- Department of Cardiovascular Diseases, The First Affiliated Hospital of Guangdong Pharmaceutical University, Guangzhou, Guangdong Province, China
Coronary heart disease is a narrowing or obstruction of the vascular cavity caused by atherosclerosis of the coronary arteries, which leads to myocardial ischemia and hypoxia. At present, percutaneous coronary intervention (PCI) is an effective treatment for coronary atherosclerotic heart disease. Restenosis is the main limiting factor of the long-term success of PCI, and it is also a difficult problem in the field of intervention. Sodium-glucose cotransporter 2 (SGLT2) inhibitor is a new oral glucose-lowering agent used in the treatment of diabetes in recent years. Recent studies have shown that SGLT2 inhibitors can effectively improve the prognosis of patients after PCI and reduce the occurrence of restenosis. This review provides an overview of the clinical studies and mechanisms of SGLT2 inhibitors in the prevention of restenosis, providing a new option for improving the clinical prognosis of patients after PCI.
1 Introduction
Coronary heart disease (CHD) is a narrowing or obstruction of the vascular cavity caused by atherosclerosis of the coronary arteries, which leads to myocardial ischemia and hypoxia. With the change in human lifestyle, the number of people with disorders of glucose and lipid metabolism has increased greatly, as well as the incidence of CHD has increased (1). With the progressive implementation of guidance-supported treatments for coronary heart disease, such as percutaneous coronary intervention (PCI), antiplatelet therapy, statins, and angiotensin converting enzyme (ACE) inhibitors, the overall prognosis for CHD has improved significantly over time. Percutaneous coronary intervention therapy for CHD has the advantages of less trauma, fast postoperative recovery, and fewer postoperative complications (2, 3). It can unclog the infarcted vessel, achieve coronary artery reperfusion, and recover the infarcted myocardium to the greatest extent, thus significantly improving the clinical symptoms of patients and increasing the long-term survival rate of patients with coronary heart disease (4). Although medications and interventional treatments greatly reduce the risk for mortality in patients with coronary heart disease, they are still at high risk of developing chronic heart failure (HF) and subsequently facing a higher risk of death and disability (5–8). After the first anniversary of PCI, dual antiplatelet therapy, cardiac rehabilitation, and cardiologist follow-up have typically ended, and the use of secondary prevention medications may be declining (8). It may lead to restenosis, recurrent myocardial infarction (MI), and other manifestations of cardiovascular disease, such as stroke. However, improvements in prognosis for coronary heart disease, especially for myocardial infarction, have slowed in recent years due to limited new treatment options. Therefore, new therapeutic approaches are needed to further improve the treatment of patients with myocardial infarction and prevent recurrence of cardiovascular events.
Restenosis is one of the main causes of recurrent cardiovascular events after PCI, and it is also a difficult problem in the field of intervention (9). Although the availability of drug-eluting stents (DES) has significantly reduced the rate of restenosis and revascularization, the incidence of intrastent restenosis (ISR) is still as high as 5%–10% (10). Therefore, prevention of restenosis has important clinical significance in improving the prognosis of CHD patients treated with PCI. Clinically, insulin resistance and endothelial dysfunction in diabetes mellitus (DM) patients make them more prone to be complicated with coronary heart disease. Patients with DM have an increased incidence of stent thrombosis and restenosis after PCI compared with non-DM patients (11–13). The main mechanisms of restenosis are summarized in Figure 1.
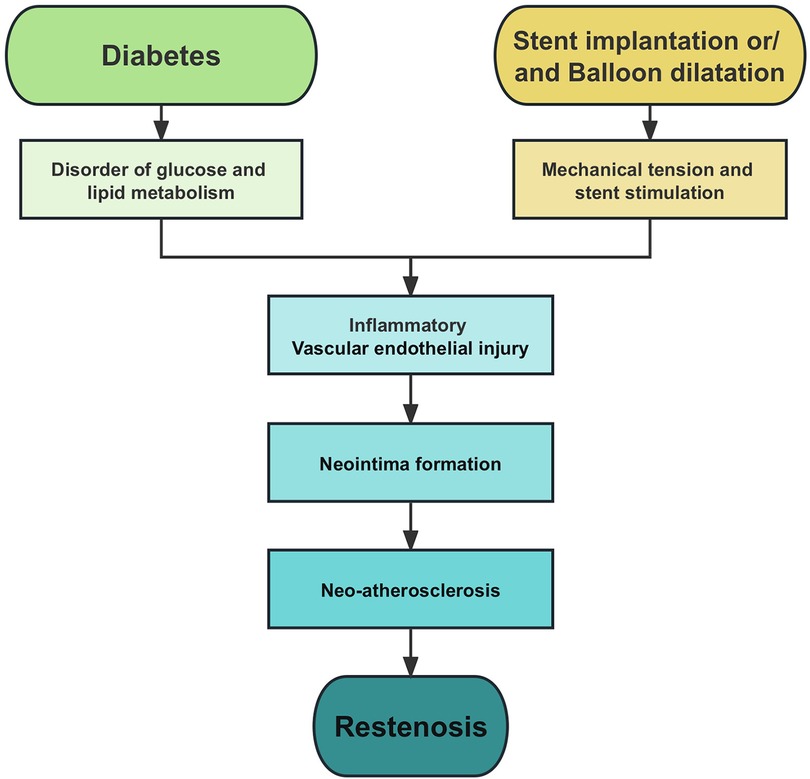
Figure 1. Pathophysiology of restenosis. Endothelial injury, inflammation, and neointima formation are the main causes of restenosis.
SGLT2 inhibitor is a new type of oral glucose-lowering agent used in the treatment of diabetes in recent years (14). They mainly combine with SGLT2 in the renal tubules to prevent the reabsorption of glucose by the kidney and increase the excretion of glucose in the urine, thus reducing the plasma glucose level (15). In addition to glucose-lowering effects, SGLT2 inhibitor has also been shown to have significant cardiovascular protective effects (16–19). The main manifestations are reducing blood pressure, regulating cardiac energy metabolism (16), improving vascular function (15, 20), inhibiting the sympathetic nervous system (21, 22), and preventing adverse myocardial remodeling (23). In addition, there is growing evidence that the use of selected SGLT2 inhibitors in patients with atherosclerotic cardiovascular disease (ASCVD) can improve cardiovascular outcomes (24). The 2023 ESC guidelines for the management of cardiovascular disease in patients with diabetes recommend that, along with GLP-1RAs, SGLT2 inhibitors are the preferred anti-hyperglycemic therapy for T2DM patients with ASCVD, independent of glycemic control considerations and independent of background metformin use (25). SGLT2 inhibitors can improve the prognosis of CHD patients undergoing PCI and reduce the occurrence of various complications, such as acute kidney injury (26, 27), lethal ventricular arrhythmias (21), and ISR (28). Previous studies have shown that SGLT2 is mainly expressed in human kidney and small intestine cells (29), but could not be detected in human myocardium (30). Recently, SGLT2 was found to be expressed in human endothelial cells (EC) and smooth muscle cells (SMC) using immunocytochemistry and western blotting techniques (31, 32). This finding may indicate that SGLT2 may be related to the occurrence and development of CHD and restenosis. This article will review the mechanisms of SGLT2 inhibitors in preventing CHD and restenosis after PCI, to provide new options for improving the clinical prognosis of CHD patients.
2 SGLT2 inhibitor improves the prognosis of patients with CHD
The positive effects of SGLT2 inhibitors in patients with heart failure, particularly in patients with reduced ejection fraction (HFrEF), have been demonstrated (33). ESC guidelines recommend SGLT2 inhibitors as the cornerstone of drug therapy for heart failure, regardless of whether they have combined diabetes (34). Recent clinical trials, including CVD-REAL Nordic (35), EMPA-REG OUTCOME (36–38), and the CANVAS project (39, 40), confirmed the effectiveness of SGLT2 inhibitors in patients with coronary heart disease.
A meta-analysis of six SGLT2 inhibitor trials demonstrated a reduction in the primary ASCVD based composite of time to first MACE (24). This was most apparent in patients with established ASCVD. Compared with the use of other glucose-lowering drugs, the use of SGLT2 inhibitors is associated with reduced major adverse cardiovascular events (MACE) and exert cardiovascular protection in patients who underwent PCI (Table 1) (41, 42).
2.1 Improve the prognosis of patients with myocardial infarction
SGLT2-I AMI PROTECT (NCT05261867) (43) enrolled 646 AMI patients with DM undergoing PCI. The patients were classified into SGLT2 inhibitors users and non-SGLT2 inhibitors users according to the glucose-lowering treatment at admission. The research results show that the use of SGLT2 inhibitors was associated with a significantly reduction in-hospital cardiovascular death, arrhythmic burden, and incidence of contrast media-induced acute kidney injury. After adjusting for confounders, the use of SGLT2 inhibitors was identified as an independent predictor of reduced incidence of MACE and HF hospitalization (44).
EMMY trial (NCT03087773) (45) is a randomized, controlled, double-blind trial to evaluate the role of empagliflozin on cardiac function in patients with acute myocardial infarction (AMI). In this multicenter study, there are 237 patients with AMI received 10 mg once daily of empagliflozin within three days after PCI, while 239 patients received a placebo. The primary outcome of the reduction in N-terminal pro-B-type natriuretic peptide (NT-proBNP) was significantly higher in the empagliflozin group alongside the absolute left ventricle ejection fraction also great improving with those receiving empagliflozin. This suggests that SGLT2 inhibitor has the potential to improve recovery after PCI and encourages related studies in patients with CHD.
EMPACT-MI trial (NCT04509674) (46) was a randomized, double-blind, placebo-controlled, multicenter trial designed to evaluate the efficacy of empagliflozin 10 mg daily in patients at high risk of new heart failure after acute myocardial infarction. Because previous clinical trials of SGLT2 inhibitors excluded patients with acute or recent myocardial infarction, the efficacy of these drugs in reducing future cardiovascular risk in the post-myocardial infarction population was unclear. The EMPACT-MI trial will further evaluate the safety and efficacy of empagliflozin in people at high risk of heart failure or death after myocardial infarction.
DAPA-MI trial (NCT04564742) (47) is another ongoing parallel, randomized, double-blind, placebo-controlled, multicenter phase 3 trial in patients with AMI. Patients with chronic symptomatic heart failure with a known reduced left ventricular ejection fraction (LVEF ≤40%) were excluded from the trial because these patients had an absolute indication for treatment with an SGLT2 inhibitor. This trial evaluated the effect of 10 mg dapagliflozin administered once daily compared with placebo in preventing death, heart failure or other adverse cardiovascular and metabolic events outcomes. The trial explores opportunities to further improve outcomes in patients with impaired left ventricular function after myocardial infarction, which may broaden the indication for the use of SGLT2 inhibitor trials in patients with acute myocardial infarction are listed in Table 2.
2.2 Reduces the risk of repeat revascularization
A subanalysis of the EMPA-REG OUTCOME randomized trial (48) elucidates the benefit of empagliflozin in patients with type 2 diabetes and a history of CABG, suggesting that empagliflozin significantly reduces important adverse cardiovascular and renal outcomes in this high-risk population. Among participants with a history of coronary artery bypass, compared with placebo, cardiovascular death was reduced by 48%, all-cause mortality by 43%, hospitalization for HF by 50% with empagliflozin. Among participants with a history of coronary artery bypass at baseline, the proportion receiving empagliflozin (7.7%) was lower than that receiving placebo (9.8%) and had undergone ≥1 coronary artery reconstruction during the trial period. Similarly, a nationwide retrospective cohort study showed that SGLT2 inhibitors, compared with dipeptidyl peptidase-4 inhibitors, were associated with a lower risk of coronary revascularization, combined renal outcomes, and all-cause mortality in T2DM patients after PCI (41).
A prospective, observational study (28) included a total of 377 patients with type 2 diabetes who underwent PCI. Comparing those who had never used SGLT2 inhibitors, the patients who were currently using SGLT2 inhibitors with a significantly lower incidence of MACE defined as cardiac death, re-infarction, and heart failure. Cox regression analysis showed that the use of SGLT2 inhibitors also significantly reduced the incidence of ISR-related adverse cardiovascular events in patients with well-controlled blood glucose (mean HbA1c <7% within 1 year). This also suggests that the mechanism by which SGLT2 inhibitors reduces the occurrence of ISR may be independent of the glucose-lowering effect. Recently, another retrospective real-world study (49) also showed that dapagliflozin significantly improved BMI, blood pressure, and glucose outcomes in patients with T2DM combined with coronary heart disease after PCI and had a beneficial effect on MACE and ISR.
3 Mechanism of action of SGLT2 inhibitor in preventing coronary heart disease and restenosis
Mechanistically, inhibition of SGLT2 leads to increased renal glucose excretion and decreased serum glucose levels. With the increase in clinical application, many studies have shown that SGLT2 inhibitors can significantly improve the prognosis of CHD patients. However, it is difficult to explain the glucose-lowering effect of SGLT2 inhibitors to inhibit arteriosclerosis and long-term pathological improvement. This suggests that SGLT2 inhibitors may play a direct role. In addition to its glucose-lowering effects, several studies, including the ongoing EMPACT-MI trial (46) and DAPA-MI trial (47), have demonstrated a positive cardiovascular protective effect of SGLT2 inhibitors in non-DM patients. Interestingly, SGLT2 inhibitors could reduce ISR-related events in type 2 DM (T2DM) patients with acute coronary syndrome and the effect was independent of glycemic control, suggesting that SGLT2 inhibitors may have a beneficial effect on coronary artery remodeling after stent implantation, perhaps by modulating a wide range of metabolic, molecular, and hemodynamic mechanisms besides its glucose-lowering effects. We summarize the main mechanism in Figure 2.
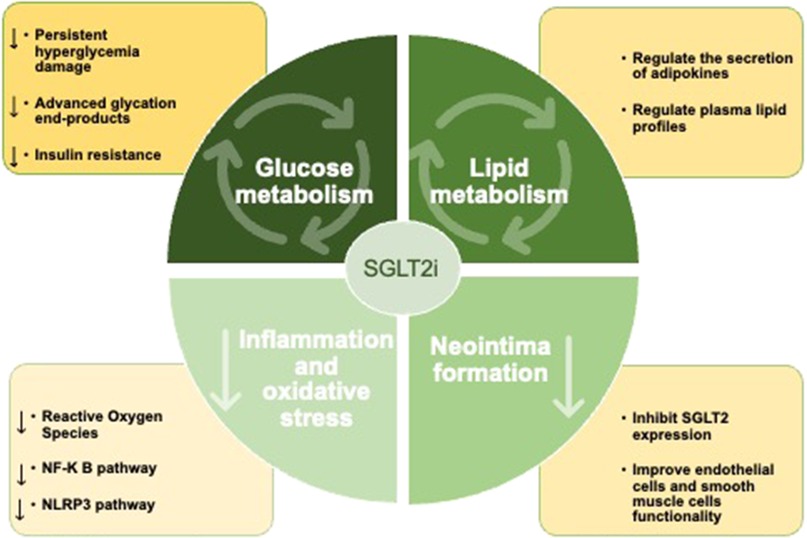
Figure 2. SGLT2 inhibitors can improve the prognosis of patients after PCI by improving glucose and lipid metabolism, inhibiting inflammatory response and oxidative stress, and inhibit neointima formation.
3.1 SGLT2 inhibitor can improve glucose metabolism
Several studies have proved that diabetes is an independent risk factor for ISR in patients after PCI (11–13). DM patients have an increased incidence of restenosis after PCI compared with non-DM patients (50). The mechanism of ISR development in DM patients may be different from non-DM patients. Diabetes results in more extensive neointimal hyperplasia, atherosclerotic plaque formation, hemodynamic changes, and inadequate compensatory vascular remodeling (51–55). Faries et al. found that VSMCs of DM patients exhibit remarkably increased rates of proliferation, adhesion, and migration, as well as abnormal cell culture morphology (56). In addition, Moreno et al. found higher levels of lipid-rich atherosclerosis, macrophage infiltration, and subsequent thrombosis in coronary artery tissue of DM patients compared to non-DM patients. These differences indicate an increased susceptibility to coronary thrombosis in patients with DM (57). These observations are consistent with the clinically observed increased incidence of atherosclerosis and restenosis in patients with diabetes.
SGLT2 inhibitor is a new type of oral glucose-lowering agent used in the treatment of diabetes in recent years (14). They mainly combine with SGLT2 in the renal tubules to prevent the reabsorption of glucose by the kidney and increase the excretion of glucose in the urine, thus reducing the plasma glucose level (15). The unique glucose-lowering mechanism of SGLT2 inhibitors independent of insulin secretion makes them ideal for the treatment of T2DM.
3.1.1 Reduce persistent hyperglycemia damage
The effect of persistent hyperglycemia on the development of macrovascular and microvascular complications has been widely discussed (58, 59). The harmful effects of glucose are already present when plasma glucose levels fall below the threshold for diagnosing DM (60). Hyperglycemia initially leads to an imbalance between nitric oxide (NO) bioavailability and reactive oxygen species (ROS) accumulation, which in turn leads to increased inflammatory response and oxidative stress, endothelial dysfunction, and vascular smooth muscle proliferation, ultimately leading to coronary atherosclerotic plaque formation and restenosis (59, 61).
As a new oral glucose-lowering agent, SGLT2 inhibitor can significantly reduce the levels of fasting plasma glucose and HbA1c, and alleviate the endothelial damage caused by persistent hyperglycemia (62). Studies in cultured endothelial cells have shown that high glucose increases inflammatory mediators and SGLT2 expression, and intervention with SGLT2 inhibitors can inhibit the development of unstable plaque phenotypes (63). A randomized clinical trial found that the use of SGLT2 inhibitors significantly reduced the absolute risk of atherosclerotic thrombosis in patients with T2DM (55). Joubert et al. found that the total levels of O-GlcNAcylated proteins were significantly lower in dapagliflozin-treated SKO mice than in untreated SKO mice (64). This suggests the notion that dapagliflozin partially corrects cardiac dysfunction through glucose-lowering effects. Long-term application of SGLT2 inhibitors has a good effect on the clinical prognosis of hospitalized patients with AMI after PCI, and it can narrow the prognostic gap still existing between DM and non-DM patients (65).
3.1.2 Reduce the harmful effects of advanced glycation end-products
Advanced glycation end-products (AGEs), called glycation or Maillard reactions, are chemicals that result from non-enzymatic interactions between proteins and sugar residues (66). AGEs are recognized by a variety of cellular receptors in the body and trigger many signaling pathways associated with inflammation and oxidative stress (66). AGEs continue to accumulate in the body as we age, and DM accelerates the development of this process (67). The accumulation of AGEs resulted in increased expression of receptors for AGEs (RAGE), inflammatory cell count, intercellular adhesion molecule (ICAM)-1, and vascular cell adhesion molecule (VCAM)-1 (68, 69). The engagement of RAGE with AGEs elicits oxidative stress generation and subsequently induces the proliferation, adhesion, and migration of SMC, thus evoking vascular injury in diabetes (68). These findings may verify that the accumulation of AGEs may contribute to the increased rate of restenosis in people with DM (50, 70).
An animal and cell experiment showed that empagliflozin reduced AGEs in serum by inhibiting the AGEs-RAGE pathway, inhibiting the expression of the RAGE, and at the same time reducing levels of cholesterol as well as proteins associated with cholesterol metabolism (71). Empagliflozin also plays an anti-inflammatory and antifibrotic role in diabetic nephropathy by inhibiting the AGEs-receptor axis (72). Dapagliflozin protects podocytes from advanced AGEs-induced inflammatory response and apoptosis via the AMPK/mTOR (mammalian target of rapamycin)-mediated autophagy pathway (73). The above studies suggest that SGLT2 inhibitors may play an anti-atherosclerotic role by inhibiting AGEs, but it needs to be further validated in vascular models.
3.1.3 Attenuate insulin resistance
Insulin resistance (IR) is a significant risk factor for CHD and is associated with inflammatory responses (74), metabolic disorders (75), elevated fibrinogen and decreased plasminogen activator (74), and endothelial dysfunction (76), leading to adverse cardiovascular events (61, 77). IR is also associated with the incidence of restenosis (75, 78–80). Adipose tissue plays a key role in the pathogenesis of IR and DM through the secretion of adipokines and inflammatory cytokines, as well as the accumulation of visceral fat (81, 82). Dysfunction and reduced metabolism of fat cells are strongly associated with IR and DM, although their role in IR is complex and poorly understood.
Drugs can lead to adaptive responses in glucose homeostasis and hormone release, and the genetic or pharmacological inhibition of SGLT2 has positive effects on hyperinsulinemia and hyperglycemia (83, 84). At present, SGLT2 inhibitors has been shown to significantly attenuate insulin resistance. The mechanism by which SGLT2 inhibitor attenuates IR can be summarized as follows: (1) by regulating the body's energy balance and metabolism, (2) by promoting the transformation of white adipose tissue into brown adipose tissue, and (3) by inhibiting the inflammation induced by polarizing M2 macrophages (85–90).
3.2 SGLT2 inhibitor can inhibit neointima formation
As a result of stent implantation, the physiological barrier function of EC is impaired due to changes in cell connectivity, and its ability to regulate vascular tone, control inflammation, maintain lipid and tissue fluid homeostasis, and prevent thrombosis is reduced (91, 92). At the same time, SMC changed from contractive phenotype to synthetic phenotype (93). The proliferation and migration of SMC were enhanced, and the secretion of cytokines and extracellular matrix was increased. Synthetic SMC can also migrate into the lipid plaque adhering to the intima to phagocytose lipids, form foam cells, synthesize and secrete fibrin, and eventually cause ISR (94).
SGLT2 inhibitors can effectively regulate the proliferation, migration, differentiation, survival, and senescence of EC (95). The DEFENCE study was the first to assess the role of dapagliflozin on vascular endothelial function in T2DM patients (96). Flow-mediated dilation (FMD) is recognized as an alternative marker of early endothelial dysfunction. dapagliflozin combined with therapy on metformin improved endothelial function in patients with inadequate glycemic control assessed by FMD. Sawada et al. found that empagliflozin can also increase FMD% and play an endothelial protective role by improving hypertriglyceridemia and IR (97). In addition, SGLT2 inhibitor also inhibits the contraction of VSMC and blocks the proliferation and migration of these cells. Hashikata et al. found that standard therapy add-on empagliflozin reduced neointimal progression compared to intensive standard therapy after DES implantation (98). Therefore, SGLT2 inhibitors might be promising for the prevention of ISR following PCI, especially in DM patients at high risk for restenosis.
3.2.1 Inhibit the expression of SGLT2 in endothelial cells and smooth muscle cells
It was previously agreed that SGLT2 is mainly expressed in human kidney and small intestine cells (29), but could not be detected in human myocardium (30). Recently, SGLT2 was found to be expressed in human endothelial cells (EC) and smooth muscle cells (SMC) using immunocytochemistry and western blotting techniques (31, 32). Interestingly, D'Onofrio N. et al. found that plaques from patients with diabetes had higher SGLT2 expression, inflammation, and oxidative stress, compared to plaques from patients without diabetes (63). This seems to be consistent with a higher risk of coronary atherosclerotic heart disease and restenosis in people with diabetes. Dutzmann et al. demonstrated that empagliflozin damages SMC proliferation and accelerates endothelial regeneration in vitro. In addition, empagliflozin prevents neointimal lesion formation and enhances reendothelialization after vascular injury in vivo (31). The mechanism may depend on vascular SGLT2 and involve the cardiovascular active peptide apelin.
Recently, dapagliflozin has also been shown to have a unique potential for cardioprotective effects by modulating apelin levels in patients and may serve as an alternative target for HF management in T2DM patients (99). Apelin is involved in the negative regulation of SMC proliferation and the improvement of EC function (100, 101), but the specific mechanism is not clear. However, the specific role of EC and SMC expression of SGLT2 is still unclear, and more studies may be needed to confirm and explore its mechanism of action in the future.
3.2.2 Enhance eNOS activation and increase the bioavailability of NO in endoderm
The release of NO by endothelial nitric oxide synthase (eNOS) plays an important role in maintaining vascular health (102). NO has been demonstrated to decrease migration and proliferation of VSMCs to attenuate the binding of inflammatory cells to the vascular wall, inhibit thrombosis by reducing platelet adhesion and aggregation, and resist angiosclerosis (103, 104). The regenerated vascular endothelial cells not only had poor cell junction formation but also significantly decreased the expression of antithrombotic molecules and NO (80). Disruption of NO synthesis leads to endothelial dysfunction and loss of homeostasis, resulting in endothelial cell apoptosis, enhanced endothelial permeability, impaired endothelium-dependent vasodilation, endothelial cell activation, inflammation, thrombosis, and neointima formation, which contribute to the development of vascular disease (105). Restenosis is closely related to the decrease of NO production by the damaged EC and the decrease in the amount of enzyme protein produced by the EC (106). Furthermore, Wu et al. showed that NO-dependent endothelial vasodilation was impaired in patients with ISR after coronary stent placement, compared with patients without restenosis (107).
SGLT2 inhibitor can enhance eNOS activation, increase the bioavailability of endodermal NO, and restore endothelium-dependent vasodilation (108, 109), these might have contributed to the beneficial vascular effects. A prospective cohort study demonstrated that empagliflozin increases blood viscosity and carotid shear stress (SS) as well as decreases carotid wall thickness (110). Within a certain range, higher levels of wall SS have a protective effect on atherosclerosis, which is conducive to the release of NO and the regulation of endothelial function and hemodynamic performance. In empagliflozin-treated mice, the activity of NO synthase in vascular endothelium increased, the content of phosphorylated cofilin and filamentous actin decreased, and the pathway involved in ROS production was down-regulated (109). Salim et al. found that ipragliflozin administration ameliorated impaired phosphorylation of eNOS, and attenuated endothelial dysfunction in diabetic mice (111). Therefore, substantial evidence supports the role of SGLT2 inhibitor in maintaining NO bioavailability and endothelial function by influencing the activity or expression of many molecules involved in eNOS, oxidative stress, inflammation, and blood pressure. The endothelial protection function of SGLT2 inhibitor plays an important role in delaying the formation of ISR.
3.3 Inhibit inflammation and oxidative stress
Inflammation is involved in the occurrence and development of atherosclerosis and affects its prognosis. There is increasing evidence that low-grade chronic inflammation is a key link in the pathogenesis of ISR (112). Endothelial injury after PCI causes inflammatory activation, thrombosis, and proliferation of vascular smooth muscle cells, and eventually leads to ISR formation (66). Inflammatory markers such as interleukin (IL)-6, IL-8, tumor necrosis factor (TNF) -α, monocyte chemotactic protein (MCP) -1, and matrix metalloproteinase (MMP) have been shown to play important roles in the pathogenesis of coronary atherosclerotic heart disease and ISR (113, 114). It can be used as a predictor of prevention and treatment of intra-stent restenosis. By monitoring changes in inflammation-related indicators, early identification of patients with ISR can be achieved, reducing the risk of sudden death in patients with coronary atherosclerotic heart disease.
The increased expression of SGLT2 was associated with higher oxidative stress, nuclear factor-κ B (NF- κ B), and proinflammatory cytokines (63). SGLT2 inhibitor treatment significantly reduces the expression of inflammatory markers TNF-α, IL-1β, and IL-6, thereby delaying the occurrence and progression of atherosclerosis (115). An international multicenter observational study found that in patients with diabetes who underwent PCI, the use of SGLT2 inhibitor was an important predictor of reduced inflammatory response (43). And recent studies have found that SGLT2 inhibitor reduces infarct size and the occurrence of ischemia-reperfusion-induced arrhythmias, and plays a cardioprotective role, which may be achieved by inhibiting inflammatory factors and oxidative stress (116). Therefore, SGLT2 inhibitor could significantly reduce the inflammatory index in patients after PCI, improving the long-term prognosis.
3.3.1 Reduce ROS generation
Inflammation and oxidative stress are both important and interdependent factors in almost all major cardiovascular diseases (117). Inflammation can induce oxidative stress directly or indirectly through a variety of cell signaling pathways, including mediators that activate ROS sources. Several molecular mechanisms have been implicated in hyperglycemic-induced endothelial damage, such as activation of PKC isoforms, increased hexosamine pathway flux, increased AGEs formation, increased polyol pathway flux, and activation of the proinflammatory NF-k B, which are associated with overproduction of ROS (19, 52, 53, 58, 118). In addition, excess glucose can be metabolized through the pentose phosphate pathway and produce nicotinamide adenine dinucleotide phosphate hydrogen (NADPH) (119). NADPH is a substrate for cytoplasmic NADPH oxidase, an enzyme complex that can produce ROS (117). Eventually, ROS can lead to endothelial dysfunction, decreased vasodilation, and atherosclerotic plaque formation, which will lead to coronary heart disease and restenosis.
Studies have shown that SGLT2 inhibitor can lead to downregulation of key proteins and pathways involved in ROS biosynthesis (109, 120). Li et al. found that SGLT2 inhibitor has an antioxidative effect and alleviates mechanical forces induced endothelial permeability in human coronary artery endothelial cells, which may be mediated by ROS clearance (121). In addition, they found that the antioxidative effect of SGLT2 inhibitors might be mediated in part by inhibition of NADPH oxidase and Na + /H + exchanger (NHE). Elevated intracellular Na + hinders mitochondrial Ca2 + processing and deteriorates energy supply and demand matching and the mitochondrial antioxidative defense systems (122). By decreasing the intracellular Na + concentrations through inhibition of the mitochondrial NHE, the production of ROS can be inhibited, thus having a favorable effect on cardiac metabolism (123, 124). Of note, NHE is also expressed in VSMC, and inhibition of NHE blunts lysophosphatidic acid-induced VSMC proliferation (125). Dapagliflozin and empagliflozin attenuate the upregulation of the cardiac NHE in mouse cardio fibroblasts stimulated with lipopolysaccharides (126, 127). The effect of dapagliflozin was blocked with an AMPK inhibitor, and it suggested that dapagliflozin may inhibit NHE by inhibiting the expression of AMPK, exerting the cardiovascular protective effect of antioxidative stress. However, in the previous studies, AMPK activation was not only observed in empagliflozin and dapagliflozin but in canagliflozin-treated cells (120). It seems paradoxical, and the involvement of AMPK phosphorylation may require further discussion.
3.3.2 Inhibit Nf-κ B pathway
The nuclear factor-κ B family of transcription factors, especially p65 and p50, participate in the pathogenesis of vascular proliferative diseases (128). Expression of NF-κ B has also been detected in the nucleus of human atherosclerotic lesions. We have previously demonstrated in restenosis animal models that inflammation induced by the NF-κ B pathway is closely relevant to the occurrence and development of coronary atherosclerosis and vascular restenosis after interventional therapy (129). Our results showed that the inhibition of NF-κ B pathway can downregulate the expression of downstream factors IL-1, IL-6, TNF-α, VCAM-1, and MCP-1, which can significantly improve the lipid profile and inhibit restenosis.
SGLT2 inhibitor may reduce the gene expression level of inflammatory factors and protein expression level of NF-κ B, thus alleviating atherosclerosis and ISR progression. Mice treated with dapagliflozin were found to have reduced atherosclerotic plaques and reduced serum levels of inflammatory markers such as IL-6, IL-8, TNF-α, and MCP-1 (130). Gaspari et al. also found that dapagliflozin-mediated attenuation of TNFα-induced NF-κ B mRNA expression implicates dapagliflozin in transcriptional regulation (131). Similarly, empagliflozin can reduce the gene expression level of inflammatory factors and protein expression level of NF-κ B, improve the protein expression level of AMPK affected by ox-LDL in cells, and delay the formation of atherosclerosis (132).
3.3.3 Inhibit NLRP3 pathway
Previous studies revealed elevated expression of the nucleotide binding domain-like receptor protein-3 (NLRP3) inflammasome in human atherosclerotic arteries and restenosis artery wall (133, 134). A study showed that NLRP3 binding to ligands promotes inflammasome formation, activates caspase-1, and eventually promotes the maturation and secretion of IL-1β, and IL-18 (135). Those cytokines are induced following vascular injury and play a role in intimal hyperplasia. Notably, compared with those of the non-DM mice, DM mice had significantly increased serum levels of NLRP3, IL-1β and IL-18 (136). Recent studies have shown that targeted inhibition of NLRP3 can dampens inflammatory leucocyte production and uptake in atherosclerosis, significantly enhancing reendothelialization and prevent neointimal formation (137, 138). It suggests that NLRP3 inflammasome may be a promising therapeutic target for restenosis.
In vitro and in vivo experiments have demonstrated that SGLT2 inhibitor might inhibit the NLRP3 inflammasome, thereby reducing the secretion of inflammatory markers. In addition, under normal glucose conditions, SGLT2 inhibitor inhibits SMC migration and proliferation by targeting IL-17A mediated IL-1β and IL-18 expression, NLRP3 expression, and inflammatory responses (32, 139). Leng et al. elucidate that dapagliflozin treatment was associated with the inhibition of the secretion of IL-1β by macrophage via the ROS-NLRP3-caspase-1 pathway in atherosclerosis for the first time (136). In addition, empagliflozin can degrade the inflammatory component NLRP3 through selective autophagy and reduce the maturation and secretion of inflammatory factors (140). SGLT2 inhibitor has been demonstrated to cause a considerable increase in serum β-hydroxybutyrate with a parallel decline in fasting serum insulin levels due to a significant improvement in insulin sensitivity (141). These effects were associated with decreased IL-1β production and inhibition of NLRP3 inflammasome activity, which might help explain its cardioprotective effects.
3.4 SGLT2 inhibitor can regulate lipid metabolism
Drug therapy strategies based on statins and supplemented by a variety of lipid-lowering drugs, including PCSK9 inhibitors, have been widely used in secondary prevention of atherosclerotic cardiovascular disease (142). Although this optimized drug therapy reduces the risk of cardiovascular disease, there is still a residual risk that cannot be controlled. The effect of dapagliflozin combined with statins on reducing triglyceride-glucose (TyG), a related indicator of glycolipid metabolism, was significantly better than that of statins alone (42). This suggests that the target of SGLT2 inhibitor may not be limited to glucose metabolism, but also has a regulatory effect on lipid metabolism.
3.4.1 Regulate the secretion of adipokines
It is known that the adipose tissue can produce and secrete a wide variety of bioactive molecules known collectively as adipocytokines, such as adiponectin (APN), leptin, IL-6, IL-1β, and MCP-1 (143, 144). Those adipocytokines are not only involved in fatty acid metabolism and glucose homeostasis but also play a role in the control of inflammatory responses (145). Adipose tissue-specific adipocytokines leptin and APN interact with AMPK to regulate fatty acid and energy metabolism (146).
In our previous study (147), we established a rabbit model of iliac artery restenosis by percutaneous intracavity balloon angioplasty. Our previous research has shown that the expressions of TNF-α, IL-6, and MCP-1 were considerably increased (P < 0.01), while the expression of APN in the restenosis group was considerably decreased (P < 0.01). We conclude that balloon angioplasty and high-fat diet can inhibit APN expression and increasing APN expression may prevent the formation of restenosis after PCI (148). In atherosclerotic ApoE(-/-) mice, changes in adipokines were consistent with our data. After treatment with empagliflozin, the circulating concentrations of TNF-α, IL-6, and MCP-1 were decreased, and APN concentrations were increased, which played an anti-atherosclerotic role (149). Interestingly, changes in these adipocytokine levels were closely related to changes in blood lipids, IR, and body weight. Compared with sitagliptin, another glucose-lowering agent, dapagliflozin can significantly increase serum APN levels in diabetic patients (150). Nishitani et al. performed gene expression microarray and metabolomic analyses of mouse adipose tissue, and the result showed that dapagliflozin improved serum glucose levels and induced 3-hydroxybutyric acid in diabetic mice (87). 3-hydroxybutyric acid has been proven to enhance adiponectin expression in adipocytes, and thus exert anti-inflammatory and anti-atherosclerosis effects (87). In addition, leptin also plays an important role in the regulation of vascular function. It has been reported that leptin can regulate the synthesis of NO in ECs and play a vascular protective role (151). Elevated leptin levels were present in patients with restenosis after stent placement, while consistent results were confirmed in patients without restenosis and in the control group (80). Recently, a meta-analysis concluded that SGLT2 inhibitors treatment was associated with decreased serum leptin levels and increased serum APN levels, which may contribute to the positive effects of SGLT2 inhibitors on metabolic homeostasis (152).
3.4.2 Regulate plasma lipid profiles
Clinical data and animal studies have shown that lipid levels are closely related to the occurrence of CHD and restenosis. It has been demonstrated that an increase in high-density lipoprotein cholesterol levels may be a predictor of anti-atherosclerotic effect (98). We have also verified in previous studies that restenosis is often accompanied by increased plasma levels of total cholesterol, low density lipoprotein cholesterol, and very low-density lipoprotein cholesterol (129, 147). Recent studies have found that improvement in hypertriglyceridemia was the strongest independent predictor of improvement in endothelial function in CAD patients with diabetes (97). There was an independent positive correlation between the TyG index and the risk of ISR in acute coronary syndrome patients after DES implantation (153).
SGLT2 inhibitor has been shown to exert a cardiovascular protective effect by affecting plasma lipid levels (21). In comparison with sitagliptin, dapagliflozin decreases low-density lipoprotein cholesterol and increases high-density lipoprotein cholesterol in patients with type 2 diabetes (150). The EMPAREG OUTCOME study also showed a sustained increase in high-density lipoprotein cholesterol levels less than 6 months after taking empagliflozin as well. In addition, empagliflozin has also been shown to play a protective role in vascular endothelium by improving hypertriglyceridemia in patients with coronary heart disease and diabetes (97).
3.5 Inhibit the sympathetic nervous system
There is strong evidence implicating sympathetic nervous system activation in the pathogenesis of cardio-metabolic illnesses including obesity, metabolic syndrome (MetS), diabetes, and hypertension (HTN) (154). The rapid increase in sympathetic nerve activity leads to increased levels of lipolysis and fatty acids in plasma, increased gluconeogenesis in the liver, inhibition of insulin release, and a shift in fuel metabolism in the muscles towards fatty acid oxidation and other directions (155, 156). However, if the sympathetic activity is chronically elevated due to a poor lifestyle, the physiological responses may move in an unfavorable direction, including elevated fasting blood glucose levels and IR (157), as well as elevated blood pressure and hypertension (158).
A recent study demonstrated that canagliflozin increased energy expenditure and fat utilization by increasing sympathetic activation of adipose tissue (159). Herat et al. also found that dapagliflozin-treated MetS mice fed a high-fat diet showed reduced blood pressure, weight loss, reduced hyperglycemia, and increased glucose tolerance (22). Interestingly, dapagliflozin treated high-fat diet mice, reducing renal tyrosine hydroxylase and norepinephrine levels in MetS mice. This suggests that dapagliflozin inhibition of SGLT2 leads to metabolic benefits in MetS mouse models via sympathetic inhibition. Matthews et al. found that SGLT2 expression increased in human renal proximal tubule cells after treated with norepinephrine for 48 h (157). The data support that sympathetic nervous system activation may up-regulate SGLT2 expression in human renal proximal tubule cells. The EMBODY trial (21) elucidates the mechanism by which empagliflozin reduces cardiovascular-related death, including sudden cardiac death in patients with AMI and T2DM. The trial identified that SGLT2 inhibitor may show significant improvements in cardiac sympathetic and parasympathetic nerve activity in heart rate variability and heart rate turbulence, and this mechanism also plays a beneficial role in the prognosis of patients after PCI.
4 The safety of SGLT2 inhibitors
Safety is the major concern after the emergence of new drugs, and attention should be paid to any problems that may arise. Known allergic reactions, pregnancy/lactation period, eGFR <20 ml/ min / 1.73 m2, symptoms of hypotension or systolic blood pressure <95 mmHg are contraindications for SGLT2 inhibitors (160). Adverse events might occur with SGLT2 inhibitors including an increased risk of orthostatic hypotension, urinary tract infection, diabetic ketoacidosis, renal impairment, and possibly limited amputations (161–163). Current guidelines and expert consensus generally agree that SGLT2 inhibitors should be avoided whenever possible during acute progression to avoid adverse events (164). However, in some clinical trials on acute and chronic HF, these adverse events were uncommon in the SGLT2 inhibitors treatment group, and the incidence of adverse events was not significantly increased compared to the control group (165, 166). Overall, SGLT2 inhibitors has many positive effects, and the benefits of using SGLT2 inhibitors far outweigh the risks. In addition, these adverse events are preventable and ameliorable, and these adverse reactions should not be used as contraindications for the use of the drug.
4.1 Orthostatic hypotension
Treatment with SGLT2 inhibitors reduces volume and sodium load through its diuretic and natriuretic properties. The resulting reduction in circulatory load, especially in ventricular filling pressure and cardiac load, may be an important mechanism by which the use of SGLT2 inhibitors can reduce mortality in patients. There was evidence that patients treated with empagliflozin and dapagliflozin had similar rates of hypotension and other adverse events related to volume reduction compared with placebo (165). However, in the CANVAS program, patients using canagliflozin showed a significant difference in volume reduction compared to placebo (26.0 vs. 18.5 event rates per 1,000 patient years, p = 0.009) (40). When symptomatic hypotension or a systolic blood pressure <95 mmHg, patients are prohibited from using SGLT2 inhibitors (160). Therefore, the volume status of patients should be assessed and treated before starting SGLT2 inhibitors. Caution should be exercised when initiating SGLT2 inhibitors in patients with decreased kidney function, older adults, and low baseline systolic blood pressure. During medication, blood pressure should be monitored and the dosage of both diuretics and drugs that affect blood pressure should be adjusted.
4.2 Urinary tract infection
Because SGLT2 inhibitors can cause significant glycosuria, especially in patients with T2DM, there is an increased risk of genital infection. Although meta-analyses have found that SGLT2 inhibitors do not increase the occurrence of urinary tract infections, these results may contain additional sources of uncertainty (167). To avoid urinary tract infection risk, prior to the use of SGLT2 inhibitors, possible previous urogenital infections and existing risk factors should be documented. In addition, SGLT2 inhibitors are not recommended for patients with recurrent genitourinary infections within 6 months.
4.3 Euglycemic diabetic ketoacidosis (EDKA)
SGLT2 inhibitors produces ketone bodies in the presence of normal blood sugar levels (168). Recent studies have suggested that the increase in ketone bodies produced by SGLT2 inhibitors creates a “super fuel” that can improve the efficiency of the heart and kidneys, thereby improving cardiac contractility and kidney function (169). Although increased ketone body formation can provide potential benefits for heart and kidney function, increased ketone body production will continue to put patients at increased risk for ketoacidosis (170). Patients with abdominal pain, nausea, vomiting, weakness, and dyspnea should be considered for EDKA during SGLT2 inhibitor use. Once EDKA is diagnosed, SGLT2 inhibitors should be discontinued. In addition, the ESC guide recommends that type 1 diabetes mellitus is not an absolute contraindication, but an individual risk of ketoacidosis should be considered when starting SGLT2 inhibitors therapy (160).
4.4 Amputation
The CANVAS study showed that canagliflozin increased the risk of lower limb amputation, while other studies have not confirmed whether SGLT2 inhibitor treatment is associated with lower limb amputation (171). Canagliflozin can damage the proliferation and migration of endothelial cells and smooth muscle cells, which may affect the re-endothelialization of arteries after PCI and play a positive role in the prevention of ISR (172, 173). On the other hand, it may exacerbate peripheral artery disease in diabetic patients by restricting angiogenesis. SGLT2 inhibitors should be used with caution in patients with risk factors for amputation, especially those with a previous history of amputation or foot ulcers, neuropathy, or peripheral vascular disease.
5 Conclusion and prospect
In summary, SGLT2 inhibitors can improve the prognosis of patients after PCI by improving glucose and lipid metabolism, inhibiting inflammatory response and oxidative stress, and improving vascular endothelial function and vascular remodeling. In addition, the early initiation of SGLT2 inhibitors can reduce the myocardial infarction size and the occurrence of reperfusion injury during AMI and can improve further outcome of impaired left ventricular function after AMI. SGLT2 inhibitors may be a new hope to improve the prognosis of patients with coronary heart disease, especially those with myocardial infarction, and prevent the recurrence of cardiovascular events. Recent studies suggest that the cardiovascular protective effect may remain significant in non-DM patients, independent of its hypoglycemic effect. In the future, more randomized controlled trials are needed to verify the beneficial effect of SGLT2 inhibitors in patients with coronary heart disease to to address the residual risks of current treatment options.
Author contributions
QZ: Methodology, Writing – original draft. ZD: Writing – original draft. TL: Writing – review & editing. KC: Writing – review & editing. ZZ: Validation, Writing – review & editing.
Funding
The author(s) declare financial support was received for the research, authorship, and/or publication of this article.
This work was supported by the Guangdong Provincial Innovation Team Project of Colleges and Universities (No. 2019KCXTD018 to TL) the Planning Projects of Scientific Technology of Guangzhou (Grant Nos. 44573039 to KC) and National Natural Science Foundation of China (Grant Nos. 82300440 to KC).
Conflict of interest
The authors declare that the research was conducted in the absence of any commercial or financial relationships that could be construed as a potential conflict of interest.
Publisher's note
All claims expressed in this article are solely those of the authors and do not necessarily represent those of their affiliated organizations, or those of the publisher, the editors and the reviewers. Any product that may be evaluated in this article, or claim that may be made by its manufacturer, is not guaranteed or endorsed by the publisher.
References
1. Tian Y, Deng P, Li B, Wang J, Li J, Huang Y, et al. Treatment models of cardiac rehabilitation in patients with coronary heart disease and related factors affecting patient compliance. Rev Cardiovasc Med. (2019) 20(1):27–33. doi: 10.31083/j.rcm.2019.01.53
2. Doenst T, Haverich A, Serruys P, Bonow RO, Kappetein P, Falk V, et al. PCI And CABG for treating stable coronary artery disease: JACC review topic of the week. J Am Coll Cardiol. (2019) 73(8):964–76. doi: 10.1016/j.jacc.2018.11.053
3. Shan L, Saxena A, McMahon R. A systematic review on the quality of life benefits after percutaneous coronary intervention in the elderly. Cardiology. (2014) 129(1):46–54. doi: 10.1159/000360603
4. Konijnenberg LSF, Damman P, Duncker DJ, Kloner RA, Nijveldt R, van Geuns RM, et al. Pathophysiology and diagnosis of coronary microvascular dysfunction in ST-elevation myocardial infarction. Cardiovas Res. (2020) 116(4): 787–805. doi: 10.1093/cvr/cvz301
5. Jernberg T, Hasvold P, Henriksson M, Hjelm H, Thuresson M, Janzon M. Cardiovascular risk in post-myocardial infarction patients: nationwide real world data demonstrate the importance of a long-term perspective. Eur Heart J. (2015) 36(19):1163–70. doi: 10.1093/eurheartj/ehu505
6. Ferrannini G, Almosawi M, Buhlin K, De Faire U, Kjellstrom B, Klinge B, et al. Long-term prognosis after a first myocardial infarction: eight years follow up of the case-control study PAROKRANK. Scand Cardiovasc J. (2022) 56(1):337–42. doi: 10.1080/14017431.2022.2112072
7. Johansson S, Rosengren A, Young K, Jennings E. Mortality and morbidity trends after the first year in survivors of acute myocardial infarction: a systematic review. BMC Cardiovasc Disord. (2017) 17(1):53. doi: 10.1186/s12872-017-0482-9
8. Rapsomaniki E, Thuresson M, Yang E, Blin P, Hunt P, Chung SC, et al. Using big data from health records from four countries to evaluate chronic disease outcomes: a study in 114 364 survivors of myocardial infarction. Eur Heart J Qual Care Clin Outcomes. (2016) 2(3):172–83. doi: 10.1093/ehjqcco/qcw004
9. Alfonso F, Coughlan JJ, Giacoppo D, Kastrati A, Byrne RA. Management of in-stent restenosis. EuroIntervention. (2022) 18(2):e103–23. doi: 10.4244/EIJ-D-21-01034
10. Byrne RA, Joner M, Kastrati A. Stent thrombosis and restenosis: what have we learned and where are we going? The Andreas Gruntzig lecture ESC 2014. Eur Heart J. (2015) 36(47):3320–31. doi: 10.1093/eurheartj/ehv511
11. Lau KW, Ding ZP, Johan A, Lim YL. Midterm angiographic outcome of single-vessel intracoronary stent placement in diabetic vs. nondiabetic patients: a matched comparative study. Am Heart J. (1998) 136(1):150–5. doi: 10.1016/S0002-8703(98)70195-4
12. Abizaid A, Kornowski R, Mintz GS, Hong MK, Abizaid AS, Mehran R, et al. The influence of diabetes mellitus on acute and late clinical outcomes following coronary stent implantation. J Am Coll Cardiol. (1998) 32(3):584–9. doi: 10.1016/S0735-1097(98)00286-1
13. Kim YH, Her AY, Jeong MH, Kim BK, Hong SJ, Kim S, et al. Effects of stent generation on clinical outcomes after acute myocardial infarction compared between prediabetes and diabetes patients. Sci Rep. (2021) 11(1):9364. doi: 10.1038/s41598-021-88593-x
14. Vasilakou D, Karagiannis T, Athanasiadou E, Mainou M, Liakos A, Bekiari E, et al. Sodium-glucose cotransporter 2 inhibitors for type 2 diabetes: a systematic review and meta-analysis. Ann Intern Med. (2013) 159(4):262–74. doi: 10.7326/0003-4819-159-4-201308200-00007
15. Connelly K, Cai S. Softening the stiff heart: SGLT2 inhibition and diastolic function. JACC Cardiovasc Imaging. (2021) 14(2):408–10. doi: 10.1016/j.jcmg.2020.08.020
16. Packer M. SGLT2 inhibitors: role in protective reprogramming of cardiac nutrient transport and metabolism. Nat Rev Cardiol. (2023) 20(7):443–62. doi: 10.1038/s41569-022-00824-4
17. McMurray JJV, Solomon SD, Inzucchi SE, Kober L, Kosiborod MN, Martinez FA, et al. Dapagliflozin in patients with heart failure and reduced ejection fraction. N Engl J Med. (2019) 381(21):1995–2008. doi: 10.1056/NEJMoa1911303
18. Youssef ME, Yahya G, Popoviciu MS, Cavalu S, Abd-Eldayem MA, Saber S. Unlocking the full potential of SGLT2 inhibitors: expanding applications beyond glycemic control. Int J Mol Sci. (2023) 24(7):6039. doi: 10.3390/ijms24076039
19. Yao D, Wang S, Wang M, Lu W. Renoprotection of dapagliflozin in human renal proximal tubular cells via the inhibition of the high mobility group box 1-receptor for advanced glycation end products-nuclear factor-kappaB signaling pathway. Mol Med Rep. (2018) 18(4):3625–30. doi: 10.3892/mmr.2018.9393
20. Xu Y, Zhang C, Jiang K, Yang X, Chen F, Cheng Z, et al. Structural repurposing of SGLT2 inhibitor empagliflozin for strengthening anti-heart failure activity with lower glycosuria. Acta Pharm Sin B. (2023) 13(4):1671–85. doi: 10.1016/j.apsb.2022.08.023
21. Shimizu W, Kubota Y, Hoshika Y, Mozawa K, Tara S, Tokita Y, et al. Effects of empagliflozin vs. placebo on cardiac sympathetic activity in acute myocardial infarction patients with type 2 diabetes mellitus: the EMBODY trial. Cardiovasc Diabetol. (2020) 19(1):148. doi: 10.1186/s12933-020-01127-z
22. Herat LY, Matthews J, Azzam O, Schlaich MP, Matthews VB. Targeting features of the metabolic syndrome through sympatholytic effects of SGLT2 inhibition. Curr Hypertens Rep. (2022) 24(3):67–74. doi: 10.1007/s11906-022-01170-z
23. Liu Z, Liu L, Zhang H, Jiang Y, Wang H. Preventive effect observation of dapagliflozin on middle and later ventricular remodeling in patients with acute ST segment elevation anterior wall myocardial infarction: a single-center, retrospective cohort study. J Healthc Eng. (2022) 2022:3955914. doi: 10.1155/2022/3955914
24. McGuire DK, Shih WJ, Cosentino F, Charbonnel B, Cherney DZI, Dagogo-Jack S, et al. Association of SGLT2 inhibitors with cardiovascular and kidney outcomes in patients with type 2 diabetes: a meta-analysis. JAMA Cardiol. (2021) 6(2):148–58. doi: 10.1001/jamacardio.2020.4511
25. Marx N, Federici M, Schutt K, Muller-Wieland D, Ajjan RA, Antunes MJ, et al. 2023 ESC guidelines for the management of cardiovascular disease in patients with diabetes. Eur Heart J. (2023) 44(39):4043–140. doi: 10.1093/eurheartj/ehad192
26. Hua R, Ding N, Guo H, Wu Y, Yuan Z, Li T. Contrast-induced acute kidney injury in patients on SGLT2 inhibitors undergoing percutaneous coronary interventions: a propensity-matched analysis. Front Cardiovasc Med. (2022) 9:918167. doi: 10.3389/fcvm.2022.918167
27. Feitosa MPM, Lima EG, Abizaid AAC, Mehran R, Lopes NHM, de Assis Fischer Ramos T, et al. The safety of SGLT-2 inhibitors in diabetic patients submitted to elective percutaneous coronary intervention regarding kidney function: SAFE-PCI pilot study. Diabetol Metab Syndr. (2023) 15(1):138. doi: 10.1186/s13098-023-01107-9
28. Marfella R, Sardu C, D’Onofrio N, Fumagalli C, Scisciola L, Sasso FC, et al. SGLT-2 inhibitors and in-stent restenosis-related events after acute myocardial infarction: an observational study in patients with type 2 diabetes. BMC Med. (2023) 21(1):71. doi: 10.1186/s12916-023-02781-2
29. Kosiborod M, Birkeland KI, Cavender MA, Fu AZ, Wilding JP, Khunti K, et al. Rates of myocardial infarction and stroke in patients initiating treatment with SGLT2-inhibitors vs. other glucose-lowering agents in real-world clinical practice: results from the CVD-REAL study. Diabetes Obes Metab. (2018) 20(8):1983–7. doi: 10.1111/dom.13299
30. Chen J, Williams S, Ho S, Loraine H, Hagan D, Whaley JM, et al. Quantitative PCR tissue expression profiling of the human SGLT2 gene and related family members. Diabetes Ther. (2010) 1(2):57–92. doi: 10.1007/s13300-010-0006-4
31. Dutzmann J, Bode LM, Kalies K, Korte L, Knopp K, Kloss FJ, et al. Empagliflozin prevents neointima formation by impairing smooth muscle cell proliferation and accelerating endothelial regeneration. Front Cardiovasc Med. (2022) 9:956041. doi: 10.3389/fcvm.2022.956041
32. Sukhanov S, Higashi Y, Yoshida T, Mummidi S, Aroor AR, Jeffrey Russell J, et al. The SGLT2 inhibitor empagliflozin attenuates interleukin-17A-induced human aortic smooth muscle cell proliferation and migration by targeting TRAF3IP2/ROS/NLRP3/caspase-1-dependent IL-1beta and IL-18 secretion. Cell Signal. (2021) 77:109825. doi: 10.1016/j.cellsig.2020.109825
33. Heidenreich PA, Bozkurt B, Aguilar D, Allen LA, Byun JJ, Colvin MM, et al. 2022 AHA/ACC/HFSA guideline for the management of heart failure: a report of the American college of cardiology/American heart association joint committee on clinical practice guidelines. Circulation. (2022) 145(18):e895–1032. doi: 10.1161/CIR.0000000000001063
34. McDonagh TA, Metra M, Adamo M, Gardner RS, Baumbach A, Bohm M, et al. 2023 focused update of the 2021 ESC guidelines for the diagnosis and treatment of acute and chronic heart failure. Eur Heart J. (2023) 44(37):3627–39. doi: 10.1093/eurheartj/ehad195
35. Birkeland KI, Jorgensen ME, Carstensen B, Persson F, Gulseth HL, Thuresson M, et al. Cardiovascular mortality and morbidity in patients with type 2 diabetes following initiation of sodium-glucose co-transporter-2 inhibitors vs. other glucose-lowering drugs (CVD-REAL Nordic): a multinational observational analysis. Lancet Diabetes Endocrinol. (2017) 5(9):709–17. doi: 10.1016/S2213-8587(17)30258-9
36. Sharma A, Ofstad AP, Ahmad T, Zinman B, Zwiener I, Fitchett D, et al. Patient phenotypes and SGLT-2 inhibition in type 2 diabetes: insights from the EMPA-REG OUTCOME trial. JACC Heart Fail. (2021) 9(8):568–77. doi: 10.1016/j.jchf.2021.03.003
37. Zinman B, Wanner C, Lachin JM, Fitchett D, Bluhmki E, Hantel S, et al. Empagliflozin, cardiovascular outcomes, and mortality in type 2 diabetes. N Engl J Med. (2015) 373(22):2117–28. doi: 10.1056/NEJMoa1504720
38. Inzucchi SE, Zinman B, Fitchett D, Wanner C, Ferrannini E, Schumacher M, et al. How does empagliflozin reduce cardiovascular mortality? Insights from a mediation analysis of the EMPA-REG OUTCOME trial. Diabetes Care. (2018) 41(2):356–63. doi: 10.2337/dc17-1096
39. Sarraju A, Bakris G, Cannon CP, Cherney D, Damaraju CV, Figtree GA, et al. Cardiovascular effects of canagliflozin in relation to renal function and albuminuria. J Am Coll Cardiol. (2022) 80(18):1721–31. doi: 10.1016/j.jacc.2022.08.772
40. Neal B, Perkovic V, Mahaffey KW, de Zeeuw D, Fulcher G, Erondu N, et al. Canagliflozin and cardiovascular and renal events in type 2 diabetes. N Engl J Med. (2017) 377(7):644–57. doi: 10.1056/NEJMoa1611925
41. Lee HF, Chan YH, Chuang C, Li PR, Yeh YH, Hsiao FC, et al. Cardiovascular, renal, and lower limb outcomes in patients with type 2 diabetes after percutaneous coronary intervention and treated with sodium-glucose cotransporter 2 inhibitors vs. dipeptidyl peptidase-4 inhibitors. Eur Heart J Cardiovasc Pharmacother. (2023) 9(4):301–10. doi: 10.1093/ehjcvp/pvad004
42. Zhu Y, Zhang JL, Yan XJ, Sun L, Ji Y, Wang FF. Effect of dapagliflozin on the prognosis of patients with acute myocardial infarction undergoing percutaneous coronary intervention. Cardiovasc Diabetol. (2022) 21(1):186. doi: 10.1186/s12933-022-01627-0
43. Paolisso P, Bergamaschi L, Santulli G, Gallinoro E, Cesaro A, Gragnano F, et al. Infarct size, inflammatory burden, and admission hyperglycemia in diabetic patients with acute myocardial infarction treated with SGLT2-inhibitors: a multicenter international registry. Cardiovasc Diabetol. (2022) 21(1):77. doi: 10.1186/s12933-022-01506-8
44. Paolisso P, Bergamaschi L, Gragnano F, Gallinoro E, Cesaro A, Sardu C, et al. Outcomes in diabetic patients treated with SGLT2-inhibitors with acute myocardial infarction undergoing PCI: the SGLT2-I AMI PROTECT registry. Pharmacol Res. (2023) 187:106597. doi: 10.1016/j.phrs.2022.106597
45. von Lewinski D, Kolesnik E, Tripolt NJ, Pferschy PN, Benedikt M, Wallner M, et al. Empagliflozin in acute myocardial infarction: the EMMY trial. Eur Heart J. (2022) 43(41):4421–32. doi: 10.1093/eurheartj/ehac494
46. Harrington J, Udell JA, Jones WS, Anker SD, Bhatt DL, Petrie MC, et al. Empagliflozin in patients post myocardial infarction rationale and design of the EMPACT-MI trial. Am Heart J. (2022) 253:86–98. doi: 10.1016/j.ahj.2022.05.010
47. James S, Erlinge D, Storey RF, McGuire DK, de Belder M, Bjorkgren I, et al. Rationale and design of the DAPA-MI trial: dapagliflozin in patients without diabetes mellitus with acute myocardial infarction. Am Heart J. (2023) 266:188–97. doi: 10.1016/j.ahj.2023.08.008
48. Verma S, Mazer CD, Fitchett D, Inzucchi SE, Pfarr E, George JT, et al. Empagliflozin reduces cardiovascular events, mortality and renal events in participants with type 2 diabetes after coronary artery bypass graft surgery: subanalysis of the EMPA-REG OUTCOME(R) randomised trial. Diabetologia. (2018) 61(8):1712–23. doi: 10.1007/s00125-018-4644-9
49. Lin Y, Zhou F, Wang X, Guo Y, Chen W. Effect of dapagliflozin on clinical outcome after drug-eluting stent implantation in elderly T2DM patients: a real-world study. Comput Math Methods Med. (2023) 2023:8441396. doi: 10.1093/eurheartj/ehac494
50. Van Belle E, Abolmaali K, Bauters C, McFadden EP, Lablanche JM, Bertrand ME. Restenosis, late vessel occlusion and left ventricular function six months after balloon angioplasty in diabetic patients. J Am Coll Cardiol. (1999) 34(2):476–85. doi: 10.1016/S0735-1097(99)00202-8
51. Niccoli G, Giubilato S, Di Vito L, Leo A, Cosentino N, Pitocco D, et al. Severity of coronary atherosclerosis in patients with a first acute coronary event: a diabetes paradox. Eur Heart J. (2013) 34(10):729–41. doi: 10.1093/eurheartj/ehs393
52. Lexis CP, Rahel BM, Meeder JG, Zijlstra F, van der Horst IC. The role of glucose lowering agents on restenosis after percutaneous coronary intervention in patients with diabetes mellitus. Cardiovasc Diabetol. (2009) 8:41. doi: 10.1186/1475-2840-8-41
53. Moreno PR, Fuster V. New aspects in the pathogenesis of diabetic atherothrombosis. J Am Coll Cardiol. (2004) 44(12):2293–300. doi: 10.1016/j.jacc.2004.07.060
54. Nicholls SJ, Tuzcu EM, Kalidindi S, Wolski K, Moon KW, Sipahi I, et al. Effect of diabetes on progression of coronary atherosclerosis and arterial remodeling: a pooled analysis of 5 intravascular ultrasound trials. J Am Coll Cardiol. (2008) 52(4):255–62. doi: 10.1016/j.jacc.2008.03.051
55. Berg DD, Moura FA, Bellavia A, Scirica BM, Wiviott SD, Bhatt DL, et al. Assessment of atherothrombotic risk in patients with type 2 diabetes Mellitus. J Am Coll Cardiol. (2023) 81(25):2391–402. doi: 10.1016/j.jacc.2023.04.031
56. Faries PL, Rohan DI, Takahara H, Wyers MC, Contreras MA, Quist WC, et al. Human vascular smooth muscle cells of diabetic origin exhibit increased proliferation, adhesion, and migration. J Vasc Surg. (2001) 33(3):601–7. doi: 10.1067/mva.2001.111806
57. Moreno PR, Murcia AM, Palacios IF, Leon MN, Bernardi VH, Fuster V, et al. Coronary composition and macrophage infiltration in atherectomy specimens from patients with diabetes mellitus. Circulation. (2000) 102(18):2180–4. doi: 10.1161/01.CIR.102.18.2180
58. Saberzadeh-Ardestani B, Karamzadeh R, Basiri M, Hajizadeh-Saffar E, Farhadi A, Shapiro AMJ, et al. Type 1 diabetes mellitus: cellular and molecular pathophysiology at A glance. Cell J. (2018) 20(3):294–301. doi: 10.22074/cellj.2018.5513
59. Paneni F, Beckman JA, Creager MA, Cosentino F. Diabetes and vascular disease: pathophysiology, clinical consequences, and medical therapy: part I. Eur Heart J. (2013) 34(31):2436–43. doi: 10.1093/eurheartj/eht149
60. Naudi A, Jove M, Ayala V, Cassanye A, Serrano J, Gonzalo H, et al. Cellular dysfunction in diabetes as maladaptive response to mitochondrial oxidative stress. Exp Diabetes Res. (2012) 2012:696215. doi: 10.1155/2012/696215
61. Beckman JA, Creager MA, Libby P. Diabetes and atherosclerosis: epidemiology, pathophysiology, and management. JAMA. (2002) 287(19):2570–81. doi: 10.1001/jama.287.19.2570
62. Salvatore T, Caturano A, Galiero R, Di Martino A, Albanese G, Vetrano E, et al. Cardiovascular benefits from gliflozins: effects on endothelial function. Biomedicines. (2021) 9(10):1356. doi: 10.3390/biomedicines9101356
63. D'Onofrio N, Sardu C, Trotta MC, Scisciola L, Turriziani F, Ferraraccio F, et al. Sodium-glucose co-transporter2 expression and inflammatory activity in diabetic atherosclerotic plaques: effects of sodium-glucose co-transporter2 inhibitor treatment. Mol Metab. (2021) 54:101337. doi: 10.1016/j.molmet.2021.101337
64. Joubert M, Jagu B, Montaigne D, Marechal X, Tesse A, Ayer A, et al. The sodium-glucose cotransporter 2 inhibitor dapagliflozin prevents cardiomyopathy in a diabetic lipodystrophic mouse model. Diabetes. (2017) 66(4):1030–40. doi: 10.2337/db16-0733
65. Trombara F, Cosentino N, Bonomi A, Ludergnani M, Poggio P, Gionti L, et al. Impact of chronic GLP-1 RA and SGLT-2I therapy on in-hospital outcome of diabetic patients with acute myocardial infarction. Cardiovasc Diabetol. (2023) 22(1):26. doi: 10.1186/s12933-023-01758-y
66. Twarda-Clapa A, Olczak A, Bialkowska AM, Koziolkiewicz M. Advanced glycation end-products (AGEs): formation, chemistry, classification, receptors, and diseases related to AGEs. Cells. (2022) 11(8):1312. doi: 10.3390/cells11081312
67. Qiu HY, Hou NN, Shi JF, Liu YP, Kan CX, Han F, et al. Comprehensive overview of human serum albumin glycation in diabetes mellitus. World J Diabetes. (2021) 12(7):1057–69. doi: 10.4239/wjd.v12.i7.1057
68. Nakamura K, Yamagishi S, Adachi H, Matsui T, Kurita-Nakamura Y, Takeuchi M, et al. Serum levels of soluble form of receptor for advanced glycation end products (sRAGE) are positively associated with circulating AGEs and soluble form of VCAM-1 in patients with type 2 diabetes. Microvasc Res. (2008) 76(1):52–6. doi: 10.1016/j.mvr.2007.09.004
69. Wautier MP, Chappey O, Corda S, Stern DM, Schmidt AM, Wautier JL. Activation of NADPH oxidase by AGE links oxidant stress to altered gene expression via RAGE. Am J Physiol Endocrinol Metab. (2001) 280(5):E685–94. doi: 10.1152/ajpendo.2001.280.5.E685
70. Aronson D, Bloomgarden Z, Rayfield EJ. Potential mechanisms promoting restenosis in diabetic patients. J Am Coll Cardiol. (1996) 27(3):528–35. doi: 10.1016/0735-1097(95)00496-3
71. Sun H, Chen J, Hua Y, Zhang Y, Liu Z. New insights into the role of empagliflozin on diabetic renal tubular lipid accumulation. Diabetol Metab Syndr. (2022) 14(1):121. doi: 10.1186/s13098-022-00886-x
72. Ojima A, Matsui T, Nishino Y, Nakamura N, Yamagishi S. Empagliflozin, an inhibitor of sodium-glucose cotransporter 2 exerts anti-inflammatory and antifibrotic effects on experimental diabetic nephropathy partly by suppressing AGEs-receptor axis. Horm Metab Res. (2015) 47(9):686–92. doi: 10.1055/s-0034-1395609
73. Yang L, Liang B, Li J, Zhang X, Chen H, Sun J, et al. Dapagliflozin alleviates advanced glycation end product induced podocyte injury through AMPK/mTOR mediated autophagy pathway. Cell Signal. (2022) 90:110206. doi: 10.1016/j.cellsig.2021.110206
74. Gruzdeva O, Uchasova E, Dyleva Y, Belik E, Shurygina E, Barbarash O. Insulin resistance and inflammation markers in myocardial infarction. J Inflamm Res. (2013) 6:83–90. doi: 10.2147/JIR.S43081
75. Liu C, Zhao Q, Zhao Z, Ma X, Xia Y, Sun Y, et al. Correlation between estimated glucose disposal rate and in-stent restenosis following percutaneous coronary intervention in individuals with non-ST-segment elevation acute coronary syndrome. Front Endocrinol (Lausanne). (2022) 13:1033354. doi: 10.3389/fendo.2022.1033354
76. King GL. The role of hyperglycaemia and hyperinsulinaemia in causing vascular dysfunction in diabetes. Ann Med. (1996) 28(5):427–32. doi: 10.3109/07853899608999103
77. Kasem SM, Saied GM, Hegazy ANM, Abdelsabour M. Impact of acute insulin resistance on myocardial blush in non-diabetic patients undergoing primary percutaneous coronary intervention. Front Cardiovasc Med. (2021) 8:647366. doi: 10.3389/fcvm.2021.647366
78. Zhao LP, Xu WT, Wang L, Li H, Shao CL, Gu HB, et al. Influence of insulin resistance on in-stent restenosis in patients undergoing coronary drug-eluting stent implantation after long-term angiographic follow-up. Coron Artery Dis. (2015) 26(1):5–10. doi: 10.1097/MCA.0000000000000170
79. On YK, Park HK, Hyon MS, Jeon ES. Serum resistin as a biological marker for coronary artery disease and restenosis in type 2 diabetic patients. Circ J. (2007) 71(6):868–73. doi: 10.1253/circj.71.868
80. Piatti P, Di Mario C, Monti LD, Fragasso G, Sgura F, Caumo A, et al. Association of insulin resistance, hyperleptinemia, and impaired nitric oxide release with in-stent restenosis in patients undergoing coronary stenting. Circulation. (2003) 108(17):2074–81. doi: 10.1161/01.CIR.0000095272.67948.17
81. Ahmed B, Sultana R, Greene MW. Adipose tissue and insulin resistance in obese. Biomed Pharmacother. (2021) 137:111315. doi: 10.1016/j.biopha.2021.111315
82. da Silva Rosa SC, Nayak N, Caymo AM, Gordon JW. Mechanisms of muscle insulin resistance and the cross-talk with liver and adipose tissue. Physiol Rep. (2020) 8(19):e14607. doi: 10.14814/phy2.14607
83. Xu B, Li S, Kang B, Zhou J. The current role of sodium-glucose cotransporter 2 inhibitors in type 2 diabetes mellitus management. Cardiovasc Diabetol. (2022) 21(1):83. doi: 10.1186/s12933-022-01512-w
84. Wang X, Ni J, Guo R, Li L, Su J, He F, et al. SGLT2 inhibitors break the vicious circle between heart failure and insulin resistance: targeting energy metabolism. Heart Fail Rev. (2022) 27(3):961–80. doi: 10.1007/s10741-021-10096-8
85. Kullmann S, Hummel J, Wagner R, Dannecker C, Vosseler A, Fritsche L, et al. Empagliflozin improves insulin sensitivity of the hypothalamus in humans with prediabetes: a randomized, double-blind, placebo-controlled, phase 2 trial. Diabetes Care. (2022) 45(2):398–406. doi: 10.2337/dc21-1136
86. Xu L, Ota T. Emerging roles of SGLT2 inhibitors in obesity and insulin resistance: focus on fat browning and macrophage polarization. Adipocyte. (2018) 7(2):121–8. doi: 10.1080/21623945
87. Nishitani S, Fukuhara A, Shin J, Okuno Y, Otsuki M, Shimomura I. Metabolomic and microarray analyses of adipose tissue of dapagliflozin-treated mice, and effects of 3-hydroxybutyrate on induction of adiponectin in adipocytes. Sci Rep. (2018) 8(1):8805. doi: 10.1038/s41598-018-27181-y
88. Bonnet F, Scheen AJ. Effects of SGLT2 inhibitors on systemic and tissue low-grade inflammation: the potential contribution to diabetes complications and cardiovascular disease. Diabetes Metab. (2018) 44(6):457–64. doi: 10.1016/j.diabet.2018.09.005
89. Yaribeygi H, Simental-Mendia LE, Barreto GE, Sahebkar A. Metabolic effects of antidiabetic drugs on adipocytes and adipokine expression. J Cell Physiol. (2019) 234(10):16987–97. doi: 10.1002/jcp.28420
90. Xu L, Nagata N, Nagashimada M, Zhuge F, Ni Y, Chen G, et al. SGLT2 inhibition by empagliflozin promotes fat utilization and browning and attenuates inflammation and insulin resistance by polarizing M2 macrophages in diet-induced obese mice. EBioMedicine. (2017) 20:137–49. doi: 10.1016/j.ebiom.2017.05.028
91. Diaz-Flores L, Gutierrez R, Garcia MP, Gonzalez-Gomez M, Diaz-Flores L Jr, Gayoso S, et al. Ultrastructural study of platelet behavior and interrelationship in sprouting and intussusceptive angiogenesis during arterial intimal thickening formation. Int J Mol Sci. (2021) 22(23):13001. doi: 10.3390/ijms222313001
92. Farb A, Shroff S, John M, Sweet W, Virmani R. Late arterial responses (6 and 12 months) after (32)P beta-emitting stent placement: sustained intimal suppression with incomplete healing. Circulation. (2001) 103(14):1912–9. doi: 10.1161/01.CIR.103.14.1912
93. Mause SF, Ritzel E, Deck A, Vogt F, Liehn EA. Endothelial progenitor cells modulate the phenotype of smooth muscle cells and increase their neointimal accumulation following vascular injury. Thromb Haemost. (2022) 122(3):456–69. doi: 10.1055/s-0041-1731663
94. McDonald RA, Hata A, MacLean MR, Morrell NW, Baker AH. MicroRNA and vascular remodelling in acute vascular injury and pulmonary vascular remodelling. Cardiovasc Res. (2012) 93(4):594–604. doi: 10.1093/cvr/cvr299
95. Oelze M, Kroller-Schon S, Welschof P, Jansen T, Hausding M, Mikhed Y, et al. The sodium-glucose co-transporter 2 inhibitor empagliflozin improves diabetes-induced vascular dysfunction in the streptozotocin diabetes rat model by interfering with oxidative stress and glucotoxicity. PLoS One. (2014) 9(11):e112394. doi: 10.1371/journal.pone.0112394
96. Shigiyama F, Kumashiro N, Miyagi M, Ikehara K, Kanda E, Uchino H, et al. Effectiveness of dapagliflozin on vascular endothelial function and glycemic control in patients with early-stage type 2 diabetes mellitus: DEFENCE study. Cardiovasc Diabetol. (2017) 16(1):84. doi: 10.1186/s12933-017-0564-0
97. Sawada T, Uzu K, Hashimoto N, Onishi T, Takaya T, Shimane A, et al. Empagliflozin’s ameliorating effect on plasma triglycerides: association with endothelial function recovery in diabetic patients with coronary artery disease. J Atheroscler Thromb. (2020) 27(7):644–56. doi: 10.5551/jat.50807
98. Hashikata T, Ikutomi M, Jimba T, Shindo A, Kakuda N, Katsushika S, et al. Empagliflozin attenuates neointimal hyperplasia after drug-eluting-stent implantation in patients with type 2 diabetes. Heart Vessels. (2020) 35(10):1378–89. doi: 10.1007/s00380-020-01621-0
99. Berezin AA, Fushtey IM, Berezin AE. The effect of SGLT2 inhibitor dapagliflozin on serum levels of apelin in T2DM patients with heart failure. Biomedicines. (2022) 10(7):1751. doi: 10.3390/biomedicines10071751
100. Kasai A, Shintani N, Oda M, Kakuda M, Hashimoto H, Matsuda T, et al. Apelin is a novel angiogenic factor in retinal endothelial cells. Biochem Biophys Res Commun. (2004) 325(2):395–400. doi: 10.1016/j.bbrc.2004.10.042
101. Zhang P, Wang AP, Yang HP, Ai L, Zhang HJ, Wang YM, et al. Apelin-13 attenuates high glucose-induced calcification of MOVAS cells by regulating MAPKs and PI3K/AKT pathways and ROS-mediated signals. Biomed Pharmacother. (2020) 128:110271. doi: 10.1016/j.biopha.2020.110271
102. Xu J, Zou MH. Molecular insights and therapeutic targets for diabetic endothelial dysfunction. Circulation. (2009) 120(13):1266–86. doi: 10.1161/CIRCULATIONAHA.108.835223
103. Heiss C, Rodriguez-Mateos A, Kelm M. Central role of eNOS in the maintenance of endothelial homeostasis. Antioxid Redox Signal. (2015) 22(14):1230–42. doi: 10.1089/ars.2014.6158
104. Yao SK, Ober JC, Krishnaswami A, Ferguson JJ, Anderson HV, Golino P, et al. Endogenous nitric oxide protects against platelet aggregation and cyclic flow variations in stenosed and endothelium-injured arteries. Circulation. (1992) 86(4):1302–9. doi: 10.1161/01.CIR.86.4.1302
105. Durante W, Behnammanesh G, Peyton KJ. Effects of sodium-glucose co-transporter 2 inhibitors on vascular cell function and arterial remodeling. Int J Mol Sci. (2021) 22(16):8786. doi: 10.3390/ijms22168786
106. Myers PR, Webel R, Thondapu V, Xu XP, Amann J, Tanner MA, et al. Restenosis is associated with decreased coronary artery nitric oxide synthase. Int J Cardiol. (1996) 55(2):183–91. doi: 10.1016/0167-5273(96)02684-8
107. Wu TC, Chen YH, Chen JW, Chen LC, Lin SJ, Ding PY, et al. Impaired forearm reactive hyperemia is related to late restenosis after coronary stenting. Am J Cardiol. (2000) 85(9):1071–6. doi: 10.1016/S0002-9149(00)00698-6
108. Aroor AR, Das NA, Carpenter AJ, Habibi J, Jia G, Ramirez-Perez FI, et al. Glycemic control by the SGLT2 inhibitor empagliflozin decreases aortic stiffness, renal resistivity index and kidney injury. Cardiovasc Diabetol. (2018) 17(1):108. doi: 10.1186/s12933-018-0750-8
109. Soares RN, Ramirez-Perez FI, Cabral-Amador FJ, Morales-Quinones M, Foote CA, Ghiarone T, et al. SGLT2 inhibition attenuates arterial dysfunction and decreases vascular F-actin content and expression of proteins associated with oxidative stress in aged mice. Geroscience. (2022) 44(3):1657–75. doi: 10.1007/s11357-022-00563-x
110. Irace C, Cutruzzola A, Parise M, Fiorentino R, Frazzetto M, Gnasso C, et al. Effect of empagliflozin on brachial artery shear stress and endothelial function in subjects with type 2 diabetes: results from an exploratory study. Diab Vasc Dis Res. (2020) 17(1):1479164119883540. doi: 10.1177/1479164119883540
111. Salim HM, Fukuda D, Yagi S, Soeki T, Shimabukuro M, Sata M. Glycemic control with ipragliflozin, a novel selective SGLT2 inhibitor, ameliorated endothelial dysfunction in streptozotocin-induced diabetic mouse. Front Cardiovasc Med. (2016) 3:43. doi: 10.3389/fcvm.2016.00043
112. Taguchi I, Yoneda S, Abe S, Toyoda S, Nasuno T, Nishino S, et al. The late-phase inflammatory response after drug-eluting stent implantation. Heart Vessels. (2014) 29(2):213–9. doi: 10.1007/s00380-013-0357-7
113. Joviliano EE, Piccinato CE, Dellalibera-Joviliano R, Moriya T, Evora PR. Inflammatory markers and restenosis in peripheral percutaneous angioplasty with intravascular stenting: current concepts. Ann Vasc Surg. (2011) 25(6):846–55. doi: 10.1016/j.avsg.2011.02.026
114. Zhou QN, Lin WH, Jing R, Liu JJ, Shi HY, Yang RF, et al. The predictive value of epicardial adipose tissue and inflammatory factors for in-stent restenosis. Zhonghua Yi Xue Za Zhi. (2019) 99(47):3732–6. doi: 10.3760/cma.j.issn.0376-2491.2019.47.011
115. Lee SG, Lee SJ, Lee JJ, Kim JS, Lee OH, Kim CK, et al. Anti-inflammatory effect for atherosclerosis progression by sodium-glucose cotransporter 2 (SGLT-2) inhibitor in a normoglycemic rabbit model. Korean Circ J. (2020) 50(5):443–57. doi: 10.4070/kcj.2019.0296
116. Lahnwong S, Palee S, Apaijai N, Sriwichaiin S, Kerdphoo S, Jaiwongkam T, et al. Acute dapagliflozin administration exerts cardioprotective effects in rats with cardiac ischemia/reperfusion injury. Cardiovasc Diabetol. (2020) 19(1):91. doi: 10.1186/s12933-020-01066-9
117. Garcia-Redondo AB, Aguado A, Briones AM, Salaices M. NADPH oxidases and vascular remodeling in cardiovascular diseases. Pharmacol Res. (2016) 114:110–20. doi: 10.1016/j.phrs.2016.10.015
118. Spadaccio C, Patti G, De Marco F, Coccia R, Di Domenico F, Pollari F, et al. Usefulness of preprocedural levels of advanced glycation end products to predict restenosis in patients with controlled diabetes mellitus undergoing drug-eluting stent implantation for stable angina pectoris (from the prospective ARMYDA-AGEs study). Am J Cardiol. (2013) 112(1):21–6. doi: 10.1016/j.amjcard.2013.02.046
119. Joubert M, Manrique A, Cariou B, Prieur X. Diabetes-related cardiomyopathy: the sweet story of glucose overload from epidemiology to cellular pathways. Diabetes Metab. (2019) 45(3):238–47. doi: 10.1016/j.diabet.2018.07.003
120. Uthman L, Homayr A, Juni RP, Spin EL, Kerindongo R, Boomsma M, et al. Empagliflozin and dapagliflozin reduce ROS generation and restore NO bioavailability in tumor necrosis factor alpha-stimulated human coronary arterial endothelial cells. Cell Physiol Biochem. (2019) 53(5):865–86. doi: 10.33594/000000178
121. Li X, Romer G, Kerindongo RP, Hermanides J, Albrecht M, Hollmann MW, et al. Sodium glucose co-transporter 2 inhibitors ameliorate endothelium barrier dysfunction induced by cyclic stretch through inhibition of reactive oxygen species. Int J Mol Sci. (2021) 22(11):6044. doi: 10.3390/ijms22116044
122. Uthman L, Baartscheer A, Bleijlevens B, Schumacher CA, Fiolet JWT, Koeman A, et al. Class effects of SGLT2 inhibitors in mouse cardiomyocytes and hearts: inhibition of na(+)/H(+) exchanger, lowering of cytosolic na(+) and vasodilation. Diabetologia. (2018) 61(3):722–6. doi: 10.1007/s00125-017-4509-7
123. Jiang K, Xu Y, Wang D, Chen F, Tu Z, Qian J, et al. Cardioprotective mechanism of SGLT2 inhibitor against myocardial infarction is through reduction of autosis. Protein Cell. (2022) 13(5):336–59. doi: 10.1007/s13238-020-00809-4
124. Bertero E, Prates Roma L, Ameri P, Maack C. Cardiac effects of SGLT2 inhibitors: the sodium hypothesis. Cardiovasc Res. (2018) 114(1):12–8. doi: 10.1093/cvr/cvx149
125. Lucchesi PA, DeRoux N, Berk BC. Na(+)-H+ exchanger expression in vascular smooth muscle of spontaneously hypertensive and Wistar-Kyoto rats. Hypertension. (1994) 24(6):734–8. doi: 10.1161/01.HYP.24.6.734
126. Ye Y, Jia X, Bajaj M, Birnbaum Y. Dapagliflozin attenuates na(+)/H(+) exchanger-1 in cardiofibroblasts via AMPK activation. Cardiovasc Drugs Ther. (2018) 32(6):553–8. doi: 10.1007/s10557-018-6837-3
127. Quagliariello V, De Laurentiis M, Rea D, Barbieri A, Monti MG, Carbone A, et al. The SGLT-2 inhibitor empagliflozin improves myocardial strain, reduces cardiac fibrosis and pro-inflammatory cytokines in non-diabetic mice treated with doxorubicin. Cardiovasc Diabetol. (2021) 20(1):150. doi: 10.1186/s12933-021-01346-y
128. Yoshida T, Yamashita M, Horimai C, Hayashi M. Smooth muscle-selective inhibition of nuclear factor-kappaB attenuates smooth muscle phenotypic switching and neointima formation following vascular injury. J Am Heart Assoc. (2013) 2(3):e000230. doi: 10.1161/JAHA.113.000230
129. Zhang R, Li T, Guo J, Zhao Y, Liu Y, Yao Y, et al. Fufang-Zhenzhu-Tiaozhi capsule reduces restenosis via the downregulation of NF-kappaB and inflammatory factors in rabbits. Lipids Health Dis. (2018) 17(1):272. doi: 10.1186/s12944-018-0921-3
130. Lee DM, Battson ML, Jarrell DK, Hou S, Ecton KE, Weir TL, et al. SGLT2 inhibition via dapagliflozin improves generalized vascular dysfunction and alters the gut microbiota in type 2 diabetic mice. Cardiovasc Diabetol. (2018) 17(1):62. doi: 10.1186/s12933-018-0708-x
131. Gaspari T, Spizzo I, Liu H, Hu Y, Simpson RW, Widdop RE, et al. Dapagliflozin attenuates human vascular endothelial cell activation and induces vasorelaxation: a potential mechanism for inhibition of atherogenesis. Diab Vasc Dis Res. (2018) 15(1):64–73. doi: 10.1177/1479164117733626
132. Fu J, Xu H, Wu F, Tu Q, Dong X, Xie H, et al. Empagliflozin inhibits macrophage inflammation through AMPK signaling pathway and plays an anti-atherosclerosis role. Int J Cardiol. (2022) 367:56–62. doi: 10.1016/j.ijcard.2022.07.048
133. Shi J, Guo J, Li Z, Xu B, Miyata M. Importance of NLRP3 inflammasome in abdominal aortic aneurysms. J Atheroscler Thromb. (2021) 28(5):454–66. doi: 10.5551/jat.RV17048
134. Liu Y, Wang K, Yang S, Xue G, Lu L. Mulberry extract upregulates cholesterol efflux and inhibits p38 MAPK-NLRP3-mediated inflammation in foam cells. Food Sci Nutr. (2023) 11(6):3141–53. doi: 10.1002/fsn3.3296
135. Yang L, Zhang X, Wang Q. Effects and mechanisms of SGLT2 inhibitors on the NLRP3 inflammasome, with a focus on atherosclerosis. Front Endocrinol (Lausanne). (2022) 13:992937. doi: 10.3389/fendo.2022.992937
136. Leng W, Ouyang X, Lei X, Wu M, Chen L, Wu Q, et al. The SGLT-2 inhibitor dapagliflozin has a therapeutic effect on atherosclerosis in diabetic ApoE(-/-) mice. Mediators Inflamm. (2016) 2016:6305735. doi: 10.1155/2016/6305735
137. Grant AJ, Yang N, Moore MJ, Lam YT, Michael PL, Chan AHP, et al. Selective NLRP3 inflammasome inhibitor MCC950 suppresses inflammation and facilitates healing in vascular materials. Adv Sci (Weinh). (2023) 10:e2300521. doi: 10.1002/advs.202300521
138. Hettwer J, Hinterdobler J, Miritsch B, Deutsch MA, Li X, Mauersberger C, et al. Interleukin-1beta suppression dampens inflammatory leucocyte production and uptake in atherosclerosis. Cardiovasc Res. (2022) 118(13):2778–91. doi: 10.1093/cvr/cvab337
139. Liu Y, Wu M, Xu B, Kang L. Empagliflozin alleviates atherosclerosis progression by inhibiting inflammation and sympathetic activity in a normoglycemic mouse model. J Inflamm Res. (2021) 14:2277–87. doi: 10.2147/JIR.S309427
140. Yu YW, Que JQ, Liu S, Huang KY, Qian L, Weng YB, et al. Sodium-glucose co-transporter-2 inhibitor of dapagliflozin attenuates myocardial ischemia/reperfusion injury by limiting NLRP3 inflammasome activation and modulating autophagy. Front Cardiovasc Med. (2021) 8:768214. doi: 10.3389/fcvm.2021.768214
141. Kim SR, Lee SG, Kim SH, Kim JH, Choi E, Cho W, et al. SGLT2 inhibition modulates NLRP3 inflammasome activity via ketones and insulin in diabetes with cardiovascular disease. Nat Commun. (2020) 11(1):2127. doi: 10.1038/s41467-020-15983-6
142. Wang CCL, Hess CN, Hiatt WR, Goldfine AB. Clinical update: cardiovascular disease in diabetes mellitus: atherosclerotic cardiovascular disease and heart failure in type 2 diabetes mellitus—mechanisms, management, and clinical considerations. Circulation. (2016) 133(24):2459–502. doi: 10.1161/CIRCULATIONAHA.116.022194
143. Kita S, Maeda N, Shimomura I. Interorgan communication by exosomes, adipose tissue, and adiponectin in metabolic syndrome. J Clin Invest. (2019) 129(10):4041–9. doi: 10.1172/JCI129193
144. Ray I, Meira LB, Michael A, Ellis PE. Adipocytokines and disease progression in endometrial cancer: a systematic review. Cancer Metastasis Rev. (2022) 41(1):211–42. doi: 10.1007/s10555-021-10002-6
145. Gleeson M, Bishop NC, Stensel DJ, Lindley MR, Mastana SS, Nimmo MA. The anti-inflammatory effects of exercise: mechanisms and implications for the prevention and treatment of disease. Nat Rev Immunol. (2011) 11(9):607–15. doi: 10.1038/nri3041
146. Zhou J, Zhang J, Li J, Guan Y, Shen T, Li F, et al. Ginsenoside F2 suppresses adipogenesis in 3T3-L1 cells and obesity in mice via the AMPK pathway. J Agric Food Chem. (2021) 69(32):9299–312. doi: 10.1021/acs.jafc.1c03420
147. Li T, Zhang R, Liu Y, Yao Y, Guo J, Zeng Z. Fufang-Zhenzhu-Tiaozhi capsule ameliorates rabbit’s iliac artery restenosis by regulating adiponectin signaling pathway. Biomed Pharmacother. (2020) 128:110311. doi: 10.1016/j.biopha.2020.110311
148. Li TD, Zeng ZH. Adiponectin as a potential therapeutic target for the treatment of restenosis. Biomed Pharmacother. (2018) 101:798–804. doi: 10.1016/j.biopha.2018.03.003
149. Han JH, Oh TJ, Lee G, Maeng HJ, Lee DH, Kim KM, et al. The beneficial effects of empagliflozin, an SGLT2 inhibitor, on atherosclerosis in ApoE (-/-) mice fed a western diet. Diabetologia. (2017) 60(2):364–76. doi: 10.1007/s00125-016-4158-2
150. Hayashi T, Fukui T, Nakanishi N, Yamamoto S, Tomoyasu M, Osamura A, et al. Dapagliflozin decreases small dense low-density lipoprotein-cholesterol and increases high-density lipoprotein 2-cholesterol in patients with type 2 diabetes: comparison with sitagliptin. Cardiovasc Diabetol. (2017) 16(1):8. doi: 10.1186/s12933-016-0491-5
151. Piatti P, Monti LD. Insulin resistance, hyperleptinemia and endothelial dysfunction in coronary restenosis. Curr Opin Pharmacol. (2005) 5(2):160–4. doi: 10.1016/j.coph.2004.10.004
152. Wu P, Wen W, Li J, Xu J, Zhao M, Chen H, et al. Systematic review and meta-analysis of randomized controlled trials on the effect of SGLT2 inhibitor on blood leptin and adiponectin level in patients with type 2 diabetes. Horm Metab Res. (2019) 51(8):487–94. doi: 10.1055/a-0958-2441
153. Zhu Y, Liu K, Chen M, Liu Y, Gao A, Hu C, et al. Triglyceride-glucose index is associated with in-stent restenosis in patients with acute coronary syndrome after percutaneous coronary intervention with drug-eluting stents. Cardiovasc Diabetol. (2021) 20(1):137. doi: 10.1186/s12933-021-01332-4
154. Carnagarin R, Tan K, Adams L, Matthews VB, Kiuchi MG, Marisol Lugo Gavidia L, et al. Metabolic dysfunction-associated fatty liver disease (MAFLD)-a condition associated with heightened sympathetic activation. Int J Mol Sci. (2021) 22(8):4241. doi: 10.3390/ijms22084241
155. Li L, Li B, Li M, Speakman JR. Switching on the furnace: regulation of heat production in brown adipose tissue. Mol Aspects Med. (2019) 68:60–73. doi: 10.1016/j.mam.2019.07.005
156. Goldstein SA, Elwyn DH. The effects of injury and sepsis on fuel utilization. Annu Rev Nutr. (1989) 9:445–73. doi: 10.1146/annurev.nu.09.070189.002305
157. Matthews VB, Elliot RH, Rudnicka C, Hricova J, Herat L, Schlaich MP. Role of the sympathetic nervous system in regulation of the sodium glucose cotransporter 2. J Hypertens. (2017) 35(10):2059–68. doi: 10.1097/HJH.0000000000001434
158. Carnagarin R, Matthews V, Zaldivia MTK, Peter K, Schlaich MP. The bidirectional interaction between the sympathetic nervous system and immune mechanisms in the pathogenesis of hypertension. Br J Pharmacol. (2019) 176(12):1839–52. doi: 10.1111/bph.14481
159. Yang X, Liu Q, Li Y, Ding Y, Zhao Y, Tang Q, et al. Inhibition of the sodium-glucose co-transporter SGLT2 by canagliflozin ameliorates diet-induced obesity by increasing intra-adipose sympathetic innervation. Br J Pharmacol. (2021) 178(8):1756–71. doi: 10.1111/bph.15381
160. McDonagh TA, Metra M, Adamo M, Gardner RS, Baumbach A, Bohm M, et al. 2021 ESC guidelines for the diagnosis and treatment of acute and chronic heart failure. Eur Heart J. (2021) 42(36):3599–726. doi: 10.1093/eurheartj/ehab368
161. Tentolouris A, Vlachakis P, Tzeravini E, Eleftheriadou I, Tentolouris N. SGLT2 inhibitors: a review of their antidiabetic and cardioprotective effects. Int J Environ Res Public Health. (2019) 16(16):2965. doi: 10.3390/ijerph16162965
162. Pollack R, Cahn A. SGLT2 inhibitors and safety in older patients. Heart Fail Clin. (2022) 18(4):635–43. doi: 10.1016/j.hfc.2022.03.002
163. Marilly E, Cottin J, Cabrera N, Cornu C, Boussageon R, Moulin P, et al. SGLT2 inhibitors in type 2 diabetes: a systematic review and meta-analysis of cardiovascular outcome trials balancing their risks and benefits. Diabetologia. (2022) 65(12):2000–10. doi: 10.1007/s00125-022-05773-8
164. Sharma A, Verma S, Bhatt DL, Connelly KA, Swiggum E, Vaduganathan M, et al. Optimizing foundational therapies in patients with HFrEF: how do we translate these findings into clinical care? JACC Basic Transl Sci. (2022) 7(5):504–17. doi: 10.1016/j.jacbts.2021.10.018
165. Bazoukis G, Papadatos SS, Thomopoulos C, Tse G, Cheilidis S, Tsioufis K, et al. Impact of SGLT2 inhibitors on major clinical events and safety outcomes in heart failure patients: a meta-analysis of randomized clinical trials. J Geriatr Cardiol. (2021) 18(10):783–95. doi: 10.11909/j.issn.1671-5411.2021.10.003
166. Voors AA, Angermann CE, Teerlink JR, Collins SP, Kosiborod M, Biegus J, et al. The SGLT2 inhibitor empagliflozin in patients hospitalized for acute heart failure: a multinational randomized trial. Nat Med. (2022) 28(3):568–74. doi: 10.1038/s41591-021-01659-1
167. Dave CV, Schneeweiss S, Kim D, Fralick M, Tong A, Patorno E. Sodium-glucose cotransporter-2 inhibitors and the risk for severe urinary tract infections: a population-based cohort study. Ann Intern Med. (2019) 171(4):248–56. doi: 10.7326/M18-3136
168. Brown E, Heerspink HJL, Cuthbertson DJ, Wilding JPH. SGLT2 inhibitors and GLP-1 receptor agonists: established and emerging indications. Lancet. (2021) 398(10296):262–76. doi: 10.1016/S0140-6736(21)00536-5
169. Mudaliar S, Alloju S, Henry RR. Can a shift in fuel energetics explain the beneficial cardiorenal outcomes in the EMPA-REG OUTCOME study? A unifying hypothesis. Diabetes Care. (2016) 39(7):1115–22. doi: 10.2337/dc16-0542
170. Burke KR, Schumacher CA, Harpe SE. SGLT2 inhibitors: a systematic review of diabetic ketoacidosis and related risk factors in the primary literature. Pharmacotherapy. (2017) 37(2):187–94. doi: 10.1002/phar.1881
171. Heyward J, Mansour O, Olson L, Singh S, Alexander GC. Association between sodium-glucose cotransporter 2 (SGLT2) inhibitors and lower extremity amputation: a systematic review and meta-analysis. PLoS One. (2020) 15(6):e0234065. doi: 10.1371/journal.pone.0234065
172. Peyton KJ, Behnammanesh G, Durante GL, Durante W. Canagliflozin inhibits human endothelial cell inflammation through the induction of heme oxygenase-1. Int J Mol Sci. (2022) 23(15):8777. doi: 10.3390/ijms23158777
Keywords: SGLT2 inhibitor, restenosis, coronary heart disease, AMI—acute myocardial infarction, ASCVD-atherosclerotic cardiovascular disease
Citation: Zhang Q, Deng Z, Li T, Chen K and Zeng Z (2024) SGLT2 inhibitor improves the prognosis of patients with coronary heart disease and prevents in-stent restenosis. Front. Cardiovasc. Med. 10:1280547. doi: 10.3389/fcvm.2023.1280547
Received: 20 August 2023; Accepted: 27 December 2023;
Published: 11 January 2024.
Edited by:
Chieko Mineo, University of Texas Southwestern Medical Center, United StatesReviewed by:
Giulia Ferrannini, Karolinska Institutet (KI), SwedenSusy Kotit, Magdi Yacoub Heart Foundation-Aswan Heart Centre, Egypt
© 2024 Zhang, Deng, Li, Chen and Zeng. This is an open-access article distributed under the terms of the Creative Commons Attribution License (CC BY). The use, distribution or reproduction in other forums is permitted, provided the original author(s) and the copyright owner(s) are credited and that the original publication in this journal is cited, in accordance with accepted academic practice. No use, distribution or reproduction is permitted which does not comply with these terms.
*Correspondence: Zhihuan Zeng gzzh@163.com