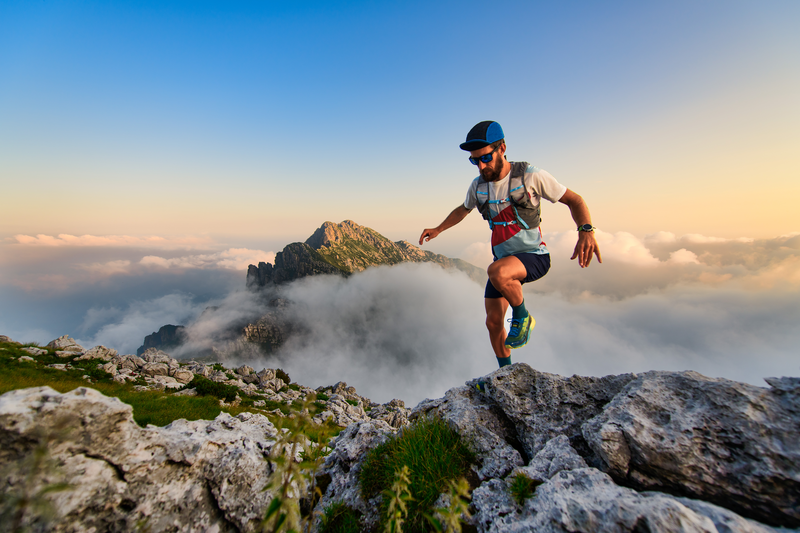
95% of researchers rate our articles as excellent or good
Learn more about the work of our research integrity team to safeguard the quality of each article we publish.
Find out more
CASE REPORT article
Front. Cardiovasc. Med. , 23 October 2023
Sec. Intensive Care Cardiovascular Medicine
Volume 10 - 2023 | https://doi.org/10.3389/fcvm.2023.1271227
This article is part of the Research Topic Extracorporeal Cardio-Pulmonary Resuscitation (ECPR) View all 9 articles
Background: Pulmonary capillary wedge pressure (PCWP) is often used as a surrogate for left-ventricular end-diastolic pressure in patients (LVEDP) who are on veno-arterial extracorporeal membrane oxygenation (V-A ECMO) support for cardiogenic shock and cardiac arrest. However, the correlation between PCWP and LVEDP is not clear in the setting of V-A ECMO usage. We sought to evaluate this correlation in this case series.
Methods: Patients were referred to our cardiac catheterization laboratory for invasive hemodynamic studies to assess their readiness for VA-ECMO decannulation. All patients underwent simultaneous left and right heart catheterization. Using standard techniques, we measured PCWP and LVEDP simultaneously. Continuous variables were reported as medians with interquartile ranges. The correlation between PCWP and LVEDP was evaluated using simple linear regression and reported as R2.
Results: Four patients underwent invasive hemodynamic studies 4 (2.5, 7) days after VA-ECMO cannulation. All four patients had suffered in-hospital cardiac arrest and had been put on VA-ECMO. At the baseline level of VA-ECMO flow of 4.1 (3.8, 4.4) L/min, the median LVEDP and PCWP were 6 (4, 7.5) mmHg and 12 (6.5, 16) mmHg, respectively. At the lowest level of VA-ECMO flow of 1.9 (1.6, 2.0) L/min, the median LVEDP and PCWP was 13.5 (8.5, 16) mmHg and 15 (13, 18) mmHg, respectively. There was a poor correlation between the simultaneously measured PCWP and LVEDP (R2 = 0.03, p = 0.66).
Conclusions: The PCWP may not correlate well with LVEDP in patients treated with VA-ECMO, particularly at high levels of VA-ECMO support.
Extracorporeal cardiopulmonary resuscitation (ECPR) with veno-arterial extracorporeal membrane oxygenation (V-A ECMO) is a strategy that has produced improvement in mortality in out-of-hospital cardiac arrest (1, 2). With the publication of recent trials showing the benefit of this strategy (3–6), it is likely that V-A ECMO will be subject to wider adoption worldwide as part of ECPR strategies.
Despite the demonstrated utility of the ECPR strategy, there is still significant debate about the effect of V-A ECMO on intracardiac hemodynamics in cardiogenic shock and cardiac arrest. Pulmonary capillary wedge pressure (PCWP) is often used as a metric of preload. This is under the assumption that PCWP reflects left atrial pressure and/or left-ventricular end-diastolic pressure (LVEDP) (7). Additionally, right heart catheterization is used in patients on V-A ECMO to evaluate intracardiac hemodynamics during the course of V-A ECMO treatment. While these principles may apply to patients that do not have mechanical support strategies in place, the utility of PCWP and its correlation to LVEDP has not yet been comprehensively evaluated in patients with V-A ECMO.
In this case series, we evaluated the relationship of PCWP with LVEDP with simultaneous left and right heart catheterization in four patients treated with V-A ECMO as part of an ECPR strategy. We sought to evaluate whether the PCWP and LVEDP varied with changes in V-A ECMO flow, the direction in which they changed with changes in V-A ECMO flow, and the degree of correlation between these two metrics.
All patients were evaluated between November 2021 and June 2022. All four patients were placed on V-A ECMO and enrolled in our local ECPR protocol after in-hospital cardiac arrest or as part of the Minnesota Resuscitation Consortium's previously published protocol (8) for out-of-hospital cardiac arrest. All patients had cannulation with the venous and arterial cannulae in the femoral vasculature (Vf-Af ECMO). Their intensive care also followed a protocolized pathway that we have published previously (9). All hemodynamic data were collected prospectively. The study was approved by our institution's Institutional Review Board (study number 00005355).
The four subjects described in this case series were referred for invasive hemodynamic case series as part of routine clinical care. The invasive hemodynamic studies were sought due to marginal recovery of the patient's cardiac function that led the referring critical care physician(s) to be uncertain about the patients' candidacy for V-A ECMO decannulation. This led the cardiac critical care team to invasively evaluate changes in LV and RV. All subjects were treated with aggressive supportive measures and volume removal prior to the invasive hemodynamic studies, and the timing of the invasive hemodynamic study was left to the treating clinician's judgement.
Our institution's echocardiographic turndown (weaning) protocol has been published previously (10). In this protocol, the V-A ECMO support is sequentially reduced from the baseline flow to 1–2 L/min while a methodical evaluation of LV and RV function is obtained. The reductions in V-A ECMO flow are done in 1 L/min increments. At each stage, the V-A ECMO flow is maintained for at least three minutes and hemodynamic stability (as measured by aortic mean arterial pressure >60 mmHg) is ensured before further decreasing the level of support. At the lowest level of support, the flow is maintained for at least five minutes to allow for a steady state to be achieved. Patients are only referred for turndown studies if they are on low or moderate doses of ≤2 vasopressors/inotropes.
In addition to our echocardiographic turndown data, we adapted our turndown protocol to include measurements of left and right heart pressures at the baseline (highest) and lowest V-A ECMO flow. Left ventricular pressures were measured by obtaining left radial access under ultrasound and fluoroscopy guidance using a 6 Fr slender sheath. A 6 Fr multipurpose diagnostic catheter was then inserted via the sheath into the aortic root. After this, an Opsens Optowire pressure wire was positioned in the LV cavity. Dual-lumen pigtail catheters were not available in our laboratory during the study period (11). All patients had right heart (Swan-Ganz) catheters inserted via the right internal jugular vein after this under ultrasound and fluoroscopic guidance. We then measured aortic, LV end-diastolic, right ventricular (RV) end-diastolic, pulmonary artery systolic, pulmonary artery diastolic, and pulmonary capillary wedge pressures in all four patients at the baseline and lowest tolerated V-A ECMO flows as part of the above turndown protocol. The RVEDP was reported as a measure of right-sided filling pressures. Right atrial pressure was not measured due to the fact that the venous VA-ECMO cannula was in the right atrium in the patients and it was assumed that the measurements would be subject to significant artifact. The left and right heart catheters were zeroed at each phase of the V-A ECMO flow. None of the hemodynamic measurements were obtained with any unloading strategy (intra-aortic balloon pump, percutaneous ventricular assist device, or left atrial venoarterial cannulation) in place. All pressure measurements were taken at the trough of the respiratory cycle. Since all patients were mechanically ventilated at the time of the examination, this was done to ensure that the pressure measurements were taken at end-expiration (12). Catheter placement was confirmed to be in West Zone 3 by ensuring that the PCWP did not change significantly with positive end-expiratory pressure. All invasive hemodynamic measurements were evaluated by two Cardiologists. The first Cardiologist measured them during the procedure and saved the hemodynamic waveforms for offline review. The second Cardiologist evaluated the pressure measurements offline from the saved waveforms and was blinded to the original measurements.
All continuous variables were represented as medians with interquartile ranges. Simple linear regression was done to evaluate the relationship between the LVEDP and PCWP at the baseline level of flow and the LVEDP and PCWP at the lowest level of flow. Results were reported as R2. The level of significance was 0.05 for all analyses. All analyses were done in Stata/MP 17.0.
Four patients with a median age of 54 (46,61) years with SCAI E cardiogenic shock were referred to us for invasive hemodynamic evaluations due to uncertainty regarding their candidacy for VA-ECMO decannulation. Their clinical history is outlined in Table 1.
All four patients were placed on V-A ECMO as part of an ECPR strategy after suffering in-hospital cardiac arrest. Three of the patients had pulseless electrical asystole and one patient had pulseless ventricular tachycardia. None of the patients had return of spontaneous circulation prior to V-A ECMO cannulation.
All four patients underwent invasive hemodynamic studies due to the treating clinicians' concerning regarding the patients' ability to be decannulated from V-A ECMO. Two patients were referred for the invasive hemodynamic study due to severe biventricular dysfunction on an echocardiogram within 24 h of the invasive hemodynamic study. The two other patients were referred for the invasive hemodynamic study due to severe RV dysfunction on an echocardiogram within 24 h of the invasive hemodynamic study despite supportive therapies. Two of the four patients required low or moderate doses of vasopressors prior to the invasive hemodynamics study. An example of the obtained pressure tracings is seen in Figure 1.
Figure 1. Example pressure tracings from patient 1. Pressure measurements from patient 1 at high V-A ECMO flow are seen. Panel A depicts the simultaneous LV and aortic pressure tracings. Panel B depicts the patient's PCWP pressure tracing.
All patients had Doppler evaluation of the mitral and tricuspid valves prior to their invasive hemodynamic study during the same hospitalization. None of the patients had mitral stenosis or tricuspid stenosis.
The median time to the invasive hemodynamic study was 4.0 (2.5, 7.0) days after V-A ECMO cannulation. During the invasive hemodynamic study, the median baseline V-A ECMO flow was 4.1 (3.8, 4.4) L/min and the median lowest V-A ECMO flow was 1.9 (1.6, 2.0) L/min. None of the patients required any adjustments to their ventilatory/oxygenation settings on their mechanical ventilators during the course of the invasive hemodynamic study. Moreover, none of the patients had any changes in the level of inotropic/vasopressive support that they required.
The PCWP and LVEDP were simultaneously evaluated in all four patients in the cardiac catheterization suite. The PCWP and LVEDP are listed in Table 2 for each patient at the baseline and lowest tolerated V-A ECMO flow. Among all measurements in all four patients, the median LVEDP was 7.5 (5.0, 13.5) mmHg and the median PCWP was 13.5 (11.5, 17.5) mmHg. At the baseline level of VA-ECMO flow, the median LVEDP was 6.0 (4.0, 7.5) mmHg, the median RVEDP was 9.0 (5.0, 14.0) mmHg, and the median PCWP was 12 (6.5, 16.0) mmHg. At the lowest level of VA-ECMO flow, the median LVEDP was 13.5 (8.5, 16.0) mmHg, the median RVEDP was 14.0 (9.5, 22.0) mmHg, and the median PCWP was 15.0 (13.0, 18.0) mmHg.
In simple linear regression, there was a poor correlation between the simultaneously measured LVEDP and PCWP (R2 = 0.03, p = 0.66; Figure 2). When the baseline and lowest levels of V-A ECMO flow were evaluated separately, there was a poor correlation between LVEDP and PCWP that were simultaneously measured at the baseline VA-ECMO flow (R2 = 0.05, p = 0.77) and the lowest tolerated VA-ECMO flow (R2 = 0.02, p = 0.86).
Figure 2. Correlation between simultaneously measured pulmonary capillary wedge pressure and left ventricular end-diastolic pressure in patients on V-A ECMO.
In this manuscript, we report the relationship between invasively measured PCWP and LVEDP at two different stages of V-A ECMO flow. In the four patients that received V-A ECMO support as part of an ECPR strategy, we found that there was a poor correlation between the PCWP and LVEDP. Not only did the simultaneously measured values of LVEDP and PCWP exhibit some variation, the direction in which the numbers changed with alterations in V-A ECMO flow also varied among the four patients.
There may be several explanations for our findings. Firstly, V-A ECMO is placed in our institution by inserting the venous cannula via a femoral approach into the right atrium or at the junction of the inferior vena cava and right atrium. This type of cannula placement, which is relatively commonly reported in the literature, is responsible for reducing the amount of venous return to the pulmonary circulation to very small amounts. Consequently, in patients where PCWP is significantly lower than the LVEDP, it is unlikely that there is significant pulmonary return that traverses the pulmonary circuit. Thus, the PCWP may be significantly lower than the LVEDP. Moreover, patients may have a relatively reduced LV compliance compared to left atrial compliance.
There were also multiple instances wherein the LVEDP was significantly lower than the PCWP. We hypothesize that this discrepancy may be due to significant lung injury and the resultant intrapleural pressure elevations in these patients. The elevated intrapleural pressures may lead to significant atrial compression, leading to elevated atrial pressures that are transmitted to the pulmonary capillaries. In contrast, the thicker-walled LV may be less prone to compression, thus leading to a relatively lower elevation in the LVEDP (13). Given that severe lung injury occurs in patients with prolonged resuscitation requiring ECPR, this may be a common source of discordance. Moreover, a reduction in atrial compliance, either due to the index OHCA or pre-morbid cardiac conditions, may also lead to a relatively higher PCWP than LVEDP, as is seen in the heart failure with preserved ejection fraction population (14). Otherwise, none of the patients had mitral stenosis, pulmonary vein stenosis, or pulmonary veno-occlusive disease during the course of their hospitalization. In patients with severe mitral regurgitation, overestimation of the PCWP due to high V waves may also occur. Similarly, patients with atrial fibrillation or increased LV afterload may also exhibit reduced atrial compliance and severely elevated V waves. However, none of the patients in this case series had severe mitral regurgitation or exhibited atrial fibrillation at the time of the invasive hemodynamic study.
We also found that the PCWP and LVEDP appeared to be better correlated at the lowest level of V-A ECMO flow. Based on the above physiologic explanations, this may be because the reduction in the level of V-A ECMO flow allowed increased transpulmonary flow, thereby raising PCWP to a level that was more similar to LVEDP. Moreover, increased flow the left atrium may have allowed for the PCWP and LVEDP to be more similar.
Prior simulation data suggest that there are elevations of PCWP soon after the initiation of V-A ECMO (15–17). In these cases, PCWP is assumed to reflect LVEDP. Further, it is assumed that the elevated afterload leads to elevations of LVEDP and this is transmitted backwards into the pulmonary vasculature. Our data suggest that this may not be universally evident. This has also been proposed in the human literature. Schrage et al. reported a significant and immediate rise in PCWP in three patients that had V-A ECMO placement. This was used as a justification to implement LV unloading strategies. While the PCWP did decline soon after implementation of the LV unloading strategy, LVEDP was not measured in these patients.
Our findings may have clinical implications. Like other ambulatory and chronic cardiovascular conditions where there may be discrepancies in LVEDP and PCWP, the hemodynamic management of V-A ECMO may warrant separate evaluation of LVEDP and PCWP. This may be particularly pertinent at important junctures in the clinical care of these patients, such as when deciding whether unloading strategies are required. It may be particularly helpful to evaluate both PCWP and LVEDP at varying levels of flow, should the patient be able to tolerate that.
Our study has several limitations. Our case series carries all of the limitations that a small number of observations may be expected to carry. Specifically, there is likely a vast spectrum of hemodynamic phenotypes that may exist and only a small portion are represented here. There may be heterogeneity in the reduction of flows as a proportion of total flow (cardiac output + V-A ECMO). Future studies should aim to evaluate a strictly protocolized turn down to similar levels of flow in homogenous cohorts. Our study is also prone to selection bias—only patients that were thought to be able to tolerate reduction in V-A ECMO flows as part of a turndown were included in our study. It is also important to note that the relationship between PCWP and LVEDP may be dynamic. Thus, the magnitude and directionality of the PCWP and LVEDP relationship may change over time during the V-A ECMO treatment course of a single patient. Given that our investigation only evaluated invasive left and right heart pressures once in each of the patients, we were unable to evaluate this. Finally, it is possible that the differences in PCWP and LVEDP were present due to human error. However, we meticulously zeroed the catheters before each V-A ECMO flow phase and did blinded, off-line reviews of saved hemodynamic waveforms in an effort to prevent bias and human error. Even still, some measurements are too discrepant to explain by human error alone.
In this small case series, we demonstrate that there is an inconsistent correlation between the magnitude and directionality of PCWP and LVEDP. Clinicians who manage V-A ECMO patients should consider monitoring both separately to guide clinical management.
The raw data supporting the conclusions of this article will be made available by the authors; all reasonable requests for data will be considered.
The studies involving humans were approved by University of Minnesota Institutional Review Board (study number 00005355). The studies were conducted in accordance with the local legislation and institutional requirements. The Ethics Committee/institutional review board waived the requirement of written informed consent for participation from the participants or the participants’ legal guardians/next of kin because data was obtained from routine clinical care. Written informed consent was not obtained from the individual(s) for the publication of any potentially identifiable images or data included in this article because all data was deidentified and was obtained as part of routine clinical care.
RK: Conceptualization, Data curation, Formal Analysis, Methodology, Visualization, Writing – original draft, Writing – review & editing. CG: Writing – original draft, Writing – review & editing, Data curation, Formal Analysis. TA: Writing – review & editing. MK: Writing – review & editing. DJ: Writing – review & editing. GS: Writing – review & editing. GR: Writing – review & editing. JB: Writing – review & editing. AG: Writing – review & editing. RJ: Writing – review & editing. TW: Writing – review & editing. DY: Writing – original draft, Writing – review & editing.
The author(s) declare financial support was received for the research, authorship, and/or publication of this article.
This project was supported by a grant from the American Heart Association (23TPA1140962; awarded to RK), a grant from the German Research Foundation (GA 3379/1-1; awarded to CG), a grant from The Helmsley Charitable Trust (awarded to DY) and a grant from the National Institute of Health (R01HL160973-01; awarded to DY).
The authors declare that the research was conducted in the absence of any commercial or financial relationships that could be construed as a potential conflict of interest.
The author(s) declared that they were an editorial board member of Frontiers, at the time of submission. This had no impact on the peer review process and the final decision.
All claims expressed in this article are solely those of the authors and do not necessarily represent those of their affiliated organizations, or those of the publisher, the editors and the reviewers. Any product that may be evaluated in this article, or claim that may be made by its manufacturer, is not guaranteed or endorsed by the publisher.
1. Kalra R, Kosmopoulos M, Goslar T, Raveendran G, Bartos JA, Yannopoulos D. Extracorporeal cardiopulmonary resuscitation for cardiac arrest. Curr Opin Crit Care. (2020) 26(3):228–35. doi: 10.1097/mcc.0000000000000717
2. Tsangaris A, Alexy T, Kalra R, Kosmopoulos M, Elliott A, Bartos JA, et al. Overview of veno-arterial extracorporeal membrane oxygenation (VA-ECMO) support for the management of cardiogenic shock. Front Cardiovasc Med. (2021) 8:686558. doi: 10.3389/fcvm.2021.686558
3. Yannopoulos D, Bartos J, Raveendran G, Walser E, Connett J, Murray TA, et al. Advanced reperfusion strategies for patients with out-of-hospital cardiac arrest and refractory ventricular fibrillation (ARREST): a phase 2, single centre, open-label, randomised controlled trial. Lancet. (2020) 396(10265):1807–16. doi: 10.1016/s0140-6736(20)32338-2
4. Yannopoulos D, Kalra R, Kosmopoulos M, Walser E, Bartos JA, Murray TA, et al. Rationale and methods of the advanced R2Eperfusion STrategies for refractory cardiac ARREST (ARREST) trial. Am Heart J. (2020) 20:29–39. doi: 10.1016/j.ahj.2020.07.006
5. Belohlavek J, Smalcova J, Rob D, Franek O, Smid O, Pokorna M, et al. Effect of intra-arrest transport, extracorporeal cardiopulmonary resuscitation, and immediate invasive assessment and treatment on functional neurologic outcome in refractory out-of-hospital cardiac arrest: a randomized clinical trial. JAMA. (2022) 327(8):737–47. doi: 10.1001/jama.2022.1025
6. Belohlavek J, Yannopoulos D, Smalcova J, Rob D, Bartos J, Huptych M, et al. Intraarrest transport, extracorporeal cardiopulmonary resuscitation, and early invasive management in refractory out-of-hospital cardiac arrest: an individual patient data pooled analysis of two randomised trials. eClinicalMedicine. (2023) 59:101988. doi: 10.1016/j.eclinm.2023.101988
7. Rao P, Khalpey Z, Smith R, Burkhoff D, Kociol RD. Venoarterial extracorporeal membrane oxygenation for cardiogenic shock and cardiac arrest. Circ Heart Fail. (2018) 11(9):e004905. doi: 10.1161/CIRCHEARTFAILURE.118.004905
8. Yannopoulos D, Bartos JA, Martin C, Raveendran G, Missov E, Conterato M, et al. Minnesota resuscitation consortium’s advanced perfusion and reperfusion cardiac life support strategy for out-of-hospital refractory ventricular fibrillation. J Am Heart Assoc. (2016) 5(6):e003732. doi: 10.1161/JAHA.116.003732
9. Bartos JA, Carlson K, Carlson C, Raveendran G, John R, Aufderheide TP, et al. Surviving refractory out-of-hospital ventricular fibrillation cardiac arrest: critical care and extracorporeal membrane oxygenation management. Resuscitation. (2018) 132:47–55. doi: 10.1016/j.resuscitation.2018.08.030
10. Kalra R, Bartos JA, Kosmopoulos M, Carlson C, John R, Shaffer A, et al. Echocardiographic evaluation of cardiac recovery after refractory out-of-hospital cardiac arrest. Resuscitation. (2020) 154:38–46. doi: 10.1016/j.resuscitation.2020.06.037
11. McKeown LA. FDA: class I recall for vascular solutions’ dual lumen catheters. TCTMD. Available at: https://www.tctmd.com/news/fda-class-i-recall-vascular-solutions-dual-lumen-catheters#:∼:text=Vascular%20Solutions%2C%20Inc.%20has%20announced,recall%2C%20the%20most%20serious%20type (Accessed September 8, 2020).
12. Saxena A, Garan AR, Kapur NK, O'Neill WW, Lindenfeld J, Pinney SP, et al. Value of hemodynamic monitoring in patients with cardiogenic shock undergoing mechanical circulatory support. Circulation. (2020) 141(14):1184–97. doi: 10.1161/CIRCULATIONAHA.119.043080
13. Hemnes AR, Opotowsky AR, Assad TR, Xu M, Doss LN, Farber-Eger E, et al. Features associated with discordance between pulmonary arterial wedge pressure and left ventricular End diastolic pressure in clinical practice: implications for pulmonary hypertension classification. Chest. (2018) 154(5):1099–107. doi: 10.1016/j.chest.2018.08.1033
14. Hanna Elias B, Smart Frank W, Hanna Deschamps E. Mechanisms of discrepancy between pulmonary artery wedge pressure and left ventricular End-diastolic pressure in heart failure with preserved ejection fraction. JACC Heart Fail. (2018) 6(3):267–9. doi: 10.1016/j.jchf.2017.11.009
15. Schrage B, Burkhoff D, Rübsamen N, Becher PM, Schwarzl M, Bernhardt A, et al. Unloading of the left ventricle during venoarterial extracorporeal membrane oxygenation therapy in cardiogenic shock. JACC Heart Fail. (2018) 6(12):1035–43. doi: 10.1016/j.jchf.2018.09.009
16. Burkhoff D, Sayer G, Doshi D, Uriel N. Hemodynamics of mechanical circulatory support. J Am Coll Cardiol. (2015) 66(23):2663–74. doi: 10.1016/j.jacc.2015.10.017
Keywords: VA-ECMO, ECLS (VA), PCWP, pulmonary capillary wedge pressure, LVEDP, left ventricular end-diastolic pressure
Citation: Kalra R, Gaisendrees C, Alexy T, Kosmopoulos M, Jaeger D, Schlachtenberger G, Raveendran G, Bartos JA, Gutierrez Bernal A, John R, Wahlers T and Yannopoulos D (2023) Case Report: Correlation between pulmonary capillary wedge pressure and left-ventricular diastolic pressure during treatment with veno-arterial extracorporeal membrane oxygenation. Front. Cardiovasc. Med. 10:1271227. doi: 10.3389/fcvm.2023.1271227
Received: 1 August 2023; Accepted: 12 October 2023;
Published: 23 October 2023.
Edited by:
Gary Schwartz, Baylor University Medical Center, United StatesReviewed by:
Miguel Alvarez Villela, Lenox Hill Hospital, United States© 2023 Kalra, Gaisendrees, Alexy, Kosmopoulos, Jaeger, Schlachtenberger, Raveendran, Bartos, Gutierrez Bernal, John, Wahlers and Yannopoulos. This is an open-access article distributed under the terms of the Creative Commons Attribution License (CC BY). The use, distribution or reproduction in other forums is permitted, provided the original author(s) and the copyright owner(s) are credited and that the original publication in this journal is cited, in accordance with accepted academic practice. No use, distribution or reproduction is permitted which does not comply with these terms.
*Correspondence: Demetris Yannopoulos eWFubm8wMDFAdW1uLmVkdQ==
†These authors have contributed equally to this work
Disclaimer: All claims expressed in this article are solely those of the authors and do not necessarily represent those of their affiliated organizations, or those of the publisher, the editors and the reviewers. Any product that may be evaluated in this article or claim that may be made by its manufacturer is not guaranteed or endorsed by the publisher.
Research integrity at Frontiers
Learn more about the work of our research integrity team to safeguard the quality of each article we publish.