- Beth Israel Deaconess Medical Center, Harvard Medical School, Boston, MA, United States
Sepsis is a life-threatening disease state characterized by organ dysfunction and a dysregulated response to infection. The heart is one of the many organs affected by sepsis, in an entity termed sepsis-induced cardiomyopathy. This was initially used to describe a reversible depression in ejection fraction with ventricular dilation but advances in echocardiography and introduction of new techniques such as speckle tracking have led to descriptions of other common abnormalities in cardiac function associated with sepsis. This includes not only depression of systolic function, but also supranormal ejection fraction, diastolic dysfunction, and right ventricular dysfunction. These reports have led to inconsistent definitions of sepsis-induced cardiomyopathy. Just as there is heterogeneity among patients with sepsis, there is heterogeneity in the cardiac response; thus resuscitating these patients with a single approach is likely suboptimal. Many factors affect the heart in sepsis including inflammatory mediators, catecholamine responsiveness, and pathogen related toxins. This review will discuss different functional effects characterized by echocardiographic changes in sepsis and their prognostic and management implications.
1. Introduction
Sepsis is a life-threatening disease state characterized by organ dysfunction and a dysregulated response during infection, with a mortality rate of approximately 27% worldwide (1). The heart is one of many organs affected in sepsis, in an entity called sepsis-induced cardiomyopathy, or sepsis-induced myocardial dysfunction, which occurs in 10%–70% of patients with sepsis (2). This range in incidence is wide likely due to differing definitions and echocardiographic techniques used to assess function, timing of echocardiography assessment during the clinical course, and the potential dynamic nature of cardiac functional changes. Fluid resuscitation, inotrope and vasopressor therapy, and mechanical ventilation can alter venous return, cardiac contractility, pulmonary vascular resistance, and left ventricular afterload, which affect traditionally measured echocardiographic parameters. In the 1980s Parker and colleagues demonstrated reversible depression in left ventricular ejection fraction (LVEF) and ventricular dilation in a subset of patients with sepsis (3). These characteristics are used most frequently to describe sepsis-induced myocardial dysfunction (3, 4). Subsequently, with advances in echocardiography, this term has expanded and other cardiac abnormalities are described and included such as diastolic dysfunction, right ventricular (RV) failure, and supranormal LVEF (Figure 1). Although no formal definition exists, sepsis-induced myocardial dysfunction is generally characterized as an acute process that is not caused by acute coronary syndrome and is typically reversible in seven to ten days which manifests as biventricular systolic and/or diastolic dysfunction with left ventricular dilation that is poorly responsive to fluid and catecholamines (5).
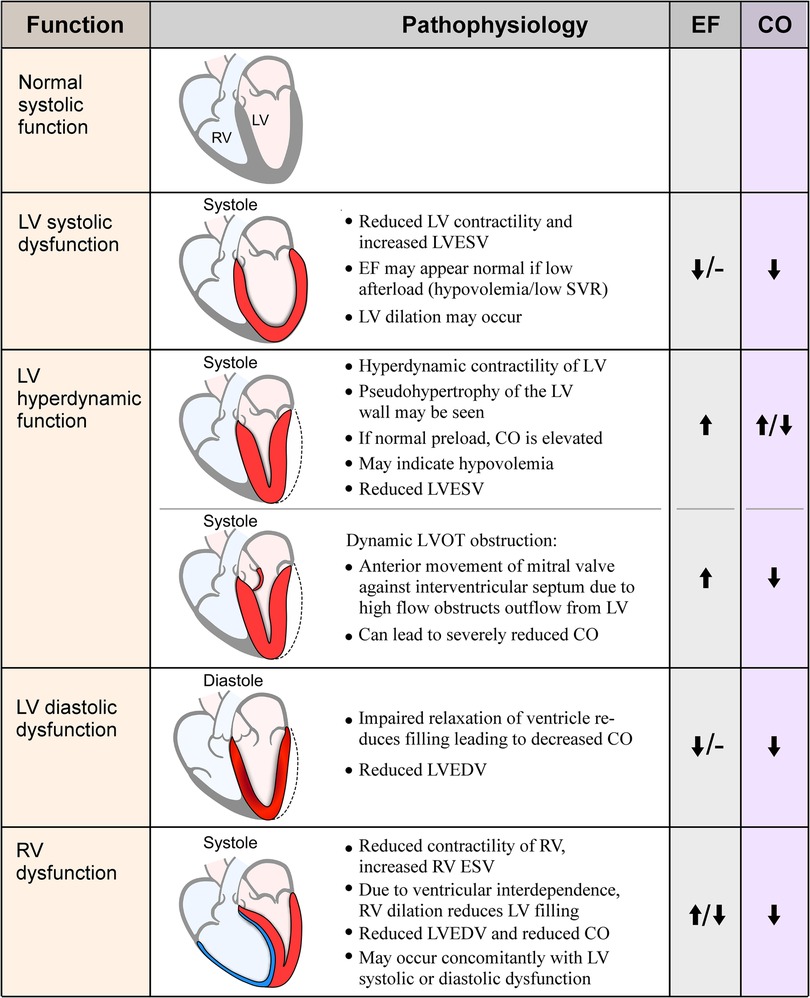
Figure 1. Visual representation and pathophysiology of echocardiographic findings in sepsis. EF, ejection fraction; CO, cardiac output; RV, right ventricle; LV, left ventricle; LVESV, left ventricular end-systolic volume; SVR, systemic vascular resistance; LVOT, left ventricular outflow tract; LVEDV, left ventricular end-diastolic volume; RVESV, right ventricular end-systolic volume.
There are many events that occur during sepsis affecting cardiac performance and with advances in echocardiography we see considerable heterogeneity in this group. There is potential benefit to a more individualized approach to the hemodynamic resuscitation of this diverse patient group. In addition to reviewing echocardiographic features observed in patients with sepsis, this review will discuss their implications for treatment and prognostication as well as possible mechanisms for the various cardiac responses. Patient and disease specific factors may result in the differences seen in the cardiac responses to sepsis, and an understanding of these should help drive a more individualized approach to care.
2. Echocardiographic features in patients with sepsis
2.1. Depressed left ventricular systolic function
While the typical clinical picture of the heart in sepsis is a hyperdynamic state with elevated cardiac output (CO), since the 1950s it was recognized that a low CO syndrome exists in some patients (6, 7). Studies have outlined numerous mechanisms to explain the reduction of CO and LV systolic function: inflammatory factors (e.g., Toll-like receptors and interleukins), mitochondrial damage, abnormal calcium handling, catecholamine desensitization, and aberration of coronary microvascular blood flow due to endothelium abnormalities, neutrophil aggregation, and procoagulant factors (8–17).
In the 1980's Parker and colleagues published findings introducing the concept of LV dilation and LVEF depression in a subset of patients with sepsis that reversed to normal within two weeks as patients recovered (3, 4). In those studies, patients with ventricular dilation and lower LVEF had lower mortality compared to patients who did not have these features. This finding was also observed in animal studies (18). The theory behind this finding is that CO is maintained in the setting of low EF by dilation of the ventricles to maintain stroke volume (SV), provided that volume resuscitation is sufficient (3). Other authors also observed reduced LV end-diastolic volume (LVEDV) in non-survivors despite adequate fluid loading; however, these authors did not observe LV dilation comparable to the Parker studies in survivors (19). The concept of ventricular dilation was subsequently challenged, as the restrictive nature of the pericardium should limit significant ventricular dilation (20). Subsequent studies failed to reproduce these findings (19, 20). This discrepancy may be due to differences in techniques used to measure LVEF and CO, however it remains unclear whether a lower LVEF is cardioprotective or represents a deleterious state (19, 20).
Most recent studies use echocardiography to evaluate LV systolic function, using <40%–50% as a definition for decreased LVEF. Studies have yielded mixed results with respect to outcomes. Some studies found that decreased LVEF was associated with increased mortality (21), others reported improved survival (19), and others found no difference in survival based on LVEF (22–24). A 2013 meta-analysis showed no difference in survival based on presence of low LVEF or LV dilation (25).
A challenge with using LVEF is its dependence on preload and afterload conditions. A patient with significant contractile dysfunction can appear to have a normal LVEF in a setting of hypovolemia or low mean arterial pressure, as is often the case in sepsis. LVEF can change rapidly and significantly depending on arterial pressure and volume status, without reflecting the change in true contractility of the myocardium (26). Thus, it is not surprising that studies based on LVEF have shown such variability in prognostication.
The more recently developed speckle-tracking echocardiography (SPE) is less dependent on loading conditions and is potentially more reflective of intrinsic myocardial function (27). SPE shows myocardium movement by tracking ultrasound echoes in the myocardium throughout the cardiac cycle. Strain is the difference between the length at rest and the final length, and represents contractility (28). There are differences in global longitudinal strain (GLS) values depending on the vendor and software so at this time there is no uniform consensus, but normal is around −20% per the American Society of Echocardiography, with less negative numbers indicating decreased contractility (29). Studies on GLS as a predictor of outcome have shown more uniform results than LVEF, with a recent meta-analysis showing that lower strain (less negative values) i.e., lower contractility, is associated with higher mortality (28, 30). In this same study LVEF was not associated with mortality differences. GLS is more sensitive than LVEF, and declining cardiac contractility by GLS is observed before LVEF or decreased CO is apparent (28). With earlier identification of dysfunction, even at a subclinical level, the question remains of how to interpret and integrate this into management decisions, and further research is needed on the significance of these findings.
There is little evidence to guide management of patients with depressed systolic function. It is an unresolved question whether the dysfunction is deleterious or protective, and accordingly how aggressively clinicians should intervene to improve systolic function in the acute setting, as opposed to treating the underlying sepsis and allowing function to recover over time. In patients with sepsis-induced myocardial dysfunction and ongoing hypoperfusion after adequate volume resuscitation the Surviving Sepsis Campaign guidelines suggest inotropic therapy, with either addition of dobutamine to norepinephrine, or epinephrine alone, based on low quality of evidence (31). Dobutamine therapy is associated with improved cardiac function parameters (32), but numerous studies including network meta-analyses show varying results with respect to mortality benefit (32–37). Use of dobutamine to achieve supranormal cardiac index has shown harm (38). Prior studies were performed in a heterogeneous septic shock population, without classification or selection by echocardiography, so it is possible there is a subgroup of patients with decreased cardiac contractility that would benefit from inotropic medications. There is an ongoing trial of dobutamine for treatment of patients with septic shock and septic cardiomyopathy with reduced LVEF which may provide clarity (NCT04166331).
2.2. Hyperdynamic left ventricular systolic function
The hyperdynamic heart with low systemic vascular resistance is considered the typical cardiac response to sepsis. Hyperdynamic cardiac function can be a compensation for vasoplegia or insufficient intravascular volume, but can also be seen in a hyperadrenergic state from excess endogenous or exogenous catecholamine stimulation.
Investigations of hyperdynamic LV function show increased mortality (20, 39, 40). When comparing groups with low, normal, and supranormal LVEF, Chotalia and colleagues found in a study of just over one thousand patients that those with supranormal (greater than 70%) LVEF had worse outcomes compared to those with normal or even low LVEF (39). In this study a supranormal EF was associated with lower SVR so it is possible this finding represented a group with persistent vasoplegia which was potentially the driver of mortality. These patients also had higher heart rates, so the hyperdynamic state may have resulted from excessive catecholamine release. It is a plausible hypothesis that this subset of hyperdynamic patients was the reason that those with lower EF seemed to fare better in comparison in earlier studies.
While tachycardia does not necessarily indicate a hyperdynamic LVEF, there is an association seen (39). Beta-blockers have been studied to reduce heart rate and increase filling time to improve SV in patients with inappropriate tachycardia. In a meta-analysis of 7 studies including 613 patients, the use of short-acting beta-blockers esmolol or landiolol in septic patients with tachycardia after resuscitation resulted in a decreased mortality (41). While these studies do not specifically investigate patients with hyperdynamic LVEF, beta blockers may represent a potential therapy for hyperdynamic LVEF but further research is warranted. There is concern that beta blockade can suppress compensatory tachycardia in the setting of low preload, causing a drop in CO and worsening hemodynamics. A potential approach to optimize risks and benefits is to identify which patients are persistently tachycardic due to hyperadrenergic states vs. due to inadequate preload, and administer targeted beta-blocker therapy only to the hyperadrenergic population. One such suggested method is using strain echocardiography, as patients with tachycardia in the setting of high preload were observed to have worse LV strain values, which identifies a subpopulation with tachycardia due to hyperadrenergic state (42).
A specific finding in some patients with hyperdynamic cardiac function is dynamic left ventricular outflow tract obstruction (LVOTO), where blood rapidly flowing through an underfilled ventricle causes anterior motion of the mitral valve and obstruction of flow through the outflow tract, similar to the obstruction seen in hypertrophic obstructive cardiomyopathy (Figure 1). LVOTO is observed in 22%–30% of septic shock patients and can occur due to high catecholamine states, inotrope administration, and hypovolemia, and typically indicates fluid responsiveness; studies show an association between LVOTO and mortality (43, 44). There is an important clinical implication as catecholamine administration typically worsens the obstruction, resulting in unintended worsening of hemodynamic status. Authors have suggested using vasopressin instead of adrenergic agents in these patients, with one small study finding hemodynamic and respiratory improvement using vasopressin and down-titrating norepinephrine in patients with severe LVOTO (45). Beta-blockers are also a plausible treatment for this patient group, as they are recommended for treatment of LVOTO in other conditions (46, 47). There are few data on their use in the septic population specifically but are a promising therapy (48–50). None-the-less, the optimal approach is in need of further research.
2.3. Left ventricular diastolic dysfunction
Sepsis can lead to abnormal ventricular relaxation due to elevated catecholamines, tachycardia, and abnormalities of calcium uptake in the sarcoplasmic reticulum which impairs the active relaxation process in cardiac myocytes (51). In the healthy heart, frequency-dependent acceleration of relaxation is observed during tachycardia to maintain ventricular filling. In sepsis this can become deranged due to disruption of normal calcium transients, and result in inadequate filling (51). Excessive or rapid fluid loading can also cause impaired diastolic function and elevated filling pressures (52). Diminished relaxation of the ventricle leads to reduced filling time, decreasing SV and coronary perfusion. Diastolic dysfunction is seen in almost half of patients with sepsis (53).
Diastolic dysfunction in sepsis is commonly assessed measuring doppler flow through the mitral valve at early diastole (E) and late diastole (A), the ratio of E/A, or by using tissue doppler imaging (TDI) to measure the velocity of the myocardium at the lateral or septal aspect of the mitral valve annulus (e'). The ratio of E/e' is used as a measurement of filling pressure (54). Lateral e' <10 cm/s and septal e'< 7 cm/s indicate dysfunction (55). The ratio of E/e' ≥13–15 is used as a measurement of elevated filling pressure (54). Although there is concern for loading conditions affecting parameters of diastolic function, TDI, particularly the lateral e' measurement, seems to be relatively unaffected by preload alterations (56). A meta-analysis of 16 studies shows an association between higher mortality and lower e' values and higher E/e' ratios which are both indicative of diastolic dysfunction (23, 53, 57).
Patients with diastolic dysfunction are particularly prone to worsening hemodynamics in the setting of tachycardia and reduced filling time (58). This group may benefit from heart rate reduction in the setting of inappropriate tachycardia but as mentioned previously more research is needed to identify these patients. Reduced compliance of the LV may make fluid loading potentially harmful in this group. If filling pressures are elevated and LV cannot relax and fill adequately in diastole to augment SV, pressure increases can cause pulmonary vascular congestion (53). However, diastolic dysfunction does not necessarily indicate fluid nonresponsiveness. In fact in one study, patients with diastolic dysfunction who were fluid responsive had improvement of LV relaxation as evidenced by an increase in the early diastolic mitral annular velocity E', compared to those who were not fluid responsive, when challenged with volume expansion (59). Since relaxation is an energy dependent process, this improvement may be from enhanced coronary artery perfusion from an increased SV (59). Thus, patients with diastolic dysfunction may particularly benefit from assessment of fluid responsiveness.
2.4. Right ventricular dysfunction
Many factors affect the RV during sepsis, in addition to those that affect the LV as described earlier. Pulmonary vasoconstriction can occur with hypoxia, hypercarbia, and acidosis which are common in sepsis (60–62). Systemic hypotension can be further detrimental and cause pulmonary vasoconstriction due to adrenergic stimulation (60). Sepsis contributes to pulmonary vasoconstriction due to elevation of factors such as endotoxin, endothelin, thromboxane, and IL-6 (63). Mechanical ventilation, increased positive end expiratory pressure, and acute respiratory distress syndrome (ARDS) also increase RV afterload. Since the RV is used to a low pressure, high compliance circuit of the normal pulmonary vasculature, it is not well equipped to handle large increases in afterload, so these factors can contribute to RV dysfunction.
Echocardiographic assessment of RV function in sepsis is conventionally defined as tricuspid annular plane systolic excursion (TAPSE) <1.6cm, TDI S'<10cm/s, increased RV/LV size ratio, and fractional area change (FAC) <35% (64). Using strain echocardiography, RV function can be assessed globally with GLS, or by RV free wall strain which does not include interventricular septal function which is affected by the LV. Although studies suggest increased prognostic value of strain imaging for the RV, it is not yet in widespread use and the choice of imaging remains unresolved (65–68). RV dysfunction is observed in approximately one third to one half of patients with sepsis and septic shock, even up to 72% using STE (67–70). Higher mortality is observed in septic patients with RV dysfunction, with a study of 393 ICU patients showing 31% 28-day mortality compared to 16% in those without RV dysfunction (67) Consistent with this, in a study of 252 Emergency Department patients with sepsis, Innocenti et al. observed a 44% 28-day mortality in patients with RV dysfunction compared to 23% in those without (70).
RV dysfunction has therapeutic implications as well. Although fluids are recommended in sepsis to maintain adequate preload, clinicians should administer fluids with caution as a dilated RV with poor systolic function and high afterload will often have difficulty accommodating an increase in volume. RV dysfunction is a demonstrated predictor of a lower probability of fluid responsiveness, so high volume fluid resuscitation is likely less helpful in these patients which supports prioritization of vasopressors in this population; albeit, there is a lack of definitive clinical data supporting this hypothesis (71).
Although vasoactive agents such as epinephrine and norepinephrine have been shown to increase pulmonary vascular resistance in in-vitro studies (72, 73) their effects in vivo are less clear. Norepinephrine is the first line vasopressor recommended in sepsis. Norepinephrine increases RV systolic function without a detrimental effect on pulmonary vascular resistance which is a favorable profile for use in patients with RV dysfunction (31, 74, 75). Vasopressin has a neutral or even vasodilatory response on the pulmonary vasculature and may be a good option as well in patients with RV dysfunction, but more data are needed (76–78). There is a paucity of evidence for how to treat RV dysfunction in sepsis and this represents an important opportunity for research.
3. Future directions: reducing heterogeneity in sepsis
Recent efforts have focused on defining subgroups of patients within the sepsis population with the goal of identifying targeted therapeutic approaches. Several groups have attempted to define subgroups using clinical data such as demographics, organ dysfunction, vital signs, and laboratory values (79–82). Others have investigated gene expression, showing differential expression of genes related to innate and adaptive immune cell function, endotoxin tolerance, and metabolic pathways (83–86). Similar approaches are implemented in other pathologies such as ARDS, with identification of a hyperinflammatory and a hypoinflammatory subphenotype, yielding important information about response to management strategies based on subphenotype (87, 88).
A recent cluster-based study of patients with sepsis by Geri and colleagues aimed to identify cardiovascular phenotypes, using a combination of echocardiographic and clinical data to determine five cardiovascular clusters (89). In this study of 360 patients, the groups were: (a) “well resuscitated” group: no dysfunction and not fluid responsive, (b) LV systolic dysfunction group: low LVEF and cardiac index, high lactate levels and norepinephrine doses, and fluid nonresponsive, (c) hyperkinetic group: elevated LVEF and not fluid responsive, (d) RV failure group: normal or high LVEF, fluid unresponsive and associated with lower PaO2/FiO2 ratios, and (e) hypovolemic group: increased LVEF and fluid responsive despite receipt of the largest amount of fluids in this group (89). Understanding differences between cardiac responses to sepsis and how to best treat them may help inform a more individualized hemodynamic resuscitation. For instance, limiting fluids in patients with LV systolic dysfunction and RV dysfunction, using inotropes only for those patients with systolic dysfunction, prioritizing vasopressin in RV dysfunction, and using beta blockers and ensuring adequate fluid resuscitation for hyperdynamic function and LVOTO, are all theoretical ways to optimize cardiac function in sepsis, but more data are needed.
4. Conclusion
In summary, there are several cardiac abnormalities in sepsis identified by echocardiography. Questions remain regarding the best diagnostic and management strategies of sepsis-induced myocardial dysfunction. Are our current definitions the best way to define sepsis-induced myocardial dysfunction? Can identification of cardiac subphenotypes to guide resuscitation result in better outcomes? A better understanding of individual patient responses in the sepsis state is an exciting potential for individualized and targeted care. This, combined with evolving technological echocardiographic assessment of the heart, holds potential for future transformative personalized approaches to resuscitation in sepsis.
Author contributions
TS: conceptualization, writing—original draft. NS: conceptualization, writing—review and editing. All authors contributed to the article and approved the submitted version.
Conflict of interest
The authors declare that the research was conducted in the absence of any commercial or financial relationships that could be construed as a potential conflict of interest.
Publisher's note
All claims expressed in this article are solely those of the authors and do not necessarily represent those of their affiliated organizations, or those of the publisher, the editors and the reviewers. Any product that may be evaluated in this article, or claim that may be made by its manufacturer, is not guaranteed or endorsed by the publisher.
References
1. Fleischmann-Struzek C, Mellhammar L, Rose N, Cassini A, Rudd KE, Schlattmann P, et al. Incidence and mortality of hospital- and icu-treated sepsis: results from an updated and expanded systematic review and meta-analysis. Intensive Care Med. (2020) 46(8):1552–62. doi: 10.1007/s00134-020-06151-x
2. Hollenberg SM, Singer M. Pathophysiology of sepsis-induced cardiomyopathy. Nat Rev Cardiol. (2021) 18(6):424–34. doi: 10.1038/s41569-020-00492-2
3. Parker MM, Shelhamer JH, Bacharach SL, Green MV, Natanson C, Frederick TM, et al. Profound but reversible myocardial depression in patients with septic shock. Ann Intern Med. (1984) 100(4):483–90. doi: 10.7326/0003-4819-100-4-483
4. Parker MM. Responses of left ventricular function in survivors and nonsurvivors of septic shock. J Crit Care. (1989) 4(1):19–25. doi: 10.1016/0883-9441(89)90087-7
5. L'Heureux M, Sternberg M, Brath L, Turlington J, Kashiouris MG. Sepsis-Induced cardiomyopathy: a comprehensive review. Curr Cardiol Rep. (2020) 22(5):35. doi: 10.1007/s11886-020-01277-2
6. Hess ML, Hastillo A, Greenfield LJ. Spectrum of cardiovascular function during gram-negative sepsis. Prog Cardiovasc Dis. (1981) 23(4):279–98. doi: 10.1016/0033-0620(81)90017-7
7. Waisbren BA. Bacteremia due to gram-negative Bacilli other than the Salmonella; a clinical and therapeutic study. AMA Arch Intern Med. (1951) 88(4):467–88. doi: 10.1001/archinte.1951.03810100051005
8. Nemoto S, Vallejo JG, Knuefermann P, Misra A, Defreitas G, Carabello BA, et al. Escherichia Coli lps-induced lv dysfunction: role of toll-like receptor-4 in the adult heart. Am J Physiol Heart Circ Physiol. (2002) 282(6):H2316–23. doi: 10.1152/ajpheart.00763.2001
9. Cain BS, Meldrum DR, Dinarello CA, Meng X, Joo KS, Banerjee A, et al. Tumor necrosis factor-alpha and interleukin-1beta synergistically depress human myocardial function. Crit Care Med. (1999) 27(7):1309–18. doi: 10.1097/00003246-199907000-00018
10. Kumar A, Thota V, Dee L, Olson J, Uretz E, Parrillo JE. Tumor necrosis factor alpha and interleukin 1beta are responsible for in vitro myocardial cell depression induced by human septic shock Serum. J Exp Med. (1996) 183(3):949–58. doi: 10.1084/jem.183.3.949
11. Crouser ED. Mitochondrial dysfunction in septic shock and multiple organ dysfunction syndrome. Mitochondrion. (2004) 4(5-6):729–41. doi: 10.1016/j.mito.2004.07.023
12. Shan J, Xie W, Betzenhauser M, Reiken S, Chen BX, Wronska A, et al. Calcium leak through ryanodine receptors leads to atrial fibrillation in 3 mouse models of catecholaminergic polymorphic ventricular tachycardia. Circ Res. (2012) 111(6):708–17. doi: 10.1161/CIRCRESAHA.112.273342
13. Bernardin G, Strosberg AD, Bernard A, Mattei M, Marullo S. Beta-Adrenergic receptor-dependent and -independent stimulation of adenylate cyclase is impaired during severe sepsis in humans. Intensive Care Med. (1998) 24(12):1315–22. doi: 10.1007/s001340050768
14. Lockyer JM, Colladay JS, Alperin-Lea WL, Hammond T, Buda AJ. Inhibition of nuclear factor-kappab-mediated adhesion molecule expression in human endothelial cells. Circ Res. (1998) 82(3):314–20. doi: 10.1161/01.res.82.3.314
15. Raeburn CD, Calkins CM, Zimmerman MA, Song Y, Ao L, Banerjee A, et al. Icam-1 and vcam-1 mediate endotoxemic myocardial dysfunction independent of neutrophil accumulation. Am J Physiol Regul Integr Comp Physiol. (2002) 283(2):R477–86. doi: 10.1152/ajpregu.00034.2002
16. Rittirsch D, Flierl MA, Ward PA. Harmful molecular mechanisms in sepsis. Nat Rev Immunol. (2008) 8(10):776–87. doi: 10.1038/nri2402
17. Semeraro F, Ammollo CT, Morrissey JH, Dale GL, Friese P, Esmon NL, et al. Extracellular histones promote thrombin generation through platelet-dependent mechanisms: involvement of platelet Tlr2 and Tlr4. Blood. (2011) 118(7):1952–61. doi: 10.1182/blood-2011-03-343061
18. Zanotti Cavazzoni SL, Guglielmi M, Parrillo JE, Walker T, Dellinger RP, Hollenberg SM. Ventricular dilation is associated with improved cardiovascular performance and survival in sepsis. Chest. (2010) 138(4):848–55. doi: 10.1378/chest.09-1086
19. Jardin F, Fourme T, Page B, Loubieres Y, Vieillard-Baron A, Beauchet A, et al. Persistent preload defect in severe sepsis despite fluid loading: a longitudinal echocardiographic study in patients with septic shock. Chest. (1999) 116(5):1354–9. doi: 10.1378/chest.116.5.1354
20. Vieillard Baron A, Schmitt JM, Beauchet A, Augarde R, Prin S, Page B, et al. Early preload adaptation in septic shock? A transesophageal echocardiographic study. Anesthesiology. (2001) 94(3):400–6. doi: 10.1097/00000542-200103000-00007
21. Hanumanthu BKJ, Nair AS, Katamreddy A, Gilbert JS, You JY, Offor OL, et al. Sepsis-Induced cardiomyopathy is associated with higher mortality rates in patients with sepsis. Acute Crit Care. (2021) 36(3):215–22. doi: 10.4266/acc.2021.00234
22. Pulido JN, Afessa B, Masaki M, Yuasa T, Gillespie S, Herasevich V, et al. Clinical Spectrum, frequency, and significance of myocardial dysfunction in severe sepsis and septic shock. Mayo Clin Proc. (2012) 87(7):620–8. doi: 10.1016/j.mayocp.2012.01.018
23. Furian T, Aguiar C, Prado K, Ribeiro RV, Becker L, Martinelli N, et al. Ventricular dysfunction and dilation in severe sepsis and septic shock: relation to endothelial function and mortality. J Crit Care. (2012) 27(3):319, e9-15.. doi: 10.1016/j.jcrc.2011.06.017
24. Landesberg G, Gilon D, Meroz Y, Georgieva M, Levin PD, Goodman S, et al. Diastolic dysfunction and mortality in severe sepsis and septic shock. Eur Heart J. (2012) 33(7):895–903. doi: 10.1093/eurheartj/ehr351
25. Huang SJ, Nalos M, McLean AS. Is early ventricular dysfunction or dilatation associated with lower mortality rate in adult severe sepsis and septic shock? A meta-analysis. Crit Care. (2013) 17(3):R96. doi: 10.1186/cc12741
26. Cikes M, Solomon SD. Beyond ejection fraction: an integrative approach for assessment of cardiac structure and function in heart failure. Eur Heart J. (2016) 37(21):1642–50. doi: 10.1093/eurheartj/ehv510
27. De Geer L, Engvall J, Oscarsson A. Strain echocardiography in septic shock—a comparison with systolic and diastolic function parameters, cardiac biomarkers and outcome. Crit Care. (2015) 19:122. doi: 10.1186/s13054-015-0857-1
28. Sanfilippo F, Corredor C, Fletcher N, Tritapepe L, Lorini FL, Arcadipane A, et al. Left ventricular systolic function evaluated by strain echocardiography and relationship with mortality in patients with severe sepsis or septic shock: a systematic review and meta-analysis. Crit Care. (2018) 22(1):183. doi: 10.1186/s13054-018-2113-y
29. Lang RM, Badano LP, Mor-Avi V, Afilalo J, Armstrong A, Ernande L, et al. Recommendations for cardiac chamber quantification by echocardiography in adults: an update from the American society of echocardiography and the European association of cardiovascular imaging. Eur Heart J Cardiovasc Imaging. (2015) 16(3):233–70. doi: 10.1093/ehjci/jev014
30. Palmieri V, Innocenti F, Guzzo A, Guerrini E, Vignaroli D, Pini R. Left ventricular systolic longitudinal function as predictor of outcome in patients with sepsis. Circ Cardiovasc Imaging. (2015) 8(11):e003865. doi: 10.1161/CIRCIMAGING.115.003865
31. Evans L, Rhodes A, Alhazzani W, Antonelli M, Coopersmith CM, French C, et al. Surviving sepsis campaign: international guidelines for management of sepsis and septic shock 2021. Crit Care Med. (2021) 49(11):e1063–e143. doi: 10.1097/CCM.0000000000005337
32. Nadeem R, Sockanathan S, Singh M, Hussain T, Kent P, AbuAlreesh S. Impact of dobutamine in patients with septic shock: a meta-regression analysis. Am J Ther. (2017) 24(3):e333–e46. doi: 10.1097/MJT.0000000000000272
33. Wilkman E, Kaukonen KM, Pettila V, Kuitunen A, Varpula M. Association between inotrope treatment and 90-day mortality in patients with septic shock. Acta Anaesthesiol Scand. (2013) 57(4):431–42. doi: 10.1111/aas.12056
34. Hernandez G, Bruhn A, Luengo C, Regueira T, Kattan E, Fuentealba A, et al. Effects of dobutamine on systemic, regional and microcirculatory perfusion parameters in septic shock: a randomized, placebo-controlled, double-blind, crossover study. Intensive Care Med. (2013) 39(8):1435–43. doi: 10.1007/s00134-013-2982-0
35. Gattinoni L, Brazzi L, Pelosi P, Latini R, Tognoni G, Pesenti A, et al. A trial of goal-oriented hemodynamic therapy in critically ill patients. Svo2 collaborative group. N Engl J Med. (1995) 333(16):1025–32. doi: 10.1056/NEJM199510193331601
36. Oba Y, Lone NA. Mortality benefit of vasopressor and inotropic agents in septic shock: a Bayesian network meta-analysis of randomized controlled trials. J Crit Care. (2014) 29(5):706–10. doi: 10.1016/j.jcrc.2014.04.011
37. Belletti A, Benedetto U, Biondi-Zoccai G, Leggieri C, Silvani P, Angelini GD, et al. The effect of vasoactive drugs on mortality in patients with severe sepsis and septic shock. A network meta-analysis of randomized trials. J Crit Care. (2017) 37:91–8. doi: 10.1016/j.jcrc.2016.08.010
38. Hayes MA, Timmins AC, Yau EH, Palazzo M, Hinds CJ, Watson D. Elevation of systemic oxygen delivery in the treatment of critically Ill patients. N Engl J Med (1994) 330(24):1717–22. doi: 10.1056/NEJM199406163302404
39. Chotalia M, Ali M, Hebballi R, Singh H, Parekh D, Bangash MN, et al. Hyperdynamic left ventricular ejection fraction in icu patients with sepsis. Crit Care Med. (2022) 50(5):770–9. doi: 10.1097/CCM.0000000000005315
40. Paonessa JR, Brennan T, Pimentel M, Steinhaus D, Feng M, Celi LA. Hyperdynamic left ventricular ejection fraction in the intensive care unit. Crit Care. (2015) 19(1):288. doi: 10.1186/s13054-015-1012-8
41. Hasegawa D, Sato R, Prasitlumkum N, Nishida K, Takahashi K, Yatabe T, et al. Effect of ultrashort-acting Beta-blockers on mortality in patients with sepsis with persistent tachycardia despite initial resuscitation: a systematic review and meta-analysis of randomized controlled trials. Chest. (2021) 159(6):2289–300. doi: 10.1016/j.chest.2021.01.009
42. Lanspa MJ, Shahul S, Hersh A, Wilson EL, Olsen TD, Hirshberg EL, et al. Associations among left ventricular systolic function, tachycardia, and cardiac preload in septic patients. Ann Intensive Care. (2017) 7(1):17. doi: 10.1186/s13613-017-0240-2
43. Elhadidy S. Dynamic left intraventricular obstruction in patients with septic shock: pathogenic role and prognostic implications. Research and Opinion in Anesthesia & Intensive Care. (2019) 6(4):424–8. doi: 10.4103/roaic.roaic_40_19
44. Chauvet JL, El-Dash S, Delastre O, Bouffandeau B, Jusserand D, Michot JB, et al. Early dynamic left intraventricular obstruction is associated with hypovolemia and high mortality in septic shock patients. Crit Care. (2015) 19:262. doi: 10.1186/s13054-015-0980-z
45. Balik M, Novotny A, Suk D, Matousek V, Maly M, Brozek T, et al. Vasopressin in patients with septic shock and dynamic left ventricular outflow tract obstruction. Cardiovasc Drugs Ther. (2020) 34(5):685–8. doi: 10.1007/s10557-020-06998-8
46. Ommen SR, Mital S, Burke MA, Day SM, Deswal A, Elliott P, et al. 2020 Aha/acc guideline for the diagnosis and treatment of patients with hypertrophic cardiomyopathy: executive summary: a report of the American college of cardiology/American heart association joint committee on clinical practice guidelines. J Am Coll Cardiol. (2020) 76(25):3022–55. doi: 10.1016/j.jacc.2020.08.044
47. Chockalingam A, Dorairajan S, Bhalla M, Dellsperger KC. Unexplained hypotension: the Spectrum of dynamic left ventricular outflow tract obstruction in critical care settings. Crit Care Med. (2009) 37(2):729–34. doi: 10.1097/CCM.0b013e3181958710
48. Chockalingam A, Dellsperger KC. Isolated dynamic left ventricular outflow tract obstruction can cause hypotension that rapidly responds to intravenous Beta blockade. Am J Ther. (2011) 18(5):e172–6. doi: 10.1097/MJT.0b013e3181cea0dd
49. Pablo CR, David AO, Carlos V, Alvaro A, Williams H, Carolina I, et al. Beta blockers as salvage treatment in refractory septic shock complicated with dynamic left ventricular outflow tract obstruction: a rare case presentation. J Investig Med High Impact Case Rep. (2021) 9:23247096211056491. doi: 10.1177/23247096211056491
50. Slama M, Tribouilloy C, Maizel J. Left ventricular outflow tract obstruction in icu patients. Curr Opin Crit Care. (2016) 22(3):260–6. doi: 10.1097/MCC.0000000000000304
51. Joulin O, Marechaux S, Hassoun S, Montaigne D, Lancel S, Neviere R. Cardiac force-frequency relationship and frequency-dependent acceleration of relaxation are impaired in lps-treated rats. Crit Care. (2009) 13(1):R14. doi: 10.1186/cc7712
52. Bouhemad B, Nicolas-Robin A, Arbelot C, Arthaud M, Feger F, Rouby JJ. Isolated and reversible impairment of ventricular relaxation in patients with septic shock. Crit Care Med. (2008) 36(3):766–74. doi: 10.1097/CCM.0B013E31816596BC
53. Sanfilippo F, Corredor C, Fletcher N, Landesberg G, Benedetto U, Foex P, et al. Diastolic dysfunction and mortality in septic patients: a systematic review and meta-analysis. Intensive Care Med. (2015) 41(6):1004–13. doi: 10.1007/s00134-015-3748-7
54. Nagueh SF, Middleton KJ, Kopelen HA, Zoghbi WA, Quinones MA. Doppler Tissue imaging: a noninvasive technique for evaluation of left ventricular relaxation and estimation of filling pressures. J Am Coll Cardiol. (1997) 30(6):1527–33. doi: 10.1016/s0735-1097(97)00344-6
55. Nagueh SF, Smiseth OA, Appleton CP, Byrd BF 3rd, Dokainish H, Edvardsen T, et al.. Recommendations for the evaluation of left ventricular diastolic function by echocardiography: an update from the American society of echocardiography and the European association of cardiovascular imaging. J Am Soc Echocardiogr. (2016) 29(4):277–314. doi: 10.1016/j.echo.2016.01.011
56. Vignon P, Allot V, Lesage J, Martaille JF, Aldigier JC, Francois B, et al. Diagnosis of left ventricular diastolic dysfunction in the setting of acute changes in loading conditions. Crit Care. (2007) 11(2):R43. doi: 10.1186/cc5736
57. Sanfilippo F, Corredor C, Arcadipane A, Landesberg G, Vieillard-Baron A, Cecconi M, et al. Tissue Doppler assessment of diastolic function and relationship with mortality in critically ill septic patients: a systematic review and meta-analysis. Br J Anaesth. (2017) 119(4):583–94. doi: 10.1093/bja/aex254
58. Westermann D, Kasner M, Steendijk P, Spillmann F, Riad A, Weitmann K, et al. Role of left ventricular stiffness in heart failure with normal ejection fraction. Circulation. (2008) 117(16):2051–60. doi: 10.1161/CIRCULATIONAHA.107.716886
59. Mahjoub Y, Benoit-Fallet H, Airapetian N, Lorne E, Levrard M, Seydi AA, et al. Improvement of left ventricular relaxation as assessed by tissue Doppler imaging in fluid-responsive critically ill septic patients. Intensive Care Med. (2012) 38(9):1461–70. doi: 10.1007/s00134-012-2618-9
60. Peterson WP, Trempy GA, Nishiwaki K, Nyhan DP, Murray PA. Neurohumoral regulation of the pulmonary circulation during circulatory hypotension in conscious dogs. J Appl Physiol. (1993) 75(4):1675–82. doi: 10.1152/jappl.1993.75.4.1675
61. Brimioulle S, Maggiorini M, Stephanazzi J, Vermeulen F, Lejeune P, Naeije R. Effects of low flow on pulmonary vascular flow-pressure curves and pulmonary vascular impedance. Cardiovasc Res. (1999) 42(1):183–92. doi: 10.1016/s0008-6363(98)00301-0
62. Wilcox SR, Kabrhel C, Channick RN. Pulmonary hypertension and right ventricular failure in emergency medicine. Ann Emerg Med. (2015) 66(6):619–28. doi: 10.1016/j.annemergmed.2015.07.525
63. Ventetuolo CE, Klinger JR. Management of acute right ventricular failure in the intensive care unit. Ann Am Thorac Soc. (2014) 11(5):811–22. doi: 10.1513/AnnalsATS.201312-446FR
64. Rudski LG, Lai WW, Afilalo J, Hua L, Handschumacher MD, Chandrasekaran K, et al. Guidelines for the echocardiographic assessment of the right heart in adults: a report from the American society of echocardiography endorsed by the European association of echocardiography, a registered branch of the European society of cardiology, and the Canadian society of echocardiography. J Am Soc Echocardiogr. (2010) 23(7):685–713. doi: 10.1016/j.echo.2010.05.010
65. Tadic M, Nita N, Schneider L, Kersten J, Buckert D, Gonska B, et al. The predictive value of right ventricular longitudinal strain in pulmonary hypertension, heart failure, and valvular diseases. Front Cardiovasc Med. (2021) 8:698158. doi: 10.3389/fcvm.2021.698158
66. Smolarek D, Gruchala M, Sobiczewski W. Echocardiographic evaluation of right ventricular systolic function: the traditional and innovative approach. Cardiol J. (2017) 24(5):563–72. doi: 10.5603/CJ.a2017.0051
67. Orde SR, Pulido JN, Masaki M, Gillespie S, Spoon JN, Kane GC, et al. Outcome prediction in sepsis: speckle tracking echocardiography based assessment of myocardial function. Crit Care. (2014) 18(4):R149. doi: 10.1186/cc13987
68. Lanspa MJ, Cirulis MM, Wiley BM, Olsen TD, Wilson EL, Beesley SJ, et al. Right ventricular dysfunction in early sepsis and septic shock. Chest. (2021) 159(3):1055–63. doi: 10.1016/j.chest.2020.09.274
69. Vallabhajosyula S, Kumar M, Pandompatam G, Sakhuja A, Kashyap R, Kashani K, et al. Prognostic impact of isolated right ventricular dysfunction in sepsis and septic shock: an 8-year historical cohort study. Ann Intensive Care. (2017) 7(1):94. doi: 10.1186/s13613-017-0319-9
70. Innocenti F, Palmieri V, Stefanone VT, Donnini C, D'Argenzio F, Cigana M, et al. Epidemiology of right ventricular systolic dysfunction in patients with sepsis and septic shock in the emergency department. Intern Emerg Med. (2020) 15(7):1281–9. doi: 10.1007/s11739-020-02325-z
71. Vieillard-Baron A, Prigent A, Repesse X, Goudelin M, Prat G, Evrard B, et al. Right ventricular failure in septic shock: characterization, incidence and impact on fluid responsiveness. Crit Care. (2020) 24(1):630. doi: 10.1186/s13054-020-03345-z
72. Currigan DA, Hughes RJ, Wright CE, Angus JA, Soeding PF. Vasoconstrictor responses to vasopressor agents in human pulmonary and radial arteries: an in vitro study. Anesthesiology. (2014) 121(5):930–6. doi: 10.1097/ALN.0000000000000430
73. Hussain A, Bennett R, Haqzad Y, Qadri S, Chaudhry M, Cowen M, et al. The differential effects of systemic vasoconstrictors on human pulmonary artery tension. Eur J Cardiothorac Surg. (2017) 51(5):880–6. doi: 10.1093/ejcts/ezw410
74. Dalla K, Bech-Hanssen O, Ricksten SE. Impact of norepinephrine on right ventricular afterload and function in septic shock-a strain echocardiography study. Acta Anaesthesiol Scand. (2019) 63(10):1337–45. doi: 10.1111/aas.13454
75. Martin C, Perrin G, Saux P, Papazian L, Gouin F. Effects of norepinephrine on right ventricular function in septic shock patients. Intensive Care Med. (1994) 20(6):444–7. doi: 10.1007/BF01710657
76. Sarkar J, Golden PJ, Kajiura LN, Murata LA, Uyehara CF. Vasopressin decreases pulmonary-to-systemic vascular resistance ratio in a porcine model of severe hemorrhagic shock. Shock. (2015) 43(5):475–82. doi: 10.1097/SHK.0000000000000325
77. Wallace AW, Tunin CM, Shoukas AA. Effects of vasopressin on pulmonary and systemic vascular mechanics. Am J Physiol. (1989) 257(4 Pt 2):H1228–34. doi: 10.1152/ajpheart.1989.257.4.H1228
78. Jin HK, Chen YF, Yang RH, McKenna TM, Jackson RM, Oparil S. Vasopressin lowers pulmonary artery pressure in hypoxic rats by releasing atrial natriuretic peptide. Am J Med Sci. (1989) 298(4):227–36. doi: 10.1097/00000441-198910000-00004
79. Seymour CW, Kennedy JN, Wang S, Chang CH, Elliott CF, Xu Z, et al. Derivation, validation, and potential treatment implications of novel clinical phenotypes for sepsis. JAMA. (2019) 321(20):2003–17. doi: 10.1001/jama.2019.5791
80. Knox DB, Lanspa MJ, Kuttler KG, Brewer SC, Brown SM. Phenotypic clusters within sepsis-associated multiple organ dysfunction syndrome. Intensive Care Med. (2015) 41(5):814–22. doi: 10.1007/s00134-015-3764-7
81. Gardlund B, Dmitrieva NO, Pieper CF, Finfer S, Marshall JC, Taylor Thompson B. Six subphenotypes in septic shock: latent class analysis of the prowess shock study. J Crit Care. (2018) 47:70–9. doi: 10.1016/j.jcrc.2018.06.012
82. Ma P, Liu J, Shen F, Liao X, Xiu M, Zhao H, et al. Individualized resuscitation strategy for septic shock formalized by finite mixture modeling and dynamic treatment regimen. Crit Care. (2021) 25(1):243. doi: 10.1186/s13054-021-03682-7
83. Scicluna BP, van Vught LA, Zwinderman AH, Wiewel MA, Davenport EE, Burnham KL, et al. Classification of patients with sepsis according to blood genomic endotype: a prospective cohort study. Lancet Respir Med. (2017) 5(10):816–26. doi: 10.1016/S2213-2600(17)30294-1
84. Wong HR, Cvijanovich N, Allen GL, Lin R, Anas N, Meyer K, et al. Genomic expression profiling across the pediatric systemic inflammatory response syndrome, sepsis, and septic shock Spectrum. Crit Care Med. (2009) 37(5):1558–66. doi: 10.1097/CCM.0b013e31819fcc08
85. Davenport EE, Burnham KL, Radhakrishnan J, Humburg P, Hutton P, Mills TC, et al. Genomic landscape of the individual host response and outcomes in sepsis: a prospective cohort study. Lancet Respir Med. (2016) 4(4):259–71. doi: 10.1016/S2213-2600(16)00046-1
86. Maslove DM, Tang BM, McLean AS. Identification of sepsis subtypes in critically ill adults using gene expression profiling. Crit Care. (2012) 16(5):R183. doi: 10.1186/cc11667
87. Calfee CS, Delucchi K, Parsons PE, Thompson BT, Ware LB, Matthay MA, et al. Subphenotypes in acute respiratory distress syndrome: latent class analysis of data from two randomised controlled trials. Lancet Respir Med. (2014) 2(8):611–20. doi: 10.1016/S2213-2600(14)70097-9
88. Calfee CS, Delucchi KL, Sinha P, Matthay MA, Hackett J, Shankar-Hari M, et al. Acute respiratory distress syndrome subphenotypes and differential response to simvastatin: secondary analysis of a randomised controlled trial. Lancet Respir Med. (2018) 6(9):691–8. doi: 10.1016/S2213-2600(18)30177-2
Keywords: sepsis, myocardial dysfunction, septic cardiac dysfunction, echocardiography, septic cardiomyopathy
Citation: Shvilkina T and Shapiro N (2023) Sepsis-Induced myocardial dysfunction: heterogeneity of functional effects and clinical significance. Front. Cardiovasc. Med. 10:1200441. doi: 10.3389/fcvm.2023.1200441
Received: 4 April 2023; Accepted: 5 June 2023;
Published: 14 July 2023.
Edited by:
Vittorio Palmieri, San Sebastiano & Sant'Anna National Hospital, ItalyReviewed by:
Mathieu Jozwiak, Centre Hospitalier Universitaire de Nice, France© 2023 Shvilkina and Shapiro. This is an open-access article distributed under the terms of the Creative Commons Attribution License (CC BY). The use, distribution or reproduction in other forums is permitted, provided the original author(s) and the copyright owner(s) are credited and that the original publication in this journal is cited, in accordance with accepted academic practice. No use, distribution or reproduction is permitted which does not comply with these terms.
*Correspondence: Tatyana Shvilkina tshvilki@bidmc.harvard.edu