- 1Liverpool Centre for Cardiovascular Science at University of Liverpool, Liverpool John Moores University and Liverpool Heart & Chest Hospital, United Kingdom
- 2Department of Cardiovascular and Metabolic Medicine, Institute of Life Course and Medical Sciences, Faculty of Health and Life Sciences, University of Liverpool, Liverpool, United Kingdom
- 3Danish Center for Clinical Health Services Research, Department of Clinical Medicine, Aalborg University, Aalborg, Denmark
- 4Department of Pharmacology and Therapeutics, Institute of Systems, Molecular and Integrative Biology, Faculty of Health and Life Sciences, University of Liverpool, Liverpool, United Kingdom
Atrial fibrillation (AF) is the most common form of cardiac arrhythmia, affecting 2%–3% of the world's population. Mental and emotional stress, as well as some mental health conditions (e.g., depression) have been shown to significantly impact the heart and have been suggested to act both as independent risk factors and triggers in the onset of AF. In this paper, we review the current literature to examine the role that mental and emotional stress have in the onset of AF and summarise the current knowledge on the interaction between the brain and heart, and the cortical and subcortical pathways involved in the response to stress. Review of the evidence suggests that mental and emotional stress negatively affect the cardiac system, potentially increasing the risk for developing and/or triggering AF. Further studies are required to further understand the cortical and sub-cortical structures involved in the mental stress response and how these interact with the cardiac system, which may help in defining new strategies and interventions to prevent the development of, and improve the management of AF.
Introduction
Atrial fibrillation (AF) is the most common arrhythmia, affecting 2%–3% of the population globally, is characterized by the presence of a rapid and irregular beating of the heart's atrial chambers (1). Common risk factors related to incident AF are hypertension, excessive/binge alcohol consumption and smoking, and the presence of other conditions such obesity, chronic obstructive pulmonary disease (COPD), coronary artery disease, congenital heart disease, sleep apnea, diabetes mellitus and thyrotoxicosis (2–5). The current management of AF has moved towards toward more holistic or integrated care approach, formulated as the ABC (Atrial Fibrillation Better Care) pathway (6), following appropriate evaluation and characterisation of the AF (7). This holistic approach is recommended in international guidelines (8).
Mental stress is an epidemic in today's society, with more than 74% of people reporting to be overwhelmed or unable to cope with daily life (9). Moreover, the recent COVID-19 pandemic has further affected the mental wellbeing of the general population (10). Psychological factors such as stress and mental disorders can affect cardiac arrhythmias and AF due to neuroendocrine (hypothalamic-pituitary-adrenal axis) and nervous (autonomic nervous system) system responses (11–14). Excessive stress can affect both frequency of arrhythmias as well as the impact of ventricular fibrillation (14). Mental health disorders may also play a role in the onset of cardiac arrhythmias (13, 15, 16).
Anxiety has been proposed as a possible trigger of cardiac arrhythmias including AF due to increased sympathetic tone and reduction of vagal tone (16). Interestingly, depression has been linked to increased risk of ventricular fibrillation and thus of sudden cardiac death (SCD), especially in patients with coronary artery disease (15). In a systematic review examining the prevalence of depression and anxiety in patients with AF, results showed that one third of AF patients have high level of depression and anxiety, negatively affect their overall quality of life (17).
The objective of this review is to summarise the current understanding on the pathophysiological brain-heart interaction, focusing on the role that psychological factors (such as negative emotions or mental disorders—e.g., depression) have on the development and progression of AF.
Atrial fibrillation and mental stress
Mental stress has negative effects on the heart and on the cardiovascular system in general (18, 19). Episodes of acute stress increase blood pressure (20), and recurrent episodes (chronic stress) may induce damage to the endothelium which increases the risk for cardiovascular events such as stroke and myocardial infarction (21). The likelihood of incident cardiac arrhythmias is strongly associated with abnormalities in electrical repolarization of the heart, where mental stress plays a role in influencing the electrical activity (22), causing increased heart rate, decreased PR and QT interval, and a prolonged QTc interval.
The role that mental stress has in altering left atrial electrophysiology (P-wave axis), a known marker of AF risk, has been observed in two studies (23, 24). In both these pre-post studies in patients with stable coronary heart disease, acute stress (administered via the speech tasks test) was associated with the development of abnormal P-wave axis (Table 1) (23, 24). Although these studies did not assess the occurrence of AF, the ECG changes during mental stress may represent a hallmark for stress-induced alteration in the left atrial electrophysiology which may trigger AF.
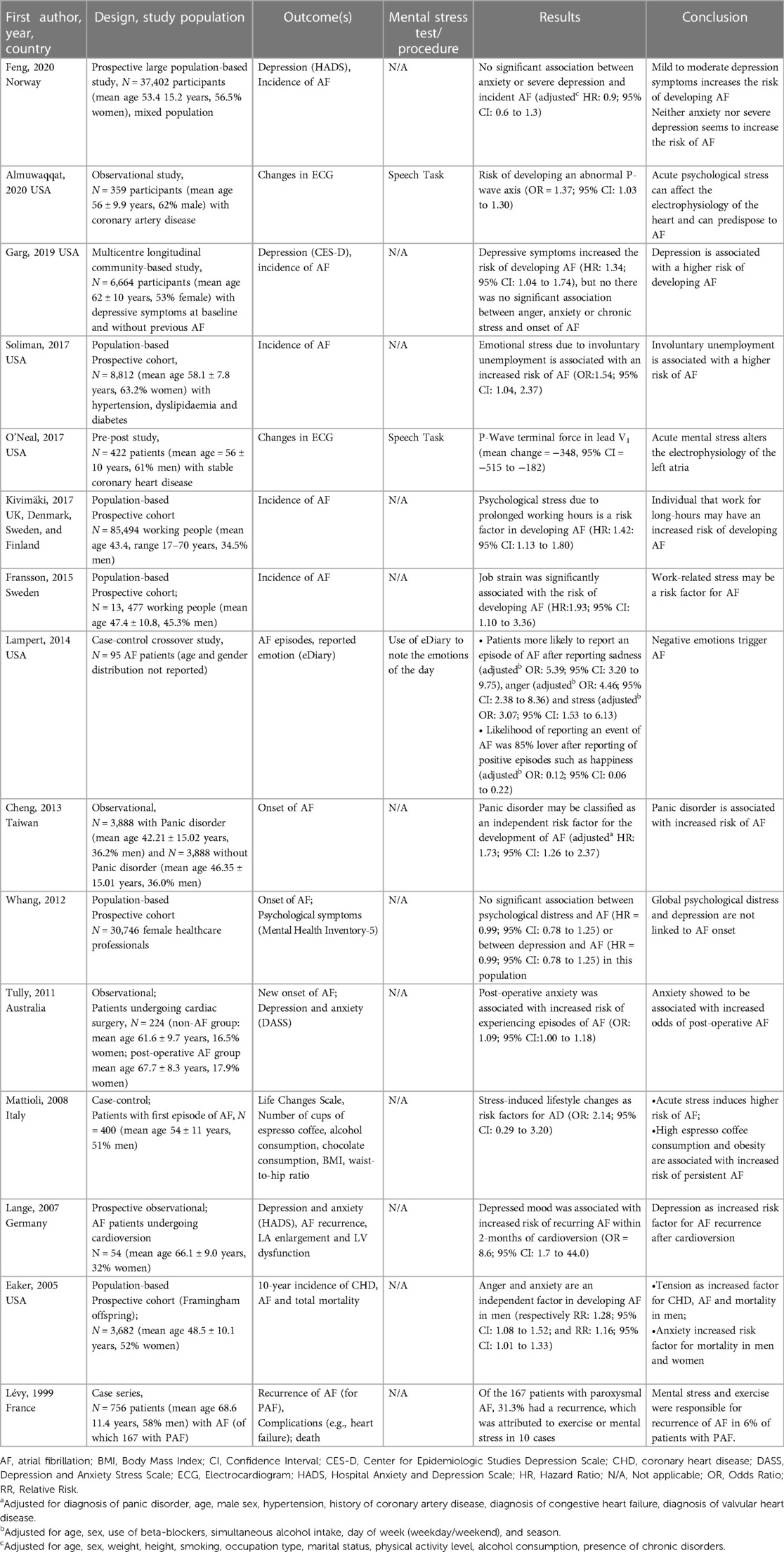
Table 1. Summary of studies investigating the role of mental stress and emotions on atrial fibrillation.
Two additional studies (25, 26) have investigated the role that mental stress has in triggering recurrent AF. Of particular relevance is a cross-over study (25) that investigated the role of negative emotions in triggering AF in a cohort of 95 patients with intermittent-persistent or paroxysmal AF, and provided patients with 24 h Holter-monitoring and an electronic diary to report the experience of positive and negative emotions daily for one year. The results showed that negative emotions are a trigger for AF, with most patients more likely to experience an episode of AF after reporting sadness, anger and stress; while the likelihood of an AF event was 85% lower after reporting of positive emotions, such happiness. Acute stress has also been identified as trigger for the recurrence of AF (26), in a prospective study of 756 patients with AF (of which 167 had paroxysmal AF) with a mean follow-up of 8.6(±3.6) months. Of the 167 patients with paroxysmal AF, 31.3% had a recurrence, which was attributed to exercise or mental stress in 10 cases.
Mental stress, mental exhaustion and negative emotions have been identified as risk factor for recurrent AF (Table 1) (27–31). A case-control study (28) reported that a high level of acute stress induces lifestyle changes (e.g., consumption of more coffee) and was identified as a risk factor for AF. Job strain (30, 31) (a measure of work stress) and prolonged working hours (29) have been both identified as risk factors for AF. Additionally, the stress due to involuntary unemployment has also been associated with an increased risk of AF occurrence (32). However, a prospective cohort study (32–33) observing female health professionals showed no significant association between psychological distress and AF in this population. Moreover, anger and anxiety have been reported as independent risk factors for AF (34–36), especially for men. However, a retrospective study (36) on a total of 3,888 patients with panic disorder and without a diagnosis of AF found no significant association between anger, anxiety or chronic stress and onset of AF, however, depressive symptoms increased the risk of developing AF. Depression has also been associated with recurring AF in another prospective cohort study (37) observing 54 patients with persistent AF undergoing electrical cardioversion, but not for incident AF (38, 39).
The quality of studies examining the relationship between mental stress and incident AF or recurrent AF varied markedly, in the quantification of mental stress (using different scales/tests), varying sample sizes, and most studies were observational in nature, with varying lengths of follow-up. To date, only one study (25) has investigated negative emotions as a trigger of episodes of AF in a crossover study design. Therefore, more studies are needed to further understand the relationship between emotions, mental stress and AF, especially the role these factors have in triggering AF. Stress biomarkers (e.g., catecholamines) and ECG readings in response to acute stress, as well as the standardisation of scales and questionnaires to assess chronic stress in this population should be assessed in large clinical trials investigating the role that emotions and stress (both acute and chronic) have in triggering AF.
Role of the immune response
It is well known that the body stress response triggers inflammatory mechanism (e.g., increasing of circulating inflammatory cytokines) (40). Chronic stress has been associated with an increased likelihood of developing cardiovascular diseases (e.g., coronary heart disease, stroke) (41), due to the inflammatory response that it activates in the body (40, 42). This is also true for the development of arrhythmias, with the later also promoting inflammation itself, leading to a cycling interaction between inflammation and disease (43). Several studies (44–47) have associated AF with increased plasma interleukins (IL-6; IL-1ϐ), suggesting the role of inflammation in the developing of AF after cardiac surgery. The role of neuroinflammation (e.g., inflammation of the brain areas related to sympathetic output) in the developing of neurogenic hypertension (48) validated further the role that inflammation has in increasing risks factors for AF. Furthermore, the increased peripheral cytokines release (such as TNF-ɑ) after acute myocardial infarction (which is commonly followed by the onset of AF), induces an increased permeability of the Blood Brain Barrier and a long persisting neuroinflammatory status that emphasize the relationship between stress, inflammation and onset of AF (49).
Heart-brain interactions
Autonomic and neurohumoral control of cardiovascular function is under the control of the central nervous system (50). Heart-brain interactions have a number of manifestations, such as the so-called “stroke-heart” syndrome, where neurological deficits induced by an ischaemic stroke affect the cardiovascular system (51). For example, a recent retrospective cohort study showed that following stroke, new-onset cardiovascular complications are very common and associate with a worsening prognosis of major adverse cardiovascular events (52).
The interaction between brain and heart in terms of cerebral influence on cardiac output has been investigated in several studies, which have focused on underlying pathophysiological processes (50, 53–72). Cerebral damage (e.g., following stroke) has been linked to the occurrence of myocardial infarction and arrhythmias, which suggests a major role of the central nervous system in regulating cardiac functions (50, 55). In recent years, the link between the heart and brain after stroke has been further described in the already mentioned “stroke-heart syndrome” (51), which induces autonomic dysfunctions resulting in reduced heart rate variability and impaired baroceptor reflex sensitivity. This post-stroke induced autonomic dysfunction is exaggerated during sleep (59). Effects of haemorrhagic stroke (i.e., subsequent subarachnoid haemorrhage) causes ECG changes such as ST-elevation or depression and QT prolongation (56). These changes have been linked to increased risk of developing AF following haemorrhagic stroke (50, 64). Dysfunction in any cortical or subcortical brain system may lead to pathological changes in the cardiovascular system (50) and cardiac abnormalities are associated with multiple neurological disorders other than stroke and haemorrhage, including brain (62, 63) and spinal cord injury (58, 65), epilepsy (66, 67, 70, 72), neurodegenerative diseases (57, 60, 68), migraine (53, 61), and sleep disorders (54, 71). It is now evident that cortical and subcortical areas of the brain interact with peripheral structures (e.g., adrenal glands) that activate a series of physiological responses which ultimately affect the heart (69) (Figure 1).
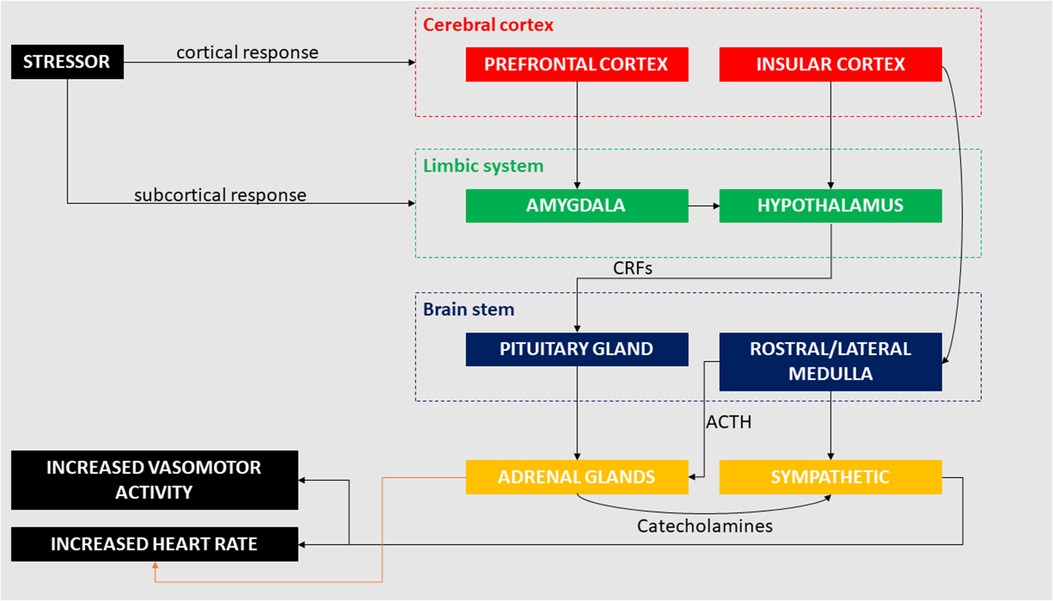
Figure 1. Cortical and subcortical pathways involved in the cardiac response to mental and emotional stress. Stressors (e.g., negative emotions, job strain) can activate conscious response through the pre-frontal cortex as well as unconscious response through the amygdala, both inducing changes in cardiac activity.
The role of neurotrophins (e.g., Brain-Derived Neurotrophic Factor—BDNF) in the brain health and development have been largely investigated (73). Several studies have also reported the crucial role of neurotrophins in non-neuronal cells (74–76), and their effects on the neuronal regulation of heart functions (76). BDNF has shown to have a protective role on the health of the heart, promoting angiogenesis and neovascularization of ischemic tissue through the recruitment of endothelial cells and by regulating their survival (77), with high serum level of BDNF being associated with a lower risk of cardiovascular disease (78).
Areas of the brain underlying individual perception of emotional stress include a network of cortical areas and subcortical nuclei. Subcortical networks have long been acknowledged to regulate stress and influence the cardiovascular response and more recently it has become apparent that the cardiovascular system is in part regulated by modulation of the cerebral cortex (79). At the cortical level, mental and emotional stress is principally regulated by the prefrontal cortex (PFC) and insular cortex.
The PFC has wide-ranging important roles in many aspects of higher order cognitive and affective functions given its extensive neural connections with other cortical and subcortical brain regions. These functions include, but are not restricted to, memory and language functioning, emotional processing, behavioural inhibition, social behaviour, personality expression, decision making, modulation of autonomic responses, and sensory integration, amongst others (80–88).
During a stressful event, the medial and orbital PFC (and cingulate cortex) plays a role in moderating the stress response via connection with limbic structures (in particular, the hippocampus and amygdala) that are involved in the neuroendocrine response to stress (89), and which may affect acute and chronic-stress induced cardiovascular response (90). Both acute and chronic stress directly impact on brain structure and function.
Acute mild stress can cause impairment of cognitive abilities mediated by the PFC and sustained chronic emotional stress may lead to damage to prefrontal neurons (91). Unlike other brain regions, even short periods of emotional stress can cause architectural changes to prefrontal neurons (92, 93). High levels of catecholamine release during stress have a deleterious impact on PFC functioning and strengthens the emotional and habitual responses of the amygdala and basal ganglia (94). Patients with heart failure have reduced grey matter density of the medial prefrontal cortex (amongst other regions), which correlates with N-terminal prohormone of brain natriuretic peptide—a biomarker of heart failure (95). Young patients with congenital heart defects have reduced volumes of the lateral, medial, and orbital PFC and concomitant cognitive deficits (96). Patients with vascular risk factors but without clinically manifest cardiovascular or cerebrovascular disease or events show evidence of prefrontal (and other regional) brain abnormalities (96). Resting-state functional MRI work has reported that temporal changes of heart rate variability are correlated with dynamic changes of PFC connectivity and that heart rate variability biofeedback leads to a drop in heart rate and concomitant increase in functional connectivity between PFC and amygdala, insula, and cingulate cortex (97). Animal studies have revealed that emotionally stressed animals with knockdown levels of glutamatergic packaging in prefrontal interneurons have increased heart rate and mean arterial pressure reactivity relative to unstressed controls (90). Further studies on animal models have highlighted the negative role that psychosocial stress has in deteriorating both cardiac structure and function in arrhythmogenic cardiopathy mice, showing an increased risk of sudden death (98).
The insular cortex shares reciprocal neural connections with PFC, amygdala and other limbic areas, acting as a hub linking large-scale brain networks, and has important roles in various sensory, emotional, motivational, and cognitive functions (99–101). The insular cortex also plays an important role in the central control of cardiac functions as it acts directly on the autonomic nervous system (103, 104), and is consistently implicated in stress-related social and anxiety disorders (105, 106). Recent work has demonstrated a site-specific regulation of cardiovascular stress response along the rostro-caudal axis of the insular cortex (107). There is also accumulating evidence indicating that acute stress impacts on the normal organisation of resting-state functional brain networks (108, 109), which include prefrontal and insular hubs, and which may serve to enable efficient coping (108). Moreover, given that the insula is proximal to the middle cerebral arteries, it is exposed to higher risk of cerebrovascular disease: insula damage typically from stroke has been associated with a multitude of cardiac complications including arrhythmia, diurnal blood pressure variation, myocardial injury, and increased brain natriuretic peptide, catecholamine, and glucose (79, 110). A significantly higher prevalence of previously undiagnosed AF is associated with stroke that impacts the insula compared to stroke that spares the insular cortex (111) and acute stroke of the insula can lead to heart failure (112). Heart rate and blood pressure changes have been reported in response to human insular stimulation (113) and there is a correlation between insula (and extra-insular) activity recorded using functional neuroimaging and heart rate variability (114–116).
Subcortical regions regulating the stress response include components of the limbic system proper and peri-limbic areas, especially including the amygdala, hippocampus and hypothalamus, and brainstem regions, including the peri-aqueductal grey (117). Subcortical pathways that involve the limbic system may regulate the body's response to mental and emotional stress, with and without cortical interaction. The amygdala and hypothalamic-pituitary-adrenal (HPA) axis play important roles in regulating the neuroendocrine response to stress (118) and can trigger the stress-mediated response without cortical initiation (89). Under a stressful situation, the amygdala sends distress signals to the hypothalamus which, through the release of corticotropin-releasing factors (CRFs), induces the pituitary gland to release adrenocorticotropic hormone (ACTH) into the bloodstream. ACTH targets the adrenal glands, which release cortisol and catecholamines in response (119). The increased level of catecholamines in the blood stream induces a series of cardiovascular effects that include increased heart rate, peripheral vasoconstriction and increased cardiac output, affecting in general the sympathetic nervous system (120). Increased adrenergic activity has been observed in the minutes preceding AF (121). Effects induced by catecholamines on the heart affect the sinoatrial node (SAN), the pacemaker of the heart, shortening the diastole and thus increasing the heart rate (122). The influence of catecholamines in promoting atrial arrhythmic activity may have a role in the onset of cardiac arrhythmias (122). Amygdala resting activity assessed using 18F-fluorodexoyglucose PET, a marker of neural glucose metabolism, was reported to be significantly related to bone-marrow activity, arterial inflammation and risk of cardiovascular disease events (123). In the same study, perceived stress was associated with amygdala resting glucose metabolism (123). In a separate 18F-fluorodexoyglucose PET study, amygdala activity was associated with major adverse cardiac events and lower socioeconomic status, further suggesting a strong link between social stress, regional brain function, and cardiac pathology (124). MRI studies have also indicated abnormal structural and functional limbic (e.g., amygdala hyperactivity, hippocampal atrophy) findings in people exposed to chronic stress (125, 126). Hippocampal atrophy has been reported in patients with heart failure (95, 127) and stroke-free patients with AF (128). Other work has reported significantly reduced total brain volume and lower memory scores in older non-demented patients with AF (129–131), particularly in those with an increasing burden of arrhythmia (129). Moreover, brain MRI perfusion studies reveal that persistent AF decreases blood flow to the brain and perfusion of brain tissue (132).
Furthermore, the interactions between the central and peripheral nervous system in response to stress, and how it affects the cardiovascular system are highly relevant. The autonomic nervous system (ANS) is a component of the peripheral nervous system designated to regulate involuntary physiological responses (e.g., heart rate; digestion) (133). It is further divided in sympathetic (SNP—which regulates the “fight or flight” response), parasympathetic (PNS—which promotes a more generic rest status), and enteric (ENS—that regulates the digestion process) (133). Under stress, the increased sympathetic activity influences the heart response, including acceleration of the heart rate, reduction of venous capacitance, increasing of cardiac contractility and peripheral vasoconstriction (134). Negative emotions cause a release of catecholamine, which increases sympathetic tone and decreases parasympathetic tone (135). In AF, the arrhythmia can be triggered by both sympathetic and parasympathetic activation (136), where patients with structurally normal hearts are more likely to show AF following vagal activation (e.g., post-exercise AF), and patients with structural heart disease more likely to show AF after sympathetic activation (e.g., during exercise) (137). In some cardiovascular conditions sympathetic activation can trigger ventricular arrhythmia and sudden cardiac death (138). Sympathetic hyper-innervation (nerve spouting) has been linked to ventricular tachycardia and ventricular fibrillation in animal models (139).
Undoubtedly, an elevated sympathetic output is the final effector of the emotional response. Its' integrated cortical and subcortical control is complex, involving the nucleus solitarius, hypothalamus and rostral ventrolateral medulla (140, 141). Of note, two relevant features of this emotional response pathway have been described: first, the activation of the sympathetic system can be triggered by external stimuli through the amygdala and hypothalamus without involvement of the cerebral cortex (140, 141). Second, the sympathetic baroreflex response is disjointed from the sympathetic activation triggered by emotional stress.
It is also important to note the bidirectional nature of the interaction brain-heart, and how the latter can influence the former, such as inducing behavioural status (e.g., anxiety-related behaviour) following peripheral physiological changes (e.g., increasing in heart rate) (142). Heart failure has shown to increase the risk for cognitive decline (143), mainly due to the induced reduction in the brain perfusion, with decreased cardiac function also changing the level of inflammatory markers in the brain (144). Considering the heart-brain axis (145). dysfunction affecting either the heart or the brain, does reflect on the other organ, creating an interconnected loop.
Can the reduction of mental stress be a preventive strategy for the recurrence of atrial fibrillation?
Psychological interventions, such as cognitive behavioural therapy, have been suggested to mitigate the effects of stress and mental health disorders on cardiovascular conditions (e.g., coronary artery disease) (146, 147). It is clear from accumulating evidence that psychological stress and negative emotions play an important role in the development of cardiac arrhythmias, and more specifically in the onset of AF (14, 16). Despite guidelines highlighting the role that psychological distress has on AF (148), the identification and prevention of mental and emotional stress is not always targeted in clinical practice and its clinical management remains elusive.
Acting on psychological stress factors (e.g., prolonged working hours) and on mental wellbeing (e.g., reduction of anxiety) can potentially reduce the incidence and recurrence of AF and positively impact the overall healthcare costs of this condition (149). Indeed, the major cost driver in the management of AF is due to hospitalization (150). It is then clear than preventing AF and reducing the recurrence of hospitalization may positively impact the healthcare costs. However, interventions that act on the patient's individual response to stress are currently difficult to implement in clinical practice due to the still widespread misconception that stress cannot be systemically identified and measured (151). Suggestions on how to assess both stressor exposure and stress response have been highlighted in the literature (151), and appropriate education needs to be provided to healthcare professionals on the topic. This may help with the design of future studies aiming to investigate the role that psychological interventions may play in the management of AF and may also reduce associated healthcare costs.
Stress reduction is a complex topic that needs to consider societal demands (such as number of working hours (31) or unemployment related stress (32), emotion-driven reactions (35) and the individual level of stress and mental health (17). The additional impact that stress has on the individual's lifestyle also has to be taken into consideration, as it may lead to the increase of other risks factors related to AF (28). From a clinical perspective, the patient's level of stress, as well as their mental health should be ascertained as standard during clinical consultations, with appropriate counselling and stress-reduction interventions such as cognitive behavioural therapy, mindfulness or yoga (152–154), where required. Additionally, the psychological distress of living with chronic conditions (155) should also be taken into consideration and addressed with appropriate educational intervention and directing the patients to discuss their worries with health professionals where needed.
In cases where psychological interventions are needed, their implementation in clinical practice may face several limitations which need to be addressed. Appropriate length and frequency of intervention, as well as the modality of administration (e.g., in person, online) should be properly investigated considering the specific needs of patients with AF. Additionally, the type of intervention should be evaluated and tailored for the specific psychological risk factors (e.g., stress, anxiety, depression). These considerations would need to be investigated in randomised controlled trials before defining appropriate guidelines for healthcare professionals.
Further investigation is also required to better understand the heart-brain interaction. Studies involving brain imaging and cardiac measurements (e.g., ECG) under laboratory-induced mental stress are required that differentiate the cerebral and cardiac response during both mental stress and emotional precipitators (e.g., anger, anxiety).
Future perspectives
Traditionally the role of the autonomic nervous system in the onset of AF has been well acknowledged. Current evidence highlights cases of sympathetic driven AF, in which episodes of arrhythmia develop in response to physical activity or emotional events, and cases of parasympathetic driven AF, in which episodes are triggered by binge eating or drinking, as well chocolate assumption (136). However, the significance of such findings is currently confined to anecdotal evidence and is difficult to translate into clinical practice. As part of the holistic management of AF, attention to psychological morbidity associated with AF is also needed, as suggested by the Atrial Fibrillation Better Care pathway (6, 156). Appropriate recognition by the cardiology community of the role that psychological factors play in the onset and progression of AF should be highlighted to promote assessment of these factors during clinical consultations and to provide empirical evidence for their impact on the management of patients with AF.
Further research should address the current lack of mental health screening in the routine clinical assessment of patients that are potentially at risk of developing AF, or of patients that are currently under management for AF. Appropriate screening methods should be defined and validated, and tailored interventions for stress reduction for those requiring further support should be investigated. Additionally, an improved understanding of the cortical and subcortical pathways driving the cardiac response under mental stress should be further investigated with the help of sophisticated neuroimaging methods. The latter may lead to the implementation and standardisation of mental stress tests in clinical practice as a tool to evaluate individual risk of developing AF.
Conclusion
AF is the most common form of cardiac arrhythmia and its increasing incidence is a worldwide burden. Mental stress has been shown to impact the cardiovascular system and to induce changes that can lead to the onset of AF. Negative emotions such as anger and anxiety and depressive symptoms have also been linked to an increased risk of developing AF in several observational studies, with their role as potential trigger of AF events suggested by laboratory-induced stress test and few observational studies. Psychological stress and emotions affect blood flow (increased heart rate and hypertension) and directly affect the heart by inducing alterations in cardiac electrical activity, which may lead to cardiac arrhythmias. Unfortunately, screening for mental health and psychological stress are not currently embedded into clinical pathways for the management of AF. However, the role that psychological stress and emotions on the onset of AF and on its recurrence need to be taken into consideration, as they can affect the management of these patients. AF has shown to be a preventable disease and helping in mange the emotional response and reducing mental stress may be effective in reducing the incidence of the disease.
Author contributions
DGL: drafting of the manuscript and critical revision. SSK: drafting of the manuscript and critical revision. HO: critical revision of the manuscript. DAL: critical revision of the manuscript. GYHL: critical revision of the manuscript. RP: conception and design of the paper and critical revision. All authors contributed to the article and approved the submitted version.
Conflict of interest
DAL has received investigator-initiated educational grants from Bristol Myers Squibb (BMS) and Pfizer; been a speaker for Boehringer Ingelheim, Bayer, and BMS/Pfizer and consulted for Boehringer Ingelheim and BMS/Pfizer; all outside the submitted work. GYHL: Consultant and speaker for BMS/Pfizer, Boehringer Ingelheim, Daiichi-Sankyo, Anthem. No fees are received personally.
The remaining authors declare that the research was conducted in the absence of any commercial or financial relationships that could be construed as a potential conflict of interest.
Publisher's note
All claims expressed in this article are solely those of the authors and do not necessarily represent those of their affiliated organizations, or those of the publisher, the editors and the reviewers. Any product that may be evaluated in this article, or claim that may be made by its manufacturer, is not guaranteed or endorsed by the publisher.
References
1. Kornej J, Börschel CS, Benjamin EJ, Schnabel RB. Epidemiology of atrial fibrillation in the 21st century. Circ Res. (2020) 127(1):4–20. doi: 10.1161/CIRCRESAHA.120.316340
2. Lau DH, Nattel S, Kalman JM, Sanders P. Modifiable risk factors and atrial fibrillation. Circulation. (2017) 136(6):583–96. doi: 10.1161/CIRCULATIONAHA.116.023163
3. Larsson SC, Drca N, Wolk A. Alcohol consumption and risk of atrial fibrillation: a prospective study and dose-response meta-analysis. J Am Coll Cardiol. (2014) 64(3):281–9. doi: 10.1016/j.jacc.2014.03.048
4. Aune D, Schlesinger S, Norat T, Riboli E. Tobacco smoking and the risk of atrial fibrillation: a systematic review and meta-analysis of prospective studies. Eur J Prev Cardiol. (2018) 25(13):1437–51. doi: 10.1177/2047487318780435
5. Anumonwo JMB, Kalifa J. Risk factors and genetics of atrial fibrillation. Cardiol Clin. (2014) 32(4):485–94. doi: 10.1016/j.ccl.2014.07.007
6. Lip GY. The ABC pathway: an integrated approach to improve AF management. Nat Rev Cardiol. (2017) 14(11):627–8. doi: 10.1038/nrcardio.2017.153
7. Potpara TS, Lip GY, Blomstrom-Lundqvist C, Boriani G, Van Gelder IC, Heidbuchel H, et al. The 4S-AF scheme (stroke risk; symptoms; severity of burden; substrate): a novel approach to in-depth characterization (rather than classification) of atrial fibrillation. Thromb Haemostasis. (2021) 121(03):270–8. doi: 10.1055/s-0040-1716408
8. Chao TF, Joung B, Takahashi Y, Lim TW, Choi EK, Chan YH, et al. 2021 focused update consensus guidelines of the Asia pacific heart rhythm society on stroke prevention in atrial fibrillation: executive summary. Thromb Haemost. (2022) 122(1):20–47. doi: 10.1055/s-0041-1739411
9. Foundation MH. Mental Health Foundation’s 2018 study (2018). Available at: https://www.mentalhealth.org.uk/explore-mental-health/statistics/stress-statistics
10. Burton A, McKinlay A, Aughterson H, Fancourt D. Impact of the COVID-19 pandemic on the mental health and well-being of adults with mental health conditions in the UK: a qualitative interview study. Journal of Mental Health. (2021):1–8. doi: 10.1080/09638237.2021.1952953
11. Galli F, Borghi L, Carugo S, Cavicchioli M, Faioni EM, Negroni MS, et al. Atrial fibrillation and psychological factors: a systematic review. PeerJ. (2017) 5:e3537. doi: 10.7717/peerj.3537
12. James PR, Taggart P, McNally ST, Newman SP, Sporton SC, Hardman SMC. Acute psychological stress and the propensity to ventricular arrhythmias. Evidence for a linking mechanism. Eur Heart J. (2000) 21(12):1023–8. doi: 10.1053/euhj.1999.1939
13. Nasser FJ, Almeida M, Silva L, Almeida R, Barbirato GB, Mendlowicz MV, et al. Psychiatric disorders and cardiovascular system: heart-brain interaction. Int J Cardiovasc Sci. (2016) 29(1):65–75. doi: 10.5935/2359-4802.20160003
14. Peacock J, Whang W. Psychological distress and arrhythmia: risk prediction and potential modifiers. Prog Cardiovasc Dis. (2013) 55(6):582–9. doi: 10.1016/j.pcad.2013.03.001
15. Shi S, Liu T, Liang J, Hu D, Yang B. Depression and risk of sudden cardiac death and arrhythmias: a meta-analysis. Psychosom Med. (2017) 79(2):153–61. doi: 10.1097/PSY.0000000000000382
16. Severino P, Mariani MV, Maraone A, Piro A, Ceccacci A, Tarsitani L, et al. Triggers for atrial fibrillation: the role of anxiety. Cardiol Res Pract. (2019) 2019:1–5. doi: 10.1155/2019/1208505
17. Thrall G, Lip GY, Carroll D, Lane D. Depression, anxiety, and quality of life in patients with atrial fibrillation. Chest. (2007) 132(4):1259–64. doi: 10.1378/chest.07-0036
18. Stalnikowicz R, Tsafrir A. Acute psychosocial stress and cardiovascular events. Am J Emerg Med. (2002) 20(5):488–91. doi: 10.1053/ajem.2002.34788
19. Steptoe A, Kivimäki M. Stress and cardiovascular disease. Nat Rev Cardiol. (2012) 9(6):360–70. doi: 10.1038/nrcardio.2012.45
20. Pickering TG. Mental stress as a causal factor in the development of hypertension and cardiovascular disease. Curr Hypertens Rep. (2001) 3(3):249–54. doi: 10.1007/s11906-001-0047-1
21. Xue Y-T, Tan Q-w, Li P, Mou S-f, Liu S-j, Bao Y, et al. Investigating the role of acute mental stress on endothelial dysfunction: a systematic review and meta-analysis. Clin Res Cardiol. (2015) 104(4):310–9. doi: 10.1007/s00392-014-0782-3
22. Bhide A, Durgaprasad R, Kasala L, Velam V, Hulikal N. Electrocardiographic changes during acute mental stress. Int J Med Sci Public Health. (2016) 5(5):835. doi: 10.5455/ijmsph.2016.19082015137
23. O'Neal WT, Hammadah M, Sandesara PB, Almuwaqqat Z, Samman-Tahhan A, Gafeer MM, et al. The association between acute mental stress and abnormal left atrial electrophysiology. J Cardiovasc Electrophysiol. (2017) 28(10):1151–7. doi: 10.1111/jce.13295
24. Almuwaqqat Z, O'Neal WT, Hammadah M, Lima BB, Bremner JD, Soliman EZ, et al. Abnormal P-wave axis and myocardial ischemia development during mental stress. J Electrocardiol. (2020) 60:3–7. doi: 10.1016/j.jelectrocard.2020.02.019
25. Lampert R, Jamner L, Burg M, Dziura J, Brandt C, Liu H, et al. Triggering of symptomatic atrial fibrillation by negative emotion. J Am Coll Cardiol. (2014) 64(14):1533–4. doi: 10.1016/j.jacc.2014.07.959
26. Lévy S, Maarek M, Coumel P, Guize L, Lekieffre J, Medvedowsky J-L, et al. Characterization of different subsets of atrial fibrillation in general practice in France. Circulation. (1999) 99(23):3028–35. doi: 10.1161/01.CIR.99.23.3028
27. Peter RH, Gracey JG, Beach TB. A clinical profile of idiopathic atrial fibrillation. Ann Intern Med. (1968) 68(6):1288–95. doi: 10.7326/0003-4819-68-6-1288
28. Mattioli AV, Bonatti S, Zennaro M, Melotti R, Mattioli G. Effect of coffee consumption, lifestyle and acute life stress in the development of acute lone atrial fibrillation. J Cardiovasc Med (Hagerstown). (2008) 9(8):794–8. doi: 10.2459/JCM.0b013e3282f64554
29. Kivimäki M, Nyberg ST, Batty GD, Kawachi I, Jokela M, Alfredsson L, et al. Long working hours as a risk factor for atrial fibrillation: a multi-cohort study. Eur Heart J. (2017) 38(34):2621–8. doi: 10.1093/eurheartj/ehx324
30. Fransson EI, Stadin M, Nordin M, Malm D, Knutsson A, Alfredsson L, et al. The association between job strain and atrial fibrillation: results from the Swedish WOLF study. BioMed Res Int. (2015) 2015:371905. doi: 10.1155/2015/371905
31. Fransson EI, Nordin M, Magnusson Hanson LL, Westerlund H. Job strain and atrial fibrillation—results from the Swedish longitudinal occupational survey of health and meta-analysis of three studies. Eur J Prev Cardiol. (2020) 25(11):1142–9. doi: 10.1177/2047487318777387
32. Soliman EZ, Zhang ZM, Judd S, Howard VJ, Howard G. Comparison of risk of atrial fibrillation among employed versus unemployed (from the REasons for geographic and racial differences in stroke study). Am J Cardiol. (2017) 120(8):1298–301. doi: 10.1016/j.amjcard.2017.07.001
33. Whang W, Davidson KW, Conen D, Tedrow UB, Everett BM, Albert CM. Global psychological distress and risk of atrial fibrillation among women: the women’s health study. J Am Heart Assoc. (2012) 1(3):e001107. doi: 10.1161/JAHA.112.001107
34. Eaker ED, Sullivan LM, Kelly-Hayes M, D’Agostino RB Sr, Benjamin EJ. Tension and anxiety and the prediction of the 10-year incidence of coronary heart disease, atrial fibrillation, and total mortality: the framingham offspring study. Psychosom Med. (2005) 67(5):692–6. doi: 10.1097/01.psy.0000174050.87193.96
35. Tully PJ, Bennetts JS, Baker RA, McGavigan AD, Turnbull DA, Winefield HR. Anxiety, depression, and stress as risk factors for atrial fibrillation after cardiac surgery. Heart Lung. (2011) 40(1):4–11. doi: 10.1016/j.hrtlng.2009.12.010
36. Cheng YF, Leu HB, Su CC, Huang CC, Chiang CH, Huang PH, et al. Association between panic disorder and risk of atrial fibrillation:a nationwide study. Psychosom Med. (2013) 75(1):30–5. doi: 10.1097/PSY.0b013e318273393a
37. Lange HW, Herrmann-Lingen C. Depressive symptoms predict recurrence of atrial fibrillation after cardioversion. J Psychosom Res. (2007) 63(5):509–13. doi: 10.1016/j.jpsychores.2007.07.010
38. Garg PK, O’Neal WT, Diez-Roux AV, Alonso A, Soliman EZ, Heckbert S. Negative affect and risk of atrial fibrillation: mESA. J Am Heart Assoc. (2019) 8(1):e010603. doi: 10.1161/JAHA.118.010603
39. Feng T, Malmo V, Laugsand LE, Strand LB, Gustad LT, Ellekjær H, et al. Symptoms of anxiety and depression and risk of atrial fibrillation-the HUNT study. Int J Cardiol. (2020) 306:95–100. doi: 10.1016/j.ijcard.2019.11.107
40. Rohleder N. Stress and inflammation—the need to address the gap in the transition between acute and chronic stress effects. Psychoneuroendocrinology. (2019) 105:164–71. doi: 10.1016/j.psyneuen.2019.02.021
41. Osborne MT, Shin LM, Mehta NN, Pitman RK, Fayad ZA, Tawakol A. Disentangling the links between psychosocial stress and cardiovascular disease. Circulation Cardiovasc Imaging. (2020) 13(8):e010931. doi: 10.1161/CIRCIMAGING.120.010931
42. Swirski FK. From clonal haematopoiesis to the CANTOS trial. Nat Rev Cardiol. (2018) 15(2):79–80. doi: 10.1038/nrcardio.2017.208
43. Lewek J, Kaczmarek K, Cygankiewicz I, Wranicz JK, Ptaszynski P. Inflammation and arrhythmias: potential mechanisms and clinical implications. Expert Rev Cardiovasc Ther. (2014) 12(9):1077–85. doi: 10.1586/14779072.2014.942286
44. Scott L, Li N, Dobrev D. Role of inflammatory signaling in atrial fibrillation. Int J Cardiol. (2019) 287:195–200. doi: 10.1016/j.ijcard.2018.10.020
45. Liu Y, Wu F, Wu Y, Elliott M, Zhou W, Deng Y, et al. Mechanism of IL-6-related spontaneous atrial fibrillation after coronary artery grafting surgery: IL-6 knockout mouse study and human observation. Transl Res. (2021) 233:16–31. doi: 10.1016/j.trsl.2021.01.007
46. Wu Q, Liu H, Liao J, Zhao N, Tse G, Han B, et al. Colchicine prevents atrial fibrillation promotion by inhibiting IL-1β-induced IL-6 release and atrial fibrosis in the rat sterile pericarditis model. Biomed Pharmacother. (2020) 129:110384. doi: 10.1016/j.biopha.2020.110384
47. Hadi HA, Alsheikh-Ali AA, Mahmeed WA, Suwaidi JMA. Inflammatory cytokines and atrial fibrillation: current and prospective views. J Inflamm Res. (2010) 3:75–97. doi: 10.2147/JIR.S10095
48. Mann SJ. Neurogenic hypertension: pathophysiology, diagnosis and management. Clin Auton Res. (2018) 28(4):363–74. doi: 10.1007/s10286-018-0541-z
49. Liu H, Luiten PG, Eisel UL, Dejongste MJ, Schoemaker RG. Depression after myocardial infarction: TNF-α-induced alterations of the blood-brain barrier and its putative therapeutic implications. Neurosci Biobehav Rev. (2013) 37(4):561–72. doi: 10.1016/j.neubiorev.2013.02.004
50. Tahsili-Fahadan P, Geocadin RG. Heart-brain axis: effects of neurologic injury on cardiovascular function. Circ Res. (2017) 120(3):559–72. doi: 10.1161/CIRCRESAHA.116.308446
51. Scheitz JF, Nolte CH, Doehner W, Hachinski V, Endres M. Stroke–heart syndrome: clinical presentation and underlying mechanisms. Lancet Neurol. (2018) 17(12):1109–20. doi: 10.1016/S1474-4422(18)30336-3
52. Buckley BJR, Harrison SL, Hill A, Underhill P, Lane DA, Lip GYH. Stroke-heart syndrome: incidence and clinical outcomes of cardiac complications following stroke. Stroke. (2022) 53(5):1759–63. doi: 10.1161/STROKEAHA.121.037316
53. Aygun D, Altintop L, Doganay Z, Guven H, Baydin A. Electrocardiographic changes during migraine attacks. Headache. (2003) 43(8):861–6. doi: 10.1046/j.1526-4610.2003.03164.x
54. Calandra-Buonaura G, Provini F, Guaraldi P, Plazzi G, Cortelli P. Cardiovascular autonomic dysfunctions and sleep disorders. Sleep Med Rev. (2016) 26:43–56. doi: 10.1016/j.smrv.2015.05.005
55. Chen Z, Venkat P, Seyfried D, Chopp M, Yan T, Chen J. Brain–heart interaction. Circ Res. (2017) 121(4):451–68. doi: 10.1161/CIRCRESAHA.117.311170
56. Colivicchi F, Bassi A, Santini M, Caltagirone C. Cardiac autonomic derangement and arrhythmias in right-sided stroke with insular involvement. Stroke. (2004) 35(9):2094–8. doi: 10.1161/01.STR.0000138452.81003.4c
57. Ding M, Qiu C. Atrial fibrillation, cognitive decline, and dementia: an epidemiologic review. Curr Epidemiol Rep. (2018) 5(3):252–61. doi: 10.1007/s40471-018-0159-7
58. Furlan JC, Fehlings MG. Cardiovascular complications after acute spinal cord injury: pathophysiology, diagnosis, and management. Neurosurg Focus. (2008) 25(5):E13. doi: 10.3171/FOC.2008.25.11.E13
59. Giubilei F, Strano S, Lino S, Calcagnini G, Tisei P, Fiorelli M, et al. Autonomic nervous activity during sleep in middle cerebral artery infarction. Cerebrovasc Dis. (1998) 8(2):118–23. doi: 10.1159/000015829
60. Idiaquez J, Roman GC. Autonomic dysfunction in neurodegenerative dementias. J Neurol Sci. (2011) 305(1-2):22–7. doi: 10.1016/j.jns.2011.02.033
61. Jalan P, Dhakal L, Pandav V, Omar AI. Status migrainosus as a potential stressor leading to takotsubo cardiomyopathy. Cephalalgia. (2012) 32(15):1140–3. doi: 10.1177/0333102412459574
62. Lump D, Moyer M. Paroxysmal sympathetic hyperactivity after severe brain injury. Curr Neurol Neurosci Rep. (2014) 14(11):494. doi: 10.1007/s11910-014-0494-0
63. Mathew MJ, Deepika A, Shukla D, Devi BI, Ramesh VJ. Paroxysmal sympathetic hyperactivity in severe traumatic brain injury. Acta Neurochir (Wien). (2016) 158(11):2047–52. doi: 10.1007/s00701-016-2934-x
64. Oppenheimer S. Cerebrogenic cardiac arrhythmias: cortical lateralization and clinical significance. Clin Auton Res. (2006) 16(1):6–11. doi: 10.1007/s10286-006-0276-0
65. Partida E, Mironets E, Hou S, Tom VJ. Cardiovascular dysfunction following spinal cord injury. Neural Regen Res. (2016) 11(2):189–94. doi: 10.4103/1673-5374.177707
66. Romigi A, Albanese M, Placidi F, Izzi F, Mercuri NB, Marchi A, et al. Heart rate variability in untreated newly diagnosed temporal lobe epilepsy: evidence for ictal sympathetic dysregulation. Epilepsia. (2016) 57(3):418–26. doi: 10.1111/epi.13309
67. Rugg-Gunn FJ, Simister RJ, Squirrell M, Holdright DR, Duncan JS. Cardiac arrhythmias in focal epilepsy: a prospective long-term study. Lancet. (2004) 364(9452):2212–9. doi: 10.1016/S0140-6736(04)17594-6
68. Sambati L, Calandra-Buonaura G, Doria A, Cortelli P. Diagnosis and management of autonomic failure in neurodegenerative disorders. Eur Neurol. (2015) 73(1-2):126–33. doi: 10.1159/000368828
69. Taggart P, Critchley H, Lambiase P. Heart–brain interactions in cardiac arrhythmia. Heart. (2011) 97(9):698–708. doi: 10.1136/hrt.2010.209304
70. Tinuper P, Bisulli F, Cerullo A, Carcangiu R, Marini C, Pierangeli G, et al. Ictal bradycardia in partial epileptic seizures: autonomic investigation in three cases and literature review. Brain. (2001) 124(Pt 12):2361–71. doi: 10.1093/brain/124.12.2361
71. Tobaldini E, Costantino G, Solbiati M, Cogliati C, Kara T, Nobili L, et al. Sleep, sleep deprivation, autonomic nervous system and cardiovascular diseases. Neurosci Biobehav Rev. (2017) 74(Pt B):321–9. doi: 10.1016/j.neubiorev.2016.07.004
72. Zijlmans M, Flanagan D, Gotman J. Heart rate changes and ECG abnormalities during epileptic seizures: prevalence and definition of an objective clinical sign. Epilepsia. (2002) 43(8):847–54. doi: 10.1046/j.1528-1157.2002.37801.x
73. Miranda M, Morici JF, Zanoni MB, Bekinschtein P. Brain-derived neurotrophic factor: a key molecule for memory in the healthy and the pathological brain. Front Cell Neurosci. (2019) 13:1–25. doi: 10.3389/fncel.2019.00363
74. Kermani P, Hempstead B. Brain-derived neurotrophic factor: a newly described mediator of angiogenesis. Trends Cardiovasc Med. (2007) 17(4):140–3. doi: 10.1016/j.tcm.2007.03.002
75. Coppola V, Barrick CA, Southon EA, Celeste A, Wang K, Chen B, et al. Ablation of TrkA function in the immune system causes B cell abnormalities. Development. (2004) 131(20):5185–95. doi: 10.1242/dev.01383
76. Caporali A, Emanueli C. Cardiovascular actions of neurotrophins. Physiol Rev. (2009) 89(1):279–308. doi: 10.1152/physrev.00007.2008
77. Pius-Sadowska E, Machaliński B. BDNF—a key player in cardiovascular system. J Mol Cell Cardiol. (2017) 110:54–60. doi: 10.1016/j.yjmcc.2017.07.007
78. Takashio S, Sugiyama S, Yamamuro M, Takahama H, Hayashi T, Sugano Y, et al. Significance of low plasma levels of brain-derived neurotrophic factor in patients with heart failure. Am J Cardiol. (2015) 116(2):243–9. doi: 10.1016/j.amjcard.2015.04.018
79. Nagai M, Hoshide S, Kario K. The insular cortex and cardiovascular system: a new insight into the brain-heart axis. J Am Soc Hypertens. (2010) 4(4):174–82. doi: 10.1016/j.jash.2010.05.001
81. Van Eden CG, Buijs RM. Functional neuroanatomy of the prefrontal cortex: autonomic interactions. Prog Brain Res. (2000) 126:49–62. doi: 10.1016/S0079-6123(00)26006-8
82. Coutlee CG, Huettel SA. The functional neuroanatomy of decision making: prefrontal control of thought and action. Brain Res. (2012) 1428:3–12. doi: 10.1016/j.brainres.2011.05.053
83. Fletcher PC, Frith CD, Rugg MD. The functional neuroanatomy of episodic memory. Trends Neurosci. (1997) 20(5):213–8. doi: 10.1016/S0166-2236(96)01013-2
84. Müller NG, Knight RT. The functional neuroanatomy of working memory: contributions of human brain lesion studies. Neuroscience. (2006) 139(1):51–8. doi: 10.1016/j.neuroscience.2005.09.018
85. Davidson RJ, Irwin W. The functional neuroanatomy of emotion and affective style. Trends Cogn Sci. (1999) 3(1):11–21. doi: 10.1016/S1364-6613(98)01265-0
86. Munakata Y, Herd SA, Chatham CH, Depue BE, Banich MT, O'Reilly RC. A unified framework for inhibitory control. Trends Cogn Sci. (2011) 15(10):453–9. doi: 10.1016/j.tics.2011.07.011
87. Gabrieli JD, Poldrack RA, Desmond JE. The role of left prefrontal cortex in language and memory. Proc Natl Acad Sci U S A. (1998) 95(3):906–13. doi: 10.1073/pnas.95.3.906
88. Goodman M, New A, Siever L. Trauma, genes, and the neurobiology of personality disorders. Ann N Y Acad Sci. (2004) 1032:104–16. doi: 10.1196/annals.1314.008
89. Buijs RM, Van Eden CG. The integration of stress by the hypothalamus, amygdala and prefrontal cortex: balance between the autonomic nervous system and the neuroendocrine system. Prog Brain Res. (2000) 126:117–32. doi: 10.1016/S0079-6123(00)26011-1
90. Schaeuble D, Packard AEB, McKlveen JM, Morano R, Fourman S, Smith BL, et al. Prefrontal cortex regulates chronic stress-induced cardiovascular susceptibility. J Am Heart Assoc. (2019) 8(24):e014451. doi: 10.1161/JAHA.119.014451
91. Arnsten AF. Stress signalling pathways that impair prefrontal cortex structure and function. Nat Rev Neurosci. (2009) 10(6):410–22. doi: 10.1038/nrn2648
92. Izquierdo A, Wellman CL, Holmes A. Brief uncontrollable stress causes dendritic retraction in infralimbic cortex and resistance to fear extinction in mice. J Neurosci. (2006) 26(21):5733–8. doi: 10.1523/JNEUROSCI.0474-06.2006
93. Brown SM, Henning S, Wellman CL. Mild, short-term stress alters dendritic morphology in rat medial prefrontal cortex. Cereb Cortex. (2005) 15(11):1714–22. doi: 10.1093/cercor/bhi048
94. Arnsten AF, Raskind MA, Taylor FB, Connor DF. The effects of stress exposure on prefrontal cortex: translating basic research into successful treatments for post-traumatic stress disorder. Neurobiol Stress. (2015) 1:89–99. doi: 10.1016/j.ynstr.2014.10.002
95. Mueller K, Thiel F, Beutner F, Teren A, Frisch S, Ballarini T, et al. Brain damage with heart failure: cardiac biomarker alterations and gray matter decline. Circ Res. (2020) 126(6):750–64. doi: 10.1161/CIRCRESAHA.119.315813
96. Badaly D, Beers SR, Ceschin R, Lee VK, Sulaiman S, Zahner A, et al. Cerebellar and prefrontal structures associated with executive functioning in pediatric patients with congenital heart defects. Front Neurol. (2022) 13:827780. doi: 10.3389/fneur.2022.827780
97. Schumann A, de la Cruz F, Köhler S, Brotte L, Bär KJ. The influence of heart rate variability biofeedback on cardiac regulation and functional brain connectivity. Front Neurosci. (2021) 15:691988. doi: 10.3389/fnins.2021.691988
98. Agrimi J, Scalco A, Agafonova J, Williams L III, Pansari N, Keceli G, et al. Psychosocial stress hastens disease progression and sudden death in mice with arrhythmogenic cardiomyopathy. J Clin Med. (2020) 9(12):1–16. doi: 10.3390/jcm9123804
99. Uddin LQ, Nomi JS, Hébert-Seropian B, Ghaziri J, Boucher O. Structure and function of the human insula. J Clin Neurophysiol. (2017) 34(4):300–6. doi: 10.1097/WNP.0000000000000377
100. Nieuwenhuys R. The insular cortex: a review. Prog Brain Res. (2012) 195:123–63. doi: 10.1016/B978-0-444-53860-4.00007-6
101. Gogolla N. The insular cortex. Curr Biol. (2017) 27(12):R580–r6. doi: 10.1016/j.cub.2017.05.010
102. Uddin LQ, Nomi JS, Hébert-Seropian B, Ghaziri J, Boucher O. Structure and function of the human insula. J Clin Neurophysiol. (2017) 34(4):300–6. doi: 10.1097/WNP.0000000000000377
103. Chouchou F, Mauguière F, Vallayer O, Catenoix H, Isnard J, Montavont A, et al. How the insula speaks to the heart: cardiac responses to insular stimulation in humans. Hum Brain Mapp. (2019) 40(9):2611–22. doi: 10.1002/hbm.24548
104. de Morree HM, Rutten G-J, Szabó BM, Sitskoorn MM, Kop WJ. Effects of insula resection on autonomic nervous system activity. J Neurosurg Anesthesiol. (2016) 28(2):153–8. doi: 10.1097/ANA.0000000000000207
105. Bruce SE, Buchholz KR, Brown WJ, Yan L, Durbin A, Sheline YI. Altered emotional interference processing in the amygdala and insula in women with post-traumatic stress disorder. NeuroImage Clin. (2013) 2:43–9. doi: 10.1016/j.nicl.2012.11.003
106. Rieger NS, Varela JA, Ng AJ, Granata L, Djerdjaj A, Brenhouse HC, et al. Insular cortex corticotropin-releasing factor integrates stress signaling with social affective behavior. Neuropsychopharmacology. (2022) 47(6):1156–68. doi: 10.1038/s41386-022-01292-7
107. Tomeo RA, Gomes-de-Souza L, Benini R, Reis-Silva LL, Crestani CC. Site-specific regulation of stress responses along the rostrocaudal axis of the insular cortex in rats. Front Neurosci. (2022) 16:878927. doi: 10.3389/fnins.2022.878927
108. Wang R, Zhen S, Zhou C, Yu R. Acute stress promotes brain network integration and reduces state transition variability. Proc Natl Acad Sci U S A. (2022) 119(24):e2204144119. doi: 10.1073/pnas.2204144119
109. Zhang W, Hashemi MM, Kaldewaij R, Koch SBJ, Beckmann C, Klumpers F, et al. Acute stress alters the “default” brain processing. Neuroimage. (2019) 189:870–7. doi: 10.1016/j.neuroimage.2019.01.063
110. Lin HB, Li FX, Zhang JY, You ZJ, Xu SY, Liang WB, et al. Cerebral-cardiac syndrome and diabetes: cardiac damage after ischemic stroke in diabetic state. Front Immunol. (2021) 12:737170. doi: 10.3389/fimmu.2021.737170
111. Scheitz JF, Erdur H, Haeusler KG, Audebert HJ, Roser M, Laufs U, et al. Insular cortex lesions, cardiac troponin, and detection of previously unknown atrial fibrillation in acute ischemic stroke: insights from the troponin elevation in acute ischemic stroke study. Stroke. (2015) 46(5):1196–201. doi: 10.1161/STROKEAHA.115.008681
112. Akkad I, Kundu S, Miller A, Ramachandran J, Shetty V. Acute stroke of the insular cortex leading to heart failure. J Med Cases. (2016) 7(3):94–7. doi: 10.14740/jmc2426w
113. Oppenheimer SM, Gelb A, Girvin JP, Hachinski VC. Cardiovascular effects of human insular cortex stimulation. Neurology. (1992) 42(9):1727–32. doi: 10.1212/WNL.42.9.1727
114. Critchley HD, Rotshtein P, Nagai Y, O'Doherty J, Mathias CJ, Dolan RJ. Activity in the human brain predicting differential heart rate responses to emotional facial expressions. Neuroimage. (2005) 24(3):751–62. doi: 10.1016/j.neuroimage.2004.10.013
115. Napadow V, Dhond R, Conti G, Makris N, Brown EN, Barbieri R. Brain correlates of autonomic modulation: combining heart rate variability with fMRI. Neuroimage. (2008) 42(1):169–77. doi: 10.1016/j.neuroimage.2008.04.238
116. Lane RD, McRae K, Reiman EM, Chen K, Ahern GL, Thayer JF. Neural correlates of heart rate variability during emotion. Neuroimage. (2009) 44(1):213–22. doi: 10.1016/j.neuroimage.2008.07.056
117. Vancheri F, Longo G, Vancheri E, Henein MY. Mental stress and cardiovascular health-part I. J Clin Med. (2022) 11(12):1–17. doi: 10.3390/jcm11123353
118. Tsigos C, Chrousos GP. Hypothalamic–pituitary–adrenal axis, neuroendocrine factors and stress. J Psychosom Res. (2002) 53(4):865–71. doi: 10.1016/S0022-3999(02)00429-4
119. Papadimitriou A, Priftis KN. Regulation of the hypothalamic-pituitary-adrenal axis. Neuroimmunomodulation. (2009) 16(5):265–71. doi: 10.1159/000216184
120. Adameova A, Abdellatif Y, Dhalla NS. Role of the excessive amounts of circulating catecholamines and glucocorticoids in stress-induced heart disease. Can J Physiol Pharmacol. (2009) 87(7):493–514. doi: 10.1139/Y09-042
121. Dimmer C, Tavernier R, Gjorgov N, Van Nooten G, Clement DL, Jordaens L. Variations of autonomic tone preceding onset of atrial fibrillation after coronary artery bypass grafting. Am J Cardiol. (1998) 82(1):22–5. doi: 10.1016/S0002-9149(98)00231-8
122. Workman AJ. Cardiac adrenergic control and atrial fibrillation. Naunyn Schmiedeberg’s Arch Pharmacol. (2010) 381(3):235–49. doi: 10.1007/s00210-009-0474-0
123. Tawakol A, Ishai A, Takx RA, Figueroa AL, Ali A, Kaiser Y, et al. Relation between resting amygdalar activity and cardiovascular events: a longitudinal and cohort study. Lancet. (2017) 389(10071):834–45. doi: 10.1016/S0140-6736(16)31714-7
124. Tawakol A, Osborne MT, Wang Y, Hammed B, Tung B, Patrich T, et al. Stress-associated neurobiological pathway linking socioeconomic disparities to cardiovascular disease. J Am Coll Cardiol. (2019) 73(25):3243–55. doi: 10.1016/j.jacc.2019.04.042
125. Williams LM, Kemp AH, Felmingham K, Barton M, Olivieri G, Peduto A, et al. Trauma modulates amygdala and medial prefrontal responses to consciously attended fear. Neuroimage. (2006) 29(2):347–57. doi: 10.1016/j.neuroimage.2005.03.047
126. McEwen BS. Plasticity of the hippocampus: adaptation to chronic stress and allostatic load. Ann N Y Acad Sci. (2001) 933:265–77. doi: 10.1111/j.1749-6632.2001.tb05830.x
127. Frey A, Homola GA, Henneges C, Mühlbauer L, Sell R, Kraft P, et al. Temporal changes in total and hippocampal brain volume and cognitive function in patients with chronic heart failure-the COGNITION.MATTERS-HF cohort study. Eur Heart J. (2021) 42(16):1569–78. doi: 10.1093/eurheartj/ehab003
128. Knecht S, Oelschläger C, Duning T, Lohmann H, Albers J, Stehling C, et al. Atrial fibrillation in stroke-free patients is associated with memory impairment and hippocampal atrophy. Eur Heart J. (2008) 29(17):2125–32. doi: 10.1093/eurheartj/ehn341
129. Stefansdottir H, Arnar DO, Aspelund T, Sigurdsson S, Jonsdottir MK, Hjaltason H, et al. Atrial fibrillation is associated with reduced brain volume and cognitive function independent of cerebral infarcts. Stroke. (2013) 44(4):1020–5. doi: 10.1161/STROKEAHA.12.679381
130. Park S, Lee S, Kim Y, Cho S, Kim K, Kim YC, et al. Causal effects of atrial fibrillation on brain white and gray matter volume: a Mendelian randomization study. BMC Med. (2021) 19(1):274. doi: 10.1186/s12916-021-02152-9
131. Moazzami K, Shao IY, Chen LY, Lutsey PL, Jack CR Jr, Mosley T, et al. Atrial fibrillation, brain volumes, and subclinical cerebrovascular disease (from the atherosclerosis risk in communities neurocognitive study [ARIC-NCS]). Am J Cardiol. (2020) 125(2):222–8. doi: 10.1016/j.amjcard.2019.10.010
132. Gardarsdottir M, Sigurdsson S, Aspelund T, Rokita H, Launer LJ, Gudnason V, et al. Atrial fibrillation is associated with decreased total cerebral blood flow and brain perfusion. Europace. (2018) 20(8):1252–8. doi: 10.1093/europace/eux220
133. McCorry LK. Physiology of the autonomic nervous system. Am J Pharm Educ. (2007) 71(4):1–11. doi: 10.5688/aj710478
134. Shen MJ, Zipes DP. Role of the autonomic nervous system in modulating cardiac arrhythmias. Circ Res. (2014) 114(6):1004–21. doi: 10.1161/CIRCRESAHA.113.302549
135. Hering D, Lachowska K, Schlaich M. Role of the sympathetic nervous system in stress-mediated cardiovascular disease. Curr Hypertens Rep. (2015) 17(10):80. doi: 10.1007/s11906-015-0594-5
136. Khan AA, Lip GYH, Shantsila A. Heart rate variability in atrial fibrillation: the balance between sympathetic and parasympathetic nervous system. Eur J Clin Investig. (2019) 49(11):e13174. doi: 10.1111/eci.13174
137. Linz D, Elliott AD, Hohl M, Malik V, Schotten U, Dobrev D, et al. Role of autonomic nervous system in atrial fibrillation. Int J Cardiol. (2019) 287:181–8. doi: 10.1016/j.ijcard.2018.11.091
138. Kiuchi MG, Nolde JM, Villacorta H, Carnagarin R, Chan JJS-Y, Lugo-Gavidia LM, et al. New approaches in the management of sudden cardiac death in patients with heart failure—targeting the sympathetic nervous system. Int J Mol Sci. (2019) 20(10):2430. doi: 10.3390/ijms20102430
139. Cao JM, Chen LS, KenKnight BH, Ohara T, Lee MH, Tsai J, et al. Nerve sprouting and sudden cardiac death. Circ Res. (2000) 86(7):816–21. doi: 10.1161/01.RES.86.7.816
140. Fontes MA, Xavier CH, Marins FR, Limborço-Filho M, Vaz GC, Müller-Ribeiro FC, et al. Emotional stress and sympathetic activity: contribution of dorsomedial hypothalamus to cardiac arrhythmias. Brain Res. (2014) 1554:49–58. doi: 10.1016/j.brainres.2014.01.043
141. Fontes MA, Tagawa T, Polson JW, Cavanagh SJ, Dampney RA. Descending pathways mediating cardiovascular response from dorsomedial hypothalamic nucleus. Am J Physiol Heart Circ Physiol. (2001) 280(6):H2891–901. doi: 10.1152/ajpheart.2001.280.6.H2891
142. Hsueh B, Chen R, Jo Y, Tang D, Raffiee M, Kim YS, et al. Cardiogenic control of affective behavioural state. Nature. (2023) 615(7951):292–9. doi: 10.1038/s41586-023-05748-8
143. Maroofi A, Moro T, Agrimi J, Safari F. Cognitive decline in heart failure: biomolecular mechanisms and benefits of exercise. Bioch Biophy Acta (BBA) Mol Basis Dis. (2022) 1868(11):166511. doi: 10.1016/j.bbadis.2022.166511
144. Toledo C, Andrade D, Díaz H, Inestrosa N, Del Rio R. Neurocognitive disorders in heart failure: novel pathophysiological mechanisms underpinning memory loss and learning impairment. Mol Neurobiol. (2019) 56:8035–51. doi: 10.1007/s12035-019-01655-0
145. Xu C, Tao X, Ma X, Zhao R, Cao Z. Cognitive dysfunction after heart disease: a manifestation of the heart-brain axis. Oxid Med Cell Longevity. (2021) 2021:4899688. doi: 10.1155/2021/4899688
146. Orth-Gomér K, Schneiderman N, Wang H-X, Walldin C, Blom M, Jernberg T. Stress reduction prolongs life in women with coronary disease. Circ Cardiovasc Qual Outcomes. (2009) 2(1):25–32. doi: 10.1161/CIRCOUTCOMES.108.812859
147. Magán I, Casado L, Jurado-Barba R, Barnum H, Redondo MM, Hernandez AV, et al. Efficacy of psychological interventions on psychological outcomes in coronary artery disease: systematic review and meta-analysis. Psychol Med. (2021) 51(11):1846–60. doi: 10.1017/S0033291720000598
148. Hindricks G, Potpara T, Dagres N, Arbelo E, Bax JJ, Blomström-Lundqvist C, et al. 2020 ESC guidelines for the diagnosis and management of atrial fibrillation developed in collaboration with the European association for cardio-thoracic surgery (EACTS): the task force for the diagnosis and management of atrial fibrillation of the European society of cardiology (ESC) developed with the special contribution of the European heart rhythm association (EHRA) of the ESC. Eur Heart J. (2021) 42(5):373–498. doi: 10.1093/eurheartj/ehaa612
149. Burdett P, Lip GYH. Atrial fibrillation in the UK: predicting costs of an emerging epidemic recognizing and forecasting the cost drivers of atrial fibrillation-related costs. Eur Heart J Qual Care Clin Outcomes. (2022) 8(2):187–94. doi: 10.1093/ehjqcco/qcaa093
150. Le Heuzey J-Y, Paziaud O, Piot O, Said MA, Copie X, Lavergne T, et al. Cost of care distribution in atrial fibrillation patients: the COCAF study. Am Heart J. (2004) 147(1):121–6. doi: 10.1016/S0002-8703(03)00524-6
151. Crosswell AD, Lockwood KG. Best practices for stress measurement: how to measure psychological stress in health research. Health Psychol Open. (2020) 7(2):2055102920933072. doi: 10.1177/2055102920933072
152. Pascoe MC, Thompson DR, Ski CF. Yoga, mindfulness-based stress reduction and stress-related physiological measures: a meta-analysis. Psychoneuroendocrinology. (2017) 86:152–68. doi: 10.1016/j.psyneuen.2017.08.008
153. Janssen M, Heerkens Y, Kuijer W, van der Heijden B, Engels J. Effects of mindfulness-based stress reduction on employees’ mental health: a systematic review. PLoS ONE. (2018) 13(1):e0191332. doi: 10.1371/journal.pone.0191332
154. Gonçalves R, Rodrigues H, Novaes F, Arbol J, Volchan E, Coutinho ESF, et al. Listening to the heart: a meta-analysis of cognitive behavior therapy impact on the heart rate of patients with anxiety disorders. J Affect Disord. (2015) 172:231–40. doi: 10.1016/j.jad.2014.09.058
155. Fortin M, Bravo G, Hudon C, Lapointe L, Dubois M-F, Almirall J. Psychological distress and multimorbidity in primary care. Ann Fam Med. (2006) 4(5):417–22. doi: 10.1370/afm.528
Keywords: atrial fibrillation, cardiac autonomic nervous system, mental health, psychological stress, stress
Citation: Leo DG, Ozdemir H, Lane DA, Lip GYH, Keller SS and Proietti R (2023) At the heart of the matter: how mental stress and negative emotions affect atrial fibrillation. Front. Cardiovasc. Med. 10:1171647. doi: 10.3389/fcvm.2023.1171647
Received: 22 February 2023; Accepted: 7 June 2023;
Published: 20 June 2023.
Edited by:
Wuxiang Xie, Peking University, ChinaReviewed by:
Jacopo Agrimi, University of Padua, ItalyLaura Calvillo, Italian Auxological Institute (IRCCS), Italy
© 2023 Leo, Ozdemir, Lane, Lip, Keller and Proietti. This is an open-access article distributed under the terms of the Creative Commons Attribution License (CC BY). The use, distribution or reproduction in other forums is permitted, provided the original author(s) and the copyright owner(s) are credited and that the original publication in this journal is cited, in accordance with accepted academic practice. No use, distribution or reproduction is permitted which does not comply with these terms.
*Correspondence: Riccardo Proietti Riccardo.Proietti@liverpool.ac.uk
†These authors share senior authorship
‡ORCID Donato Giuseppe Leo orcid.org/0000-0002-0709-3073 Hizir Ozdemir orcid.org/0000-0001-9830-689X Deirdre A. Lane orcid.org/0000-0002-5604-9378 Gregory Y. H. Lip orcid.org/0000-0002-7566-1626 Simon S. Keller orcid.org/0000-0001-5247-9795 Riccardo Proietti orcid.org/0000-0003-4113-7030