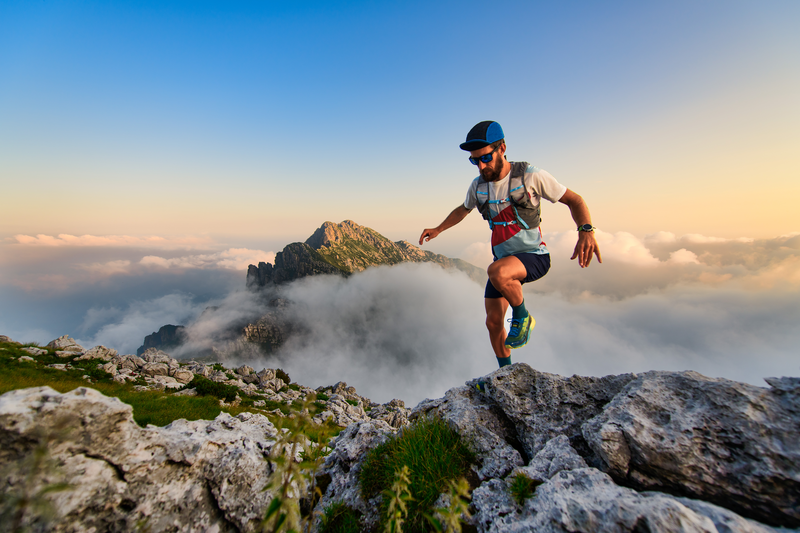
94% of researchers rate our articles as excellent or good
Learn more about the work of our research integrity team to safeguard the quality of each article we publish.
Find out more
ORIGINAL RESEARCH article
Front. Cardiovasc. Med. , 11 August 2023
Sec. General Cardiovascular Medicine
Volume 10 - 2023 | https://doi.org/10.3389/fcvm.2023.1164524
Introduction: Corin is a protease in the natriuretic peptide system. Deleterious CORIN variants are associated with hypertension and heart disease. It remains unclear if and to what extent corin deficiency may contribute to heart failure (HF).
Methods: Corin knockout (KO) mice were used as a model. Cardiac function was assessed by echocardiography and tissue analysis in Corin KO mice at different ages or subjected to transverse aortic constriction (TAC), which increased pressure overload. Heart and lung tissues were analyzed for cardiac hypertrophy and lung edema using wheat germ agglutinin, Sirius red, Masson's trichrome, and Prussian blue staining. Recombinant corin was tested for its effect on cardiac function in the TAC-operated Corin KO mice. Selected gene expression in the heart was examined by RT-PCR. ELISA was used to analyze factors in plasma.
Results: Corin KO mice had progressive cardiac dysfunction with cardiac hypertrophy and fibrosis after 9 months of age, likely due to chronic hypertension. When Corin KO mice were subjected to TAC at 10–12 weeks of age, cardiac function decreased more rapidly than in similarly treated wild-type mice. When the TAC-operated Corin KO mice were treated with recombinant corin protein, cardiac dysfunction, hypertrophy, and fibrosis were ameliorated. The corin treatment also decreased the gene expression associated with cardiac hypertrophy and fibrosis, increased plasma cGMP levels, lowered plasma levels of N-terminal pro-atrial natriuretic peptide, angiotensin II, and aldosterone, and lessened lung edema in the Corin KO mice subjected to TAC.
Conclusion: Corin deficiency impairs cardiac function and exacerbates HF development in mice. Corin protein may be used to reduce cardiac hypertrophy and fibrosis, suppress the renin-angiotensin-aldosterone system, and improve cardiac function in HF.
Heart failure (HF) is a major disease characterized by the progressive loss of cardiac function (1). Atrial and B-type natriuretic peptides (ANP and BNP) are key hormones in the cardiac endocrine system that preserves body fluid balance and cardiac function (2–4). ANP also acts locally in the heart to regulate cellular homeostasis, preventing cardiac hypertrophy (5–7). In failing hearts, upregulation of natriuretic peptide expression is a compensatory mechanism to counter body fluid retention and maintain cardiomyocyte morphology and function. In HF patients, elevated plasma levels of unprocessed natriuretic peptides are well documented (8, 9), suggesting that defects in natriuretic peptide processing may be an importance underlying mechanism in the pathogenesis of HF.
Corin is a transmembrane serine protease expressed in the heart and non-cardiac tissues, where it converts the ANP precursor, pro-ANP, to mature ANP (10–14). The corin function is essential for body fluid-electrolyte balance and tissue homeostasis. In mice, corin deficiency prevents ANP generation, causing sodium retention, salt-sensitive hypertension, and cardiac hypertrophy (15–17). In the pregnant uterus, corin-mediated ANP activation is a key mechanism in endometrium decidualization and spiral artery remodeling, which is essential for maternal and fetal health (18, 19). In cell-based experiments, corin also activated BNP (20–22). This function, however, is dispensable in vivo (23), where furin, a proprotein convertase, has been identified as a primary enzyme for pro-BNP processing in cardiomyocytes (20, 24, 25).
To date, variants in the NPPA gene, encoding prepro-ANP, have been shown to be a key determinant in blood pressure levels and risks for cardiovascular disease (26–29). Similarly, variants in the CORIN gene have been reported in individuals with hypertension and heart disease (30–34). In HF patients, CORIN variants with impaired activity are associated with poor clinical outcomes (35). Circulating corin levels have also been identified as biomarkers in stroke, coronary artery disease, myocardial infarction, and HF (36–46). These findings suggest that defects in corin expression and/or function may be an underlying mechanism in heart disease. It remains unclear if and to what extent corin defects may contribute to the development of HF.
In this study, we examined the role of corin deficiency in the pathogenesis of HF. We used Corin knockout (KO) mice as an animal model to examine if corin deficiency exacerbates HF, particularly under pathological conditions. We studied cardiac morphology and function in Corin KO mice at different ages or subjected to transverse aortic constriction (TAC) that induces HF. We also tested if recombinant corin could be used as a therapeutic agent to improve cardiac function in Corin KO mice. Our findings should help to define the role of corin deficiency in the pathophysiology of heart disease.
All experimental procedures conducted in this study were approved by the Animal Use and Ethics Committee of Soochow University (201603A181).
The strain of the Corin KO mice was published (17, 47). Briefly, two loxP loci were inserted in the Corin gene, flanking exon 4, to generate Corflox mice, which were crossed with CMV-Cre mice expressing Cre in all tissues. The resultant mice with the null Corin allele were verified by DNA genotyping and analysis of Corin mRNA and protein expression by RT-PCR and western blotting, respectively (17, 47). The Corin KO mice were hypertensive [systolic blood pressure: ∼118 vs. ∼107 mm Hg in wild-type (WT) mice] and bred into the C57BL/6J background, as reported previously (17, 47). High levels of blood pressure in the Corin KO mice remained similar at different ages (Supplementary Figure S1). The Corin KO and C57BL/6J WT mice were housed at a temperature controlled specific-pathogen-free facility with 12:12-h light-dark cycles and ventilated cages containing bedding materials. The mice had free access to water and a regular chow diet with 0.59% NaCl.
A TAC model was performed to induce HF in mice, as described previously (48). Briefly, Corin KO and C57BL/6 WT mice (male; 10–12 weeks old) (n = 13–15 per group) were anesthetized with isoflurane in oxygen (1.5%; flow rate of 0.3 L/min). The aortic arch and a 27-gauge needle were tied with a 7-0 silk suture at the level between the brachiocephalic trunk and the left carotid artery. As a sham control, similar surgical procedures were carried out except the suture constriction. Buprenorphine (0.1 mg/kg) was given to the mice as post-surgery analgesia up to 48 h. Echocardiography was conducted weekly to evaluate cardiac function, as described below. The experiment lasted 8 weeks.
Echocardiograph was carried out to evaluate cardiac function (49). Briefly, mice were anesthetized via inhalation of 1.5% isoflurane in oxygen (flow rate: 0.3 L/min). Transthoracic echocardiogram was conducted using VisualSonics equipment (Vevo2100) with a 30-MHz probe. Representative echocardiographic images were taken and shown in Supplementary Figure S2. Each echocardiographic measurement was done in triplicate. The data were analyzed by computer software (Vevo2100, VisualSonics). To assess cardiac hypertrophy, isolated hearts were weighed, and the data were normalized to body weights and tibia lengths.
Mice were euthanized by exsanguination after isoflurane inhalation. Blood samples were collected in anti-coagulated tubes and centrifuged at 2,095g for 10 min at room temperature to obtain plasma. Tissues, including hearts and lungs, were dissected, weighed, and used for gene expression studies. For histological analysis, the tissues were treated with 4% (v/v) paraformaldehyde and embedded in paraffin. Tissue sections at 4 μm in thickness were prepared and stained with hematoxylin and eosin (H&E) (for general histology), rhodamine-conjugated wheat germ agglutinin (WGA) (Vector Laboratories, RL-1022-5) (for cardiac sarcolemma) or Sirius red and Masson's trichrome (Solarbio, G1340) (for fibrosis). To examine cardiomyocyte sizes, five randomly selected fields in each WGA-stained section were analyzed in a blinded manner. At least three sections were examined for each mouse. Diameters of at least 200 cardiomyocytes at short axis were measured at the nucleus plane with Image-Pro-Plus software (48). To evaluate cardiac fibrosis, percentages of Masson's trichrome-positive area in left ventricular (LV) sections were analyzed using Image-Pro-Plus software (Media Cybernetics, V6.0). To analyze lung edema, isolated lungs were weighed, and the data were normalized to body weights and tibia lengths. Lung sections were stained with Prussian blue (BBI Life Sciences, e670108) to assess iron-containing macrophages.
To test the effect of exogenous corin on cardiac function in Corin KO mice, a soluble form of corin (sCorin) was prepared, consisting of the entire extracellular region of human corin (residues 124-1042) and a C-terminal V5 tag (48). sCorin also contained an engineered zymogen activation site that can be cleaved by enterokinase (EK) (48). sCorin was expressed in Chinese hamster ovary (CHO)-K1 cells (NingBoMingZhou Tech, China) and purified from the conditioned medium with an affinity column, as described previously (48). The purified sCorin was quantified using a Bradford assay kit (Thermo Fisher Scientific, 23236) and activated by recombinant EK (BBI Life Sciences, C620005) (48). Corin KO mice were subjected to TAC or sham procedures. After 1 week, the mice with the TAC procedure were injected with sCorin (3 mg/kg, i.p., daily) or equal volume of vehicle (Supplementary Figure S3). Cardiac function was assessed weekly by echocardiography. As reported previously (48), the intraperitoneal injection resulted in >20 µg/ml of sCorin in plasma with a half-life >8 h. After 7 weeks, the mice were euthanized. Blood and tissue samples were used for further gene and protein analyses.
At 8 weeks post-TAC or sham operation, LV tissues were isolated from the mice without or with sCorin or vehicle treatment. Total RNAs were isolated from tissue homogenates with Trizol reagents (Ambion, 15596018) and used to make cDNAs using reverse transcriptase (Thermo Fisher Scientific, K1622). Quantitative RT-PCR (Thermo Fisher Scientific, 4485689) was used to examine expression levels of selected genes, including Nppa (encoding the ANP precursor), Nppb (encoding the BNP precursor), Myh7 (encoding myosin heavy chain-β), and Ctgf (encoding connective tissue growth factor). The sequences of the oligonucleotide primers used in the RT-PCR are listed in Supplementary Table S1.
Plasma samples were prepared from the mice 8 weeks after sham or TAC procedures. Plasma levels of cGMP (Enzo Life Sciences, ADI-900-013; Shanghai MLBIO Biotechnology, ml001887), ANP (Shanghai Animalunion Biotechnology, LV30662), N-terminal (NT)-pro-ANP (Cloud-Clone Corp, SEA484Mu), angiotensin II (RayBio, EIAM-ANGII-1), and aldosterone (Elabscience, E-EL-0070c) were examined by ELISA. In the NT-pro-ANP ELISA, the antibody was raised against a pro-ANP fragment (Asn25-Arg122). Experimental procedures for the ELISA measurements were based on manufacturers' instructions.
Statistical analysis was done using Graphpad Prism 8.0 software (San Diego, CA, USA). Data were tested for normality and equal variance with Anderson-Darling, D'Agostino–Pearson, Kolmogorov–Smirnov, and Shapiro–Wilk tests. If passed, comparisons were done with Student's t test between two groups or one-way ANOVA followed by Tukey's post hoc analysis among three or more groups. Otherwise, the Kruskal–Wallis test was used. Quantitative data are presented in mean ± SEM. P values <0.05 were considered as statistically significant.
To examine the effect of corin deficiency on cardiac morphology and function, we performed echocardiography in WT and Corin KO mice at different ages. When tested between 3 and 9 months of age, male WT and Corin KO mice had similar values in parameters of cardiac function, including ejection fraction (EF) (Figure 1A), fractional shortening (FS) (Figure 1B), LV end diastolic dimension (LVEDD) (Figure 1C), LV end systolic dimension (LVESD) (Figure 1D), and LV mass (Figure 1E). When tested at 12 and 15 months of age, male WT mice had no apparent decline in cardiac function, whereas male Corin KO mice had reduced EF and FS and increased LVEDD, LVESD, and LV mass, compared to those in Corin KO mice at younger ages or in WT mice at similar ages (Figures 1A–E). Similar findings were also observed in female WT and Corin KO mice (Supplementary Figure S4), indicating that the effect of corin deficiency on cardiac function is gender independent.
Figure 1. Cardiac function in WT and Corin KO mice at different ages. Echocardiography was conducted to examine ejection fraction (EF) (A), fractional shortening (FS) (B), left ventricular end diastolic dimension (LVEDD) (C), LV end systolic dimension (LVESD) (D), and LV mass (E) in male WT and Corin KO mice between 3 and 15 months of age. Data are mean ± SEM; n = 8–23 per group. P values were analyzed by one-way ANOVA and Tukey's post hoc analysis (A–C,E) or Kruslal-Wallis test (D). ns, not significant.
In tissue analysis, hearts from 15-month-old Corin KO mice appeared larger (Figure 2A) and were heavier than those from age-matched WT mice, as indicated by greater heart weights (HW), normalized to body weights (BW) (Figure 2B) or tibia lengths (TL) (Figure 2C) (Supplementary Table S2). In H&E- and WGA-stained heart sections, cardiomyocytes from the Corin KO mice were larger in diameters, compared to those from WT mice (Figures 2D,E). Sirius red staining revealed increased fibrosis in heart sections from the Corin KO mice, whereas no apparent fibrosis was detected in heart sections from the aged-matched WT mice (Figures 2F,G). These results indicate that Corin KO mice at 12 months of age or older developed cardiac hypertrophy, cardiac fibrosis, and HF.
Figure 2. Cardiac hypertrophy and fibrosis in Corin KO mice. Hearts were isolated from 15-month-old male WT and Corin KO mice. Tissue sections were stained with hematoxylin and eosin (H&E), wheat germ agglutinin (WGA), or Sirius red. (A) Representative pictures of hearts and H&E-stained sections from WT and Corin KO mice are shown. (B,C) Heart weights (HW) were normalized to body weights (BW) (B) or tibia length (TL) (C). (D,E) Cardiomyocyte diameters in H&E- or WGA-stained LV sections were measured at the nucleus plane in 200 individual cells randomly selected from three sections. At least three sections per mouse were analyzed. (F,G) Connective tissues (purple) in at least five randomly selected fields from Sirius red-stained LV sections were analyzed. Percentages of fibrotic area were calculated using Image-Pro-Plus software. Scale bars are indicated. Quantitative data are mean ± SEM analyzed by Student's t test.
HF may result from many pathological insults. To examine if corin deficiency exacerbates HF under pathological conditions, we tested a HF model induced by TAC in 10–12-week-old WT and Corin KO mice (Supplementary Figure S2). By echocardiography, we found that cardiac function decreased progressively after WT and Corin KO mice were subjected to TAC, whereas cardiac function remained unchanged in the corresponding mice subjected to sham operation (Figure 3A) (Supplementary Figure S5). The rate of the decline was much faster in the Corin KO than WT mice after TAC; the times for EF to fall below 50% was 3.9 ± 0.1 and 6.0 ± 0.2 weeks (n = 8, P < 0.001) in the Corin KO and WT mice, respectively (Figure 3A). At 8 weeks post-TAC, the Corin KO mice had markedly reduced EF (Figure 3B) and FS (Figure 3C) and increased LVEDD (Figure 3D) and LVESD (Figure 3E), compared to those in the Corin KO mice at the baseline or 8 weeks post-sham operation. In tissue and histological examinations, hearts isolated from the Corin KO mice at 8 weeks post-TAC were larger (Figures 4A,B) and heavier (Figures 4C,D) (Supplementary Table S3), compared with those from the corresponding sham-operated Corin KO mice or the TAC-operated WT mice (Supplementary Figure S6). These results indicate that corin deficiency accelerated cardiac hypertrophy and HF in mice upon aortic constriction.
Figure 3. Cardiac function in Corin KO mice subjected to TAC. TAC was done in 10–12-week-old male WT and Corin KO mice (n = 8 per group). Cardiac function was assessed weekly with echocardiography. (A) EF in sham- or TAC-operated WT and Corin KO mice after the surgery. Comparisons were done by two-way ANOVA and Tukey's post hoc analysis between sham- or TAC-operated WT or Corin KO mice and between TAC-operated WT and Corin KO mice. Data are mean ± SEM; n = 8 per group. Times when EF values were 50% (t50%) in WT (black) and Corin KO (red) mice were calculated. (B–E) EF (B), FS (C), LVEDD (D), and LVESD (E) in sham- or TAC-operated Corin KO mice before (baseline) and 8 weeks post-surgery were examined by one-way ANOVA and Tukey's post hoc analysis. Data are mean ± SEM; n = 8 per group.
Figure 4. Cardiac hypertrophy in TAC-operated Corin KO mice. Hearts were isolated from sham- or TAC-operated Corin KO mice at 8 weeks post-surgery. Tissue sections were stained with H&E. (A) Representative pictures of hearts and H&E-stained sections from the sham- and TAC-operated Corin KO mice are shown. (B) Heart section areas were analyzed by Image-Pro-Plus software. (C,D) Values of HW were normalized to BW (C) or TL (D). Data in B–D are mean ± SEM analyzed by Student's t test.
Previously, transgenic Corin expression in the heart or recombinant corin protein administration improved cardiac function in mouse models of HF (48, 50). We tested if a similar approach could improve cardiac function in Corin KO mice with TAC. We performed TAC or sham procedures in 10–12-week-old Corin KO mice, which had normal cardiac function, followed by weekly peritoneal injection of vehicle or a soluble form of corin (sCorin) consisting of the full length extracellular region of corin (48) (Supplementary Figure S3). The sCorin injection increased plasma ANP levels (Supplementary Figure S7). As indicated by echocardiographic measurements, including EF (Figure 5A), FS (Figure 5B), LVEDD (Figure 5C), LV end diastolic volume (LVEDV) (Figure 5D), LVESD (Figure 5E), and LV end systolic volume (LVESV) (Figure 5F), cardiac function declined progressively in the Corin KO mice subjected to TAC, compared to those in the sham-operated controls. After 7 weeks of sCorin injection, cardiac function was improved in the TAC-operated Corin KO mice, compared to that in the vehicle-treated corresponding controls (Figures 5A–F).
Figure 5. Cardiac function in TAC-operated Corin KO mice treated with sCorin. Corin KO mice (male, 10–12 weeks old) were subjected to sham or TAC operation (n = 13–15 per group). The TAC-operated mice were treated with a control vehicle (TAC + Veh) or sCorin (TAC + sCorin), starting at 1-week post-surgery. Cardiac function was assessed weekly with echocardiography. Data of EF (A), FS (B), LVEDD (C), LV end diastolic volume (LVEDV) (D), LVESD (E), and LV end systolic volume (LVESV) (F) were analyzed by one-way ANOVA and Tukey's post hoc analysis.
Analysis of hearts isolated at 8 weeks post-TAC showed that cardiac hypertrophy occurred in the TAC-operated Corin KO mice, compared to the sham-operated mice (Figures 6A–D). In the TAC-operated Corin KO mice that received sCorin injection, cardiac hypertrophy was markedly reduced, as indicated by smaller hearts (Figures 6A,B) and reduced ratios of HW to BW (Figure 6C) or TL (Figure 6D) (Supplementary Table S4), compared to those in the TAC-operated Corin KO mice receiving the vehicle.
Figure 6. Increased heart size and weight in TAC-operated Corin KO mice treated with sCorin. Hearts were isolated from the sham- or TAC-operated Corin KO mice with vehicle (TAC + Veh) or sCorin (TAC + sCorin) treatment at 8 weeks post-surgery. Tissue sections were stained with H&E. (A) Representative pictures of hearts and H&E-stained sections from the sham- and TAC-operated Corin KO mice are shown. (B) Heart section areas were analyzed by Image-Pro-Plus software. (C,D) Values of HW were normalized to BW (C) or TL (D). Data in B–D are mean ± SEM analyzed by one-way ANOVA and Tukey's post hoc analysis.
Analysis of H&E- and WGA-stained heart sections revealed that cardiomyocytes in the TAC-operated Corin KO mice were larger in diameters, compared to those in the sham-operated Corin KO mice (Figures 7A,B). In the TAC-operated Corin KO mice with sCorin treatment, cardiomyocyte diameters were reduced, compared to those in the TAC-operated Corin KO mice with vehicle treatment (Figures 7A,B). Similarly, analysis of Sirius red- or Masson's trichrome-stained heart sections revealed increased fibrosis in the TAC-, but not sham-, operated Corin KO mice (Figures 7C,D). In the TAC-operated Corin KO mice with sCorin injection, the area of fibrosis was markedly reduced, compared to that in the vehicle-treated mice (Figures 7C,D). These results indicate that administration of exogenous corin protein reduced cardiac hypertrophy and fibrosis and improved cardiac function in the Corin KO mice subjected to TAC.
Figure 7. Cardiac hypertrophy and fibrosis in TAC-operated Corin KO mice treated with sCorin. Corin KO mice (male, 10–12 weeks old) were subjected to sham or TAC operation. The TAC-operated Corin KO mice were treated with a control vehicle (TAC + Veh) or sCorin (TAC + sCorin). Hearts were isolated at 8 weeks post-surgery (n = 7 per group). (A,B) To assess cardiac hypertrophy, heart sections were stained with H&E and WGA (A). Diameters of at least 200 cardiomyocytes in randomly selected LV sections were measured at the nucleus plane (B). At least three sections per mouse were analyzed. (C,D) To assess cardiac fibrosis, heart sections were stained with Sirius red and Masson's trichrome (C). Fibrotic areas in at least three randomly selected LV section fields were analyzed by Image-Pro-Plus software (D). The data in (B,D) were analyzed by one-way ANOVA and Tukey's post hoc analysis.
To verify our findings, we analyzed a set of selected genes associated with cardiac hypertrophy and fibrosis in the Corin KO mice subjected to TAC. By quantitative RT-PCR, we found that hearts from the TAC-operated Corin KO mice had increased levels of Nppa, Nppb, Myh7, which were associated cardiac hypertrophy, and Ctgf, which was associated with cardiac fibrosis, compared with those in the sham-operated Corin KO mice (Figures 8A–D). The expression levels of these genes were reduced after the TAC-operated Corin KO mice received the sCorin treatment (Figures 8A–D). These results are consistent with the findings that the sCorin treatment reduced cardiac hypertrophy and fibrosis in the Corin KO mice subjected to TAC.
Figure 8. Cardiac gene expression in TAC-operated Corin KO mice treated with sCorin. Hearts were isolated from the sham- or TAC-operated Corin KO mice with vehicle (TAC + Veh) or sCorin (TAC + sCorin) treatment at 8 weeks post-surgery. Quantitative RT-PCR was done to assess expression levels of Nppa (A), Nppb (B), Myh7 (C), and Ctgf (D), which are associated with cardiac hypertrophy and fibrosis (n = 5 per group). Data were analyzed by one-way ANOVA and Tukey's post hoc analysis.
We next measured plasma cGMP levels, an indicator of natriuretic peptide activity. We found reduced plasma cGMP levels in the TAC-operated Corin KO mice, compared to those in the sham-operated Corin KO mice (Figure 9A). The levels were increased after the TAC-operated Corin KO mice were treated with sCorin. In contrast, levels of plasma NT-pro-ANP, angiotensin II, and aldosterone were increased in the TAC-operated Corin KO mice, compared with those in the sham-operated mice. The levels were decreased after the TAC-operated Corin KO mice were treated with sCorin (Figures 9B–D). These results are consistent, indicating that the TAC-operated Corin KO mice had increased Nppa expression, impaired natriuretic peptide processing, and enhanced angiotensin and aldosterone levels and that such a phenotype was ameliorated after the sCorin treatment.
Figure 9. Changes of plasma factor levels in TAC-operated Corin KO mice treated with sCorin. Plasma samples were isolated from the sham- or TAC-operated Corin KO mice with vehicle (TAC + Veh) or sCorin (TAC + sCorin) treatment at 8 weeks post-surgery. Levels of cGMP (A), N-terminal (NT)-pro-ANP (B), angiotensin II (C), and aldosterone (D) were measured by ELISA (n = 13–15 per group). Data were analyzed by one-way ANOVA and Tukey's post hoc analysis.
Lung congestion and edema are common pathological features in HF. Compared to the sham-operated mice, the TAC-operated Corin KO mice had increased lung weights (LW), normalized to BW or TL (Figures 10A,B). By Prussian blue staining for macrophages containing hemosiderin, we found more blue spots in lung sections from the TAC-operated Corin KO mice (Figures 10C,D). The lung weights and Prussian blue staining were reduced in the TAC-operated Corin KO mice after the sCorin treatment (Figures 10C,D). These results indicate that TAC-induced HF resulted in lung edema in the TAC-operated Corin KO mice, which was ameliorated after the sCorin treatment.
Figure 10. Analysis of lungs from TAC-operated Corin KO mice treated with sCorin. Lungs were isolated from the sham- or TAC-operated Corin KO mice with vehicle (TAC + Veh) or sCorin (TAC + sCorin) treatment at 8 weeks post-surgery. Lung weights (LW) were normalized to BW (A) or TL (B) (n = 13–15 per group). Lung sections were stained with Prussian blue for macrophages containing hemosiderin (blue dots) (C). Blue dots in at least three randomly selected fields from at least three sections per mouse were counted (n = 7 mice per group). Data in (A,B,D) were analyzed by one-way ANOVA and Tukey's post hoc analysis.
Hypertension is an important risk factor in the pathogenesis of HF (1, 51). Corin and ANP-mediated signaling is critical for preserving normal blood pressure. Deficiencies in Corin and Nppa genes cause salt-sensitive hypertension in mice (17, 52–54). In this study, we examined to what extent corin deficiency may impair cardiac function and morphology in mice with a null Corin allele. We found that the Corin KO mice had normal cardiac function up to 9 months of age, after which cardiac function declined progressively. The abnormal cardiac function was associated with cardiac hypertrophy and fibrosis, as indicated by echocardiography and histological analysis of heart tissues in the Corin KO mice of 12–15 months of age. In the Corin KO mice, blood pressure levels were continuously high, as measured between 3 and 15 months of age. These findings suggest that impaired cardiac function and morphology in the aged Corin KO mice may result primarily from chronic pressure overload, but not intrinsic structural defects in the heart. Previously, corin was reported to protect against ischemia and oxidative stress-induced apoptosis in cultured cardiomyocytes and neuronal cells (55, 56). Based on the findings in our study, it seems unlikely that enhanced cardiomyocyte apoptosis is a major cellular event in the Corin KO mice, at least up to 9 months of age without additional pathological challenges.
Consistent with the findings in the aged Corin KO mice, we found that TAC, which causes pressure overload (57, 58), led to a marked decline in cardiac function in 10–12-week-old Corin KO mice, indicating that corin deficiency exacerbates HF development upon aortic constriction. Previously, a strain of mast cell-deficient mice, i.e., C57BL/6-KitW−sh (Wsh) mice, was reported to contain an inversion breakpoint in a genetic locus containing Kit, Corin, Pdgfra (encoding platelet-derived growth factor receptor-α), and other genes (59). The Wsh mice also exhibited a phenotype of cardiac hypertrophy (59) and, when subjected to TAC, a rapid decline in cardiac function (60), resembling our findings in Corin KO mice. Unlike in the Corin KO mice subjected to TAC, however, there was no detectable cardiac fibrosis in the Wsh mice after TAC (60). Mast cells are known to produce many proteases, cytokines, and growth factors that are important in immune responses, inflammation, and tissue homeostasis (61, 62). It remains to be determined if some of those mast cell-derived molecules are involved in tissue remodeling and cardiac fibrosis in failing hearts, which may account for the apparent difference in cardiac fibrosis between the mast cell-deficient Wsh mice and the Corin KO mice subjected to TAC.
Genetic variants that impair corin expression and/or function have been reported in patients with hypertension and cardiovascular disease (30–34). A CORIN variant allele (T555I/Q568P), for example, has been identified in ∼10%–12% African Americans (63, 64), a population with a high prevalence for hypertension and heart disease (65). Individuals with this CORIN allele had an increased cardiac hypertrophic response to pressure overload, as indicated by greater LV mass normalized to fat-free mass or body surface area as a function of systolic blood pressure (66, 67). In cell-based experiments, the corin variant T555I/Q568P was defective in proprotein convertase subtilisin/kexin-6 mediated zymogen activation and pro-ANP/pro-BNP processing (23, 68). In a transgenic model, mice expressing the corin variant also exhibited cardiac hypertrophy that was exacerbated by high-salt diets or during pregnancy (49, 52). These results are consistent with our findings in this study, suggesting that individuals with corin defects may be more susceptible to developing cardiac hypertrophy and HF, particularly in the presence of additional pathological challenges.
In patients with late stages of HF, body fluid retention is common, as manifested by shortness of breath, orthopnea, and low leg swelling (1). Approaches to enhance natriuretic peptide activity or to reduce natriuretic peptide degradation have been used to improve body fluid balance in individuals with failing hearts (69–72). In mouse models of myocardial infarction and cardiomyopathy, transgenic corin expression in the heart decreased cardiac dysfunction, lowered edema, and improved survival (50, 73, 74). Similarly, our recent studies also found that in WT mice, cardiac hypertrophy and dysfunction induced by left coronary artery ligation or TAC were ameliorated by administration of recombinant sCorin (48). In this study, we showed that sCorin injection improved cardiac function, reduced cardiac hypertrophy and fibrosis, and lessened lung edema in the Corin KO mice subjected to TAC. Moreover, the sCorin treatment also led to changes in cardiac gene expression and circulating factors, including the reduced levels of Nppa, Nppb, Myh7 and Cfgf expression in the heart, increased plasma cGMP levels, and suppressed plasma levels of NT-pro-ANP, angiotensin II, and aldosterone in these mice. These findings are consistent, indicating the improved cardiac morphology and function, which are associated with enhanced natriuretic peptide processing and signaling and suppressed renin-angiotensin-aldosterone system in the Corin KO mice treated with sCorin. In patients with HF, impaired natriuretic peptide processing is common, as indicated by elevated levels of circulating pro-ANP and pro-BNP (8, 9). Together, these results suggest that strategies to enhance corin activity may be explored to improve cardiac function in HF patients, particularly those with deficiencies in corin expression and/or activity.
In conclusion, we showed that the Corin KO mice had cardiac dysfunction beyond 12 months of age, which was likely caused by chronic hypertension. When subjected to TAC at a young age, the Corin KO mice exhibited worsening cardiac hypertrophy, cardiac fibrosis, HF, and lung edema, indicating that corin deficiency exacerbated HF development in the presence of additional pathological challenges. In the Corin KO mice subjected to TAC, administration of recombinant corin protein enhanced natriuretic peptide activity, suppressed the renin-angiotensin-aldosterone system, improved cardiac morphology and function, and lessened lung edema. These results indicate that corin deficiency is an underlying factor in the pathogenesis of HF, suggesting that recombinant corin protein may be used to improve the function of failing hearts in patients with impaired natriuretic peptide processing.
The original contributions presented in the study are included in the article/Supplementary Material, further inquiries can be directed to the corresponding authors.
The animal study was reviewed and approved by the Animal Use and Ethics Committee of Soochow University.
ND and QW contributed to the conception and research design. YN, TZ, SZ, WL, and KW contributed to the experiments. YN, TZ, ND, and QW contributed to data analysis. TZ and ND contributed to funding. YN, TZ, ND, and QW contributed to manuscript writing and revision. All authors contributed to the article and approved the submitted version.
This work was supported in part by grants from the National Natural Science Foundation of China (32171112 to ND and 31500636 to TZ) and the Priority Academic Program Development of Jiangsu Higher Education Institutes (to Soochow University).
QW is an inventor on several corin-related patents that are owned by Bayer Healthcare. QW does not own Bayer stock and has not and will not receive any royalties from those patents.
The remaining authors declare that the research was conducted in the absence of any commercial or financial relationships that could be construed as a potential conflict of interest.
All claims expressed in this article are solely those of the authors and do not necessarily represent those of their affiliated organizations, or those of the publisher, the editors and the reviewers. Any product that may be evaluated in this article, or claim that may be made by its manufacturer, is not guaranteed or endorsed by the publisher.
The Supplementary Material for this article can be found online at: https://www.frontiersin.org/articles/10.3389/fcvm.2023.1164524/full#supplementary-material
1. Metra M, Teerlink JR. Heart failure. Lancet. (2017) 390(10106):1981–95. doi: 10.1016/S0140-6736(17)31071-1
2. Goetze JP, Bruneau BG, Ramos HR, Ogawa T, de Bold MK, de Bold AJ. Cardiac natriuretic peptides. Nat Rev Cardiol. (2020) 17(11):698–717. doi: 10.1038/s41569-020-0381-0
3. Nishikimi T, Kato J. Cardiac peptides-current physiology, pathophysiology, biochemistry, molecular biology, and clinical application. Biology. (2022) 11(2):330. doi: 10.3390/biology11020330
4. Matsuo A, Nagai-Okatani C, Nishigori M, Kangawa K, Minamino N. Natriuretic peptides in human heart: novel insight into their molecular forms, functions, and diagnostic use. Peptides. (2019) 111:3–17. doi: 10.1016/j.peptides.2018.08.006
5. Forte M, Marchitti S, Di Nonno F, Stanzione R, Schirone L, Cotugno M, et al. NPPA/atrial natriuretic peptide is an extracellular modulator of autophagy in the heart. Autophagy. (2022) 19:1087–99. doi: 10.1080/15548627.2022.2115675
6. Song W, Wang H, Wu Q. Atrial natriuretic peptide in cardiovascular biology and disease (NPPA). Gene. (2015) 569(1):1–6. doi: 10.1016/j.gene.2015.06.029
7. Kuhn M. Cardiac actions of atrial natriuretic peptide: new visions of an old friend. Circ Res. (2015) 116(8):1278–80. doi: 10.1161/CIRCRESAHA.115.306325
8. Xu-Cai YO, Wu Q. Molecular forms of natriuretic peptides in heart failure and their implications. Heart. (2010) 96(6):419–24. doi: 10.1136/hrt.2008.164145
9. Davidovski FS, Goetze JP. ProANP and proBNP in plasma as biomarkers of heart failure. Biomark Med. (2019) 13(13):1129–35. doi: 10.2217/bmm-2019-0158
10. Yan W, Sheng N, Seto M, Morser J, Wu Q. Corin, a mosaic transmembrane serine protease encoded by a novel cDNA from human heart. J Biol Chem. (1999) 274(21):14926–35. doi: 10.1074/jbc.274.21.14926
11. Yan W, Wu F, Morser J, Wu Q. Corin, a transmembrane cardiac serine protease, acts as a pro-atrial natriuretic peptide-converting enzyme. Proc Natl Acad Sci U S A. (2000) 97(15):8525–9. doi: 10.1073/pnas.150149097
12. Dong N, Niu Y, Chen Y, Sun S, Wu Q. Function and regulation of corin in physiology and disease. Biochem Soc Trans. (2020) 48(5):1905–16. doi: 10.1042/BST20190760
13. Hooper JD, Scarman AL, Clarke BE, Normyle JF, Antalis TM. Localization of the mosaic transmembrane serine protease corin to heart myocytes. Eur J Biochem. (2000) 267(23):6931–7. doi: 10.1046/j.1432-1033.2000.01806.x
14. Ichiki T, Huntley BK, Heublein DM, Sandberg SM, McKie PM, Martin FL, et al. Corin is present in the normal human heart, kidney, and blood, with pro-B-type natriuretic peptide processing in the circulation. Clin Chem. (2011) 57(1):40–7. doi: 10.1373/clinchem.2010.153908
15. Chan JC, Knudson O, Wu F, Morser J, Dole WP, Wu Q. Hypertension in mice lacking the proatrial natriuretic peptide convertase corin. Proc Natl Acad Sci U S A. (2005) 102(3):785–90. doi: 10.1073/pnas.0407234102
16. Wang W, Shen J, Cui Y, Jiang J, Chen S, Peng J, et al. Impaired sodium excretion and salt-sensitive hypertension in corin-deficient mice. Kidney Int. (2012) 82(1):26–33. doi: 10.1038/ki.2012.41
17. Zhou T, Zhang S, Du C, Wang K, Gu X, Sun S, et al. Renal corin is essential for normal blood pressure and sodium homeostasis. Int J Mol Sci. (2022) 23(19):11251. doi: 10.3390/ijms231911251
18. Cui Y, Wang W, Dong N, Lou J, Srinivasan DK, Cheng W, et al. Role of corin in trophoblast invasion and uterine spiral artery remodelling in pregnancy. Nature. (2012) 484(7393):246–50. doi: 10.1038/nature10897
19. Zhang W, Li S, Lou J, Li H, Liu M, Dong N, et al. Atrial natriuretic peptide promotes uterine decidualization and a TRAIL-dependent mechanism in spiral artery remodeling. J Clin Invest. (2021) 131(20):e151053. doi: 10.1172/JCI151053
20. Nishikimi T, Nakagawa Y, Minamino N, Ikeda M, Tabei K, Fujishima A, et al. Pro-B-type natriuretic peptide is cleaved intracellularly: impact of distance between O-glycosylation and cleavage sites. Am J Physiol Regul Integr Comp Physiol. (2015) 309(6):R639–49. doi: 10.1152/ajpregu.00074.2015
21. Ichiki T, Huntley BK, Burnett JC Jr. BNP molecular forms and processing by the cardiac serine protease corin. Adv Clin Chem. (2013) 61:1–31. doi: 10.1016/B978-0-12-407680-8.00001-4
22. Semenov AG, Tamm NN, Seferian KR, Postnikov AB, Karpova NS, Serebryanaya DV, et al. Processing of pro-B-type natriuretic peptide: furin and corin as candidate convertases. Clin Chem. (2010) 56(7):1166–76. doi: 10.1373/clinchem.2010.143883
23. Chen S, Cao P, Dong N, Peng J, Zhang C, Wang H, et al. PCSK6-mediated corin activation is essential for normal blood pressure. Nat Med. (2015) 21(9):1048–53. doi: 10.1038/nm.3920
24. Fu S, Ping P, Zhu Q, Ye P, Luo L. Brain natriuretic peptide and its biochemical, analytical, and clinical issues in heart failure: a narrative review. Front Physiol. (2018) 9:692. doi: 10.3389/fphys.2018.00692
25. Sawada Y, Suda M, Yokoyama H, Kanda T, Sakamaki T, Tanaka S, et al. Stretch-induced hypertrophic growth of cardiocytes and processing of brain-type natriuretic peptide are controlled by proprotein-processing endoprotease furin. J Biol Chem. (1997) 272(33):20545–54. doi: 10.1074/jbc.272.33.20545
26. Newton-Cheh C, Larson MG, Vasan RS, Levy D, Bloch KD, Surti A, et al. Association of common variants in NPPA and NPPB with circulating natriuretic peptides and blood pressure. Nat Genet. (2009) 41(3):348–53. doi: 10.1038/ng.328
27. Cannone V, Huntley BK, Olson TM, Heublein DM, Scott CG, Bailey KR, et al. Atrial natriuretic peptide genetic variant rs5065 and risk for cardiovascular disease in the general community: a 9-year follow-up study. Hypertension. (2013) 62(5):860–5. doi: 10.1161/HYPERTENSIONAHA.113.01344
28. Barbato E, Bartunek J, Mangiacapra F, Sciarretta S, Stanzione R, Delrue L, et al. Influence of rs5065 atrial natriuretic peptide gene variant on coronary artery disease. J Am Coll Cardiol. (2012) 59(20):1763–70. doi: 10.1016/j.jacc.2012.02.017
29. Pereira NL, Tosakulwong N, Scott CG, Jenkins GD, Prodduturi N, Chai Y, et al. Circulating atrial natriuretic peptide genetic association study identifies a novel gene cluster associated with stroke in whites. Circ Cardiovasc Genet. (2015) 8(1):141–9. doi: 10.1161/CIRCGENETICS.114.000624
30. Chen YL, Li TJ, Hao Y, Wu BG, Li H, Geng N, et al. Association of rs2271037 and rs3749585 polymorphisms in CORIN with susceptibility to hypertension in a Chinese Han population: a case-control study. Gene. (2018) 651:79–85. doi: 10.1016/j.gene.2018.01.080
31. Zhang Y, Zhou T, Niu Y, He M, Wang C, Liu M, et al. Identification and functional analysis of CORIN variants in hypertensive patients. Hum Mutat. (2017) 38(12):1700–10. doi: 10.1002/humu.23318
32. Dong N, Fang C, Jiang Y, Zhou T, Liu M, Zhou J, et al. Corin mutation R539C from hypertensive patients impairs zymogen activation and generates an inactive alternative ectodomain fragment. J Biol Chem. (2013) 288(11):7867–74. doi: 10.1074/jbc.M112.411512
33. Zhao Y, Yuan X, Zhong Y, Zhang Y, Zhang S, Li S, et al. Single-nucleotide polymorphisms in the 3’ untranslated region of CORIN associated with cardiovascular diseases in a Chinese Han population: a case-control study. Front Cardiovasc Med. (2021) 8:625072. doi: 10.3389/fcvm.2021.625072
34. Dong N, Zhou T, Zhang Y, Liu M, Li H, Huang X, et al. Corin mutations K317E and S472G from preeclamptic patients alter zymogen activation and cell surface targeting. J Biol Chem. (2014) 289(25):17909–16. doi: 10.1074/jbc.M114.551424
35. Rame JE, Tam SW, McNamara D, Worcel M, Sabolinski ML, Wu AH, et al. Dysfunctional corin i555(p568) allele is associated with impaired brain natriuretic peptide processing and adverse outcomes in blacks with systolic heart failure: results from the genetic risk assessment in heart failure substudy. Circ Heart Fail. (2009) 2(6):541–8. doi: 10.1161/CIRCHEARTFAILURE.109.866822
36. Chen L, Zhang Q, Zhang M, Yu J, Ren L, Li J, et al. Soluble corin predicts the risk of cardiovascular disease: a 10-year follow-up study. JACC Asia. (2022) 2(4):490–501. doi: 10.1016/j.jacasi.2022.01.004
37. Peng H, Zhu F, Shi J, Han X, Zhou D, Liu Y, et al. Serum soluble corin is decreased in stroke. Stroke. (2015) 46(7):1758–63. doi: 10.1161/STROKEAHA.114.008368
38. Gommans DHF, Revuelta-Lopez E, Lupon J, Cserkóová A, Domingo M, Vart P, et al. Soluble neprilysin and corin concentrations in relation to clinical outcome in chronic heart failure. JACC Heart Fail. (2021) 9(2):85–95. doi: 10.1016/j.jchf.2020.08.015
39. Yu R, Han X, Zhang X, Wang Y, Wang T. Circulating soluble corin as a potential biomarker for cardiovascular diseases: a translational review. Clin Chim Acta. (2018) 485:106–12. doi: 10.1016/j.cca.2018.06.036
40. Dong N, Chen S, Wang W, Zhou Y, Wu Q. Corin in clinical laboratory diagnostics. Clin Chim Acta. (2012) 413(3-4):378–83. doi: 10.1016/j.cca.2011.10.032
41. Ibebuogu UN, Gladysheva IP, Houng AK, Reed GL. Decompensated heart failure is associated with reduced corin levels and decreased cleavage of pro-atrial natriuretic peptide. Circ Heart Fail. (2011) 4(2):114–20. doi: 10.1161/CIRCHEARTFAILURE.109.895581
42. Dong N, Chen S, Yang J, He L, Liu P, Zheng D, et al. Plasma soluble corin in patients with heart failure. Circ Heart Fail. (2010) 3(2):207–11. doi: 10.1161/CIRCHEARTFAILURE.109.903849
43. Yang SF, Chou RH, Li SY, Huang SS, Huang PH. Serum corin level is associated with subsequent decline in renal function in patients with suspected coronary artery disease. J Am Heart Assoc. (2018) 7(10):e008157. doi: 10.1161/JAHA.117.008157
44. Wang D, Gladysheva IP, Sullivan RD, Fan TM, Mehta RM, Tripathi R, et al. Increases in plasma corin levels following experimental myocardial infarction reflect the severity of ischemic injury. PLoS One. (2018) 13(9):e0202571. doi: 10.1371/journal.pone.0202571
45. Zhou X, Chen J, Zhang Q, Shao J, Du K, Xu X, et al. Prognostic value of plasma soluble corin in patients with acute myocardial infarction. J Am Coll Cardiol. (2016) 67(17):2008–14. doi: 10.1016/j.jacc.2016.02.035
46. Lu Y, Wang W, Tang Z, Chen L, Zhang M, Zhang Q, et al. A prediction model for rapid identification of ischemic stroke: application of serum soluble corin. J Multidiscip Healthc. (2022) 15:2933–43. doi: 10.2147/JMDH.S395896
47. He M, Zhou T, Niu Y, Feng W, Gu X, Xu W, et al. The protease corin regulates electrolyte homeostasis in eccrine sweat glands. PLoS Biol. (2021) 19(2):e3001090. doi: 10.1371/journal.pbio.3001090
48. Niu Y, Zhang S, Gu X, Zhou T, Li F, Liu M, et al. Recombinant soluble corin improves cardiac function in mouse models of heart failure. J Am Heart Assoc. (2021) 10(7):e019961. doi: 10.1161/JAHA.120.019961
49. Baird RC, Li S, Wang H, Naga Prasad SV, Majdalany D, Perni U, et al. Pregnancy-associated cardiac hypertrophy in corin-deficient mice: observations in a transgenic model of preeclampsia. Can J Cardiol. (2019) 35(1):68–76. doi: 10.1016/j.cjca.2018.11.001
50. Gladysheva IP, Wang D, McNamee RA, Houng AK, Mohamad AA, Fan TM, et al. Corin overexpression improves cardiac function, heart failure, and survival in mice with dilated cardiomyopathy. Hypertension. (2013) 61(2):327–32. doi: 10.1161/HYPERTENSIONAHA.112.193631
51. Carretero OA, Oparil S. Essential hypertension. Part I: definition and etiology. Circulation. (2000) 101(3):329–35. doi: 10.1161/01.CIR.101.3.329
52. Wang W, Cui Y, Shen J, Jiang J, Chen S, Peng J, et al. Salt-sensitive hypertension and cardiac hypertrophy in transgenic mice expressing a corin variant identified in blacks. Hypertension. (2012) 60(5):1352–8. doi: 10.1161/HYPERTENSIONAHA.112.201244
53. Melo LG, Veress AT, Chong CK, Ackermann U, Sonnenberg H. Salt-sensitive hypertension in ANP knockout mice is prevented by AT1 receptor antagonist losartan. Am J Physiol. (1999) 277(3):R624–30. doi: 10.1152/ajpregu.1999.277.3.R624
54. Feng JA, Perry G, Mori T, Hayashi T, Oparil S, Chen YF. Pressure-independent enhancement of cardiac hypertrophy in atrial natriuretic peptide-deficient mice. Clin Exp Pharmacol Physiol. (2003) 30(5-6):343–9. doi: 10.1046/j.1440-1681.2003.03836.x
55. Li Y, Xia J, Jiang N, Xian Y, Ju H, Wei Y, et al. Corin protects H(2)O(2)-induced apoptosis through PI3K/AKT and NF-κB pathway in cardiomyocytes. Biomed Pharmacother. (2018) 97:594–9. doi: 10.1016/j.biopha.2017.10.090
56. Yin R, Qiu C, Shen Q, Wang Z. Corin is regulated by circ-0012397/miR-200a-3p and inhibits the oxygen-glucose deprivation-induced apoptosis of SHSY5Y neuroblastoma cells. Ann Transl Med. (2022) 10(22):1242. doi: 10.21037/atm-22-4943
57. Rockman HA, Ross RS, Harris AN, Knowlton KU, Steinhelper ME, Field LJ, et al. Segregation of atrial-specific and inducible expression of an atrial natriuretic factor transgene in an in vivo murine model of cardiac hypertrophy. Proc Natl Acad Sci U S A. (1991) 88(18):8277–81. doi: 10.1073/pnas.88.18.8277
58. Deng H, Ma LL, Kong FJ, Qiao Z. Distinct phenotypes induced by different degrees of transverse aortic constriction in C57BL/6N mice. Front Cardiovasc Med. (2021) 8:641272. doi: 10.3389/fcvm.2021.641272
59. Nigrovic PA, Gray DH, Jones T, Hallgren J, Kuo FC, Chaletzky B, et al. Genetic inversion in mast cell-deficient (wsh) mice interrupts corin and manifests as hematopoietic and cardiac aberrancy. Am J Pathol. (2008) 173(6):1693–701. doi: 10.2353/ajpath.2008.080407
60. Buckley CL, Stokes AJ. Corin-deficient W-sh mice poorly tolerate increased cardiac afterload. Regul Pept. (2011) 172(1-3):44–50. doi: 10.1016/j.regpep.2011.08.006
61. Krystel-Whittemore M, Dileepan KN, Wood JG. Mast cell: a multi-functional master cell. Front Immunol. (2015) 6:620. doi: 10.3389/fimmu.2015.00620
62. Wu Q, Kuo HC, Deng GG. Serine proteases and cardiac function. Biochim Biophys Acta. (2005) 1751(1):82–94. doi: 10.1016/j.bbapap.2004.09.005
63. Dries DL, Victor RG, Rame JE, Cooper RS, Wu X, Zhu X, et al. Corin gene minor allele defined by 2 missense mutations is common in blacks and associated with high blood pressure and hypertension. Circulation. (2005) 112(16):2403–10. doi: 10.1161/CIRCULATIONAHA.105.568881
64. Parcha V, Irvin MR, Lange LA, Armstrong ND, Pampana A, Meyer M, et al. Corin missense variants, blood pressure, and hypertension in 11 322 black individuals: insights from REGARDS and the Jackson heart study. J Am Heart Assoc. (2022) 11(12):e025582. doi: 10.1161/JAHA.121.025582
65. Carnethon MR, Pu J, Howard G, Albert MA, Anderson CAM, Bertoni AG, et al. Cardiovascular health in African Americans: a scientific statement from the American heart association. Circulation. (2017) 136(21):e393–423. doi: 10.1161/CIR.0000000000000534
66. Rame JE, Drazner MH, Post W, Peshock R, Lima J, Cooper RS, et al. Corin I555(P568) allele is associated with enhanced cardiac hypertrophic response to increased systemic afterload. Hypertension. (2007) 49(4):857–64. doi: 10.1161/01.HYP.0000258566.95867.9e
67. Burnett JC Jr, Olson TM. Natriuretic peptides and myocardial structure: insights from population genetics. Hypertension. (2007) 49(4):765–6. doi: 10.1161/01.HYP.0000258567.67263.96
68. Wang W, Liao X, Fukuda K, Knappe S, Wu F, Dries DL, et al. Corin variant associated with hypertension and cardiac hypertrophy exhibits impaired zymogen activation and natriuretic peptide processing activity. Circ Res. (2008) 103(5):502–8. doi: 10.1161/CIRCRESAHA.108.177352
69. Kuwahara K. The natriuretic peptide system in heart failure: diagnostic and therapeutic implications. Pharmacol Ther. (2021) 227:107863. doi: 10.1016/j.pharmthera.2021.107863
70. Cannone V, Cabassi A, Volpi R, Burnett JC. Atrial natriuretic peptide: a molecular target of novel therapeutic approaches to cardio-metabolic disease. Int J Mol Sci. (2019) 20(13):3264. doi: 10.3390/ijms20133265
71. Ichiki T, Jinno A, Tsuji Y. Natriuretic peptide-based novel therapeutics: long journeys of drug developments optimized for disease states. Biology. (2022) 11(6):859. doi: 10.3390/biology11060859
72. McMurray JJ, Packer M, Desai AS, Gong J, Lefkowitz MP, Rizkala AR, et al. Angiotensin-neprilysin inhibition versus enalapril in heart failure. N Engl J Med. (2014) 371(11):993–1004. doi: 10.1056/NEJMoa1409077
73. Tripathi R, Sullivan RD, Fan TM, Houng AK, Mehta RM, Reed GL, et al. Cardiac-specific overexpression of catalytically inactive corin reduces edema, contractile dysfunction, and death in mice with dilated cardiomyopathy. Int J Mol Sci. (2019) 21(1):203. doi: 10.3390/ijms21010203
Keywords: cardiac function, cardiac fibrosis, corin, heart failure, mouse models
Citation: Niu Y, Zhou T, Zhang S, Li W, Wang K, Dong N and Wu Q (2023) Corin deficiency impairs cardiac function in mouse models of heart failure. Front. Cardiovasc. Med. 10:1164524. doi: 10.3389/fcvm.2023.1164524
Received: 12 February 2023; Accepted: 1 August 2023;
Published: 11 August 2023.
Edited by:
Junjie Xiao, Shanghai University, ChinaReviewed by:
Johji Kato, University of Miyazaki, Japan© 2023 Niu, Zhou, Zhang, Li, Wang, Dong and Wu. This is an open-access article distributed under the terms of the Creative Commons Attribution License (CC BY). The use, distribution or reproduction in other forums is permitted, provided the original author(s) and the copyright owner(s) are credited and that the original publication in this journal is cited, in accordance with accepted academic practice. No use, distribution or reproduction is permitted which does not comply with these terms.
*Correspondence: Ningzheng Dong bmluZ3poZW5nZG9uZ0BzdWRhLmVkdS5jbg== Qingyu Wu d3VxeUBzdWRhLmVkdS5jbg==
†These authors have contributed equally to this work and share first authorship
Disclaimer: All claims expressed in this article are solely those of the authors and do not necessarily represent those of their affiliated organizations, or those of the publisher, the editors and the reviewers. Any product that may be evaluated in this article or claim that may be made by its manufacturer is not guaranteed or endorsed by the publisher.
Research integrity at Frontiers
Learn more about the work of our research integrity team to safeguard the quality of each article we publish.