- 1Xiyuan Hospital, China Academy of Chinese Medical Sciences, Beijing, China
- 2Graduate School, Beijing University of Chinese Medicine, Beijing, China
- 3National Clinical Research Center for Chinese Medicine Cardiology, Beijing, China
Background: Myocardial ischemia/reperfusion injury (MIRI) refers to the more severe damage that occurs in the previously ischemic myocardium after a short-term interruption of myocardial blood supply followed by restoration of blood flow within a certain period of time. MIRI has become a major challenge affecting the therapeutic efficacy of cardiovascular surgery.
Methods: A scientific literature search on MIRI-related papers published from 2000 to 2023 in the Web of Science Core Collection database was conducted. VOSviewer was used for bibliometric analysis to understand the scientific development and research hotspots in this field.
Results: A total of 5,595 papers from 81 countries/regions, 3,840 research institutions, and 26,202 authors were included. China published the most papers, but the United States had the most significant influence. Harvard University was the leading research institution, and influential authors included Lefer David J., Hausenloy Derek J., Yellon Derek M., and others. All keywords can be divided into four different directions: risk factors, poor prognosis, mechanisms and cardioprotection.
Conclusion: Research on MIRI is flourishing. It is necessary to conduct an in-depth investigation of the interaction between different mechanisms and multi-target therapy will be the focus and hotspot of MIRI research in the future.
Introduction
The heart is a vital organ in maintaining the body's circulatory system, and the heart muscle requires an adequate supply of blood and oxygen to maintain its function. Myocardial infarction (MI) is a consequence of coronary artery occlusion, resulting in irreversible damage to the myocardium due to ischemia and hypoxia, and poses a severe threat to human health, with high rates of disability and mortality worldwide (1–3).
As early as the 1970s, Ginks et al. (4) performed myocardial ischemia-reperfusion mapping in dogs and found that reperfusion therapy was effective in restoring blood flow and reducing myocardial injury after MI. After years of research and observation, reperfusion therapy such as percutaneous coronary intervention (PCI) and coronary artery bypass grafting (CABG) have become the first-line treatment strategy for MI (5, 6). However, it has also been found that these therapies may induce myocardial, vascular, or electrophysiological dysfunction, leading to worsened cardiac function, and is responsible for up to 50% of the final infarct size. This phenomenon is known as myocardial ischemia/reperfusion injury (MIRI), which reduces the efficacy of myocardial reperfusion therapy (7–10).
Although more effective reperfusion techniques and drugs that improve MIRI have emerged, the incidence of secondary myocardial damage after blood flow restoration remains high due to narrow intervention windows and individual differences in susceptibility to reperfusion injury (11, 12). Therefore, the pathogenesis and prevention of MIRI remain a research hotspot in the cardiovascular field.
Bibliometrics presents the knowledge structure and frontier trends of a research field by modern techniques to visualize countries, institutions, authors, journals, documents and keywords (13, 14). Therefore, we reviewed the literature on Myocardial Ischemia-Reperfusion in the Web of Science Core Collection (WoSCC) database to provide a reference for future research on Myocardial Ischemia-Reperfusion.
Methods
Data sources
The data for this study was obtained from WoSCC. In order to more accurately capture the topic, we conducted subject searches in SCI-Expanded, SSCI, CCR-Expanded, IC, and ESCI. The search formula for this study was set as follows: TS = (myocardial NEAR/1 “reperfusion injur*”) OR TS = (Cardiac NEAR/1 “reperfusion injur*”). The search was conducted from January 1, 2000 to January 7, 2023, with article and review types selected, and English language limited. A total of 5,595 papers were obtained. The results were exported in txt. format as “Full Record and Cited References”. To prevent data deviation due to database updates, the data search and export were completed on January 7, 2023.
Analysis method
We primarily employed VOSviewer for data visualization, in conjunction with Excel, CiteSpace 6.1.R6, and Pajek 5.16 (15, 16). Firsty import the data into the CiteSpace software and check the duplicate data. Second, Synonyms are modified and merged before each visualization to show results more accurately. Third, we selected an appropriate number of nodes and set corresponding “Layout” parameters in VOSviewer, while leaving other options at their default values. We select the appropriate number of nodes for data visualization.
Results
Analysis of annual publications distribution
According to the search results, a total of 5,595 papers related to MIRI were collected by WoSCC from January 1st, 2000 to January 7th, 2023, as shown in Figure 1. Among them, there were 4,732 articles and 863 reviews, with a total citation count of 160,289 (excluding self-citations), an average citation frequency of 33.51 times per article, and an h-index of 165. Figure 2 shows the annual publication and citation volume. Overall, research related to MIRI showed an increasing trend, with the highest number of publications (589) and citations (24,492) in 2021.
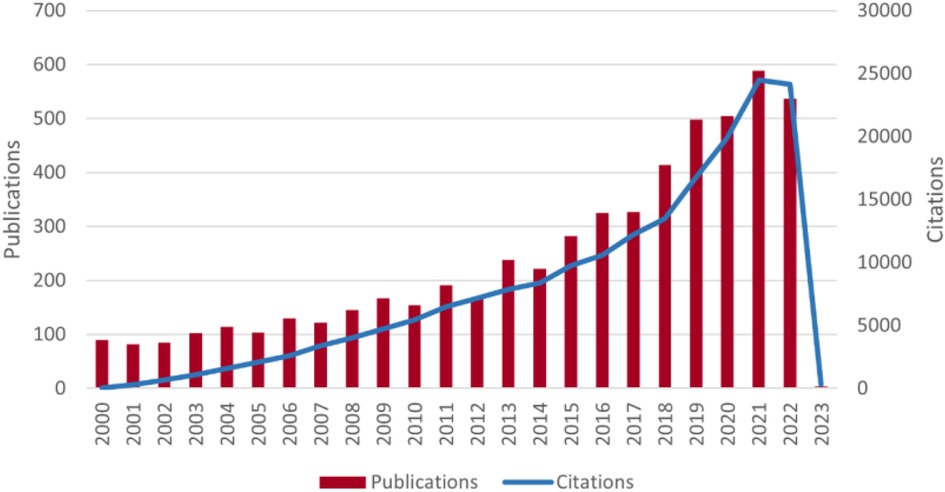
Figure 2. Trends in the growth of publications and the number of cited papers worldwide from 2000 to 2023. The data for 2023 are not complete.
Contribution of countries/regions
A total of 81 countries/regions participated in research on MIRI. Table 1 and Figure 3A show the top 10 countries/regions in terms of publication volume, as well as their citation counts, centrality, and annual publication volume. According to the statistics, China (2,845 papers) surpassed the United States (1,325 papers) in publication volume since 2011, followed by Germany (305 papers), Japan (278 papers), and England (233 papers). The United States had the highest citation count (75,508 times), followed by China (56,807 times), England (18,535 times), Germany (15,967 times), and Japan (13,349 times). The citation frequency of other countries was less than 10,000. Centrality of countries/regions is an important indicator of their importance. From the perspective of centrality, the United States, China, England, Italy, and Germany have high centrality and play important roles in this field.
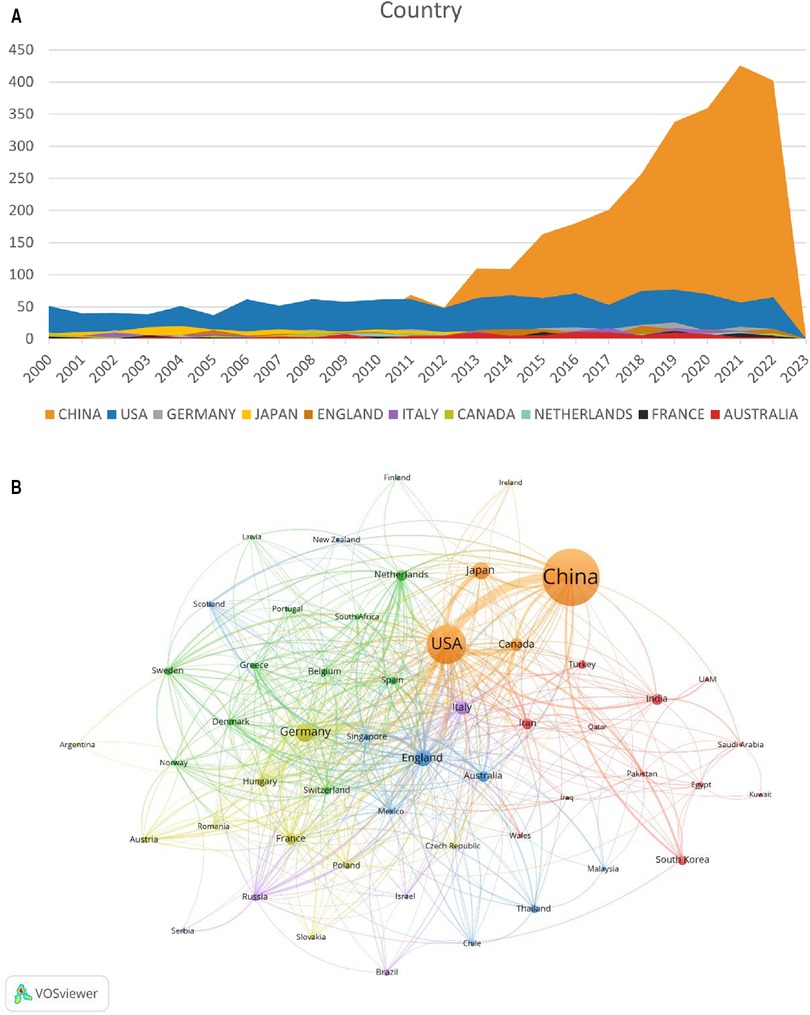
Figure 3. Distribution of countries/regions involved in MIRI. (A) The annual number of papers published by the top 10 countries. (B) Cooccurrence diagram of countries/regions with more than 5 publications. The size of the nodes corresponds to the publication volume of countries/regions. The lines represent the connections between countries/regions, and their thickness represents the link strength. Different colors represent different clusters.
Figure 3B shows a visualization analysis of 52 countries/regions with publication volume exceeding 5 papers. The size of the nodes corresponds to the publication volume, and the lines represent the connections between countries/regions. The countries/regions are roughly divided into six clusters based on the degree of cooperation, which are represented by different colors. The connections between countries/regions are mainly focused on the cooperation between the US and other countries, including China, Germany and Japan.
Contribution of institutions
A total of 3,840 institutions participated in research on MIRI after merging and eliminating meaningless nodes. Statistics and visual analysis were performed on 134 institutions with a publication volume of more than 15. Table 2 shows the top 10 institutions by publication volume, of which 9 are from China, but their centrality is low (<0.1). Harvard University is the only research institution that exceeds 0.1 and has the highest citation volume (7,372 times). It is worth noting that although University College London has only 59 publications, it ranks third in terms of citation volume (6,295 times). According to Figure 4A and Figure 4B, it can be seen that European and American countries, represented by Harvard University with relatively stable publication volume, began research in this area earlier; while Chinese research institutions have shown a significant fluctuating growth trend in the past decade.
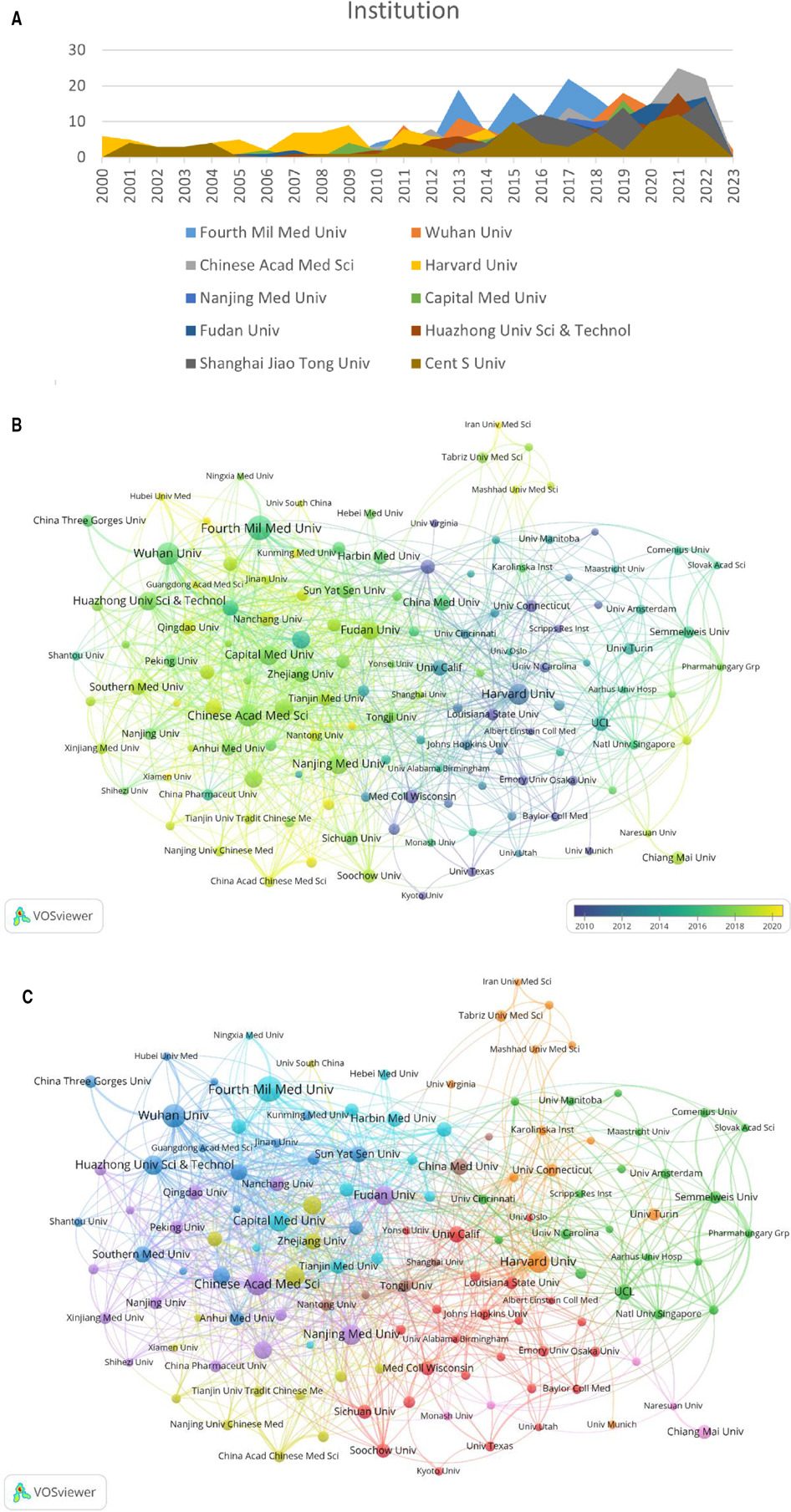
Figure 4. Distribution of institutions involved in MIRI. (A) The annual number of papers published by the top 10 institutions. (B) The time-overlay map of the cooperation network among the institutions. The color of an item is determined by the average year, where colors range from blue (2000y) to green to yellow (2023y). (C) Cooccurrence diagram of institutions with more than 15 publications. The size of the nodes corresponds to the publication volume of institutions. The lines represent the connections between institutions, and their thickness represents the link strength. Different colors represent different clusters.
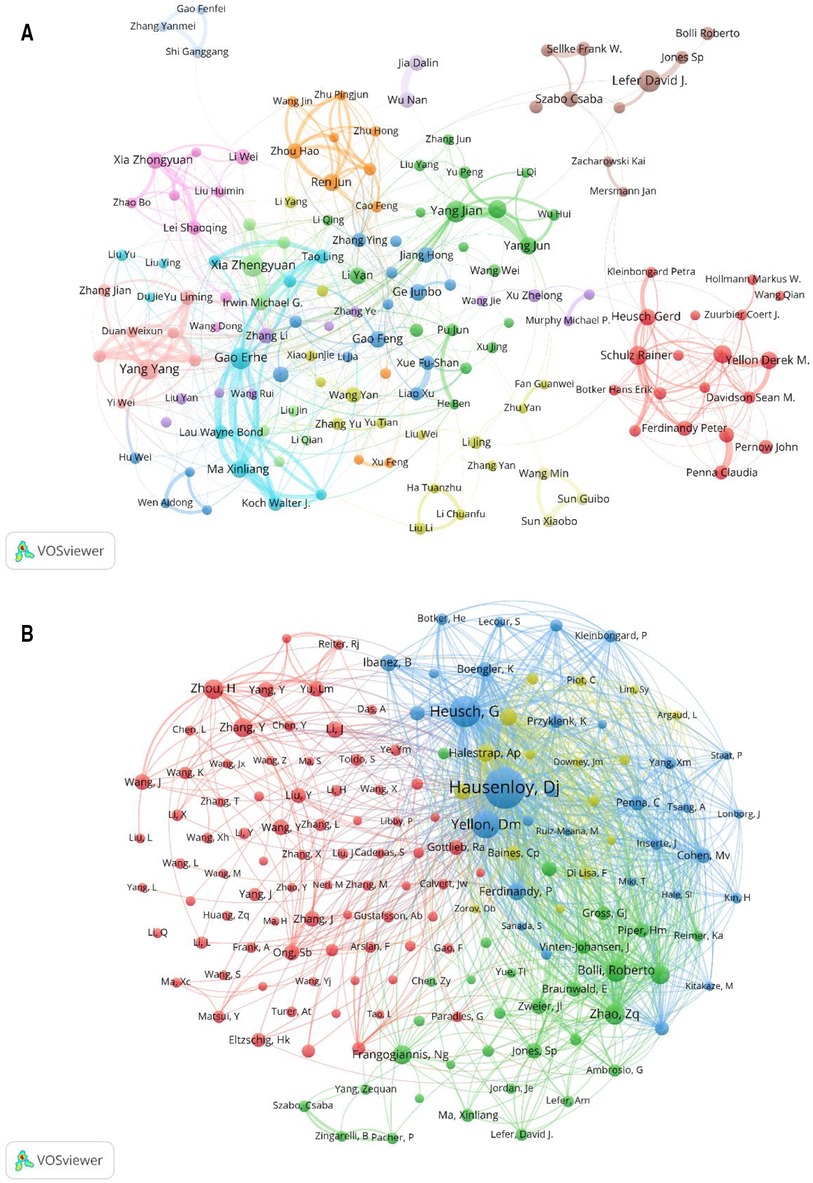
Figure 5. Distribution of authors involved in MIRI. (A) Cooccurrence diagram of authors. with more than 10 publications. The size of the nodes corresponds to the publications by the author, and the connections between the nodes reflect the collaboration relationship. Different colors represent different clusters. (B) Cooccurrence diagram of co-cited authors. The size of the node in the map represents the total frequency of co-citation. The larger the node, the more frequently it is co-cited.
In this field, the cooperation between institutions is relatively close and international exchanges are frequent. For example, the Fourth Military Medical University has cooperated with other institutions 80 times, especially with Temple University (14 times). In addition, two academic groups have been formed, one represented by Fudan Univ, Chinese Acad Med Sci and Nanjing Med Univ, and the other by Wuhan Univ and Huazhong Univ Sci & Technol, as shown in Figure 4C.
Authors and co-cited authors
Over the past 20 years, a total of 26,202 authors have participated in research related to MIRI. Among them, 168 authors with more than 10 publications were selected for visualization analysis. The largest number of papers was published by Lefer, David J. and Xia, Zhengyuan (45), followed by Gao, Erhe and Yang, Jian (42). The most co-cited author was Yellon, Derek M. (6,774), followed by Hausenloy, Derek J. (6,601), as shown in Table 3.
The collaboration among the authors of MIRI-related literature was displayed in VOSviewer. The same cluster often represents close collaboration and provides information for finding research partners. Several academic groups with relatively fixed collaborations have emerged in this field, as shown in Figure 5A. Furthermore, we can see that academic groups represented by Xia Zhengyuan, Gao Erhe, Yang Jian have more frequently with the outside world, while academic groups represented by Lefer David J. and Schulz Rainer are relatively isolated.
When two or more authors are cited by the same article, a co-citation relationship exists. The size of the node in the map represents the total frequency of co-citation. The larger the node, the more frequently it is co-cited, indicating greater influence in the field. Figure 5B shows that the research hotspots of the authors are highly homogeneous. The authors are mainly divided into 4 clusters: Hausenloy Derek J, Heusch Gerd etc. (blue); Bolli Roberto, Zhao ZQ etc. (green); Zhou Hao, Zhang Y etc. (red); Halestrap AP, Murry CE etc. (yellow).
Highly co-cited references
Co-citation analysis is a dynamic process that changes over time. It is used to study the internal connections between literature and depict the dynamic structure of scientific development.
The top 10 co-cited papers, totaling 11 papers, are listed in Table 4. In addition, 94 papers with co-citations exceeding 60 times were subjected to visual analysis, where the size of the nodes was proportional to the number of co-citations (Figure 6). The papers were divided into three clusters. The green cluster was led by “Mechanisms of disease: Myocardial reperfusion injury” (Yellon DM, 2007), with the highest number of co-citations (631 times). It mainly described four types of cardiac dysfunction caused by reperfusion injury, and summarizes the reasons for the discrepancies in outcomes of single-target interventions for MIRI in animal models and clinical studies. The study also confirmed new strategies to prevent lethal reperfusion injury by reperfusion injury salvage kinase (RISK) pathway and mitochondrial permeability transition pore (mPTP) (1).
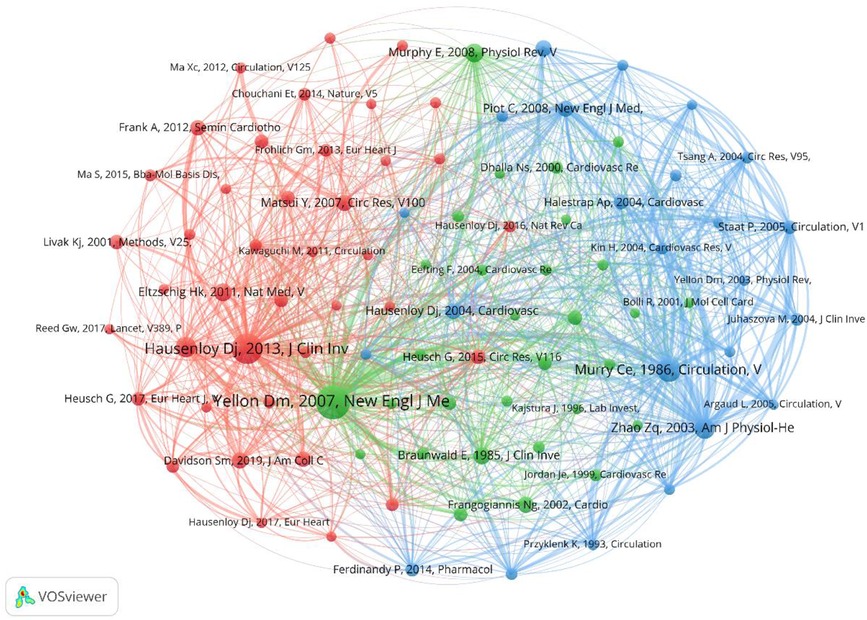
Figure 6. Cooccurrence diagram of references with co-citations exceeding 60 times. The size of the node indicates the cooccurrence frequencies of references, and the link reflects the cooccurrence relationship. The color of the node represents the respective cluster.
The red cluster was led by “Myocardial ischemia-reperfusion injury: a neglected therapeutic target” (Hausenloy DJ, 2013), with the highest number of co-citations (529 times). The article identified four recognized forms of MIRI, namely, reperfusion-induced arrhythmias, myocardial stunning, microvascular obstruction (MVO), and lethal myocardial reperfusion injury. It discussed in detail pathological mechanisms such as oxidative stress, calcium overload, pH value correction, mPTP, inflammation, and cell apoptosis, as well as new therapies. However, the article did not affirm the view that MI area would increase with prolonged reperfusion time. In addition, the study confirmed the position of cardiac magnetic resonance (CMR) imaging in the diagnosis and efficacy evaluation of MIRI (17).
The blue cluster was led by “Preconditioning with ischemia: a delay of lethal cell injury in ischemic myocardium” (Murry CE, 1986), followed by “Inhibition of myocardial injury by ischemic postconditioning during reperfusion: comparison with ischemic preconditioning” (Zhao ZQ, 2003) with 322 and 238 co-citations, respectively. The former was proposed by Murry et al. (18) using a canine model, which found that multiple brief ischemic episodes over a period of time could protect the heart from subsequent sustained ischemic injury, thereby introducing the concept of ischemic preconditioning. The latter, proposed by Zhi-Qing Zhao et al. (19), compared the effects of ischemic postconditioning and ischemic preconditioning and demonstrated that both were equally effective in reducing infarct size and protecting endothelial function.
Distribution of journals
The dual-map overlay of journals reveal the relatives position of the topic of study to the main research disciplines. Each point on the map represents a journal, with the citation graph on the left and the cited graph on the right. The curve represents the validation line, with different colors representing different citation relationships.
Figure 7 identifies three main paths, indicating that papers published in the “4 Molecular Biology, Biology, and Immunology” journal primarily reference papers in the fields of “8 Molecular Biology, Biology, and Genetics” and “5 Health, Nursing, and Medicine”. In addition, papers published in journals such as “8 Molecular Biology, Biology, and Genetics” are also commonly cited in papers in the “2 Medicine, Medicine, and Clinical” field. Currently, research on MIRI is mainly focused on clinical and molecular biology aspects.
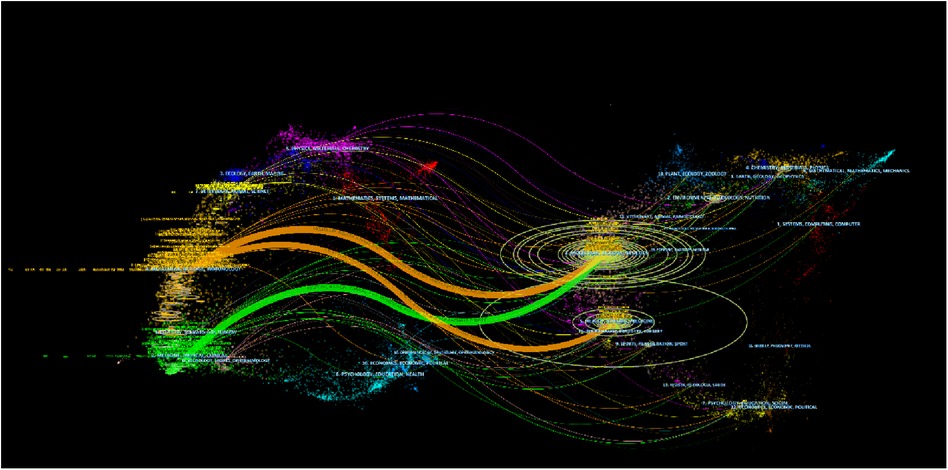
Figure 7. The dual-map overlay of journals. Each node on the map represents a journal, with the citation graph on the left and the cited graph on the right. The curve represents the validation line, with different colors representing different citation relationships.
Analysis of research hotspots
The keywords summarize the research topic of a paper and can be used to analyze the research hotspots and directions in the field of MIRI. Before visualization, synonyms (e.g., salvia miltiorrhiza and danshen), different spellings (e.g., ischemia, ischemic, and ischaemic), abbreviations (eg, IL-6 and interleukin-6), and singular/plural forms (e.g., arrhythmia and arrhythmias) should be merged. In VOSviewer, the keyword threshold was set at 15, resulting in a total of 142 keywords. The most frequent keyword was “MIRI” (2,918 times), followed by “myocardial ischemia” (734 times) and “apoptosis” (700 times), as shown in Table 5. These keywords can be divided into four different directions: (1) keywords related to risk factors are diabetes mellitus, hyperlipidemia, hypertension, and aging; (2) keywords related to poor prognosis, such as arrhythmia, myocardial stunning, cardiac function, and heart failure; (3) the study of pathological and physiological mechanisms mainly revolves around cell death, oxidative stress, inflammation, endoplasmic reticulum and mitochondria, non-coding RNAs (miRNA, lncRNA), and biomarkers, involving hot signaling pathways such as the PI3K/AKT pathway, Nf-κB pathway, and TLRs signaling pathway; (4) the main treatment-related keywords are cardiac protection, ischemic preconditioning, ischemic postconditioning, melatonin, dexmedetomidine, resveratrol, and Danshen, among others.
In the overlay visualization of keyword time series (Figure 8), each column represents a cluster, and the color represents the average time. The closer the color is to blue, the more frequently the keyword appeared in the early stage, and the closer to yellow, the more frequently the keyword appeared in recent years, which can reflect the research hotspot in a field to some extent. Keywords such as ferroptosis (2021.472), pyroptosis (2021.095), NLRP3 (2020.4), lncRNA (2020.25), mitochondrial dynamics (2020.2353), exosomes (2019.9643), mitophagy (2019.804), sirtuins (2019.7273), and necroptosis (2019.6522) have been frequently appearing in recent years, indicating that they are hotspots in the field of MIRI in recent years. It is worth noting that traditional Chinese medicine (TCM) (2019.2727) has gradually attracted attention worldwide for its role in treating MIRI. Representative drugs and effective ingredients include danshen, berberine, flavonoids and so on.
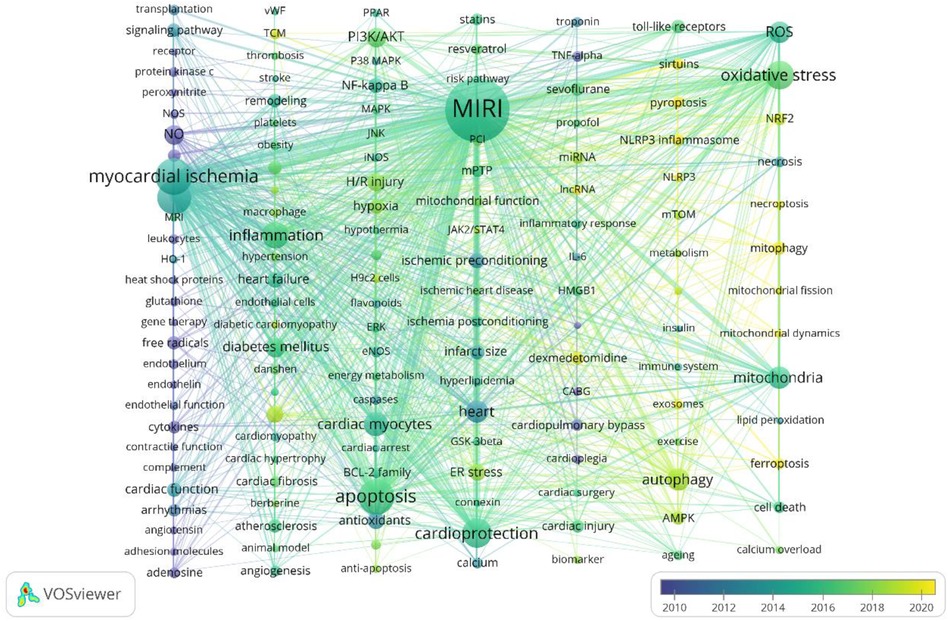
Figure 8. VOSviewer overlay visualization of keywords. Each column represents a cluster, and the color represents the average time. The closer the blue item is, the earlier it appears.
Discussion
General distribution
In terms of publications, the quantity of papers on myocardial ischemia- reperfusion injury has surpassed 100 papers per year since 2003. Since 2021, it has further increased to over 500 papers per year, indicating that research on MIRI remains a major focus in the cardiovascular field. Among them, the total number of papers published in China is more than 2,850, accounting for more than half of the total publication output. Especially since 2013, the number of publications has rapidly increased, indicating that research on MIRI has received increasing attention from the Chinese in the past decade. However, there is still a problem of insufficient influence. As one of the earliest institutions to begin research on myocardial ischemia reperfusion injury, the United States is another country with more than 1,000 publications, with the highest centrality. Among the top 10 most co-cited authors, 4 are from the United States.
In terms of cooperative relationships, frequent collaborations exist between countries/regions and institutions. For example, European and American academic institutions represented by Harvard University and University College London, and Chinese academic institutions represented by Fudan University and the Chinese Academy of Medical Sciences, all maintaining frequent collaborative relationships with other institutions.
Hotspots and frontiers
In risk factors
In risk factors, the most common keywords related to MIRI are diabetes mellitus, hyperlipidemias, hypertension, and aging, all of which have been reported to be associated with MIRI (20–23). Diabetes mellitus is a common comorbidity in cardiovascular disease patients and increases the risk of cardiovascular disease by 2–4 times compared to non-diabetic patients (24, 25). However, the controversy remains as to whether it increases the susceptibility of the heart to ischemia-reperfusion injury (26). In the co-morbidity study of MIRI and diabetes mellitus, a popular target is AMPK, which has been found to improve cardiovascular complications related to diabetes mellitus by stimulating AMPK. The mechanism mainly involves the downregulation of AMPK in the heart tissue of animals and humans with type 2 diabetes mellitus or metabolic syndrome, leading to energy metabolism disorder, aggravated inflammation, and intensified cellular autophagy, apoptosis, ferroptosis, and necroptosis pathways (27–30).
In addition, MVO as a potentially preexisting risk factor worsens within minutes after reperfusion and persists for at least 1 week (31), resulting in myocardial damage due to inadequate perfusion, known as the “no-reflow” phenomenon (32–34). Approximately 50% of acute MI patients experience ischemia-reperfusion injury in cardiac microvascular endothelial cells (CMECs), which is the main factor leading to the final infarct size and adverse cardiovascular outcomes (35, 36).
On the prognosis
On the prognosis, research indicates that reperfusion injury accounts for up to 50% of the final myocardial damage in acute MI (37). Common sequelae of reperfusion injury include heart failure, remodeling, arrhythmias, and myocardial stunning, which are consistent with our survey results.
Acute ST-segment elevation myocardial infarction (STEMI) patients who undergo primary PCI are prone to develop ventricular arrhythmias following ischemia-reperfusion injury, which are usually easily managed or self-terminated (38). The underlying cause may be related to the instability of action potential resulting from the inability of mitochondria to recover or maintain their inner membrane potential after prolonged ischemia (39–41). Moreover, this phenomenon is more common and severe in elderly female rats (42), which may be associated with the decline of estrogen receptors and antioxidant activity in their myocardium, rather than the decrease in serum estrogen levels (43).
Myocardial stunning refers to systolic and diastolic dysfunction in patients with acute myocardial ischemia after reperfusion, and the severity is proportional to the duration of ischemia (17). The mechanism of myocardial stunning is relatively mature. It mainly attributes to the massive formation of reactive oxygen species and calcium overload in cardiomyocytes and microvascular endothelial cells after reperfusion, which leads to endothelial dysfunction and decreased responsiveness to calcium in the excitation-contraction coupling mechanism. However this process is entirely reversible and generally lasts for several hours or days (44–46).
Infarct size is the main determinant of patient prognosis, and MIRI may further increase the infarct size (47, 48). There are two recognized forms of irreversible MIRI: MVO and lethal myocardial reperfusion injury (17, 49, 50). In the early stages of MI, ventricular compensatory mechanisms are activated, and cardiac function remains normal or slightly reduced. As the infarct size expands and non-infarcted areas remodel, the risk of developing heart failure or death remains high (51, 52). A questionnaire survey involving 850 patients showed that 2 out of every 5 patients had heart failure-related quality of life impairment after MI, causing a significant social and economic burden (53).
Regarding the mechanisms
Regarding the mechanisms, we found that the research fields with higher output focus on regulated cell death, oxidative stress, inflammatory response, non-coding RNA, as well as mitochondrial and endoplasmic reticulum stress. They form an intertwined association between different pathways that affect MIRI by regulating common pathway molecules. As the main type of cell death during reperfusion, regulated cell death plays an important role in the pathogenesis of MIRI (54). In our VOSviewer visualization, apoptosis has received the most attention. Nevertheless, non-apoptotic forms of regulated cell death, such as ferroptosis, pyroptosis, necroptosis, and autophagy have increasingly received the attention of researchers in recent years. They can operate alone or coexist with other forms of cell death, thereby playing a role in the enlargement of infarct size and the deterioration of heart function caused by ischemia-reperfusion (26, 55). The generation of reactive oxygen species (ROS) is the central pathogenic mechanism of MIRI. Ferroptosis is an iron overload and iron-dependent ROS accumulation process, and its regulatory mechanisms involve multiple signaling pathways and metabolic pathways, especially the glutathione peroxidase 4 (GPX4) axis (10, 26, 56). During myocardial ischemia-reperfusion, the increase in intracellular free iron levels and the decrease in GPX4 activity lead to the massive release of ROS within myocardial cells, causing lipid peroxidation (57–60). In addition, under the chemotactic effect of ROS, neutrophils accumulate in infarcted myocardial tissue several hours after reperfusion, and ferroptosis also promotes this process through Toll-like receptor 4-dependent signaling pathways, triggering harmful inflammatory responses and ultimately leading to cell death (17, 61). Studies have shown that this process mainly occurs during the reperfusion phase of MIRI rather than the ischemic phase (62, 63). It has been found that ferroptosis can be effectively inhibited by ferrostatin 1, liproxstatin 1, iron chelators, and antioxidants during reperfusion, which can protect against myocardial injury, reduce infarct size, and improve cardiac function in acute or chronic MIRI (62, 64–69).
Pyroptosis is a highly inflammatory form of cell death contributing to ischemia-reperfusion injury when overactivated. After reperfusion, the increased levels of calcium ions and ROS lead to the formation of NLRP3 inflammasomes that activate caspases, initiating the pyroptotic pathway (26, 70). On the hand, activated caspase-1/11 can activate Gasdermin D (GSDMD), a pore-forming protein that mediates cell death, increasing cell permeability and resulting in cell lysis typically (71–73). On the other hand, activated caspases cleave IL-1β and IL-18, releasing them outside the cell through GSDMD membrane pores, further triggering inflammation (26, 74). However, Shi et al. (75) found that caspase-11 may be the only pathway to trigger pyroptosis in cardiac myocytes. They also demonstrated that knocking out the GSDMD gene significantly reduced the levels of LDH and IL-18 after hypoxia/reoxygenation, and reduced the area of MI induced by ischemia-reperfusion in mice.
Necrosis has long been considered an uncontrolled form of cell death, but it has been found to occur in a regulated manner as well, known as necroptosis. Necroptosis involves the activation of the RIPK1/RIPK3/MLKL pathway and is recognized as another major programmed cell death type in MIRI now (76, 77). The process involves ischemia and oxidative stress-induced cardiac injury, as follows: the classical necroptotic pathway is usually initiated by the phosphorylation of RIPK1, which further phosphorylates RIPK3. The complex formed by RIPK1 and RIPK3 can induce MLKL oligomerization and translocation to the plasma membrane, leading to Ca2+ or Na+ ion influx and directly forming a pore, releasing damage-associated molecular patterns and causing membrane rupture (78, 79). However, RIPK3-induced myocardial necrosis can also occur independently of RIPK1 (80). When MIRI occurs, RIPK3 can be directly activated. Then through the RIPK3-CaMKII or RIPK3-PGAM5-CypD cascade, promoting the opening of the mPTP and participating in multiple signaling pathways that induce myocardial death (26, 81–84).
Multiple studies prove autophagy is primarily a pro-survival mechanism during short-term ischemia and hypoxia (9, 85). When the supply of oxygen and nutrients to cardiomyocytes is reduced and ATP is depleted quickly, the AMPK/mTOR pathway is activated (86, 87). At this time, cardiomyocytes utilize autophagy to degrade excessive or potentially dangerous cytosolic entities, such as damaged organelles or misfolded proteins, and acquire metabolic substrates to increase ATP production (88, 89). At the same time, an appropriate level of autophagy can reduce ROS production, decrease NLRP3-related inflammatory responses, and decrease other types of cell death, including necroptosis and apoptosis (90). However, research indicates that autophagy plays a dual role in MIRI, depending on the degree of its activation (91, 92). Unlike the ischemic phase, ROS accumulation is deemed the primary factor affecting autophagic flux during the reperfusion phase (93, 94). Elevated levels of ROS during reperfusion cause the opening of the mitochondrial permeability transition pore (MPTP), which promotes ROS release, activates Bnip3 (95–97), and induces the expression of the autophagy-related protein Beclin1 (93, 98). In normal conditions, the anti-apoptotic protein Bcl-2 binds to Beclin1, preventing autophagy. However, this balance could be disrupted by Bnip3, promoting autophagosome formation and increasing the autophagy rate (99, 100), eventually leading to cell death caused by excessive degradation of cellular components (54). Nevertheless, some perspectives propose that ischemia-reperfusion injury is associated with deficiencies in autophagosome-lysosome fusion (88, 101), which lead to cell death by impaired clearance of autophagosomes.
As an entrance to molecular regulators, non-coding RNAs (including miRNAs, lncRNAs, circRNAs) affect cellular function through targeting various molecules in signalling pathways and have been widely studied in cardiovascular disease (102, 103). Among them, miRNAs are the most widely studied ncRNAs. Elevated levels of ROS can cause DNA damage and regulate miRNAs, which can negatively regulate gene expression by inducing mRNA degradation or inhibiting their translation (104, 105). Previous studies have shown that during MIRI, miR-29c and miR-125a are significantly downregulated (106, 107), while miR-135b-3p is upregulated (108), playing roles in promoting autophagy and ferroptosis, respectively. In vivo/in vitro experiments showed that modulation of miR-1, miR-126, miR-140-3p, miR-214-5p, miR-125b and miR-24 could exert anti-apoptotic effects (109–114). Regulation of miR-133a, miR-15 exert anti-apoptotic effects (115–117). Moreover, miRNAs can bind to several mRNA molecules, allowing them to play multiple cellular functions. For example, miR-29b binds to PTEN. Its overexpression can reduce PTEN expression level and increased the protein levels of p-Akt/Akt and p-eNOS/eNOS, thereby exerting Anti-oxidative stress, Anti-inflammatory and Anti-apoptosis effects (118). MiR-125a-5p targets KLF13, TGFBR1, and DAAM1, promoting M2 macrophage polarization, inhibiting fibroblast proliferation and activation, and promoting angiogenesis, subsequently improving myocardial cell apoptosis and inflammation (107). Table 6 provides a summary of some important miRNAs. Table 6 provides a summary of some important miRNAs.
Cardioprotection
MI commonly causes two processes of myocardial injury, the first occurring during ischemia and the second possibly after reperfusion. The following treatment strategies can be summarized in light of these two processes. The first is mechanical ischemic conditioning, including brief ischemia-reperfusion cycles in the heart or tissues away from the heart, which can be achieved by ischemic preconditioning or postconditioning methods (138–141).
The second strategy involves drug therapy proven to protect the myocardium. For patients with pre-existing coronary artery disease, long-term and standardized treatment has been shown to effectively prevent major adverse cardiovascular events (MACE). Such as aspirin and ticagrelor, which can prevent reperfusion injury when given before reperfusion and effectively limit the area of MI (142, 143). The protective effect of simvastatin on contractile function in acute MIRI models may be related to the inhibition of the RhoA/ROCK pathway. Research shows SGLT2 inhibitors can alleviate the damage of MI in diabetic and non-diabetic hearts (144–146), reduce MIRI by inhibiting cardiomyocyte autophagy and protecting mitochondrial function, and reduce cardiovascular mortality and heart failure (HF) rehospitalization rate of patients after myocardial ischemia-reperfusion by targeting multiple pathways (26, 146–148). Nicorandil is widely used in the treatment of coronary heart disease and has a dual effect as a nitric oxide (NO) donor and increases cell membrane permeability to potassium ions. It has been shown to alleviate oxidative stress, inflammation, and apoptosis induced by ischemia-reperfusion (149, 150). A recent randomized double-blind controlled trial showed that nicorandil administered before primary PCI could improve the myocardial perfusion grade and increase the ejection fraction, and reduce myocardial infarct size in patients with ST-segment elevation MI (151). In addition, the soluble guanylate cyclase (sGC) stimulator vericiguat has been shown to reduce MIRI by improving microcirculation (152). Moreover, there are increasing studies and reports on the use of single or compound traditional Chinese medicine in the prevention and treatment of MIRI (153, 154). Resveratrol can reduce oxidative stress levels, Fe2+ content and inhibit ferroptosis induced by ischemia-reperfusion (155). Yang et al. (156) first reported that neocryptotanshinone can promote autophagolysosome clearance of protein aggregates via the ERK1/2-Nrf2-LAMP2 pathway, exerting therapeutic advantages for MIRI. Other Chinese medicines and effective ingredients, such as Madder, Calenduloside E, and Tubeimoside I, can also reduce infarct size and alleviate MIRI through different mechanisms, such as reducing inflammation, oxidative stress, or inhibiting cell death (157–159).
Thirdly, with the advancement of technology, research on novel therapeutic methods such as nanomedicine has gradually been carried out. Compared to free drugs, nanomedicine has better therapeutic effects and safety, attributed to its multifunctional carrier selection, targeted and controlled drug release, and improved bioavailability (160). Currently, common nanocarriers include liposomes (161–163), polymer nanoparticles (164, 165), inorganic nanoparticles (166) and extracellular vesicles (167–169). Carvedilol, a nonselective β-blocker, was encapsulated into platelet membrane vesicles (PMVs). Targeted administration of PMVs@Carvedilol may be a promising treatment for myocardial reperfusion injury, as it significantly improves postinjury cardiac function and increases drug utilization compared to other delivery methods (170). MicroRNAs use exosomes as a carrier to enable cell-to-cell communication (171). As previously mentioned, MIRI is often accompanied by abnormal expression of miRNAs. Enrichment of specific miRNAs by mesenchymal stem cell-secreted extracellular vesicles has shown promising results in regulating miRNA levels in cardiomyocytes in various preclinical experiments, making it a potential therapeutic approach (102, 172). It is worth noting that some miRNAs are dysregulated in multiple cardiovascular diseases. Therefore, selecting miRNAs that are dysregulated throughout the entire disease process leading to MIRI may have a stronger therapeutic effect. At the same time, it is essential to consider the safe and effective translation of preclinical experiments to clinical practice.
Limitations
Firstly, this study's bibliometric analysis only includes papers in the WoSCC database, while other databases such as PubMed, Cochrane library, and Google Scholar are excluded. However, it should be noted that WoSCC is widely recognized as one of the most authoritative scientific literature search platforms, covering the vast majority of research on MIRI and still maintaining a certain degree of representativeness. Secondly, papers published in recent years are rarely cited, which may lead to the omission of some recently published papers with significant contributions when analyzing highly co-cited papers, indicating the necessity of updating future research. In addition, changing job positions or using different names within the same institution during an author's career can also have a significant impact on research results.
Conclusion
Using information visualization techniques, we have attempted to elucidate the research progress, hot topics, and frontiers in MIRI over the past two decades. Although the annual publication output in China has far exceeded that in the United States in recent years, its academic influence is far behind. In addition, we have identified scholars, institutions, and representative literature that play important roles in this field. Keyword analysis shows that the main research direction is the pathogenesis of MIRI and corresponding protective strategies, with ferroptosis and pyroptosis as the latest hot topics.
Author contributions
YW and LG designed the study and co-wrote the manuscript. ZZ and PH collected the data. SF and AW re-examined the data. YW and ZZ analyzed the data. XM and ML reviewed and revised the manuscript. All authors contributed to the article and approved the submitted version.
Funding
This study was supported by the National Key Research and Development Program of China (No. 2018YFC1707401).
Conflict of interest
The authors declare that the research was conducted in the absence of any commercial or financial relationships that could be construed as a potential conflict of interest.
Publisher's note
All claims expressed in this article are solely those of the authors and do not necessarily represent those of their affiliated organizations, or those of the publisher, the editors and the reviewers. Any product that may be evaluated in this article, or claim that may be made by its manufacturer, is not guaranteed or endorsed by the publisher.
References
1. Yellon DM, Hausenloy DJ. Mechanisms of disease: myocardial reperfusion injury. N Engl J Med. (2007) 357(11):1121–35. doi: 10.1056/NEJMra071667
2. Thygesen K, Alpert JS, Jaffe AS, Chaitman BR, Bax JJ, Morrow DA, et al. Fourth universal definition of myocardial infarction (2018). Circulation. (2018) 138(20):e618–51. doi: 10.1161/CIR.0000000000000617
3. Roth GA, Mensah GA, Johnson CO, Addolorato G, Ammirati E, Baddour LM, et al. Global burden of cardiovascular diseases and risk factors, 1990-2019: update from the GBD 2019 study. J Am Coll Cardiol. (2020) 76(25):2982–3021. doi: 10.1016/j.jacc.2020.11.010
4. Ginks WR, Sybers HD, Maroko PR, Covell JW, Sobel BE, Ross J. Coronary artery reperfusion. II. Reduction of myocardial infarct size at 1 week after the coronary occlusion. J Clin Invest. (1972) 51(10):2717–23. doi: 10.1172/JCI107091
5. Jernberg T, Johanson P, Held C, Svennblad B, Lindbäck J, Wallentin L, et al. Association between adoption of evidence-based treatment and survival for patients with ST-elevation myocardial infarction. J Am Med Assoc. (2011) 305(16):1677–84. doi: 10.1001/jama.2011.522
6. Hausenloy DJ, Botker HE, Engstrom T, Erlinge D, Heusch G, Ibanez B, et al. Targeting reperfusion injury in patients with ST-segment elevation myocardial infarction: trials and tribulations. Eur Heart J. (2017) 38(13):935–41. doi: 10.1093/eurheartj/ehw145
7. Maron DJ, Hochman JS, Reynolds HR, Bangalore S, O’Brien SM, Boden WE, et al. Initial invasive or conservative strategy for stable coronary disease. N Engl J Med. (2020) 382(15):1395–407. doi: 10.1056/NEJMoa1915922
8. Braunwald E, Kloner RA. Myocardial reperfusion: a double-edged sword? J Clin Invest. (1985) 76(5):1713–9. doi: 10.1172/JCI112160
9. Heusch G. Myocardial ischaemia-reperfusion injury and cardioprotection in perspective. Nat Rev Cardiol. (2020) 17(12):773–89. doi: 10.1038/s41569-020-0403-y
10. Han X, Zhang J, Liu J, Wang H, Du F, Zeng X, et al. Targeting ferroptosis: a novel insight against myocardial infarction and ischemia-reperfusion injuries. Apoptosis. (2022) 6:108–23. doi: 10.1007/s10495-022-01785-2
11. Fordyce CB, Gersh BJ, Stone GW, Granger CB. Novel therapeutics in myocardial infarction: targeting microvascular dysfunction and reperfusion injury. Trends Pharmacol Sci. (2015) 36:605–16. doi: 10.1016/j.tips.2015.06.004
12. Safiri S, Karamzad N, Singh K, Carson-Chahhoud K, Adams C, Nejadghaderi SA, et al. Burden of ischemic heart disease and its attributable risk factors in 204 countries and territories, 1990-2019. Eur J Prev Cardiol. (2022) 29:420–31. doi: 10.1093/eurjpc/zwab213
13. Broadus RN. Toward a definition of “bibliometrics”. Scientometrics. (1987) 12(5-6):373–9. doi: 10.1007/BF02016680
14. Ninkov A, Frank JR, Maggio LA. Bibliometrics: methods for studying academic publishing. Perspect Med Educ. (2022) 11(3):173–6. doi: 10.1007/s40037-021-00695-4
15. Moral-Munoz JA, Herrera-Viedma E, Santisteban-Espejo A, Cobo MJ. Software tools for conducting bibliometric analysis in science: an up-to-date review. Prof Inf. (2020) 29(1):e290103. doi: 10.3145/epi.2020.ene.03
16. van Eck NJ, Waltman L. Software survey: VOSviewer, a computer program for bibliometric mapping. Scientometrics. (2010) 84(2):523–38. doi: 10.1007/s11192-009-0146-3
17. Hausenloy DJ, Yellon DM. Myocardial ischemia-reperfusion injury: a neglected therapeutic target. J Clin Invest. (2013) 123(1):92–100. doi: 10.1172/JCI62874
18. Murry CE, Jennings RB, Reimer KA. Preconditioning with ischemia: a delay of lethal cell injury in ischemic myocardium. Circulation. (1986) 74(5):1124–36. doi: 10.1161/01.cir.74.5.1124
19. Zhao ZQ, Corvera JS, Halkos ME, Kerendi F, Wang NP, Guyton RA, et al. Inhibition of myocardial injury by ischemic postconditioning during reperfusion: comparison with ischemic preconditioning. Am J Physiol Heart Circ Physiol. (2003) 285(2):H579–88. doi: 10.1152/ajpheart.01064.2002
20. Ferdinandy P, Schulz R, Baxter GF. Interaction of cardiovascular risk factors with myocardial ischemia/reperfusion injury, preconditioning, and postconditioning. Pharmacol Rev. (2007) 59(4):418–58. doi: 10.1124/pr.107.06002
21. Penna C, Andreadou I, Aragno M, Beauloye C, Bertrand L, Lazou A, et al. Effect of hyperglycaemia and diabetes on acute myocardial ischaemia-reperfusion injury and cardioprotection by ischaemic conditioning protocols. Br J Pharmacol. (2020) 177(23):5312–35. doi: 10.1111/bph.14993
22. Andreadou I, Schulz R, Badimon L, Adameová A, Kleinbongard P, Lecour S, et al. Hyperlipidaemia and cardioprotection: animal models for translational studies. Br J Pharmacol. (2020) 177(23):5287–311. doi: 10.1111/bph.14931
23. Lin Z, Zhang YH. Ischemic reperfusion injury in hypertensive rat heart. J Hypertens. (2022) 40(Suppl 1):e114. doi: 10.1097/01.hjh.0000836412.18071.b8
24. Lejay A, Fang F, John R, Van JAD, Barr M, Thaveau F, et al. Ischemia reperfusion injury, ischemic conditioning and diabetes mellitus. J Mol Cell Cardiol. (2016) 91:11–22. doi: 10.1016/j.yjmcc.2015.12.020
25. Verma S, Pandey A, Bhatt DL. Forecasting heart failure risk in diabetes. J Am Coll Cardiol. (2022) 79(23):2294–7. doi: 10.1016/j.jacc.2022.04.011
26. Adameova A, Horvath C, Abdul-Ghani S, Varga ZV, Saadeh Suleiman M, Dhalla NS. Interplay of oxidative stress and necrosis-like cell death in cardiac ischemia/reperfusion injury: a focus on necroptosis. Biomedicines. (2022) 10(1):127. doi: 10.3390/biomedicines10010127
27. Gan L, Xie D, Liu J, Lau WB, Christopher TA, Lopez B, et al. Small extracellular microvesicles mediated pathological communications between dysfunctional adipocytes and cardiomyocytes as a novel mechanism exacerbating ischemia/reperfusion injury in diabetic mice. Circulation. (2020) 141(12):968–83. doi: 10.1161/CIRCULATIONAHA.119.042640
28. Paskeh MDA, Asadi A, Mirzaei S, Hashemi M, Entezari M, Raesi R, et al. Targeting AMPK signaling in ischemic/reperfusion injury: from molecular mechanism to pharmacological interventions. Cell Signal. (2022) 94:110323. doi: 10.1016/j.cellsig.2022.110323
29. Kandula N, Kumar S, Mandlem VKK, Siddabathuni A, Singh S, Kosuru R. Role of AMPK in myocardial ischemia-reperfusion injury-induced cell death in the presence and absence of diabetes. Oxid Med Cell Longev. (2022) 2022:7346699. doi: 10.1155/2022/7346699
30. Yao M, Wang Z, Jiang L, Wang L, Yang Y, Wang Q, et al. Oxytocin ameliorates high glucose- and ischemia/reperfusion-induced myocardial injury by suppressing pyroptosis via AMPK signaling pathway. Biomed Pharmacother. (2022) 153:113498. doi: 10.1016/j.biopha.2022.113498
31. Ibáñez B, Heusch G, Ovize M, Van de Werf F. Evolving therapies for myocardial ischemia/reperfusion injury. J Am Coll Cardiol. (2015) 65(14):1454–71. doi: 10.1016/j.jacc.2015.02.032
32. Kloner RA, Ganote CE, Jennings RB. The “no-reflow” phenomenon after temporary coronary occlusion in the dog. J Clin Invest. (1974) 54(6):1496–508. doi: 10.1172/JCI107898
33. Rezkalla SH, Kloner RA. No-reflow phenomenon. Circulation. (2002) 105(5):656–62. doi: 10.1161/hc0502.102867
34. Allencherril J, Jneid H, Atar D, Alam M, Levine G, Kloner RA, et al. Pathophysiology, diagnosis, and management of the no-reflow phenomenon. Cardiovasc Drugs Ther. (2019) 33(5):589–97. doi: 10.1007/s10557-019-06901-0
35. Niccoli G, Burzotta F, Galiuto L, Crea F. Myocardial no-reflow in humans. J Am Coll Cardiol. (2009) 54(4):281–92. doi: 10.1016/j.jacc.2009.03.054
36. Zhang B, Liu G, Huang B, Liu H, Jiang H, Hu Z, et al. KDM3A attenuates myocardial ischemic and reperfusion injury by ameliorating cardiac microvascular endothelial cell pyroptosis. Oxid Med Cell Longev. (2022) 2022:4622520. doi: 10.1155/2022/4622520
37. Hausenloy DJ, Bøtker HE, Condorelli G, Ferdinandy P, Garcia-Dorado D, Heusch G, et al. Translating cardioprotection for patient benefit: position paper from the working group of cellular biology of the heart of the European society of cardiology. Cardiovasc Res. (2013) 98(1):7–27. doi: 10.1093/cvr/cvt004
38. Neri M, Riezzo I, Pascale N, Pomara C, Turillazzi E. Ischemia/reperfusion injury following acute myocardial infarction: a critical issue for clinicians and forensic pathologists. Mediators Inflamm. (2017) 2017:7018393. doi: 10.1155/2017/7018393
39. Akar FG, Aon MA, Tomaselli GF, O’Rourke B. The mitochondrial origin of postischemic arrhythmias. J Clin Invest. (2005) 115(12):3527–35. doi: 10.1172/JCI25371
40. Consolini AE, Ragone MI, Bonazzola P, Colareda GA. Mitochondrial bioenergetics during ischemia and reperfusion. Adv Exp Med Biol. (2017) 982:141–67. doi: 10.1007/978-3-319-55330-6_8
41. Solhjoo S, O’Rourke B. Mitochondrial instability during regional ischemia-reperfusion underlies arrhythmias in monolayers of cardiomyocytes. J Mol Cell Cardiol. (2015) 78:90–9. doi: 10.1016/j.yjmcc.2014.09.024
42. Bozdogan Ö, Kaya ST, Yasar S, Orallar H. Effect of ATP-dependent channel modulators on ischemia-induced arrhythmia change depending on age and gender. Exp Biol Med. (2013) 238(10):1170–79. doi: 10.1177/1535370213498980
43. Bozdogan O, Bozcaarmutlu A, Kaya ST, Sapmaz C, Ozarslan TO, Eksioglu D, et al. Decreasing myocardial estrogen receptors and antioxidant activity may be responsible for increasing ischemia- and reperfusion-induced ventricular arrhythmia in older female rats. Life Sci. (2021) 271:119190. doi: 10.1016/j.lfs.2021.119190
44. Verma S, Fedak PWM, Weisel RD, Butany J, Rao V, Maitland A, et al. Fundamentals of reperfusion injury for the clinical cardiologist. Circulation. (2002) 105(20):2332–6. doi: 10.1161/01.cir.0000016602.96363.36
45. Guaricci AI, Bulzis G, Pontone G, Scicchitano P, Carbonara R, Rabbat M, et al. Current interpretation of myocardial stunning. Trends Cardiovasc Med. (2018) 28(4):263–71. doi: 10.1016/j.tcm.2017.11.005
46. Heusch G. Myocardial stunning and hibernation revisited. Nat Rev Cardiol. (2021) 18(7):522–36. doi: 10.1038/s41569-021-00506-7
47. Stone GW, Selker HP, Thiele H, Patel MR, Udelson JE, Ohman EM, et al. Relationship between infarct size and outcomes following primary PCI: patient-level analysis from 10 randomized trials. J Am Coll Cardiol. (2016) 67(14):1674–83. doi: 10.1016/j.jacc.2016.01.069
48. Liu T, Howarth AG, Chen Y, Nair AR, Yang HJ, Ren D, et al. Intramyocardial hemorrhage and the “wave front” of reperfusion injury compromising myocardial salvage. J Am Coll Cardiol. (2022) 79(1):35–8. doi: 10.1016/j.jacc.2021.10.034
49. Ibanez B, Fuster V, Jimenez-Borreguero J, Badimon JJ. Lethal myocardial reperfusion injury: a necessary evil? Int J Cardiol. (2011) 151(1):3–11. doi: 10.1016/j.ijcard.2010.10.056
50. de Waha S, Patel MR, Granger CB, Ohman EM, Maehara A, Eitel I, et al. Relationship between microvascular obstruction and adverse events following primary percutaneous coronary intervention for ST-segment elevation myocardial infarction: an individual patient data pooled analysis from seven randomized trials. Eur Heart J. (2017) 38(47):3502–10. doi: 10.1093/eurheartj/ehx414
51. Del Buono MG, Moroni F, Montone RA, Azzalini L, Sanna T, Abbate A. Ischemic cardiomyopathy and heart failure after acute myocardial infarction. Curr Cardiol Rep. (2022) 24(10):1505–15. doi: 10.1007/s11886-022-01766-6
52. Heidenreich PA, Bozkurt B, Aguilar D, Allen LA, Byun JJ, et al. 2022 AHA/ACC/HFSA guideline for the management of heart failure: a report of the American college of cardiology/American heart association joint committee on clinical practice guidelines. J Am Coll Cardiol. (2022) 79(17):e263–421. doi: 10.1016/j.jacc.2021.12.012
53. Wohlfahrt P, Jenca D, Stehlik J, Melenovský V, Mrázková J, Stanek V, et al. Heart failure-related quality-of-life impairment after myocardial infarction. Clin Res Cardiol. (2023) 112(1):39–48. doi: 10.1007/s00392-022-02008-z
54. Del Re DP, Amgalan D, Linkermann A, Liu Q, Kitsis RN. Fundamental mechanisms of regulated cell death and implications for heart disease. Physiol Rev. (2019) 99(4):1765–817. doi: 10.1152/physrev.00022.2018
55. Lu L-Q, Tian J, Luo X-J, Peng J. Targeting the pathways of regulated necrosis: a potential strategy for alleviation of cardio-cerebrovascular injury. Cell Mol Life Sci. (2021) 78(1):63–78. doi: 10.1007/s00018-020-03587-8
56. Li N, Jiang W, Wang W, Xiong R, Wu X, Geng Q. Ferroptosis and its emerging roles in cardiovascular diseases. Pharmacol Res. (2021) 166:105466. doi: 10.1016/j.phrs.2021.105466
57. Granger DN, Kvietys PR. Reperfusion injury and reactive oxygen species: the evolution of a concept. Redox Biol. (2015) 6:524–51. doi: 10.1016/j.redox.2015.08.020
58. Park E, Chung SW. ROS-mediated autophagy increases intracellular iron levels and ferroptosis by ferritin and transferrin receptor regulation. Cell Death Dis. (2019) 10(11):822. doi: 10.1038/s41419-019-2064-5
59. Li X, Ma N, Xu J, Zhang Y, Yang P, Su X, et al. Targeting ferroptosis: pathological mechanism and treatment of ischemia-reperfusion injury. Oxid Med Cell Longev. (2021) 2021:1587922. doi: 10.1155/2021/1587922
60. Miyamoto HD, Ikeda M, Ide T, Tadokoro T, Furusawa S, Abe K, et al. Iron overload via heme degradation in the endoplasmic Reticulum triggers ferroptosis in myocardial ischemia-reperfusion injury. JACC Basic Transl Sci. (2022) 7(8):800–19. doi: 10.1016/j.jacbts.2022.03.012
61. Li W, Feng G, Gauthier JM, Lokshina I, Higashikubo R, Evans S, et al. Ferroptotic cell death and TLR4/trif signaling initiate neutrophil recruitment after heart transplantation. J Clin Invest. (2019) 129(6):2293–304. doi: 10.1172/JCI126428
62. Tang L-J, Luo X-J, Tu H, Chen H, Xiong XM, Li NS, et al. Ferroptosis occurs in phase of reperfusion but not ischemia in rat heart following ischemia or ischemia/reperfusion. Naunyn Schmiedebergs Arch Pharmacol. (2021) 394(2):401–10. doi: 10.1007/s00210-020-01932-z
63. Zhou L, Han S, Guo J, Qiu T, Zhou J, Shen L. Ferroptosis-A new dawn in the treatment of organ ischemia-reperfusion injury. Cells. (2022) 11(22):3653. doi: 10.3390/cells11223653
64. Gao M, Monian P, Quadri N, Ramasamy R, Jiang X. Glutaminolysis and transferrin regulate ferroptosis. Mol Cell. (2015) 59(2):298–308. doi: 10.1016/j.molcel.2015.06.011
65. Wu X, Li Y, Zhang S, Zhou X. Ferroptosis as a novel therapeutic target for cardiovascular disease. Theranostics. (2021) 11(7):3052–9. doi: 10.7150/thno.54113
66. Fang X, Ardehali H, Min J, Wang F. The molecular and metabolic landscape of iron and ferroptosis in cardiovascular disease. Nat Rev Cardiol. (2023) 20(1):7–23. doi: 10.1038/s41569-022-00735-4
67. Fang X, Wang H, Han D, Xie E, Yang X, Wei J, et al. Ferroptosis as a target for protection against cardiomyopathy. Proc Natl Acad Sci U S A. (2019) 116(7):2672–80. doi: 10.1073/pnas.1821022116
68. Zhang Y, Xin L, Xiang M, Shang C, Wang Y, Wang Y, et al. The molecular mechanisms of ferroptosis and its role in cardiovascular disease. Biomed Pharmacother. (2022) 145:112423. doi: 10.1016/j.biopha.2021.112423
69. Feng Y, Madungwe NB, Imam Aliagan AD, Tombo N, Bopassa JC. Liproxstatin-1 protects the mouse myocardium against ischemia/reperfusion injury by decreasing VDAC1 levels and restoring GPX4 levels. Biochem Biophys Res Commun. (2019) 520(3):606–11. doi: 10.1016/j.bbrc.2019.10.006
70. Lu F, Lan Z, Xin Z, He C, Guo Z, Xia X, et al. Emerging insights into molecular mechanisms underlying pyroptosis and functions of inflammasomes in diseases. J Cell Physiol. (2020) 235(4):3207–21. doi: 10.1002/jcp.29268
71. Broz P, Pelegrín P, Shao F. The gasdermins, a protein family executing cell death and inflammation. Nat Rev Immunol. (2020) 20(3):143–57. doi: 10.1038/s41577-019-0228-2
72. Chen X, He WT, Hu L, Li J, Fang Y, Wang X, et al. Pyroptosis is driven by non-selective gasdermin-D pore and its morphology is different from MLKL channel-mediated necroptosis. Cell Res. (2016) 26(9):1007–20. doi: 10.1038/cr.2016.100
73. Galluzzi L, Vitale I, Aaronson SA, Abrams JM, Adam D, Agostinis P, et al. Molecular mechanisms of cell death: recommendations of the nomenclature committee on cell death 2018. Cell Death Differ. (2018) 25(3):486–541. doi: 10.1038/s41418-017-0012-4
74. Zhang X, Qu H, Yang T, Kong X, Zhou H. Regulation and functions of NLRP3 inflammasome in cardiac fibrosis: current knowledge and clinical significance. Biomed Pharmacother. (2021) 143:112219. doi: 10.1016/j.biopha.2021.112219
75. Shi H, Gao Y, Dong Z, Yang J, Gao R, Li X, et al. GSDMD-mediated cardiomyocyte pyroptosis promotes myocardial I/R injury. Circ Res. (2021) 129(3):383–96. doi: 10.1161/CIRCRESAHA.120.318629
76. Degterev A, Huang Z, Boyce M, Li Y, Jagtap P, Mizushima N, et al. Chemical inhibitor of nonapoptotic cell death with therapeutic potential for ischemic brain injury. Nat Chem Biol. (2005) 1(2):112–9. doi: 10.1038/nchembio711
77. Vandenabeele P, Galluzzi L, Berghe TV, Kroemer G. Molecular mechanisms of necroptosis: an ordered cellular explosion. Nat Rev Mol Cell Biol. (2010) 11(10):700–14. doi: 10.1038/nrm2970
78. Chen H, Tang LJ, Tu H, Zhou YJ, Li NS, Luo XJ, et al. Arctiin protects rat heart against ischemia/reperfusion injury via a mechanism involving reduction of necroptosis. Eur J Pharmacol. (2020) 875:173053. doi: 10.1016/j.ejphar.2020.173053
79. Li L, Lin L, Lei S, Shi S, Chen C, Xia Z. Maslinic acid inhibits myocardial ischemia-reperfusion injury-induced apoptosis and necroptosis via promoting autophagic flux. DNA Cell Biol. (2022) 41(5):487–97. doi: 10.1089/dna.2021.0918
80. Luedde M, Lutz M, Carter N, Sosna J, Jacoby C, Vucur M, et al. RIP3, A kinase promoting necroptotic cell death, mediates adverse remodelling after myocardial infarction. Cardiovasc Res. (2014) 103(2):206–16. doi: 10.1093/cvr/cvu146
81. Zhang T, Zhang Y, Cui M, Jin L, Wang Y, Lv F, et al. CaMKII is a RIP3 substrate mediating ischemia- and oxidative stress-induced myocardial necroptosis. Nat Med. (2016) 22(2):175–82. doi: 10.1038/nm.4017
82. Zhou H, Li D, Zhu P, Ma Q, Toan S, Wang J, et al. Inhibitory effect of melatonin on necroptosis via repressing the Ripk3-PGAM5-CypD-mPTP pathway attenuates cardiac microvascular ischemia-reperfusion injury. J Pineal Res. (2018) 65(3):e12503. doi: 10.1111/jpi.12503
83. Zhu P, Hu S, Jin Q, Li D, Tian F, Toan S, et al. Ripk3 promotes ER stress-induced necroptosis in cardiac IR injury: a mechanism involving calcium overload/XO/ROS/mPTP pathway. Redox Biol. (2018) 16:157–68. doi: 10.1016/j.redox.2018.02.019
84. Ying L, Benjanuwattra J, Chattipakorn SC, Chattipakorn N. The role of RIPK3-regulated cell death pathways and necroptosis in the pathogenesis of cardiac ischaemia-reperfusion injury. Acta Physiol. (2021) 231(2):e13541. doi: 10.1111/apha.13541
85. Levine B, Yuan J. Autophagy in cell death: an innocent convict? J Clin Invest. (2005) 115(10):2679–88. doi: 10.1172/JCI26390
86. Egan D, Kim J, Shaw RJ, Guan K-L. The autophagy initiating kinase ULK1 is regulated via opposing phosphorylation by AMPK and mTOR. Autophagy. (2011) 7(6):643–4. doi: 10.4161/auto.7.6.15123
87. Matsui Y, Takagi H, Qu X, Abdellatif M, Sakoda H, Asano T, et al. Distinct roles of autophagy in the heart during ischemia and reperfusion: roles of AMP-activated protein kinase and beclin 1 in mediating autophagy. Circ Res. (2007) 100(6):914–22. doi: 10.1161/01.RES.0000261924.76669.36
88. Mokhtari B, Badalzadeh R. The potentials of distinct functions of autophagy to be targeted for attenuation of myocardial ischemia/reperfusion injury in preclinical studies: an up-to-date review. J Physiol Biochem. (2021) 77(3):377–404. doi: 10.1007/s13105-021-00824-x
89. Mishra PK, Adameova A, Hill JA, Baines CP, Kang PM, Downey JM, et al. Guidelines for evaluating myocardial cell death. Am J Physiol Heart Circ Physiol. (2019) 317(5):H891–922. doi: 10.1152/ajpheart.00259.2019
90. Mehto S, Jena KK, Nath P, Chauhan S, Kolapalli SP, Das SK, et al. The crohn’s disease risk factor IRGM limits NLRP3 inflammasome activation by impeding its assembly and by mediating its selective autophagy. Mol Cell. (2019) 73(3):429–445.e7. doi: 10.1016/j.molcel.2018.11.018
91. Ma S, Wang Y, Chen Y, Cao F. The role of the autophagy in myocardial ischemia/reperfusion injury. Biochim Biophys Acta. (2015) 1852(2):271–6. doi: 10.1016/j.bbadis.2014.05.010
92. Yu Y-W, Que J-Q, Liu S, Huang KY, Qian L, Weng YB, et al. Sodium-glucose co-transporter-2 inhibitor of dapagliflozin attenuates myocardial ischemia/reperfusion injury by limiting NLRP3 inflammasome activation and modulating autophagy. Front Cardiovasc Med. (2022) 8:768214. doi: 10.3389/fcvm.2021.768214
93. Xiang M, Lu Y, Xin L, Gao J, Shang C, Jiang Z, et al. Role of oxidative stress in reperfusion following myocardial ischemia and its treatments. Oxid Med Cell Longev. (2021) 2021:6614009. doi: 10.1155/2021/6614009
94. Popov SV, Mukhomedzyanov AV, Voronkov NS, Derkachev IA, Boshchenko AA, Fu F, et al. Regulation of autophagy of the heart in ischemia and reperfusion. Apoptosis. (2023) 28(1-2):55–80. doi: 10.1007/s10495-022-01786-1
95. Gottlieb RA, Carreira RS. Autophagy in health and disease. 5. Mitophagy as a way of life. Am J Physiol Cell Physiol. (2010) 299(2):C203–210. doi: 10.1152/ajpcell.00097.2010
96. Zhao J, Zhang J, Liu Q, Wang Y, Jin Y, Yang Y, et al. Hongjingtian injection protects against myocardial ischemia reperfusion-induced apoptosis by blocking ROS induced autophagic- flux. Biomed Pharmacother. (2021) 135:111205. doi: 10.1016/j.biopha.2020.111205
97. Cadenas S. ROS and redox signaling in myocardial ischemia-reperfusion injury and cardioprotection. Free Radic Biol Med. (2018) 117:76–89. doi: 10.1016/j.freeradbiomed.2018.01.024
98. Hariharan N, Zhai P, Sadoshima J. Oxidative stress stimulates autophagic flux during ischemia/reperfusion. Antioxid Redox Signal. (2011) 14(11):2179–90. doi: 10.1089/ars.2010.3488
99. Mokhtari B, Badalzadeh R. Protective and deleterious effects of autophagy in the setting of myocardial ischemia/reperfusion injury: an overview. Mol Biol Rep. (2022) 49(11):11081–99. doi: 10.1007/s11033-022-07837-9
100. Pattingre S, Tassa A, Qu X, Garuti R, Liang XH, Mizushima N, et al. Bcl-2 antiapoptotic proteins inhibit beclin 1-dependent autophagy. Cell. (2005) 122(6):927–39. doi: 10.1016/j.cell.2005.07.002
101. Ma X, Liu H, Foyil SR, Godar RJ, Weinheimer CJ, Hill JA, et al. Impaired autophagosome clearance contributes to cardiomyocyte death in ischemia/reperfusion injury. Circulation. (2012) 125(25):3170–81. doi: 10.1161/CIRCULATIONAHA.111.041814
102. Marinescu M-C, Lazar A-L, Marta MM, Cozma A, Catana C-S. Non-coding RNAs: prevention, diagnosis, and treatment in myocardial ischemia-reperfusion injury. Int J Mol Sci. (2022) 23(5):2728. doi: 10.3390/ijms23052728
103. Poller W, Dimmeler S, Heymans S, Zeller T, Haas J, Karakas M, et al. Non-coding RNAs in cardiovascular diseases: diagnostic and therapeutic perspectives. Eur Heart J. (2018) 39(29):2704–16. doi: 10.1093/eurheartj/ehx165
104. Kabekkodu SP, Shukla V, Varghese VK, D’Souza J, Chakrabarty S, Satyamoorthy K. Clustered miRNAs and their role in biological functions and diseases. Biol Rev Camb Philos Soc. (2018) 93(4):1955–86. doi: 10.1111/brv.12428
105. Bartel DP. MicroRNAs: genomics, biogenesis, mechanism, and function. Cell. (2004) 116(2):281–97. doi: 10.1016/s0092-8674(04)00045-5
106. Li T, Gu J, Yang O, Wang J, Wang Y, Kong J. Bone marrow mesenchymal stem cell-derived exosomal miRNA-29c decreases cardiac ischemia/reperfusion injury through inhibition of excessive autophagy via the PTEN/akt/mTOR signaling pathway. Circ J. (2020) 84(8):1304–11. doi: 10.1253/circj.CJ-19-1060
107. Wu Q, Shang Y, Bai Y, Wu Y, Wang H, Shen T. Sufentanil preconditioning protects against myocardial ischemia/reperfusion injury via miR-125a/DRAM2 axis. Cell Cycle. (2021) 20(4):383–91. doi: 10.1080/15384101.2021.1875668
108. Sun W, Shi R, Guo J, Wang H, Shen L, Shi H, et al. miR-135b-3p promotes cardiomyocyte ferroptosis by targeting GPX4 and aggravates myocardial ischemia/reperfusion injury. Front Cardiovasc Med. (2021) 8:663832. doi: 10.3389/fcvm.2021.663832
109. Zhu WS, Guo W, Zhu JN, Tang CM, Fu YH, Lin QX, et al. Hsp90aa1: a novel target gene of miR-1 in cardiac ischemia/reperfusion injury. Sci Rep. (2016) 6(1):24498. doi: 10.1038/srep24498
110. Wang W, Zheng Y, Wang M, Yan M, Jiang J, Li Z. Exosomes derived miR-126 attenuates oxidative stress and apoptosis from ischemia and reperfusion injury by targeting ERRFI1. Gene. (2019) 690:75–80. doi: 10.1016/j.gene.2018.12.044
111. Yang D, Wang M, Hu Z, Ma Y, Shi Y, Cao X, et al. Extracorporeal cardiac shock wave-induced exosome derived from endothelial colony-forming cells carrying miR-140-3p alleviate cardiomyocyte hypoxia/reoxygenation injury via the PTEN/PI3K/AKT pathway. Front Cell Dev Biol. (2021) 9:779936. doi: 10.3389/fcell.2021.779936
112. Lu Y, Xi J, Zhang Y, Li C, Chen W, Hu X, et al. MicroRNA-214-5p protects against myocardial ischemia reperfusion injury through targeting the FAS ligand. Arch Med Sci. (2020) 16(5):1119–29. doi: 10.5114/aoms.2019.85405
113. Chen Q, Liu Y, Ding X, Li Q, Qiu F, Wang M, et al. Bone marrow mesenchymal stem cell-secreted exosomes carrying microRNA-125b protect against myocardial ischemia reperfusion injury via targeting SIRT7. Mol Cell Biochem. (2020) 465(1-2):103–14. doi: 10.1007/s11010-019-03671-z
114. Xiao X, Lu Z, Lin V, May A, Shaw DH, Wang Z, et al. MicroRNA miR-24-3p reduces apoptosis and regulates Keap1-Nrf2 pathway in mouse cardiomyocytes responding to ischemia/reperfusion injury. Oxid Med Cell Longev. (2018) 2018:7042105. doi: 10.1155/2018/7042105
115. Liu X-M, Zhang Z, Zhong J, Li N, Wang T, Wang L, et al. Long non-coding RNA MALAT1 modulates myocardial ischemia-reperfusion injury through the PI3K/akt/eNOS pathway by sponging miRNA-133a-3p to target IGF1R expression. Eur J Pharmacol. (2022) 916:174719. doi: 10.1016/j.ejphar.2021.174719
116. Liu N, Xie L, Xiao P, Chen X, Kong W, Lou Q, et al. Cardiac fibroblasts secrete exosome microRNA to suppress cardiomyocyte pyroptosis in myocardial ischemia/reperfusion injury. Mol Cell Biochem. (2022) 477(4):1249–60. doi: 10.1007/s11010-021-04343-7
117. Xu J, Chen X, Nie W. miR-15b-5p regulates the NLRP3 inflammasome signal through targeting SIRT3 to regulate hypoxia/reoxygenation-induced cardiomyocyte pyroptosis process. Shock. (2022) 58(2):147–57. doi: 10.1097/SHK.0000000000001961
118. Li K, Zhou P, Li S, Zheng S, Wang D. MicroRNA-29b reduces myocardial ischemia-reperfusion injury in rats via down-regulating PTEN and activating the akt/eNOS signaling pathway. J Thromb Thrombolysis. (2022) 53(1):123–35. doi: 10.1007/s11239-021-02535-y
119. Gao L, Qiu F, Cao H, Li H, Dai G, Ma T, et al. Therapeutic delivery of microRNA-125a-5p oligonucleotides improves recovery from myocardial ischemia/reperfusion injury in mice and swine. Theranostics. (2023) 13(2):685–703. doi: 10.7150/thno.73568
120. Zhao D, Shun E, Ling F, Liu Q, Warsi A, Wang B, et al. Plk2 regulated by miR-128 induces ischemia-reperfusion injury in cardiac cells. Mol Ther Nucleic Acids. (2020) 19:458–67. doi: 10.1016/j.omtn.2019.11.029
121. Chen B, Zheng L, Zhu T, Jiao K. LncRNA FOXD3-AS1 aggravates myocardial ischemia/reperfusion injury by inactivating the Redd1/AKT/GSK3β/Nrf2 signaling pathway via the miR-128/TXNIP axis. J Biochem Mol Toxicol. (2022) 36(12):e23218. doi: 10.1002/jbt.23218
122. Wan X, Yao B, Ma Y, Liu Y, Tang Y, Hu J, et al. MicroRNA-128-1-5p attenuates myocardial ischemia/reperfusion injury by suppressing Gadd45g-mediated apoptotic signaling. Biochem Biophys Res Commun. (2020) 530(1):314–21. doi: 10.1016/j.bbrc.2020.07.009
123. Dai Z-H, Jiang Z-M, Tu H, Mao L, Song G-L, Yang Z-B, et al. miR-129 attenuates myocardial ischemia reperfusion injury by regulating the expression of PTEN in rats. Biomed Res Int. (2021) 2021:5535788. doi: 10.1155/2021/5535788
124. Zheng S, Wang L, Ma H, Sun F, Wen F. microRNA-129 overexpression in endothelial cell-derived extracellular vesicle influences inflammatory response caused by myocardial ischemia/reperfusion injury. Cell Biol Int. (2021) 45(8):1743–56. doi: 10.1002/cbin.11614
125. Liu S, Liao Q, Xu W, Zhang Z, Yin M, Cao X. MiR-129-5p protects H9c2 cardiac myoblasts from hypoxia/reoxygenation injury by targeting TRPM7 and inhibiting NLRP3 inflammasome activation. J Cardiovasc Pharmacol. (2021) 77(5):586–93. doi: 10.1097/FJC.0000000000000991
126. Chen Z-X, He D, Mo Q-W, Xie L-P, Liang J-R, Liu L, et al. MiR-129-5p protects against myocardial ischemia-reperfusion injury via targeting HMGB1. Eur Rev Med Pharmacol Sci. (2020) 24(8):4440–50. doi: 10.26355/eurrev_202004_21026
127. Huang C, Qu Y, Feng F, Zhang H, Shu L, Zhu X, et al. Cardioprotective effect of circ_SMG6 knockdown against myocardial ischemia/reperfusion injury correlates with miR-138-5p-mediated EGR1/TLR4/TRIF inactivation. Oxid Med Cell Longev. (2022) 2022:1927260. doi: 10.1155/2022/1927260
128. Chang Y, Xing L, Zhou W, Zhang W. Up-regulating microRNA-138-5p enhances the protective role of dexmedetomidine on myocardial ischemia-reperfusion injury mice via down-regulating Ltb4r1. Cell Cycle. (2021) 20(4):445–58. doi: 10.1080/15384101.2021.1878330
129. Yang S, Li H, Chen L. MicroRNA-140 attenuates myocardial ischemia-reperfusion injury through suppressing mitochondria-mediated apoptosis by targeting YES1. J Cell Biochem. (2019) 120(3):3813–21. doi: 10.1002/jcb.27663
130. Ge X, Meng Q, Wei L, Liu J, Li M, Liang X, et al. Myocardial ischemia-reperfusion induced cardiac extracellular vesicles harbour proinflammatory features and aggravate heart injury. J Extracell Vesicles. (2021) 10(4):e12072. doi: 10.1002/jev2.12072
131. Hu C, Liao J, Huang R, Su Q, He L. MicroRNA-155-5p in serum derived-exosomes promotes ischaemia-reperfusion injury by reducing CypD ubiquitination by NEDD4. ESC Heart Fail. (2023) 10(2):1144–57. doi: 10.1002/ehf2.14279
132. Yan X, Huang Y. Mechanism of total glucosides of paeony in hypoxia/reoxygenation-induced cardiomyocyte pyroptosis. J Bioenerg Biomembr. (2021) 53(6):643–53. doi: 10.1007/s10863-021-09921-4
133. Li X, Jin Y. Inhibition of miR-182-5p attenuates ROS and protects against myocardial ischemia-reperfusion injury by targeting STK17A. Cell Cycle. (2022) 21(15):1639–50. doi: 10.1080/15384101.2022.2060640
134. Yuan J, Fu X. MicroRNA-21 mediates the protective role of emulsified isoflurane against myocardial ischemia/reperfusion injury in mice by targeting SPP1. Cell Signal. (2021) 86:110086. doi: 10.1016/j.cellsig.2021.110086
135. Han J, Zhang Z, Zhang Z, Yang S. Artemisinin relieves myocardial ischemia-reperfusion injury via modulating miR-29b-3p and hemicentin 1. Front Pharmacol. (2022) 13:918966. doi: 10.3389/fphar.2022.918966
136. Wang K, An T, Zhou L-Y, Liu C-Y, Zhang X-J, Feng C, et al. E2F1-regulated miR-30b suppresses cyclophilin D and protects heart from ischemia/reperfusion injury and necrotic cell death. Cell Death Differ. (2015) 22(5):743–54. doi: 10.1038/cdd.2014.165
137. Bei Y, Lu D, Bär C, Chatterjee S, Costa A, Riedel I, et al. miR-486 attenuates cardiac ischemia/reperfusion injury and mediates the beneficial effect of exercise for myocardial protection. Mol Ther. (2022) 30(4):1675–91. doi: 10.1016/j.ymthe.2022.01.031
138. Bøtker HE, Kharbanda R, Schmidt MR, Bøttcher M, Kaltoft AK, Terkelsen CJ, et al. Remote ischaemic conditioning before hospital admission, as a complement to angioplasty, and effect on myocardial salvage in patients with acute myocardial infarction: a randomised trial. Lancet. (2010) 375(9716):727–34. doi: 10.1016/S0140-6736(09)62001-8
139. Ma L-L, Kong F-J, Dong Z, Xin K-Y, Wang X-X, Sun A-J, et al. Hypertrophic preconditioning attenuates myocardial ischaemia-reperfusion injury by modulating SIRT3-SOD2-mROS-dependent autophagy. Cell Prolif. (2021) 54(7):e13051. doi: 10.1111/cpr.13051
140. Stiermaier T, Jensen J-O, Rommel K-P, de Waha-Thiele S, Fuernau G, Desch S, et al. Combined intrahospital remote ischemic perconditioning and postconditioning improves clinical outcome in ST-elevation myocardial infarction. Circ Res. (2019) 124(10):1482–91. doi: 10.1161/CIRCRESAHA.118.314500
141. Zhao Z, Shi Q, Guo Q, Peng L, Li X, Rao L, et al. Remote ischemic preconditioning can extend the tolerance to extended drug-coated balloon inflation time by reducing myocardial damage during percutaneous coronary intervention. Int J Cardiol. (2022) 353:3–8. doi: 10.1016/j.ijcard.2022.01.049
142. Basili S, Tanzilli G, Raparelli V, Calvieri C, Pignatelli P, Carnevale R, et al. Aspirin reload before elective percutaneous coronary intervention: impact on serum thromboxane b2 and myocardial reperfusion indexes. Circ Cardiovasc Interv. (2014) 7(4):577–84. doi: 10.1161/CIRCINTERVENTIONS.113.001197
143. Ye Y, Birnbaum GD, Perez-Polo JR, Nanhwan MK, Nylander S, Birnbaum Y. Ticagrelor protects the heart against reperfusion injury and improves remodeling after myocardial infarction. Arterioscler Thromb Vasc Biol. (2015) 35(8):1805–14. doi: 10.1161/ATVBAHA.115.305655
144. Sayour AA, Celeng C, Oláh A, Ruppert M, Merkely B, Radovits T. Sodium-glucose cotransporter 2 inhibitors reduce myocardial infarct size in preclinical animal models of myocardial ischaemia-reperfusion injury: a meta-analysis. Diabetologia. (2021) 64(4):737–48. doi: 10.1007/s00125-020-05359-2
145. Lim VG, Bell RM, Arjun S, Kolatsi-Joannou M, Long DA, Yellon DM. SGLT2 inhibitor, canagliflozin, attenuates myocardial infarction in the diabetic and nondiabetic heart. JACC Basic Transl Sci. (2019) 4(1):15–26. doi: 10.1016/j.jacbts.2018.10.002
146. Nikolaou PE, Mylonas N, Makridakis M, Makrecka-Kuka M, Iliou A, Zerikiotis S, et al. Cardioprotection by selective SGLT-2 inhibitors in a non-diabetic mouse model of myocardial ischemia/reperfusion injury: a class or a drug effect? Basic Res Cardiol. (2022) 117(1):27. doi: 10.1007/s00395-022-00934-7
147. Andreadou I, Bell RM, Bøtker HE, Zuurbier CJ. SGLT2 Inhibitors reduce infarct size in reperfused ischemic heart and improve cardiac function during ischemic episodes in preclinical models. Biochim Biophys Acta Mol Basis Dis. (2020) 1866(7):165770. doi: 10.1016/j.bbadis.2020.165770
148. Zhang F, Cao X, Zhao C, Chen L, Chen X. Empagliflozin activates JAK2/STAT3 signaling and protects cardiomyocytes from hypoxia/reoxygenation injury under high glucose conditions. J Thromb Thrombolysis. (2022) 17:116–25. doi: 10.1007/s11239-022-02719-0
149. Pearce L, Carr RD, Yellon DM, Davidson SM. Nicorandil - an effective multitarget drug for cardioprotection? Cardiovasc Drugs Ther. (2023) 37(1):5–8. doi: 10.1007/s10557-022-07397-x
150. Peng Y-W, Major T, Deatrick KB, Mohammed A, Jeakle M, Charpie JR. Nicorandil attenuates ventricular dysfunction and organ injury after cardiopulmonary bypass. Int J Cardiol. (2022) 368:62–8. doi: 10.1016/j.ijcard.2022.08.030
151. Qian G, Zhang Y, Dong W, Jiang ZC, Li T, Cheng LQ, et al. Effects of nicorandil administration on infarct size in patients with ST-segment-elevation myocardial infarction undergoing primary percutaneous coronary intervention: the CHANGE trial. J Am Heart Assoc. (2022) 11(18):e026232. doi: 10.1161/JAHA.122.026232
152. Cai Y, Zhang B, Shalamu A, Gao T, Ge J. Soluble guanylate cyclase (sGC) stimulator vericiguat alleviates myocardial ischemia-reperfusion injury by improving microcirculation. Ann Transl Med. (2022) 10(12):662. doi: 10.21037/atm-22-2583
153. Chen J, Huang Q, Li J, Yao Y, Sun W, Zhang Z, et al. Panax ginseng against myocardial ischemia/reperfusion injury: a review of preclinical evidence and potential mechanisms. J Ethnopharmacol. (2023) 300:115715. doi: 10.1016/j.jep.2022.115715
154. Dong L, Shen Z, Chi H, Wang Y, Shi Z, Fang H, et al. Research progress of Chinese medicine in the treatment of myocardial ischemia-reperfusion injury. Am J Chin Med. (2023) 51(1):1–17. doi: 10.1142/S0192415X23500015
155. Li T, Tan Y, Ouyang S, He J, Liu L. Resveratrol protects against myocardial ischemia-reperfusion injury via attenuating ferroptosis. Gene. (2022) 808:145968. doi: 10.1016/j.gene.2021.145968
156. Yang Y, Shao M, Yao J, Yang S, Cheng W, Ma L, et al. Neocryptotanshinone protects against myocardial ischemia-reperfusion injury by promoting autolysosome degradation of protein aggregates via the ERK1/2-Nrf2-LAMP2 pathway. Phytomedicine. (2023) 110:154625. doi: 10.1016/j.phymed.2022.154625
157. Gao J, Wang Z, Ye Z. Madder (Rubia cordifolia L.) alleviates myocardial ischemia-reperfusion injury by protecting endothelial cells from apoptosis and inflammation. Mediators Inflamm. (2023) 2023:5015039. doi: 10.1155/2023/5015039
158. Wang R, Wang M, Zhou J, Dai Z, Sun G, Sun X. Calenduloside E suppresses calcium overload by promoting the interaction between L-type calcium channels and Bcl2-associated athanogene 3 to alleviate myocardial ischemia/reperfusion injury. J Adv Res. (2021) 34:173–86. doi: 10.1016/j.jare.2020.10.005
159. Lv D, Luo M, Cheng Z, Wang R, Yang X, Guo Y, et al. Tubeimoside I ameliorates myocardial ischemia-reperfusion injury through SIRT3-dependent regulation of oxidative stress and apoptosis. Oxid Med Cell Longev. (2021) 2021:5577019. doi: 10.1155/2021/5577019
160. Wang J, Liu Y, Liu Y, Huang H, Roy S, Song Z, et al. Recent advances in nanomedicines for imaging and therapy of myocardial ischemia-reperfusion injury. J Controlled Release. (2023) 353:563–90. doi: 10.1016/j.jconrel.2022.11.057
161. Chen J, Song Y, Wang Q, Li Q, Tan H, Gao J, et al. Targeted neutrophil-mimetic liposomes promote cardiac repair by adsorbing proinflammatory cytokines and regulating the immune microenvironment. J Nanobiotechnology. (2022) 20(1):218. doi: 10.1186/s12951-022-01433-6
162. Mukhamadiyarov RA, Senokosova EA, Krutitsky SS, Voevoda DV, Pyshnaya IA, Ivanov VV, et al. Size-dependent ability of liposomes to accumulate in the ischemic myocardium and protect the heart. J Cardiovasc Pharmacol. (2018) 72(3):143–52. doi: 10.1097/FJC.0000000000000606
163. Weng X, Tan H, Huang Z, Chen J, Zhang N, Wang Q, et al. Targeted delivery and ROS-responsive release of resolvin D1 by platelet chimeric liposome ameliorates myocardial ischemia-reperfusion injury. J Nanobiotechnology. (2022) 20(1):454. doi: 10.1186/s12951-022-01652-x
164. Huang C, Zhou S, Chen C, Wang X, Ding R, Xu Y, et al. Biodegradable redox-responsive AIEgen-based-covalent organic framework nanocarriers for long-term treatment of myocardial ischemia/reperfusion injury. Small. (2022) 18(47):e2205062. doi: 10.1002/smll.202205062
165. Wei Y, Zhu M, Li S, Hong T, Guo X, Li Y, et al. Engineered biomimetic nanoplatform protects the myocardium against ischemia/reperfusion injury by inhibiting pyroptosis. ACS Appl Mater Interfaces. (2021) 13(29):33756–66. doi: 10.1021/acsami.1c03421
166. Sharma AK, Kumar A, Sahu M, Sharma G, Datusalia AK, Rajput SK. Exercise preconditioning and low dose copper nanoparticles exhibits cardioprotection through targeting GSK-3β phosphorylation in ischemia/reperfusion induced myocardial infarction. Microvasc Res. (2018) 120:59–66. doi: 10.1016/j.mvr.2018.06.003
167. Gao L, Qiu F, Cao H, Li H, Dai G, Ma T, et al. Therapeutic delivery of microRNA-125a-5p oligonucleotides improves recovery from myocardial ischemia/reperfusion injury in mice and swine. Theranostics. (2023) 13(2):685–703. doi: 10.7150/thno.73568
168. Majka M, Kleibert M, Wojciechowska M. Impact of the main cardiovascular risk factors on plasma extracellular vesicles and their influence on the heart’s vulnerability to ischemia-reperfusion injury. Cells. (2021) 10(12):3331. doi: 10.3390/cells10123331
169. Yu S, Tang X, Zheng T, Li S, Ren H, Wu H, et al. Plasma-derived extracellular vesicles transfer microRNA-130a-3p to alleviate myocardial ischemia/reperfusion injury by targeting ATG16L1. Cell Tissue Res. (2022) 389(1):99–114. doi: 10.1007/s00441-022-03605-0
170. Zhou T, Yang X, Wang T, Xu M, Huang Z, Yu R, et al. Platelet-membrane-encapsulated carvedilol with improved targeting ability for relieving myocardial ischemia-reperfusion injury. Membranes. (2022) 12(6):605. doi: 10.3390/membranes12060605
171. Asgarpour K, Shojaei Z, Amiri F, Ai J, Mahjoubin-Tehran M, Ghasemi F, et al. Exosomal microRNAs derived from mesenchymal stem cells: cell-to-cell messages. Cell Commun Signal. (2020) 18(1):149. doi: 10.1186/s12964-020-00650-6
Keywords: myocardial ischemia/reperfusion injury, reperfusion therapy, myocardial infarction, mechanism, multi-target therapy, bibliometric analysis
Citation: Wang Y, Guo L, Zhang Z, Fu S, Huang P, Wang A, Liu M and Ma X (2023) A bibliometric analysis of myocardial ischemia/reperfusion injury from 2000 to 2023. Front. Cardiovasc. Med. 10:1180792. doi: 10.3389/fcvm.2023.1180792
Received: 6 March 2023; Accepted: 22 May 2023;
Published: 13 June 2023.
Edited by:
Tommaso Gori, Johannes Gutenberg University Mainz, GermanyReviewed by:
Peng Zhu, Southern Medical University, ChinaYang Yang, First Affiliated Hospital of Zhengzhou University, China
© 2023 Wang, Guo, Zhang, Fu, Huang, Wang, Liu and Ma. This is an open-access article distributed under the terms of the Creative Commons Attribution License (CC BY). The use, distribution or reproduction in other forums is permitted, provided the original author(s) and the copyright owner(s) are credited and that the original publication in this journal is cited, in accordance with accepted academic practice. No use, distribution or reproduction is permitted which does not comply with these terms.
*Correspondence: Xiaochang Ma maxiaochang@x263.net Mi Liu liumi177@qq.com