- 1Department of Biotherapy, State Key Laboratory of Biotherapy, Cancer Center, West China Hospital, Sichuan University, Chengdu, China
- 2Department of Cardiology, The First Affiliated Hospital of China Medical University, Shenyang, China
Background & aims: Coronavirus disease 2019 (COVID-19) is strongly associated with myocarditis or pericarditis risk in observational studies, however, there are still studies that do not support the above conclusion. Whether the observed association reflects causation needs to be confirmed. We performed a bidirectional Mendelian randomization (MR) study to assess the causal relationship of COVID-19, which was divided into three groups, namely severe COVID-19, hospitalized COVID-19, and COVID-19 infection, measured by myocarditis or pericarditis.
Methods: We extracted summary genome-wide association statistics for the severe COVID-19 (case: 13,769, control: 1,072,442), hospitalized COVID-19 (case: 32,519, control: 2,062,805), COVID-19 infection (case: 122,616, control: 2,475,240), myocarditis (case 1,521, control 191,924), and pericarditis (case 979, control 286,109) among individuals of European ancestry. Independent genetic variants that exhibited a significant association with each phenotype at the genome-wide level of significance were utilized as instrumental variables. Estimation of the causal effect was mainly performed using the random effects inverse-variance weighted method (IVW). Additionally, other tests such as MR-Egger intercept, MR-PRESSO, Cochran's Q-test, “Leave-one-out”, and funnel plots were conducted to assess the extent of pleiotropy and heterogeneity.
Results: Non-associations in the IVW and sensitivity analyses were observed for COVID-19 with myocarditis or pericarditis. Severe COVID-19 was not associated with myocarditis [odds ratio (OR), 1.00; 95% confidence interval (CI), 0.89–1.12; P = 0.99], pericarditis (OR = 0.90, 95% CI, 0.78–1.04, P = 0.17). Similar results can be observed in hospitalized COVID-19, and COVID-19 infection. At the same time, null associations were observed for myocarditis or pericarditis with COVID-19 traits in the reverse direction. The main results are kept stable in the sensitivity analysis.
Conclusion: There is no evidence that COVID-19 is independently and causally associated with myocarditis or pericarditis.
1. Introduction
Coronavirus disease 2019 (COVID-19) is an epidemic disease caused by SARS-CoV-2, which is a sizable enveloped RNA virus, exhibiting approximately 80% sequence similarity with SARS-CoV, and predominantly impacts the respiratory system owing to its main attachment to angiotensin converting enzyme-2 (ACE-2) receptors (1, 2). SARS-CoV-2 can also commonly affect the cardiovascular, brain, kidney, liver, and immune systems, leading to their compromise (3). Myocarditis, pericarditis, acute coronary syndrome, arrhythmias, cardiomyopathy; heart failure, and thromboembolic disease are common cardiovascular damage manifestations of COVID-19 (2, 4).
Myocarditis is an inflammatory disease of the cardiac muscle, mainly diagnosed by histological, immunological, and immunohistochemical criteria (5). Pericarditis the most common form of pericardial disease refers to the inflammation of the pericardial layers (6). The etiology of myocarditis and pericarditis includes infectious and non-infectious forms. Viral infection and postviral immune-mediated responses are the most common causes of myocarditis (5). At the same time, viral infection is also a common cause of pericarditis. Sometimes myocarditis and pericarditis caused by viral infection can coexist (6, 7). According to some reports, myocarditis or pericarditis is a common cardiac complication caused by COVID-19 (8–10). During the COVID-19 pandemic, a study conducted by the Centers for Disease Control and Prevention (CDC) observed that the incidence of myocarditis infected with COVID-19 is 15 times that of non-infected COVID-19 (11). In European and American countries, it is estimated that the annual morbidity of acute pericarditis in the general population is about 27.7 cases per 100,000 people (12, 13). However, in the context of COVID-19 infection, real-world data demonstrate that new-onset pericarditis developed in approximately 1.5% of cases (14). More attention has been paid to myocarditis or pericarditis during the prevalence of COVID-19. Although there is growing evidence suggesting a potential association between COVID-19 and myocarditis and pericarditis, the precise causal relationship has not been definitively established. To date, limited reports with pathological evidence have demonstrated direct myocardial or pericardium invasion by the SARS-CoV-2 virus (15, 16). It is notable that even three years after the COVID-19 epidemic, our understanding of the pathogenesis of myocardial or pericardium injury in relation to COVID-19 remains incomplete. Several experts suggest that systemic inflammation may be the primary cause of myocardial injury, rather than direct viral infection of the heart (4, 17). The potential for long-term evolution into forms of inflammatory cardiomyopathy remains also unclear (18). Therefore, the relationship between COVID-19 and myocarditis and pericarditis still deserves further study, especially new research protocols, such as Mendelian randomization (MR).
Clinical research is susceptible to confounding factors and reverse causation. MR as a new and promising approach uses genetic variants as instrumental variables (IVs) to assess the genetic relationship between two phenotypes while avoiding potential confounding factors and reverse causation (19), which can be used to reveal the genetic sensitivity between COVID-19 and myocarditis or pericarditis. The reverse direction MR analysis was also performed (i.e., evaluating the causal association between myocarditis or pericarditis on COVID-19).
2. Materials and methods
2.1. Study design
In Figure 1, the study design and the three indispensable assumptions of MR are portrayed, which are: (1) a robust association exists between single nucleotide polymorphisms (SNPs) and COVID-19; (2) SNPs are unaffected by any known confounding variables; and (3) the development of myocarditis or pericarditis is exclusively influenced by the association between SNPs and COVID-19. This assumption is also applicable in the reverse direction.
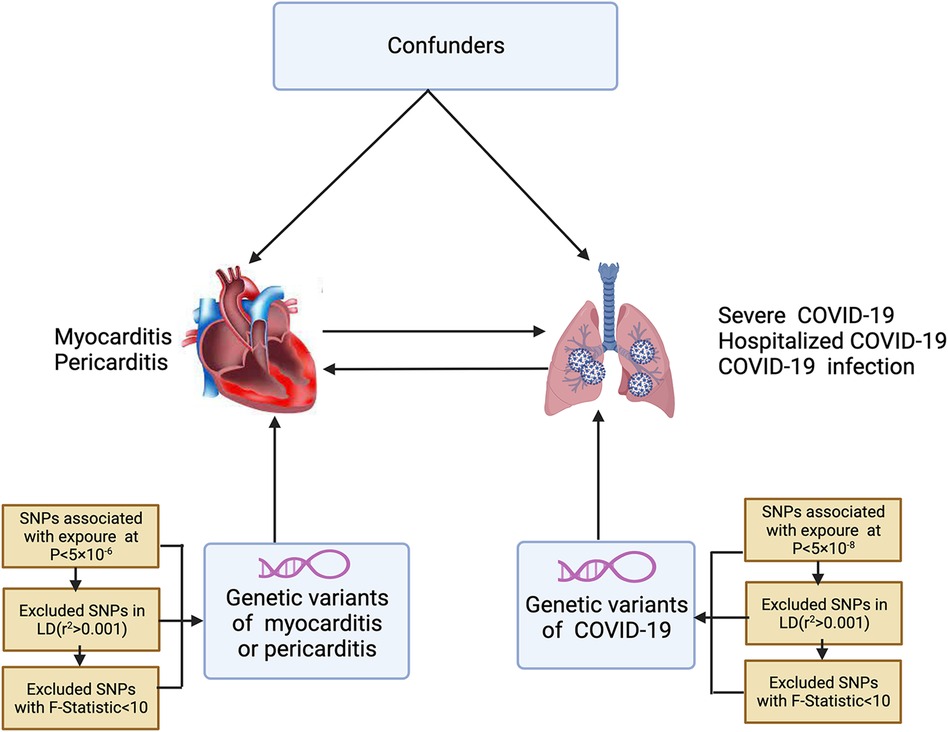
Figure 1. The conceptual framework for the bidirectional Mendelian randomization analysis. SNPs, single nucleotide polymorphisms; LD, linkage disequilibrium.
2.2. Data sources
In this two-sample MR study, the genetic association is the causal relationship between host genetic liability to COVID-19 on myocarditis or pericarditis. The exposures were severe COVID-19 (case: 13,769, control: 1,072,442), hospitalized COVID-19 (case: 32,519, control: 2,062,805), and COVID-19 infection (case: 122,616, control: 2,475,240). The outcomes were myocarditis (case 1,521, control 191,924) or pericarditis (case 979, control 286,109). The reverse MR analysis evaluates the possibility of reverse causal relationships. Myocarditis or pericarditis was treated as exposure, while COVID-19 events were treated as the outcome. Genome-wide association studies (GWAS) summary statistics of COVID-19 were obtained from the COVID-19 Host Genetic Initiative (HGI) (Round 7). Summary statistics of myocarditis or pericarditis were extracted from a GWAS conducted in finngen_R9_I9.
2.3. Selection and validation of SNPs
To identify suitable SNPs, we applied three criteria. First, we selected SNPs associated with COVID-19 at the genome-wide significance threshold with P < 5 × 10−8. In the reverse direction, independent instruments of myocarditis or pericarditis traits (P < 5 × 10−6) identified from the original GWAS were used as instruments. Second, we assessed the independence between selected SNPs using pairwise-linkage disequilibrium with a clumping window of 10,000 kb [22]. Third, the individual SNPs were assessed using the F-statistic to confirm their strength in mitigating potential biases. SNPs with F-statistics greater than ten were considered sufficiently powerful. Before conducting the MR analysis, data-harmonization measures were undertaken to ensure that the SNP's effects on exposure and outcome corresponded to the same allele.
2.4. MR analysis
An inverse-variance weighted (IVW) meta-analysis under a random-effect model was regarded as the primary analysis. MR-Egger, simple mode, weighted mode, and weighted median were used to complement IVW estimates. MR-Pleiotropy Residual Sum and Outlier methods (MR-PRESSO) were used to select abnormal SNPs. The intercept obtained from the MR-Egger regression was an index of directional pleiotropy. MR-PRESSO was also used to assess and correct horizontal pleiotropy (20). Cochrane's Q-value can detect heterogeneity. A leave-one-out sensitivity analysis was used to determine whether a single SNP affected the main causal relationship. Additionally, funnel plots assess whether causal associations are subject to potential bias. All statistical analyses used the “Two Sample MR” packages in R version 023.03.0 + 386.
3. Results
Using GWAS summary statistics, the 2-sample MR analyses did not indicate any causal effect of genetically predicted COVID-19 on the risk of myocarditis or pericarditis in individuals of European descent. The F-values of individual SNP and total SNPs are greater than 10. IVW models indicated that genetically predicted severe COVID-19 was not associated with an increased risk of myocarditis (OR = 1.00, 95% CI, 0.89–1.12; P = 0.99), and pericarditis (OR = 0.90, 95% CI, 0.78–1.04, P = 0.17). Similar results can be observed in hospitalized COVID-19, and COVID-19 infection (Figure 2). At the same time, null associations were observed for myocarditis or pericarditis with COVID-19 traits (Figure 3). IVW models showed thatmyocarditis was not associated with an increased risk of severe COVID-19 (OR = 0.96, 95% CI, 0.91–1.00; P = 0.07), hospitalized COVID-19 (OR = 0.97, 95% CI, 0.94–1.00, P = 1.00), and COVID-infection (OR = 0.99, 95% CI, 0.97–1.00; P = 0.09). Similar results can be observed in pericarditis. No directional pleiotropic effect across the genetic variants was detected in the MR-Egger regression (Tables 1, 2). No horizontal pleiotropic effect across the genetic variants was detected in the MR-PRESSO (Tables 1, 2). The results of the IVW analysis did not suggest the existence of heterogeneity (Tables 1, 2). Associations of each variant with exposure and the risk of outcome are shown in Supplementary Tables S1–S12. The scatter plot for exposure on outcome was shown in Supplementary Figures S1–S12A. A forest plot of exposure on outcome was shown in Supplementary Figures S1–S12B. The leave-one-out sensitivity analysis showed that the association between exposure and outcome was not substantially driven by any individual SNP (Supplementary Figures S1–S12C). The funnel plot was symmetrical around the IVW estimates, revealing that the causal association was less susceptible to potential bias (Supplementary Figures S1–S12D).
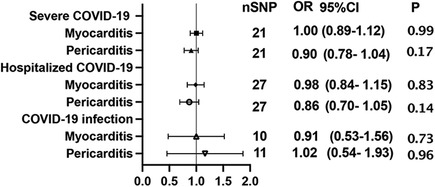
Figure 2. Mendelian randomization estimates the causal effect of COVID-19 on myocarditis or pericarditis. OR, odds ratio; CI, confidence interval.
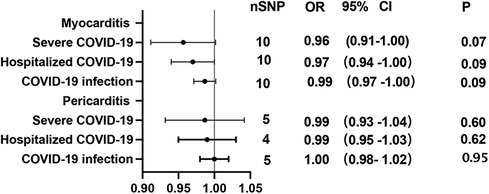
Figure 3. Mendelian randomization in the reverse direction estimates the causal effect of myocarditis or pericarditis on COVID-19. OR, odds ratio; CI, confidence interval.
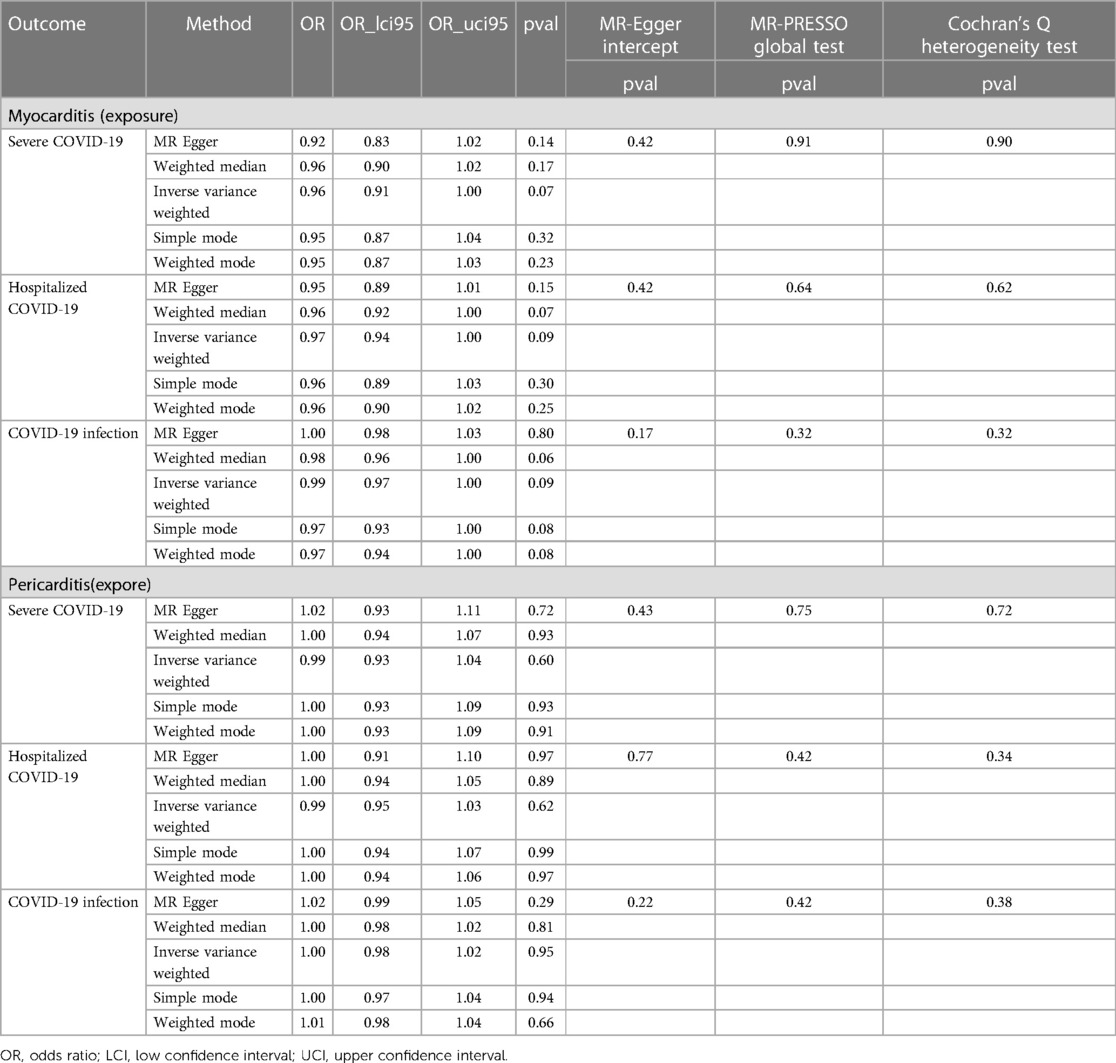
Table 2. The reverse direction MR analysis evaluates the association of myocarditis or pericarditis with COVID-19 traits.
4. Discussion
This study conducted the first-ever 2-sample MR analysis examining the association between COVID-19 and myocarditis or pericarditis utilizing large-scale GWAS data. There is no evidence that COVID-19 is independently and causally associated with myocarditis or pericarditis.
Some studies indicated that during the COVID-19 pandemic, there is a substantial increase in the incidence of myocarditis and pericarditis. In a study from March 2020 to January 2021, the incidence of myocarditis was 0.146% among patients diagnosed with COVID-19 and 0.009% among patients without COVID-19, with a 15-fold difference compared to each other (11). In another study, among 718,365 patients diagnosed with COVID-19, 35,820 (5.0%) occurred myocarditis, and 10,706 (1.5%) occurred pericarditis (14). However, in a study, the incidence of myocarditis and pericarditis among 181,656 hospitalized COVID-19 patients was 0.08% (21). Another large study reported that among 277 postmortem examinations, the true incidence of myocarditis was likely less than 2% (22). A published meta-analysis about the rate of myocarditis in COVID-19 fatalities was also less than 2% (23). Our careful analysis of the above articles can conclude that the large variation in the incidence of COVID-19-associated myocarditis is due to diagnostic heterogeneity. The definitive diagnosis of myocarditis should be based on established histological and immunohistochemical (ICH) criteria with endomyocardial biopsy or autopsy (24). Due to the relatively lower rates of endomyocardial biopsies (EMBs) performed in patients with COVID-19, the diagnosis of myocarditis relies more extensively on evidence of myocardial injury or the use of cardiac magnetic resonance imaging (CMR) in conjunction with clinical symptoms (25, 26). These methods are employed to meet the criteria outlined in the CDC working definition of myocarditis. For example, in an Italy study, researchers conducted an autopsy series on 40 patients who had died from SARS-CoV-2 infection. The authors performed a thorough histological analysis of the heart tissues. Interestingly, only two out of the 40 patients exhibited indications of myocarditis, and only one patient had clear evidence of lymphocytic myocarditis. Notably, despite observing histological signs of lymphocytic infiltrate in three cases, the researchers did not detect the presence of the virus using reverse transcription polymerase chain reaction (RT-PCR), in situ hybridization (ISH), and IHC techniques (27). At the same time, the diagnosis of most patients with COVID-19 associated pericarditis is still based on symptoms and imaging examinations, but not pathological examinations (28, 29). Differences in diagnostic criteria have a greater impact on the incidence of myocarditis and pericarditis during the COVID-19 epidemic (20). We analyzed that this reason may significantly affect the result of this Mendelian analysis.
The mechanisms of COVID-19-associated myocarditis or pericarditis are complex and mainly include direct viral damage, cytokine storm, immune system dysregulation, and endothelial dysfunction (9, 30). Firstly, direct viral damage includes endocytosis of the virus and degradation of cellular structures, and overall myocyte injury (31, 32). Secondly, cytokine storm, as a well-known physiological mechanism associated with SARS-CoV-2 infection, leads to the excessive production of pro-inflammatory cytokines. This overproduction of pro-inflammatory cytokines has been linked to myocardial injury, as evidenced by elevated troponin levels observed during these conditions. Following the initial infection of the lungs by SARS-CoV-2, a cytokine storm is generated, characterized by the release of various cytokines such as tumor necrosis factor-alpha (TNFα), interleukin-1β (IL-1β), and IL-6. Alongside this, extracellular vesicles (EVs) carrying the virus or virus particles are also released. These EVs have the potential to travel through the bloodstream or lymphatic system to the heart, where they can infect cardiac cells expressing specific receptors such as ACE2, transmembrane serine protease-2 (TMPRSS2), and neuropilin-1 receptor (NRP1) (33). Thirdly, immune-mediated myocarditis is another important mechanism wherein both the innate and acquired immune responses lead to myocardial injury, ultimately leading to dilated cardiomyopathy. Autoimmune-mediated myocarditis can occur when cryptic antigens in cardiomyocytes, are released following virus-mediated injury. In cases where viral antigens successfully evade the innate immune system, they replicate and produce virus-associated proteins that directly cause myocardial injury by inducing apoptosis and necrosis. Similar to other viral pathogens, SARS-CoV-2 may induce myocarditis in humans through a similar pathway. Noteworthily, cytokine storm promotes the activation of T-cells and releases cytokines to maintain the exaggerated immune response (34). The fourth mechanism involves the direct entry of the virus into endothelial cells within the heart, without necessarily infecting myocytes. This direct infection of endothelial cells has been observed in autopsy hearts and glomerular endothelial cells, through the use of electron microscopy. However, in some cases, the appearance and location of these virus particles within cells were not typical of those observed in coronavirus-infected cells (30, 35).
Although myocarditis or pericarditis caused by COVID-19 may be related to the above mechanisms, there is still some controversy. First, some studies believed that the evidence for direct damage to myocardial cells caused by viruses is not sufficient. In a study involving 39 consecutive autopsy cases from Germany, the viral load of SARS-CoV-2 was quantified. The results showed that SARS-CoV-2 was detected in the hearts of 24 out of 39 cases (61.5%), with 16 out of 39 cases (41%) exhibiting viral copy numbers higher than 1,000 copies per microgram of RNA. Virus replication was observed in five patients with the highest viral load. However, ISH confirmed the presence of the virus in interstitial cells within the cardiac tissue, rather than in the myocytes themselves. Notably, the presence of the virus was not associated with increased infiltration of mononuclear cells into the myocardium, and no cases of myocarditis were identified according to the Dallas criteria (15). At the same time, other researchers also have not found a SARS-CoV-2 genome detected within the myocardium (16, 36). Second, drug-induced myocarditis should also be considered because it has been confirmed in autopsy reports of COVID-19 deaths receiving antivirals (37, 38). Third, Takotsubo cardiomyopathy and myocarditis have similar CMR manifestations, mainly including injury to myocytes, and infiltration of lymphocytes and macrophages in the autopsied specimens. Some so-called COVID-19-related myocarditis cannot be ruled out as Takotsubo cardiomyopathy, especially in patients with a lack of evident myocardial SARS-CoV-2 viral genome (39).
Another concerning issue is confounding factors, such as immunodeficiency. A study showed an increased risk of more severe presentations and higher mortality caused by COVID-19 for patients with solid-organ transplants or cancer (40). According to a recent report, patients with cancer treated with checkpoint inhibitors were at particularly high risk of severe COVID-19 (41). Another study including 60 patients with primary immunodeficiency (PID) and 40 patients with secondary immunodeficiency (SID) included that, compared with the general population, adult patients with PID and symptomatic SID display greater morbidity and mortality from COVID-19 (42). A study concluded that myocarditis was found in 37 cases (52%) according to a retrospective evaluation of 71 consecutive necropsy patients who died from acquired immunodeficiency syndrome (AIDS) (43). Another study included that among 181 consecutive patients at all stages of HIV infection, 109 patients (60%) occurred different degrees of pericarditis (44). We could find that immunodeficiency as a confounding factor is not only associated with COVID-19 infection but also increases the incidence of myocarditis and pericarditis. Therefore, we cannot ignore that confounding factors have a great effect on the results of MR analysis.
Although there is already a large number of literature on the relationship between COVID-19 and myocarditis or pericarditis, our article still has outstanding novelty. Firstly, this is the first time that we have used a new research method—MR to clarify the causal relationship between them. Compared to traditional clinical studies, MR is less prone to potential confounding and avoids reverse causation because genetic variation is allocated at conception, and thus, it can strengthen the evidence for causal inference (45, 46). At the same time, in order to verify whether there is a reverse causal relationship, our article uses bidirectional MR. Secondly, our data comes from the latest GWAS database. The huge sample size and the latest data further improve the stability and credibility of the results. Finally, we conducted an assessment of pleiotropy, and heterogeneity and invited a professional from the data center to assist and verify our statistical and computational processes to ensure the stability and reliability of the results.
5. Limitation
Our study has several limitations. Firstly, most of the included patients with myocarditis or pericarditis were diagnosed by clinical symptoms and signs, auxiliary examinations (electrocardiogram, myocardial enzymes, cardiac color Doppler ultrasound, CMR, etc.), and no EMB or autopsy was performed. This may have a significant impact on our analysis. Secondly, myocarditis or pericarditis is equivalent to the number of COVID-19 patients being too small, and the screened SNPs are relatively insufficient. Finally, the majority of participants were of European ancestry. Hence, the generalizability of the results cannot be derived for other races.
6. Conclusion
Our 2-sample MR analysis did not yield any supportive genetic evidence for a causal association between COVID-19 and an elevated risk of myocarditis or pericarditis. Although growing evidence indicates a causal relationship between COVID-19 and myocarditis or pericarditis, we need to consider the diagnostic heterogeneity of myocarditis or pericarditis and the effect of confounders. According to our conclusions and other evidence, the relationship between COVID-19 and myocarditis or pericarditis still deserves further research and observation.
Data availability statement
The original contributions presented in the study are included in the article/Supplementary Material, further inquiries can be directed to the corresponding author.
Ethics statement
Ethical approval was not required for the study involving humans in accordance with the local legislation and institutional requirements. Written informed consent to participate in this study was not required from the participants or the participants’ legal guardians/next of kin in accordance with the national legislation and the institutional requirements.
Author contributions
GL: Formal analysis, Software, Writing – original draft, Writing – review & editing. TC: Data curation, Formal analysis, Writing – review & editing. XZ: Methodology, Software, Writing – original draft. BH: Data curation, Writing – review & editing. HS: Data curation, Funding acquisition, Project administration, Supervision, Writing – original draft, Writing – review & editing.
Funding
The author(s) declare financial support was received for the research, authorship, and/or publication of this article.
This work was financially supported by National Natural Science Foundation of China (81201788).
Acknowledgments
We acknowledged the participants and investigators of the COVID-19 Host Genetics Initiative and FinnGen study. We would like to thank Chunyang Li of the West China Biomedical Big Data Center for her guidance on MR methods and statistical methods.
Conflict of interest
The authors declare that the research was conducted in the absence of any commercial or financial relationships that could be construed as a potential conflict of interest.
Publisher's note
All claims expressed in this article are solely those of the authors and do not necessarily represent those of their affiliated organizations, or those of the publisher, the editors and the reviewers. Any product that may be evaluated in this article, or claim that may be made by its manufacturer, is not guaranteed or endorsed by the publisher.
Supplementary material
The Supplementary Material for this article can be found online at: https://www.frontiersin.org/articles/10.3389/fcvm.2023.1271959/full#supplementary-material
Supplementary Figure S1
(A) Regression lines of MR tests, (B) forrest plot of single SNP from hospitalized COVID-19 on myocarditis, (C) MR leave-one-out sensitivity analysis, (D) funnel of single SNP from hospitalized COVID-19 on myocarditis.
Supplementary Figure S2
(A) Regression lines of MR tests, (B) forrest plot of single SNP from hospitalized COVID-19 on myocarditis, (C) MR leave-one-out sensitivity analysis, (D) funnel of single SNP from hospitalized COVID-19 on pericarditis.
Supplementary Figure S3
(A) Regression lines of MR tests, (B) forrest plot of single SNP from hospitalized COVID-19 on myocarditis, (C) MR leave-one-out sensitivity analysis, (D) Funnel of single SNP from COVID-19 infection on myocarditis.
Supplementary Figure S4
(A) Regression lines of MR tests, (B) forrest plot of single SNP from hospitalized COVID-19 on myocarditis, (C) MR leave-one-out sensitivity analysis, (D) funnel of single SNP from COVID-19 infection on pericarditis.
Supplementary Figure S5
(A) Regression lines of MR tests, (B) forrest plot of single SNP from hospitalized COVID-19 on myocarditis, (C) MR leave-one-out sensitivity analysis, (D) funnel of single SNP from severe COVID-19 on myocarditis.
Supplementary Figure S6
(A) Regression lines of MR tests, (B) forrest plot of single SNP from hospitalized COVID-19 on myocarditis, (C) MR leave-one-out sensitivity analysis, (D) funnel of single SNP from severe COVID-19 on pericarditis.
Supplementary Figure S7
(A) Regression lines of MR tests, (B) forrest plot of single SNP from hospitalized COVID-19 on myocarditis, (C) MR leave-one-out sensitivity analysis, (D) Funnel of single SNP from myocarditis on hospitalized COVID-19.
Supplementary Figure S8
(A) Regression lines of MR tests, (B) forrest plot of single SNP from hospitalized COVID-19 on myocarditis, (C) MR leave-one-out sensitivity analysis, (D) funnel of single SNP from myocarditis on COVID-19 infection.
Supplementary Figure S9
(A) Regression lines of MR tests, (B) forrest plot of single SNP from hospitalized COVID-19 on myocarditis, (C) MR leave-one-out sensitivity analysis, (D) funnel of single SNP from myocarditis on severe COVID-19.
Supplementary Figure S10
(A) Regression lines of MR tests, (B) forrest plot of single SNP from hospitalized COVID-19 on myocarditis, (C) MR leave-one-out sensitivity analysis, (D) funnel of single SNP from pericarditis on hospitalized COVID-19.
Supplementary Figure S11
(A) Regression lines of MR tests, (B) forrest plot of single SNP from hospitalized COVID-19 on myocarditis, (C) MR leave-one-out sensitivity analysis, (D) funnel of single SNP from pericarditis on COVID-19 infection.
Supplementary Figure S12
(A) Regression lines of MR tests, (B) forrest plot of single SNP from hospitalized COVID-19 on myocarditis, (C) MR leave-one-out sensitivity analysis, (D) funnel of single SNP from pericarditis on severe COVID-19.
Supplementary Table S1
Association of the genetic instruments with severe COVID-19 and myocarditis.
Supplementary Table S2
Association of the genetic instruments with severe COVID-19 and pericarditis.
Supplementary Table S3
Association of the genetic instruments with hospitalized COVID-19 and myocarditis.
Supplementary Table S4
Association of the genetic instruments with hospitalized COVID-19 and pericarditis.
Supplementary Table S5
Association of the genetic instruments with COVID-19 infection and myocarditis.
Supplementary Table S6
Association of the genetic instruments with COVID-19 infection and pericarditis.
Supplementary Table S7
Association of the genetic instruments with myocarditis and hospitalized COVID-19.
Supplementary Table S8
Association of the genetic instruments with myocarditis and COVID-19 infection.
Supplementary Table S9
Association of the genetic instruments with myocarditis and severe COVID-19.
Supplementary Table S10
Association of the genetic instruments with pericarditis and hospitalized COVID-19.
Supplementary Table S11
Association of the genetic instruments with pericarditis and COVID-19 infection.
Supplementary Table S12
Association of the genetic instruments with pericarditis and severe COVID-19.
References
1. Jackson CB, Farzan M, Chen B, Choe H. Mechanisms of SARS-CoV-2 entry into cells. Nat Rev Mol Cell Biol. (2022) 23(1):3–20. doi: 10.1038/s41580-021-00418-x
2. Keri VC, Hooda A, Kodan P, Brunda RL, Jorwal P, Wig N. Intricate interplay between COVID-19 and cardiovascular diseases. Rev Med Virol. (2021) 31(4):e2188. doi: 10.1002/rmv.2188
3. Borczuk AC, Yantiss RK. The pathogenesis of coronavirus-19 disease. J Biomed Sci. (2022) 29(1):87. doi: 10.1186/s12929-022-00872-5
4. Nishiga M, Wang DW, Han Y, Lewis DB, Wu JC. COVID-19 and cardiovascular disease: from basic mechanisms to clinical perspectives. Nat Rev Cardiol. (2020) 17(9):543–58. doi: 10.1038/s41569-020-0413-9
5. Sagar S, Liu PP, Cooper LT Jr. Myocarditis. Lancet. (2012) 379(9817):738–47. doi: 10.1016/S0140-6736(11)60648-X
6. Adler Y, Charron P, Imazio M, Badano L, Baron-Esquivias G, Bogaert J, et al. 2015 ESC guidelines for the diagnosis and management of pericardial diseases: the task force for the diagnosis and management of pericardial diseases of the European society of cardiology (ESC) endorsed by: the European association for cardio-thoracic surgery (EACTS). Eur Heart J. (2015) 36(42):2921–64. doi: 10.1093/eurheartj/ehv318
7. Imazio M, Spodick DH, Brucato A, Trinchero R, Adler Y. Controversial issues in the management of pericardial diseases. Circulation. (2010) 121(7):916–28. doi: 10.1161/CIRCULATIONAHA.108.844753
8. Nappi F, Avtaar Singh SS. SARS-CoV-2-induced myocarditis: a state-of-the-art review. Viruses. (2023) 15(4):916. doi: 10.3390/v15040916
9. Fairweather D, Beetler DJ, Di Florio DN, Musigk N, Heidecker B, Cooper LT Jr. COVID-19, myocarditis and pericarditis. Circ Res. (2023) 132(10):1302–19. doi: 10.1161/CIRCRESAHA.123.321878
10. Artico J, Shiwani H, Moon JC, Gorecka M, McCann GP, Roditi G, et al. Myocardial involvement after hospitalization for COVID-19 complicated by troponin elevation: a prospective, multicenter, observational study. Circulation. (2023) 147(5):364–74. doi: 10.1161/CIRCULATIONAHA.122.060632
11. Boehmer TK KL, Lavery AM, Hsu J, Ko JY, Yusuf H, Romano SD, et al. Association between COVID-19 and myocarditis using hospital-based administrative data—united States, March 2020–January 2021. MMWR Morb Mortal Wkly Rep. (2021) 70:1228–32. doi: 10.15585/mmwr.mm7035e5
12. Imazio M, Cecchi E, Demichelis B, Chinaglia A, Ierna S, Demarie D, et al. Myopericarditis versus viral or idiopathic acute pericarditis. Heart. (2008) 94(4):498–501. doi: 10.1136/hrt.2006.104067
13. Kyto V, Sipila J, Rautava P. Clinical profile and influences on outcomes in patients hospitalized for acute pericarditis. Circulation. (2014) 130(18):1601–6. doi: 10.1161/CIRCULATIONAHA.114.010376
14. Buckley BJR, Harrison SL, Fazio-Eynullayeva E, Underhill P, Lane DA, Lip GYH. Prevalence and clinical outcomes of myocarditis and pericarditis in 718,365 COVID-19 patients. Eur J Clin Invest. (2021) 51(11):e13679. doi: 10.1111/eci.13679
15. Lindner D, Fitzek A, Brauninger H, Aleshcheva G, Edler C, Meissner K, et al. Association of cardiac infection with SARS-CoV-2 in confirmed COVID-19 autopsy cases. JAMA Cardiol. (2020) 5(11):1281–5. doi: 10.1001/jamacardio.2020.3551
16. Sala S, Peretto G, Gramegna M, Palmisano A, Villatore A, Vignale D, et al. Acute myocarditis presenting as a reverse Tako-tsubo syndrome in a patient with SARS-CoV-2 respiratory infection. Eur Heart J. (2020) 41(19):1861–2. doi: 10.1093/eurheartj/ehaa286
17. Cenko E, Badimon L, Bugiardini R, Claeys MJ, De Luca G, de Wit C, et al. Cardiovascular disease and COVID-19: a consensus paper from the ESC working group on coronary pathophysiology & microcirculation, ESC working group on thrombosis and the association for acute CardioVascular care (ACVC), in collaboration with the European heart rhythm association (EHRA). Cardiovasc Res. (2021) 117(14):2705–29. doi: 10.1093/cvr/cvab298
18. Vidal-Perez R, Brandao M, Pazdernik M, Kresoja KP, Carpenito M, Maeda S, et al. Cardiovascular disease and COVID-19, a deadly combination: a review about direct and indirect impact of a pandemic. World J Clin Cases. (2022) 10(27):9556–72. doi: 10.12998/wjcc.v10.i27.9556
19. Sekula P, Del Greco MF, Pattaro C, Kottgen A. Mendelian randomization as an approach to assess causality using observational data. J Am Soc Nephrol. (2016) 27(11):3253–65. doi: 10.1681/ASN.2016010098
20. Verbanck M, Chen CY, Neale B, Do R. Detection of widespread horizontal pleiotropy in causal relationships inferred from Mendelian randomization between complex traits and diseases. Nat Genet. (2018) 50(5):693–8. doi: 10.1038/s41588-018-0099-7
21. Park H, Yun KW, Kim KR, Song SH, Ahn B, Kim DR, et al. Epidemiology and clinical features of myocarditis/pericarditis before the introduction of mRNA COVID-19 vaccine in Korean children: a multicenter study. J Korean Med Sci. (2021) 36(32):e232. doi: 10.3346/jkms.2021.36.e232
22. Halushka MK, Vander Heide RS. Myocarditis is rare in COVID-19 autopsies: cardiovascular findings across 277 postmortem examinations. Cardiovasc Pathol. (2021) 50:107300. doi: 10.1016/j.carpath.2020.107300
23. Merugu GP, Nesheiwat Z, Balla M, Patel M, Fatima R, Sheikh T, et al. Predictors of mortality in 217 COVID-19 patients in northwest Ohio, United States: a retrospective study. J Med Virol. (2021) 93(5):2875–82. doi: 10.1002/jmv.26750
24. Pollack A, Kontorovich AR, Fuster V, Dec GW. Viral myocarditis–diagnosis, treatment options, and current controversies. Nat Rev Cardiol. (2015) 12(11):670–80. doi: 10.1038/nrcardio.2015.108
25. Huang L, Zhao P, Tang D, Zhu T, Han R, Zhan C, et al. Cardiac involvement in patients recovered from COVID-2019 identified using magnetic resonance imaging. JACC Cardiovasc Imaging. (2020) 13(11):2330–9. doi: 10.1016/j.jcmg.2020.05.004
26. Puntmann VO, Carerj ML, Wieters I, Fahim M, Arendt C, Hoffmann J, et al. Outcomes of cardiovascular magnetic resonance imaging in patients recently recovered from coronavirus disease 2019 (COVID-19). JAMA Cardiol. (2020) 5(11):1265–73. doi: 10.1001/jamacardio.2020.3557
27. Dal Ferro M, Bussani R, Paldino A, Nuzzi V, Collesi C, Zentilin L, et al. SARS-CoV-2, myocardial injury and inflammation: insights from a large clinical and autopsy study. Clin Res Cardiol. (2021) 110(11):1822–31. doi: 10.1007/s00392-021-01910-2
28. Dini FL, Baldini U, Bytyci I, Pugliese NR, Bajraktari G, Henein MY. Acute pericarditis as a major clinical manifestation of long COVID-19 syndrome. Int J Cardiol. (2023) 374:129–34. doi: 10.1016/j.ijcard.2022.12.019
29. Diaz-Arocutipa C, Saucedo-Chinchay J, Imazio M. Pericarditis in patients with COVID-19: a systematic review. J Cardiovasc Med (Hagerstown). (2021) 22(9):693–700. doi: 10.2459/JCM.0000000000001202
30. Varga Z, Flammer AJ, Steiger P, Haberecker M, Andermatt R, Zinkernagel AS, et al. Endothelial cell infection and endotheliitis in COVID-19. Lancet. (2020) 395(10234):1417–8. doi: 10.1016/S0140-6736(20)30937-5
31. Blauwet LA, Cooper LT. Myocarditis. Prog Cardiovasc Dis. (2010) 52(4):274–88. doi: 10.1016/j.pcad.2009.11.006
32. Guzik TJ, Mohiddin SA, Dimarco A, Patel V, Savvatis K, Marelli-Berg FM, et al. COVID-19 and the cardiovascular system: implications for risk assessment, diagnosis, and treatment options. Cardiovasc Res. (2020) 116(10):1666–87. doi: 10.1093/cvr/cvaa106
33. Ou X, Liu Y, Lei X, Li P, Mi D, Ren L, et al. Characterization of spike glycoprotein of SARS-CoV-2 on virus entry and its immune cross-reactivity with SARS-CoV. Nat Commun. (2020) 11(1):1620. doi: 10.1038/s41467-020-15562-9
34. Tschope C, Ammirati E, Bozkurt B, Caforio ALP, Cooper LT, Felix SB, et al. Myocarditis and inflammatory cardiomyopathy: current evidence and future directions. Nat Rev Cardiol. (2021) 18(3):169–93. doi: 10.1038/s41569-020-00435-x
35. Fox SE, Li G, Akmatbekov A, Harbert JL, Lameira FS, Brown JQ, et al. Unexpected features of cardiac pathology in COVID-19 infection. Circulation. (2020) 142(11):1123–5. doi: 10.1161/CIRCULATIONAHA.120.049465
36. Xu Z, Shi L, Wang Y, Zhang J, Huang L, Zhang C, et al. Pathological findings of COVID-19 associated with acute respiratory distress syndrome. Lancet Respir Med. (2020) 8(4):420–2. doi: 10.1016/S2213-2600(20)30076-X
37. Russell B, Moss C, George G, Santaolalla A, Cope A, Papa S, et al. Associations between immune-suppressive and stimulating drugs and novel COVID-19-a systematic review of current evidence. Ecancermedicalscience. (2020) 14:1022. doi: 10.3332/ecancer.2020.1022
38. Alijotas-Reig J, Esteve-Valverde E, Belizna C, Selva-O'Callaghan A, Pardos-Gea J, Quintana A, et al. Immunomodulatory therapy for the management of severe COVID-19. Beyond the anti-viral therapy: a comprehensive review. Autoimmun Rev. (2020) 19(7):102569. doi: 10.1016/j.autrev.2020.102569
39. Kawai S, Shimada T. Inflammation in takotsubo cardiomyopathy? Inquiry from “guidelines for diagnosis and treatment of myocarditis (JCS 2009)”. J Cardiol. (2014) 63(4):247–9. doi: 10.1016/j.jjcc.2013.11.002
40. Fung M, Babik JM. COVID-19 in immunocompromised hosts: what we know so far. Clin Infect Dis. (2021) 72(2):340–50. doi: 10.1093/cid/ciaa863
41. Robilotti EV, Babady NE, Mead PA, Rolling T, Perez-Johnston R, Bernardes M, et al. Determinants of COVID-19 disease severity in patients with cancer. Nat Med. (2020) 26(8):1218–23. doi: 10.1038/s41591-020-0979-0
42. Shields AM, Burns SO, Savic S, Richter AG, Consortium UPC. COVID-19 in patients with primary and secondary immunodeficiency: the United Kingdom experience. J Allergy Clin Immunol. (2021) 147(3):870–875.e1. doi: 10.1016/j.jaci.2020.12.620
43. Anderson DW, Virmani R, Reilly JM, O'Leary T, Cunnion RE, Robinowitz M, et al. Prevalent myocarditis at necropsy in the acquired immunodeficiency syndrome. J Am Coll Cardiol. (1988) 11(4):792–9. doi: 10.1016/0735-1097(88)90213-6
44. Silva-Cardoso J, Moura B, Martins L, Mota-Miranda A, Lecour H. Pericardial involvement in human immunodeficiency virus infection. Chest. (1999) 115:418–22. doi: 10.1378/chest.115.2.418
45. Sanderson E, Glymour MM, Holmes MV, Kang H, Morrison J, Munafo MR, et al. Mendelian randomization. Nat Rev Methods Primers. (2022) 2:6. doi: 10.1038/s43586-021-00092-5
Keywords: bidirectional two-sample Mendelian randomization, COVID-19, myocarditis, pericarditis, causal effect
Citation: Liu G, Chen T, Zhang X, Hu B and Shi H (2023) Causal relationship between COVID-19 and myocarditis or pericarditis risk: a bidirectional Mendelian randomization study. Front. Cardiovasc. Med. 10:1271959. doi: 10.3389/fcvm.2023.1271959
Received: 3 August 2023; Accepted: 28 November 2023;
Published: 14 December 2023.
Edited by:
Stamatios Lerakis, Mount Sinai Hospital, United StatesReviewed by:
Sebastiano Cicco, University of Bari Aldo Moro, ItalySaverio Muscoli, Policlinico Tor Vergata, Italy
© 2023 Liu, Chen, Zhang, Hu and Shi. This is an open-access article distributed under the terms of the Creative Commons Attribution License (CC BY). The use, distribution or reproduction in other forums is permitted, provided the original author(s) and the copyright owner(s) are credited and that the original publication in this journal is cited, in accordance with accepted academic practice. No use, distribution or reproduction is permitted which does not comply with these terms.
*Correspondence: Huashan Shi shihuashan@scu.edu.cn