- 1Biological Work and Health Psychology, University of Konstanz, Konstanz, Germany
- 2Centre for the Advanced Study of Collective Behaviour, University of Konstanz, Konstanz, Germany
Objective: Exaggerated physiological reactions to acute mental stress (AMS) are associated with hypertension (development) and have been proposed to play an important role in mediating the cardiovascular disease risk with hypertension. A variety of studies compared physiological reactivity to AMS between essential hypertensive (HT) and normotensive (NT) individuals. However, a systematic review of studies across stress-reactive physiological systems including intermediate biological risk factors for cardiovascular diseases is lacking.
Methods: We conducted a systematic literature search (PubMed) for original articles and short reports, published in English language in peer-reviewed journals in November and December 2022. We targeted studies comparing the reactivity between essential HT and NT to AMS in terms of cognitive tasks, public speaking tasks, or the combination of both, in at least one of the predefined stress-reactive physiological systems.
Results: We included a total of 58 publications. The majority of studies investigated physiological reactivity to mental stressors of mild or moderate intensity. Whereas HT seem to exhibit increased reactivity in response to mild or moderate AMS only under certain conditions (i.e., in response to mild mental stressors with specific characteristics, in an early hyperkinetic stage of HT, or with respect to certain stress systems), increased physiological reactivity in HT as compared to NT to AMS of strong intensity was observed across all investigated stress-reactive physiological systems.
Conclusion: Overall, this systematic review supports the proposed and expected generalized physiological hyperreactivity to AMS with essential hypertension, in particular to strong mental stress. Moreover, we discuss potential underlying mechanisms and highlight open questions for future research of importance for the comprehensive understanding of the observed hyperreactivity to AMS in essential hypertension.
1. Introduction
Essential hypertension (EHT), the chronic elevation of blood pressure (BP) without secondary causes, is one of the most prevalent risk factors for cardiovascular disease (CVD) (1, 2). Exaggerated physiological reactions to acute stress (AMS), or more precisely acute mental stress, have been proposed to be involved in mediating the CVD risk with hypertension (3, 4). Specifically, several lines of evidence suggest that exaggerated mental stress-induced cardiovascular and neuroendocrine reactions predict not only premature development of hypertension and other precursors of coronary heart disease, but also an accelerated progression of atherosclerosis and the likelihood of having a future acute coronary syndrome such as myocardial infarction (3–6). In addition to cardiovascular and neuroendocrine hyperreactivity, heightened stress reactivity of intermediate biological CVD risk factors (e.g., blood lipids and lipoproteins, hemostatic factors, inflammatory activity) may play an important role in hypertension and hypertension development (7–9) as well as in the interface between stress and heightened risk for CVD morbidity and mortality (10–13). Since the 1970s, a variety of studies have compared the physiological reactivity to AMS in essential hypertensive individuals (HT) and normotensive individuals (NT) applying mental stressors of different severity and considering different stress-reactive physiological systems. However, a systematic summary of the literature is lacking so far. Here, we therefore summarize for the first time the hitherto published evidence on physiological reactivity to AMS in HT as compared to NT regarding the primary endocrine stress-axes, i.e., the sympathetic-adrenal-medullary (SAM) axis, the hypothalamus-pituitary-adrenal (HPA) axis, and the renin-angiotensin-aldosterone system (RAAS), in addition to major stress-reactive intermediate biological risk factors for CVD (coagulation, blood lipids and lipoproteins, and inflammatory measures). Based the hitherto reported cardiovascular hyperreactivity in EHT to different psychological and physiological stressors including AMS [for review see: (14, 15)] on the one hand, and the interrelation of stress-reactive physiological systems (16–20) on the other hand, we hypothesized a generalized physiological hyperreactivity to AMS in EHT across different stress-reactive physiological systems. Moreover, we discuss potential mechanisms underlying the obtained summarized stress reactivity findings in EHT.
1.1. Essential hypertension as a major CVD risk factor
Arterial hypertension is a highly prevalent medical condition defined as a chronic elevation of systolic BP (SBP) ≥140 mmHg, a chronic elevation of diastolic BP (DBP) ≥90 mmHg, or both (21). The worldwide prevalence of hypertension among adults is about 30% with noticeable but stable differences across countries and with a steep increase with ageing (21, 22). Hypertension presents a major if not the greatest risk factor for CVD (23). With BP increase from normal to severe levels, the risk for accelerated atherosclerosis, coronary heart disease, left-ventricular hypertrophy, and stroke increases markedly (24, 25). Moreover, high BP was identified to be the leading single risk factor globally in 2015 accounting for 10.7 million deaths (26). Intriguingly, most individuals diagnosed with arterial hypertension suffer from EHT, also referred to as primary, idiopathic, or systemic hypertension, meaning that there is no medical cause for their condition (27).
1.2. Acute mental stress
According to Lazarus & Folkman (28), stress is the result of cognitive appraisal processes concerning the interaction between the individual and the environment. More precisely, stress occurs when an individual experiences demands or threats, i.e., encounters a so-called stressor, without sufficient resources to meet these demands or mitigate the threats. This perceived imbalance between situational demands and personal resources triggers stress responses comprising a wide variety of psychophysiological reactions in order to counteract the stressful circumstances (29). Stress can occur acutely, i.e., lasting for minutes to hours (i.e., acute stress) or chronically, i.e., persisting for days to months (i.e., chronic stress) (30). In human acute stress research, most studies focus on AMS. Mental stressors commonly used comprise cognitive tasks, public speaking tasks (PST), and the combinations of cognitive and PST (31, 32). Typical cognitive tasks are mental arithmetic tasks (MA), Raven's progressive matrices (33), general knowledge quizzes, visual puzzles, and interference tasks such as the mirror tracing task or the Stroop color word interference task (SCWT) (34). In PST, participants are instructed to prepare and deliver a short speech in front of an audience on an assigned, often controversial topic. Notably, cognitive tasks have been shown to induce physiological stress reactions of minor intensity as compared to PST (35). Strongest physiological stress reactions have been observed in reaction to the combination of cognitive tasks with PST (31). Central for induction of strong stress reactions is the combination of social-evaluative threat (i.e., potential negative evaluation of the self, e.g., by an evaluative audience) and uncontrollability (i.e., perception that avoidance of negative consequences, termination of aversive experiences or succession is not or will not be possible, e.g., false feedback or time-pressure) (31). A frequently used psychosocial stressor that combines PST and MA is the Trier Social Stress Test [TSST (36)]. The TSST comprises a short introduction phase followed by a 3-min preparation phase, a 5-min mock job interview, and a 5-min MA in front of an audience with video and audio recording. Also, the Montreal Imaging Stress Task [MIST (37)] that comprises a series of computerized mental arithmetic challenges, was initially developed for inducing AMS in a functional imaging setting and includes social evaluation and uncontrollability (31).
Given these differences in stressor potency to elicit physiological stress reactions, we discriminate between reactivity to mild, moderate, and strong mental stressors. We refer to cognitive tasks as mild, to PST as moderate, and to the TSST and the MIST (that both combine PST with MA) as strong mental stressors.
1.3. Major stress-reactive physiological systems
Following stress encounter, multiple interrelated physiological systems are activated, comprising neuroendocrine stress axes, i.e., the SAM axis, the HPA axis, and the RAAS, as well as stress-reactive intermediate biological risk factors for CVD.
The first activated stress-reactive system is the SAM axis (38). Here, stress perception activates preganglionic sympathetic neurons in the intermediolateral cell column of the spinal cord. This subsequently leads on the one hand the activation of end organs via direct sympathetic signaling pathways where norepinephrine (NEP) acts as postganglionic transmitter and on the other hand to stimulation of the secretion of the catecholamines NEP and epinephrine (EP) from chromaffin cells of the adrenal medulla into the circulation (39). Both, the NEP released directly at sympathetic synapses and the circulating catecholamines released from the adrenal medulla, induce rapid physiological adaptions in order to allow to “fight-or-flight”, e.g., increases of BP, heart rate (HR), or vasodilation of central blood vessels (e.g., in skeletal muscles, heart, and brain) (38). Measures of acute SAM axis reactivity comprise on the endocrine level the plasma catecholamines NEP and EP, on the cardiovascular level BP, HR, cardiac output, stroke volume, or total peripheral resistance. Also, the enzyme salivary alpha amylase (sAA), secreted from the salivary glands under sympathetic stimulation, is considered a surrogate marker of catecholamine release, in particular of NE (40). Notably, all these parameters reach their maximum stress reactivity during or immediately after stress. Recovery to baseline levels can be observed within 5–20 min after stress cessation for most parameters except for BP where delayed recovery periods have been reported (41–44).
Simultaneously to the sympathetic activation, the activity of the parasympathetic nervous system (PNS) as counterpart of the SNS, predominant under quiet, resting conditions, decreases. Parasympathetic (re)activity can be assessed via heart rate variability (HRV) reflecting beat-to-beat changes in RR intervals (45, 46). HRV parameters reflecting predominantly PNS (re)activity comprise the square root of the mean of the sum of the squares of differences between adjacent normal-to-normal interval’ (RMSSD), the percentage difference between adjacent normal-to-normal intervals that are greater than 50 ms (PNN50), and the high frequency (HF) band (0.15–0.40 Hz) (45, 46). Notably, given that HRV depends on resting heart rate as well as the frequency and depth of respiration, reliable HRV assessment requires adjustment for these potentially influencing factors (47). In reaction to AMS, immediate significant decreases in RMSSD, pNN50, and HF have been observed in healthy individuals (48–50).
In addition the activation of the SAM axis, perception of stress activates hypophysiotropic neurons in the paraventricular nucleus of the hypothalamus that lead to the release of the hypothalamic hormones, corticotropin-releasing hormone (CRH) and arginine vasopressin (AVP), which are the principal regulators of the HPA axis (39). CRH and AVP stimulate the secretion of adrenocorticotropin hormone (ACTH) from the anterior pituitary (51). ACTH primarily acts on the zona fasciculata of the adrenal cortex, where it initiates the synthesis and secretion of cortisol (52). Cortisol acts on intracellular cytosolic glucocorticoid and mineralocorticoid receptors throughout the entire body, in order to prepare the body for exposure to stress (53). In human stress research, assessment of acute HPA axis (re)activity usually comprises peripheral ACTH and/or cortisol measurements (54, 55). While ACTH is usually measured from blood plasma, cortisol can reliably be measured from both, blood plasma and serum (bound and free fractions of cortisol), as well as from saliva (biologically active free fraction of cortisol) (56, 57). With respect to stress reactivity, stress exposure by TSST induces fast increases in ACTH that peak immediately after TSST cessation and return to baseline levels about 40 min after stress cessation (36, 58). Salivary cortisol shows a comparably delayed stress reactivity with highest levels peaking about 30 min after TSST beginning, or about 10 min after its cessation, respectively, that last for another 10–20 min and then slowly return to baseline levels about 60 min after stress cessation (36, 59). Reactivity of plasma and serum cortisol starts a bit earlier but the subsequent reactivity pattern is comparable (36, 59).
Both, the SAM axis and the HPA axis, can interact with the RAAS. With respect to the SAM axis, catecholamines released from the adrenal medulla induce the synthesis and secretion of renin via β-adrenergic receptors on juxtaglomerular cells in the kidney. Subsequently, renin catalyzes the hydrolysis of angiotensinogen produced in the liver and fat tissues to angiotensin-I (ANG-I) (60). ANG-I is transported to lung capillaries, endothelial cells, and kidney endothelial cells via the blood circulation where it is converted to angiotensin-II (ANG-II) by angiotensin-converting enzyme (ACE) (61). ANG-II then stimulates the production of aldosterone in the zona glomerulosa of adrenal cortex (62). With respect to the HPA axis, the stress-induced release of ACTH stimulates in addition to the production of cortisol in the zona fasciculata also the production of aldosterone in the zona glomerulosa of the adrenal cortex (60). Parameters indicative of RAAS activation comprise plasma renin and plasma renin activity (PRA), ANG-II, and aldosterone that can be measured in blood plasma. Notably, we recently showed that aldosterone can also be validly assessed from saliva (18). While plasma renin measures and ANG-II increased immediately after stress with quick return to baseline levels, highest levels of both, plasma and salivary aldosterone, were observed during the 20 min after TSST cessation (18, 63). Stress-induced aldosterone level increases returned to baseline within about 90–120 min after stress with respect to salivary aldosterone and within 180 min after stress for plasma aldosterone (18).
In addition to the activation of endocrine stress axes, AMS elicits increases in intermediate biological risk factors for CVD. Acute stress induces activation of coagulation molecules, platelets, and fibrinolysis resulting in net hypercoagulability (11, 64) that presumably aims at protecting the organism from lethal hemorrhage in case of a potentially resulting injury in fight-or-flight situations (65). Major clotting-related targets of stress-induced catecholamine-release include platelet activation, the hepatic release of clotting factors VII (FVII:C) and VIII (FVIII:C), as well as excretion of hemostatically active von Willebrand factor antigen (VWF) and the pro-fibrinolytic tissue-type plasminogen activator (t-PA) from endothelial storage pools into the circulation (66, 67). Notably, further stress-induced t-PA increases in the circulation origin from sympathetic innervated artery walls (68). Subsequently, various resulting processes involving the clotting factors IX (FIX:C), X (FX:C), XI (FXI:C), and XII (FXII:C), lead to further platelet activation and the formation of a critical amount of thrombin, initiating the conversion of fibrinogen to fibrin and clot formation (69). Termination of clot formation involves several anticoagulant mechanisms leading to inactivation of thrombin by formation of thrombin-antithrombin III (TAT) complexes (70). Laboratory studies in healthy humans show increased stress-induced activation of the clotting parameters FVII:C, FVIII:C, platelets, t-PA, TAT complexes, and fibrinogen with highest levels immediately after stress and recovery back to prestress levels about 20–45 min after stress (12, 66, 71, 72). Notably, stress reactivity kinetics of the fibrin cleavage product and hypercoagulability parameter D-Dimer are not fully understood yet with some studies reporting highest D-dimer levels immediately after stress and other study observing delayed increases (71, 73–75).
Blood lipids and lipoproteins represent further stress-reactive intermediate biological risk factors. Stress-induced changes in the prothrombotic lipid parameters total cholesterol (TC), triglycerides (TG), and low density lipoprotein-cholesterol (LDL-C) as well as the antithrombotic high density lipoprotein-cholesterol (HDL-C) presumably aim at providing energy for muscles activity in fight-or-flight responses (76). Studies investigating blood lipid and lipoprotein reactivity to AMS report stress-induced increases in prothrombotic and antithrombotic blood lipid parameters (13, 77–82). However, not all these studies controlled for stress-induced hemoconcentration as an important confounding factor (79). Notably, stress can induce transient acute loss of plasma volume into the extravascular space, which results in the concentration and passive increase of larger (>69 kDa) and thus non-diffusible blood constituents such as lipids (83, 84). After control for stress hemoconcentration effects on blood lipid reactivity (85), stress-induced increases in prothrombotic blood lipids and lipoproteins have been observed in reaction to mental stressors of moderate or strong intensity (13, 78, 79, 81) but not in reaction to mild mental stressors (79). With respect to HDL-C, results of studies controlling for stress hemoconcentration remain inconclusive (13, 78, 79). Stress hemoconcentration-independent changes in blood lipid parameters in reaction to AMS have been proposed to relate to stress-induced SNS activation (86, 87). With respect to the kinetics of lipid stress reactivity to moderate and strong mental stress, most studies point to highest levels of prothrombotic blood lipids and lipoproteins at or shortly after stress cessation (13, 78, 79, 81).
Finally, acute stress is capable of inducing immune activation. A recent systematic review and meta-analysis summarized the increasing number of studies investigating reactivity of inflammatory markers in reaction to AMS in healthy individuals (88). Robust stress-induced increases of moderate to large effect size were found for the cytokines interleukin (IL)-6 and IL-1β (88, 89), in addition to small to moderate stress-induced increases in tumor necrosis factor (TNF)-α (88). Stress-induced C-reactive protein (CRP) increases however could not be confirmed (88). It is assumed that the stress-induced proinflammatory response has evolved in order to protect an individual from potential immediate injury and infection due to stressors such as e.g., a predator attack (30, 90). Stress reactivity kinetics differ between cytokines [likely due to differences in half-lives or clearance mechanisms (91)] and are not fully understood yet given the investigation period of only up to 2 h in existing studies. Notably, inflammatory markers were found to increase delayed, i.e., not before 10 min after stress cessation. Peak levels of circulating IL-1β and TNF-α were mostly observed 40–50 min after stress, while peak levels of IL-6 occurred later, at 90–120 min after stress (88). The exact mechanisms underlying the stress-induced increases in inflammatory markers are beginning to be understood. It has been speculated that cytokine increases in reaction to acute stress are mediated via the SNS and intracellular proinflammatory activity (88, 89, 92). With respect to down-regulation or delay of the inflammatory response to acute stress, cortisol, and thus HPA axis reactivity, has been proposed to play an important role (88, 93–95).
2. Systematic review: physiological reactivity to AMS in essential hypertension
Exaggerated stress-induced physiological reactions have not only been associated with premature development of hypertension (6, 96) but also been proposed to play an important role in mediating the CVD risk with hypertension (3). In the following, we summarize the hitherto existing literature on the physiological reactivity to AMS with respect to the SAM and HPA axes, the RAAS as well as intermediate biological risk factors in HT compared to NT. In line with existing meta-analytic evidence for a cardiovascular hyperreactivity with EHT to different stressors including AMS (14) and the interrelation of stress-reactive physiological systems (16–20), we expect our systematic review to support a generalized physiological hyperreactivity to AMS with EHT across different stress-reactive physiological systems.
2.1. Methods
We performed a systematic review according to the “preferred reporting items for systematic reviews and meta-analysis guidelines” (PRISMA) (97). Our literature search was conducted in November and December 2022.
2.1.1. Search strategy
In our main literature search on studies investigating physiological reactivity to AMS in manifest EHT, we conducted a systematic search on PubMed using the combinations of search terms “hypertension” AND (“mental stress” OR “psychological stress” OR “psychosocial stress” OR “acute stress”) together with the keywords of each of the considered stress-reactive physiological systems: the SNS (sympathetic reactivity, sympathetic nervous system, catecholamines, norepinephrine, epinephrine, cardiovascular reactivity, blood pressure, heart rate, salivary alpha-amylase), the PNS (parasympathetic reactivity, parasympathetic nervous system, heart rate variability, RMSSD, HF, pNN50), the HPA axis (HPA axis, CRH, ACTH, cortisol), the RAAS (renin-angiotensin-aldosterone-system, renin, angiotensin, aldosterone), the coagulation system (coagulation, procoagulant, d-dimer, fibrinogen, FVII, FVIII), blood lipids and lipoproteins (blood lipids, LDL, HDL, triglycerides, cholesterol), the immune system (inflammation, inflammatory marker, interleukin, t-PA, TNF-alpha, NF-kappaB, CRP). All identified publications were screened to exclude duplicates. Subsequently, both, abstracts and full texts of remaining publications were reviewed manually for formal and content-related inclusion criteria. We moreover manually searched the reference lists of included publications for further relevant publications not identified by the initial search.
Moreover, we a-priori defined that in case of less than 10 identified eligible publications for a stress-reactive physiological systems, our main literature search for this system will be extended to a secondary literature search on hypertension-prone individuals. Study participants were considered as hypertension-prone if at least one of their parents had EHT (parental hypertension) and/or if they were designated as hypertension-prone by the authors of the respective publication. Based on this definition, we repeated the previous systematic literature for all stress-reactive systems except the SNS (see Figure 1) by using the search terms “hypertension-prone” OR “parental hypertension” instead of “hypertension”.
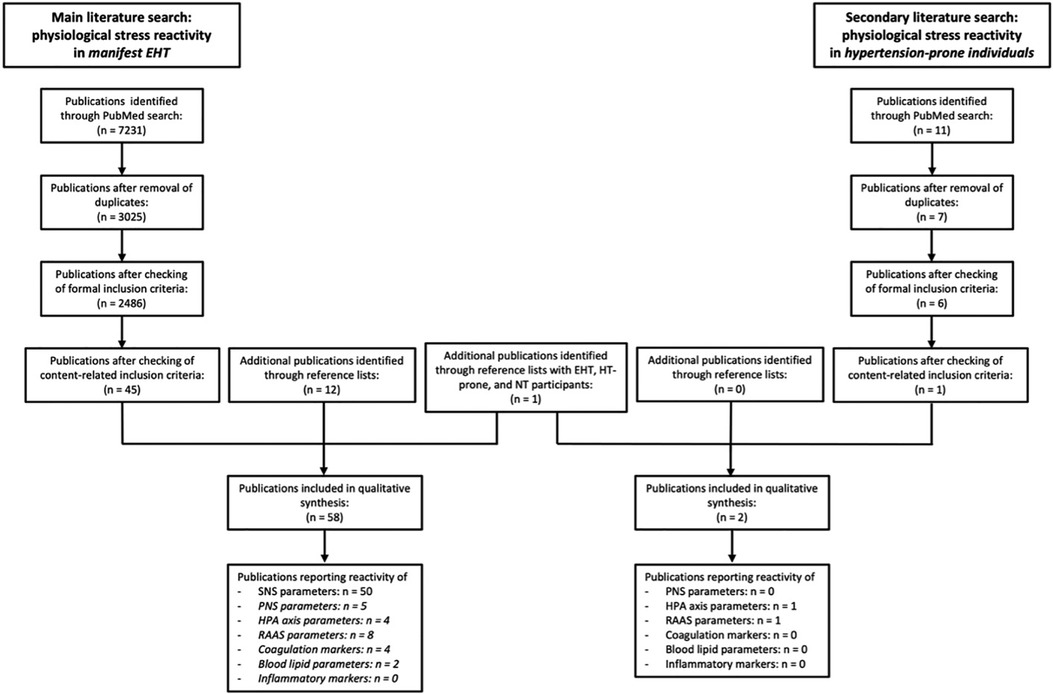
Figure 1. Flow diagram of the process of study selection. A secondary literature search was conducted for endocrine stress systems and biological risk factors with <10 publications (in italics) included in qualitative synthesis of main literature search. EHT, essential hypertension; SNS, sympathetic nervous system; PNS, parasympathetic nervous system; HPA axis, hypothalamus-pituitary-adrenal axis; RAAS, renin-angiotensin-aldosterone-system; n = number of publications.
2.1.2. Eligibility and inclusion criteria
With respect to formal inclusion criteria, we considered original articles and short reports published in English language that were published in peer-reviewed journals. Meta-analyses, reviews, case reports, editorials, and conference abstracts were not considered. With respect to content-related inclusion criteria, we included studies reporting experimental data on human physiological reactivity in reaction to AMS assessed in essential hypertensive (main literature search) or hypertension-prone (secondary literature search) individuals of at least 18 years as compared to a normotensive control group. Acute mental stressors considered in this review comprise cognitive tasks (MA, Raven's progressive matrices, general knowledge quizzes, visual puzzles, mirror tracing tasks, SCWT) (mild mental stressors), PST (moderate mental stressors), and the combinations of cognitive and PST (strong mental stressors). Notably, publications that did not differentiate between reactivity to mental stressors and other stressor types were not further considered. Regarding the physiological reactivity assessment, publications had to capture at least one of the previously defined physiological parameters of interest (plasma EP, plasma NEP, BP, HR, sAA, RMSSDN, PNN50, HF, ACTH, plasma or salivary cortisol, plasma renin or plasma renin activity, ANG-II, aldosterone, any coagulation or inflammatory marker, or blood lipid parameter). Notably, some studies have resulted in multiple publications, addressing different physiological systems and parameters with respect to AMS reactivity in EHT. To prevent a resulting bias, we included the first published data of the respective physiological systems and parameters.
2.1.3. Data collection and synthesis
We retrieved and tabulated the following data from publications with respect to each defined physiological parameter: participant characteristics, stressor characteristics, and findings with respect to physiological stress reactivity.
2.2. Results
With respect to the main literature search, our search yielded 2,486 potentially relevant publications after removal of duplicates and checking of formal inclusion criteria (see Figure 1). Ultimately, 45 publications met content-related inclusion criteria and 13 additional eligible publications were identified from reference lists, rendering a total of 58 publications included in the qualitative synthesis of literature on physiological reactivity to AMS in EHT. 50 publications report reactivity of SNS parameters, 5 of PNS parameters, 4 of HPA axis parameters, 8 of RAAS parameters, 4 of coagulation markers, 2 of blood lipid parameters and 0 of inflammatory markers (see Tables 1–5). With respect to the secondary literature search, we identified 6 potentially relevant publications after removal of duplicates and checking of formal inclusion criteria (see Figure 1). Only 1 of these 6 publications met content-related inclusion criteria. We additionally identified 1 eligible publication from reference lists, rendering a total of 2 articles included in the qualitative synthesis of literature on reactivity to AMS in hypertension-prone individuals with respect to PNS, HPA axis, RAAS, and intermediate biological risk factors.
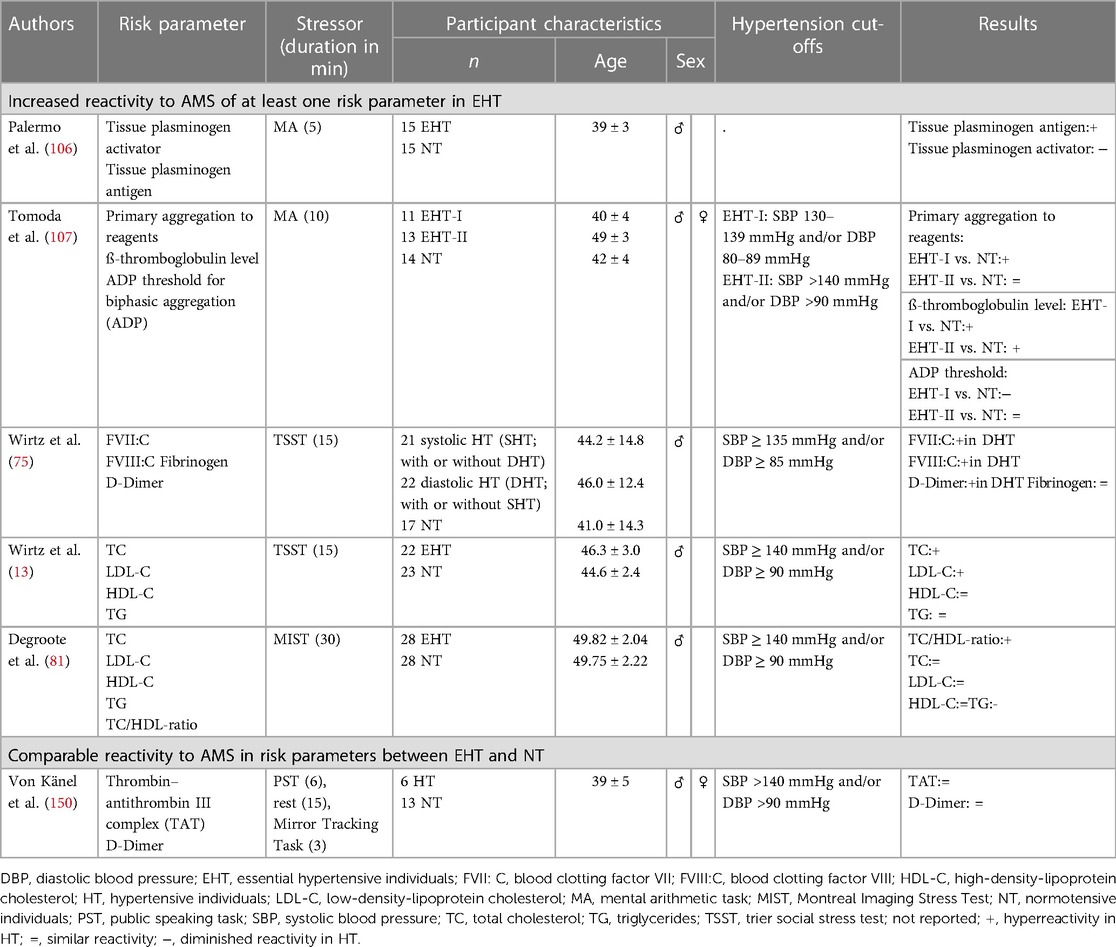
Table 5. Summary of studies on stress reactivity to AMS in EHT with respect to intermediate biological risk factors for cardiovascular disease.
In the following, we report the results of our systematic review for the defined physiological parameters. For a condensed summary of our results, see Table 6.
2.2.1. Stress reactivity of the autonomic nervous system
2.2.1.1. Sympathetic adrenal medullary (SAM) axis
With respect to sympathetic parameters or SAM axis parameters, respectively, reactivity to AMS has been extensively studied in EHT. Given the multitude of studies, we restricted our review to studies that investigated sympathetic stress reactivity in manifest HT. We hereby focused our literature search on catecholamines, cardiovascular reactivity in terms of BP and HR as the major sympathetic measures, and sAA (see Table 1).
2.2.1.1.1. Catecholamine reactivity
Norepinephrine: In reaction to AMS including either mild or strong mental stressors, the majority of studies failed to find reactivity differences in plasma NEP between HT and NT (42, 105, 107, 109, 118, 119, 127, 129–133, 142, 143) despite the overall higher plasma NEP levels with EHT (42, 142, 143). Notably, the participants of these studies were mostly aged between 35 and 50 years of age. However, few studies investigated reactivity to mild AMS by means of MA in younger subjects mostly aged between 19 and 29 (98–104). Results of these studies show enhanced NEP reactivity in the young HT as compared to NT. Interestingly, Lenders et al. (101) explicitly examined potential age-related differences in MA stress-induced plasma catecholamine reactivity in EHT. In line with the above summarized findings, they found increased NEP responses to MA in young HT aged 20–29 years, but not in older HT aged 30–39 or 40–55 years, all as compared to NT. These findings are in accordance with the hypothesis that in particular young HT and those in the early stages of hypertension are characterized by a hyperkinetic state with elevations in HR and cardiac output as well as increased sympathetic tone (151, 152). This hyperkinetic state is supposed to attenuate with increasing age and the progression of hypertension (151). In later stages of hypertension, BP elevations can be achieved with less sympathetic firing/tone because of alterations in the wall-lumen ratio and hyperresponsiveness of arterioles. This results in the combination of normal cardiac output and increased vascular resistance; characteristic for advanced hypertension (151, 153).
Epinephrine: Similar to NEP reactivity, the majority of studies did not observe differences in plasma EP reactivity to AMS between HT and NT (99–102, 104, 118, 119, 127, 129–133, 142, 143), apart from overall higher plasma EP levels with hypertension in some studies (99, 142). Notably, all these studies applied mild mental stressors. So far, only five studies report increased EP reactivity to AMS in HT with four studies employing mild mental stressors (98, 103, 105–107) and one study applying a strong stressor (42). To the best of our knowledge, the latter study is the hitherto only study investigating EP reactivity to a strong mental stressor. In that study, middle-aged EHT and NT men underwent the TSST, and EP reactivity was assessed repeatedly before and up to 60 min after stress cessation. Results showed higher EP reactivity in EHT as compared to NT with most pronounced reactivity differences up to 10 min after stress cessation pointing to EP hyperreactivity in reaction to strong AMS in middle-aged EHT. With respect to mild stressors, two studies investigated EP reactivity in younger subjects with an average age of 20 years to 2–5 min of MA and found higher EP reactivity in HT as compared to NT (98, 103) while other comparable studies did not (100, 104, 119, 129, 142). Notably, investigating EP reactivity in young men in the low, normal, and high (hypertension grade 2) BP ranges, Flaa et al. (98) found increasing EP reactivity with increasing BP. The last study investigated EP reactivity to the SCWT in two groups of EHT individuals, including grade 2 HT, aged from 18 to 59 years as compared to NT (105). In this study, the SCWT induced significant EP increases in the HT groups but not in NT. Other studies on EP reactivity to the SCWT, notably in HT grade 1, failed to find reactivity differences (99, 104, 118, 131). It remains to be elucidated whether an elevated EP reactivity to mild AMS may depend on HT severity.
2.2.1.1.2. Cardiovascular reactivity
Blood pressure: In the majority of studies on BP reactivity to AMS, HT and NT exhibited similar SBP (20, 42, 99, 102, 105, 119, 130, 131, 133–136, 138–142, 144, 145), DBP (20, 42, 99, 101, 102, 107, 112–114, 116, 118, 120, 122, 130, 131, 133–136, 138–141, 143–145), and/or mean BP (MBP) (129, 137) stress reactivity with HT having comparatively higher overall BP levels. In contrast, comparably fewer studies report elevated SBP (100, 101, 107, 109–114, 116–118, 120–122, 124–126), DBP (100, 105, 109–111, 117, 119, 121, 124–126), and/or MBP (98, 103, 108, 115, 123, 127) reactivity to AMS in EHT. A more detailed look on methodological differences between the studies revealed that elevated SBP (100, 101, 107, 109–112, 114, 116, 117, 120–122, 125), DBP (100, 109–111, 117, 119, 121, 125), or MBP reactivity (98, 108, 115) was predominantly observed in studies using MA with a duration between 4 and 15 min. Neither studies using MA of shorter duration [increased BP reactivity in HT (103, 113, 127); similar BP reactivity (112, 113, 136, 138, 142, 144, 145); decreased BP reactivity in HT (142)], nor other similarly mild mental stressors [increased BP reactivity in HT (105, 118, 123, 124, 126); similar BP reactivity (99, 105, 118, 131, 135, 137, 140, 141)], nor stronger stress induction tasks [increased BP reactivity in HT: (126); similar BP reactivity (20, 42)]: were capable of reliably inducing higher BP reactivity in HT as compared to NT. Moreover, there was no clear evidence for effects of age or stressor severity (i.e., mild vs. moderate vs. strong) on BP stress reactivity in HT as have been associated with catecholamine reactivity (see above). Taken together, these findings suggest that elevated BP reactivity in HT seems to specifically relate to MA of a minimum length. Given that HT did not show a higher BP reactivity to other cognitive or stronger mental stressors, it might be speculated that the stressfulness of a task does not account for BP reactivity differences in HT. Whether the BP reactivity difference in HT relates to cognitive processes and related brain activity or psychophysical demands associated with MA or other mild mental stressors (e.g., SCWT) remains to be elucidated (154–156).
Heart rate: With respect to HR reactivity, the majority of studies could not reveal significant reactivity differences either to mild (20, 42, 99, 101, 102, 105, 110–112, 114–116, 118–120, 124–127, 129–131, 133, 134, 136–140, 142, 143, 145), moderate (20, 126), or strong (42) AMS between HT and NT. Increased HR reactivity to mild AMS in terms of MA in HT was observed in five studies that were all performed in younger HT (98, 100, 103, 107, 109). This further supports the above outlined hyperkinetic state in young HT and those in the early stages of hypertension (151, 152) (see Catecholamine reactivity). Moreover, one study reports increased HR reactivity to MA in individuals with hypertension grade 2 but not with hypertension grade 1 as compared to NT (107). Of all studies only two studies found diminished HR reactivity in reaction to MA in middle-aged EHT (144, 145). Taken together, there is weak evidence for a HR hyperreactivity to AMS in EHT, except for a subgroup of hyperkinetic HT.
2.2.1.1.3. Salivary alpha amylase
In the hitherto only study investigating sAA reactivity to AMS in EHT, we recently found that HT exhibit greater sAA reactivity to the TSST as a strong mental stressor as compared to NT with higher sAA increases +1 min and +10 min after TSST cessation (128). This suggest that the stress-induced physiological hyperreactivity in EHT also extends to the SNS parameter sAA.
2.2.1.2. Parasympathetic nervous system (PNS)
So far, PNS reactivity to AMS in manifest EHT without further comorbidity has been investigated in four studies that applied mild mental stressors and assessed the PNS parameter HF (see Table 2). Two of the studies could not find significant stress-induced HF changes, neither in NT nor in HT to MA (115) or SCWT (99). However, the other two studies observed greater HF decreases in HT as compared to NT in reaction to MA pointing to a reduced parasympathetic cardiac control during stress in HT (117, 146). In line with the latter, a recent study investigated the RMSSD response to the TSST as strong mental stressor in patients with cardiometabolic risk factors, i.e., with EHT and/or either prior evidence of prediabetes or type-2 diabetes as compared to healthy NT (147). Patients with cardiometabolic risk factors had overall lower RMSSD values during rest as well as during stress. PNS reactivity to AMS in hypertension-prone individuals has not yet been investigated. Taken together, these studies may suggest that the reduced parasympathetic cardiac control under resting conditions (157, 158) seem to extend to AMS, in terms of a greater reduction of PNS activity under stress, most likely dependent on the intensity of the stressor.
Taken together, the above summarized findings regarding stress reactivity of the autonomic nervous system suggests that SAM axis hyperreactivity cannot generally be observed in HT but occurs only in response to specific mental stressors or in an early hyperkinetic stage of HT (see Table 6).
2.2.2. Hypothalamus pituitary adrenal (HPA) axis
Regarding HPA axis reactivity to AMS in human EHT (see Tables 3, 6), previous studies focused on the HPA axis stress hormone cortisol whereas ACTH has hitherto not been investigated in EHT. Compared to NT, HT exhibited larger elevations in plasma (109, 148) as well as salivary cortisol (20, 42) during and after mild, moderate, and strong mental stress, however not unequivocally (137). Also, hypertension-prone individuals have been reported to show higher salivary cortisol responses to moderate mental stress (148). There are hitherto no studies investigating ACTH reactivity to AMS in hypertension-prone individuals. Notably, animal studies point to an HPA axis hormone-spanning stress hyperreactivity with hypertension (159, 160). Whether the heightened HPA axis stress reactivity in human EHT already occurs at the level of the pituitary gland where ACTH is secreted from the portal system into the circulation, remains to be elucidated. Moreover, given the observed prospective association between higher cortisol reactivity to AMS and incident hypertension (6), it can be assumed that increased HPA axis reactivity is present before the onset of hypertension. Thus, the magnitude of HPA axis stress reactivity may constitute one possible mechanism through which acute stress may influence the risk of hypertension and CVD (4, 6).
2.2.3. Renin-angiotensin-aldosterone system
In the context of human hypertension, reactivity of RAAS parameters to AMS has so far hardly been investigated (see Table 4). With respect to mental stress-induced plasma renin reactivity, studies applying mild AMS could not find significant differences between HT and NT (107, 109, 131, 135, 141, 144). Notably three of them found negligible PRA changes in both, HT and NT (131, 135, 144) and three observed slightly but not significantly higher PRA increases in HT as compared to NT (107, 109, 141). In response to a mild stress-inducing IQ-Test however, HT exhibited increases in PRA as compared to the decreases observed in the NT (137). This study is also the only study investigating RAAS reactivity to AMS in hypertension-prone individuals. Hypertension-prone individuals showed PRA increases in-between NT and HT (137). Moreover, this study is also the hitherto only one considering ANG-II reactivity to AMS so far, revealing a similar reactivity pattern for ANG-II as observed for PRA, with increases in the hypertension-group, decreases in the normotensive-group, and hypertension-prone individuals to be in-between (137). So far, renin or ANG-II reactivity to stronger AMS induction procedures have not yet been investigated in or hypertension-prone individuals as compared to NT. With respect to plasma aldosterone reactivity, two of the three studies applying mild mental stressors, did not reveal reactivity differences between HT and NT in reaction to the SCWT (141) or in reaction to MA (107). In the second study however, HT showed stress-induced aldosterone increases after an IQ-test significantly differing from the decreases observed in NT (137). We recently investigated aldosterone reactivity in EHT, applying the TSST as a strong psychosocial stressor and overserved significantly greater aldosterone increases in EHT as compared to NT (149). There are hitherto no studies investigating aldosterone reactivity to AMS in hypertension-prone individuals.
Taken together, in reaction to mild AMS induction, results are mixed but point to a (slightly) higher reactivity of all RAAS parameters in hypertension, whereas strong AMS induction induced significantly higher reactivity of the RAAS end-product aldosterone in EHT (see Table 6). Overall, these results suggest that the stress-induced physiological hyperreactivity in EHT also extends to the RAAS depending on the intensity of the stressor.
2.2.4. Intermediate biological risk factors for cardiovascular disease
2.2.4.1. Coagulation activity
So far, four studies investigated coagulation activity in response to AMS in HT as compared to NT (see Table 5). In terms of mild mental stress, one study observed attenuated fibrinolysis activation in terms of t-PA reactivity (106) and another study found greater platelet activity (107) in HT as compared to NT in response to MA. A third study applied a speech task and a mirror tracking task in randomized order as mild and moderate mental stressors, respectively, and could not find group differences in stress-induced increases in TAT and D-Dimer, notably in a rather small sample of 6 HT and 13 NT (150). There is one study examining coagulation reactivity in EHT in reaction to strong mental stress (75). In this study, HT exhibited exaggerated acute procoagulant responses as compared to NT. These responses became most apparent during recovery of hypercoagulability from stress depending on hypertension subtype with diastolic HT showing higher FVII:C levels immediately post-stress and at 20-min recovery, higher FVIII:C levels at 20- and 60-min recovery as well as higher D-dimer recovery (75). Coagulation activity in response to AMS in hypertension-prone individuals as compared to NT has hitherto not been investigated. Taken together, there is compiling evidence for an exaggerated coagulation reactivity to AMS in EHT, especially in diastolic HT, that seems to be most apparent during recovery from prothrombotic changes from stress (see Table 6).
2.2.4.2. Blood lipids and lipoproteins
Blood lipid reactivity to AMS has hardly been investigated in EHT. There are hitherto no studies investigating blood lipid reactivity in reaction to mild or moderate AMS in EHT, however, two studies investigated blood lipid reactivity in response to strong mental stress (13, 81) (see Table 5). In reaction to the TSST, hypertensive men exhibited greater TC and LDL-C responses as compared to normotensive controls, with group differences sustaining up to 60 min after TSST cessation and most pronounced differences immediately after TSST cessation (13). There were no reactivity differences in HDL-C and TG. In reaction to the MIST, notably performed within an fMRI environment in lying position wearing goggles, HT showed a more pronounced rise in TC/HDL-C ratio scores and a slower decrease in TG levels as compared to NT but no reactivity differences in TC, HDL-D, and LDL-C (81). Notably, complementary analyses using MAP as continuous measure of hypertension assessment confirmed the linear nature of the observed effects in both studies. Whether the observed reactivity differences in some of the lipid markers are generalizable and relate to differences between the TSST and the MIST, e.g., such as perceived stressor intensity, remains to be elucidated. There are no studies investigating blood lipid reactivity in response to AMS in hypertension-prone individuals.
Taken together, the above outlined studies indicate an exaggerated blood lipid stress reactivity with hypertension, at least to strong mental stress (see Table 6). Whether this extends to mild mental stressors remains to be elucidated.
2.2.4.3. Inflammatory markers
So far, there are no studies investigating reactivity of inflammatory markers to AMS in human EHT or in hypertension-prone individuals (see Table 5). Notwithstanding, there is few evidence pointing to a potentially exaggerated inflammatory responses to AMS in EHT. Two studies in manifest HT investigated stress reactivity of immune parameters related to secretion of inflammatory markers (20, 161). In reaction to mild mental stress, HT exhibited a circulatory environment conducive to increased leukocyte adhesion including higher increases in T-lymphocytes (161), both central to atherogenesis, as well as higher levels of the antibody type immunoglobulin A measured from saliva (20) as compared to NT controls. Moreover, studies investigating associations between inflammatory markers and BP instead of EHT, similarly point to a potentially exaggerated inflammatory stress response with increasing BP and thus EHT. Two cross-sectional studies, notably in NT, report associations between cardiovascular and inflammatory reactivity to mild mental stress (162, 163). Increasing BP stress reactivity was found to be associated with higher levels of IL-6 and IL-1 receptor antagonist (162) as well as higher stress-induced IL-1β gene expression (163).
To sum it up, differences in inflammatory reactivity to AMS between HT and NT have not been investigated so far (see Table 6). However, results from studies investigating stress reactivity of immune parameters in HT or associations between inflammatory stress reactivity and BP stress reactivity suggest that elevated stress reactivity of inflammatory markers might relate to EHT, in particular prospectively.
3. Potential mechanisms underlying physiological hyperreactivity to AMS in essential hypertension
Potential mechanisms underlying the above summarized physiological hyperreactivity to AMS in EHT have conceptually been divided into three different systemic levels of influence (164): the cognitive-emotional level, the hypothalamic and brainstem level, and the peripheral level. Alterations in physiological stress reactivity with EHT may arise from systematic differences at the cognitive-emotional level encompassing perception and evaluation of and affective responses to (mental) stressors as well as the concomitant cortical processing. Notably, these processes depend on the activity of the corticolimbic system and are important determinants of the physiological reactivity. Differences in physiological stress reactivity may (additionally or alternatively) emerge at the hypothalamic and brainstem level, where inputs from the corticolimbic systems are converted into autonomic or neuroendocrine (re)activity (39). Last, stress reactivity differences may result from tissue alterations at the peripheral level, such as alterations in vascular or receptor functioning.
3.1. Cognitive-emotional level
Various psychological factors have been proposed to affect the perception and evaluation of a stressor as well as the affective response that may contribute to the tonic elevation of sympathetic drive and to the frequent and intense bouts of stressor-evoked reactivity observed in HT (164, 165). These psychological factors include social support, affect management or emotional regulation respectively, chronic stress, in addition to cognitive appraisal of the stress situation (165). Interestingly, differences between HT and NT have repeatedly been observed with respect to some of these variables with HT reporting lower levels of social support and higher levels of perceived chronic stress (42, 166–168). They were found to differ in emotional regulation strategies, in particular with deficits in positive strategies, but interestingly not in the cognitive appraisal of a standardized stressor (42). Despite these observed differences, the role of psychological factors in physiological stress reactivity in HT has hardly been investigated so far. The hitherto only study investigating associations of psychological factors with physiological AMS reactivity in EHT examined associations of social support, hedonistic emotional regulation (HER), and cognitive appraisal with endocrine stress reactivity to strong mental stress (42). With respect to social support, HT with lower perceived social support showed higher EP reactivity to the TSST as compared to NT and HT with higher perceived social support. With respect to HER, HT with lower HER exhibited higher NEP reactivity and higher cortisol reactivity to the TSST as compared to NT and HT with high HER. In sum, findings of that study suggest a possible role for social support and emotional regulation in modulating endocrine stress reactivity in EHT. Whether such modulated endocrine stress reactivity in turn affects intermediate biological CVD risk factors and whether such a mechanism may link HT with poor cardiovascular outcomes remains to be elucidated. With respect to chronic stress, some studies in NT found increased cardiovascular or neuroendocrine reactivity with higher chronic stress (169, 170), but the role of chronic stress in physiological reactivity to AMS in EHT has not yet been investigated.
In addition to psychological factors, the cognitive emotional level is affected by the neural structure, function, and connectivity of the corticolimbic system (171). In particular, alterations of the corticolimbic system may relate to the stress reactivity differences with EHT (172). In reaction to mild acute stress, HT exhibited altered (re)activity in corticolimbic brain areas proposed to be involved in stress regulation (39, 173), including prefrontal and cingulate cortex, insula, hippocampus, as well as the amygdala (174–176). While imaging studies that additionally assess physiological stress reactivity are lacking in HT, evidence from studies in NT points to a neural basis of elevated cardiovascular and cortisol stress reactivity (173, 177, 178). Future studies are needed to test for a potential neural basis of the observed elevated cardiovascular and cortisol stress reactivity in EHT.
3.2. Hypothalamic and brainstem level
Multiple functional alterations of subcortical structures including hypothalamus, medulla, and pons, have been observed in HT and may relate to the stress reactivity differences with EHT (179, 180). For instance, increased NEP release from subcortical brain regions has been observed in HT as compared to NT (181). This finding is in line with animal studies investigating brain catecholamines and points to a disturbance of catecholaminergic metabolism in the inferior part of the brain in EHT (179, 182, 183). Further, functioning of the hypothalamic paraventricular nucleus involved in sympathetic and parasympathetic control of cardiovascular reactivity, have been proposed to be altered with HT (184, 185). Moreover, investigating CRH-producing neurons of the paraventricular nucleus using post-mortem quantitative immunohistochemical and in situ hybridization techniques, higher numbers of CRH-producing neurons and higher amount of CRH mRNA have been found in EHT as compared to healthy controls (186). Given the central role of CRH in the activation the HPA axis but also in the activation of the SNS (187), the suggested elevated CRH synthesis and release may contribute to the hyperreactivity of the SNS and the HPA axis. However, investigation of associations between stress reactivity on the cerebral level with endocrine and/or cardiovascular stress reactivity in EHT so lacking so far.
3.3. Peripheral level
At the peripheral level, we investigated whether stress hormone reactivity in terms of NEP, EP, and cortisol, contributes to blood lipid and lipoprotein or procoagulant stress reactivity with elevated BP (12, 13). We assessed plasma NEP, EP, cortisol as well as FVII:C, FVIII:C, fibrinogen, D-dimer, and plasma lipid profiles before and +1, +20, and +60 min after cessation of the TSST in hypertensive men as compared to NT (12, 13). We additionally considered the influence of resting mean arterial BP (MAP) as a continuous indicator of hypertension status.
With respect to blood lipid parameter reactivity, we observed that stress-induced NEP increases independently predicted higher immediate stress increases in TC, TG, and LDL-C (13). We hypothesized this association to result from NEP induced lipolysis and release of free fatty acids which in turn may serve as a substrate for the resynthesis of TG and hepatic production of very-low-density lipoprotein cholesterol (13, 86). Moreover, MAP interacted with NEP increases in predicting immediate changes in HDL-C and LDL-C (13). Given this, NEP stress reactivity seems to elicit proatherogenic changes of blood lipids and lipoproteins in response to AMS, either alone or in interaction with MAP, pointing to a further potential mechanism by which stress might increase cardiovascular risk in HT.
With respect to coagulation activity, we observed that NEP changes from rest to immediately after stress independently predicted immediate D-Dimer changes (12). Also, cortisol changes from rest to 10 min after stress independently predicted immediate FVIII:C changes. In terms of associations between integrated reactivity of stress hormone and coagulation factors between rest and 60 min after stress cessation (total stress reactivity), MAP interacted with the integrated release of EP in predicting total stress reactivity of FVII:C and of fibrinogen. MAP further interacted with integrated NEP release in predicting total FVIII:C stress reactivity while D-dimer total stress reactivity was predicted by NEP stress reactivity alone. These findings suggest a sustained hypercoagulability over about 1 h after stress in EHT which may represent a psychobiological mechanism through which stress and hypertension may interact in increasing the risk of atherothrombotic events (188).
Moreover, alterations in adrenergic receptor (AR) number and/or functioning have been observed with EHT (189–191). Notably, these AR alterations may add to the physiological reactivity differences in EHT. With respect to cardiovascular reactivity, β-AR mechanisms do not seem to mediate the higher reactivity in HT as compared to NT (192–194), but we recently found evidence pointing to a role for α-AR mechanisms (195). We investigated α-AR mechanisms in the cardiovascular reactivity to a standardized stress reactivity-mimicking NEP-infusion in EHT as compared to NT by using the non-selective α-AR blocker phentolamine. Non-selective α-AR blockade attenuated DBP hyperreactivity to NEP-infusion observed in HT but not SBP hyperreactivity in HT, suggesting a mediating effect of α-AR in DBP hyperreactivity to stress in EHT. Whether alterations in α- and/or β-AR number and/or functioning is involved in the observed hyperreactivity of other stress-reactive parameters in EHT remains to be elucidated.
In sum, there are several potential mechanisms operating at different levels that may contribute to the altered physiological reactivity to AMS with EHT (for summary see Figure 2). However, the exact mechanisms and their interactions are not fully understood and need to be investigated in more detail.
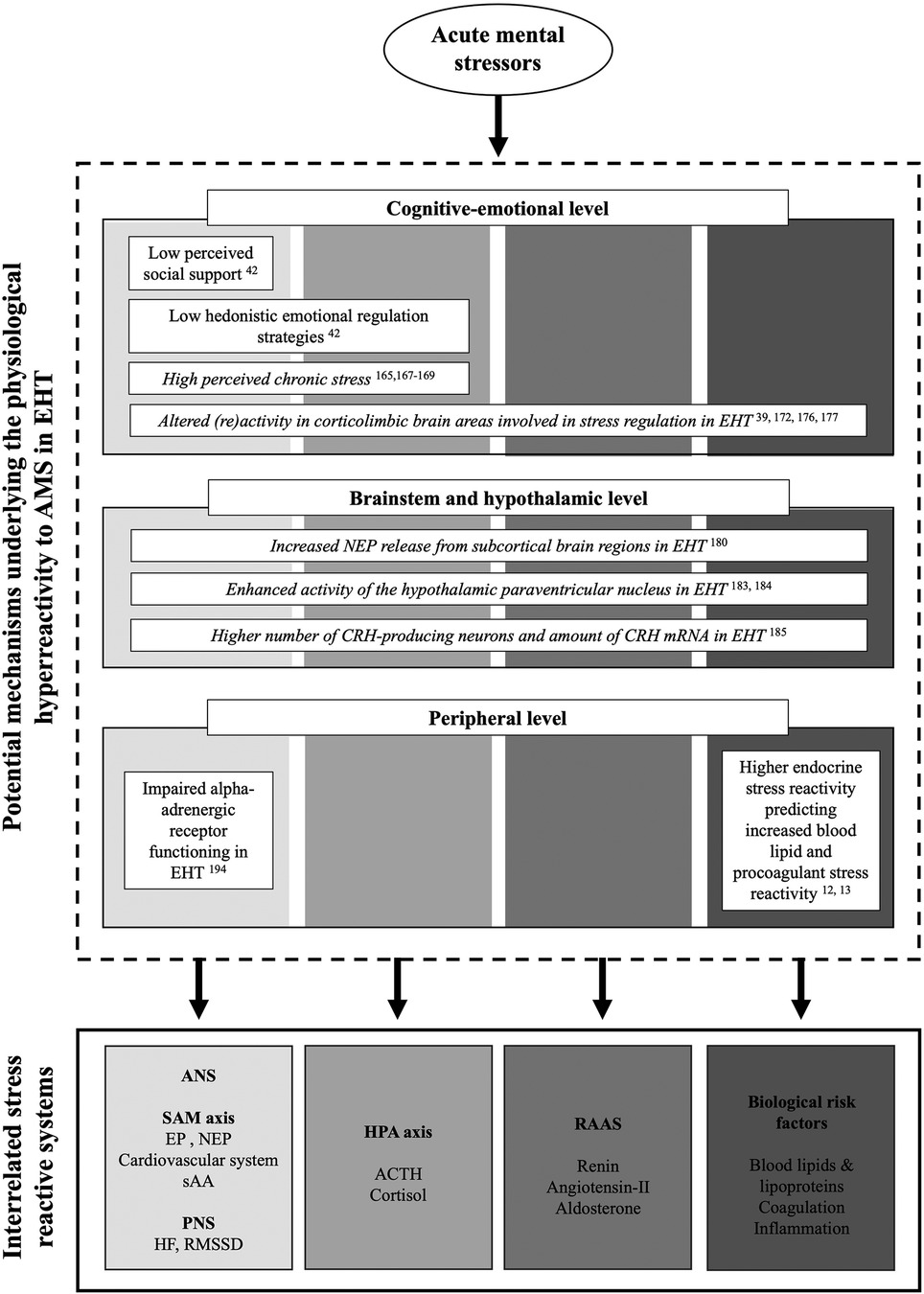
Figure 2. Overview over potential mechanisms underlying the physiological hyperreactivity to acute mental stress in essential hypertension with respect to the cognitive-emotional, brainstem and hypothalamic, as well as peripheral levels and the different stress reactive physiological systems. Potential mechanisms with respective evidence are presented in regular font. Potential suggested mechanisms but not yet investigated in the context of acute stress reactivity are presented in italics.
4. Conclusion and future directions
In this comprehensive systematic review, we summarized the hitherto published evidence on increased physiological reactivity to AMS in EHT as compared to NT with respect to the SAM axis, the HPA axis, the RAAS, as well as stress-reactive intermediate biological risk factors for CVD. Taken together, our review indicates that the expected physiological hyperreactivity to AMS cannot generally (i.e., to mental stressors of all intensities, across all stress-reactive physiological systems, and in all HT) be observed in EHT. In fact, in reaction to acute mental stressors of mild or moderate intensity, as applied by most studies investigating physiological, in particular sympathetic, reactivity to AMS in EHT, results were inconclusive, presumably due to methodological issues. More precisely, our review indicates that exaggerated reactivity to mild AMS occurs only in response to mild mental stressors with specific characteristics, in an early hyperkinetic stage of HT, or with respect to certain stress systems. However, in reaction to acute mental stressors of strong intensity, such as the TSST or the MIST, evidence strongly suggests a physiological hyperreactivity across the investigated stress-reactive physiological systems. Our review revealed that there is considerable variation in the number of studies investigating reactivity to AMS in EHT with respect to the reviewed physiological systems. While reactivity of SAM axis parameters has been extensively studied, there are comparatively few studies examining reactivity to AMS with respect to PNS, HPA axis, or RAAS as well as with respect to reactivity of biological risk factors, pointing to the need for future research. Further, given that the first studies on physiological stress reactivity in EHT were published in the 1970s, the studies included in this review employed a strikingly broad methodology including statistical analysis methods. Future research replicating previous findings or further investigating differences in stress reactivity between HT and NT should apply state-of-the-art methods for hypertension classification, AMS induction, assessment of physiological parameters, as well as statistical analyses. As a limitation, it should be noted that the majority of studies included in our systematic review and the discussion of potential underlying mechanisms of stress hyperreactivity in EHT are cross-sectional studies and do not demonstrate a potential causal role with respect to hypertension severity or cardiovascular risk. Moreover, systematic reviews always entail the risk of publication bias, which means that only studies with significant results have been published.
The mechanisms underlying the observed differences in physiological reactivity to AMS between HT and NT are beginning to be understood and warrant further study. We discussed several potential mechanisms that may contribute to the observed reactivity differences at different levels, the cognitive-emotional level, the hypothalamic and brain stem level, and the peripheral level. Notably, the discussed potential mechanisms mainly come from study findings in samples of healthy NT participating in laboratory experiments and population-based studies, respectively. Further elucidation of the mechanisms in HT as compared to NT subjects is of vital importance, especially with regard to potential therapeutical targets for cardiovascular risk prevention and/or reduction. Concomitantly, potential modulating factors of the physiological hyperreactivity to AMS with EHT remain to be elucidated.
Author contributions
L-MW: investigation and formal analysis (literature search and data synthesis); visualization; writing. PHW: idea and structure of the review article; contribution to investigation and formal analysis (literature search and data synthesis); writing; supervision. All authors contributed to the article and approved the submitted version.
Funding
This work was supported by the German Research Foundation under Germany's Excellence Strategy (EXC2117 – 422037984) (to PHW).
Conflict of interest
The authors declare that the research was conducted in the absence of any commercial or financial relationships that could be construed as a potential conflict of interest.
Publisher's note
All claims expressed in this article are solely those of the authors and do not necessarily represent those of their affiliated organizations, or those of the publisher, the editors and the reviewers. Any product that may be evaluated in this article, or claim that may be made by its manufacturer, is not guaranteed or endorsed by the publisher.
References
1. Roth GA, Mensah GA, Johnson CO, Addolorato G, Ammirati E, Baddour LM, et al. Global burden of cardiovascular diseases and risk factors, 1990–2019. J Am Coll Cardiol. (2020) 76(25):2982–3021. doi: 10.1016/j.jacc.2020.11.010
2. Kjeldsen SE. Hypertension and cardiovascular risk: general aspects. Pharmacol Res. (2018) 129:95–9. doi: 10.1016/j.phrs.2017.11.003
3. Chida Y, Steptoe A. Greater cardiovascular responses to laboratory mental stress are associated with poor subsequent cardiovascular risk status. Hypertension. (2010) 55(4):1026–32. doi: 10.1161/HYPERTENSIONAHA.109.146621
4. Hamer M, Endrighi R, Venuraju SM, Lahiri A, Steptoe A. Cortisol responses to mental stress and the progression of coronary artery calcification in healthy men and women. PLoS One. (2012) 7(2):e31356. doi: 10.1371/journal.pone.0031356
5. Carroll D, Phillips AC, Der G, Hunt K, Benzeval M. Blood pressure reactions to acute mental stress and future blood pressure status: data from the 12-year follow-up of the west of Scotland study. Psychosom Med. (2011) 73(9):737–42. doi: 10.1097/PSY.0b013e3182359808
6. Hamer M, Steptoe A. Cortisol responses to mental stress and incident hypertension in healthy men and women. J Clin Endocrinol Metab. (2012) 97(1):E29–34. doi: 10.1210/jc.2011-2132
7. Halperin RO, Sesso HD, Ma J, Buring JE, Stampfer MJ, Gaziano JM. Dyslipidemia and the risk of incident hypertension in men. Hypertension. (2006) 47(1):45–50. doi: 10.1161/01.HYP.0000196306.42418.0e
8. Harrison DG, Guzik TJ, Lob HE, Madhur MS, Marvar PJ, Thabet SR, et al. Inflammation, immunity, and hypertension. Hypertension. (2011) 57(2):132–40. doi: 10.1161/HYPERTENSIONAHA.110.163576
9. Tabak O, Gelisgen R, Uzun H, Kalender B, Balci H, Curgunlu A, et al. Hypertension and hemostatic/fibrinolytic balance disorders. Clin Invest Med. (2009) 32(6):E285–E92. doi: 10.25011/cim.v32i6.10664
10. Kop WJ, Weissman NJ, Zhu J, Bonsall RW, Doyle M, Stretch MR, et al. Effects of acute mental stress and exercise on inflammatory markers in patients with coronary artery disease and healthy controls. Am J Cardiol. (2008) 101(6):767–73. doi: 10.1016/j.amjcard.2007.11.006
11. von Känel R, Mills PJ, Fainman C, Dimsdale JE. Effects of psychological stress and psychiatric disorders on blood coagulation and fibrinolysis: a biobehavioral pathway to coronary artery disease? Psychosom Med. (2001) 63(4):531–44. doi: 10.1097/00006842-200107000-00003
12. Wirtz PH, Ehlert U, Emini L, Rüdisüli K, Groessbauer S, Mausbach BT, et al. The role of stress hormones in the relationship between resting blood pressure and coagulation activity. J Hypertens. (2006) 24(12):2409–16. doi: 10.1097/HJH.0b013e32801098e5
13. Wirtz PH, Ehlert U, Bärtschi C, Redwine LS, von Känel R. Changes in plasma lipids with psychosocial stress are related to hypertension status and the norepinephrine stress response. Metab Clin Exp. (2009) 58(1):30–7. doi: 10.1016/j.metabol.2008.08.003
14. Fredrikson M, Matthews KA. Cardiovascular responses to behavioral stress and hypertension: a meta-analytic review. Ann Behav Med. (1990) 12(1):30–9. doi: 10.1207/s15324796abm1201_3
15. Manuck SB, Kasprowicz AL, Muldoon MF. Behaviorally-evoked cardiovascular reactivity and hypertension: conceptual issues and potential associations. Ann Behav Med. (1990) 12(1):17–29. doi: 10.1207/s15324796abm1201_2
16. al'Absi M, Arnett DK. Adrenocortical responses to psychological stress and risk for hypertension. Biomed Pharmacother. (2000) 54(5):234–44. doi: 10.1016/S0753-3322(00)80065-7
17. Gideon A, Sauter C, Pruessner JC, Farine DR, Wirtz PH. Determinants and mechanisms of the renin-aldosterone stress response. Psychosom Med. (2022) 84(1):50–63. doi: 10.1097/PSY.0000000000001018
18. Gideon A, Sauter C, Fieres J, Berger T, Renner B, Wirtz PH. Kinetics and interrelations of the renin aldosterone response to acute psychosocial stress: a neglected stress system. J Clin Endocrinol Metab. (2020) 105(3):dgz190. doi: 10.1210/clinem/dgz190
19. Godoy LD, Rossignoli MT, Delfino-Pereira P, Garcia-Cairasco N, de Lima Umeoka EH. A comprehensive overview on stress neurobiology: basic concepts and clinical implications. Front Behav Neurosci. (2018) 12:127. doi: 10.3389/fnbeh.2018.00127
20. Nyklíček I, Bosch JA, Amerongen AVN. A generalized physiological hyperreactivity to acute stressors in hypertensives. Biol Psychol. (2005) 70(1):44–51. doi: 10.1016/j.biopsycho.2004.11.013
21. Williams B, Mancia G, Spiering W, Agabiti Rosei E, Azizi M, Burnier M, et al. ESC/ESH guidelines for the management of arterial hypertension: the task force for the management of arterial hypertension of the European society of cardiology (ESC) and the European society of hypertension (ESH). Eur Heart J. (2018) 39(33):3021–104. doi: 10.1093/eurheartj/ehy339
22. Mills KT, Bundy JD, Kelly TN, Reed JE, Kearney PM, Reynolds K, et al. Global disparities of hypertension prevalence and control: a systematic analysis of population-based studies from 90 countries. Circulation. (2016) 134(6):441–50. doi: 10.1161/CIRCULATIONAHA.115.018912
23. Lim SS, Vos T, Flaxman AD, Danaei G, Shibuya K, Adair-Rohani H, et al. A comparative risk assessment of burden of disease and injury attributable to 67 risk factors and risk factor clusters in 21 regions, 1990-2010: a systematic analysis for the global burden of disease study 2010. Lancet. (2012) 380(9859):2224–60. doi: 10.1016/S0140-6736(12)61766-8
24. Frohlich ED, Apstein C, Chobanian AV, Devereux RB, Dustan HP, Dzau V, et al. The heart in hypertension. N Engl J Med. (1992) 327(14):998–1008. doi: 10.1056/NEJM199210013271406
25. Perk J, De Backer G, Gohlke H, Graham I, Reiner Z, Verschuren M, et al. European guidelines on cardiovascular disease prevention in clinical practice (version 2012). The fifth joint task force of the European society of cardiology and other societies on cardiovascular disease prevention in clinical practice (constituted by representatives of nine societies and by invited experts). Eur Heart J. (2012) 33(13):1635–701. doi: 10.1093/eurheartj/ehs092
26. Mensah GA. Epidemiology and global burden of hypertension. In: Camm AJ, Lüscher TF, Maurer G, Serruys PW, editors. The ESC textbook of cardiovascular medicine. Oxford: Oxford University Press (2018). p. 291–7.
27. Carretero OA, Oparil S. Essential hypertension. Circulation. (2000) 101(3):329–35. doi: 10.1161/01.CIR.101.3.329
29. Everly GS, Lating JM. The anatomy and physiology of the human stress response. In: Everly GS, Lating JM, editors. A clinical guide to the treatment of the human stress response. New York: Springer (2019). p. 19–56.
30. Dhabhar FS, McEwen BS. Acute stress enhances while chronic stress suppresses cell-mediated immunity in vivo: a potential role for leukocyte trafficking. Brain Behav Immun. (1997) 11(4):286–306. doi: 10.1006/brbi.1997.0508
31. Dickerson SS, Kemeny ME. Acute stressors and cortisol responses: a theoretical integration and synthesis of laboratory research. Psychol Bull. (2004) 130(3):355–91. doi: 10.1037/0033-2909.130.3.355
32. Bali A, Jaggi AS. Clinical experimental stress studies: methods and assessment. Rev Neurosci. (2015) 26(5):555–79. doi: 10.1515/revneuro-2015-0004
33. Raven JC, Court JH. Raven’s progressive matrices and vocabulary scales. Oxford: Oxford pyschologists Press (1998).
34. Stroop JR. Studies of interference in serial verbal reactions. J Exp Psychol. (1935) 18(6):643–62. doi: 10.1037/h0054651
35. Al’Absi M, Bongard S, Buchanan T, Pincomb GA, Licinio J, Lovallo WR. Cardiovascular and neuroendocrine adjustment to public speaking and mental arithmetic stressors. Psychophysiology. (1997) 34(3):266–75. doi: 10.1111/j.1469-8986.1997.tb02397.x
36. Kirschbaum C, Pirke K-M, Hellhammer DH. The ‘trier social stress test’–a tool for investigating psychobiological stress responses in a laboratory setting. Neuropsychobiology. (1993) 28(1-2):76–81. doi: 10.1159/000119004
37. Dedovic K, Renwick R, Mahani NK, Engert V, Lupien SJ, Pruessner JC. The Montreal imaging stress task: using functional imaging to investigate the effects of perceiving and processing psychosocial stress in the human brain. J Psychiatry Neurosci. (2005) 30(5):319–25.16151536
38. Romero ML, Butler LK. Endocrinology of stress. Int J Comp Psychol. (2007) 20(2):89–95. doi: 10.46867/IJCP.2007.20.02.15
39. Ulrich-Lai YM, Herman JP. Neural regulation of endocrine and autonomic stress responses. Nat Rev Neurosci. (2009) 10(6):397–409. doi: 10.1038/nrn2647
40. Kuebler U, von Känel R, Heimgartner N, Zuccarella-Hackl C, Stirnimann G, Ehlert U, et al. Norepinephrine infusion with and without alpha-adrenergic blockade by phentolamine increases salivary alpha amylase in healthy men. Psychoneuroendocrinology. (2014) 49:290–8. doi: 10.1016/j.psyneuen.2014.07.023
41. Nater UM, Rohleder N. Salivary alpha-amylase as a non-invasive biomarker for the sympathetic nervous system: current state of research. Psychoneuroendocrinology. (2009) 34(4):486–96. doi: 10.1016/j.psyneuen.2009.01.014
42. Wirtz PH, von Känel R, Mohiyeddini C, Emini L, Ruedisueli K, Groessbauer S, et al. Low social support and poor emotional regulation are associated with increased stress hormone reactivity to mental stress in systemic hypertension. J Clin Endocrinol Metab. (2006) 91(10):3857–65. doi: 10.1210/jc.2005-2586
43. Steptoe A, Marmot M. Psychosocial, hemostatic, and inflammatory correlates of delayed poststress blood pressure recovery. Psychosom Med. (2006) 68(4):531–7. doi: 10.1097/01.psy.0000227751.82103.65
44. Liu X, Ishimatsu K, Sotoyama M, Iwakiri K. Positive emotion inducement modulates cardiovascular responses caused by mental work. J Physiol Anthropol. (2016) 35(1):27. doi: 10.1186/s40101-016-0116-4
45. Cygankiewicz I, Zareba W. Chapter 31—heart rate variability. In: Buijs RM, Swaab DF, editors. Handbook of clinical neurology. vol 117. Amsterdam: Elsevier (2013). p. 379–93.
46. Kuusela T. Methodological aspects of heart rate variability analysis. In: Kamath MV, Watanabe M, Upton A, editors. Heart rate variability (HRV) signal analysis: Clinical applications. Boca Raton, Florida (2013). p. 10–42.
47. Monfredi O, Lyashkov AE, Johnsen A-B, Inada S, Schneider H, Wang R, et al. Biophysical characterization of the underappreciated and important relationship between heart rate variability and heart rate. Hypertension. (2014) 64(6):1334–43. doi: 10.1161/HYPERTENSIONAHA.114.03782
48. Weber CS, Thayer JF, Rudat M, Wirtz PH, Zimmermann-Viehoff F, Thomas A, et al. Low vagal tone is associated with impaired post stress recovery of cardiovascular, endocrine, and immune markers. Eur J Appl Physiol. (2010) 109(2):201–11. doi: 10.1007/s00421-009-1341-x
49. Visnovcova Z, Mestanik M, Javorka M, Mokra D, Gala M, Jurko A, et al. Complexity and time asymmetry of heart rate variability are altered in acute mental stress. Physiol Meas. (2014) 35(7):1319. doi: 10.1088/0967-3334/35/7/1319
50. Taelman J, Vandeput S, Spaepen A, Van Huffel S. Influence of mental stress on heart rate and heart rate variability. 4th European conference of the international federation for medical and biological engineering; Springer (2009). p. 1366–9
51. Charmandari E, Tsigos C, Chrousos G. Endocrinology of the stress response. Annu Rev Physiol. (2005) 67:259–84. doi: 10.1146/annurev.physiol.67.040403.120816
52. Russell G, Lightman S. The human stress response. Nat Rev Endocrinol. (2019) 15(9):525–34. doi: 10.1038/s41574-019-0228-0
53. Sapolsky RM, Romero LM, Munck AU. How do glucocorticoids influence stress responses? Integrating permissive, suppressive, stimulatory, and preparative actions. Endocr Rev. (2000) 21(1):55–89. doi: 10.1210/edrv.21.1.0389
54. Kudielka BM, Wüst S. Human models in acute and chronic stress: assessing determinants of individual hypothalamus–pituitary–adrenal axis activity and reactivity. Stress. (2010) 13(1):1–14. doi: 10.3109/10253890902874913
55. Spencer RL, Deak T. A users guide to HPA axis research. Physiol Behav. (2017) 178:43–65. doi: 10.1016/j.physbeh.2016.11.014
56. Hellhammer DH, Wüst S, Kudielka BM. Salivary cortisol as a biomarker in stress research. Psychoneuroendocrinology. (2009) 34(2):163–71. doi: 10.1016/j.psyneuen.2008.10.026
57. Törnhage CJ. Salivary cortisol for assessment of hypothalamic-pituitary-adrenal axis function. Neuroimmunomodulation. (2009) 16(5):284–9. doi: 10.1159/000216186
58. Stephens MAC, Mahon PB, McCaul ME, Wand GS. Hypothalamic–pituitary–adrenal axis response to acute psychosocial stress: effects of biological sex and circulating sex hormones. Psychoneuroendocrinology. (2016) 66:47–55. doi: 10.1016/j.psyneuen.2015.12.021
59. Kudielka BM, Schommer NC, Hellhammer DH, Kirschbaum C. Acute HPA axis responses, heart rate, and mood changes to psychosocial stress (TSST) in humans at different times of day. Psychoneuroendocrinology. (2004) 29(8):983–92. doi: 10.1016/j.psyneuen.2003.08.009
60. Connell JM, Davies E. The new biology of aldosterone. J Endocrinol. (2005) 186(1):1–20. doi: 10.1677/joe.1.06017
61. Atlas SA. The renin-angiotensin aldosterone system: pathophysiological role and pharmacologic inhibition. J Manag Care Pharm. (2007) 13(8 Suppl B):9–20. doi: 10.18553/jmcp.2007.13.s8-b.9
62. Yatabe J, Yoneda M, Yatabe MS, Watanabe T, Felder RA, Jose PA, et al. Angiotensin III stimulates aldosterone secretion from adrenal gland partially via angiotensin II type 2 receptor but not angiotensin II type 1 receptor. Endocrinology. (2011) 152(4):1582–8. doi: 10.1210/en.2010-1070
63. Kosunen KJ. Plasma renin activity, angiotensin II, and aldosterone after mental arithmetic. Scand J Clin Lab Invest. (1977) 37(5):425–9. doi: 10.3109/00365517709091502
64. von Känel R. Acute mental stress and hemostasis: when physiology becomes vascular harm. Thromb Res. (2015) 135:S52–S5. doi: 10.1016/S0049-3848(15)50444-1
65. Cannon WB, Mendenhall WL. Factors affecting the coagulation time of blood: IV. The hastening of coagulation in pain and emotional excitement. Am J Physiol. (1914) 34(2):251–61. doi: 10.1152/ajplegacy.1914.34.2.251
66. Austin AW, Wissmann T, von Kanel R. Stress and hemostasis: an update. Semin Thromb Hemost. (2013) 39(08):902–12. doi: 10.1055/s-0033-1357487
67. Von Känel R, Dimsdale JE. Effects of sympathetic activation by adrenergic infusions on hemostasis in vivo. Eur J Haematol. (2000) 65(6):357–69. doi: 10.1034/j.1600-0609.2000.065006357.x
68. Hao Z, Jiang X, Sharafeih R, Shen S, Hand AR, Cone RE, et al. Stimulated release of tissue plasminogen activator from artery wall sympathetic nerves: implications for stress-associated wall damage. Stress. (2005) 8(2):141–9. doi: 10.1080/10253890500168098
69. Hoffman M, Monroe DM. A cell-based model of hemostasis. Thromb Haemost. (2001) 85(06):958–65. doi: 10.1055/s-0037-1615947
70. Preckel D, von Känel R. Regulation of hemostasis by the sympathetic nervous system: any contribution to coronary artery disease? Heart Drug. (2004) 4(3):123–30. doi: 10.1159/000078415
71. von Känel R, Preckel D, Zgraggen L, Mischler K, Kudielka BM, Haeberli A, et al. The effect of natural habituation on coagulation responses to acute mental stress and recovery in men. Thromb Haemost. (2004) 92(12):1327–35. doi: 10.1160/TH04-04-0223
72. Thrall G, Lane D, Carroll D, Lip GYH. A systematic review of the effects of acute psychological stress and physical activity on haemorheology, coagulation, fibrinolysis and platelet reactivity: implications for the pathogenesis of acute coronary syndromes. Thromb Res. (2007) 120(6):819–47. doi: 10.1016/j.thromres.2007.01.004
73. von Känel R, Bellingrath S, Kudielka BM. Association of vital exhaustion and depressive symptoms with changes in fibrin D-dimer to acute psychosocial stress. J Psychosom Res. (2009) 67(1):93–101. doi: 10.1016/j.jpsychores.2008.12.009
74. Wirtz PH, Ehlert U, Emini L, Rüdisüli K, Groessbauer S, Gaab J, et al. Anticipatory cognitive stress appraisal and the acute procoagulant stress response in men. Psychosom Med. (2006) 68(6):851–8. doi: 10.1097/01.psy.0000245866.03456.aa
75. Wirtz PH, Ehlert U, Emini L, Rüdisüli K, Groessbauer S, von Känel R. Procoagulant stress reactivity and recovery in apparently healthy men with systolic and diastolic hypertension. J Psychosom Res. (2007) 63(1):51–8. doi: 10.1016/j.jpsychores.2007.01.014
76. Stoney CM, West S. Lipids, personality, and stress: mechanisms and modulators. In: Hillbrand M, Spitz RT, editors. Lipids, health, and behavior. Washington, DC, United States: American Psychological Association (1997). p. 47–66.
77. Grant N, Hamer M, Steptoe A. Social isolation and stress-related cardiovascular, lipid, and cortisol responses. Ann Behav Med. (2009) 37(1):29–37. doi: 10.1007/s12160-009-9081-z
78. Stoney CM, Finney ML. Social support and stress: influences on lipid reactivity. Int J Behav Med. (2000) 7(2):111–26. doi: 10.1207/S15327558IJBM0702_2
79. Stoney CM, Bausserman L, Niaura R, Marcus B, Flynn M. Lipid reactivity to stress: II. Biological and behavioral influences. Health Psychol. (1999) 18:251–61. doi: 10.1037/0278-6133.18.3.251
80. Bachen EA, Muldoon MF, Matthews KA, Manuck SB. Effects of hemoconcentration and sympathetic activation on serum lipid responses to brief mental stress. Psychosom Med. (2002) 64(4):587–94. doi: 10.1097/00006842-200207000-00008
81. Degroote C, von Känel R, Thomas L, Zuccarella-Hackl C, Pruessner JC, Wiest R, et al. Acute stress-induced blood lipid reactivity in hypertensive and normotensive men and prospective associations with future cardiovascular risk. J Clin Med. (2021) 10(15):3400. doi: 10.3390/jcm10153400
82. Stoney CM, Matthews KA, Mcdonald RH, Johnson CA. Sex differences in lipid, lipoprotein, cardiovascular, and neuroendocrine responses to acute stress. Psychophysiology. (1988) 25(6):645–56. doi: 10.1111/j.1469-8986.1988.tb01902.x
83. Allen M, Patterson S. Hemoconcentration and stress: a review of physiological mechanisms and relevance for cardiovascular disease risk. Biol Psychol. (1995) 41(1):1–27. doi: 10.1016/0301-0511(95)05123-R
84. Austin AW, Patterson S, von Känel R. Hemoconcentration and hemostasis during acute stress: interacting and independent effects. Ann Behav Med. (2011) 42(2):153–73. doi: 10.1007/s12160-011-9274-0
85. Dill DB, Costill DL. Calculation of percentage changes in volumes of blood, plasma, and red cells in dehydration. J Appl Physiol. (1974) 37(2):247–8. doi: 10.1152/jappl.1974.37.2.247
86. Arner P. Human fat cell lipolysis: biochemistry, regulation and clinical role. Best Pract Res Clin Endocrinol Metab. (2005) 19(4):471–82. doi: 10.1016/j.beem.2005.07.004
87. Brindley DN, McCann BS, Niaura R, Stoney CM, Suarez EC. Stress and lipoprotein metabolism: modulators and mechanisms. Metab Clin Exp. (1993) 42(9, Supplement 1):3–15. doi: 10.1016/0026-0495(93)90255-M
88. Marsland AL, Walsh C, Lockwood K, John-Henderson NA. The effects of acute psychological stress on circulating and stimulated inflammatory markers: a systematic review and meta-analysis. Brain Behav Immun. (2017) 64:208–19. doi: 10.1016/j.bbi.2017.01.011
89. Steptoe A, Hamer M, Chida Y. The effects of acute psychological stress on circulating inflammatory factors in humans: a review and meta-analysis. Brain Behav Immun. (2007) 21(7):901–12. doi: 10.1016/j.bbi.2007.03.011
90. Segerstrom SC, Miller GE. Psychological stress and the human immune system: a meta-analytic study of 30 years of inquiry. Psychol Bull. (2004) 130(4):601. doi: 10.1037/0033-2909.130.4.601
91. Oliver JC, Bland L, Oettinger CW, Arduino MJ, McAllister S, Aguero S, et al. Cytokine kinetics in an in vitro whole blood model following an endotoxin challenge. Lymphokine Cytokine Res. (1993) 12(2):115–20.8324076
92. Wirtz PH, von Känel R. Psychological stress, inflammation, and coronary heart disease. Curr Cardiol Rep. (2017) 19(11):111. doi: 10.1007/s11886-017-0919-x
93. Meyer T, Wirtz PH. Mechanisms of mitochondrial redox signaling in psychosocial stress-responsive systems: new insights into an old story. Antioxid Redox Signal. (2018) 28(9):760–72. doi: 10.1089/ars.2017.7186
94. Wolf JM, Rohleder N, Bierhaus A, Nawroth PP, Kirschbaum C. Determinants of the NF-κB response to acute psychosocial stress in humans. Brain Behav Immun. (2009) 23(6):742–9. doi: 10.1016/j.bbi.2008.09.009
95. Kunz-Ebrecht SR, Mohamed-Ali V, Feldman PJ, Kirschbaum C, Steptoe A. Cortisol responses to mild psychological stress are inversely associated with proinflammatory cytokines. Brain Behav Immun. (2003) 17(5):373–83. doi: 10.1016/S0889-1591(03)00029-1
96. Flaa A, Eide IK, Kjeldsen SE, Rostrup M. Sympathoadrenal stress reactivity is a predictor of future blood pressure. Hypertension. (2008) 52(2):336–41. doi: 10.1161/HYPERTENSIONAHA.108.111625
97. Page MJ, McKenzie JE, Bossuyt PM, Boutron I, Hoffmann TC, Mulrow CD, et al. The PRISMA 2020 statement: an updated guideline for reporting systematic reviews. Int J Surg. (2021) 88:105906. doi: 10.1016/j.ijsu.2021.105906
98. Flaa A, Mundal HH, Eide I, Kjeldsen S, Rostrup M. Sympathetic activity and cardiovascular risk factors in young men in the low, normal, and high blood pressure ranges. Hypertension. (2006) 47(3):396–402. doi: 10.1161/01.HYP.0000203952.27988.79
99. Garafova A, Penesova A, Cizmarova E, Marko A, Vlcek M, Jezova D. Cardiovascular and sympathetic responses to a mental stress task in young patients with hypertension and/or obesity. Physiol Res. (2014) 63:S459. doi: 10.33549/physiolres.932931
100. Kawabe H, Saito I, Hasegawa C, Nagano S, Saruta T. Circulatory and plasma catecholamine responses to mental stress in young subjects with two different types of hypertension. Angiology. (1994) 45(6):435–41. doi: 10.1177/0003319794045006043
101. Lenders JW, Willemsen JJ, de Boo T, Lemmens WA, Thien T. Disparate effects of mental stress on plasma noradrenaline in young normotensive and hypertensive subjects. J Hypertens. (1989) 7(4):317–23. doi: 10.1097/00004872-198904000-00012
102. Lindvall K, Kahan T, De U, Östergren J, Hjemdahl P. Stress-induced changes in blood pressure and left ventricular function in mild hypertension. Clin Cardiol. (1991) 14(2):125–32. doi: 10.1002/clc.4960140208
103. Matsukawa T, Gotoh E, Uneda S, Miyajima E, Shionoiri H, Tochikubo O, et al. Augmented sympathetic nerve activity in response to stressors in young borderline hypertensive men. Acta Physiol Scand. (1991) 141(2):157–65. doi: 10.1111/j.1748-1716.1991.tb09064.x
104. Perini C, Müller FB, Rauchfleisch U, Battegay R, Bühler FR. Effects of psychological and physical covariates on plasma catecholamines in borderline hypertensives and offspring of hypertensive parents. Clin Exp Hypertens A. (1990) 12(2):137–50. doi: 10.3109/10641969009074724
105. Eliasson K, Hjemdahl P, Kahan T. Circulatory and sympatho-adrenal responses to stress in borderline and established hypertension. J Hypertens. (1983) 1(2):131–9. doi: 10.1097/00004872-198308000-00004
106. Palermo A, Bertalero P, Pizza N, Amelotti R, Libretti A. Decreased fibrinolytic response to adrenergic stimulation in hypertensive patients. J Hypertens Suppl. (1989) 7(6):S162–3. doi: 10.1097/00004872-198900076-00077
107. Tomoda F, Takata M, Kagitani S, Kinuno H, Yasumoto K, Tomita S, et al. Different platelet aggregability during mental stress in two stages of essential hypertension. Am J Hypertens. (1999) 12(11):1063–70. doi: 10.1016/S0895-7061(99)00131-4
108. Bahlmann A, Brod J, Cachovan M. Stress-induced changes of the venous circulation. Contrib Nephrol. (1981) 30:43–8. doi: 10.1159/000406417
109. Baumann R, Ziprian H, Gödicke W, Hartrodt W, Naumann E, Läuter J. The influence of acute psychic stress situations on biochemical and vegetative parameters of essential hypertensives at the early stage of the disease. Psychother Psychosom. (1973) 22(2-6):131–40. doi: 10.1159/000286484
110. Brod J, Cachovan M, Bahlmann J, Bauer GE, Celsen B, Sippel R, et al. Haemodynamic changes during acute emotional stress in man with special reference to the capacitance vessels. Klin Wochenschr. (1979) 57(11):555–65. doi: 10.1007/BF01491134
111. Drummond PD. Cardiovascular reactivity in mild hypertension. J Psychosom Res. (1983) 27(4):291–7. doi: 10.1016/0022-3999(83)90051-X
112. Drummond PD. Cardiovascular reactivity in borderline hypertensives during behavioural and orthostatic stress. Psychophysiology. (1985) 22(6):621–8. doi: 10.1111/j.1469-8986.1985.tb01656.x
113. Fredrikson M, Dimberg U, Frisk-Holmberg M. Arterial blood pressure and electrodermal activity in hypertensive and normotensive subjects during inner- and outer-directed attention. Acta Med Scand Suppl. (1981) 646:73–6. doi: 10.1111/j.0954-6820.1981.tb02624.x
114. Georgiades A, Lemne C, Faire U, Lindvall K, Fredrikson M. Stress-induced laboratory blood pressure in relation to ambulatory blood pressure and left ventricular mass among borderline hypertensive and normotensive individuals. Hypertension. (1996) 28(4):641–6. doi: 10.1161/01.HYP.28.4.641
115. Itoh H, Takeda K, Nakamura K, Fujita H, Uchida A, Kuwahara T, et al. Young borderline hypertensives are hyperreactive to mental arithmetic stress: spectral analysis of R-R intervals. J Auton Nerv Syst. (1995) 54(2):155–62. doi: 10.1016/0165-1838(95)00008-L
116. Köhler T, Fricke M, Ritz T, Scherbaum N. Psychophysiological reactivity of borderline hypertensives and their recovery after mental stress. Psychother Psychosom. (1997) 66(5):261–7. doi: 10.1159/000289145
117. Langewitz W, Rüddel H, Schächinger H. Reduced parasympathetic cardiac control in patients with hypertension at rest and under mental stress. Am Heart J. (1994) 127(1):122–8. doi: 10.1016/0002-8703(94)90517-7
118. Lindqvist M, Kahan T, Melcher A, Hjemdahl P. Cardiovascular and sympatho-adrenal responses to mental stress in primary hypertension. Clin Sci. (1993) 85(4):401–9. doi: 10.1042/cs0850401
119. Reims H, Sevre K, Fossum E, Høieggen A, Eide I, Kjeldsen S. Plasma catecholamines, blood pressure responses and perceived stress during mental arithmetic stress in young men. Blood Press. (2004) 13(5):287–94. doi: 10.1080/08037050410016474
120. Schmieder RE, Rüddel H, Schächinger H, Bruns J, Schulte W. Renal hemodynamics and cardiovascular reactivity in the prehypertensive stage. Behav Med. (1993) 19(1):5–12. doi: 10.1080/08964289.1993.9937558
121. Schulte W, Neus H. Hemodynamics during emotional stress in borderline and mild hypertension. Eur Heart J. (1983) 4(11):803–9. doi: 10.1093/oxfordjournals.eurheartj.a061401
122. Schulte W, Neus H, Thönes M, von Eiff AW. Basal blood pressure variability and reactivity of blood pressure to emotional stress in essential hypertension. Basic Res Cardiol. (1984) 79(1):9–16. doi: 10.1007/BF01935802
123. Shapiro AP, Moutsos SE, Krifcher E. Patterns of pressor response to noxious stimuli in nornmal, hypertensive, and diabetic subjects. J Clin Invest. (1963) 42(12):1890–8. doi: 10.1172/JCI104874
124. Steptoe A, Melville D, Ross A. Behavioral response demands, cardiovascular reactivity, and essential hypertension. Psychosom Med. (1984) 46(1):33–48. doi: 10.1097/00006842-198401000-00006
125. Tuomisto MT. Intra-arterial blood pressure and heart rate reactivity to behavioral stress in normotensive, borderline, and mild hypertensive men. Health Psychol. (1997) 16(6):554–65. doi: 10.1037/0278-6133.16.6.554
126. Markovic N, Matthews KA, Huston SL, Egbagbe E, Ukoli FAM, Bunker CH. Blood pressure reactivity to stress varies by hypertensive status and sex in Nigerians. Am J Epidemiol. (1995) 142(10):1020–8. doi: 10.1093/oxfordjournals.aje.a117554
127. Murakami E, Matsuzaki K, Sumimoto T, Mukai M, Kazatani Y, Kodama K. Clinical significance of pressor responses to laboratory stressor testing in hypertension. Hypertens Res. (1996) 19(2):133–7. doi: 10.1291/hypres.19.133
128. Walther L-M, von Känel R, Zuccarella-Hackl C, Wirtz PH. Hyperreactivity of salivary alpha-amylase to acute psychosocial stress and norepinephrine infusion in essential hypertension. Biomedicines. (2022) 10(7):1762. doi: 10.3390/biomedicines10071762
129. Fossum E, Høieggen A, Reims H, Moan A, Rostrup M, Eide I, et al. High screening blood pressure is related to sympathetic nervous system activity and insulin resistance in healthy young men. Blood Press. (2004) 13(2):89–94. doi: 10.1080/08037050310031008
130. Graafsma SJ, van Tits LJ, van Heijst P, Reyenga J, Lenders JW, Rodrigues de Miranda JF, et al. Adrenoceptors on blood cells in patients with essential hypertension before and after mental stress. J Hypertens. (1989) 7(7):519–24. doi: 10.1097/00004872-198907000-00002
131. Hjemdahl P, Eliasson K. Sympatho-adrenal and cardiovascular response to mental stress and orthostatic provocation in latent hypertension. Clin Sci. (1979) 57(Suppl 5):189s–91s. doi: 10.1042/cs057189s
132. Januszewicz W, Sznajderman M, Wocial B, Feltynowski T, Klonowicz T. The effect of mental stress on catecholamines, their metabolites and plasma renin activity in patients with essential hypertension and in healthy subjects. Clin Sci. (1979) 57(s5):229s–31s. doi: 10.1042/cs057229s
133. Köhler T, Scherbaum N, Ritz T. Psychophysiological responses of borderline hypertensives in two experimental situations. Psychother Psychosom. (1995) 63(1):44–53. doi: 10.1159/000288936
134. Carroll D, Harris MG, Cross G. Haemodynamic adjustments to mental stress in normotensives and subjects with mildly elevated blood pressure. Psychophysiology. (1991) 28(4):438–46. doi: 10.1111/j.1469-8986.1991.tb00728.x
135. Esler MD, Nestel PJ. Renin and sympathetic nervous system responsiveness to adrenergic stimuli in essential hypertension. Am J Cardiol. (1973) 32(5):643–9. doi: 10.1016/S0002-9149(73)80057-8
136. Fredrikson M, Dimberg U, Frisk-Holmberg M, Ström G. Haemodynamic and electrodermal correlates of psychogenic stimuli in hypertensive and normotensive subjects. Biol Psychol. (1982) 15(1-2):63–73. doi: 10.1016/0301-0511(82)90031-X
137. Hollenberg NK, Williams GH, Adams DF. Essential hypertension: abnormal renal vascular and endocrine responses to a mild psychological stimulus. Hypertension. (1981) 3(1):11–7. doi: 10.1161/01.HYP.3.1.11
138. Naqvi TZ, Hyuhn HK. Cerebrovascular mental stress reactivity is impaired in hypertension. Cardiovasc Ultrasound. (2009) 7:32. doi: 10.1186/1476-7120-7-32
139. Scheuch K, Hanefeld M, Gräßler J, Seibt R, Naumann HJ. Hypertriglyceridaemia in mild hypertension: impact on cardiovascular and hormonal reactivity under different stress tests. J Hum Hypertens. (1999) 13(8):533–9. doi: 10.1038/sj.jhh.1000877
140. Seibt R, Boucsein W, Scheuch K. Effects of different stress settings on cardiovascular parameters and their relationship to daily life blood pressure in normotensives, borderline hypertensives and hypertensives. Ergonomics. (1998) 41(5):634–48. doi: 10.1080/001401398186801
141. Ducher M, Bertram D, Pozet N, Laville M, Fauvel JP. Stress-induced renal alterations in normotensives offspring of hypertensives and in hypertensives. Am J Hypertens. (2002) 15(4):346–50. doi: 10.1016/S0895-7061(01)02333-0
142. Horikoshi Y, Tajima I, Igarashi H, Inui M, Kasahara K, Noguchi T. The adreno-sympathetic system, the genetic predisposition to hypertension, and stress. Am J Med Sci. (1985) 289(5):186–91. doi: 10.1097/00000441-198505000-00002
143. Sullivan P, Schoentgen S, DeQuattro V, Procci W, Levine D, Meulen J, et al. Anxiety, anger, and neurogenic tone at rest and in stress in patients with primary hypertension. Hypertension. (1981) 3(6_pt_2):II-119. doi: 10.1161/01.HYP.3.6_Pt_2.II-119
144. Dimsdale JE, Mills P, Ziegler M, Leitz K, Nelesen R. Converting enzyme inhibition and blood pressure reactivity to psychological stressors. Hypertension. (1992) 20(2):210–3. doi: 10.1161/01.HYP.20.2.210
145. Fredrikson M, Dimberg U, Frisk-Holmberg M, Störm G. Arterial blood pressure and general sympathetic activation in essential hypertension during stimulation. Acta Med Scand. (1985) 217(3):309–17. doi: 10.1111/j.0954-6820.1985.tb02701.x
146. Ruediger H, Seibt R, Scheuch K, Krause M, Alam S. Sympathetic and parasympathetic activation in heart rate variability in male hypertensive patients under mental stress. J Hum Hypertens. (2004) 18(5):307–15. doi: 10.1038/sj.jhh.1001671
147. Seipäjärvi SM, Tuomola A, Juurakko J, Rottensteiner M, Rissanen A-PE, Kurkela JL, et al. Measuring psychosocial stress with heart rate variability-based methods in different health and age groups. Physiol Meas. (2022) 43(5):055002. doi: 10.1088/1361-6579/ac6b7c
148. Al’Absi M, Wittmers LE. Enhanced adrenocortical responses to stress in hypertension-prone men and women. Ann Behav Med. (2003) 25(1):25–33. doi: 10.1207/S15324796ABM2501_04
149. Gideon A, Sauter C, Ehlert U, von Känel R, Wirtz PH. Aldosterone hyperreactivity to acute psychosocial stress induction in men with essential hypertension. Horm Behav. (2021) 134:105018. doi: 10.1016/j.yhbeh.2021.105018
150. von Känel R, Mills PJ, Ziegler MG, Dimsdale JE. Effect of β2-adrenergic receptor functioning and increased norepinephrine on the hypercoagulable state with mental stress. Am Heart J. (2002) 144(1):68–72. doi: 10.1067/mhj.2002.123146
151. Julius S. Autonomic nervous system dysregulation in human hypertension. Am J Cardiol. (1991) 67(10):B3–7. doi: 10.1016/0002-9149(91)90813-Z
152. Esler MD. Catecholamines and essential hypertension. Baillieres Clin Endocrinol Metab. (1993) 7(2):415–38. doi: 10.1016/S0950-351X(05)80182-X
153. Julius S, Majahalme S. The changing face of sympathetic overactivity in hypertension. Ann Med. (2000) 32(5):365–70. doi: 10.3109/07853890008995939
154. Mestanik M, Mestanikova A, Visnovcova Z, Calkovska A, Tonhajzerova I. Cardiovascular sympathetic arousal in response to different mental stressors. Physiol res. (2015) 64:S585. doi: 10.33549/physiolres.933217
155. Arsalidou M, Taylor MJ. Is 2+2 = 4? Meta-analyses of brain areas needed for numbers and calculations. NeuroImage. (2011) 54(3):2382–93. doi: 10.1016/j.neuroimage.2010.10.009
156. MacLeod CM, MacDonald PA. Interdimensional interference in the stroop effect: uncovering the cognitive and neural anatomy of attention. Trends Cogn Sci. (2000) 4(10):383–91. doi: 10.1016/S1364-6613(00)01530-8
157. Sevre K, Lefrandt JD, Nordby G, Os I, Mulder M, Gans ROB, et al. Autonomic function in hypertensive and normotensive subjects. Hypertension. (2001) 37(6):1351–6. doi: 10.1161/01.HYP.37.6.1351
158. Yu Y, Xu Y, Zhang M, Wang Y, Zou W, Gu Y. Value of assessing autonomic nervous function by heart rate variability and heart rate turbulence in hypertensive patients. Int J Hypertens. (2018) 2018:4067601. doi: 10.1155/2018/4067601
159. Djordjevic J, Vuckovic T, Jasnic N, Cvijic G. Effect of various stressors on the blood ACTH and corticosterone concentration in normotensive Wistar and spontaneously hypertensive Wistar-Kyoto rats. Gen Comp Endocrinol. (2007) 153(1):217–20. doi: 10.1016/j.ygcen.2007.02.004
160. Roman O, Seres J, Pometlova M, Jurcovicova J. Neuroendocrine or behavioral effects of acute or chronic emotional stress in Wistar Kyoto (WKY) and spontaneously hypertensive (SHR) rats. Endocr Regul. (2004) 38(4):151–5.15841794
161. Mills PJ, Farag NH, Hong S, Kennedy BP, Berry CC, Ziegler MG. Immune cell CD62l and CD11a expression in response to a psychological stressor in human hypertension. Brain Behav Immun. (2003) 17(4):260–7. doi: 10.1016/S0889-1591(03)00055-2
162. Steptoe A, Willemsen G, Owen N, Flower L, Mohamed-Ali V. Acute mental stress elicits delayed increases in circulating inflammatory cytokine levels. Clin Sci. (2001) 101(2):185–92. doi: 10.1042/cs1010185
163. Brydon L, Edwards S, Jia H, Mohamed-Ali V, Zachary I, Martin JF, et al. Psychological stress activates interleukin-1β gene expression in human mononuclear cells. Brain Behav Immun. (2005) 19(6):540–6. doi: 10.1016/j.bbi.2004.12.003
164. Lovallo WR, Gerin W. Psychophysiological reactivity: mechanisms and pathways to cardiovascular disease. Psychosom Med. (2003) 65(1):36–45. doi: 10.1097/01.PSY.0000033128.44101.C1
165. Jorgensen RS, Johnson BT, Kolodziej ME, Schreer GE. Elevated blood pressure and personality: a meta-analytic review. Psychol Bull. (1996) 120(2):293. doi: 10.1037/0033-2909.120.2.293
166. Spruill TM, Butler MJ, Thomas SJ, Tajeu GS, Kalinowski J, Castañeda SF, et al. Association between high perceived stress over time and incident hypertension in black adults: findings from the Jackson heart study. J Am Heart Assoc. (2019) 8(21):e012139. doi: 10.1161/JAHA.119.012139
167. Harding BN, Hawley CN, Kalinowski J, Sims M, Muntner P, Young BA, et al. Relationship between social support and incident hypertension in the Jackson heart study: a cohort study. BMJ Open. (2022) 12(3):e054812. doi: 10.1136/bmjopen-2021-054812
168. Roohafza H, Sattari N, Nouri F, Talaei M, Masoumi G, Sarrafzadegan N, et al. Do any kinds of perceived stressors lead to hypertension? A longitudinal cohort study. Hypertens Res. (2022) 45(6):1058–66. doi: 10.1038/s41440-022-00895-3
169. Low CA, Salomon K, Matthews KA. Chronic life stress, cardiovascular reactivity, and subclinical cardiovascular disease in adolescents. Psychosom Med. (2009) 71(9):927–31. doi: 10.1097/PSY.0b013e3181ba18ed
170. Fleming I, Baum A, Davidson LM, Rectanus E, McArdle S. Chronic stress as a factor in physiologic reactivity to challenge. Health Psychol. (1987) 6(3):221–37. doi: 10.1037/0278-6133.6.3.221
171. Myers B. Corticolimbic regulation of cardiovascular responses to stress. Physiol Behav. (2017) 172:49–59. doi: 10.1016/j.physbeh.2016.10.015
172. Jennings JR, Zanstra Y. Is the brain the essential in hypertension? NeuroImage. (2009) 47(3):914–21. doi: 10.1016/j.neuroimage.2009.04.072
173. Pruessner JC, Dedovic K, Pruessner M, Lord C, Buss C, Collins L, et al. Stress regulation in the central nervous system: evidence from structural and functional neuroimaging studies in human populations - 2008 curt Richter award winner. Psychoneuroendocrinology. (2010) 35(1):179–91. doi: 10.1016/j.psyneuen.2009.02.016
174. Nagai M, Hoshide S, Kario K. The insular cortex and cardiovascular system: a new insight into the brain-heart axis. J Am Soc Hypertens. (2010) 4(4):174–82. doi: 10.1016/j.jash.2010.05.001
175. Yoris A, Abrevaya S, Esteves S, Salamone P, Lori N, Martorell M, et al. Multilevel convergence of interoceptive impairments in hypertension: new evidence of disrupted body–brain interactions. Hum Brain Mapp. (2018) 39(4):1563–81. doi: 10.1002/hbm.23933
176. Feng R, Rolls ET, Cheng W, Feng J. Hypertension is associated with reduced hippocampal connectivity and impaired memory. EBioMedicine. (2020) 61:103082. doi: 10.1016/j.ebiom.2020.103082
177. Gianaros PJ, Sheu LK. A review of neuroimaging studies of stressor-evoked blood pressure reactivity: emerging evidence for a brain-body pathway to coronary heart disease risk. NeuroImage. (2009) 47(3):922–36. doi: 10.1016/j.neuroimage.2009.04.073
178. Wheelock MD, Harnett NG, Wood KH, Orem TR, Granger DA, Mrug S, et al. Prefrontal cortex activity is associated with biobehavioral components of the stress response. Front Hum Neurosci. (2016) 10:583. doi: 10.3389/fnhum.2016.00583
179. Wardener HED. The hypothalamus and hypertension. Physiol Rev. (2001) 81(4):1599–658. doi: 10.1152/physrev.2001.81.4.1599
180. Goncharuk VD. Chapter 24—the hypothalamus and its role in hypertension. In: Swaab DF, Buijs RM, Kreier F, Lucassen PJ, Salehi A, editors. Handbook of clinical neurology. Vol 182. Amsterdam: Elsevier (2021). p. 333–54.
181. Ferrier C, Jennings GL, Eisenhofer G, Lambert G, Cox HS, Kalff V, et al. Evidence for increased noradrenaline release from subcortical brain regions in essential hypertension. J Hypertens. (1993) 11(11):1217–27. doi: 10.1097/00004872-199311000-00009
182. Kawasaki S, Takeda K, Tanaka M, Itoh H, Hirata M, Nakata T, et al. Enhanced norepinephrine release in hypothalamus from locus coeruleus in SHR. Jpn Heart J. (1991) 32(2):255–62. doi: 10.1536/ihj.32.255
183. Kaehler ST, Sinner C, Philippu A. Release of catecholamines in the locus coeruleus of freely moving and anaesthetized normotensive and spontaneously hypertensive rats: effects of cardiovascular changes and tail pinch. Naunyn Schmiedebergs Arch Pharmacol. (2000) 361(4):433–9. doi: 10.1007/s002109900210
184. Dyavanapalli J, Dergacheva O, Wang X, Mendelowitz D. Parasympathetic vagal control of cardiac function. Curr Hypertens Rep. (2016) 18(3):22. doi: 10.1007/s11906-016-0630-0
185. Benarroch EE. Paraventricular nucleus, stress response, and cardiovascular disease. Clin Auton Res. (2005) 15(4):254–63. doi: 10.1007/s10286-005-0290-7
186. Goncharuk VD, Van Heerikhuize J, Swaab DF, Buijs RM. Paraventricular nucleus of the human hypothalamus in primary hypertension: activation of corticotropin-releasing hormone neurons. J Comp Neurol. (2002) 443(4):321–31. doi: 10.1002/cne.10124
188. Catena C, Novello M, Lapenna R, Baroselli S, Colussi G, Nadalini E, et al. New risk factors for atherosclerosis in hypertension: focus on the prothrombotic state and lipoprotein(a). J Hypertens. (2005) 23(9):1617–31. doi: 10.1097/01.hjh.0000178835.33976.e7
189. Laurent S, Boutouyrie P. The structural factor of hypertension. Circ Res. (2015) 116(6):1007–21. doi: 10.1161/CIRCRESAHA.116.303596
190. Michel MC, Brodde OE, Insel PA. Peripheral adrenergic receptors in hypertension. Hypertension. (1990) 16(2):107–20. doi: 10.1161/01.HYP.16.2.107
191. Brodde OE, Michel MC. Adrenergic receptors and their signal transduction mechanisms in hypertension. J Hypertens Suppl. (1992) 10(7):S133–45. doi: 10.1097/00004872-199212000-00015
192. Heidbreder E, Pagel G, Röckel A, Heidland A. Beta-adrenergic blockade in stress protection. Limited effect of metoprolol in psychological stress reaction. Eur J Clin Pharmacol. (1978) 14(6):391–8. doi: 10.1007/BF00716379
193. Floras JS, Hassan MO, Jones JV, Sleight P. Cardioselective and nonselective beta-adrenoceptor blocking drugs in hypertension: a comparison of their effect on blood pressure during mental and physical activity. J Am Coll Cardiol. (1985) 6(1):186–95. doi: 10.1016/S0735-1097(85)80273-4
194. Nyberg G, Graham R, Stokes G. The effect of mental arithmetic in normotensive and hypertensive subjects, and its modification by beta-adrenergic receptor blockade. Br J Clin Pharmacol. (1977) 4(4):469–74. doi: 10.1111/j.1365-2125.1977.tb00764.x
Keywords: essential hypertension, physiological stress reactivity, acute mental stress, systematic review, stress
Citation: Walther L-M and Wirtz PH (2023) Physiological reactivity to acute mental stress in essential hypertension—a systematic review. Front. Cardiovasc. Med. 10:1215710. doi: 10.3389/fcvm.2023.1215710
Received: 2 May 2023; Accepted: 27 July 2023;
Published: 11 August 2023.
Edited by:
Gaurav Kumar, Medical College of Wisconsin, United StatesReviewed by:
Stefan Gross, University Medicine Greifswald, GermanyAravind Parthasarathy, Medical College of Wisconsin, United States
Daisuke Kume, Osaka Institute of Technology, Japan
© 2023 Walther and Wirtz. This is an open-access article distributed under the terms of the Creative Commons Attribution License (CC BY). The use, distribution or reproduction in other forums is permitted, provided the original author(s) and the copyright owner(s) are credited and that the original publication in this journal is cited, in accordance with accepted academic practice. No use, distribution or reproduction is permitted which does not comply with these terms.
*Correspondence: Petra H. Wirtz cGV0cmEud2lydHpAdW5pLWtvbnN0YW56LmRl