- Department of Cardiology, The Second Hospital of Jilin University, Changchun, China
Heat shock protein 27 (HSP27) is a small chaperone protein that is overexpressed in a variety of cellular stress states. It is involved in regulating proteostasis and protecting cells from multiple sources of stress injury by stabilizing protein conformation and promoting the refolding of misfolded proteins. Previous studies have confirmed that HSP27 is involved in the development of cardiovascular diseases and plays an important regulatory role in this process. Herein, we comprehensively and systematically summarize the involvement of HSP27 and its phosphorylated form in pathophysiological processes, including oxidative stress, inflammatory responses, and apoptosis, and further explore the potential mechanisms and possible roles of HSP27 in the diagnosis and treatment of cardiovascular diseases. Targeting HSP27 is a promising future strategy for the treatment of cardiovascular diseases.
1. Introduction
According to the World Health Organization (WHO), cardiovascular diseases (CVDs) lead the world in terms of morbidity and mortality. By 2030, approximately 23.6 million people are predicted to die from these diseases, mainly stroke and heart disease (1). The cardiovascular system is highly energy-dependent, and homeostatic imbalances due to various causes can severely damage to the heart in a short period of time. Therefore, the cardiovascular system possesses a well-established set of protective mechanisms, including collateral circulation, the antioxidant system, and other intracellular stress responses that protect cardiovascular function. Among these defense schemes, the heat shock response (HSR) is a well-preserved evolutionary feature that induces the expression of heat shock proteins (HSPs) (2).
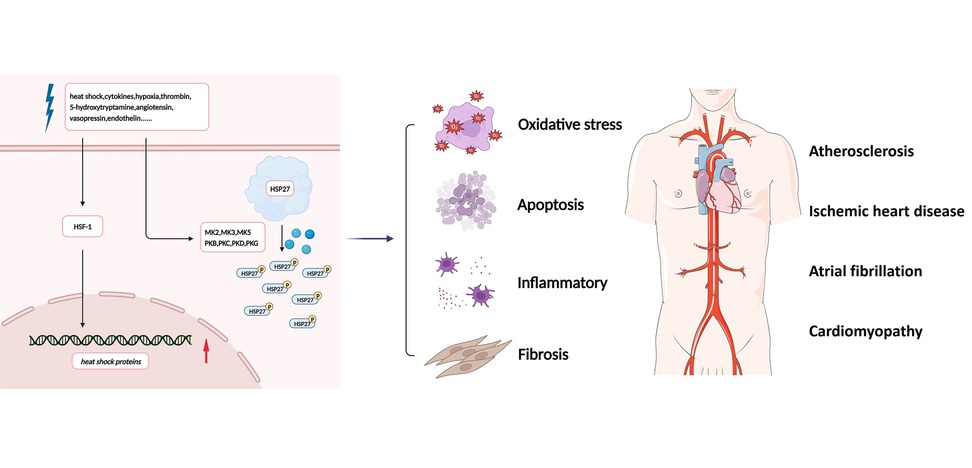
Figure 1. Simplified overview of the effects of HSP27. In the presence of stress stimuli such as heat shock, cytokines, hypoxia, thrombin, 5-hydroxytryptamine, angiotensin II, vasopressin, and endothelin, HSP27 is rapidly phosphorylated, leading to conformational changes and depolymerization, associated with signaling processes involving oxidative stress, inflammatory, apoptosis, and fibrosis. Future HSP27-related research in cardiovascular diseases remains to be conducted. HSF-1, heat shock transcription factor 1; HSP27, heat shock protein 27; MK2, MAPK activated protein kinase 2; PKB, protein kinase B; PKC, protein kinase C; PKD, protein kinase D; PKG, protein kinase G.
HSPs are a highly conserved family of proteins expressed by many cell types after exposure to stressful environmental conditions. The expression of HSPs increases with stresses such as hyperthermia, hypoxia, nutritional deficiencies, oxidative stress, and UV radiation, as well as under conditions associated with systemic stress, such as in response to ischemia-reperfusion injury (IRI) (3–5). Many HSPs have been shown to be molecular chaperones involved in the refolding of other damaged proteins. Small heat shock proteins (sHSPs) are dynamic polypeptides that are predominantly β-folded and form large oligomers. Some important functions that they exhibit include preventing protein aggregation, refolding proteins, conferring heat resistance to cells, and anti-apoptotic functions. sHSPs are the most stress-inducible molecular chaperones that prevent stress-induced aggregation of partially denatured proteins. They also exhibit refolding abilities similar to large HSPs but are not dependent on adenosine triphosphate (ATP) hydrolysis (6).
Heat shock protein 27 (HSP27) is a ubiquitous sHSP with chaperone activity. Previous studies have found that HSP27 is involved in and plays a protective role in CVD-related pathophysiological processes such as oxidative stress, inflammatory responses, and apoptosis (7). In this review, we first introduce the origin of HSP27 and the phosphorylation reactions closely related to its function, followed by a comprehensive and systematic summary of the pathophysiological mechanisms involved in the regulation of HSP27. We finally summarize and discuss the latest research advances in its use as a diagnostic and prognostic biomarker for CVDs. The prospects of targeting HSP27 for CVD treatment are promising.
2. Overview of HSP27
2.1. Heat shock proteins
The HSR is an evolutionarily highly conserved process that induces the expression of molecular chaperones in response to cellular stress and promotes the refolding of denatured proteins. As early as the 1930s, researchers have conducted experiments on the effects of heat stress on Drosophila, and the appearance of chromosome expansion after heat stress indicated enhanced local gene transcription (8). In addition to elevated temperatures, HSR can be activated by a variety of stressors, such as oxidative stress, glucose depletion, and overexpression of misfolded proteins (9). Heat shock transcription factor 1 (HSF1) activates the genes encoding HSPs when host cells are exposed to stress conditions such as heat shock, local ischemia, or toxic substances (10). This family of proteins, together with other chaperone proteins, prevents intracellular protein misfolding and aggregation, and promotes cellular repair after stress injury. Although they are known as heat shock or heat stress proteins, many HSPs are also commonly expressed in cells under physiological conditions and are essential for the maintenance of cellular homeostasis, such as proteostasis (11).
HSPs have molecular weights of 10–150 kDa. Mammalian HSPs can be divided into different families based on their molecular weights, including the chaperone protein families of sHSPs (HSP27), HSP40, HSP60, HSP70, HSP90, and large HSPs (HSP110 and GRP170) (12). In contrast to other major chaperone protein families, sHSPs bind and sequester non-natural and denatured proteins in an ATP-independent manner, facilitating subsequent substrate depolymerization and/or refolding (13). sHSPs are characterized by low molecular weights (12–43 kDa, average length of 160 residues) and a conserved alpha-crystallin protein structural domain (alpha-crystallin domain, ACD). The highly conserved ACD consists of 90–100 amino acids and forms an immunoglobulin-like β-sandwich structural domain with seven to eight antiparallel β-sheet layers. The ACDs of human sHSPs are rich in histidine, which improves the ability to respond to changes in pH and metal ion effectiveness and regulates sHSP activity. ACDs are flanked by a highly variable amino-terminal region (NTR) and a flexible carboxy-terminal region (CTR). The NTR is rich in hydrophobic residues and is highly disordered, while the CTR is rich in charged polar residues, which allows extremely high concentrations of sHSPs to remain soluble in the cell. Both the NTRs and CTRs exhibit high flexibility and wide sequence variation, and contribute to the oligomerization of sHSPs (14), a process that will be elaborated in the next section.
2.2. HSP27 and its phosphorylation
HSP27 (also known as HSPB1) is a member of the sHSP family. In addition to the conserved ACD region, the amino-terminal WDPF structural domain of HSP27 is essential for oligomerization, and the carboxy-terminal flexible variable region is thought to be involved in interactions with target proteins, regulation of solubility, and oligomerization. The presence of 10.2% serine, 6.8% threonine, and 2.4% tyrosine residues in the primary sequence of HSP27 provides a structural basis for HSP27 phosphorylation. The amino-terminal Ser15, Ser78, and Ser82 are now known to be important phosphorylation acceptor sites (15). HSP27 phosphorylation regulates its oligomerization. Typically, unphosphorylated HSP27 exists as large multimers that can oligomerize into large aggregates of up to 800 kDa or form heterodimeric structures with other members of the sHSP family. In the presence of stress stimuli such as heat shock, cytokines, hypoxia, thrombin, 5-hydroxytryptamine, angiotensin II, vasopressin, and endothelin, HSP27 is rapidly phosphorylated, leading to conformational changes and depolymerization (16). In cells, HSP27 phosphorylation is required for most of its functional activities (17). Phosphorylated HSP27 exists mainly as a monomer to a tetramer and acts as an inhibitor of apoptosis and an inducer of autophagy (18).
Under physiological conditions, HSP27 is constitutively expressed in a variety of cells and is upregulated under stress conditions, helping to maintain intracellular protein conformations and stabilize protein functions. HSP27 is also associated with signaling processes involving cell proliferation, cell cycle regulation, apoptosis, autophagy, and fibrosis (19–21). In recent years, clinical studies have identified HSP27 as a potential target and biological marker for the diagnosis, treatment, and prognosis of a variety of diseases, including cancer (15, 22, 23), neurological diseases (24–26), and CVDs (27–29). In the following section, the role of HSP27 and its phosphorylation in the regulation of pathophysiological processes, such as oxidative stress and inflammatory responses, will be discussed and analyzed in a comprehensive and systematic manner (Figure 1).
3. Pathophysiological processes regulated by HSP27
3.1. Oxidative stress
In recent years, HSP27 has been extensively studied in redox biology. HSP27 has been shown to regulate intracellular redox homeostasis and enhance cellular resistance to oxidative damage by protecting against lipid peroxidation (LPO) and protein oxidation (30).
3.1.1. Oxidative stress and HSP27 expression levels
In the cardiovascular system, protein quality control system (PQS) disorders are critical for the progression of hypertrophic cardiomyopathy (HCM) (31). Elevated HSP27 expression and its hyperphosphorylation are present in HCM hearts. Exogenous supplementation with HSP27 reduces oxidative stress levels in HCM myocytes, ameliorates PQS disorders, and reduces myocardial stiffness (32). In vitro experiments revealed that HSP27 prevents oxidative stress by increasing glucose-6-phosphate dehydrogenase (G6PD) activity and maintaining reduced glutathione (GSH) levels (33). After administration of ethanol and methylenedioxymethamphetamine to adolescent mice, sympathetic pathways were activated and cellular stress was increased. Increased HSP27 in the right ventricle may increase thioredoxin-1 (Trx1) levels and reduce the oxidative stress induced by the co-stimulation with both drugs (34).
HSP27 overexpression significantly ameliorated oxidative stress. Upregulation of HSP27 improved the reduced state of glutathione reductase (GR), peroxiredoxin-1 (Prx1), and Trx1 in H9c2 cells treated with hydrogen peroxide, increased the GSH/ glutathione disulfide (GSSG) ratio, promoted the formation of a complex between HSP27 and oxidized Prx1, regulated the redox status of key proteins involved in the cytosolic antioxidant pathway, and protected H9c2 cells from oxidative stress (35). In addition, HSP27 regulated the Hippo pathway by enhancing dephosphorylation of mammalian STE20-like kinase 1, leading to reduced phosphorylation of large tumor suppressor kinase 1 (LATS1) and Yes-associated protein (YAP) and increased nuclear localization of YAP, which ameliorated the hydrogen peroxide-induced imbalance in the cytoplasmic redox state and drove the transcription of genes associated with cytoprotection (35). Drosophila larvae exposed to nonylphenol exhibited significantly reduced midgut HSP27 and ecdysone receptor mRNA levels, decreased GSH levels and thioredoxin reductase activity, and increased levels of reactive oxygen species (ROS). This was accompanied by a nonylphenol concentration-dependent increase in LPO, protein carbonyl (PC) content, and cell death. Overexpression of HSP27 ameliorated oxidative stress by decreasing nonylphenol-induced intracellular ROS, LPO, and PC levels in the midgut, and decreased GSH levels (36). Cyclo(His-Pro) increased soluble superoxide dismutase (SOD) activity by upregulating HSP70 and HSP27 and decreasing lipopolysaccharide (LPS)-induced ROS production (37).
Altering HSP27 cross-linking altered normal HSP27 dimerization, inhibited HSP27 function. HSP27 inhibitors downregulated radiation-induced ROS production, inhibited immune cell infiltration in lung tissue (38). HSP27 knockdown decreased human dermal fibroblast viability and increased ultraviolet B (UVB)-induced ROS production. Compared to UVB exposure alone, HSP27 knockdown in UVB-exposed cells resulted in significantly lower LC3B expression, significantly higher p62 expression, and reduced expression of some antioxidants, such as SOD and catalase, accelerating UVB-induced ROS release (39). The use of intermittent pressure-imitating, rolling manipulation to simulate traditional Chinese medicine manipulation in a human skeletal muscle cell line increased SOD activity and decrease malondialdehyde (MDA) content and creatine kinase activity by downregulating CD36, HSP27, and FABP4 expression. This improved oxidative stress and lipid metabolic imbalances (40).
3.1.2. Oxidative stress and HSP27 phosphorylation
There is also a degree of modulation between HSP27 phosphorylation levels and oxidative stress signaling pathways. As a stress response, freezing stimulation can lead to enhanced p38 kinase activity and increased HSP27 phosphorylation, which inhibits oxidative stress and exerts a protective effect by increasing cytoplasmic SOD1 levels (41). Yusuke et al. found (42) that during cerebral ischemia/reperfusion (I/R), ataxia telangiectasia mutated (ATM) kinase, via phosphorylation of HSP27 on serine 85, induced G6PD activity in the pentose phosphate pathway to increase the NADPH/NAD+ ratio and exert antioxidant effects. ATM inhibitors decreased HSP27 phosphorylation and G6PD upregulation after I/R, significantly increasing PC levels and leading to an increased infarct size. Geranylgeranylacetone did not affect HSP27 levels in brain I/R-injured rats but significantly increased HSP27 phosphorylation and reduced PC levels by increasing G6PD activity (43). However, the exact mechanism needs to be confirmed in future studies.
These results indicate that HSP27 expression and its phosphorylation regulate many enzymes involved in oxidative stress, thus reducing ROS production. However, the specific mechanism by which HSP27 regulates enzymes and how it affects their expression levels are unclear and need to be determined by more research.
3.2. Inflammatory responses
HSP27 is a major intracellular molecular chaperone and a regulator of inflammatory signaling responses. Exogenous HSP27 prevents neutrophil apoptosis in a dose-dependent manner without altering the levels of inflammatory cytokines such as interleukin (IL) 12 and IL-10, prolonging neutrophil survival and exacerbating acute inflammation and tissue destruction (44). The combination of inflammatory markers, soluble suppression of tumorigenicity 2 (sST2), HSP27, and high-sensitivity C-reactive protein is an important factor in patients with heart failure (HF) as an independent predictor of cardiovascular death and unplanned HF-related hospitalization (45). A positive correlation can be found between systemic HSP27 levels and the pro-inflammatory cytokines tumor necrosis factor alpha (TNF-α) and IL-6 (p < 0.001) (46).
3.2.1. Inflammatory responses and HSP27 expression
Upregulation of intracellular HSP27 expression inhibits the inflammatory response. Clostridium difficile (CD) causes severe gastrointestinal inflammatory diarrhea in patients treated with antibiotics. It was found that overexpression of HSP27 protected the levels of cystic fibrosis transmembrane regulator (CFTR), Na+/H+ exchange regulatory factor-1 (NHERF1), and Na+/H+ exchange regulatory factor-2 (NHERF2), rendering them unaffected by toxin CD B (TcdB). Thus, the binding of CFTR to NHERF1 and NHERF2 was reduced, preventing a decrease in transepithelial resistance, and protecting intestinal barrier function (47). Upregulation of HSP27 is involved in the regulation of cellular resistance to Escherichia coli F18 by inhibiting adhesion of the bacteria to porcine small intestinal epithelial cells and reducing pro-inflammatory factor concentrations that drive exogenous stress-induced inflammatory responses. In addition, upregulation of HSP27 gene expression led to upregulation of the ribosomal S6 kinase 2 (RSK2) gene in the mitogen-activated protein kinase (MAPK) pathway, which synergistically counteracted stress-induced inflammatory responses in the body (48). Valdecoxib attenuated nuclear factor-κB (NF-κB) and IκB phosphorylation in mouse skeletal muscle by increasing 5′-adenosine monophosphate-activated protein kinase (AMPK) phosphorylation and HSP27 expression via the AMPK pathway. Valdecoxib also reduced eukaryotic initiation factor 2 alpha phosphorylation and C/EBP homologous protein (CHOP) expression, decreased serum levels of the pro-inflammatory cytokines TNF-α and IL-6, and improved the inflammatory response and endoplasmic reticulum (ER) stress (49). In rats (50), renal IRI increased serum creatinine and urea nitrogen concentrations; promoted LPO by elevating MDA levels; inhibited SOD activity; induced NF-κB, IL-2, and TLR-4 expression to stimulate inflammatory responses; and increased renal cell apoptosis. Hydrogen sulfide protected the renal tissue from LPO, inflammation, and apoptosis, possibly due to the upregulation of HSP70, heme oxygenase 1, and HSP27. HSPB1 knockout mice subjected to myocardial infarction (MI) exhibited enhanced and prolonged leukocyte infiltration, enhanced inflammatory cytokine expression, and enhanced toll-like receptor 4 (TLR4)/myeloid differentiation factor 88 (MyD88)/NF-κB activation in their heart tissue. Further analysis of primary mouse cardiomyocytes showed that cardiomyocyte-specific HSPB1 knockdown increased NF-κB activation, promoted the expression of proinflammatory mediators, increased leukocyte recruitment, and activated excessive inflammatory responses, ultimately leading to poor remodeling, cardiac dysfunction, and cardiac rupture after MI (51). HSP27 inhibited the expression of transcriptional repressor E2F4/p130 to promote cell cycle progression. HSP27 knockdown resulted in increased expression of E2F4/p130 and downregulation of the expression of six G2/M-related genes (CCNA2, CCNB1, CCNB2, CDC25C, CDCA3, and CDK1), leading to G2 blockade and upregulation of inflammatory factors and cellular senescence (52).
3.2.2. Inflammatory responses and HSP27 phosphorylation
Inflammatory mediators trigger the inflammatory response through G protein-coupled receptors (GPCRs), including protease-activated receptor 1 (PAR1). Thrombin ligand activation of PAR1 stimulates pro-inflammatory p38 MAPK signaling and promotes endothelial barrier disruption. GPCRs induce the p38-dependent MAPK-activated protein kinase 2 (MAPKAPK2)-MAPKAPK3 inflammatory signaling pathway that mediates HSP27 phosphorylation and activation to control endothelial barrier restoration and vascular leakage. Knockdown of HSP27 or blockade of HSP27 oligomer formation enhances endothelial barrier permeability in vitro and vascular leakage in vivo in response to PAR1 activation (53). Noxa inhibits the nuclear translocation and activation of NF-κB by inhibiting the degradation of ubiquitinated IκBα through the interaction of covalent disulfide bonds with phosphorylated HSP27, thereby suppressing the inflammatory response to allergens (54).
HSP27 phosphorylation promotes CREB-binding protein (CBP) transcriptional output via p53 activation during early inflammation in LPS-stimulated THP-1 cells, regulates NF-κB activation, and increases the release of the inflammatory factors TNF-α, IL-6, interferon-beta (IFN-β), and IL-10. In late inflammation, phosphorylated HSP27 inhibits an excessive increase in CBP by suppressing the excessive accumulation of ROS. The phosphorylated state of HSP27 controls the LPS-induced inflammatory response by regulating CBP (55). HSP27 was phosphorylated at serine 78 and 82 after exposure to LPS. Inhibition of HSP27 phosphorylation significantly exacerbated LPS-induced apoptosis, growth inhibition, and inflammatory factor expression by inhibiting IκBα phosphorylation and NF-κB activation (56). High mobility group box-1 protein (HMGB1) is a pro-inflammatory cytokine associated with death during sepsis and other inflammatory diseases. After acetylation by CBP at transcriptional junctions, HMGB1 is translocated from the nucleus to the cytoplasm. In the cytoplasm of static monocytes, HSP27, in a non-phosphorylated state, interacts with CBP as a natural regulator to promote ubiquitin-mediated CBP degradation. After LPS stimulation, HSP27 is phosphorylated through the p38 MAPK/MK2 signaling pathway, translocates to the nucleus to bind CBP, inhibits CBP acetyltransferase activity and subsequent CBP-dependent acetylation of HMGB1, prevents HMGB1 translocation to the cytoplasm, and inhibits inflammatory signaling (57).
3.2.3. Inflammatory responses and HSP27 immune complex
Blood levels of HSP27 and natural IgG autoantibodies (anti-HSP27 antibodies, AAb) were higher in healthy controls than in patients with CVD (58). The interaction of the HSP27 immune complex (IC) with TLR4 was found to enhance binding to the membrane of THP-1 macrophages (MΦ), activate the NF-κB pathway, and competitively displace the interaction of LPS with this receptor. The activation of NF-κB via this external pathway affects the anti-inflammatory milieu, with increased secreted IL-10 and decreased IL-1β levels. In addition, HSP27 IC competes with oxidized low-density lipoprotein (oxLDL) to bind the scavenger receptors SR-AI and CD-36, reducing oxLDL internalization and foam cell formation. This finding suggests that HSP27 IC can act as a natural antagonist of inflammatory signaling and MΦ foam cell formation (59). Further studies on THP-1 MΦ showed (60) that HSP27 IC increased the number of secreted exosomes and the concentration of cholesterol in exosomes. HSP27 immunotherapy is not only a preventive measure for atherosclerosis but also a potential treatment for atherosclerosis by reducing the cholesterol content of plaques that have already formed.
3.2.4. Inflammatory responses and exosomal HSP27
In addition to intracellular chaperone functions, cellular stress proteins can be released by cells and are present in extracellular environments, such as in exosomes or body fluids, and are increasingly used as biomarkers of human disease states (61). Shi et al. (62) found that HSP27 is present on exosomal membranes and that exosomes containing HSP27, as an important exosomal cargo exerting anti-inflammatory effects, stimulate NF-κB activation and IL-10 release. This finding suggests that the future use of exosomes to target specific tissues and organs for the delivery of protein cargoes for precision therapy is an interesting possibility.
3.3. Apoptosis
It is well known that HSP27 is involved in endogenous and exogenous apoptotic pathways and interacts with apoptotic mediators to exert anti-apoptotic effects.
3.3.1. Apoptosis and HSP27 expression levels
HSP27 plays an active antiapoptotic role in the pathophysiology of several diseases. HSP27, Bax, and caspase-3 expression was upregulated in AS mouse plaques and ROS levels were elevated in the aorta. HSP27 knockdown resulted in increased plaque size, increased apoptosis, and enhanced aortic ROS levels. HSP27 maintained oxidative stress homeostasis and mitigated progression of atherosclerosis by inhibiting the mitochondrial apoptosis pathway (63). In a mouse I/R injury model (64), miR-410 inhibited mitochondrial autophagy after cardiac I/R injury by directly targeting HMGB1 to regulate HSP27 activity. Inhibition of HSP27 significantly attenuated the promoting effect of HMGB1 upregulation on cell viability and ATP production, as well as its inhibitory effect on apoptosis. Zhou et al. (65) found that HSP27 expression was elevated in rat neurons after lumbosacral nerve root avulsion injury. In a oxygen-glucose deprivation (OGD) model, upregulation of HSP27 was found to increase GSH content and SOD activity and to inhibit oxidative stress, suppressing OGD-induced apoptosis. HSP27 knockdown significantly reduced p65 phosphorylation and eliminated OGD-induced NF-κB activation. HSP27 may inhibit OGD-induced apoptosis by activating the NF-κB signaling pathway.
In a rat subarachnoid hemorrhage (SAH) model (66), HSP27 overexpression attenuated neurological defects and apoptosis in the basal cortex and effectively inhibited activation of MAP kinase kinase 4, c-Jun N-terminal kinase, c-Jun, and caspase-3, exerting neuroprotective effects. UVB irradiation triggered changes in HSP27 phosphorylation and localization, inducing the translocation of HSP27 from the cytoplasm to the nucleus and protecting the cells from UVB damage (67). HSP27 knockdown was found to increase levels of a chronic product of photoaging, MDA; decrease hydroxyproline content; upregulate protein expression of the aging markers p16, p53, and p21; and promote the expression of the pro-apoptotic factor Bax. These changes resulted in a significant decrease in collagen fibers and thickening and distortion of elastic fibers (68). Further studies have revealed that HSP27 regulates the subcellular localization of p21 by activating the phosphorylated protein kinase B (AKT)-dependent pathway and inhibiting the p53/Bax/Bcl-2-dependent mitochondrial apoptotic pathway to promote the transfer of p21 to the cytoplasm (69). Semisynthetic penicillinase-resistant antibiotics (PRAs) induce ER stress in hepatocytes, resulting in pure cholestasis. HSP27 activates the phosphatidylinositol 3-kinase (PI3K)/AKT pathway, which inhibits caspase-3 activity and prevents ER stress-triggered apoptosis (70). However, intravitreal injection of HSP27 also caused elevated levels of caspase-3, -8, and -9 in retinal cells, activation of intrinsic and extrinsic apoptotic pathways, and increased apoptosis, leading to retinal damage (71). The mechanism by which exogenous injection of HSP27 increases apoptosis remains to be investigated.
Since HSP27 is able to inhibit apoptosis and autophagy, this biological function can then be exploited by reducing HSP27 expression to promote tumor cell apoptosis. Resveratrol combined with small interfering RNA (siRNA)-mediated silencing of HSP27 promotes caspase-3-induced apoptosis (72). Widespread chemotherapy resistance can lead to a decrease in the effectiveness of anticancer drug therapy. Inhibition of HSP27 decreases NOTCH1 expression and the phosphorylation of AKT and mechanistic target of rapamycin (mTOR), enhances the effects of 5-fluorouracil (5-FU) and vincristine on the downstream AKT-mTOR pathway, and increases chemotherapeutic drug-related apoptosis (73). Both apoptosis and autophagy were strongly induced curcumin treatment, resulting in the effective treatment. Knockdown of HSP27 increased the resistance of cells to curcumin through the p-AKT/AKT signaling pathway; decreased reactive oxygen/nitrogen species, superoxide, and autophagy levels and reduced reactive oxidative stress and apoptosis (74). Radiation therapy is the treatment of choice in patients with limited prostate cancer (PCa). MiR-541–3p inhibited the expression of β-catenin by targeting HSP27 mRNA and its 3′ untranslated region (3′UTR), upregulated cell apoptosis after radiation (75). Heat stress was shown to downregulate miR-541 levels, leading to increased translation of HSP27. This promoted autophagy in normal lung bronchial epithelial cells through ATG7, increased mitochondrial membrane potential, reduced cytochrome C release from mitochondria into the cytoplasm (thereby inhibiting apoptosis), and acted as an oncogene in the heat stress-induced transformation of lung epithelial cells (76).Silencing HSPB1 increased the radiosensitivity by decreasing cell viability, depolarizing the mitochondrial membrane potential, arresting the cell cycle in the G2/M phase, and promoting apoptosis (77).
The promotion of HSP27 expression can play a positive cytoprotective role and reduce the occurrence of adverse drug reactions. Overexpression of HSP27 prevented apoptosis and maintained axonal integrity, leading to reversal of aberrant pain responses in paclitaxel-treated transgenic mice overexpressing human HSP27 (hHsp27 Tg mice). HSP27 overexpression exerted its anti-apoptotic function by inactivating caspase-3 and maintained axonal integrity by inactivating RhoA and its downstream effectors to prevent peripheral neuropathy (78). Vincristine-induced mechanical and cold abnormal pain was also prevented in the hHsp27 Tg mice by blocking axonal degeneration, demyelination, mitochondrial dysfunction, and apoptosis (79). HSP27 protected cardiomyocytes against doxorubicin-induced toxicity by upregulating the cytoprotective cascade system, specifically by upregulating enzyme activity or activating PI3K/AKT to attenuate the p53 cascade (80).
3.3.2. Apoptosis and HSP27 phosphorylation
Phosphorylation of overexpressed HSP27 at Ser82 and its association with p53 are critical for cardioprotection in doxorubicin -induced dilated cardiomyopathy. Only phosphorylated HSP27 could protect the heart by inhibiting the p53-dependent apoptotic pathway (81). Glucocorticoids reduced TNF-α-induced apoptosis in human aortic smooth muscle cells and maintained aortic wall homeostasis by increasing the levels of unbound soluble tumor necrosis factor receptor-2 (TNFR2) and inhibiting phosphorylation of HSP27 induced by activation of the p38 MAPK-HSP27 pathway (82). Endolipin exerted a protective effect against high glucose-induced apoptosis in human umbilical vein endothelial cells (HUVECs) by promoting HSP27 phosphorylation through PI3K/AKT and ERK1/2 signaling pathways, and this protective effect could be significantly blocked by siRNA-HSP27 (83). Tanshinone IIA (TIIA) induces phosphorylation of HSP27 at serine 82, leading to activation of the unfolded protein response and accumulation of ROS, which induces the expression of HSF1 and its target genes and leads to cell apoptosis (84). The chemotherapy regimen FIRINOX (a combination of 5-fluorouracil, irinotecan, and oxaliplatin) leads to pancreatic ductal adenocarcinoma (PDAC)-induced DNA damage, activating the NF-κB pathway and TNF-α production. The resultant autocrine TNFR1 activity triggers apoptotic signaling and mediates the treatment-resistant TAK1-p38-MK2-HSP27 pathway. Targeting kinases that directly phosphorylate HSP27, thus blocking HSP27 phosphorylation, can promote apoptosis (85). Studies have found (86) that dioscin promotes ROS-induced apoptosis via the p38 MAPK/HSP27 pathway and that inhibition of HSP27 phosphorylation attenuates dioscin-mediated apoptosis. Therapeutic regimens targeting HSP27 and its phosphorylation can play an active role by regulating the apoptotic process.
3.4. Fibrosis
Fibrosis is the end stage of persistent tissue damage and chronic inflammatory responses, characterized by excessive accumulation of extracellular matrix and destruction of normal tissue structure. HSP27 has been shown to be overexpressed in the pleura of patients with pulmonary fibrosis and other fibrotic diseases (87). In a study of two mouse models of thrombopoietin and constitutively active JAK2 mutant (JAKV617F)-induced myelofibrosis (88), HSP27 was found to regulate the proliferation of JAK2V617F-positive cells and interact directly with JAK2/STAT5 to protect STAT5 from dephosphorylation. Thus, HSP27 may serve as a potential therapeutic target. In transforming growth factor beta (TGF-β)-stimulated human renal proximal tubule HK-11 cells and in the kidneys of diabetic mice (89), researchers demonstrated that pSer82 HSP27 can bind to nuclear factor erythroid 2 (NF-E2), which may promote NF-E2 degradation by targeting the protein to the proteasome, thereby promoting fibrosis. Recombinant HSP27 can be combined with antibodies targeting the angiotensin II type 1 (AT1) receptor to form a ZZ-TAT-GFP fusion protein that specifically targets cardiomyocytes, and can reduce apoptosis, reduce the area of myocardial fibrosis, increase ejection fraction, reduce end-systolic volume and end-diastolic pressure, and improve cardiac function after MI (90).
Epithelial-mesenchymal transition (EMT) is an important event in cell development in which epithelial cells acquire mesenchymal fibroblast-like features, including reduced intercellular adhesion and increased motility (91). Among the three subtypes of EMT, type 2 is associated with wound healing, tissue regeneration, and organ fibrosis as a repair-related process. HSP27 is known to increase cell migration and invasion and to mediate EMT in cancer cells, leading to cancer progression. The inhibition of HSP27 is considered a promising therapeutic approach for controlling cancer growth and fibrosis. Oh et al. (92) found that the HSP27 inhibitor J2 increased the lysosomal degradation of HSP27 and promoted STAT6-induced Ym1 expression in M2 macrophages, inhibited IL-8 production, and attenuated inflammatory lung fibrosis in mice. Further studies have revealed that HSP27 activates the NF-κB pathway through direct interaction with IκBα, leading to increased expression of Twist, IL-1β, and IL-6, and promoting the EMT process that is closely associated with the development of radiation-induced pulmonary fibrosis (93). EMT is also an important contributor to the pathogenesis of liver fibrosis. Mangiferin prevented CCl4 and TGF-β1-induced EMT and hepatic fibrosis by reducing HSP27 expression to inhibit the JAK2/STAT3 pathway, which in turn inhibited TGF-β1/Smad pathway activation (94). It was found that HSP27 interacted directly with small ubiquitin-related modifier 2/3, regulating pyruvate kinase M2 and promoting the migration and motility through the EMT pathway (95). Studies related to the regulation of EMT by HSP27 have mainly focused on tumor therapy (96, 97), finding that HSP27 is directly involved in EMT in lung mesothelial, epithelial, and colon adenocarcinoma cells. The regulatory role of HSP27 in the fibrosis of other tissues and organs needs to be explored further (Table 1).
4. The therapeutic intervention of HSP27
4.1. Atherosclerosis
HSP27 is a traditional intracellular chaperone protein, but it also functions as an extracellular signal that regulates lipid accumulation and foam cell formation, contributing to the prevention of atherosclerosis (98). Clinical studies found that patients with atherosclerosis with >50% coronary stenosis had lower levels of HSP27 than those without atherosclerosis. Follow-up found that low HSP27 levels were associated with the presence of coronary artery disease and the occurrence of future adverse clinical events (99). In a study of 852 patients with stroke and coronary atherosclerosis (100), vitamin D was found to improve oxidative stress by reducing serum anti-HSP27 antibody levels, which may reduce CVD risk; however, further evaluation in larger populations is needed. Circulating HSP27 was positively correlated with carotid intima-media thickness, an independent predictor of early diabetic atherosclerotic lesions, and may represent a novel marker of subclinical atherosclerosis in type 2 diabetes (101). Serum HSP27 and its phosphorylation levels were lower in patients with lower extremity occlusive atherosclerosis and negatively correlated with disease severity (102). Low serum HSP27 levels were independently associated with the occurrence of CVD and sudden cardiac death in patients undergoing dialysis as well as with carotid atherosclerosis and oxidative stress. HSP27 may affect oxLDL-stimulated atherosclerosis by competing with oxLDL for entry into macrophages, reducing ROS production in endothelial cells, and reducing oxidative modifications of LDL (103).
HSP27 exerts a potential therapeutic effect on atherosclerosis by improving endothelial function. Intermittent hypoxia exposure (IHE) can increase the production of ROS and erythropoietin, which is adapted to strenuous exercise. Several studies have demonstrated the protective effects of moderate hypoxia on CVD. Researchers have demonstrated for the first time that IHE combined with physical activity can reduce endothelial dysfunction and atherosclerosis risk by increasing nitric oxide bioavailability and circulating HSP27 levels (104). However, further research is needed to translate hypoxic exposure interventions to the clinical setting. In a study of HSP27-deficient and overexpressing mice, Venu et al. (105) found that HSP27 can affect mRNA levels of endothelial nitric oxide synthase (eNOS) and soluble guanylate cyclase, as well as the proportion of vasodilation that is sensitive to the action of L-NG-nitro arginine methyl ester (L-NAME), thereby influencing nitric oxide-mediated vascular relaxation by regulating intact vascular endothelial function.
Additionally, HSP27 can improve atherosclerosis through anti-inflammatory, anti-stress, and anti-aging pathways. Heat treatment can improve atherosclerotic lesions by upregulating the expression of aortic SIRT1, HSF1, HSP27, HSP72, and HSP73, significantly reducing the plasma levels of triacylglycerol, total cholesterol, and LDL cholesterol, and restoring anti-inflammatory and anti-aging HSRs (106). 4-Phenyl butyric acid (4-PBA) is a small chemical molecular chaperone that was shown to inhibit the growth of atherosclerotic plaques by increasing the expression of HSP27 in ApoE−/− mice. In vitro experiments indicated that 4-PBA treatment inhibited macrophage attachment to human aortic endothelial cells, prevented ER stress-induced cell death, increased the nuclear localization of HSF1 and the expression of HSP27, and may have exerted a therapeutic effect on atherosclerosis through this pathway; however, the exact mechanism needs to be investigated further (107).
In recent years, immunotherapy has become a popular topic in the field of oncology. Next, we review immunotherapy with HSP27 for atherosclerosis that may have efficacy. Previous studies have confirmed that HSP27 is an estrogen receptor β-associated protein and that estrogen induces elevated levels of HSP27 and anti-HSP27 antibodies in healthy subjects compared to the levels in patients with CVD. Experiments on ovariectomized ApoE−/− mice to mimic human menopause found a 65% increase in atherosclerosis burden compared to that in the sham-operated group (28). However, after administration of recombinant HSP27 (rHSP27), atherosclerotic lesions significantly improved. In-depth studies have revealed that the IC formed by rHSP27 vaccination upregulated hepatocyte low-density lipoprotein receptor (LDLR) expression via the NF-κB pathway, leading to increased LDLR for binding proprotein convertase subtilisin/kexin type 9 (PCSK9) and mediating its lysosomal processing (108). Thus, HSP27 immunotherapy is a promising therapeutic strategy. It lowers cholesterol levels by upregulating LDLR expression, promotes clearance of LDL cholesterol, and reduces atherosclerosis formation; however, in-depth studies for clinical applications are warranted.
4.2. Ischemic heart disease
Numerous studies have demonstrated the protective role of HSP27 in reducing ischemic oxidative stress, its high level of endogenous expression in human myocardial tissue, and its apparently important role in improving the development of ischemic heart disease (IHD). Clinical studies in large cohorts have noted that serum HSP27 concentrations are elevated in the first few hours after acute coronary syndrome but fall to levels close to those of healthy individuals approximately 12 h after the onset of chest pain (109). The level of HSP27 expression in peripheral blood mononuclear cells of patients with IHD correlated significantly with disease severity in patients with ≥50% coronary stenosis and can be used as an early prognostic biomarker (110). A study of 400 patients with IHD (111) found that anti-HSP27 titers were significantly higher in patients with IHD than in the control patients. In addition, within the IHD group, anti-HSP27 titers were higher in patients with three-vessel disease than in those with two-vessel and one-vessel disease. Serum anti-HSP27 titers may be associated with the presence and severity of coronary artery disease.
Platelet hyperactivity is a well-known risk factor for IHD and thrombosis. The function of HSP27 is regulated by phosphorylation, which may reflect its cytoprotective function; however, the precise mechanism is not fully understood. Compared with patients with non-ischemic chest pain, patients with MI have significantly increased platelet HSP27 levels and phosphorylation, accompanied by characteristic intracellular translocation of HSP27 from the cytoskeleton to the platelet membrane (112). The future extension of this platelet HSP27 phenotype to other acute ischemic events would be interesting. Chemokine (C-C motif) ligand 2 (CCL2) promotes inflammatory responses and accelerates the progression of IHD. CCL2 knockout mice exhibited markedly diminished platelet aggregation and secretion, accompanied by reduced phosphorylation of protein kinase C alpha (PKCα), p38 MAPK, and HSP27 (113). In-depth studies have revealed that CCL2 increases platelet aggregation, activation, and granule secretion by triggering the PKCα/p38 MAPK/HSP27 signaling pathway in platelets, regulating platelet function, and thus affecting arterial thrombosis. The tomato extract Fruitflow® restored collagen-activated platelet cyclic AMP levels, reduced collagen-induced phosphorylation of protein kinase A substrates, and reduced platelet AKT, glycogen synthase kinase 3β, p38 MAPK, and HSP27 phosphorylation, which is beneficial in people at risk of platelet hyperactivity-induced thrombosis (114). Altered ion homeostasis during I/R is an important trigger of cell death. Inhibition of electrogenic sodium bicarbonate co-transporter isoform 1 (NBCe1) can increase HSP27 phosphorylation while attenuating Drp1-dependent mitochondrial fission by attenuating calcium overload and activating the calcium-regulated neurophosphatase/p38 MAPK/HSP27-dependent pathway, which can improve mitochondrial status and cardiac function after myocardial ischemia (115). Adenine nucleotide translocase (ANT) plays a central role in cellular energy supply and mutations in ANT1 in the heart have been associated with IHD (116). In a rat MI model and a primary cardiomyocyte hypoxia model (117), cardiomyocytes overexpressing ANT1 had enhanced HSP27 expression and secretion under hypoxic conditions; the secreted HSP27 induced the expression of HSP27 and ANT1 by stimulating TLR4-dependent AKT activation. Increased levels of ANT1 and HSP27 expression increased the stability of the mitochondrial membrane potential and inhibited caspase-3/7 activity, exerting cardioprotective effects.
4.3. Atrial fibrillation
Atrial fibrillation (AF) is the most common arrhythmia worldwide and is associated with high morbidity and mortality from ischemic stroke and HF. Disruption of protein homeostasis is the basis of structural and electrical conduction damage. Cells respond to loss of proteostasis by inducing HSR, leading to HSP expression. The sHSP family, which includes HSP27, may be important in maintaining cardiomyocyte proteostasis by stabilizing contractile proteins and may serve as a potential biomarker for predicting AF recurrence after treatment (118).
In a study of 114 patients with AF (119), higher baseline HSP27 levels after catheter ablation were found to predict sinus rhythm maintenance in patients with paroxysmal AF. Baseline HSP27 levels also correlated with IL-10 and TNF-α levels, suggesting that the predictive value of HSP27 in postoperative AF patients may be related to inflammatory responses. A study of 300 patients with AF (120) found that serum HSP27 levels were significantly increased at 3, 6, and 12 months after ablation in patients with AF recurrence compared to those in patients without AF recurrence within 1 year after ablation. Serum HSP27 levels were significantly elevated in patients with AF recurrence within one year of pulmonary vein isolation, and HSP27 levels were predictive of AF recurrence after ablation therapy. In patients with rheumatic heart disease and AF, HSP27 levels were negatively correlated with AF duration and left atrial diameter. Left atrial enlargement and low HSP27 expression were independent predictors of AF in patients with rheumatic heart disease (121).
By establishing an experimental model of chronic AF in dogs, it was found (122) that atrial HSP27 mRNA and protein expression levels were significantly higher in the paced group than in the sham-operated group, while those in the paced + angiotensin 1–7 (Ang-(1–7)) group were significantly lower than those in the paced group. These findings suggest that overexpression of HSP27 is an atrial tissue response to rapid atrial pacing, and Ang-(1–7) may indirectly downregulate the expression of HSP27 by improving atrial remodeling. Previous studies have revealed that HSP-inducing compounds, such as L-glutamine, can reduce the onset and progression of AF. L-glutamine supplementation reduced the serum levels of HSP27 and HSP70 over 3 months and normalized the levels of several metabolites associated with carbohydrate, nucleotide, amino acid, vitamin, and cofactor metabolic pathways (123). Future in-depth investigations of the regulatory role of L-glutamine in AF may be beneficial for its application in clinically therapeutic areas.
4.4. Cardiomyopathy
Cardiomyopathies include a heterogeneous group of diseases characterized by mechanical or electrical disturbances of the myocardium. The etiology of cardiomyopathy is diverse, with the end result being ventricular dysfunction and progressive HF. HSP27 has potential therapeutic value in myocardial diseases with multiple etiologies. Reduced myocardial diastolic function is an early manifestation of diabetic cardiomyopathy. Studies in diabetic rats found that aerobic exercise increased the left ventricular end-diastolic internal diameter and left ventricular end-diastolic volume, upregulated myocardial HSP27 expression, increased HSP27 phosphorylation at the Ser82 site, and enhanced myocardial pHSP27-myosin co-localization in diabetic rats, suggesting that exercise reduces cardiac diastolic dysfunction and repairs damaged myocardial proteins in diabetes (124). Cardiomyocytes from patients with HCM exhibited higher passive stiffness (Fpassive). Protein kinase D treatment increased HSP27 phosphorylation and restored intracellular localization of HSP27 to the Z-disc and I-band, decreasing Fpassive and producing a therapeutic effect on the diastolic left ventricular dysfunction associated with high cardiomyocyte stiffness (125). The term “desminopathies” refer to a clinically heterogeneous group of familial and sporadic myopathies and cardiomyopathies that are caused by mutations in the human desmin gene on chromosome 2q35 (126). Studies using an R349P desmin knock-in mouse model revealed that the mutant desmin increased proteasome activity, stimulated macroautophagy, induced chaperone-assisted selective autophagy dysregulation, and increased protein levels of αB-crystallin and HSP27, which translocated from the Z-disc to the level of I-band myelin. This finding provides a basis for further pharmacological and genetic intervention studies (127) (Table 2).
5. Prospects and outlook
As an intracellular chaperone protein, HSP27 can be upregulated under a variety of stressful stimuli and mediates pathophysiological processes, such as cellular oxidative stress, inflammatory responses, apoptosis, autophagy, and fibrosis. Numerous studies have revealed its cytoprotective role; however, there are still some questions that need to be addressed, including: Are intracellular and extracellular HSP27 functions consistent? Is the presence of other proteins necessary for HSP27 to perform its physiological function? What is the effect of post-translational modifications other than phosphorylation on HSP27 function? The resolution of these issues is necessary to deeply explore the physiological functions of HSP27 and thus develop disease therapeutics to target it.
In addition, HSP27 can influence the developmental processes of various diseases. HSP27 is strongly induced by physiological stress and anticancer drugs. The powerful cytoprotective function of HSP27 and the fact that this protein is overexpressed in most cancers make this chaperone an attractive target in cancer therapy. HSP27 depletion in various cancer models induces tumor regression, and the antisense oligonucleotide OGX-427, a specific inhibitor of HSP27, is currently undergoing phase II clinical trials. However, its application in the field of cardiovascular disease treatment is still lacking, and therapeutic protocols for improving diseases including atherosclerosis, ischemic heart disease, cardiac arrhythmias, and cardiomyopathies are still at the stage of cell or animal experiments, and the results of relevant clinical studies are needed. Thus, future HSP27-related research in cardiovascular diseases remains to be conducted.
Author contributions
BL contributed to conception, design and drafting of the manuscript. YZ, HS, NL, HW, and XS contributed to data collection and manuscript drafting. All authors contributed to the article and approved the submitted version.
Funding
This work was supported by the Jilin Provincial Department of Science and Technology (grant numbers 20220303002SF); Jilin Provincial Health Care Commission (grant numbers 2021LC016); and Jilin Provincial Development and Reform Commission (grant numbers 2022C003).
Conflict of interest
The authors declare that the research was conducted in the absence of any commercial or financial relationships that could be construed as a potential conflict of interest.
Publisher's note
All claims expressed in this article are solely those of the authors and do not necessarily represent those of their affiliated organizations, or those of the publisher, the editors and the reviewers. Any product that may be evaluated in this article, or claim that may be made by its manufacturer, is not guaranteed or endorsed by the publisher.
References
1. Krishnan-Sivadoss I, Mijares-Rojas IA, Villarreal-Leal RA, Torre-Amione G, Knowlton AA, Guerrero-Beltran CE. Heat shock protein 60 and cardiovascular diseases: an intricate love-hate story. Med Res Rev. (2021) 41(1):29–71. doi: 10.1002/med.21723
2. Sun X, Siri S, Hurst A, Qiu H. Heat shock protein 22 in physiological and pathological hearts: small molecule, large potentials. Cells. (2021) 11(1):114. doi: 10.3390/cells11010114
3. Dabravolski SA, Sukhorukov VN, Kalmykov VA, Orekhov NA, Grechko AV, Orekhov AN. Heat shock protein 90 as therapeutic target for cvds and heart ageing. Int J Mol Sci. (2022) 23(2):649. doi: 10.3390/ijms23020649
4. Angelini G, Castagneto-Gissey L, Salinari S, Bertuzzi A, Anello D, Pradhan M, et al. Upper gut heat shock proteins Hsp70 and Grp78 promote insulin resistance, hyperglycemia, and non-alcoholic steatohepatitis. Nat Commun. (2022) 13(1):7715. doi: 10.1038/s41467-022-35310-5
5. Yoon S, Gergs U, McMullen JR, Eom GH. Overexpression of heat shock protein 70 improves cardiac remodeling and survival in protein phosphatase 2a-expressing transgenic mice with chronic heart failure. Cells. (2021) 10(11):3180. doi: 10.3390/cells10113180
6. Nandi SK, Panda AK, Chakraborty A, Rathee S, Roy I, Barik S, et al. Role of Atp-small heat shock protein interaction in human diseases. Front Mol Biosci. (2022) 9:844826. doi: 10.3389/fmolb.2022.844826
7. Szyller J, Bil-Lula I. Heat shock proteins in oxidative stress and ischemia/reperfusion injury and benefits from physical exercises: a review to the current knowledge. Oxid Med Cell Longev. (2021) 2021:6678457. doi: 10.1155/2021/6678457
8. Roberts RJ, Hallee L, Lam CK. The potential of Hsp90 in targeting pathological pathways in cardiac diseases. J Pers Med. (2021) 11(12):1373. doi: 10.3390/jpm11121373
9. Kurop MK, Huyen CM, Kelly JH, Blagg BSJ. The heat shock response and small molecule regulators. Eur J Med Chem. (2021) 226:113846. doi: 10.1016/j.ejmech.2021.113846
10. Lang BJ, Guerrero ME, Prince TL, Okusha Y, Bonorino C, Calderwood SK. The functions and regulation of heat shock proteins; key orchestrators of proteostasis and the heat shock response. Arch Toxicol. (2021) 95(6):1943–70. doi: 10.1007/s00204-021-03070-8
11. Reinle K, Mogk A, Bukau B. The diverse functions of small heat shock proteins in the proteostasis network. J Mol Biol. (2022) 434(1):167157. doi: 10.1016/j.jmb.2021.167157
12. Johnston CL, Marzano NR, van Oijen AM, Ecroyd H. Using single-molecule approaches to understand the molecular mechanisms of heat-shock protein chaperone function. J Mol Biol. (2018) 430(22):4525–46. doi: 10.1016/j.jmb.2018.05.021
13. Yu C, Leung SKP, Zhang W, Lai LTF, Chan YK, Wong MC, et al. Structural basis of substrate recognition and thermal protection by a small heat shock protein. Nat Commun. (2021) 12(1):3007. doi: 10.1038/s41467-021-23338-y
14. Janowska MK, Baughman HER, Woods CN, Klevit RE. Mechanisms of small heat shock proteins. Cold Spring Harb Perspect Biol. (2019) 11(10):a034025. doi: 10.1101/cshperspect.a034025
15. Ju H, Hu Z, Wei D, Huang J, Zhang X, Rui M, et al. A novel intronic circular rna, Circgng7, inhibits head and neck squamous cell carcinoma progression by blocking the phosphorylation of heat shock protein 27 at Ser78 and Ser82. Cancer Commun (Lond). (2021) 41(11):1152–72. doi: 10.1002/cac2.12213
16. Hwang SY, Choi SK, Seo SH, Jo H, Shin JH, Na Y, et al. Specific roles of Hsp27 S15 phosphorylation augmenting the nuclear function of Her2 to promote trastuzumab resistance. Cancers (Basel). (2020) 12(6):1540. doi: 10.3390/cancers12061540
17. Hoffman LM, Jensen CC, Beckerle MC. Phosphorylation of the small heat shock protein Hspb1 regulates cytoskeletal recruitment and cell motility. Mol Biol Cell. (2022) 33(11):ar100. doi: 10.1091/mbc.E22-02-0057
18. Sun X, Ou Z, Xie M, Kang R, Fan Y, Niu X, et al. Hspb1 as a novel regulator of ferroptotic cancer cell death. Oncogene. (2015) 34(45):5617–25. doi: 10.1038/onc.2015.32
19. Yang S, Xiao H, Cao L. Recent advances in heat shock proteins in cancer diagnosis, prognosis, metabolism and treatment. Biomed Pharmacother. (2021) 142:112074. doi: 10.1016/j.biopha.2021.112074
20. Holguin BA, Hildenbrand ZL, Bernal RA. Insights into the role of heat shock protein 27 in the development of neurodegeneration. Front Mol Neurosci. (2022) 15:868089. doi: 10.3389/fnmol.2022.868089
21. Alexander CC, Munkascy E, Tillmon H, Fraker T, Scheirer J, Holstein D, et al. Hspb1 overexpression improves life span and stress resistance in an invertebrate model. J Gerontol A Biol Sci Med Sci. (2022) 77(2):268–75. doi: 10.1093/gerona/glab296
22. Heiserman JP, Nallanthighal S, Gifford CC, Graham K, Samarakoon R, Gao C, et al. Heat shock protein 27, a novel downstream target of collagen type Xi alpha 1, synergizes with fatty acid oxidation to confer cisplatin resistance in ovarian cancer cells. Cancers (Basel). (2021) 13(19):4855. doi: 10.3390/cancers13194855
23. Hrudka J, Jelinkova K, Fiserova H, Matej R, Mandys V, Waldauf P. Heat shock proteins 27, 70, and 110: expression and prognostic significance in colorectal cancer. Cancers (Basel). (2021) 13(17):4407. doi: 10.3390/cancers13174407
24. Hayashi J, Ton J, Negi S, Stephens D, Pountney DL, Preiss T, et al. The effect of oxidized dopamine on the structure and molecular chaperone function of the small heat-shock proteins, alphab-crystallin and Hsp27. Int J Mol Sci. (2021) 22(7):3700. doi: 10.3390/ijms22073700
25. Navarro-Zaragoza J, Cuenca-Bermejo L, Almela P, Laorden ML, Herrero MT. Could small heat shock protein Hsp27 be a first-line target for preventing protein aggregation in Parkinson’s disease? Int J Mol Sci. (2021) 22(6):3038. doi: 10.3390/ijms22063038
26. Kaku H, Balaj AR, Rothstein TL. Small heat shock proteins collaborate with faim to prevent accumulation of misfolded protein aggregates. Int J Mol Sci. (2022) 23(19):11841. doi: 10.3390/ijms231911841
27. Shan R, Liu N, Yan Y, Apoptosis LB. Autophagy and atherosclerosis: relationships and the role of Hsp27. Pharmacol Res. (2021) 166:105169. doi: 10.1016/j.phrs.2020.105169
28. Maarouf N, Chen YX, Shi C, Deng J, Diao C, Rosin M, et al. Unlike estrogens that increase Pcsk9 levels post-menopause Hsp27 vaccination lowers cholesterol levels and atherogenesis due to divergent effects on Pcsk9 and ldlr. Pharmacol Res. (2020) 161:105222. doi: 10.1016/j.phrs.2020.105222
29. Inia JA, O’Brien ER. Role of heat shock protein 27 in modulating atherosclerotic inflammation. J Cardiovasc Transl Res. (2021) 14(1):3–12. doi: 10.1007/s12265-020-10000-z
30. Martinez-Laorden E, Navarro-Zaragoza J, Milanes MV, Laorden ML, Almela P. Cardiac protective role of heat shock protein 27 in the stress induced by drugs of abuse. Int J Mol Sci. (2020) 21(10):3623. doi: 10.3390/ijms21103623
31. Wenzl FA, Ambrosini S, Paneni F. Molecular underpinnings of myocardial stiffness in patients with hypertrophic cardiomyopathy. Int J Cardiol. (2021) 343:80–2. doi: 10.1016/j.ijcard.2021.09.024
32. Hassoun R, Budde H, Zhazykbayeva S, Herwig M, Sieme M, Delalat S, et al. Stress activated signalling impaired protein quality control pathways in human hypertrophic cardiomyopathy. Int J Cardiol. (2021) 344:160–9. doi: 10.1016/j.ijcard.2021.09.009
33. Terra LF, Wailemann RAM, Dos Santos AF, Gomes VM, Silva RP, Laporte A, et al. Heat shock protein B1 is a key mediator of prolactin-induced Beta-cell cytoprotection against oxidative stress. Free Radic Biol Med. (2019) 134:394–405. doi: 10.1016/j.freeradbiomed.2019.01.023
34. Navarro-Zaragoza J, Ros-Simo C, Milanes MV, Valverde O, Laorden ML. Binge ethanol and mdma combination exacerbates Hsp27 and Trx-1 (biomarkers of toxic cardiac effects) expression in right ventricle. Life Sci. (2019) 220:50–7. doi: 10.1016/j.lfs.2019.01.050
35. Liu X, Liu K, Li C, Cai J, Huang L, Chen H, et al. Heat-shock protein B1 upholds the cytoplasm reduced state to inhibit activation of the hippo pathway in H9c2 cells. J Cell Physiol. (2019) 234(4):5117–33. doi: 10.1002/jcp.27322
36. Dwivedi S, D’Souza LC, Shetty NG, Raghu SV, Sharma A. Hsp27, a potential ecr target, protects nonylphenol-induced cellular and organismal toxicity in Drosophila melanogaster. Environ Pollut. (2022) 293:118484. doi: 10.1016/j.envpol.2021.118484
37. Grottelli S, Mezzasoma L, Scarpelli P, Cacciatore I, Cellini B, Bellezza I. Cyclo(his-pro) inhibits Nlrp3 inflammasome cascade in als microglial cells. Mol Cell Neurosci. (2019) 94:23–31. doi: 10.1016/j.mcn.2018.11.002
38. Kim JY, An YM, Yoo BR, Kim JM, Han SY, Na Y, et al. Hsp27 inhibitor attenuates radiation-induced pulmonary inflammation. Sci Rep. (2018) 8(1):4189. doi: 10.1038/s41598-018-22635-9
39. Wang ZY, Li A, Huang X, Bai GL, Jiang YX, Li RL, et al. Hsp27 protects skin from ultraviolet B -induced photodamage by regulating autophagy and reactive oxygen Species production. Front Cell Dev Biol. (2022) 10:852244. doi: 10.3389/fcell.2022.852244
40. Zhao LJ, Dong BS, Zhang H, Ding DF, Guan HZ, Li YF, et al. Intermittent pressure imitating rolling manipulation ameliorates injury in skeletal muscle cells through oxidative stress and lipid metabolism signalling pathways. Gene. (2021) 778:145460. doi: 10.1016/j.gene.2021.145460
41. Wu CW, Tessier SN, Storey KB. Stress-induced antioxidant defense and protein chaperone response in the freeze-tolerant wood frog rana Sylvatica. Cell Stress Chaperones. (2018) 23(6):1205–17. doi: 10.1007/s12192-018-0926-x
42. Yamamoto Y, Hosoda K, Imahori T, Tanaka J, Matsuo K, Nakai T, et al. Pentose phosphate pathway activation via Hsp27 phosphorylation by Atm kinase: a putative endogenous antioxidant defense mechanism during cerebral ischemia-reperfusion. Brain Res. (2018) 1687:82–94. doi: 10.1016/j.brainres.2018.03.001
43. Matsuo K, Hosoda K, Tanaka J, Yamamoto Y, Imahori T, Nakai T, et al. Geranylgeranylacetone attenuates cerebral ischemia-reperfusion injury in rats through the augmentation of Hsp 27 phosphorylation: a preliminary study. BMC Neurosci. (2021) 22(1):9. doi: 10.1186/s12868-021-00614-7
44. Fouani M, Basset CA, Mangano GD, Leone LG, Lawand NB, Leone A, et al. Heat shock proteins alterations in rheumatoid arthritis. Int J Mol Sci. (2022) 23(5):2806. doi: 10.3390/ijms23052806
45. Traxler D, Zimmermann M, Simader E, Veraar CM, Moser B, Mueller T, et al. The inflammatory markers Sst2, Hsp27 and Hscrp as a prognostic biomarker panel in chronic heart failure patients. Clin Chim Acta. (2020) 510:507–14. doi: 10.1016/j.cca.2020.07.050
46. Kaiser F, Donos N, Henderson B, Alagarswamy R, Pelekos G, Boniface D, et al. Association between circulating levels of heat-shock protein 27 and aggressive periodontitis. Cell Stress Chaperones. (2018) 23(5):847–56. doi: 10.1007/s12192-018-0891-4
47. Yanda MK, Guggino WB, Cebotaru L. A new role for heat shock factor 27 in the pathophysiology of Clostridium Difficile toxin B. Am J Physiol Gastrointest Liver Physiol. (2020) 318(1):G120–G9. doi: 10.1152/ajpgi.00166.2019
48. Zong Q, Jing P, Sun S, Wang H, Wu S, Bao W. Effects of Hsp27 gene expression on the resistance to Escherichia Coli infection in piglets. Gene. (2021) 773:145415. doi: 10.1016/j.gene.2021.145415
49. Kim TJ, Lee HJ, Pyun DH, Abd El-Aty AM, Jeong JH, Jung TW. Valdecoxib improves lipid-induced skeletal muscle insulin resistance via simultaneous suppression of inflammation and endoplasmic Reticulum stress. Biochem Pharmacol. (2021) 188:114557. doi: 10.1016/j.bcp.2021.114557
50. Du Y, Liu XH, Zhu HC, Wang L, Wang ZS, Ning JZ, et al. Hydrogen sulfide treatment protects against renal ischemia-reperfusion injury via induction of heat shock proteins in rats. Iran J Basic Med Sci. (2019) 22(1):99–105. doi: 10.22038/ijbms.2018.29706.7170
51. Wang Y, Liu J, Kong Q, Cheng H, Tu F, Yu P, et al. Cardiomyocyte-specific deficiency of Hspb1 worsens cardiac dysfunction by activating nfkappab-mediated leucocyte recruitment after myocardial infarction. Cardiovasc Res. (2019) 115(1):154–67. doi: 10.1093/cvr/cvy163
52. Park AM, Tsunoda I, Yoshie O. Heat shock protein 27 promotes cell cycle progression by down-regulating E2f transcription factor 4 and retinoblastoma family protein P130. J Biol Chem. (2018) 293(41):15815–26. doi: 10.1074/jbc.RA118.003310
53. Rada CC, Mejia-Pena H, Grimsey NJ, Canto Cordova I, Olson J, Wozniak JM, et al. Heat shock protein 27 activity is linked to endothelial barrier recovery after proinflammatory gpcr-induced disruption. Sci Signal. (2021) 14(698):eabc1044. doi: 10.1126/scisignal.abc1044
54. Zhang C, Jones JT, Chand HS, Wathelet MG, Evans CM, Dickey B, et al. Noxa/Hsp27 Complex delays degradation of ubiquitylated ikbalpha in airway epithelial cells to reduce pulmonary inflammation. Mucosal Immunol. (2018) 11(3):741–51. doi: 10.1038/mi.2017.117
55. Bi X, Jiang B, Zhou J, Luo L, Yin Z. Phosphorylated Hsp27 prevents lps-induced excessive inflammation in thp-1 cells via suppressing ros-mediated upregulation of cbp. Cell Biol Int. (2020) 44(1):253–67. doi: 10.1002/cbin.11228
56. Zhang Y, Wang X, Wang S, Yan Z, Li C, Zheng Y, et al. Heat shock protein 27 regulates the inflammatory response of intestinal epithelial cells by the nuclear factor-kappab pathway. Dig Dis Sci. (2020) 65(12):3514–20. doi: 10.1007/s10620-020-06074-z
57. Bi X, Xu M, Li J, Huang T, Jiang B, Shen L, et al. Heat shock protein 27 inhibits Hmgb1 translocation by regulating cbp acetyltransferase activity and ubiquitination. Mol Immunol. (2019) 108:45–55. doi: 10.1016/j.molimm.2019.02.008
58. Batulan Z, Pulakazhi Venu VK, Li Y, Koumbadinga G, Alvarez-Olmedo DG, Shi C, et al. Extracellular release and signaling by heat shock protein 27: role in modifying vascular inflammation. Front Immunol. (2016) 7:285. doi: 10.3389/fimmu.2016.00285
59. Shi C, Deng J, Chiu M, Chen YX, O’Brien ER. Heat shock protein 27 immune complex altered signaling and transport (icast): novel mechanisms of attenuating inflammation. FASEB J. (2020) 34(11):14287–301. doi: 10.1096/fj.202001389RR
60. Shi C, Alvarez-Olmedo D, Zhang Y, Pattar BSB, O’Brien ER. The heat shock protein 27 immune complex enhances exosomal cholesterol efflux. Biomedicines. (2020) 8(8):290. doi: 10.3390/biomedicines8080290
61. Reddy VS, Madala SK, Trinath J, Reddy GB. Extracellular small heat shock proteins: exosomal biogenesis and function. Cell Stress Chaperones. (2018) 23(3):441–54. doi: 10.1007/s12192-017-0856-z
62. Shi C, Ulke-Lemee A, Deng J, Batulan Z, O’Brien ER. Characterization of heat shock protein 27 in extracellular vesicles: a potential anti-inflammatory therapy. FASEB J. (2019) 33(2):1617–30. doi: 10.1096/fj.201800987R
63. Zhang HL, Jia KY, Sun D, Yang M. Protective effect of Hsp27 in atherosclerosis and coronary heart disease by inhibiting reactive oxygen Species. J Cell Biochem. (2019) 120(3):2859–68. doi: 10.1002/jcb.26575
64. Yang F, Li T, Dong Z, Mi R. Microrna-410 is involved in mitophagy after cardiac ischemia/reperfusion injury by targeting high-mobility group box 1 protein. J Cell Biochem. (2018) 119(2):2427–39. doi: 10.1002/jcb.26405
65. Zhou ZB, Huang GX, Lu JJ, Ma J, Yuan QJ, Cao Y, et al. Up-regulation of heat shock protein 27 inhibits apoptosis in lumbosacral nerve root avulsion-induced neurons. Sci Rep. (2019) 9(1):11468. doi: 10.1038/s41598-019-48003-9
66. Zhou XY, Sun JY, Wang WQ, Li SX, Li HX, Yang HJ, et al. Tat-Hsp27 peptide improves neurologic deficits via reducing apoptosis after experimental subarachnoid hemorrhage. Front Cell Neurosci. (2022) 16:878673. doi: 10.3389/fncel.2022.878673
67. Cui B, Wang Y, Jin J, Yang Z, Guo R, Li X, et al. Resveratrol treats uvb-induced photoaging by anti-mmp expression, through anti-inflammatory, antioxidant, and antiapoptotic properties, and treats photoaging by upregulating vegf-B expression. Oxid Med Cell Longev. (2022) 2022:6037303. doi: 10.1155/2022/6037303
68. Liu Y, Huang X, Wang P, Pan Y, Cao D, Liu C, et al. The effects of Hsp27 against uvb-induced photoaging in rat skin. Biochem Biophys Res Commun. (2019) 512(3):435–40. doi: 10.1016/j.bbrc.2019.03.076
69. Liu C, Huang X, Wang P, Pan Y, Cao D, Liu YY, et al. Interference of Hsp27 results in apoptosis induced by photodamage via regulation of subcellular localization of P21 in immortalized human keratinocytes. Med Sci Monit. (2019) 25:7557–66. doi: 10.12659/MSM.917164
70. Burban A, Sharanek A, Guguen-Guillouzo C, Guillouzo A. Endoplasmic reticulum stress precedes oxidative stress in antibiotic-induced cholestasis and cytotoxicity in human hepatocytes. Free Radic Biol Med. (2018) 115:166–78. doi: 10.1016/j.freeradbiomed.2017.11.017
71. Grotegut P, Hoerdemann PJ, Reinehr S, Gupta N, Dick HB, Joachim SC. Heat shock protein 27 injection leads to caspase activation in the visual pathway and retinal T-cell response. Int J Mol Sci. (2021) 22(2):513. doi: 10.3390/ijms22020513
72. Onay Ucar E, Sengelen A. Resveratrol and sirna in combination reduces Hsp27 expression and induces caspase-3 activity in human glioblastoma cells. Cell Stress Chaperones. (2019) 24(4):763–75. doi: 10.1007/s12192-019-01004-z
73. Liu Z, Liu Y, Long Y, Liu B, Wang X. Role of Hsp27 in the multidrug sensitivity and resistance of colon cancer cells. Oncol Lett. (2020) 19(3):2021–7. doi: 10.3892/ol.2020.11255
74. Liang HH, Huang CY, Chou CW, Makondi PT, Huang MT, Wei PL, et al. Heat shock protein 27 influences the anti-cancer effect of curcumin in colon cancer cells through ros production and autophagy activation. Life Sci. (2018) 209:43–51. doi: 10.1016/j.lfs.2018.07.047
75. He Z, Shen F, Qi P, Zhai Z, Wang Z. Mir-541-3p enhances the radiosensitivity of prostate cancer cells by inhibiting Hsp27 expression and downregulating Beta-catenin. Cell Death Discov. (2021) 7(1):18. doi: 10.1038/s41420-020-00387-8
76. Shen C, Liu W, Zhang S, Pu L, Deng B, Zeng Q, et al. Downregulation of Mir-541 induced by heat stress contributes to malignant transformation of human bronchial epithelial cells via Hsp27. Environ Res. (2020) 184:108954. doi: 10.1016/j.envres.2019.108954
77. Xu L, Lin X, Zheng Y, Zhou H. Silencing of heat shock protein 27 increases the radiosensitivity of non-small cell lung carcinoma cells. Mol Med Rep. (2019) 20(1):613–21. doi: 10.3892/mmr.2019.10263
78. Chine VB, Au NPB, Kumar G, Ma CHE. Targeting axon integrity to prevent chemotherapy-induced peripheral neuropathy. Mol Neurobiol. (2019) 56(5):3244–59. doi: 10.1007/s12035-018-1301-8
79. Chine VB, Au NPB, Ma CHE. Therapeutic benefits of maintaining mitochondrial integrity and calcium homeostasis by forced expression of Hsp27 in chemotherapy-induced peripheral neuropathy. Neurobiol Dis. (2019) 130:104492. doi: 10.1016/j.nbd.2019.104492
80. Ramani S, Park S. Hsp27 role in cardioprotection by modulating chemotherapeutic doxorubicin-induced cell death. J Mol Med (Berl). (2021) 99(6):771–84. doi: 10.1007/s00109-021-02048-4
81. Kanagasabai R, Karthikeyan K, Zweier JL, Ilangovan G. Serine mutations in overexpressed Hsp27 abrogate the protection against doxorubicin-induced P53-dependent cardiac apoptosis in mice. Am J Physiol Heart Circ Physiol. (2021) 321(5):H963–75. doi: 10.1152/ajpheart.00027.2020
82. Zhang L, Zhou J, Jing Z, Xiao Y, Sun Y, Wu Y, et al. Glucocorticoids regulate the vascular remodeling of aortic dissection via the P38 Mapk-Hsp27 pathway mediated by soluble Tnf-Rii. EBioMedicine. (2018) 27:247–57. doi: 10.1016/j.ebiom.2017.12.002
83. Chen H, Lu N, Xu C, Zheng M. The role of heat shock protein 27 phosphorylation in the proliferation and apoptosis of human umbilical vein endothelial cells induced by visfatin. Microvasc Res. (2019) 121:30–6. doi: 10.1016/j.mvr.2018.08.003
84. Yin CF, Kao SC, Hsu CL, Chang YW, Cheung CHY, Huang HC, et al. Phosphoproteome analysis reveals dynamic heat shock protein 27 phosphorylation in tanshinone iia-induced cell death. J Proteome Res. (2020) 19(4):1620–34. doi: 10.1021/acs.jproteome.9b00836
85. Grierson PM, Dodhiawala PB, Cheng Y, Chen TH, Khawar IA, Wei Q, et al. The Mk2/Hsp27 axis is a major survival mechanism for pancreatic ductal adenocarcinoma under genotoxic stress. Sci Transl Med. (2021) 13(622):eabb5445. doi: 10.1126/scitranslmed.abb5445
86. Yao Y, Cui L, Ye J, Yang G, Lu G, Fang X, et al. Dioscin facilitates ros-induced apoptosis via the P38-mapk/Hsp27-mediated pathways in lung squamous cell carcinoma. Int J Biol Sci. (2020) 16(15):2883–94. doi: 10.7150/ijbs.45710
87. Wang G, Jiao H, Zheng JN, Sun X. Hsp27 regulates Tgf-Beta mediated lung fibroblast differentiation through the Smad3 and erk pathways. Int J Mol Med. (2017) 39(1):183–90. doi: 10.3892/ijmm.2016.2813
88. Sevin M, Kubovcakova L, Pernet N, Causse S, Vitte F, Villeval JL, et al. Hsp27 is a partner of Jak2-Stat5 and a potential therapeutic target in myelofibrosis. Nat Commun. (2018) 9(1):1431. doi: 10.1038/s41467-018-03627-9
89. Jin S, Li J, Barati M, Rane S, Lin Q, Tan Y, et al. Loss of Nf-E2 expression contributes to the induction of profibrotic signaling in diabetic kidneys. Life Sci. (2020) 254:117783. doi: 10.1016/j.lfs.2020.117783
90. Kim N, Ullah I, Chung K, Lee D, Cha MJ, Ban H, et al. Targeted delivery of recombinant heat shock protein 27 to cardiomyocytes promotes recovery from myocardial infarction. Mol Pharm. (2020) 17(6):2034–43. doi: 10.1021/acs.molpharmaceut.0c00192
91. Marconi GD, Fonticoli L, Rajan TS, Pierdomenico SD, Trubiani O, Pizzicannella J, et al. Epithelial-mesenchymal transition (Emt): the type-2 emt in wound healing, tissue regeneration and organ fibrosis. Cells. (2021) 10(7):1587. doi: 10.3390/cells10071587
92. Oh A, Jeon S, Jeong MG, Kim HK, Kang J, Lee YS, et al. Hspb1 inhibitor J2 attenuates lung inflammation through direct modulation of Ym1 production and paracrine signaling. Biomed Pharmacother. (2021) 143:112225. doi: 10.1016/j.biopha.2021.112225
93. Kim JY, Jeon S, Yoo YJ, Jin H, Won HY, Yoon K, et al. The Hsp27-mediated ikbalpha-nfkappab signaling axis promotes radiation-induced lung fibrosis. Clin Cancer Res. (2019) 25(17):5364–75. doi: 10.1158/1078-0432.CCR-18-3900
94. Zhang XL, Zhang XY, Ge XQ, Liu MX. Mangiferin prevents hepatocyte epithelial-mesenchymal transition in liver fibrosis via targeting Hsp27-mediated Jak2/Stat3 and Tgf-Beta1/smad pathway. Phytother Res. (2022) 36(11):4167–82. doi: 10.1002/ptr.7549
95. Zhang X, Liu T, Zheng S, Liu Q, Shen T, Han X, et al. Sumoylation of Hsp27 regulates Pkm2 to promote esophageal squamous cell carcinoma progression. Oncol Rep. (2020) 44(4):1355–64. doi: 10.3892/or.2020.7711
96. Gao J, Ma Y, Yang G, Li G. Translationally controlled tumor protein: the mediator promoting cancer invasion and migration and its potential clinical prospects. J Zhejiang Univ Sci B. (2022) 23(8):642–54. doi: 10.1631/jzus.B2100910
97. Kabakov A, Yakimova A, Matchuk O. Molecular chaperones in cancer stem cells: determinants of stemness and potential targets for antitumor therapy. Cells. (2020) 9(4):892. doi: 10.3390/cells9040892
98. Hashikawa N, Ido M, Morita Y, Hashikawa-Hobara N. Effects from the induction of heat shock proteins in a murine model due to progression of aortic atherosclerosis. Sci Rep. (2021) 11(1):7025. doi: 10.1038/s41598-021-86601-8
99. Seibert TA, Hibbert B, Chen YX, Rayner K, Simard T, Hu T, et al. Serum heat shock protein 27 levels represent a potential therapeutic target for atherosclerosis: observations from a human cohort and treatment of female mice. J Am Coll Cardiol. (2013) 62(16):1446–54. doi: 10.1016/j.jacc.2013.05.041
100. Ghazizadeh H, Mirinezhad MR, Seyedi SMR, Sadabadi F, Ahmadnezhad M, Jaberi N, et al. Prognostic factors associating with pro-oxidant-antioxidant balance; neutrophils to lymphocytes ratio, vitamin D, heat shock protein 27, and red cell distribution width. Arch Med Res. (2020) 51(3):261–7. doi: 10.1016/j.arcmed.2020.02.006
101. Wang X, Shi J, Lu B, Zhang W, Yang Y, Wen J, et al. Circulating heat shock protein 27 as a novel marker of subclinical atherosclerosis in type 2 diabetes: a cross-sectional community-based study. BMC Cardiovasc Disord. (2020) 20(1):198. doi: 10.1186/s12872-020-01456-7
102. Bai J, Wang F, Wang X, Mutu E, Duan C, Qi Y, et al. Expression and clinical significance of Hsp27 and its phosphorylation in lower extremity arteriosclerosis obliterans. PeerJ. (2020) 8:e9305. doi: 10.7717/peerj.9305
103. Jaroszynski A, Jaroszynska A, Zaborowski T, Drelich-Zbroja A, Zapolski T, Dabrowski W. Serum heat shock protein 27 levels predict cardiac mortality in hemodialysis patients. BMC Nephrol. (2018) 19(1):359. doi: 10.1186/s12882-018-1157-1
104. Zembron-Lacny A, Tylutka A, Wacka E, Wawrzyniak-Gramacka E, Hiczkiewicz D, Kasperska A, et al. Intermittent hypoxic exposure reduces endothelial dysfunction. Biomed Res Int. (2020) 2020:6479630. doi: 10.1155/2020/6479630
105. Pulakazhi Venu VK, Saifeddine M, Mihara K, El-Daly M, Belke D, Dean JLE, et al. Heat shock protein-27 and sex-selective regulation of muscarinic and proteinase-activated receptor 2-mediated vasodilatation: differential sensitivity to endothelial nos inhibition. Br J Pharmacol. (2018) 175(11):2063–76. doi: 10.1111/bph.14200
106. Bruxel MA, Tavares AMV, Zavarize Neto LD, de Souza Borges V, Schroeder HT, Bock PM, et al. Chronic whole-body heat treatment relieves atherosclerotic lesions, cardiovascular and metabolic abnormalities, and enhances survival time restoring the anti-inflammatory and anti-senescent heat shock response in mice. Biochimie. (2019) 156:33–46. doi: 10.1016/j.biochi.2018.09.011
107. Lynn EG, Lhotak S, Lebeau P, Byun JH, Chen J, Platko K, et al. 4-Phenylbutyrate protects against atherosclerotic lesion growth by increasing the expression of Hsp25 in macrophages and in the circulation of apoe(−/−) mice. FASEB J. (2019) 33(7):8406–22. doi: 10.1096/fj.201802293RR
108. Chen YX, Shi C, Deng J, Diao C, Maarouf N, Rosin M, et al. Hsp25 vaccination attenuates atherogenesis via upregulation of ldlr expression, lowering of Pcsk9 levels and curbing of inflammation. Arterioscler Thromb Vasc Biol. (2021) 41(6):e338–53. doi: 10.1161/ATVBAHA.121.315933
109. Heidari-Bakavoli AR, Sahebkar A, Mobara N, Moohebati M, Tavallaie S, Rahsepar AA, et al. Changes in plasma level of heat shock protein 27 after acute coronary syndrome. Angiology. (2012) 63(1):12–6. doi: 10.1177/0003319711406104
110. Abaspour AR, Taghikhani M, Parizadeh SMR, Seyedi SMR, Ghazizadeh H, Kazemi E, et al. Hsp27 expression in the human peripheral blood mononuclear cells as an early prognostic biomarker in coronary artery disease patients. Diabetes Metab Syndr. (2019) 13(3):1791–5. doi: 10.1016/j.dsx.2019.04.010
111. Pourghadamyari H, Moohebati M, Parizadeh SM, Falsoleiman H, Dehghani M, Fazlinezhad A, et al. Serum antibody titers against heat shock protein 27 are associated with the severity of coronary artery disease. Cell Stress Chaperones. (2011) 16(3):309–16. doi: 10.1007/s12192-010-0241-7
112. Kraemer BF, Mannell H, Lamkemeyer T, Franz-Wachtel M, Lindemann S. Heat-shock protein 27 (Hspb1) is upregulated and phosphorylated in human platelets during st-elevation myocardial infarction. Int J Mol Sci. (2019) 20(23):5968. doi: 10.3390/ijms20235968
113. Liu D, Cao Y, Zhang X, Peng C, Tian X, Yan C, et al. Chemokine Cc-motif ligand 2 participates in platelet function and arterial thrombosis by regulating pkcalpha-P38mapk-Hsp27 pathway. Biochim Biophys Acta Mol Basis Dis. (2018) 1864(9 Pt B):2901–12. doi: 10.1016/j.bbadis.2018.05.025
114. Zhang S, Chen H, Li C, Chen B, Gong H, Zhao Y, et al. Water-soluble tomato extract fruitflow alters the phosphoproteomic profile of collagen-stimulated platelets. Front Pharmacol. (2021) 12:746107. doi: 10.3389/fphar.2021.746107
115. Ciocci Pardo A, Gonzalez Arbelaez LF, Fantinelli JC, Aiello EA, Mosca SM. Calcineurin/P38mapk/Hsp27-dependent pathways are involved in the attenuation of postischemic mitochondrial injury afforded by sodium bicarbonate co-transporter (Nbce1) inhibition. Biochem Pharmacol. (2019) 161:26–36. doi: 10.1016/j.bcp.2019.01.002
116. Yergoz F, Friebel J, Krankel N, Rauch-Kroehnert U, Schultheiss HP, Landmesser U, et al. Adenine nucleotide translocase 1 expression modulates the immune response in ischemic hearts. Cells. (2021) 10(8):2130. doi: 10.3390/cells10082130
117. Winter J, Hammer E, Heger J, Schultheiss HP, Rauch U, Landmesser U, et al. Adenine nucleotide translocase 1 expression is coupled to the Hsp27-mediated Tlr4 signaling in cardiomyocytes. Cells. (2019) 8(12):1588. doi: 10.3390/cells8121588
118. van Wijk SW, Ramos KS, Brundel B. Cardioprotective role of heat shock proteins in atrial fibrillation: from mechanism of action to therapeutic and diagnostic target. Int J Mol Sci. (2021) 22(1):442. doi: 10.3390/ijms22010442
119. Hu YF, Yeh HI, Tsao HM, Tai CT, Lin YJ, Chang SL, et al. Electrophysiological correlation and prognostic impact of heat shock protein 27 in atrial fibrillation. Circ Arrhythm Electrophysiol. (2012) 5(2):334–40. doi: 10.1161/CIRCEP.111.965996
120. Marion D, Lanters EAH, Ramos KS, Li J, Wiersma M, Baks-Te Bulte L, et al. Evaluating Serum heat shock protein levels as novel biomarkers for atrial fibrillation. Cells. (2020) 9(9):2105. doi: 10.3390/cells9092105
121. Wu W, Lu Z, Li Y, Chen Z, Jiang H, Li Y. Decreased cardiac expression of heat shock protein 27 is associated with atrial fibrillation in patients with rheumatic heart disease. Acta Cardiol Sin. (2015) 31(1):1–7. doi: 10.6515/acs20140526a
122. Wang X, Shangguan W, Li G. Angiotensin-(1-7) prevents atrial tachycardia induced-heat shock protein 27 expression. J Electrocardiol. (2018) 51(1):117–20. doi: 10.1016/j.jelectrocard.2017.08.015
123. Starreveld R, Ramos KS, Muskens A, Brundel B, de Groot NMS. Daily supplementation of L-glutamine in atrial fibrillation patients: the effect on heat shock proteins and metabolites. Cells. (2020) 9(7):1729. doi: 10.3390/cells9071729
124. Li S, Liang M, Pan Y, Wang M, Gao D, Shang H, et al. Exercise modulates heat shock protein 27 activity in diabetic cardiomyopathy. Life Sci. (2020) 243:117251. doi: 10.1016/j.lfs.2019.117251
125. Herwig M, Kolijn D, Lodi M, Holper S, Kovacs A, Papp Z, et al. Modulation of titin-based stiffness in hypertrophic cardiomyopathy via protein kinase D. Front Physiol. (2020) 11:240. doi: 10.3389/fphys.2020.00240
126. Clemen CS, Herrmann H, Strelkov SV, Schroder R. Desminopathies: pathology and mechanisms. Acta Neuropathol. (2013) 125(1):47–75. doi: 10.1007/s00401-012-1057-6
Keywords: heat shock protein 27, phosphorylation, cardiovascular diseases, cardiovascular pathophysiology, therapy
Citation: Zou Y, Shi H, Liu N, Wang H, Song X and Liu B (2023) Mechanistic insights into heat shock protein 27, a potential therapeutic target for cardiovascular diseases. Front. Cardiovasc. Med. 10:1195464. doi: 10.3389/fcvm.2023.1195464
Received: 28 March 2023; Accepted: 25 April 2023;
Published: 12 May 2023.
Edited by:
Johnson Rajasingh, University of Tennessee Health Science Center (UTHSC), United StatesReviewed by:
Giuseppina Milano, Centre Hospitalier Universitaire Vaudois (CHUV), SwitzerlandGobinath Shanmugam, University of Alabama at Birmingham, United States
Ioanna-Katerina Aggeli, National and Kapodistrian University of Athens, Greece
© 2023 Zou, Shi, Liu, Wang, Song and Liu. This is an open-access article distributed under the terms of the Creative Commons Attribution License (CC BY). The use, distribution or reproduction in other forums is permitted, provided the original author(s) and the copyright owner(s) are credited and that the original publication in this journal is cited, in accordance with accepted academic practice. No use, distribution or reproduction is permitted which does not comply with these terms.
*Correspondence: Bin Liu bGl1YmluMzMzM0B2aXAuc2luYS5jb20=