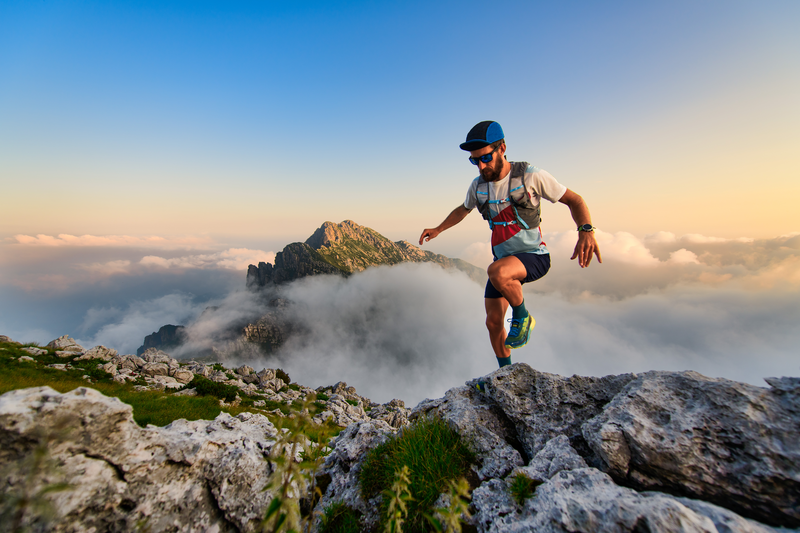
94% of researchers rate our articles as excellent or good
Learn more about the work of our research integrity team to safeguard the quality of each article we publish.
Find out more
ORIGINAL RESEARCH article
Front. Cardiovasc. Med. , 19 December 2023
Sec. Coronary Artery Disease
Volume 10 - 2023 | https://doi.org/10.3389/fcvm.2023.1292153
Objective: Red cell distribution width (RDW) and serum calcium (Ca) levels are predictors of in-hospital mortality in acute myocardial infarction (AMI) patients. However, their sensitivity and specificity are limited. Therefore, this study aimed to determine whether the RDW to Ca ratio (RCR) acquired on admission can be used to predict the in-hospital mortality of AMI patients.
Methods: This retrospective cohort study extracted clinical information from the Medical Information Market for Intensive IV (MIMIC-IV) database on 2,910 AMI patients enrolled via propensity score matching (PSM). Prognostic values were assessed using a multivariate logistic model and three PSM approaches. Analysis was performed based on stratified variables and interactions among sex, age, ethnicity, anemia, renal disease, percutaneous transluminal coronary intervention (PCI), coronary artery bypass grafting (CABG), atrial fibrillation, congestive heart failure, dementia, diabetes, paraplegia, hypertension, cerebrovascular disease, and Sequential Organ Failure Assessment (SOFA) score.
Results: A total of 4,105 ICU-admitted AMI patients were analyzed. The optimal cut-off value of the RCR for in-hospital mortality was 1.685. The PSM was performed to identify 1,455 pairs (2,910) of score-matched patients, with balanced differences exhibited for nearly all variables.The patients’ median age was 72 years (range, 63–82 years) and 60.9% were male. The risk of in-hospital mortality incidence increased with increasing RCR levels. After adjusting for confounders, the risk ratio for the incidence of in-hospital mortality for high RCR was 1.75 [95% confidence interval (CI): 1.60–1.94, P = 0.0113] compared to that associated with low RCR in the PSM cohort. High RCR was also substantially implicated in in-hospital mortality incidence in the weighted cohorts [odds ratio (OR) = 1.76, 95% CI: 1.62–1.94, P = 0.0129]. Assessment of RCR in three groups showed that patients with high RCR also had a higher risk of in-hospital mortality (OR = 3.04; 95% CI, 2.22–4.16; P < 0.0001) than in patients with RCR in the adjusted model. In the sensitivity analysis, both the original and weighted groups showed similar results.
Conclusion: The RCR at admission may be useful for predicting in-hospital mortality in ICU-admitted AMI patients.
Acute myocardial infarction (AMI) is the leading cause of death due to cardiovascular disease (1). An estimated 800,000 people in the United States alone develop AMI annually (2, 3). Despite significant advances in medical technology, particularly in the field of coronary revascularization, the mortality rate of AMI remains concerning (4). Severely ill AMI patients experience a greater occurrence of death and complications owing to the sudden worsening of symptoms and a limited window for providing life-saving interventions (5, 6). Early detection and effective management are essential for preventing life-threatening circumstances and improving patient prognosis. The development of non-invasive, inexpensive tests to identify patients at increased risk of in-hospital mortality is essential to further reduce in-hospital mortality.
Coronary atherosclerosis is a chronic disease which has stable and unstable periods. People during an unstable period may develop a MI if the vascular wall is stimulated by inflammation. Red cell distribution width (RDW) can reflect the inflammatory state of the body, suggesting that it can be used as a marker of inflammation (7). RDW measures the variability in circulating erythrocyte size and is associated with increased in-hospital mortality rates in AMI patients (7–10). Additionally, Cao et al. found that high serum calcium (Ca) levels are also associated with an increased risk of death from any cause and cardiac death after AMI (11). Shiyovich et al. also suggested that serum Ca level is an independent predictor of in-hospital mortality after AMI, showing a U-shaped relationship (12). Many prospective studies have found an association between serum calcium and in-hospital mortality in patients with AMI (12). Although RDW and Ca levels can serve as indicators of AMI severity, their sensitivity and specificity are relatively low (13, 14). The RDW/Ca ratio(RCR) is defined as the ratio of RDW to serum Ca. The RCR is a widely used and straightforward index in clinical settings as it solely necessitates venous blood. As a new biomarker, the RCR has emerged as an independent prognostic indicator of poor outcomes in acute pancreatitis (13). An elevated RCR can quickly, conveniently, economically, sensitively, and accurately predict severity and mortality in patients with acute pancreatitis (13, 14).
To date, no study has evaluated the association between RCR and in-hospital mortality among AMI patients. Thus, the present study aimed to examine the prognostic significance of RCR in a large population of ICU-admitted AMI patients and identified high-risk patients using a simple and convenient indicator.
Data were gathered from the Medical Information Mart for Intensive Care IV (MIMIC-IV) database, a retrospective cohort study that included critical care information of 76,540 patients who had been admitted to intensive care units and treated in hospitals at the Beth Israel Deaconess Medical Center (Boston, MA, USA) from 2008 to 2019 (15). One author (XD) had access to the data and was responsible for its extraction (certification number: 6182750).
The participants underwent at least one RDW and Ca measurement during the admission period. We observed the participants until they died or were discharged. We included adult patients aged ≥18 years with AMI based on International Classification of Diseases, 9th revision (ICD-9) codes 410.0 (410.00, 410.01, and 410.02), 410.1 (410.10, 410.11, and 410.12), 410.2 (410.20, 410.21, and 410.22), 410.3 (410.30, 410.31, and 410.32), 410.4 (410.40, 410.41, and 410.42), 410.5 (410.50, 410.51, and 410.52), 410.6 (410.60, 410.61, and 410.62), 410.7 (410.70, 410.71, and 410.72), 410.8 (410.80, 410.81, and 410.82), and 410.9 (410.90, 410.91, and 410.92), and ICD-10 codes: I21.0 (I21.01, I21.02, and I21.09), I21.1 (I21.11 and I21.19), I21.2 (I21.21 and I21.29), I21.3, and I21.4. We identified 5,085 ICU-admitted AMI patients. 1,458 patients came from coronary care unit(CCU). The exclusion criteria included patients (1) admitted to the hospital without an RDW measurement (n = 16), (2) who did not undergo Ca measurement during their hospital stay (n = 407), (3) with malignant tumors (n = 446), (4) with AIDS (n = 12), and (5) with severe liver diseases (n = 99). This study included 4,105 AMI patients (Figure 1). As a baseline, data from the first 24 h after ICU admission were collected. Troponin T were peak levels.
Figure 1. Participant flowchart. The analysis included a total of 4,105 patients with acute myocardial infarction (AMI).
AMI was defined as an increase or decrease in troponin T values with at least one value exceeding the 99th percentile upper reference limit and at least one of the following symptoms of myocardial ischemia: development of pathological Q-wave imaging evidence of new loss of viable myocardium, new ischemic electrocardiographic changes, or an abnormality of regional wall motion consistent with an ischemic cause (16).
In-hospital death was defined as death due to any cause during admission.To make the data more reliable, serum calcium (mg/ml) was corrected to albumin (g/dl) using the Payne formula: corrected serum calcium (corrected sCa) = Serum calcium + 0.8 × (4.0—albumin) (12).
Variables with continuous values were reported as averages ± standard deviations (SD) or medians (interquartile range). Based on the normality of the data distribution, Student's t- or Mann–Whitney U-tests were used for comparisons. Categorical variables were analyzed using the chi-square test and reported as case numbers (%).
By calculating the maximum Youden index for survival status, the optimal cutoff value of RCR was determined using receiver operating curve (ROC) curve analysis, in which the Youden index was equal to the sum of sensitivity and specificity minus 1. The best cutoff value of RCR was 1.685, with 88.1% sensitivity, 37.1% specificity, 28.7% positive predictive value, 88.2% negative predictive value, and 0.252 Youden index. The patients were divided into two groups according to the RCR cutoff value.
Propensity score matching (PSM) was employed because it was difficult to achieve complete stochasticity during patient screening. PSM balances the selection bias and underlying confounding factors. PSM analysis was conducted in the present study using a logistic regression model that included patient age and sex, the presence of congestive heart failure, mild liver disease, renal disease, peripheral vascular disease, cerebrovascular disease, dementia, chronic pulmonary disease, rheumatic disease, peptic ulcer disease, and diabetes, mean corpuscular volume (MCV), mean corpuscular-hemoglobin concentration (MCHC), Sequential Organ Failure Assessment (SOFA) score, thiazide use, and parathyroid diseases. In order to assess PSM levels, we used standardized mean differences (SMDs). For a given covariate, an SMD <10.0% represents a relatively small imbalance (17, 18). We considered a threshold of 0.01 to be an acceptable PSM degree. Matching was conducted with a caliper of 0.01 for patients grouped into low RCR (<1.685) and high RCR (≥1.685) pairs. A total of 2,910 propensity score-matched patients (1,455 pairs) with matching propensity scores were identified. Univariate and multivariate logistic regression analyses were performed to evaluate the prognostic relevance of the RCR. After considering clinical significance, the final adjusted model retained the covariates that caused significant changes in the effect estimate of >10% (P < 0.05) (19). The propensity score (PS) was estimated to perform sensitivity analyses to calculate the inverse probability of treatment weight (IPTW). In this example, 1/PS is the weight of high RCR, whereas 1/(1–PS) corresponds to the weight of low RCR. The IPTW model was then used to create a weighted cohort. Sensitivity analysis was conducted using two relationship inference models in the original and weighted cohorts, respectively (20, 21). Next, we analyzed the modifications and interactions of the subgroups based on the likelihood ratio test.
The statistical analyses were performed using IBM SPSS Statistics for Windows, version 26.0 (IBM Corp., Armonk, NY, USA), R (http://www.R-project.org, The R Foundation), and Empower Stats software (www.empowerstats.com, X&Y Solutions, Inc., Boston, MA, USA).
As shown Table 1, the average age of the study population in the original cohort was 71.4 ± 13.3 years. Our preliminary analysis of the confounding variables identified significant differences between the high and low RCR groups before PSM. Patients with high RCR had a lower male ratio, proportion of white ethnicity, systolic blood pressure (SBP), diastolic blood pressure (DBP), Ca, RBC, glucose, and length of hospital stay, had a higher age, male ratio, proportion of white, heart rate, respiratory rate, SOFA, RCR, WBC, creatinine, creatine kinase-myocardial band (CK-MB), and troponin T, and were more likely to have a high prevalence of congestive heart failure, dementia, chronic pulmonary disease, chronic pulmonary disease, mild liver disease, hypertension,cardiogenic shock, and in-hospital mortality (P < 0.05 for all).
The PSM-matched population showed differences in the proportions of white ethnicity, heart rate, SBP, DBP, temperature, respiratory rate, RCR, RBC, creatinine level, glucose level, aspartate transaminase (AST) level, percutaneous transluminal coronary angioplasty (PTCA), prevalence of mild liver disease, hyperlipidemia, in-hospital mortality, and length of hospital stay (all P < 0.05).
Table 2 shows the estimated odds ratios (ORs) and 95% confidence interval (CIs) for RCR in relation to in-hospital mortality after PSM. As a categorical variable, the prevalence of in-hospital mortality increased remarkably among patients in the high RCR group compared to that observed in the low RCR group (crude model: OR = 1.74, 95% CI: 1.61–1.88, P = 0.0010; Model I: OR = 1.74, 95% CI: 1.61–1.89, P = 0.0013; Model II: OR = 1.75, 95% CI: 1.60–1.94, P = 0.0113). As a successive variable, after adjusting for the clinical confounders listed, an SD increase in serum uric acid (SUA) level was related to a 158% increased risk of in-hospital mortality (OR = 2.58; 95% CI:1.92–3.47; P < 0.0001) in the fully adjusted model. When RCR was assessed in the three models, patients with high RCR also had a higher risk of in-hospital mortality (OR = 3.04; 95% CI, 2.22–4.16; P < 0.0001) than that observed in patients in the low RCR group in the adjusted model.
Table 2. Adjusted odds ratios for the incidence of in-hospital mortality according to the presence of high RCR in the PS-matched cohort.
According to these findings, a sensitivity analysis was applied to further confirm the association between RCR and the incidence of in-hospital mortality in the two cohorts examined, and a weighted cohort was developed with the estimated PS through the development of an IPTW model (Table 3). We also used non-adjusted, partially adjusted, and fully adjusted models across the two cohorts. In the original cohort, a high risk of in-hospital mortality was noted in cases with a high RCR (crude model: OR = 1.73, 95% CI:1.63–1.86, P < 0.001; Model I: OR = 1.75, 95% CI:1.64–1.88, P < 0.001; Model II: OR = 1.74, 95% CI: 0.54–2.02, P = 0.0635) after adjusting for different covariates. The results remained marked in the weighted cohort (crude model: OR = 1.78, 95% CI:1.70–1.87, P < 0.001; Model I: OR = 1.78, 95% CI: 1.72–1.88, P < 0.001; Model II: OR = 1.76, 95% CI: 1.62–1.94, P = 0.0129). The results were also significant when SUA was used as a successive variable. When RCR was assessed in the three models, patients with high RCR also had a higher risk of in-hospital mortality than that observed in patients with low RCR in both original and weighted cohorts.
Table 3. Association of RCR and in-hospital mortality among AMI in the original (A) and weighted cohorts (B).
Using Van der Weele and Ding's E-value methodology, we conducted a sensitivity analysis to identify possible confounding effects (22). In the current study, the adjusted OR for the associations between RCR and in-hospital mortality was 1.75 (95% CI 1.60–1.94). An unmeasured confounder could fully account for the association of intensification with in-hospital mortality if it was associated with the exposure and outcome with an OR of 1.98 (lower confidence limit, 1.59) (Figure 2). Considering this E-value, fewer confounding factors seemed to affect the current association between RCR and in-hospital mortality.
Figure 2. E-value analyses. An unmeasured confounder could fully account for the association of intensification with red cell distribution width to serum calcium ratio (RCR) if it was associated with the exposure and outcome with an odds ratio (OR) of 1.98 (lower confidence limit, 1.59).
We performed stratified analysis to evaluate the robustness of our findings in diverse subgroups following PSM. After adjusting for potential confounders, we found no interactions between sex, age, ethnicity, anemia, renal disease, PCI, CABG, atrial fibrillation, congestive heart failure, diabetes, hypertension, cerebrovascular disease, hyperparathyroidism, SOFA score, cardiogenic shock, and AMI categories (Table 4 and Figure 3). The high RCR (≥1.685) group had a higher in-hospital mortality rate than that in the low RCR (<1.685) group for all subgroups. However, whether RCR interacted with any of the subgroup factors (P > 0.05) was not evident when we analyzed the interaction between RCR and each factor.
Figure 3. Forest plot of the association between red cell distribution width to serum calcium ratio (RCR) and in-hospital mortality of patients with acute myocardial infarction (AMI).
Finally, we examined the performance of RCR as a predictor of in-hospital mortality. ROC curves were constructed using the RCR in the original and matched populations (Supplementary Figures S1 and S2, respectively). The results showed AUC values of 0.65 (95% CI: 0.63–0.67) and 0.66 (95% CI: 0.63–0.68), respectively.
Furthermore,the predictive values for in-hospital mortality of troponin T and RCR were compared in patients with AMI. ROC curves generated for RCR and troponin T are plotted in Supplementary Figure S3. The AUC for RCR was 0.66 (range, 0.63-0.67), whereas it was 0.51 (range, 0.48-0.53) for troponin T (P < 0.001).
Finally, ROC curves were also used to evaluate the prognostic value of RCR, RDW, and Ca in patients with AMI. The AUCs of RCR, RDW, and Ca for predicting the in-hospital mortality were 0.66, 0.65, and 0.53, respectively (Supplementary Figure S4).
To our knowledge, this is the first study to investigate the prognostic value of RCR on admission in a large population of ICU-admitted AMI patients. Our findings demonstrated that AMI patients with a high RCR were at an increased risk of in-hospital mortality. The multivariate models showed adverse outcomes associated with RCR after adjusting for confounding factors. Thus, RCR may be useful to evaluate the prognosis of AMI patients. Additionally, our analysis of the PSM cohorts revealed an association between RCR and in-hospital mortality, in which the risk of in-hospital mortality increased by 75% after adjusting for PS, and the association persisted even after adjusting for all other confounders. Similarly, the original and weighted cohorts showed similar results after sensitivity analysis. The results obtained by stratifying by the chosen variables in addition to adjusting for PS were also consistent. As a further measure of the association of RCR with in-hospital mortality, we analyzed RCR associations in different subgroups of traditional cardiovascular risk factors.
The RCR is a straightforward index widely used in clinical settings because it only requires venous blood. Thus, RDW and Ca are regularly assessed in AMI patients admitted to the intensive care unit (ICU). RCR was a blend of two parameters that had been demonstrated to possess a superior capability in forecasting the disease's prognosis. The RDW, along with other standard complete blood count parameters, is used to identify diseases of the hematological system (23). Various diseases can be prognosticated by RDW, including cardiovascular and cerebrovascular disorders, respiratory diseases, cancer, and critical illness (24–26). The RDW reflects the inflammation level. Inflammatory reactions play a significant role in AMI occurrence and progression, which may lead to an elevation in RDW (8, 27, 28).We believe that the RDW can be used to predict the prognosis of AMI patients because it reflects the level of inflammation. Similarly, serum Ca level is an independent predictor of in-hospital death in patients with ST elevation myocardial infarction (STEMI) (29). Fang et al. reported that both decreased and increased corrected serum Ca levels in AMI patients are associated with increased in-hospital mortality; moreover, patients with corrected serum Ca levels of 2.35 mmol/L showed the lowest risk of in-hospital death (30). As predictors of in-hospital mortality from AMI, most previous studies included either RDW or Ca; however, we also considered the ratio of RDW to Ca. RCR is a blend of two parameters that have been demonstrated to possess superior capability in predicting disease prognosis. However, few studies have investigated the prognostic value of RCR in acute pancreatitis (13, 14, 31, 32). Han et al. suggested that the RCR may play a valuable role in predicting acute pancreatitis severity (31). Additionally, Gravito-Soares et al. conducted a study of 666 acute pancreatitis patients and determined that RCR may be a new method to identify acute pancreatitis patients requiring transferred to the ICU, with accompanying complications requiring surgical treatment, or at risk for early-stage in-hospital death (32). These outcomes suggest that a high RCR is associated with more severe circumstances than those associated with a low RCR. Our study results suggest that RCR can be used for the early identification of ICU-admitted AMI patients with poor prognoses. These findings are consistent with those reported previously. It is suggested that more attention should be given to the effect of RCR levels on the early prognosis of AMI patients.
Our findings revealed higher in-hospital mortality in AMI patients with high RDW and low serum Ca level. However, the mechanism behind the close correlation between RCR and in-hospital mortality in AMI patients is currently unknown. High RDW is associated with a poor prognosis in AMI, but the mechanisms underlying this association are also unknown. This process is influenced by factors that impair the hematopoietic function of the bone marrow. Most of these risk factors are shared with those associated with worsening coronary artery disease prognoses. Several hypotheses have been proposed, including the potential roles of anemia (33), inflammatory cytokines (34), free cholesterol (35), oxidative stress (36), thrombosis (37), nutritional deficiency (8), microvascular disorders (38), and neurohumoral and adrenergic systems (39). AMI patients with low serum Ca levels have higher in-hospital mortality for several reasons. First, these factors are directly related to the electrophysiological properties of cardiomyocyte membranes in which Ca levels are determined. When Ca levels are low, Ca channels may not close as quickly as they should, leading to prolonged plateaus (40). Furthermore, patients with low serum Ca levels are more likely to experience cardiac arrhythmias and arrests (41). Second, there is a possibility of reversing heart failure and cardiomyopathy when Ca levels are low (42). Third, low Ca levels are associated with multiple cardiovascular risk factors including hypertension and decreased left ventricular systolic function (43). Moreover, evidence suggests that Ca administered to STEMI patients with hypocalcemia may improve their short-term outcomes after AMI; however, further research is needed (29).
Few studies have explored the clinical value of RCR in AMI patients. Identifying the precise mechanisms underlying the strong association between RCR and inpatient death in AMI patients is challenging. We demonstrated that RDW/Ca (AUC = 0.66) had better predictive power in predicting in-hospital mortality of AMI than the single factors: RDW(AUC = 0.65, P < 0.05) and Ca (AUC = 0.53, P < 0.05). We also clarified that RDW/Ca was a risk factor for clinical deterioration in AMI. However, the AUC of RCR was subtle greater than RDW. For a more robust conclusion and to define a precise cut-off value of RCR, we recommend further multicenter prospective trials with a larger sample size.
Our study had several strengths. To our knowledge, this was the first study to examine the relationship between RCR and mortality in a large cohort of ICU-admitted AMI patients. The significant relationship between RCR and in-hospital death remained after a multiple logistic regression analysis adjusted for several confounding factors, indicating a good level of stability. This study used rigorous statistical adjustments to mitigate the residual confounding factors to which observational studies are prone.
However, our study also had several limitations. First, causal inferences were not possible because of the study design. Although PSM is among the most effective methods for mitigating inherent biases in non-randomized studies, caution is needed when interpreting our findings as residual biases might have remained. Second, the analysis might have introduced measurement errors due to the influence of specific factors on RCR and the exclusion of various RCR measurements. Third, data on the clinical outcome of cardiac death, which is more consequential, was not obtained. Finally, not all patients were from CCU, which is potential limitation affecting generalizability. The data were from the United States, and thus the results may not apply fully to ICUs elsewhere with different practices or resources. As a single-center study, caution should be exercised in interpreting findings from other populations and regions. Therefore, large prospective multicenter studies and follow-ups are needed to confirm these results in the future.
We demonstrated a significant positive association between RCR and in-hospital mortality in ICU-admitted AMI patients. Further research is necessary to determine whether the RCR predicts in-hospital mortality in AMI patients.
Publicly available datasets were analyzed in this study. This data can be found here: https://mimic.physionet.org.
The study was approved by the Ethic Committee of the First People's Hospital of Changde City (No. 2023-203-01). The studies were conducted in accordance with the local legislation and institutional requirements. Written informed consent for participation was not required from the participants or the participants' legal guardians/next of kin in accordance with the national legislation and institutional requirements.
SH: Data curation, Writing – original draft, Writing – review & editing. HZ: Data curation, Visualization, Writing – review & editing. ZZ: Conceptualization, Data curation, Writing – review & editing. NG: Software, Writing – review & editing. QZ: Data curation, Writing – original draft. XD: Data curation, Writing – review & editing. LG: Conceptualization, Visualization, Writing – original draft.
The author(s) declare financial support was received for the research, authorship, and/or publication of this article.
This project was supported by the Hunan Science and Technology Innovation Project (2021SK4042), the Hunan Provincial Natural Science Foundation of China (2022JJ30086), the Scientific Research Project of Hunan Provincial Health Commission (B202303018255), and the First People’s Hospital of Changde Wings Spreading Program Scientific Research Fund (2022ZZ07 and 2022ZZ04).
The authors declare that the research was conducted in the absence of any commercial or financial relationships that could be construed as a potential conflict of interest.
All claims expressed in this article are solely those of the authors and do not necessarily represent those of their affiliated organizations, or those of the publisher, the editors and the reviewers. Any product that may be evaluated in this article, or claim that may be made by its manufacturer, is not guaranteed or endorsed by the publisher.
The Supplementary Material for this article can be found online at: https://www.frontiersin.org/articles/10.3389/fcvm.2023.1292153/full#supplementary-material
SUPPLEMENTARY FIGURE S1
ROC curve for the prediction of in-hospital mortality in the original population.
SUPPLEMENTARY FIGURE S2
ROC curve for the prediction of in-hospital mortality in the matched population.
SUPPLEMENTARY FIGURE S3
ROC curves of RCR and troponin T.
SUPPLEMENTARY FIGURE S4
ROC curves of RCR, RDW, and Ca.
1. Barrère-Lemaire S, Vincent A, Jorgensen C, Piot C, Nargeot J, Djouad F. Mesenchymal stromal cells for improvement of cardiac function following acute myocardial infarction: a matter of timing. Physiol Rev. (2023). doi: 10.1152/physrev.00009.2023
2. Fryar CD, Chen TC, Li X. Prevalence of uncontrolled risk factors for cardiovascular disease: United States, 1999–2010. NCHS Data Brief. (2012) 103:1–8.
3. Guan Y, Niu H, Wen J, Dang Y, Zayed M, Guan J. Rescuing cardiac cells and improving cardiac function by targeted delivery of oxygen-releasing nanoparticles after or even before acute myocardial infarction. ACS Nano. (2022) 16:19551–66. doi: 10.1021/acsnano.2c10043
4. Wang TKM, Grey C, Jiang Y, Selak V, Bullen C, Jackson RT, et al. Trends in cardiovascular outcomes after acute coronary syndrome in New Zealand 2006–2016. Heart. (2020) 8:1–7. doi: 10.1136/heartjnl-2020-316891
5. Valley TS, Iwashyna TJ, Cooke CR, Sinha SS, Ryan AM, Yeh RW, et al. Intensive care use and mortality among patients with ST elevation myocardial infarction: retrospective cohort study. Br Med J. (2019) 365:l1927. doi: 10.1136/bmj.l1927
6. Lu T, Tan L, Xu K, Liu J, Liu C, Zhang G, et al. Outcomes of hyperlactatemia on admission in critically ill patients with acute myocardial infarction: a retrospective study from MIMIC-IV. Front Endocrinol (Lausanne). (2022) 13:1015298. doi: 10.3389/fendo.2022.1015298
7. Lippi G, Mattiuzzi C, Cervellin G. Learning more and spending less with neglected laboratory parameters: the paradigmatic case of red blood cell distribution width. Acta Biomed. (2016) 87:323–8.28112703
8. Huang S, Zhou Q, Guo N, Zhang Z, Luo L, Luo Y, et al. Association between red blood cell distribution width and in-hospital mortality in acute myocardial infarction. Med (Baltim). (2021) 100:e25404. doi: 10.1097/MD.0000000000025404
9. Xu N, Peng C. Association between red cell distribution width-to-platelet ratio and short-term and long-term mortality risk in patients with acute ischemic stroke. BMC Neurol. (2023) 23:191. doi: 10.1186/s12883-023-03219-1
10. Yao H, Lian L, Zheng R, Chen C. Red blood cell distribution width/platelet ratio on admission as a predictor for in-hospital mortality in patients with acute myocardial infarction: a retrospective analysis from MIMIC-IV database. BMC Anesthesiol. (2023) 23:113. doi: 10.1186/s12871-023-02071-7
11. Cao W, Li Y, Wen Y, Fang S, Zhao B, Zhang X, et al. Higher serum phosphorus and calcium levels provide prognostic value in patients with acute myocardial infarction. Front Cardiovasc Med. (2022) 9:929634. doi: 10.3389/fcvm.2022.929634
12. Shiyovich A, Plakht Y, Gilutz H. Serum calcium levels independently predict in-hospital mortality in patients with acute myocardial infarction. Nutr Metab Cardiovasc Dis. (2018) 28:510–6. doi: 10.1016/j.numecd.2018.01.013
13. Gupta V, Narang SS, Gill CS, Selhi PK, Gupta M. Red cell distribution width and ratio of red cell distribution width-to-total serum calcium as predictors of outcome of acute pancreatitis. Int J Appl Basic Med Res. (2023) 13:5–9. doi: 10.4103/ijabmr.ijabmr_286_22
14. Han T, Cheng T, Liao Y, He Y, Liu B, Lai Q, et al. The ratio of red blood cell distribution width to serum calcium predicts severity of patients with acute pancreatitis. Am J Emerg Med. (2022) 53:190–5. doi: 10.1016/j.ajem.2022.01.024
15. Johnson AEW, Bulgarelli L, Shen L, Gayles A, Shammout A, Horng S, et al. MIMIC-IV, a freely accessible electronic health record dataset. Sci Data. (2023) 10:1. doi: 10.1038/s41597-022-01899-x
16. Byrne RA, Rossello X, Coughlan JJ, Barbato E, Berry C, Chieffo A, et al. 2023 ESC guidelines for the management of acute coronary syndromes. Eur Heart J. (2023) 44:3720–826. doi: 10.1093/eurheartj/ehad191
17. Han Y, Hu H, Liu Y, Li Q, Huang Z, Wang Z, et al. The association between congestive heart failure and one-year mortality after surgery in Singaporean adults: a secondary retrospective cohort study using propensity-score matching, propensity adjustment, and propensity-based weighting. Front Cardiovasc Med. (2022) 9:858068. doi: 10.3389/fcvm.2022.858068
18. Normand ST, Landrum MB, Guadagnoli E, Ayanian JZ, Ryan TJ, Cleary PD, et al. Validating recommendations for coronary angiography following acute myocardial infarction in the elderly: a matched analysis using propensity scores. J Clin Epidemiol. (2001) 54:387–98. doi: 10.1016/S0895-4356(00)00321-8
19. Kernan WN, Viscoli CM, Brass LM, Broderick JP, Brott T, Feldmann E, et al. Phenylpropanolamine and the risk of hemorrhagic stroke. N Engl J Med. (2000) 343:1826–32. doi: 10.1056/NEJM200012213432501
20. Koch B, Vock DM, Wolfson J. Covariate selection with group lasso and doubly estimation of causal effects. Biometrics. (2018) 74:8–17. doi: 10.1111/biom.12736
21. Geleris J, Sun Y, Platt J, Zucker J, Baldwin M, Hripcsak G, et al. Observational study of hydroxychloroquine in hospitalized patients with COVID-19. N Engl J Med. (2020) 382:2411–8. doi: 10.1056/NEJMoa2012410
22. VanderWeele TJ, Ding P. Sensitivity analysis in observational research: introducing the E-value. Ann Intern Med. (2017) 167:268–74. doi: 10.7326/M16-2607
23. Parizadeh SM, Jafarzadeh-Esfehani R, Bahreyni A, Ghandehari M, Shafiee M, Rahmani F, et al. The diagnostic and prognostic value of red cell distribution width in cardiovascular disease; current status and prospective. BioFactors. (2019) 45:507–16. doi: 10.1002/biof.1518
24. Wang L, Wang C, Wu S, Li Y, Guo W, Liu M. Red blood cell distribution width is associated with mortality after acute ischemic stroke: a cohort study and systematic review. Ann Transl Med. (2020) 8:81. doi: 10.21037/atm.2019.12.142
25. Yu XS, Chen ZQ, Hu YF, Chen JX, Xu WW, Shu J, et al. Red blood cell distribution width is associated with mortality risk in patients with acute respiratory distress syndrome based on the Berlin definition:a propensity score matched cohort study. Heart Lung. (2020) 49:641–5. doi: 10.1016/j.hrtlng.2020.04.008
26. Ma W, Mao S, Bao M, Wu Y, Guo Y, Liu J, et al. Prognostic significance of red cell distribution width in bladder cancer. Transl Androl Urol. (2020) 9:295–302. doi: 10.21037/tau.2020.03.08
27. Fang L, Moore XL, Dart AM, Wang LM. Systemic inflammatory response following acute myocardial infarction. J Geriatr Cardiol. (2015) 12:305–12. doi: 10.11909/j.issn.1671-5411.2015.03.020
28. Tong L, Liu YQ, Shen JH, BO M, Zhou Q, Duan XJ, et al. Relationship between the red cell distribution width-to-platelet ratio and in-hospital mortality among critically ill patients with acute myocardial infarction: a retrospective analysis of the MIMIC-IV database. BMJ (Open). (2022) 12:e062384. doi: 10.1136/bmjopen-2022-062384
29. Lu X, Wang Y, Meng H, Chen P, Huang Y, Wang Z, et al. Association of admission serum calcium levels and in-hospital mortality in patients with acute ST-elevated myocardial infarction: an eight-year, single-center study in China. PLoS One. (2014) 9:e99895. doi: 10.1371/journal.pone.0099895
30. Fang D, Chen H. Association between serum calcium level and in-hospital mortality in patients with acute myocardial infarction: a retrospective cohort study. Sci Rep. (2022) 12:19954. doi: 10.1038/s41598-022-24566-y
31. Gravito-Soares M, Gravito-Soares E, Gomes D, Almeida N, Tomé L. Red cell distribution width and red cell distribution width to total serum calcium ratio as major predictors of severity and mortality in acute pancreatitis. BMC Gastroenterol. (2018) 18:108. doi: 10.1186/s12876-018-0834-7
32. Han TY, Cheng T, Liu BF, He YR, Pan P, Yang WT, et al. Evaluation of the prognostic value of red cell distribution width to total serum calcium ratio in patients with acute pancreatitis. Gastroenterol Res Pract. (2021) 2021:6699421. doi: 10.1155/2021/6699421
33. Muñoz-Bravo C, Gutiérrez-Bedmar M, Gómez-Aracena J, García-Rodríguez A, Navajas JF. Iron:protector or risk factor for cardiovascular disease? Still controversial. Nutrients. (2013) 5:2384–404. doi: 10.3390/nu5072384
34. Tomaiuolo G, Lanotte L, D’Apolito R, Cassinese A, Guido S. Microconfined flow behavior of red blood cells. Med Eng Phys. (2016) 38:11–6. doi: 10.1016/j.medengphy.2015.05.007
35. Tziakas D, Chalikias G, Grapsa A, Gioka T, Tentes I, Konstantinides S. Red blood cell distribution width: a strong prognostic marker in cardiovascular disease: is associated with cholesterol content of erythrocyte membrane. Clin Hemorheol Microcirc. (2012) 51:243–54. doi: 10.3233/CH-2012-1530
36. Eryd S A, Smith JG, Melander O, Hedblad B, Engström G. Inflammation-sensitive proteins and risk of atrial fibrillation: a population-based cohort study. Eur J Epidemiol. (2011) 26:449–55. doi: 10.1007/s10654-011-9565-6
37. Zöller B, Melander O, Svensson P, Engström G. Red cell distribution width and risk for venous thromboembolism: a population-based cohort study. Thromb Res. (2014) 133:334–9. doi: 10.1016/j.thromres.2013.12.013
38. Buchanan DM, Arnold SV, Gosch KL, Jones PG, Longmore LS, Spertus JA, et al. Association of smoking status with angina and health-related quality of life after acute myocardial infarction. Circ Cardio Vasc Qual Outcomes. (2015) 8:493–500. doi: 10.1161/CIRCOUTCOMES.114.001545
39. Okonko DO, Marley SB, Anker SD, Poole-Wilson PA, Gordon MY. Suppression of erythropoiesis in patients with chronic heart failure and anemia of unknown origin:evidence of an immune basis. Int J Cardiol. (2013) 166:664–71. doi: 10.1016/j.ijcard.2011.11.081
40. Yan SD, Liu XJ, Peng Y, Xia TL, Liu W, Tsauo JY, et al. Admission serum calcium levels improve the GRACE risk score prediction of hospital mortality in patients with acute coronary syndrome. Clin Cardiol. (2016) 39:516–23. doi: 10.1002/clc.22557
41. Thongprayoon C, Cheungpasitporn W, Chewcharat A, Mao MA, Thirunavukkarasu S, Kashani KB. Hospital mortality and long-term mortality among hospitalized patients with various admission serum ionized calcium levels. Postgrad Med. (2020) 132:385–90. doi: 10.1080/00325481.2020.1728980
42. Hurley K, Baggs D. Hypocalcemic cardiac failure in the emergency department. J Emerg Med. (2005) 28:155–9. doi: 10.1016/j.jemermed.2004.06.014
Keywords: red cell distribution width, serum calcium, in-hospital mortality, acute myocardial infarction, propensity score analysis
Citation: Huang S, Zhang H, Zhuang Z, Guo N, Zhou Q, Duan X and Ge L (2023) Propensity score analysis of red cell distribution width to serum calcium ratio in acute myocardial infarction as a predictor of in-hospital mortality. Front. Cardiovasc. Med. 10:1292153. doi: 10.3389/fcvm.2023.1292153
Received: 15 September 2023; Accepted: 7 December 2023;
Published: 19 December 2023.
Edited by:
Arthur Shiyovich, Rabin Medical Center, IsraelReviewed by:
Diaa Hakim, Harvard Medical School, United States© 2023 Huang, Zhang, Zhuang, Guo, Zhou, Duan and Ge. This is an open-access article distributed under the terms of the Creative Commons Attribution License (CC BY). The use, distribution or reproduction in other forums is permitted, provided the original author(s) and the copyright owner(s) are credited and that the original publication in this journal is cited, in accordance with accepted academic practice. No use, distribution or reproduction is permitted which does not comply with these terms.
*Correspondence: Sulan Huang aHVhbmdzdWxhbmppYXlvdTNAMTI2LmNvbQ== Liangqing Ge Z2VsaWFuZ3FpbmdAMTYzLmNvbQ==
†These authors have contributed equally to this work
Abbreviations AMI, acute myocardial infarction; RDW, red blood cell distribution width; Ca, calcium; RCR, red blood cell distribution width to serum calcium; ratio; PSM, propensity score matching; SMD, standardized mean difference; PCI, percutaneous transluminal coronary intervention; CABG, coronary artery bypass grafting; IPTW, inverse probability of treatment weight; ROC, receiver operating characteristic; AUC, area under curve; SBP, systolic blood pressure; DBP, diastolic blood pressure; SPO2, blood oxygen saturation; SOFA, Sequential Organ Failure Assessment; WBC, white blood cell; RBC, red blood cell; ALT, alanine transaminase; AST, aspartate transaminase; CK-MB, creatine kinase-myocardial band; OR, odds ratio; CI, confidence interval.
Disclaimer: All claims expressed in this article are solely those of the authors and do not necessarily represent those of their affiliated organizations, or those of the publisher, the editors and the reviewers. Any product that may be evaluated in this article or claim that may be made by its manufacturer is not guaranteed or endorsed by the publisher.
Research integrity at Frontiers
Learn more about the work of our research integrity team to safeguard the quality of each article we publish.