contraction (31). Compared with RV-GS, RV-FWS shows less dependence on LV motion (17, 32). These features highlight the advantage of RV-FWS in evaluating myocardial function.
Infection causes reversible or irreversible damage to the microcirculation and endothelial cells, resulting in the release of inflammatory mediators and myocardial depressant factors into the bloodstream. This can induce partial myocardial cell apoptosis and a negative inotropic effect to a certain extent (5, 33). Significantly elevated myocardial injury biomarkers in patients with sepsis support this hypothesis (Table 3). However, the pathophysiological mechanisms responsible for RVSD are complex, and cardiomyocyte injury alone does not entirely account for this phenomenon. The RV's long-term low-pressure ejection makes it less tolerant to acute increases in afterload (34, 35). We revealed an increased lactate level and relatively insufficient tissue perfusion in patients with RVSD (Table 3). Hypoxia and acidosis-induced pulmonary vasoconstriction may cause increased RV afterload (36, 37). Furthermore, while we did not observe a correlation between mechanical ventilation and increased mortality, the use of mechanical ventilation also leads to an increase in pulmonary vascular resistance to some extent (38, 39). In addition, individuals with RVSD demonstrated elevated right atrial pressure, emphasizing the effect of volume overload. Therefore, further research is required to determine whether RVSD in sepsis is a concomitant phenomenon of increased load.
The prognostic significance of RVSD remains unclear. Orde et al. (20) revealed that nonsurvivors demonstrated significantly impaired RV-FWS compared with survivors. RV-FWS of >−13% persisted as an independent predictor of 6-month mortality in patients with sepsis after adjusting for factors such as mechanical ventilation. Our study revealed that the mortality rate for patients with RV-FWS of ≥−19% increased by 2.7 times (P = 0.002) (Table 3), but impaired RV-FWS was not a determinant of mortality outcomes after adjusting for clinically pertinent covariates (Table 2). The use of an RV-focused apical 4-chamber view for strain analysis may have caused our higher absolute values of strain. Moreover, our research incorporated more covariate parameters, and we used a short follow-up period, which may have mitigated the effect of secondary diseases and treatment modalities on survival outcomes. Lanspa et al. (21) adopted RV-FWS of −20% as the threshold and revealed no correlation between impaired RV-FWS and 28-day mortality in patients with sepsis. Our study corroborates their findings and also demonstrates that the presence of isolated RVSD (with preserved LVEF) may increase the risk of mortality (Table 4). Isolated RVSD has been suggested as a maladaptive response to stress that may be associated with the development of secondary cardiogenic shock in sepsis (40, 41). We used LVEF to assess LV systolic function and found that the incidence of LV systolic dysfunction in patients with sepsis was approximately 32% (Table 4). However, in multivariate analysis, neither LV systolic dysfunction nor biventricular systolic dysfunction emerged as a risk factor for mortality outcomes (Tables 2, 4). The prognostic value of LVEF in sepsis is limited and it may not be a reliable indicator of intrinsic myocardial contractile function (42, 43), particularly in the context of interventions, such as aggressive fluid resuscitation or vasoactive medications. In addition, some studies have suggested that concurrent biventricular dysfunction is a tolerable adaptive response (44, 45).
Our study had several limitations. First, we did not include assessment data for the RV outflow tract, thereby the effect of RV-pulmonary artery decoupling was not investigated. Second, we could not evaluate the trend of changes in RV function as treatment progressed due to the lack of follow-up examinations during hospitalization. Third, although we used consistent diagnostic criteria, the Berkson bias remained. In addition, we did not eliminate the potential impact of confounding factors such as secondary lung infection, antibiotic use, and fluid balance on patient outcomes. Fourth, LV performance was assessed by LVEF rather than strain. Fifth, this single-center study included a relatively small sample size and we did not perform a power analysis. Furthermore, strain measurement necessitates high-quality images (17), and different analysis software demonstrated substantial heterogeneity (46).
5 Conclusion
In the present study, 26% of patients with sepsis had RVSD, which was defined as RV-FWS of ≥−19%. Nonsurvivors exhibited a higher incidence of RVSD than survivors. However, impaired RV-FWS was not identified as an independent risk factor for 30-day mortality, suggesting that RVSD may not affect the short-term prognosis of patients with sepsis. To validate this finding, research with larger sample sizes and multicentre designs is required in the future.
Data availability statement
The original contributions presented in the study are included in the article/Supplementary Material, further inquiries can be directed to the corresponding author.
Ethics statement
The studies involving humans were approved by Ethics Committee of the Yichang Central People's Hospital. The studies were conducted in accordance with the local legislation and institutional requirements. The participants provided their written informed consent to participate in this study. Written informed consent was obtained from the individual(s) for the publication of any potentially identifiable images or data included in this article.
Author contributions
HC: Writing – original draft, Writing – review & editing, Data curation, Investigation, Software. LH: Writing – original draft, Writing – review & editing. BX: Methodology, Project administration, Writing – original draft. YG: Data curation, Software, Writing – review & editing. JZ: Data curation, Investigation, Writing – original draft. BZ: Formal Analysis, Funding acquisition, Project administration, Resources, Writing – review & editing.
Funding
The author(s) declare financial support was received for the research, authorship, and/or publication of this article.
This work was supported by the Health Commission of Hubei Province Scientific Research Project (No. WJ2019H509) and the Yichang Medical and Health Talent Special Program.
Acknowledgments
The authors thank the Department of Ultrasound at The First College of Clinical Medical Science, China Three Gorges University for assistance with this work.
Conflict of interest
The authors declare that the research was conducted in the absence of any commercial or financial relationships that could be construed as a potential conflict of interest.
Publisher's note
All claims expressed in this article are solely those of the authors and do not necessarily represent those of their affiliated organizations, or those of the publisher, the editors and the reviewers. Any product that may be evaluated in this article, or claim that may be made by its manufacturer, is not guaranteed or endorsed by the publisher.
References
1. Lv X, Wang H. Pathophysiology of sepsis-induced myocardial dysfunction. Mil Med Res. (2016) 3:30. doi: 10.1186/s40779-016-0099-9
2. Sanfilippo F, Corredor C, Fletcher N, Tritapepe L, Lorini FL, Arcadipane A, et al. Left ventricular systolic function evaluated by strain echocardiography and relationship with mortality in patients with severe sepsis or septic shock: a systematic review and meta-analysis. Crit Care. (2018) 22(1):183. doi: 10.1186/s13054-018-2113-y
3. Martin L, Derwall M, Al Zoubi S, Zechendorf E, Reuter DA, Thiemermann C, et al. The septic heart: current understanding of molecular mechanisms and clinical implications. Chest. (2019) 155(2):427–37. doi: 10.1016/j.chest.2018.08.1037
4. Hoffman MJ, Greenfield LJ, Sugerman HJ, Tatum JL. Unsuspected right ventricular dysfunction in shock and sepsis. Ann Surg. (1983) 198(3):307–19. doi: 10.1097/00000658-198309000-00007
5. Dhainaut JF, Lanore JJ, de Gournay JM, Huyghebaert MF, Brunet F, Villemant D, et al. Right ventricular dysfunction in patients with septic shock. Intensive Care Med. (1988) 14(Suppl 2):488–91. doi: 10.1007/BF00256967
6. Arrigo M, Huber LC, Winnik S, Mikulicic F, Guidetti F, Frank M, et al. Right ventricular failure: pathophysiology, diagnosis and treatment. Card Fail Rev. (2019) 5(3):140–6. doi: 10.15420/cfr.2019.15.2
7. Pulido JN, Afessa B, Masaki M, Yuasa T, Gillespie S, Herasevich V, et al. Clinical spectrum, frequency, and significance of myocardial dysfunction in severe sepsis and septic shock. Mayo Clin Proc. (2012) 87(7):620–8. doi: 10.1016/j.mayocp.2012.01.018
8. Hamada-Harimura Y, Seo Y, Ishizu T, Nishi I, Machino-Ohtsuka T, Yamamoto M, et al. Incremental prognostic value of right ventricular strain in patients with acute decompensated heart failure. Circ Cardiovasc Imaging. (2018) 11(10):e007249. doi: 10.1161/CIRCIMAGING.117.007249
9. Haddad F, Hunt SA, Rosenthal DN, Murphy DJ. Right ventricular function in cardiovascular disease, part I: anatomy, physiology, aging, and functional assessment of the right ventricle. Circulation. (2008) 117(11):1436–48. doi: 10.1161/CIRCULATIONAHA.107.653576
10. Lahm T, Douglas IS, Archer SL, Bogaard HJ, Chesler NC, Haddad F, et al. Assessment of right ventricular function in the research setting: knowledge gaps and pathways forward. An official American thoracic society research statement. Am J Respir Crit Care Med. (2018) 198(4):e15–43. doi: 10.1164/rccm.201806-1160ST
11. Rudski LG, Lai WW, Afilalo J, Hua L, Handschumacher MD, Chandrasekaran K, et al. Guidelines for the echocardiographic assessment of the right heart in adults: a report from the American society of echocardiography endorsed by the European association of echocardiography, a registered branch of the European society of cardiology, and the Canadian society of echocardiography. J Am Soc Echocardiogr. (2010) 23(7):685–713. doi: 10.1016/j.echo.2010.05.010
12. Spencer KT, Flachskampf FA. Focused cardiac ultrasonography. JACC Cardiovasc Imaging. (2019) 12(7 Pt 1):1243–53. doi: 10.1016/j.jcmg.2018.12.036
13. Hundley WG, Bluemke DA, Finn JP, Flamm SD, Fogel MA, Friedrich MG, et al. ACCF/ACR/AHA/NASCI/SCMR 2010 expert consensus document on cardiovascular magnetic resonance: a report of the American college of cardiology foundation task force on expert consensus documents. Circulation. (2010) 121(22):2462–508. doi: 10.1161/CIR.0b013e3181d44a8f
14. Korosoglou G, Giusca S, Montenbruck M, Patel AR, Lapinskas T, Götze C, et al. Fast strain-encoded cardiac magnetic resonance for diagnostic classification and risk stratification of heart failure patients. JACC Cardiovasc Imaging. (2021) 14(6):1177–88. doi: 10.1016/j.jcmg.2020.10.024
15. Muehlberg F, Blaszczyk E, Will K, Wilczek S, Brederlau J, Schulz-Menger J. Characterization of critically ill patients with septic shock and sepsis-associated cardiomyopathy using cardiovascular MRI. ESC Heart Fail. (2022) 9(4):2147–56. doi: 10.1002/ehf2.13938
16. Kelle S, Bourantas CV, Korosoglou G. Editorial: insights in cardiovascular imaging: 2022. Front Cardiovasc Med. (2023) 10:1231842. doi: 10.3389/fcvm.2023.1231842
17. Badano LP, Kolias TJ, Muraru D, Abraham TP, Aurigemma G, Edvardsen T, et al. Standardization of left atrial, right ventricular, and right atrial deformation imaging using two-dimensional speckle tracking echocardiography: a consensus document of the EACVI/ASE/industry task force to standardize deformation imaging. Eur Heart J Cardiovasc Imaging. (2018) 19(6):591–600. doi: 10.1093/ehjci/jey042
18. de Braga Lima Carvalho Canesso M, Borges IN, de Deus Queiroz Santos TA, Ris TH, de Barros MVL, Nobre V, et al. Value of speckle-tracking echocardiography changes in monitoring myocardial dysfunction during treatment of sepsis: potential prognostic implications. Int J Cardiovasc Imaging. (2019) 35(5):855–9. doi: 10.1007/s10554-018-01525-1
19. Lang RM, Badano LP, Mor-Avi V, Afifilalo J, Armstrong A, Ernande L, et al. Recommendations for cardiac chamber quantification by echocardiography in adults: an update from the American society of echocardiography and the European association of, cardiovascular imaging. Eur Heart J Cardiovasc Imaging. (2016) 17(4):412. doi: 10.1093/ehjci/jew041
20. Orde SR, Pulido JN, Masaki M, Gillespie S, Spoon JN, Kane GC, et al. Outcome prediction in sepsis: speckle tracking echocardiography based assessment of myocardial function. Crit Care. (2014) 18(4):R149. doi: 10.1186/cc13987
21. Lanspa MJ, Cirulis MM, Wiley BM, Olsen TD, Wilson EL, Beesley SJ, et al. Right ventricular dysfunction in early sepsis and septic shock. Chest. (2021) 159(3):1055–63. doi: 10.1016/j.chest.2020.09.274
22. Singer M, Deutschman CS, Seymour CW, Shankar-Hari M, Annane D, Bauer M, et al. The third international consensus definitions for sepsis and septic shock (sepsis-3). JAMA. (2016) 315(8):801–10. doi: 10.1001/jama.2016.0287
23. Lang RM, Badano LP, Mor-Avi V, Afilalo J, Armstrong A, Ernande L, et al. Recommendations for cardiac chamber quantification by echocardiography in adults: an update from the American society of echocardiography and the European association of cardiovascular imaging. Eur Heart J Cardiovasc Imaging. (2015) 16(3):233–70. doi: 10.1093/ehjci/jev014
24. Innocenti F, Palmieri V, Stefanone VT, Donnini C, D'Argenzio F, Cigana M, et al. Epidemiology of right ventricular systolic dysfunction in patients with sepsis and septic shock in the emergency department. Intern Emerg Med. (2020) 15(7):1281–9. doi: 10.1007/s11739-020-02325-z
25. Zhang H, Huang W, Zhang Q, Chen X, Wang X, Liu D. Prevalence and prognostic value of various types of right ventricular dysfunction in mechanically ventilated septic patients. Ann Intensive Care. (2021) 11(1):108. doi: 10.1186/s13613-021-00902-9
26. Vallabhajosyula S, Kumar M, Pandompatam G, Sakhuja A, Kashyap R, Kashani K, et al. Prognostic impact of isolated right ventricular dysfunction in sepsis and septic shock: an 8-year historical cohort study. Ann Intensive Care. (2017) 7(1):94. doi: 10.1186/s13613-017-0319-9
27. Seo J, Jung IH, Park JH, Kim GS, Lee HY, Byun YS, et al. The prognostic value of 2D strain in assessment of the right ventricle in patients with dilated cardiomyopathy. Eur Heart J Cardiovasc Imaging. (2019) 20(9):1043–50. doi: 10.1093/ehjci/jez015
28. Nagai T, Horinouchi H, Yoshioka K, Ikari Y. Right ventricular free wall longitudinal strain assessment using offline speckle tracking in COVID-19 patients requiring intensive medical care. J Med Ultrason (2001). (2023) 50(3):417–25. doi: 10.1007/s10396-023-01305-y
29. Sanz J, Sánchez-Quintana D, Bossone E, Bogaard HJ, Naeije R. Anatomy, function, and dysfunction of the right ventricle: JACC state-of-the-art review. J Am Coll Cardiol. (2019) 73(12):1463–82. doi: 10.1016/j.jacc.2018.12.076
30. Hulshof HG, Eijsvogels TMH, Kleinnibbelink G, van Dijk AP, George KP, Oxborough DL, et al. Prognostic value of right ventricular longitudinal strain in patients with pulmonary hypertension: a systematic review and meta-analysis. Eur Heart J Cardiovasc Imaging. (2019) 20(4):475–84. doi: 10.1093/ehjci/jey120
31. Naeije R, Badagliacca R. The overloaded right heart and ventricular interdependence. Cardiovasc Res. (2017) 113(12):1474–85. doi: 10.1093/cvr/cvx160
32. Amsallem M, Mercier O, Kobayashi Y, Moneghetti K, Haddad F. Forgotten No more: a focused update on the right ventricle in cardiovascular disease. JACC Heart Fail. (2018) 6(11):891–903. doi: 10.1016/j.jchf.2018.05.022
33. Flierl MA, Rittirsch D, Huber-Lang MS, Sarma JV, Ward PA. Molecular events in the cardiomyopathy of sepsis. Mol Med. (2008) 14(5-6):327–36. doi: 10.2119/2007-00130.Flierl
34. Maughan WL, Shoukas AA, Sagawa K, Weisfeldt ML. Instantaneous pressure-volume relationship of the canine right ventricle. Circ Res. (1979) 44(3):309–15. doi: 10.1161/01.res.44.3.309
35. Hurford WE, Zapol WM. The right ventricle and critical illness: a review of anatomy, physiology, and clinical evaluation of its function. Intensive Care Med. (1988) 14(Suppl 2):448–57. doi: 10.1007/BF00256958
36. Brimioulle S, Maggiorini M, Stephanazzi J, Vermeulen F, Lejeune P, Naeije R. Effects of low flow on pulmonary vascular flow-pressure curves and pulmonary vascular impedance. Cardiovasc Res. (1999) 42(1):183–92. doi: 10.1016/s0008-6363(98)00301-0
37. Wilcox SR, Kabrhel C, Channick RN. Pulmonary hypertension and right ventricular failure in emergency medicine. Ann Emerg Med. (2015) 66(6):619–28. doi: 10.1016/j.annemergmed.2015.07.525
38. Peterson WP, Trempy GA, Nishiwaki K, Nyhan DP, Murray PA. Neurohumoral regulation of the pulmonary circulation during circulatory hypotension in conscious dogs. J Appl Physiol (1985). (1993) 75(4):1675–82. doi: 10.1152/jappl.1993.75.4.1675
39. Vieillard-Baron A, Matthay M, Teboul JL, Bein T, Schultz M, Magder S, et al. Experts’ opinion on management of hemodynamics in ARDS patients: focus on the effects of mechanical ventilation. Intensive Care Med. (2016) 42(5):739–49. doi: 10.1007/s00134-016-4326-3
40. Vieillard-Baron A. Septic cardiomyopathy. Ann Intensive Care. (2011) 1(1):6. doi: 10.1186/2110-5820-1-6
41. Piazza G, Goldhaber SZ. The acutely decompensated right ventricle: pathways for diagnosis and management. Chest. (2005) 128(3):1836–52. doi: 10.1378/chest.128.3.1836
42. Berrios RA S, O'Horo JC, Velagapudi V, Pulido JN. Correlation of left ventricular systolic dysfunction determined by low ejection fraction and 30-day mortality in patients with severe sepsis and septic shock: a systematic review and meta-analysis. J Crit Care. (2014) 29(4):495–9. doi: 10.1016/j.jcrc.2014.03.007
43. Kim JS, Kim YJ, Kim M, Ryoo SM, Kim WY. Association between right ventricle dysfunction and poor outcome in patients with septic shock. Heart. (2020) 106(21):1665–71. doi: 10.1136/heartjnl-2020-316889
44. Jardin F, Fourme T, Page B, Loubières Y, Vieillard-Baron A, Beauchet A, et al. Persistent preload defect in severe sepsis despite fluid loading: a longitudinal echocardiographic study in patients with septic shock. Chest. (1999) 116(5):1354–9. doi: 10.1378/chest.116.5.1354
45. Antonucci E, Fiaccadori E, Donadello K, Taccone FS, Franchi F, Scolletta S. Myocardial depression in sepsis: from pathogenesis to clinical manifestations and treatment. J Crit Care. (2014) 29(4):500–11. doi: 10.1016/j.jcrc.2014.03.028
Keywords: strain, right ventricular dysfunction, sepsis, echocardiography, prognosis
Citation: Chen H, Huang L, Xing B, Gao Y, Zhang J and Zhang B (2024) Prognostic value of right ventricular free wall strain in patients with sepsis. Front. Cardiovasc. Med. 11:1334759. doi: 10.3389/fcvm.2024.1334759
Received: 7 November 2023; Accepted: 5 February 2024;
Published: 21 February 2024.
Edited by:
Grigorios Korosoglou, GRN Klinik Weinheim, GermanyReviewed by:
Francesca Innocenti, Careggi Univeristy Hospital, ItalySuzan Hatipoglu, Royal Free Hospital, United Kingdom
© 2024 Chen, Huang, Xing, Gao, Zhang and Zhang. This is an open-access article distributed under the terms of the Creative Commons Attribution License (CC BY). The use, distribution or reproduction in other forums is permitted, provided the original author(s) and the copyright owner(s) are credited and that the original publication in this journal is cited, in accordance with accepted academic practice. No use, distribution or reproduction is permitted which does not comply with these terms.
*Correspondence: Bingyi Zhang emhhbmdjYXJsc21pdGhAMTI2LmNvbQ==
†These authors share first authorship