- 1Department of Internal Medicine, Universities of Giessen and Marburg Lung Center (UGMLC), Institute for Lung Health (ILH), Cardio-Pulmonary Institute (CPI), Member of the German Center for Lung Research (DZL), Giessen, Germany
- 2Department of Internal Medicine, Justus-Liebig-University Giessen, Giessen, Germany
Background: Volume overload is often associated with clinical deterioration in precapillary pulmonary hypertension (PH). However, thorough assessment of volume overload is complex and therefore not routinely performed. We examined whether estimated plasma volume status (ePVS) is associated with central venous congestion and prognosis in patients with idiopathic pulmonary arterial hypertension (IPAH) or chronic thromboembolic PH (CTEPH).
Methods: We included all patients with incident IPAH or CTEPH enrolled in the Giessen PH Registry between January 2010 and January 2021. Plasma volume status was estimated using the Strauss formula.
Results: In total, 381 patients were analyzed. Patients with high ePVS (≥4.7 vs. <4.7 ml/g) at baseline showed significantly increased central venous pressure (CVP; median [Q1, Q3]: 8 [5, 11] mmHg vs. 6 [3, 10] mmHg) and pulmonary arterial wedge pressure (10 [8, 15] mmHg vs. 8 [6, 12] mmHg), while right ventricular function was not altered. In multivariate stepwise backward Cox regression, ePVS was independently associated with transplant-free survival at baseline and during follow-up (hazard ratio [95% confidence interval]: 1.24 [0.96, 1.60] and 2.33 [1.49, 3.63], respectively). An intra-individual decrease in ePVS was associated with a decrease in CVP and predicted prognosis in univariate Cox regression. Patients with high ePVS without edema had lower transplant-free survival than those with normal ePVS without edema. In addition, high ePVS was associated with cardiorenal syndrome.
Conclusions: In precapillary PH, ePVS is associated with congestion and prognosis. High ePVS without edema may represent an under-recognized subgroup with poor prognosis.
1. Introduction
Pulmonary hypertension (PH) is a devastating vascular disease characterized by increased pulmonary arterial pressure and resistance (1). The right ventricle adapts to the increased right ventricular afterload through various mechanisms such as hypertrophy and increased contractility (2). However, even slight changes in pulmonary hemodynamics can unbalance the fragile interaction between the right ventricle and the pulmonary artery, resulting in so-called uncoupling.
One of the most common causes of the previously described congestion and, therefore, exacerbations of PH is plasma volume overload (3). However, optimal adjustment of the patient's fluid status is time-consuming and complex, since it currently depends on parameters such as the diameter of the inferior vena cava or even the invasive measurement of central venous pressure (CVP) by right heart catheterization (4). Clinical signs such as edema or body weight gain are considered uncertain signs of volume overload (5, 6). A volume overload assessment is therefore not always performed, especially in an ambulatory setting where clinicians sometimes rely only on the brain natriuretic peptide (BNP) concentration to indicate right ventricular stress.
Recent data suggest that the plasma volume status can be estimated using different formulas (7, 8). In patients with left heart failure, the estimated plasma volume status (ePVS) is associated with prognosis, and the intra-individual change in estimated plasma volume can be used to monitor congestion (8). However, the utility and prognostic relevance of ePVS in patients with precapillary PH are not yet fully understood. Thus, we aimed to assess the clinical relevance of (i) edema and (ii) ePVS in patients with idiopathic pulmonary arterial hypertension (IPAH) or chronic thromboembolic PH (CTEPH).
2. Methods
2.1. Study population
All consecutive patients with newly diagnosed IPAH (PH classification group 1) or CTEPH (PH classification group 4) who were enrolled in the prospectively recruiting Giessen PH registry (9) between January 2010 and January 2021 were included in this study. Patients without right heart catheterization or hemoglobin/hematocrit values at the time of diagnosis were excluded from further analysis. The diagnosis was made by a multidisciplinary panel of physicians, surgeons, and radiologists. All patients gave their written informed consent to be enrolled in the Giessen PH registry (and subsequent analyses of registry data). The study was approved by the local ethics committee of the University of Giessen (#266/11).
2.2. Right heart catheterization
Right heart catheterization was performed as previously described (10). Briefly, a sheath was placed in the right internal jugular vein under local anesthesia. In rare cases, the left internal jugular vein or femoral vein was used. CVP, pulmonary arterial pressure, and pulmonary arterial wedge pressure (PAWP) were measured during stopped expiration using a Swan-Ganz catheter (11). Cardiac output was determined with the direct Fick method and thermodilution, and was used to calculate pulmonary vascular resistance {[mean pulmonary arterial pressure (mPAP)—PAWP]/cardiac output}. If oxygen consumption was not measurable, resting oxygen uptake was estimated using the LaFarge and Bergstra formula (12).
2.3. Echocardiography, laboratory measurements, and estimation of plasma volume status
Echocardiography was performed by experts according to the guidelines that were valid at the time of the measurement (1, 13, 14). The echocardiographic assessment included, among others, the systolic excursion of the tricuspid annular plane (TAPSE) and the right atrial area. The laboratory measurements included, among others, BNP and creatinine levels and were performed in the central laboratory of the University Hospital Giessen. Glomerular filtration rate was estimated (eGFR) using the Chronic Kidney Disease Epidemiology Collaboration formula (142 * min[serum creatinine/kappa, 1]alpha * max[serum creatinine/kappa, 1]−1.2 * 0.9938Age * Sex Factor, where alpha = −0.241 [female] or −0.302 [male], kappa = 0.7 [female] or 0.9 [male], and Sex Factor = 1.012 [female] or 1 [male]) (15, 16).
Plasma volume status was estimated at baseline and during follow-up using the Strauss formula: ePVS = (1-hematocrit)/hemoglobin (g/dl) * 100 (8). This formula considers hematocrit and hemoglobin concentration as estimators for plasma volume status. Both hematocrit and hemoglobin concentration are known predictors of cardiovascular events (17, 18). In addition, the relative change of ePVS can be determined by the following formula: ePVSfollow−up–ePVSbaseline.
2.4. Statistical analyses
The Shapiro-Wilk test was used to assess whether the included variables were normally distributed. Normally distributed continuous data are presented as mean ± standard deviation and t-tests were used to compare means between groups. Non-normal continuous data are shown as median [first quartile, third quartile] and Wilcoxon rank tests were used to compare medians between groups. Chi-square tests were used to compare categorical data between groups. We considered p < 0.05 to indicate statistical significance. Survival time and transplant status were censored at either 12 months (short-term transplant-free survival) or 60 months (long-term transplant-free survival). Kaplan-Meier estimators with log-rank tests and univariate and multivariate (stepwise backward) Cox regression analyses were used to examine the prognostic relevance of parameters. In the multivariate analyses, the stepwise backward model was chosen based on Akaike information criteria using the R stats package. Cox regression coefficients were used to develop new prognostic models based on independent predictors of mortality/transplant, as described previously (19). Multivariate, backward binary logistic regression was performed using a p value threshold of 0.10. Missing data were not imputed. Statistical analyses were performed with R version 4.0.4 (The R Foundation, Vienna) and SPSS 29.0 (IBM, Armonk, USA).
3. Results
3.1. Baseline characteristics
A total of 381 patients were enrolled in the study, of whom 145 (38%) were diagnosed with IPAH and 236 (62%) with CTEPH. The median age of the study population was 69 [55, 76] years and 217 (57%) were female. The included patients showed severe impairment of pulmonary hemodynamics (Table 1). The median CVP was 7 [4, 10] mmHg and the median ePVS was 4.14 [3.53, 4.92] ml/g.
3.2. Prognostic relevance of edema in incident and prevalent patients with PH
First, we evaluated whether the clinical assessment of edema in precapillary PH is prognostically relevant. Data on edema status were available for 317 patients at baseline (incident patients) and 154 patients at follow-up (median 8 [6, 9] months after baseline; prevalent patients). As shown in the Kaplan-Meier curves in Figure 1, patients with edema had comparable transplant-free survival rates to those without edema, both at baseline and at follow-up. In addition, there was no difference in the transplant-free survival distribution between patients whose edema resolved and those who developed edema during follow-up (Supplementary Figure S1A). In patients with edema at baseline, resolution of the edema was also not associated with increased transplant-free survival (Supplementary Figure S1B).
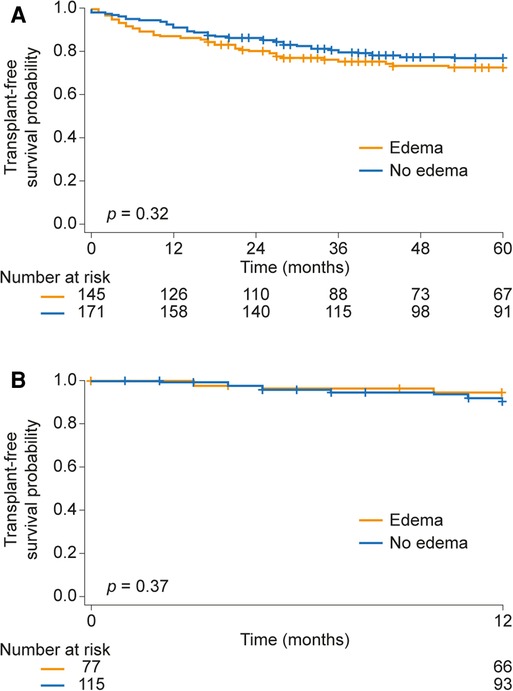
Figure 1. Prognostic relevance of edema (A) at baseline and (B) during follow-up in patients with precapillary pulmonary hypertension.
3.3. Association of high ePVS with congestion and chronic cardiorenal syndrome in incident patients with PH
Second, we investigated whether elevated ePVS is associated with congestion in patients with precapillary PH. We classified ePVS in the lowest and intermediate thirds (below 4.7 ml/g) as normal, and ePVS ≥4.7 ml/g as high.
Baseline characteristics of patients with normal and high ePVS are compared in Table 1. Interestingly, patients with high ePVS showed significantly increased PAWP and CVP, while mPAP and (concordantly) pulmonary vascular resistance were lower than in patients with normal ePVS. In turn, patients with high ePVS had edema significantly more often than those with normal ePVS. In addition, BNP levels tended to be higher and eGFR was significantly lower in patients with high ePVS compared with patients with normal ePVS. However, TAPSE, the diameter of the inferior vena cava, and right atrial area were not affected. Interestingly, patients with high ePVS had chronic cardiorenal syndrome, defined by an eGFR < 60ml/min/1.73 m2, significantly more often than patients with normal ePVS (44% vs. 28%, X2 p = 0.003).
3.4. Prognostic relevance of ePVS in incident patients with PH
Next, Kaplan-Meier analysis was performed to assess the prognostic relevance of ePVS at baseline (Figure 2A). The 1-, 3-, and 5-year transplant-free survival rates of patients with normal ePVS at baseline were 90%, 82%, and 79%, respectively, while patients with high ePVS at baseline showed significantly lower transplant-free survival rates (84%, 70%, and 69%, respectively; log-rank p = 0.023). Consistent with this, univariate Cox regression analysis showed a significantly increased hazard ratio (HR) for mortality/transplant (HR per unit increase of baseline ePVS: 1.26 [95% confidence interval (CI): 1.08, 1.47], p = 0.004). Regarding non-invasive parameters, BNP concentration, eGFR, TAPSE, inferior vena cava diameter, hematocrit, hemoglobin concentration, and right atrial area were also associated with prognosis in univariate Cox regression as shown in Table 2. Multivariate (stepwise backward) Cox regression showed that ePVS, eGFR, inferior vena cava diameter, and BNP concentration were independently associated with transplant-free survival in patients with precapillary PH (Table 2). Next, we performed a Kaplan-Meier analysis based on thirds of a Cox regression score (regression score , for n independent predictors of mortality/transplant in backward regression). The 1-, 3-, and 5-year transplant-free survival rates were 98%, 88%, and 88%, respectively, in the low-score group and 91%, 82%, and 78%, respectively, in the intermediate-score group, while the patients in the high-score group had significantly reduced transplant-free survival rates (71%, 52%, and 46% at 1, 3, and 5 years, respectively), as shown in Supplementary Figure S2A.
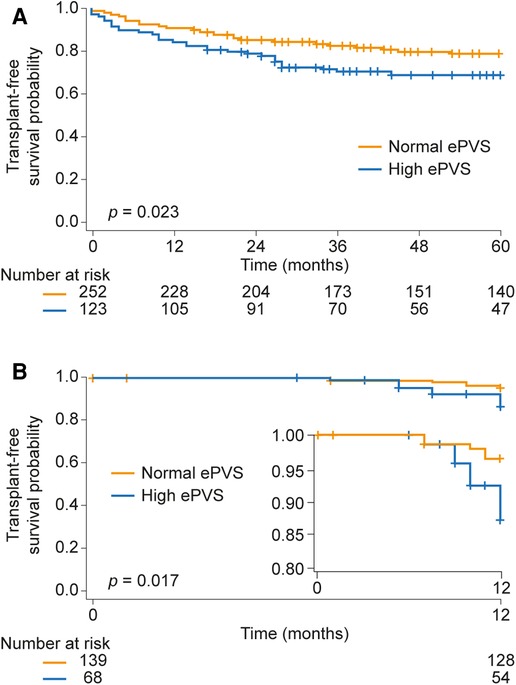
Figure 2. Prognostic relevance of ePVS (A) at baseline and (B) during follow-up in patients with precapillary pulmonary hypertension. In panel (B), the inset shows the same data on an enlarged y axis. ePVS, estimated plasma volume status.
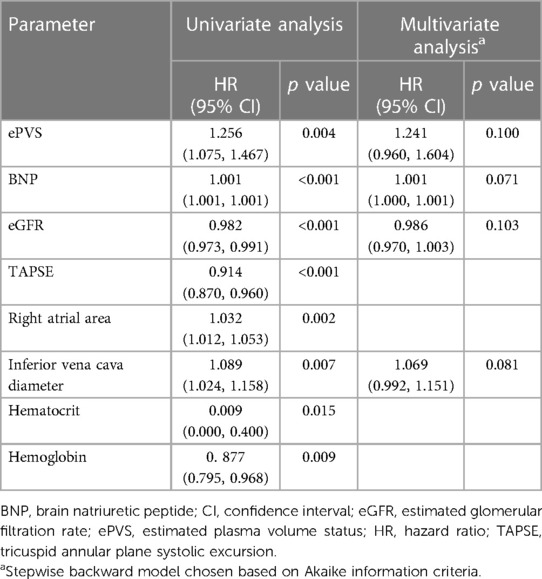
Table 2. Cox regression analysis of the association of baseline parameters with mortality/transplant.
3.5. Prognostic relevance of ePVS in prevalent patients with PH
Follow-up data were available for 221 patients. The median follow-up time was 8 [6, 9] months. Patient characteristics at follow-up are presented in Table 3. At follow-up, PAWP, CVP, and BNP concentration were significantly elevated and eGFR was significantly decreased in patients with high ePVS compared with patients with normal ePVS, although the prevalence of edema did not differ significantly between the two groups. TAPSE, inferior vena cava diameter, and right atrial area at follow-up also showed no difference between the two groups. In addition, ePVS showed moderate correlation with CVP (spearman rho = 0.32, p = 0.005).
High ePVS during follow-up (defined as >4.8 ml/g) was associated with significantly reduced transplant-free survival in a Kaplan-Meier analysis truncated at 12 months (Figure 2B; log-rank p = 0.017), and ePVS predicted short-term (1-year) mortality/transplant in univariate Cox regression analysis (HR per unit increase of ePVS during follow-up: 2.12 [95% CI: 1.54, 2.93], p < 0.001). BNP concentration, eGFR, TAPSE, hematocrit, and hemoglobin concentration were also associated with transplant-free survival in prevalent patients with PH in univariate Cox regression analysis (Table 4). However, only ePVS, TAPSE, BNP concentration, and eGFR were independently associated with prognosis in multivariate (stepwise backward) Cox regression (Table 4). The resulting Cox regression score showed high concordance (0.912 ± 0.036). The short-term Kaplan-Meier analysis based on thirds of the Cox regression score is shown in Supplementary Figure S2B. The 1-year transplant-free survival rate was 100% and 98% in the low- and intermediate-score groups, respectively, and was significantly compromised (77%) in the high-score group.
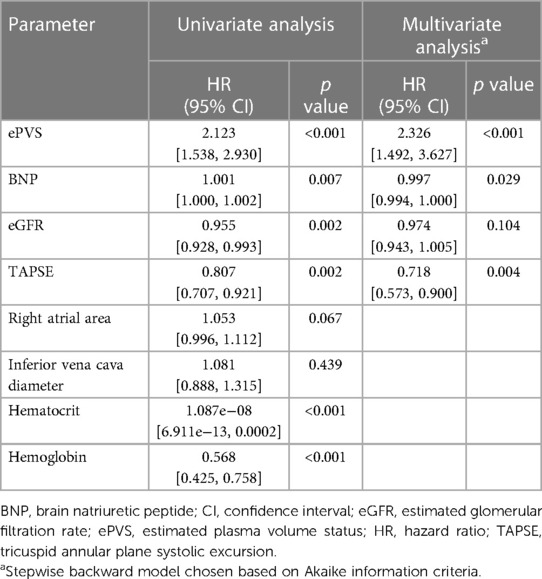
Table 4. Cox regression analysis of the association of follow-up parameters with mortality/transplant.
Next, we assessed the prognostic relevance of the change in ePVS from baseline during follow-up in PH. The median change in ePVS was 0.31 [−0.16, 0.87] ml/g. Patients with large decreases in ePVS (below −0.16 ml/g) showed significantly greater decreases in CVP and PAWP than patients with lower decreases in ePVS during follow-up (CVP: −4 ± 5 vs. 0 ± 5 mmHg, p = 0.019; PAWP: −2 [−3, −1] mmHg vs. 1 [−1, 4] mmHg, p = 0.008). Consistent with these findings, the change in ePVS was associated with prognosis in univariate Cox regression analysis (HR: 1.55 [95% CI: 1.00, 2.38], p = 0.048).
3.6. Newly described phenotype of high ePVS without edema in patients with incident PH
Of the 317 patients with information available on the presence/absence of edema at baseline, 131 patients (41%) had neither edema nor high ePVS, while 63 patients (20%) had both edema and high ePVS. Interestingly, 39 patients (12%) had high ePVS but no edema. We further characterized this subset of patients.
Patients with high ePVS but no edema had significantly lower mPAP and pulmonary vascular resistance and elevated PAWP, cardiac index, and TAPSE/pulmonary arterial systolic pressure (PASP) ratio compared with patients with normal ePVS and no edema (Table 5). Other pulmonary hemodynamic and echocardiographic parameters were comparable in both groups (Table 5). BNP concentration was not significantly increased. Notably, patients with high ePVS without edema had significantly impaired transplant-free survival, as shown in the Central Illustration. The univariate HR for mortality/transplant in this group compared with patients with normal ePVS without edema was 2.28 (95% CI: 1.18, 4.42). The HR remained significantly increased even after adjustment for age, tricuspid regurgitation, pulmonary vascular resistance, mixed venous oxygen saturation, BNP, and TAPSE/PASP (HR: 2.58 [95% CI: 1.08, 6.17]).
3.7. Reason for development of edema in patients with high ePVS and chronic cardiorenal syndrome
Of 54 patients with high ePVS and chronic cardiorenal syndrome, 45 patients had edema (83%). To investigate the underlying mechanism leading to edema in this subgroup, we performed backward binary logistic regression. Interestingly, only TAPSE/PASP (B = −6.34, p = 0.066) and sex (B = −1.51, p = 0.086) were independently associated with edema while age, eGFR, inferior vena cava diameter, right atrial area, pulmonary vascular resistance, cardiac index, mixed venous oxygen saturation, and BNP were not.
4. Discussion
Our study assesses the relevance of ePVS in a homogeneous study population of patients with precapillary PH (IPAH and CTEPH). High ePVS was associated with congestion and cardiorenal syndrome and predicted mortality/transplant both at baseline and during follow-up. Furthermore, a decrease in ePVS was associated with a decrease in volume overload (i.e., decongestion) and with improved prognosis. Notably, the prognostic utility of ePVS was independent of other non-invasive biomarkers such as eGFR, BNP concentration, or echocardiographic parameters such as TAPSE and right atrial area. A newly defined phenotype of high ePVS without edema was characterized and its prognostic relevance was demonstrated. Thus, in addition to the approaches currently used, ePVS enables physicians to assess the volume (over)load and prognosis of patients with precapillary PH.
Although the reserve of right ventricular-pulmonary arterial coupling is high in patients with PH, both chronic and acute volume overload result in clinical deterioration accompanied by a worsened prognosis (19, 20). Non-invasive assessment of volume overload in patients with heart failure is therefore crucial, but is complicated by the imprecision of clinical signs such as edema and the lack of accurate biomarkers. Our study shows that ePVS is a feasible tool for estimating central venous congestion in PH, as high ePVS was associated with increased CVP. Notably, right ventricular systolic function (reflected by TAPSE) was not consistently impaired in patients with PH and plasma volume overload. In addition, there was no right atrial dilatation. Therefore, one can speculate that ePVS is an early biomarker of volume overload, before contractility worsens and remodeling occurs in the right side of the heart. In contrast to ePVS, BNP concentrations (currently widely used to assess congestion in patients with PH) are strongly influenced by right ventricular function (21). Furthermore, multivariate (stepwise backward) Cox regression showed that ePVS is an independent predictor of mortality/transplant in incident patients with PH and can therefore be used in addition to the existing (non-invasive) methods of risk assessment in PH (1, 22).
At follow-up examinations, high ePVS was associated with increased central venous congestion (reflected by invasively measured CVP). Similarly, ePVS predicted mortality/transplant in patients with precapillary PH. Once again, ePVS was not meaningfully dependent on right ventricular systolic function and right atrial enlargement. In addition, ePVS remained as an independent predictor of mortality/transplant in multivariate (stepwise backward) Cox regression and can therefore be used as an additional biomarker to assess congestion during follow-up. Notably, concordance of a new risk score including ePVS was high, exceeding 0.900.
Interestingly, decongestion (reflected by an intra-patient decrease in ePVS) was associated with decreases in CVP and PAWP. In addition, the intra-patient decrease in ePVS was a predictor of transplant-free survival. From this, it can be concluded that the intra-individual course of ePVS can be used to monitor the effect and sufficiency of treatment in patients with IPAH or CTEPH.
We have for the first time described a phenotype of high ePVS without edema, which had a high prevalence in our study cohort. Patients with this phenotype showed less severe elevation of mPAP and pulmonary vascular resistance and no significant difference in right ventricular function and BNP concentration compared with patients with normal ePVS without edema. Nevertheless, transplant-free survival was poor and comparable to that in patients with edema and high ePVS, underscoring the clinical relevance of a thorough assessment of plasma volume in patients with precapillary PH. Special attention needs to be paid to this newly described phenotype because it is easily underdiagnosed due to normal echocardiographic right heart morphology and function. The associated risk of mortality is overlooked in risk assessments based on the usual clinical parameters. We thus expect clinical (risk) assessment of patients with precapillary PH to be improved by inclusion of ePVS. However, due to the lack of prospective studies, it remains unclear how patients with this phenotype should be managed and whether escalation of diuretic therapy can increase the probability of transplant-free survival. In patients with high plasma volume and impaired renal function in our study cohort, impaired RV-PA coupling (besides sex) was the main reason for the development of edema. Other parameters, including eGFR, inferior vena cava diameter, right atrial area, right ventricular function, and pulmonary hemodynamics were not associated with edema.
From a pathophysiological point of view, it is known that an increased right ventricular afterload leads to both venous congestion and reduced forward stroke volume (4, 19, 23). Both lead to impaired renal function and thus to fluid retention (Figure 3) (23). Patients with impaired right ventricular function show edema due to high plasma volume. These patients can be identified through clinical examination and treated appropriately. Conversely, patients with preserved right ventricular function do not show edema but still have an increased plasma volume. These patients cannot be identified through routine clinical examination because their clinical and echocardiographic findings appear normal, although they have a significantly reduced survival time.
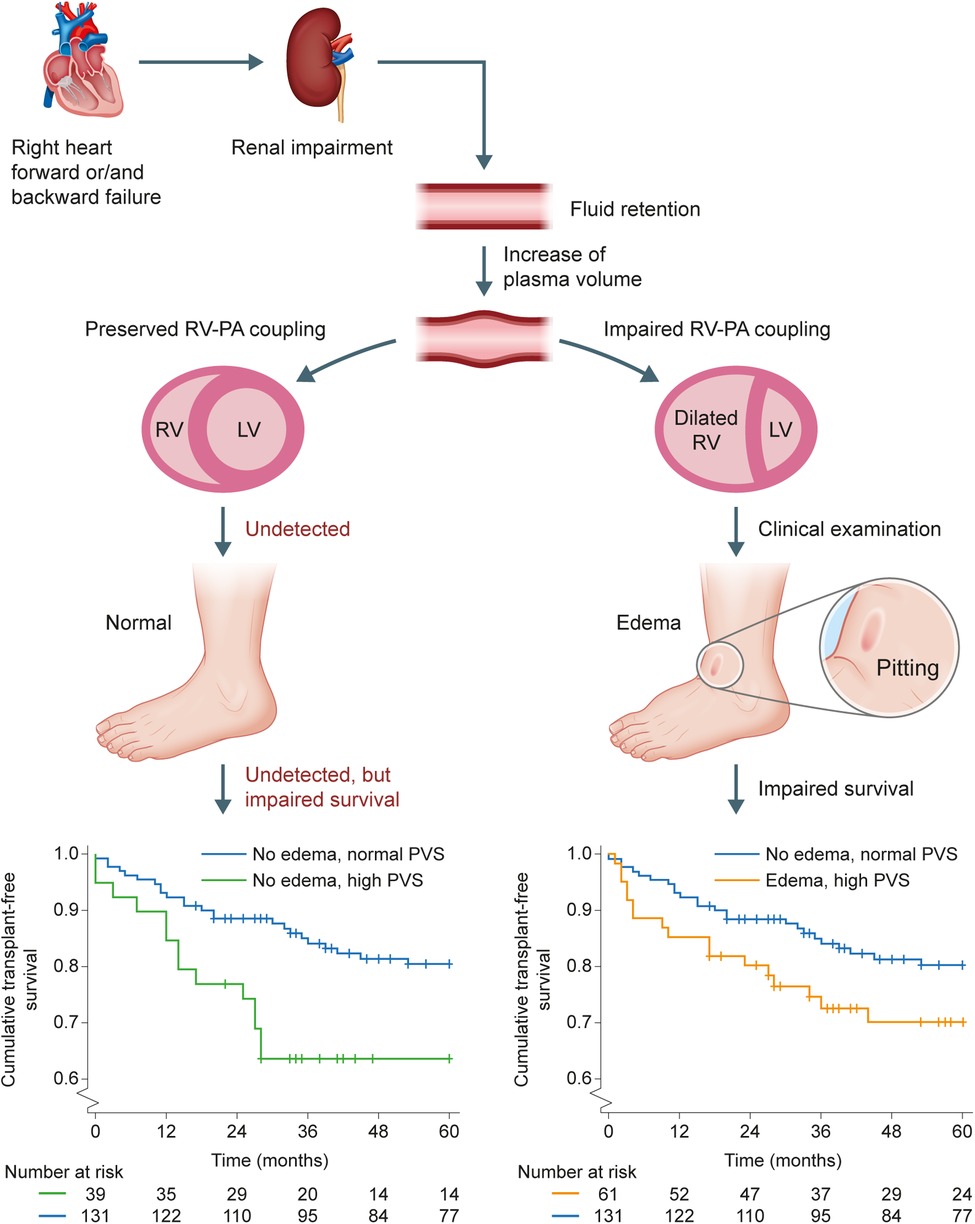
Figure 3. Prognostic relevance of high ePVS in the absence of edema. Forward and backward failure in the right side of the heart both lead to impaired renal function and thus to fluid retention. Patients with impaired right ventricular function show edema due to high plasma volume and have impaired transplant-free survival (lower Kaplan-Meier plot). Patients with preserved right ventricular function do not show edema but still have a high plasma volume (undetected in routine clinical examination) and significantly impaired transplant-free survival (upper Kaplan-Meier plot; p = 0.012). In both Kaplan-Meier plots, the comparator is the group of patients with normal ePVS without edema. ePVS, estimated plasma volume status; LV, left ventricle; PA, pulmonary artery; RV, right ventricle.
It should be noted that hemoglobin concentration and hematocrit—which are included in the calculation of ePVS (8, 24)—depend not only on plasma volume, but also on constellations driving erythropoiesis such as chronic hypoxemia or PH-targeted therapy during follow-up (e.g., endothelin receptor antagonists causing anemia), in particular in patients with PH and (right-sided) heart failure (25). Under these circumstances, ePVS decreases since the hemoglobin concentration is in the denominator of the formula for estimating plasma volume (8). By including hematocrit in the multivariate regression analysis, our study gives evidence for the superiority of ePVS. In this context, it is also important to consider that the ePVS formula has shown a good correlation with the measured plasma volume in patients with heart failure and preserved ejection fraction (7). Nevertheless, ePVS must always be evaluated in the overall clinical context and should not be considered as a stand-alone parameter.
Our study is limited due to its retrospective study design. Missing data and subsequent exclusion of patients may have resulted in significant bias. Furthermore, the usefulness of ePVS for guiding treatment strategy/diuretic escalation remains unclear. Chronic cardiorenal syndrome was solely defined by eGFR without exclusion of other reasons which may have led to increased creatinine concentrations, owing to the retrospective study design. The definitive mechanism leading to the association of ePVS with congestion and cardiorenal syndrome cannot be directly assessed due to the retrospective nature of the study. Therefore, a prospective assessment is warranted.
In conclusion, we believe that the estimation of plasma volume status may be a feasible additional method to assess and monitor volume overload and congestion in patients with precapillary PH. It is a novel finding that elevated ePVS values are linked with poor survival, even in the absence of right heart decompensation and overt edema formation. Owing to its added prognostic value in combination with existing non-invasive biomarkers, ePVS is a promising clinical assessment tool.
Data availability statement
The raw data supporting the conclusions of this article will be made available by the authors, without undue reservation.
Ethics statement
The studies involving human participants were reviewed and approved by local ethics committee of the University of Giessen (#266/11). The patients/participants provided their written informed consent to participate in this study.
Author contributions
Study conceptualization: AY, HG, MJR; Study design and data collection: All authors; Data analysis: AY and HG; Drafting of the manuscript: AY, HG, MJR; Critical revision of the manuscript: All authors; Approval of manuscript for submission: All authors; Agreement to be accountable for all aspects of the work in ensuring that questions related to the accuracy or integrity of any part of the work are appropriately investigated and resolved: All authors. All authors contributed to the article and approved the submitted version.
Funding
This work was supported by the Excellence Cluster Cardio-Pulmonary System (ECCPS) and the Collaborative Research Center (SFB) 1213 - Pulmonary Hypertension and Cor Pulmonale, grant number SFB1213/1, project B08 (German Research Foundation, Bonn, Germany).
Acknowledgments
Editorial assistance was provided by Claire Mulligan (Beacon Medical Communications Ltd, Brighton, UK), funded by the University of Giessen.
Conflict of interest
AY reports personal fees from MSD outside the submitted work. KT reports personal fees from Actelion and Bayer outside the submitted work. NS reports personal fees from Actelion outside the submitted work. WS reports personal fees from Pfizer and Bayer Pharma AG outside the submitted work. HAG reports personal fees from Bayer, Actelion, Pfizer, Merck, GSK, and Takeda, grants and personal fees from Novartis, Bayer HealthCare, and Encysive/Pfizer, and grants from Aires outside the submitted work. HG reports personal fees from Actelion, AstraZeneca, Bayer, BMS, GSK, Janssen-Cilag, Lilly, MSD, Novartis, OMT, Pfizer, and United Therapeutics outside the submitted work. MR reports grants from United Therapeutics, grants and personal fees from Bayer, and personal fees from Actelion, Mundipharma, Roche, and OMT outside the submitted work.
The remaining authors declare that the research was conducted in the absence of any commercial or financial relationships that could be construed as a potential conflict of interest.
Publisher's note
All claims expressed in this article are solely those of the authors and do not necessarily represent those of their affiliated organizations, or those of the publisher, the editors and the reviewers. Any product that may be evaluated in this article, or claim that may be made by its manufacturer, is not guaranteed or endorsed by the publisher.
Supplementary material
The Supplementary Material for this article can be found online at: https://www.frontiersin.org/articles/10.3389/fcvm.2023.1161041/full#supplementary-material.
Abbreviations
BNP, brain natriuretic peptide; CI, confidence interval; CTEPH, chronic thromboembolic pulmonary hypertension; CVP, central venous pressure; eGFR, estimated glomerular filtration rate; ePVS, estimated plasma volume status; HR, hazard ratio; IPAH, idiopathic pulmonary arterial hypertension; mPAP, mean pulmonary arterial pressure; PASP, pulmonary arterial systolic pressure; PAWP, pulmonary arterial wedge pressure; PH, pulmonary hypertension; TAPSE, tricuspid annular plane systolic excursion.
References
1. Galie N, Humbert M, Vachiery JL, Gibbs S, Lang I, Torbicki A, et al. 2015 ESC/ERS guidelines for the diagnosis and treatment of pulmonary hypertension: the joint task force for the diagnosis and treatment of pulmonary hypertension of the European society of cardiology (ESC) and the European respiratory society (ERS): endorsed by: association for European paediatric and congenital cardiology (AEPC), international society for heart and lung transplantation (ISHLT). Eur Heart J. (2016) 37:67–119. doi: 10.1093/eurheartj/ehv317
2. Ryan JJ, Archer SL. The right ventricle in pulmonary arterial hypertension: disorders of metabolism, angiogenesis and adrenergic signaling in right ventricular failure. Circ Res. (2014) 115:176–88. doi: 10.1161/CIRCRESAHA.113.301129
3. Hansen L, Burks M, Kingman M, Stewart T. Volume management in pulmonary arterial hypertension patients: an expert pulmonary hypertension clinician perspective. Pulm Ther. (2018) 4:13–27. doi: 10.1007/s41030-018-0052-z
4. Humbert M, Kovacs G, Hoeper MM, Badagliacca R, Berger RMF, Brida M, et al. 2022 ESC/ERS guidelines for the diagnosis and treatment of pulmonary hypertension. Eur Heart J. (2022) 43:3618–731. doi: 10.1093/eurheartj/ehac237
5. Miller WL. Fluid volume overload and congestion in heart failure: time to reconsider pathophysiology and how volume is assessed. Circ Heart Fail. (2016) 9:e002922. doi: 10.1161/CIRCHEARTFAILURE.115.002922
6. McDonald K. Monitoring fluid status at the outpatient level: the need for more precision. Congest Heart Fail. (2010) 16(Suppl 1):S52–5. doi: 10.1111/j.1751-7133.2010.00171.x
7. Fudim M, Miller WL. Calculated estimates of plasma volume in patients with chronic heart failure-comparison with measured volumes. J Card Fail. (2018) 24:553–60. doi: 10.1016/j.cardfail.2018.07.462
8. Duarte K, Monnez JM, Albuisson E, Pitt B, Zannad F, Rossignol P. Prognostic value of estimated plasma volume in heart failure. JACC Heart Fail. (2015) 3:886–93. doi: 10.1016/j.jchf.2015.06.014
9. Gall H, Felix JF, Schneck FK, Milger K, Sommer N, Voswinckel R, et al. The Giessen pulmonary hypertension registry: survival in pulmonary hypertension subgroups. J Heart Lung Transplant. (2017) 36:957–67. doi: 10.1016/j.healun.2017.02.016
10. Yogeswaran A, Richter MJ, Sommer N, Ghofrani HA, Seeger W, Gall H, et al. Evaluation of pulmonary hypertension by right heart catheterisation: does timing matter? Eur Respir J. (2020) 56:1901892. doi: 10.1183/13993003.01892-2019
11. Gassanov N, Er F. Right heart catheter examination: step by step. Dtsch Med Wochenschr. (2021) 146:1064–9. doi: 10.1055/a-1199-7446
12. Narang N, Thibodeau JT, Levine BD, Gore MO, Ayers CR, Lange RA, et al. Inaccuracy of estimated resting oxygen uptake in the clinical setting. Circulation. (2014) 129:203–10. doi: 10.1161/CIRCULATIONAHA.113.003334
13. Kaul S, Tei C, Hopkins JM, Shah PM. Assessment of right ventricular function using two-dimensional echocardiography. Am Heart J. (1984) 107:526–31. doi: 10.1016/0002-8703(84)90095-4
14. Lang RM, Badano LP, Mor-Avi V, Afilalo J, Armstrong A, Ernande L, et al. Recommendations for cardiac chamber quantification by echocardiography in adults: an update from the American society of echocardiography and the European association of cardiovascular imaging. Eur Heart J Cardiovasc Imaging. (2015) 16:233–70. doi: 10.1093/ehjci/jev014
15. Inker LA, Eneanya ND, Coresh J, Tighiouart H, Wang D, Sang Y, et al. New creatinine- and cystatin c-based equations to estimate GFR without race. N Engl J Med. (2021) 385:1737–49. doi: 10.1056/NEJMoa2102953
16. Levey AS, Stevens LA, Schmid CH, Zhang YL, Castro AF 3rd, Feldman HI, et al. A new equation to estimate glomerular filtration rate. Ann Intern Med. (2009) 150:604–12. doi: 10.7326/0003-4819-150-9-200905050-00006
17. Angraal S, Mortazavi BJ, Gupta A, Khera R, Ahmad T, Desai NR, et al. Machine learning prediction of mortality and hospitalization in heart failure with preserved ejection fraction. JACC Heart Fail. (2020) 8:12–21. doi: 10.1016/j.jchf.2019.06.013
18. Erikssen G, Thaulow E, Sandvik L, Stormorken H, Erikssen J. Haematocrit: a predictor of cardiovascular mortality? J Intern Med. (1993) 234:493–9. doi: 10.1111/j.1365-2796.1993.tb00783.x
19. Yogeswaran A, Tello K, Lund J, Klose H, Harbaum L, Sommer N, et al. Risk assessment in pulmonary hypertension based on routinely measured laboratory parameters. J Heart Lung Transplant. (2022) 41:400–10. doi: 10.1016/j.healun.2021.10.018
20. Tello K, Dalmer A, Axmann J, Vanderpool R, Ghofrani HA, Naeije R, et al. Reserve of right ventricular-arterial coupling in the setting of chronic overload. Circ Heart Fail. (2019) 12:e005512. doi: 10.1161/CIRCHEARTFAILURE.118.005512
21. Legris V, Thibault B, Dupuis J, White M, Asgar AW, Fortier A, et al. Right ventricular function and its coupling to pulmonary circulation predicts exercise tolerance in systolic heart failure. ESC Heart Fail. (2022) 9:450–64. doi: 10.1002/ehf2.13726
22. Forfia PR, Fisher MR, Mathai SC, Housten-Harris T, Hemnes AR, Borlaug BA, et al. Tricuspid annular displacement predicts survival in pulmonary hypertension. Am J Respir Crit Care Med. (2006) 174:1034–41. doi: 10.1164/rccm.200604-547OC
23. Husain-Syed F, Birk HW, Ronco C, Schormann T, Tello K, Richter MJ, et al. Doppler-derived renal venous stasis index in the prognosis of right heart failure. J Am Heart Assoc. (2019) 8:e013584. doi: 10.1161/JAHA.119.013584
24. Strauss MB, Davis RK, Rosenbaum JD, Rossmeisl EC. Water diuresis produced during recumbency by the intravenous infusion of isotonic saline solution. J Clin Invest. (1951) 30:862–8. doi: 10.1172/JCI102501
Keywords: pulmonary arterial hypertension, chronic thromboembolic pulmonary hypertension, glomerular filtration rate, fluid balance, survival
Citation: Yogeswaran A, Richter MJ, Husain-Syed F, Rako Z, Sommer N, Grimminger F, Seeger W, Ghofrani HA, Gall H and Tello K (2023) Estimated plasma volume status: association with congestion, cardiorenal syndrome and prognosis in precapillary pulmonary hypertension. Front. Cardiovasc. Med. 10:1161041. doi: 10.3389/fcvm.2023.1161041
Received: 9 February 2023; Accepted: 26 April 2023;
Published: 10 May 2023.
Edited by:
Francesco Gentile, Sant’Anna School of Advanced Studies, Italy© 2023 Yogeswaran, Richter, Husain-Syed, Rako, Sommer, Grimminger, Seeger, Ghofrani, Gall and Tello. This is an open-access article distributed under the terms of the Creative Commons Attribution License (CC BY). The use, distribution or reproduction in other forums is permitted, provided the original author(s) and the copyright owner(s) are credited and that the original publication in this journal is cited, in accordance with accepted academic practice. No use, distribution or reproduction is permitted which does not comply with these terms.
*Correspondence: Athiththan Yogeswaran YXRoaXRodGhhbi55b2dlc3dhcmFuQGlubmVyZS5tZWQudW5pLWdpZXNzZW4uZGU=
†These authors have contributed equally to this work and share senior authorship