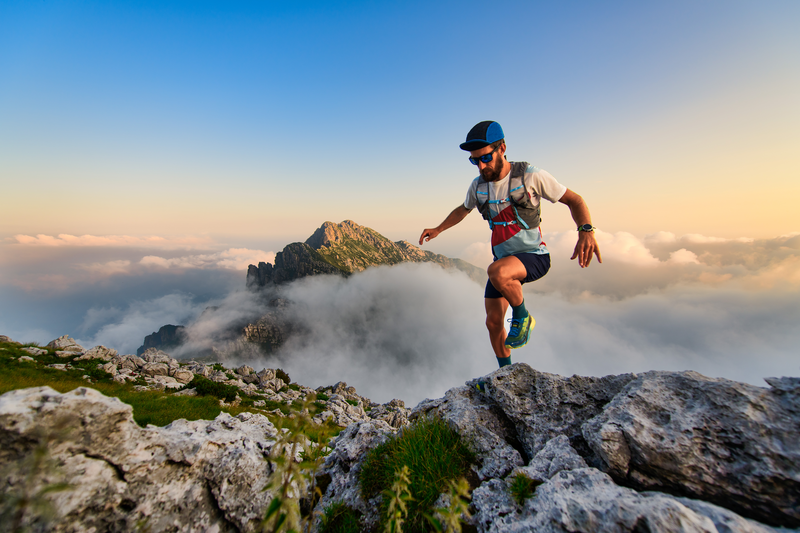
94% of researchers rate our articles as excellent or good
Learn more about the work of our research integrity team to safeguard the quality of each article we publish.
Find out more
REVIEW article
Front. Cardiovasc. Med. , 27 July 2023
Sec. Cardiovascular Metabolism
Volume 10 - 2023 | https://doi.org/10.3389/fcvm.2023.1187735
Obesity is a heterogeneous disease that affects almost one-third of the global population. A clear association has been established between obesity and cardiovascular disease (CVD). However, CVD risk is known to be related more to the local distribution of fat than to total body fat. Visceral adipose tissue (VAT) in particular has a high impact on CVD risk. This manuscript reviews the role of VAT in residual CV risk and the available therapeutic strategies for decreasing residual CV risk related to VAT accumulation. Among the many pathways involved in residual CV risk, obesity and particularly VAT accumulation play a major role by generating low-grade systemic inflammation, which in turn has a high prognostic impact on all-cause mortality and myocardial infarction. In recent years, many therapeutic approaches have been developed to reduce body weight. Orlistat was shown to reduce both weight and VAT but has low tolerability and many drug-drug interactions. Naltrexone-bupropion combination lowers body weight but has frequent side effects and is contraindicated in patients with uncontrolled hypertension. Liraglutide and semaglutide, glucagon-like peptide 1 (GLP-1) agonists, are the latest drugs approved for the treatment of obesity, and both have been shown to induce significant body weight loss. Liraglutide, semaglutide and other GLP-1 agonists also showed a positive effect on CV outcomes in diabetic patients. In addition, liraglutide showed to specifically reduce VAT and inflammatory biomarkers in obese patients without diabetes. GLP-1 agonists are promising compounds to limit inflammation in human visceral adipocytes.
Obesity is a heterogeneous disease that affects almost one-third of the world's population (1). Kelly et al. showed that if there is no change in this trend by 2030, 57.8% of the world's population will be overweight or obese (2). Consequently, obesity can be considered a pandemic (3). Obesity is a disease that affects all age groups. The prevalence of obesity is higher in women of any age and older people (4). Moreover, obesity in adolescence, in recent years, has become a global health emergency with increased cardiovascular disease (CVD) risk in adulthood (5).
Probably the most critical risk factors for developing obesity are the adoption of a sedentary lifestyle and a fat-rich diet (6). The increased availability of more nutrient-dense foods (with more critical marketing in recent years) could explain the weight gain among populations (7). Changes in food culture are considered the primary driver of the increased prevalence. Conversely, changes in lifestyle and less physical activity are cofactors of this expansion (7).
According to the WHO's BMI classification criteria, overweight is defined as a BMI > 25 kg/m2 and obesity as a BMI > 30 kg/m2 (8). The large inter-individual variability in the percent of fat according to age, sex, or ethnicity illustrated the lack of sensibility of this criterion (9, 10). Hence, in 2004, WHO experts proposed lower BMI cutoffs for the diagnosis of obesity for Asian people (11). Furthermore, BMI does not correlate precisely with the prevalence of metabolic abnormalities among ethnic groups. Indeed, Caucasian people have an equivalent prevalence of metabolic abnormalities to other ethnic groups with higher BMI (12).
Epidemiologic studies have depicted the relationship between obesity and 20 health outcomes (4). A strong association is clear with CVD, such as ischemic heart disease, ischemic and hemorrhagic stroke, and hypertensive heart disease, as well as with conditions that could be risk factors for impaired cardiac function, such as chronic kidney disease (CKD) or diabetes mellitus (DM) (4). About 30% of deaths worldwide are due to CVD; nowadays, CVD is the primary cause of mortality and early mobility globally (13).
The detrimental effects of obesity on cardiovascular health are well-known and mediated through intermediate conditions, like diabetes mellitus, dyslipidemia, and hypertension (13). Conversely an increased muscle mass is associated with better insulin sensitivity and glucose metabolism, lower rates of type 2 diabetes mellitus and cardiovascular events (14).
The inability to discriminate between fat mass and fat free mass by BMI may lead to erroneous assumptions about cardiovascular risk: the so-called “obesity paradox”. Lean subjects with preserved muscle mass are at lower cardiovascular risk than obese subjects (15).
For years, it has been known that CVD risk is related more to the local distribution of fat than to total body fat. There are anatomical, cellular, molecular, metabolic, clinical, and prognostic distinctions between visceral adipose tissue (VAT) and subcutaneous adipose tissue (SAT). The first has a high weight in CVD risk (16). Consequently, this manuscript aims to convey the available therapeutic strategies for clinicians to decrease the residual CVD risk related to VAT accumulation.
Obesity is a heterogeneous disease related to adipose tissue (AT) accumulation. In women, the accumulation of AT is maximal in the hips and thighs, whereas in men it tends to accumulate in the trunk and upper body (16, 17). It depends mainly on the influence of hormones, especially estrogen, and on the activity of lipoprotein lipase (LPL, which allows TG accumulation in AT), which is more intense for women in the gluteal region. The literature describes two types of adipose tissue: subcutaneous (SAT) and ectopic (EAT) adipose tissue. There are different EAT types, including VAT (18).
Regarding total AT, 80%–90% is subcutaneous (16, 19); that is, it tends to form beneath the skin. It is divided into superficial and deep layers. The latter grows when obesity develops and correlates more strongly with CVD and obesity-related insulin resistance (20). Pubertal and post-pubertal women especially accumulate SAT. However, in postmenopausal women, the percentage of VAT increases (21). SAT works as a metabolic sink with the accumulation of TG in adipocytes, and it grows with surplus energy intake (i.e., a high-calorie diet). The most frequent locations of SAT storage are the abdominal, femoral–gluteal, and back (16). Abdominal AT is divided into subcutaneous and intra-abdominal (16). When the ability of SAT to store AT is exceeded (or impaired), AT starts to accumulate in locations that generally are not usual for adipose tissue storage, forming the EAT (22).
Visceral adipose tissue is classically ectopic, because lipid accumulation occurs in normally lean tissues such as the liver, the heart (pericardial, epicardial, and intramyocardial), and skeletal muscle or in areas of the body usually not associated with adipose tissue storage and containing only small amounts of fat (renal sinus, pancreas, thoracic, periaortic, perivascular) (18, 22, 23). Even within the abdominal superficial adipose tissue, the deep adiposity exhibits a metabolic phenotype closer to that of VAT (24). Any ectopic fat may be a “dysfunctional” adipose tissue, even if the debate is unresolved about ectopic fat as a marker or mediator of cardiometabolic diseases (23, 24).
Ectopic fat deposits are VAT, intramuscular fat, fatty liver, perivascular and pericardial fat, myocardial steatosis, and renal situs fat. They may be subdivided into those causing systemic or local metabolic effects (18). These effects explain the relationship between EAT accumulation and CVD. Some of the most crucial systemic effects of EAT are the development of a low-grade systemic inflammatory status and/or insulin resistance (22). The contribution of VAT to their development is greater than fatty-liver or intra-muscular fat (indeed, they have smaller volume) (18). Nevertheless, a strong relationship between nonalcoholic steatohepatitis (NASH) and metabolic syndrome (MS) is known (25). Non-alcoholic fatty liver disease (NAFLD) is explicated as the liver's storage of lipids (especially TGs). The liver's fat content could be quantified with ultrasonography but is more accurate with magnetic resonance imaging (MRI) or computed tomography (CT) (26). NASH may reveal the risk of developing DM II and CVD. Indeed, this condition is characterized by the greater release of very low-density lipoproteins (VLDLs) and, hence, the heightened concentration of Apo-B lipoproteins (27), the development of impaired insulin sensitivity (28), and the release of inflammatory factors (29). Several studies have displayed that NASH could provoke coronary atherosclerosis and narrowing carotid arteries (29).
The relationship between adipose tissue storage and the heart could have two possible implications. One is the storage around the heart between the myocardium and pericardium (epicardial adipose tissue), and the other is the potential storage of AT into cardiomyocytes (myocardial steatosis) (30). Under physiological conditions, epicardial adipose tissue has an important function for the myocardium as thermogenic, mechanic, or metabolic support (30). Furthermore, perivascular fat has vasoactive properties (31). This valuable work of the perivascular/epicardial adipose tissue becomes dysfunctional when obesity develops (32). Indeed, excess EAT around the heart causes hypertrophy of the myocardial tissue, fibrosis, and the reduction of the synthesis of adiponectin through the heightened release of inflammatory factors (32, 33). The Framingham Heart Study clarifies the role of epicardial AT as an independent cardiovascular (CV) risk factor (34). Wang et al. also showed the relationship between this AT and components of MS as DM II, decreased HDL-C levels, hypertension, coronary disease, greater TG levels, and VAT (35). Moreover, several recent studies conveyed the positive relationship between the thickening of the pericardial AT and the prevalence of atrial fibrillation (33).
Conversely, myocardial steatosis (storage of lipids in cardiomyocytes) contributes, with local effects, to cardiac dysfunction. More lipids accumulate in cardiomyocytes in the hearts of patients with DM II or impaired glucose intolerance (36).
Another perivascular location of EAT is the accumulation of lipids around renal arteries (renal situs fat). It is associated with poor control of hypertension and chronic kidney disease (37, 38).
The reasons for the correlations between VAT and clinical diseases are unknown. The most recognizable adverse effects of the accumulation of VAT are the development of metabolic alterations, insulin resistance and systemic inflammatory status (39).
Anatomically, a more significant number of large and dysfunctional adipocytes form VAT. All types of visceral fat are metabolically active. They are insulin-resistant (few insulin receptors), hyper-lipolytic, and resistant to the anti-lipolytic effect of insulin, so they favor the accumulation of AT in ectopic tissues (40, 41). The high lipolytic activity of the abdominal VAT constantly releases free fatty acids (FFAs), drained through the portal vein, so FFAs accumulate in the liver.
This accumulation of FFAs and glycerol in the liver leads to alterations in the lipid and glucose metabolism. The liver reduces the binding of the insulin and increases the content of lipids and the secretion of lipoproteins rich in triglycerides (VLDL, Apo-B) (42, 43). Increased production of glucose and hyperinsulinemia, could explain the relationship between VAT and DM II (43).
Metabolically, VAT has a greater density of glucocorticoid (44) and androgen receptors (44). Subsequently, in men after 50 years, VAT tends to increase (4). By contrast, the binding capacity of estrogens is greater in SAT (45). In women, VAT tends to increase in adulthood (44). In addition, VAT is more sensitive to catecholamine-induced lipolysis (46). More lipolysis and higher levels of FFAs lead to hyperglycemia, hyperinsulinemia, and hypertriglyceridemia by propagation of insulin resistance to the liver.
VAT is regarded as endocrine system secreting several adipokines, e.g., leptin, adiponectin, omentin, visfatin, resistin, and apelin (47). Adipokines are mediators of body composition, due to their pleitropic effects on metabolism. In adiposity, especially in VAT, production of adipokines are altered.
Leptin is involved in the regulation of body weight and fat distribution (48). In overweight and obesity hyperleptinemia and resistance to leptin increase, resulting in reduced energy expenditure, hyperinsulinemia and hyperlipidemia (49). The greater the VAT, the higher the blood levels of leptin, a marker of MS that predicts CV risk) (45, 46, 50, 51).
Adiponectin is a hormone which plasma levels decrease with visceral fat accumulation, although it is secreted only by adipose tissue (52). By reducing the levels of circulating adiponectin, the stimulation of fatty acid oxidation and glucose uptake in skeletal muscle are reduced, worsening whole-body energy homeostasis (53). Also in the liver fatty acid oxidation is blunted, together with inhibition of glucose production (53). Therefore obesity-associated hypoadiponectinemia may contribute to hepatic steatosis. Adiponectin exemplifies the less production of protective adipokines (23).
Omentin enhances insulin action by stimulating insulin-mediated glucose uptake, without effect on basal glucose transport (54). Serum omentin level is significantly lower in overweight subjects (55). Other adipokines that lost their protective effects are vaspin (inhibition of reactive oxygen species) and apelin (enhancement of cholesterol efflux) (47). In contrast, adipokines that accentuate their detriment effects include resistin (interference with insulin action) and visfatin (insulin-mimetic actions) (56).
Overall, adipokines have different origins (adipocyte, stromal cells and type of VAT), mechanisms of action (direct, mediated, positive or negative) and effects on various metabolic circuits (56). Upstream, adipokine dysregulation due to adipose tissue dysfunction contributes to obesity-related metabolic diseases.
Expansion of visceral adipose tissue depots is accompanied by inflammation (57). One of the first step is infiltration of new macrophages, which increase up to 10 times (23, 58). Adypocytes secrete high levels of monocyte chemotactic protein-1 (MCP-1), a potent chemiotactic chemokine promoting monocytes/macrophages accumulation (59). Also the macrophages derived from monocytes produce additional MCP-1 because they switch to a pro-inflammatory phenotype (60). Also the increase of production of TNFα reflects the influx of inflammatory cells within expending adipose tissue (61). It has long been known that obesity is associated with elevated circulating levels of IL-6 released from visceral fat, providing a strong link with inflammation (62). Visceral adipose tissue released greater amounts of IL-6 compared with abdominal subcutaneous tissue (63).
Local inflammation is further promoted by immune cells resident in adipose tissue. Various types of T cells (CD4+, CD8+, natural killer) enrich VAT and produce interferon-γ (IFNγ). INFγ stimulates the differentiation of monocytes in activated macrophages (64).
Local VAT inflammation progresses to low-grade systemic inflammation, that is characterized as mildly elevated levels of circulating cytokines, chemokines, and acute phase reactants (61).
These signals from dysfunctional adipose tissue are primary stimuli for hepatic macrophages (Kupffer cells). Polarization to inflammatory phenotype of Kupffer cells propagates inflammation to liver (65). Furthermore, the liver starts to produce inflammatory mediators (41).
The release of interleukin-6 (IL-6) and tumor necrosis factor-alfa (TNF-alfa), with a proatherogenic and prodiabetic function, is higher in obese patients (16). Moreover, IL-6 is the primary mediator of CRP production in the liver, and blood levels of CRP will be increased in obese patients (16).
There is a close relationship between inflammatory and atherosclerotic disease (66). Visceral obesity is also related to hypercoagulability (67), peripheral arterial disease (68), atherosclerosis in carotid arteries (69, 70) and microalbuminuria (71).
The release of FFA from dysfunctional adipocytes into the circulation is also diverted to muscle and other tissues (72). Decreased muscle glucose uptake and hepatic insulin resistance are other consequences of excess of FFA from visceral fat and can contribute metabolic complications, such as dyslipidemia and risk for type 2 diabetes (T2DM) (73). Fat accumulates ectopically pancreatic β-cells and occurs tipically with elevated levels of lipotoxic intermediates such as diacylglycerol (73). Lipotoxicity contributes to insulin impairment.
It has been reported that FFA levels were lower in metabolically healthy obesity subjects than those with metabolically unhealthy obesity (74), high level of FFA are associated with a higher incidence of T2DM and almost all patients with type 2 diabetes have fatty liver (75).
The association of visceral adiposity with dyslipidemia, inflammation, insulin resistance, glycemic abnormalities, hepatic steatosis and many other metabolic and vascular disturbances configure a picture of metabolic syndrome, contributing to increased atherosclerosis and cardiometabolic risk.
Insulin resistance, principally through the activation of the renin-angiotensin-aldosterone system and sympathetic activation, leads to hypertension (46). Obesity and visceral adiposity in particular account for most of the risk of hypertension (46, 50).
To show the role of VAT as an independent risk factor for metabolic and CV diseases, Ruiz-Castell et al. (60) divided a cohort of patients (n = 1,529) into quartiles based on estimated VAT according to Samouda's anthropometric measure (76). This trial showed that the odds ratio (OR) increased in parallel with the rise in VAT size. This relationship was more robust for men with hypertension (OR 11.83 vs. 8.21 for the fourth quartile), though the correlation was stronger for women than men for prediabetes and diabetes (OR = 7.57 vs. 5.41, for the fourth quartile), for hypercholesterolemia (OR = 5.28 vs. 2.26, for the fourth quartile), and for hypertriglyceridemia (OR = 14.62 vs. 6.78, for the fourth quartile).
VAT forms around the abdominal visceral organs, and constitutes about 5%–15% of the total AT (77). Several methods are available for measuring VAT. The most commonly used are anthropometric measures or bioelectrical impedance analysis (BIA) (78). However, these are inaccurate. Only CT and MRI could give a direct measurement of VAT, and the point for optimal image acquisition to estimate the VAT is 5–6 cm above the L4–L5 disk (78). A good estimation of VAT could also be obtained thanks to the development of dual-energy x-ray absorptiometry (DEXA) (23). It calculates VAT as the difference between total AT and SAT. In addition, ultrasound could help measure VAT by measuring the distance between the aorta and the internal face of the abdomen wall. Nonetheless, it is a method that depends strongly on the operator's skill (78). In real-world experience, these methods are reserved only for research scopes, so several anthropometric measures are created to allow an indirect measure of VAT. Perhaps the most used is waist circumference (WC), but the waist-to-hip ratio (WHR) and sagittal abdominal diameter are also available in clinical practice (78). If waist circumference represents VAT and SAT, hip circumference represents SAT only. Accordingly, a high WHR value may be significant for a large VAT (78). Nevertheless, a study showed that the most reliable surrogate for the measurement of VAT is WC (78). Several thresholds are accessible for WC. In the U.S., 102 cm in men and 88 cm in women are necessary for a diagnosis of MS (79). However, there may be ethnicity-specific differences in the WC threshold (80). Another anthropometric measure of VAT is neck circumference, but there is no solid literature on the topic (23). Samouda et al. described a new anthropometric measure for estimating VAT based on thigh and waist circumference and adjusted for BMI and age (43). It is: (6 × waist circumference −4.41 × proximal thigh circumference +1.19 × age −213.65) for men and (2.15 × waist circumference −3.63 × proximal thigh circumference +1.46 × age +6.22 × BMI −92.713) for women (76).
Ectopic fat localized around abdominal visceral organs is a significant risk factor for metabolic and CV disease, independent of total AT (19, 23).
Residual CV risk comprises persistent CV risk; it is the possibility of incident vascular events or the progression of established vascular damage, despite optimized care (81). This could be associated with known CV risk factors not adequately treated with therapy or unknown CV risk factors (81, 82). This concept originated from the evidence of people experiencing new CV events or the recurrence of CV events despite evidence-based pharmacological therapy (61). Since the emergence of interventional trials, mainly statin trials, science has started to discuss residual CV risk (83).
Many pathways could be involved in residual CV risk (Figure 1). The most important are lipidic, thrombotic, inflammatory, and diabetic patterns (84). Obesity, particularly the accumulation of VAT through the generation of low-grade systemic inflammation, increases the residual CV risk (77). The relationship between the development of atherosclerosis and inflammation has been known since studies demonstrated the presence of inflammatory cells, such as macrophages, in the arterial plaque and since activated T-lymphocytes were found at the site of plaque rupture or erosion (85). Furthermore, the risk of plaque rupture could depend more on the number of macrophages than on plaque size (86).
The prognostic value of inflammation in patients undergoing percutaneous coronary intervention (PCI) was evaluated by Kalman et al. among 7,000 patients (66). Subsequently, 38% of these patients had persistently high residual inflammatory risk (RIR; defined as high blood levels of high sensitive C-reactive protein ≥2 mg/L before and after >4 weeks after the PCI). All-cause mortality and myocardial infarction (MI) incidence rates are higher in these patients at the 1-year follow-up (87). Therapy targeting interleukin-1β with canakinumab at a dose of 150 mg/die proved in the CANTOS trial to reduce the incidence rate of the primary endpoint (composite of nonfatal MI, nonfatal stroke, or CV death). The incidence rate for events was 3.86 per 100 person-years in the 150 mg group, despite 4.50 per 100 persons in the placebo group, with a hazard ratio of 0.85 in the 150 mg group (p = 0.021) (88). Nowadays, no drugs are available that act selectively on RIR.
Furthermore, there is a known relationship between adiposity and dyslipidemia. Regarding the lipidic pathway, the most crucial part of residual CV risk is associated with abnormal triglyceride levels (TG)-rich lipoproteins (TGRLs), their remnants, or alterations in the number or function of HDL (high density lipoprotein) (89). Dyslipidemia associated with non-LDL (low-density lipoprotein) is called atherogenic dyslipidemia or atherogenic dyslipoproteinemia (90).
TGRLs derive from the diet (chylomicrons and remnants) and the liver (very low-density lipoprotein and their remnants) (59). The hydrolyzation of TGRLs by LPL transforms these lipoproteins into relatively cholesterol-rich molecules, termed cholesterol remnants and measured with the formula: total cholesterol-HDL-C—LDL-C (89, 90).
Even in patients well-treated for hypertension, some data support the persistence of residual CV risk, even with an optimal control of blood pressure (91–93).
In recent years, many therapeutic approaches have been developed to reduce body weight.
Molecules of novel therapeutic classes have emerged, such as tirzepatide, the first dual glucose-dependent insulinotropic polypeptide/glucagon-like peptide-1 receptor agonist (GIP)/GLP-1 RA), which has recently demonstrated a body weight reduction exceeding 20% in people with obesity, coupled with improved cardiometabolic measures (94). Extensively studied for weight management is lorcaserin, a selective 5-HT2C receptor agonist (95). Also the insulin sensitizers thiazolidinediones such as pioglitazone have shown in combination with simvastatin to reduce adipose tissue inflammation in subjects with MS (96).
There are five Food and Drug Administration (FDA)-approved medications for managing body weight (97). A summary of the main drugs and their characteristics is provided in Table 1.
The first is orlistat, an oral drug shown to reduce body weight by 5% or more. It is a pancreatic and gastric lipase inhibitor (98). Several studies have shown the efficacy of orlistat. Khera et al. showed a reduction of 5% of body weight in 44% of patients treated with orlistat despite 23% of those in treatment with placebo and, after 1 year of follow-up, a more significant reduction of body weight (−2.6 kg) in patients receiving orlistat despite a placebo (102). Orlistat's tolerability is not optimal. Indeed, this drug may cause steatorrhea to impair the absorption of fat as well as some vitamins and nutrients (76). Special attention must be given to patients receiving levothyroxine, warfarin, antiepileptics, and antiretroviral medications (97). Orlistat may interfere with their function. Furthermore, it is contraindicated for pregnant and breastfeeding women, and there are no data about its use for children (97). In a 2011 multicenter study, orlistat 60 mg showed the ability to reduce VAT to a greater extent than placebo in patients with a BMI of 25–34.9 kg/m2 without DM (65). Indeed, after 24 weeks of treatment, people receiving orlistat with a low-calorie diet and physical exercise reached a median reduction of VAT of 15.7%, compared to 9.4% of people receiving a placebo. In addition, orlistat-treated patients reached a more significant total mass fat loss than placebo-treated patients (−4.65 vs. −3.01 kg, p < 0.05) (100).
Another drug in use against obesity is the combination of an opioid receptor antagonist (naltrexone) and a norepinephrine–dopamine reuptake inhibitor (bupropion) (103). In a meta-analysis 55% of patients receiving naltrexone–bupropion lowered their body weight by 5% compared to 23% in the placebo-treated population (104). After 1 year of follow-up in naltrexone–bupropion-treated patients, there was 5 kg excess weight loss compared to placebo (104). Problems with the tolerability of this drug are frequent. Mainly, it is contraindicated in patients with uncontrolled hypertension (105).
In a phase 2 trial naltrexone/bupropion obtained a reduction of VAT mass (−15.0%) greater than placebo (−4.6%) and proportional with weight loss in 80 obese subjects treated for 24 weeks (106).
In 2012, the FDA approved the commercialization of the association between phentermine–topiramate. The first is a sympathomimetic that causes an increase in blood and central nervous system levels of norepinephrine. The second is a gamma–aminobutyric (GABA) receptor agonist, also used for treating epilepsy or headaches (83). The fixed drug combination showed optimal results in reducing body weight, 4.7%–10.4% weight loss (99) and also improvements in waist circumference measures, suggesting possible diminution in visceral fat depots (107). However some questions about its long-term safety remain (97). The principal side effects concern the nervous system, such as depression, paresthesia, and insomnia (99, 107). Furthermore, it is contraindicated in pregnant women. Due to the lack of long-term safety (99), the European Medicines Agency (EMA) did not allow its commercialization.
Liraglutide and semaglutide are the last two drugs approved by the FDA and EMA to treat obesity (97, 108). They belong to the same antidiabetic drug family: the Glucagon-Like Peptide 1 (GLP-1) agonists (97). Between those, there are some distinctions in the efficacy of weight loss and in the frequency of subcutaneous administration.
The efficacy of weight loss with the daily administration of liraglutide 3.0 mg/daily was assessed in 2015 by the SCALE trial (109). A cohort of 3,731 obese patients (BMI > 30 or >27 kg/m2 with dyslipidemia or hypertension) were enrolled and divided into subjects receiving a daily dose of liraglutide 3 mg and subjects receiving a placebo as an adjunct to diet and exercise. After 56 weeks of follow-up, the liraglutide group achieved a mean loss of 8.4 ± 7.3 kg, and the placebo group lost a mean of 2.8 ± 6.5 kg (p < 0.001). Additionally, 63.2% of patients in the liraglutide group lost at least −5% of their body weight compared with 27.1% in the placebo group. A difference of 22.5% between the two groups was also seen for the rate of patients achieving −10% of body weight (109).
In 2022, Rubino et al. showed a more significant loss in body weight with semaglutide than with liraglutide in obese patients without diabetes (−15.8% vs. −6.4%, p < 0.01) with a higher rate of obtaining 10% or more weight loss (70.9% vs. 25.6%, p < 0.01). The rate of subjects discontinuing treatment was higher for liraglutide than semaglutide (27.6% vs. 13.5%) (110). Nevertheless, compared to placebo, semaglutide demonstrated a higher proportion of subjects discontinuing treatment due to side effects (especially gastrointestinal) (101, 111). Semaglutide is administered once a week; liraglutide is administered daily (97).
In 2016, liraglutide showed a positive role in CV outcomes in diabetic patients. In the LEADER trial, 9,340 diabetic patients with high CV risk were randomized to receive a placebo or liraglutide (112). The primary composite outcome was the first occurrence of death from CV causes, nonfatal myocardial infarction, or nonfatal stroke. After 3.8 years of follow-up, the primary outcome rate was 13% in the liraglutide group compared to 14.9% in the placebo group (p < 0.01 for noninferiority; p = 0.01 for superiority) (113).
In addition to liraglutide, semaglutide (114), dulaglutide (115) and efpeglenatide (116) in patients affected by DM II also showed the ability to reduce the rate of major adverse cardiovascular events (MACEs) compared to placebo.
In a post-hoc analysis of five trials, Davies et al. evaluated whether the CV risk increased during treatment with liraglutide despite comparators (placebo or orlistat) in a population both with and without DM (117). The primary composite outcome was the first occurrence of CV death, nonfatal myocardial infarction, or nonfatal stroke. Two of the five trials were adjudicated retrospectively. In liraglutide 3.0 mg, eight participants experienced adverse CV events, despite 10 participants in the comparators group. The hazard ratio (HR) for liraglutide 3.0 mg vs. its comparators was 0.42 (p = 0.07). For all liraglutide doses vs. all comparators, the HR increased to 0.50 (p = 0.11) (94).
Only with the ongoing Semaglutide Effects on Heart Disease and Stroke in Patients With Overweight or Obesity (SELECT) study (NCT03574597) will clinicians understand whether GLP-1 agonists (semaglutide) can reduce MACEs in obese patients with an established CV event (prior myocardial infarction, prior stroke, or peripheral arterial disease) without DM (118).
The once-daily administration of liraglutide 3.0 mg with physical exercise and a 500-kcal deficient diet, compared to a placebo over a median of 36 weeks on treatment, reduced the VAT measured with MRI (96). In a cohort of 128 obese patients (BMI > 30 or >27 kg/m2 with MS) without DM, in the liraglutide group there was a mean reduction in VAT of −12.49% compared with −1.63% in the placebo group (p < 0.0001). The liraglutide 3.0 mg group also obtained better results concerning secondary outcomes. Indeed, the estimated treatment disparities between the two groups were −33% for liver fat lost, −9.10% for abdominal SAT lost, −8.66% for lower body fatty tissue lost, and −8.64% for total body adipose tissue lost (119). Furthermore, in the liraglutide group, there was a reduction in CPR blood levels (−19.91%, p = 0.038) and in fasting blood glucose (−5.62%, p = 0.0048). In patients receiving liraglutide, the most frequent adverse events were gastrointestinal-related and upper respiratory tract infections but were low-grade episodes (grades 1–2). During the treatment, no patient had episodes of hypoglycemia (119).
Similar results came from Yu et al. (120). They enrolled a population of patients with a BMI > 28 kg/m2 with a prior diagnosis of DM II who had not undergone lifestyle interventions. They were divided into a study group (receiving liraglutide) and a control group (undergoing lifestyle interventions). After 12 weeks of follow-up, the liraglutide group obtained a more significant reduction of VTA calculated by the energy spectrum CT (−7.1 ± 10.17 cm2 in the study group vs. 0.91 ± 16.59 cm2 in the control group) (120).
The effects of liraglutide on visceral adipose tissue reduction are summarized in Figure 2.
Figure 2. Effects of liraglutide on visceral adipose tissue and weight reduction (data from three reviews) (121–123).
The use of dietary supplements promoted for weight management is very common among consumers, attracted by promises on thermogenic properties (e.g., caffeine, green tea extracts), effects on satiety (e.g., glucomannan), increased fat oxidation and energy expenditure (e.g., pepper) (124).
Dietary weight-loss supplements might interact with prescription drugs, over-the-counter medications and herbal compounds. The potential for possible interactions should always be considered (125).
These interactions can be of pharmacokinetic or pharmacodynamic nature, or both. At pharmacokinetic level, interferences with hepatic cytochrome P450 (CYP450) enzymes are typical of St. John's wort or bitter orange, used for promoting a sense of satiety or increasing energy expenditure, respectively (125). Futhermore many supplements may interfere with absorption of drugs, such as laxatives (senna) (124). At pharmacodynamic level, alteration of dose–response relationship of insulin has been well described for supplements containing caffeine (124).
New natural constituents with anti-obesity effects are continuously being proposed, e.g., Vaccinium corymbosum, Vaccinium myrtillus, Tripterygium wilfordi (celastrol), astaxanthin, artemisinin, Ananas comosus, papain, saffron, tocopherol, etc. (126).
Few interactions are described between herbal compounds and approved anti-obesity drugs, such as orlistat, phentermine/topiramate, naltrexone/bupropion, liraglutide, semaglutide, and setmelanotide (127). Pharmacological interactions are more studied for older compounds, but this doesn't mean that interactions with herbs won't be recognized in the future for new treatments.
Orlistat reduces the absorption of beta-carotene and vitamin E when using for long-term. Other fat soluble vitamins (A, D, and K) may show small reductions in circulating concentrations, so that multivitamin supplements are recommended (128). Interestingly, psyllium natural fibers are helpful in controlling the gastrointestinal side effects of orlistat, reduced significantly in intensity after 60 days of supplementation (129).
There is a warning about the use with caution of St. John's wort with lorcaserin (10 mg) (130).
No data supporting herb-drug interactions are available specifically related to use of topiramate at low doses as an anorectic agent (125). Caffeine should be avoided or used cautiously with phentermine and some, but not all, green tea products contain caffeine (131).
Naltrexone has been shown to interact with several cytochrome P450 enzymes, particularly 2C9 and 2D6, in preclinical studies. However, the clinical significance of these interactions is not known (132).
Although Gimko biloba extract treatment appears to reduce significantly the half-life and increase the maximal plasmatic concentration of hydroxybupropion, no bupropion dose adjustments appear warranted when the drug is administered orally with G. biloba extract (133).
Interactions of liraglutide or semaglutide with herbal products have not been established (113, 134). Liraglutide has shown very low potential to be involved in pharmacokinetic drug-drug interactions related to cytochrome P450 and plasma protein binding (135).
Setmelanotide did not appear to be metabolized by human hepatic microsomes (X35).
The main visceral adipose tissue is fatty tissue around the abdominal visceral organs. Waist circumference (WC) is the best anthropometric measure of VAT; CT and MRI are the most precise diagnostic techniques but are not always available. Several studies have shown a positive relationship between the size of VAT and CV disease. Indeed, VAT favors the development of atherogenic dyslipidemia, insulin resistance, and a low-grade systemic inflammatory state. Among anti-obesity drugs, a GLP-1 agonist called liraglutide (a subcutaneous antidiabetic drug), in addition to diet and physical exercise, demonstrated the ability to obtain a significant decrease in the size of VAT in a population of obese patients without diabetes mellitus. This positive result was also enhanced by reducing the blood level of CRP in patients receiving liraglutide. Similar results came from a recent trial of obese patients with DM.
Conceptualization: AC, GM, VA, OS, and PC; methodology: GSi, GSc, FR, DP; investigation: FS; data curation: GM, DP, CM; writing—original draft preparation: AC, GM, VA, FG, EM; writing—review and editing: OS; PC; visualization: CM, FS; supervision: OS, PC. All authors contributed to the article and approved the submitted version.
Editorial assistance was provided by Airon Communication srl through an unconditional support from Novo Nordisk S.p.A. The authors bear full responsibility for the contents and conclusions of the publication. Novo Nordisk S.p.A. did not influence nor was it involved in the analysis and interpretation of the data presented in the publication.
The remaining authors declare that the research was conducted in the absence of any commercial or financial relationships that could be construed as a potential conflict of interest.
The reviewer AM declared a past co-authorship with the authors AC and PC to the handling editor.
All claims expressed in this article are solely those of the authors and do not necessarily represent those of their affiliated organizations, or those of the publisher, the editors and the reviewers. Any product that may be evaluated in this article, or claim that may be made by its manufacturer, is not guaranteed or endorsed by the publisher.
1. Chooi YC, Ding C, Magkos F. The epidemiology of obesity. Metab Clin Exp. (2019) 92:6–10. doi: 10.1016/J.METABOL.2018.09.005
2. Kelly T, Yang W, Chen CS, Reynolds K, He J. Global burden of obesity in 2005 and projections to 2030. Int J Obes. (2008) 32:1431–7. doi: 10.1038/IJO.2008.102
3. Mi YJ, Zhang B, Wang HJ, Yan J, Han W, Zhao J, et al. Prevalence and secular trends in obesity among Chinese adults, 1991-2011. Am J Prev Med. (2015) 49:661–9. doi: 10.1016/J.AMEPRE.2015.05.005
4. GBD 2015 Obesity Collaborators, Afshin A, Forouzanfar MH, Reitsma MB, Sur P, Estep K, Lee A, et al. Health effects of overweight and obesity in 195 countries over 25 years. N Engl J Med. (2017) 377:13–27. doi: 10.1056/NEJMOA1614362
5. Cardel MI, Atkinson MA, Taveras EM, Holm JC, Kelly AS. Obesity treatment among adolescents: a review of current evidence and future directions. JAMA Pediatr. (2020) 174:609–17. doi: 10.1001/JAMAPEDIATRICS.2020.0085
6. He Y, Pan A, Wang Y, Yang Y, Xu J, Zhang Y, et al. Prevalence of overweight and obesity in 15.8 million men aged 15-49 years in rural China from 2010 to 2014. Sci Rep. (2017) 7:5012. doi: 10.1038/S41598-017-04135-4
7. Swinburn BA, Sacks G, Hall KD, McPherson K, Finegood DT, Moodie ML, et al. The global obesity pandemic: shaped by global drivers and local environments. Lancet. (2011) 378:804–14. doi: 10.1016/S0140-6736(11)60813-1
8. Pi-Sunyer FX. Obesity: criteria and classification. Proc Nutr Soc. (2000) 59:505–9. doi: 10.1017/S0029665100000732
9. Gallagher D, Visser M, Sepúlveda D, Pierson RN, Harris T, Heymsfieid SB. How useful is body mass index for comparison of body fatness across age, sex, and ethnic groups? Am J Epidemiol. (1996) 143:228–39. doi: 10.1093/OXFORDJOURNALS.AJE.A008733
10. Gallagher D, Heymsfield SB, Heo M, Jebb SA, Murgatroyd PR, Sakamoto Y. Healthy percentage body fat ranges: an approach for developing guidelines based on body mass index. Am J Clin Nutr. (2000) 72:694–701. doi: 10.1093/AJCN/72.3.694
11. WHO Expert Consultation. Appropriate body-mass index for Asian populations and its implications for policy and intervention strategies. Lancet. (2004) 363(9403):157–63. doi: 10.1016/S0140-6736(03)15268-3; Erratum in: Lancet. (2004) 363(9412):902.14726171
12. Wen CP, David Cheng TY, Tsai SP, Chan HT, Hsu HL, Hsu CC, et al. Are Asians at greater mortality risks for being overweight than caucasians? Redefining obesity for asians. Public Health Nutr. (2009) 12(4):497–506. doi: 10.1017/S1368980008002802
13. Naghavi M, Abajobir AA, Abbafati C, Abbas KM, Abd-Allah F, Abera SF, et al. Global, regional, and national age-sex specifc mortality for 264 causes of death, 1980-2016: a systematic analysis for the global burden of disease study 2016. Lancet. (2017) 390:1151–1210. doi: 10.1016/S0140-6736(17)32152-9
14. Medina-Inojosa JR, Somers VK, Thomas RJ, Jean N, Jenkins SM, Gomez-Ibarra MA, et al. Association between adiposity and lean mass with long-term cardiovascular events in patients with coronary artery disease: no paradox. J Am Heart Assoc. (2018) 7(10):e007505. doi: 10.1161/JAHA.117.007505
15. Prado CM, Gonzalez MC, Heymsfield SB. Body composition phenotypes and obesity paradox. Curr Opin Clin Nutr Metab Care. (2015) 18(6):535–51. doi: 10.1097/MCO.0000000000000216
16. Ibrahim MM. Subcutaneous and visceral adipose tissue: structural and functional differences. Obes Rev. (2010) 11:11–8. doi: 10.1111/J.1467-789X.2009.00623.X
17. Després JP. Body fat distribution and risk of cardiovascular disease: an update. Circulation. (2012) 126:1301–13. doi: 10.1161/CIRCULATIONAHA.111.067264
18. Britton KA, Fox CS. Ectopic fat depots and cardiovascular disease. Circulation. (2011) 124(24):e837–41. doi: 10.1161/CIRCULATIONAHA.111.077602
19. Gastaldelli A, Basta G. Ectopic fat and cardiovascular disease: what is the link? Nutr Metab Cardiovasc Dis. (2010) 20:481–90. doi: 10.1016/J.NUMECD.2010.05.005
20. Marinou K, Hodson L, Vasan SK, Fielding BA, Banerjee R, Brismar K, et al. Structural and functional properties of deep abdominal subcutaneous adipose tissue explain its association with insulin resistance and cardiovascular risk in men. Diabetes Care. (2014) 37:821–9. doi: 10.2337/DC13-1353
21. Mittal B. Subcutaneous adipose tissue & visceral adipose tissue. Indian J Med Res. (2019) 149:571. doi: 10.4103/IJMR.IJMR_1910_18
22. Longo M, Zatterale F, Naderi J, Parrillo L, Formisano P, Raciti GA, et al. Adipose tissue dysfunction as determinant of obesity-associated metabolic complications. Int J Mol Sci. (2019) 20(9):2358. doi: 10.3390/ijms20092358
23. Neeland IJ, Ross R, Després JP, Matsuzawa Y, Yamashita S, Shai I, et al. Visceral and ectopic fat, atherosclerosis, and cardiometabolic disease: a position statement. Lancet Diabetes Endocrinol. (2019) 7:715–25. doi: 10.1016/S2213-8587(19)30084-1
24. Oikonomou EK, Antoniades C. The role of adipose tissue in cardiovascular health and disease. Nat Rev Cardiol. (2019) 16(2):83–99. doi: 10.1038/s41569-018-0097-6
25. Capeau J. Insulin resistance and steatosis in humans. Diabetes Metab. (2008) 34:649–57. doi: 10.1016/S1262-3636(08)74600-7
26. Zhang YN, Fowler KJ, Hamilton G, Cui JY, Sy EZ, Balanay M, et al. Liver fat imaging-a clinical overview of ultrasound, CT, and MR imaging. Br J Radiol. (2018) 91(1089):20170959. doi: 10.1259/bjr.20170959
27. Deprince A, Haas JT, Staels B. Dysregulated lipid metabolism links NAFLD to cardiovascular disease. Mol Metab. (2020) 42:101092. doi: 10.1016/j.molmet.2020.101092
28. Sung KC, Wild SH, Kwag HJ, Byrne CD. Fatty liver, insulin resistance, and features of metabolic syndrome: relationships with coronary artery calcium in 10,153 people. Diabetes Care. (2012) 35:2359–64. doi: 10.2337/DC12-0515
29. Gaggini M, Morelli M, Buzzigoli E, DeFronzo RA, Bugianesi E, Gastaldelli A. Non-alcoholic fatty liver disease (NAFLD) and its connection with insulin resistance, dyslipidemia, atherosclerosis and coronary heart disease. Nutrients. (2013) 5:1544. doi: 10.3390/NU5051544
30. Iozzo P. Myocardial, perivascular, and epicardial fat. Diabetes Care. (2011) 34(Suppl 2):S371–9. doi: 10.2337/dc11-s250
31. Löhn M, Dubrovska G, Lauterbach B, Luft FC, Gollasch M, Sharma AM. Periadventitial fat releases a vascular relaxing factor. FASEB J. (2002) 16:1057–63. doi: 10.1096/FJ.02-0024COM
32. Packer M. Epicardial adipose tissue may mediate deleterious effects of obesity and inflammation on the myocardium. J Am Coll Cardiol. (2018) 71(20):2360–72. doi: 10.1016/j.jacc.2018.03.509
33. Guglielmi V, Sbraccia P. Epicardial adipose tissue: at the heart of the obesity complications. Acta Diabetol. (2017) 54(9):805–12. doi: 10.1007/s00592-017-1020-z
34. Mahabadi AA, Massaro JM, Rosito GA, Levy D, Murabito JM, Wolf PA, et al. Association of pericardial fat, intrathoracic fat, and visceral abdominal fat with cardiovascular disease burden: the framingham heart study. Eur Heart J. (2009) 30:850–6. doi: 10.1093/EURHEARTJ/EHN573
35. Wang CP, Hsu HL, Hung WC, Yu TH, Chen YH, Chiu CA, et al. Increased epicardial adipose tissue (EAT) volume in type 2 diabetes mellitus and association with metabolic syndrome and severity of coronary atherosclerosis. Clin Endocrinol. (2009) 70(6):876–82. doi: 10.1111/j.1365-2265.2008.03411.x
36. McGavock JM, Lingvay I, Zib I, Tillery T, Salas N, Unger R, et al. Cardiac steatosis in diabetes mellitus: a 1H-magnetic resonance spectroscopy study. Circulation. (2007) 116:1170–5. doi: 10.1161/CIRCULATIONAHA.106.645614
37. Foster MC, Hwang SJ, Porter SA, Massaro JM, Hoffmann U, Fox CS. Fatty kidney, hypertension, and chronic kidney disease: the framingham heart study. Hypertension. (2011) 58:784–90. doi: 10.1161/HYPERTENSIONAHA.111.175315
38. Chughtai HL, Morgan TM, Rocco M, Stacey B, Brinkley TE, Ding J, et al. Renal sinus fat and poor blood pressure control in middle-aged and elderly individuals at risk for cardiovascular events. Hypertension. (2010) 56:901. doi: 10.1161/HYPERTENSIONAHA.110.157370
39. Mathieu P, Poirier P, Pibarot P, Lemieux I, Després JP. Visceral obesity: the link among inflammation, hypertension, and cardiovascular disease. Hypertension. (2009) 53:577–84. doi: 10.1161/HYPERTENSIONAHA.108.110320
40. Misra A, Vikram NK. Clinical and pathophysiological consequences of abdominal adiposity and abdominal adipose tissue depots. Nutrition. (2003) 19:457–66. doi: 10.1016/S0899-9007(02)01003-1
41. Mårin P, Andersson B, Ottosson M, Olbe L, Chowdhury B, Kvist H, et al. The morphology and metabolism of intraabdominal adipose tissue in men. Metab Clin Exp. (1992) 41:1242–8. doi: 10.1016/0026-0495(92)90016-4
42. Neeland IJ, Hughes C, Ayers CR, Malloy CR, Jin ES. Effects of visceral adiposity on glycerol pathways in gluconeogenesis. Metab Clin Exp. (2017) 67:80–9. doi: 10.1016/J.METABOL.2016.11.008
43. Rebuffé-Scrive M, Lundholm K, Björntorp P. Glucocorticoid hormone binding to human adipose tissue. Eur J Clin Invest. (1985) 15(5):267–71. doi: 10.1111/j.1365-2362.1985.tb00182.x
44. Freedland ES. Role of a critical visceral adipose tissue threshold (CVATT) in metabolic syndrome: implications for controlling dietary carbohydrates: a review. Nutr Metab (Lond). (2004) 1(1):12. doi: 10.1186/1743-7075-1-12
45. Hellmér J, Marcus C, Sonnenfeld T, Arner P. Mechanisms for differences in lipolysis between human subcutaneous and omental fat cells. J Clin Endocrinol Metab. (1992) 75:15–20. doi: 10.1210/JCEM.75.1.1320047
46. Sharma AM, Engeli S, Pischon T. New developments in mechanisms of obesity-induced hypertension: role of adipose tissue. Curr Hypertens Rep. (2001) 3:152–6. doi: 10.1007/S11906-001-0030-X
47. Qi XY, Qu SL, Xiong WH, Rom O, Chang L, Jiang ZS. Perivascular adipose tissue (PVAT) in atherosclerosis: a double-edged sword. Cardiovasc Diabetol. (2018) 17:134. doi: 10.1186/s12933-018-0777-x
49. Myers MG, Cowley MA, Münzberg H. Mechanisms of leptin action and leptin resistance. Annu Rev Physiol. (2008) 70:537–56. doi: 10.1146/annurev.physiol.70.113006.100707
50. Hall JE, do Carmo JM, da Silva AA, Wang Z, Hall ME. Obesity-induced hypertension: interaction of neurohumoral and renal mechanisms. Circ Res. (2015) 116(6):991–1006. doi: 10.1161/CIRCRESAHA.116.305697
51. Park HK, Ahima RS. Physiology of leptin: energy homeostasis, neuroendocrine function and metabolism. Metab Clin Exp. (2015) 64(1):24–34. doi: 10.1016/j.metabol.2014.08.004
52. Fang H, Judd RL. Adiponectin regulation and function. Compr Physiol. (2018) 8(3):1031–63. doi: 10.1002/cphy.c170046
53. Matsuzawa J. Obesity and metabolic syndrome: the contribution of visceral fat and adiponectin. Diabet Manag. (2014) 4:391–401. doi: 10.2217/DMT.14.30
54. Yang RZ, Lee MJ, Hu H, Pray J, Wu HB, Hansen BC, et al. Identification of omentin as a novel depot-specific adipokine in human adipose tissue: possible role in modulating insulin action. Am J Physiol Endocrinol Metab. (2006) 290(6):E1253–61. doi: 10.1152/ajpendo.00572.2004
55. Arab A, Moosavian SP, Hadi A, Karimi E, Nasirian M. The association between serum omentin level and bodyweight: a systematic review and meta-analysis of observational studies. Clin Nutr ESPEN. (2020) 39:22–9. doi: 10.1016/j.clnesp.2020.06.014
56. Würfel M, Blüher M, Stumvoll M, Ebert T, Kovacs P, Tönjes A, et al. Adipokines as clinically relevant therapeutic targets in obesity. Biomedicines. (2023) 11(5):1427. doi: 10.3390/biomedicines11051427
57. Kolb H. Obese visceral fat tissue inflammation: from protective to detrimental? BMC Med. (2022) 20:494. doi: 10.1186/s12916-022-02672-y
58. Weisberg SP, McCann D, Desai M, Rosenbaum M, Leibel RL, Ferrante AW Jr. Obesity is associated with macrophage accumulation in adipose tissue. J Clin Invest. (2003) 112:1796–808. doi: 10.1172/JCI19246
59. Kaplan JL, Marshall MA, McSkimming CC, Harmon DB, Garmey JC, Oldham SN, et al. Adipocyte progenitor cells initiate monocyte chemoattractant protein-1-mediated macrophage accumulation in visceral adipose tissue. Mol Metab. (2015) 4(11):779–94. doi: 10.1016/j.molmet.2015.07.010
60. Kratz M, Coats BR, Hisert KB, Hagman D, Mutskov V, Peris E, et al. Metabolic dysfunction drives a mechanistically distinct proinflammatory phenotype in adipose tissue macrophages. Cell Metab. (2014) 20(4):614–25. doi: 10.1016/j.cmet.2014.08.010
61. Macdougall CE, Wood EG, Loschko J, Scagliotti V, Cassidy FC, Robinson ME, et al. Visceral adipose tissue immune homeostasis is regulated by the crosstalk between adipocytes and dendritic cell subsets. Cell Metab. (2018) 27(3):588–601.e4. doi: 10.1016/j.cmet.2018.02.007
62. Fontana L, Eagon JC, Trujillo ME, Scherer PE, Klein S. Visceral fat adipokine secretion is associated with systemic inflammation in obese humans. Diabetes. (2007) 56(4):1010–3. doi: 10.2337/db06-1656
63. Fain JN, Madan AK, Hiler ML, Cheema P, Bahouth SW. Comparison of the release of adipokines by adipose tissue, adipose tissue matrix, and adipocytes from visceral and subcutaneous abdominal adipose tissues of obese humans. Endocrinology. (2004) 145:2273–82. doi: 10.1210/en.2003-1336
64. Mathis D. Immunological goings-on in visceral adipose tissue. Cell Metab. (2013) 17:851–9. doi: 10.1016/j.cmet.2013.05.008
65. Jager J, Aparicio-Vergara M, Aouadi M. Liver innate immune cells and insulin resistance: the multiple facets of Kupffer cells. J Intern Med. (2016) 280:209–20. doi: 10.1111/joim.12483
66. Auer J, Berent R, Lassnig E, Eber B. C-reactive protein and coronary artery disease. Jpn Heart J. (2002) 43:607–19. doi: 10.1536/JHJ.43.607
67. Chitongo PB, Roberts LN, Yang L, Patel RK, Lyall R, Luxton R, et al. Visceral adiposity is an independent determinant of hypercoagulability as measured by thrombin generation in morbid obesity. TH Open. (2017) 1(2):e146–54. doi: 10.1055/s-0037-1608942
68. Planas A, Clará A, Pou JM, Vidal-Barraquer F, Gasol A, de Moner A, et al. Relationship of obesity distribution and peripheral arterial occlusive disease in elderly men. Int J Obes Relat Metab Disord. (2001) 25:1068–70. doi: 10.1038/SJ.IJO.0801638
69. Harris MM, Stevens J, Thomas N, Schreiner P, Folsom AR. Associations of fat distribution and obesity with hypertension in a bi-ethnic population: the ARIC study. Atherosclerosis risk in communities study. Obes Res. (2000) 8:516–24. doi: 10.1038/OBY.2000.64
70. Lear SA, Humphries KH, Kohli S, Frohlich JJ, Birmingham CL, Mancini GB. Visceral adipose tissue, a potential risk factor for carotid atherosclerosis: results of the multicultural community health assessment trial (M-CHAT). Stroke. (2007) 38(9):2422–9. doi: 10.1161/STROKEAHA.107.484113
71. Mulyadi L, Stevens C, Munro S, Lingard J, Bermingham M. Body fat distribution and total body fat as risk factors for microalbuminuria in the obese. Ann Nutr Metab. (2001) 45:67–71. doi: 10.1159/000046708
72. Perry RJ, Samuel VT, Petersen KF, Shulman GI. The role of hepatic lipids in hepatic insulin resistance and type 2 diabetes. Nature. (2014) 510:84–91. doi: 10.1038/nature13478
73. Henderson GC. Plasma free fatty acid concentration as a modifiable risk factor for metabolic disease. Nutrients. (2021) 13(8):2590. doi: 10.3390/nu13082590
74. Chait A, den Hartigh LJ. Adipose tissue distribution, inflammation and its metabolic consequences, including diabetes and cardiovascular disease. Front Cardiovasc Med. (2020) 7:22. doi: 10.3389/fcvm.2020.00022
75. Shitole SG, Biggs ML, Ix JH, Fretts AM, Tracy RP, Siscovick DS, et al. Fasting and postload nonesterified fatty acids and glucose dysregulation in older adults. Am J Epidemiol. (2022) 191(7):1235–47. doi: 10.1093/aje/kwac044
76. Samouda H, Dutour A, Chaumoitre K, Panuel M, Dutour O, Dadoun F. VAT=TAAT-SAAT: innovative anthropometric model to predict visceral adipose tissue without resort to CT-scan or DXA. Obesity. (2013) 21:E41–50. doi: 10.1002/OBY.20033
77. le Jemtel TH, Samson R, Milligan G, Jaiswal A, Oparil S. Visceral adipose tissue accumulation and residual cardiovascular risk. Curr Hypertens Rep. (2018) 20:1–14. doi: 10.1007/S11906-018-0880-0/FIGURES/2
78. Shuster A, Patlas M, Pinthus JH, Mourtzakis M. The clinical importance of visceral adiposity: a critical review of methods for visceral adipose tissue analysis. Br J Radiol. (2012) 85:1–10. doi: 10.1259/BJR/38447238
79. Grundy SM, Cleeman JI, Daniels SR, Donato KA, Eckel RH, Franklin BA, et al. Diagnosis and management of the metabolic syndrome: an American heart association/national heart, lung, and blood institute scientific statement. Circulation. (2005) 112:2735–52. doi: 10.1161/CIRCULATIONAHA.105.169404
80. Alberti KGMM, Zimmet P, Shaw J. The metabolic syndrome–a new worldwide definition. Lancet. (2005) 366:1059–62. doi: 10.1016/S0140-6736(05)67402-8
81. Hermans MP, Fruchart JC. Reducing vascular events risk in patients with dyslipidaemia: an update for clinicians. Ther Adv Chronic Dis. (2011) 2:307. doi: 10.1177/2040622311413952
82. Powell-Wiley TM, Poirier P, Burke LE, Després JP, Gordon-Larsen P, Lavie CJ, et al. Obesity and cardiovascular disease: a scientific statement from the American heart association. Circulation. (2021) 143(21):e984–e1010. doi: 10.1161/CIR.0000000000000973
83. Vanuzzo D. The epidemiological concept of residual risk. Intern Emerg Med. (2011) 6(Suppl 1)):45–51. doi: 10.1007/S11739-011-0669-5
84. Dhindsa DS, Sandesara PB, Shapiro MD, Wong ND. The evolving understanding and approach to residual cardiovascular risk management. Front Cardiovasc Med. (2020) 7:88. doi: 10.3389/fcvm.2020.00088
85. Robertson AK, Hansson GK. T cells in atherogenesis: for better or for worse? Arterioscler Thromb Vasc Biol. (2006) 26(11):2421–32. doi: 10.1161/01.ATV.0000245830.29764.84
86. Moreno PR, Falk E, Palacios IF, Newell JB, Fuster V, Fallon JT. Macrophage infiltration in acute coronary syndromes. Implications for plaque rupture. Circulation. (1994) 90:775–8. doi: 10.1161/01.CIR.90.2.775
87. Kalkman DN, Aquino M, Claessen BE, Baber U, Guedeney P, Sorrentino S, et al. Residual inflammatory risk and the impact on clinical outcomes in patients after percutaneous coronary interventions. Eur Heart J. (2018) 39:4101–8. doi: 10.1093/EURHEARTJ/EHY633
88. Ridker PM, Everett BM, Thuren T, MacFadyen JG, Chang WH, Ballantyne C, et al. Antiinflammatory therapy with canakinumab for atherosclerotic disease. N Engl J Med. (2017) 377:1119–31. doi: 10.1056/NEJMoa1707914
89. Toth PP. Triglyceride-rich lipoproteins as a causal factor for cardiovascular disease. Vasc Health Risk Manag. (2016) 12:171. doi: 10.2147/VHRM.S104369
90. Grundy SM. Hypertriglyceridemia, atherogenic dyslipidemia, and the metabolic syndrome. Am J Cardiol. (1998) 81(4A):18B–25B. doi: 10.1016/S0002-9149(98)00033-2
91. Glynn RJ, L'Italien GJ, Sesso HD, Jackson EA, Buring JE. Development of predictive models for long-term cardiovascular risk associated with systolic and diastolic blood pressure. Hypertension. (2002) 39:105–10. doi: 10.1161/HY1201.097199
92. Andersson OK, Almgren T, Persson B, Samuelsson O, Hedner T, Wilhelmsen L. Survival in treated hypertension: follow up study after two decades. Br Med J. (1998) 317:167. doi: 10.1136/BMJ.317.7152.167
93. Jones DW. Hypertension and cardiovascular disease: is a treatment strategy focused on high risk sufficient? J Clin Hypertens. (2018) 20(8):1146–8. doi: 10.1111/jch.13324
94. Blüher M, Aras M, Aronne LJ, Batterham RL, Giorgino F, Ji L, et al. New insights into the treatment of obesity. Diabetes Obes Metab. (2023) 25(8):2058–72. doi: 10.1111/dom.15077
95. Higgins GA, Fletcher PJ, Shanahan WR. Lorcaserin: a review of its preclinical and clinical pharmacology and therapeutic potential. Pharmacol Ther. (2020) 205:107417. doi: 10.1016/j.pharmthera.2019.107417
96. Grosso AF, de Oliveira SF, Higuchi Mde L, Favarato D, Dallan LA, da Luz PL. Synergistic anti-inflammatory effect: simvastatin and pioglitazone reduce inflammatory markers of plasma and epicardial adipose tissue of coronary patients with metabolic syndrome. Diabetol Metab Syndr. (2014) 6(1):47. doi: 10.1186/1758-5996-6-47
97. Idrees Z, Cancarevic I, Huang L. FDA-approved pharmacotherapy for weight loss over the last decade. Cureus. (2022) 14(9):e29262–00. doi: 10.7759/CUREUS.29262
98. Heck AM, Yanovski JA, Calis KA. Orlistat, a new lipase inhibitor for the management of obesity. Pharmacotherapy. (2000) 20:270–9. doi: 10.1592/PHCO.20.4.270.34882
99. Jordan J, Astrup A, Engeli S, Narkiewicz K, Day WW, Finer N. Cardiovascular effects of phentermine and topiramate: a new drug combination for the treatment of obesity. J Hypertens. (2014) 32:1178–88. doi: 10.1097/HJH.0000000000000145
100. Smith SR, Stenlof KS, Greenway FL, McHutchison J, Schwartz SM, Dev VB, et al. Orlistat 60 mg reduces visceral adipose tissue: a 24-week randomized, placebo-controlled, multicenter trial. Obesity. (2011) 19:1796–803. doi: 10.1038/OBY.2011.143
101. Wadden TA, Bailey TS, Billings LK, Davies M, Frias JP, Koroleva A, et al. Effect of subcutaneous semaglutide vs placebo as an adjunct to intensive behavioral therapy on body weight in adults with overweight or obesity: the STEP 3 randomized clinical trial. J Am Med Assoc. (2021) 325:1403–13. doi: 10.1001/JAMA.2021.1831
102. Khera R, Murad MH, Chandar AK, Dulai PS, Wang Z, Prokop LJ, et al. Association of pharmacological treatments for obesity with weight loss and adverse events: a systematic review and meta-analysis. J Am Med Assoc. (2016) 315:2424–34. doi: 10.1001/JAMA.2016.7602
103. Kim KK. Understanding the mechanism of action and clinical implications of anti-obesity drugs recently approved in Korea. Korean J Fam Med. (2019) 40:63–71. doi: 10.4082/KJFM.19.0013
104. Kulak-Bejda A, Bejda G, Waszkiewicz N. Safety and efficacy of naltrexone for weight loss in adult patients - a systematic review. Arch Med Sci. (2020) 17(4):940–53. doi: 10.5114/aoms.2020.96908
105. Pedersen SD, Manjoo P, Wharton S. Canadian adult obesity clinical practice guidelines: pharmacotherapy in obesity management. Available at: https://obesitycanada.ca/guidelines/pharmacotherapy (Accessed April 25, 2023)
106. Smith SR, Fujioka K, Gupta AK, Billes SK, Burns C, Kim D, et al. Combination therapy with naltrexone and bupropion for obesity reduces total and visceral adiposity. Diabetes Obes Metab. (2013) 15(9):863–6. doi: 10.1111/dom.12095
107. Cosentino G, Conrad AO, Uwaifo GI. Phentermine and topiramate for the management of obesity: a review. Drug Des Devel Ther. (2013) 7:267–78. doi: 10.2147/DDDT.S31443
108. Blind E, Janssen H, Dunder K, de Graeff PA. The European medicines agency’s approval of new medicines for type 2 diabetes. Diabetes Obes Metab. (2018) 20(9):2059–63. doi: 10.1111/dom.13349
109. Pi-Sunyer X, Astrup A, Fujioka K, Greenway F, Halpern A, Krempf M, et al. A randomized, controlled trial of 3.0 mg of liraglutide in weight management. N Engl J Med. (2015) 373:11–22. doi: 10.1056/NEJMOA1411892
110. Rubino DM, Greenway FL, Khalid U, O'Neil PM, Rosenstock J, Sørrig R, et al. Effect of weekly subcutaneous semaglutide vs daily liraglutide on body weight in adults with overweight or obesity without diabetes: the STEP 8 randomized clinical trial. J Am Med Assoc. (2022) 327:138–50. doi: 10.1001/JAMA.2021.23619
111. Rubino D, Abrahamsson N, Davies M, Hesse D, Greenway FL, Jensen C, et al. Effect of continued weekly subcutaneous semaglutide vs placebo on weight loss maintenance in adults with overweight or obesity: the STEP 4 randomized clinical trial. J Am Med Assoc. (2021) 325:1414–25. doi: 10.1001/JAMA.2021.3224
112. Marso SP, Daniels GH, Brown-Frandsen K, Kristensen P, Mann JFE, Nauck MA, et al. Liraglutide and cardiovascular outcomes in type 2 diabetes. N Engl J Med. (2016) 375:311–22. doi: 10.1056/NEJMOA1603827
114. Marso SP, Bain SC, Consoli A, Eliaschewitz FG, Jódar E, Leiter LA, et al. Semaglutide and cardiovascular outcomes in patients with type 2 diabetes. N Engl J Med. (2016) 375:1834–44. doi: 10.1056/NEJMOA1607141
115. Gerstein HC, Colhoun HM, Dagenais GR, Diaz R, Lakshmanan M, Pais P, et al. Dulaglutide and cardiovascular outcomes in type 2 diabetes (REWIND): a double-blind, randomised placebo-controlled trial. Lancet. (2019) 394:121–30. doi: 10.1016/S0140-6736(19)31149-3
116. Gerstein HC, Sattar N, Rosenstock J, Ramasundarahettige C, Pratley R, Lopes RD, et al. Cardiovascular and renal outcomes with efpeglenatide in type 2 diabetes. N Engl J Med. (2021) 385:896–907. doi: 10.1056/NEJMOA2108269
117. Davies MJ, Aronne LJ, Caterson ID, Thomsen AB, Jacobsen PB, Marso SP. Liraglutide and cardiovascular outcomes in adults with overweight or obesity: a post hoc analysis from SCALE randomized controlled trials. Diabetes Obes Metab. (2018) 20:734–9. doi: 10.1111/DOM.13125
118. Ryan DH, Lingvay I, Colhoun HM, Deanfield J, Emerson SS, Kahn SE, et al. Semaglutide effects on cardiovascular outcomes in people with overweight or obesity (SELECT) rationale and design. Am Heart J. (2020) 229:61–9. doi: 10.1016/J.AHJ.2020.07.008
119. Neeland IJ, Marso SP, Ayers CR, Lewis B, Oslica R, Francis W, et al. Effects of liraglutide on visceral and ectopic fat in adults with overweight and obesity at high cardiovascular risk: a randomised, double-blind, placebo-controlled, clinical trial. Lancet Diabetes Endocrinol. (2021) 9:595–605. doi: 10.1016/S2213-8587(21)00179-0
120. Yu D, Zou M, Pan Q, Song Y, Li M, Zhang X, et al. Effects of liraglutide or lifestyle interventions combined with other antidiabetic drugs on abdominal fat distribution in people with obesity and type 2 diabetes mellitus evaluated by the energy spectrum CT: a prospective randomized controlled study. Front Endocrinol. (2022) 13:951570. doi: 10.3389/FENDO.2022.951570
121. Cercato C, Fonseca FA. Cardiovascular risk and obesity. Diabetol Metab Syndr. (2019) 11:74. doi: 10.1186/s13098-019-0468-0
122. Anderson SL, Marrs JC. Antihyperglycemic medications and cardiovascular risk reduction. Eur Endocrinol. (2017) 13:86–90. doi: 10.17925/EE.2017.13.02.86
123. Gadde KM, Heymsfield SB. Targeting visceral adiposity with pharmacotherapy. Lancet Diabetes Endocrinol. (2021) 9:551–2. doi: 10.1016/S2213-8587(21)00204-7
124. Mah E, Chen O, Liska DJ, Blumberg JB. Dietary supplements for weight management: a narrative review of safety and metabolic health benefits. Nutrients. (2022) 14(9):1787. doi: 10.3390/nu14091787
125. Jordan MA. Interactions with drugs and dietary supplements used for weight loss. In: El-Shemy HA, editors. Drug discovery. InTech (2013). Avaibale at: https://www.intechopen.com/chapters/42071
126. Gasmi A, Mujawdiya PK, Nehaoua A, Shanaida M, Semenova Y, Piscopo S, et al. Pharmacological treatments and natural biocompounds in weight management. Pharmaceuticals (Basel). (2023) 16(2):212. doi: 10.3390/ph16020212
127. Chakhtoura M, Haber R, Ghezzawi M, Rhayem C, Tcheroyan R, Mantzoros CS. Pharmacotherapy of obesity: an update on the available medications and drugs under investigation. EClinicalMedicine. (2023) 58:101882. doi: 10.1016/j.eclinm.2023.101882
128. Qi X. Review of the clinical effect of orlistat. IOP Conf Ser Mater Sci Eng. (2018) 301:012063. doi: 10.1088/1757-899X/301/1/012063
129. Cavaliere H, Floriano I, Medeiros-Neto G. Gastrointestinal side effects of orlistat may be prevented by concomitant prescription of natural fibers (psyllium mucilloid). Int J Obes Relat Metab Disord. (2001) 25(7):1095–9. doi: 10.1038/sj.ijo.0801645
130. Yanovski SZ, Yanovski JA. Long-term drug treatment for obesity: a systematic and clinical review. J Am Med Assoc. (2014) 311:74–86. doi: 10.1001/jama.2013.281361
131. Brown NJ, Ryder D, Branch RA. A pharmacodynamic interaction between caffeine and phenylpropanolamine. Clin Pharmacol Ther. (1991) 50:363–71. doi: 10.1038/clpt.1991.152
132. AlRabiah H, Ahad A, Mostafa GAE, Al-Jenoobi FI. Effect of naltrexone hydrochloride on cytochrome P450 1A2, 2C9, 2D6, and 3A4 activity in human liver microsomes. Eur J Drug Metab Pharmacokinet. (2018) 43:707–13. doi: 10.1007/s13318-018-0482-x
133. Lei HP, Ji W, Lin J, Chen H, Tan ZR, Hu DL, et al. Effects of Ginkgo biloba extract on the pharmacokinetics of bupropion in healthy volunteers. Br J Clin Pharmacol. (2009) 68(2):201–6. doi: 10.1111/j.1365-2125.2009.03442.x
135. EMA/CHMP. Assessment report imcivree. Available at: www.ema.europa.eu/en/documents/assessment-report/imcivree-epar-public-assessment-report_en.pdf (Accessed July 10, 2023).
Keywords: obesity, visceral adipose tissue, residual cardiovascular risk, GLP-1 agonists, liraglutide, semaglutide
Citation: Cesaro A, De Michele G, Fimiani F, Acerbo V, Scherillo G, Signore G, Rotolo FP, Scialla F, Raucci G, Panico D, Gragnano F, Moscarella E, Scudiero O, Mennitti C and Calabrò P (2023) Visceral adipose tissue and residual cardiovascular risk: a pathological link and new therapeutic options. Front. Cardiovasc. Med. 10:1187735. doi: 10.3389/fcvm.2023.1187735
Received: 16 March 2023; Accepted: 13 July 2023;
Published: 27 July 2023.
Edited by:
Tatsuya Morimoto, University of Shizuoka, JapanReviewed by:
Sujith Rajan, New York University, United States© 2023 Cesaro, De Michele, Fimiani, Acerbo, Scherillo, Signore, Rotolo, Scialla, Raucci, Panico, Gragnano, Moscarella, Scudiero, Mennitti and Calabrò. This is an open-access article distributed under the terms of the Creative Commons Attribution License (CC BY). The use, distribution or reproduction in other forums is permitted, provided the original author(s) and the copyright owner(s) are credited and that the original publication in this journal is cited, in accordance with accepted academic practice. No use, distribution or reproduction is permitted which does not comply with these terms.
*Correspondence: Paolo Calabrò cGFvbG8uY2FsYWJyb0B1bmljYW1wYW5pYS5pdA==
†ORCID Arturo Cesaro orcid.org/0000-0003-4435-1235 Paolo Calabrò orcid.org/0000-0002-5018-830X
Disclaimer: All claims expressed in this article are solely those of the authors and do not necessarily represent those of their affiliated organizations, or those of the publisher, the editors and the reviewers. Any product that may be evaluated in this article or claim that may be made by its manufacturer is not guaranteed or endorsed by the publisher.
Research integrity at Frontiers
Learn more about the work of our research integrity team to safeguard the quality of each article we publish.