- Division of Cardiology, National Defense Medical College, Tokorozawa, Saitama, Japan
Coronary microcirculation has multiple layers of autoregulatory function to maintain resting flow and augment hyperemic flow in response to myocardial demands. Functional or structural alterations in the coronary microvascular function are frequently observed in patients with heart failure with preserved or reduced ejection fraction, which may lead to myocardial ischemic injury and resultant worsening of clinical outcomes. In this review, we describe our current understanding of coronary microvascular dysfunction in the pathogenesis of heart failure with preserved and reduced ejection fraction.
Introduction
Percutaneous revascularization for epicardial coronary artery disease (CAD) as an initial treatment strategy did not improve survival even in chronic coronary syndrome patients with evidence of moderate-severe ischemia (ISCHEMIA trial) (1). Revascularizing epicardial CAD with percutaneous coronary intervention (PCI) also failed to show additional benefit in reducing all-cause death or heart failure hospitalization beyond optimal medical therapy in patients with left ventricular systolic dysfunction (REVIVED-BCIS2) (2). Given the fact that the myocardial oxygen extraction from the coronary circulation is approximately 70%–80% at rest and that increased myocardial oxygen demands need to be met by increasing coronary flow (3, 4), the above mentioned negative results of epicardial revascularization may potentially underline the importance of the coronary microvasculature to augment coronary flow to meet the myocardial demands. Recently, coronary microvascular dysfunction has been rigorously studied and appears to be involved not only in the development of ischemic symptoms but also in various pathological processes and conditions, including heart failure (5–8). In this short review, we will discuss the relationship between coronary microvascular dysfunction and heart failure with preserved or reduced ejection fraction (Figure 1).
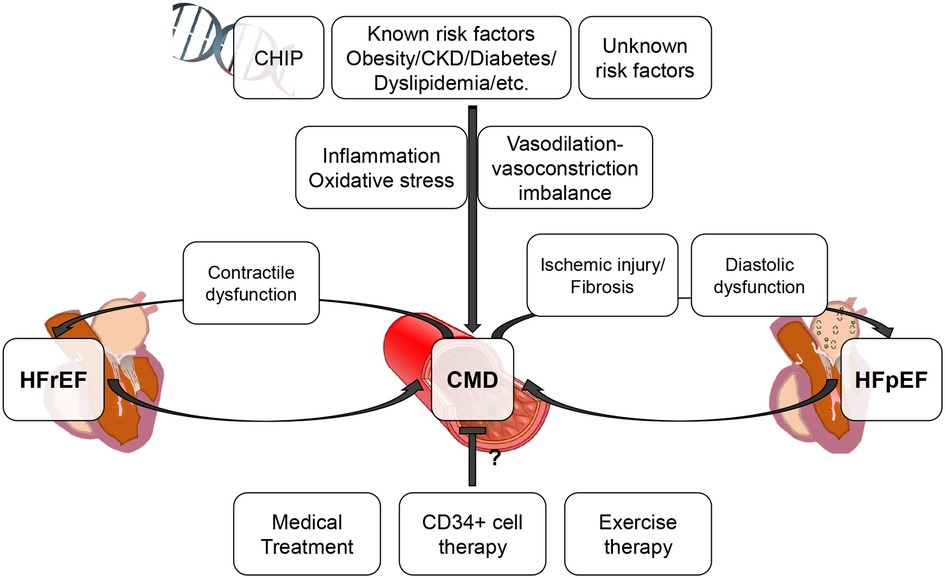
Figure 1. Conceptual association between CMD and HFpEF/HFrEF. This figure illustrates the conceptual association between CMD and HFpEF/HFrEF. CMD can be involved in the progression of HFpEF/HFrEF and associated with worse prognosis. CHIP, clonal hematopoiesis of indeterminate potential; CKD, chronic kidney disease; CMD, coronary microvascular dysfunction; HFpEF, heart failure with preserved ejection fraction; HFrEF, heart failure with reduced ejection fraction.
Incidence of CMD in patients with HFpEF
With recent advances in imaging modalities and techniques, it has been uncovered that coronary microvascular dysfunction (CMD) can frequently manifests in patients with heart failure with preserved ejection fraction (HFpEF). PROMIS-HFpEF (Prevalence of Microvascular Dysfunction in HFpEF) trial is a prospective multinational multi-center observational study enrolling 202 patients with HFpEF (left ventricular ejection fraction [LVEF] ≥40%), revealing that 75% of patients with HFpEF had CMD defined as adenosine stress transthoracic Doppler echocardiography-derived coronary flow reserve (CFR) <2.5 in the absence of unrevascularized epicardial CAD (9). One-year outcomes in patients from PROMIS-HFpEF trial showed that HFpEF patients with CMD had a significantly higher incidence rates of cardiovascular death and heart failure hospitalization than those without. Even though adverse events were detected in only 15 patients within 1 year, CMD was an independent risk factor for cardiovascular death and heart failure hospitalization (10). A caveat of this study was that the CFR measurements were performed non-invasively with echocardiography. However, another group performed invasive assessments of CMD to separately evaluate endothelium-dependent and endothelium-independent CMD, and reported a consistent prevalence of CMD in patients with HFpEF. Among 162 HFpEF patients (LVEF ≥50%), 29% had isolated endothelium-dependent CMD (defined as an increase of coronary blood flow ≤0% in response to incremental doses of intracoronary acetylcholine [10−6, 10−5, and 10−4 mol/L]: %change in CBF), 33% had isolated endothelium-independent CMD (defined as CFR <2.5 in response to incremental doses of intracoronary adenosine [18–72 μg]), and 10% had combined CMD, leaving a total of 72% with abnormal coronary microvascular function. Importantly, no single clinical characteristics could identify the presence of either endothelium-dependent or endothelium-independent CMD. Both endothelium-dependent and endothelium-independent CMD were associated with higher all-cause mortality; however, patients with endothelium-dependent CMD displayed a greater left ventricular end-diastolic dimension, while those with endothelium-independent CMD displayed impaired left ventricular diastolic function with a higher E/e’ ratio, indicating that both pathways have different effects on the pathophysiology of HFpEF (11).
Effects of CMD on hemodynamics during exercise and on myocardial injury in HFpEF
The effects of the endothelium-dependent and endothelium-independent CMD on hemodynamics during exercise were rigorously analyzed in 51 patients who underwent exercise right heart catheterization and concurrent invasive coronary reactivity testing for assessment of unexplained exertional symptoms in the absence of obstructive CAD (<50% stenosis) and impaired systolic left ventricular function (LVEF >50%). Pulmonary artery wedge pressure (PAWP) at peak exercise was significantly higher in patients with endothelium-dependent CMD or endothelium-independent CMD than those without, with a significant negative correlation between mean PAWP at peak exercise and %change in CBF in response to acetylcholine (an indicator of endothelium-dependent coronary microvascular function) or CFR (an indicator of endothelium-independent coronary microvascular function). Furthermore, this study uniquely demonstrated that higher CFR was significantly correlated with a greater exercise capacity, while there was no significant correlation between %change in CBF and exercise capacity, underlining the mechanistic importance of endothelium-independent pathway in HFpEF pathology (12). In fact, CFR <2.0 assessed with positron emission tomography was significantly associated with E/e′>15, which is an echocardiographic feature of left ventricular diastolic dysfunction in 201 patients with normal LVEF in the absence of significant epicardial CAD. Negative correlation between CFR and E/e′ was more pronounced in patients with troponin elevation compared to those without. Moreover, patients with both CFR <2 and E/e′ >15 had significantly higher incidence of HFpEF hospitalization than the other groups, indicating a causal link between myocardial ischemic injury due to CMD and diastolic dysfunction, the main underlying pathology of HFpEF (13). Another study assessing myocardial oxygen supply and demand calculated from invasively obtained PAWP and aortic pressure waveform at rest and during exercise demonstrated that HFpEF patients had markedly decreased myocardial oxygen supply which augmented during exercise, leading to an elevated oxygen supply-demand mismatch. The greater the oxygen supply-demand mismatch, the higher the troponin levels during exercise, suggesting a vicious cycle in which ischemic myocardial damage induces diastolic dysfunction, which in turn induces further myocardial damage through oxygen supply-demand mismatch (14). In contrast to previous studies, which have reported the link between myocardial ischemic injury induced by CMD and the development of HFpEF, Arnold et al. demonstrated that myocardial perfusion reserve, as assessed by cardiac magnetic resonance imaging, was not significantly associated with either late gadolinium enhancement or extracellular volume - markers of myocardial fibrosis (15). These inconsistent findings may be due to the fact that both CMD and HFpEF are heterogeneous conditions for which a single underlying mechanism cannot fully account.
Vascular dysfunction as a potential link between atrial fibrillation and HFpEF
Atrial fibrillation (AF) is frequently observed in patients with HFpEF, and its presence has been associated with poorer long-term clinical outcomes (16, 17). Comorbid AF is more strongly correlated with incident HFpEF than with heart failure with reduced ejection fraction (HFrEF) (18). Additionally, studies have demonstrated that left atrial compliance and function progressively deteriorate with increasing AF burden in HFpEF patients during the transition from paroxysmal to permanent AF (19). These findings lend support to the hypothesis that AF may serve as an indicator of the underlying pathophysiological processes in patients with HFpEF. One potential mechanism linking AF and HFpEF is endothelial dysfunction (20). A previous study demonstrated that patients with epicardial and/or coronary microvascular endothelial dysfunction had a 5.8-fold increased relative risk of developing AF compared to those without coronary endothelial dysfunction (21). Indeed, inflammatory endothelial activation characterized by upregulation of E-selectin and intercellular adhesion molecule was observed in HFpEF patients, accompanied by increased oxidative stress in the endothelial cells and impaired NO-dependent signaling in the myocardium, potentially leading to cardiomyocyte stiffening and hypertrophy in HFpEF patients (22, 23). Inflammation in the heart or systemic circulation may in turn alter the electrophysiology and structural substrate of the atria, thus increasing their vulnerability to atrial fibrillation (24). The potential interplay between vascular dysfunction and AF development in HFpEF warrants further investigation.
Risk factors and potential treatment of CMD in HFpEF patients
Endothelium-independent CMD, characterized by a decline in coronary flow reserve - defined as the ratio of hyperemic flow to resting flow - can be attributed to either increased resting flow or decreased hyperemic flow. The former subtype has been linked to dysfunctional autoregulation of the coronary microvasculature, while the latter has been associated with structural microvascular alterations in CMD. In diabetic patients, a decline in CFR has been observed in conjunction with increasing resting coronary flow (25, 26). In a swine model of CMD induced by multiple risk factors (diabetes mellitus, hypercholesterolemia, and chronic kidney disease), a decline in CFR due to increased resting flow was observed, and myocardial efficiency was found to be lower, requiring higher oxygen consumption for a given level of myocardial work (27). This animal model also exhibited left ventricular diastolic dysfunction, a key feature of HFpEF, potentially through systemic inflammation and oxidative stress accompanied by metabolic alterations in glucose and fatty acid (28, 29). Interestingly, a recent study reported a possible beneficial effect of sodium-glucose cotransporter 2 inhibitors on CMD by decreasing resting coronary flow and thereby increasing myocardial flow reserve in diabetic patients (30). However, another study assessing the effects of sodium-glucose cotransporter 2 inhibitors on microvascular flow reserve in diabetic patients failed to improve it (31). The difference between these two studies is that the former included diabetic patients with diabetes duration of less than 10 years, while the latter included those with a median diabetes duration of more than 10 years. One study indicated that CMD subtypes could alter from functional to structural as the duration of diabetes exceeds ten years (32). The underlying pathophysiology of the decrease in CFR may differ between patients with increased resting flow (functional CMD) and those with decreased hyperemic flow (structural CMD), both of which equally contribute to worsening clinical outcomes (33); however, response to therapeutic agents can vary between these two distinct CMD subtypes. Obesity has also been linked to functional and structural CMD in clinical and experimental studies (34). Vasodilation-vasoconstriction imbalance associated with obesity can lead to functional microvascular vasomotor dysfunction (35). Further, histological analyses showed that coronary capillary rarefaction was observed in obese patients and animals (36–38). Chronic kidney disease is another risk factor that has induced CMD and HFpEF in experimental animals. A large community-based cohort study has also shown an association between chronic kidney disease and new-onset HFpEF (39). Impaired renal clearance of uremic toxins can lead to a chronic inflammatory state and systemic endothelial dysfunction, including coronary microvascular endothelial dysfunction (40). Coronary endothelial cells can modulate left ventricular diastolic function via the paracrine effect (41); therefore, coronary microvascular endothelial dysfunction with parallel pathological changes in systemic endothelium can be the link between chronic kidney disease and HFpEF. Various studies are ongoing to evaluate drug therapy for CMD. The CorMicA trial reported the efficacy of stratified medical treatment based on the assessment using invasive coronary function testing in patients with angina and no obstructive CAD (42, 43). However, there is currently no established treatment selection based on different CMD subtypes. Furthermore, data is lacking on whether CMD can be a therapeutic target for HFpEF patients (44). In fact, rarefaction of the coronary microvasculature has been observed in postmortem HFpEF patients, and the extent of coronary rarefaction has been found to correlate with myocardial fibrosis (45). CMD patients with advanced structural alterations may have limited benefits from pharmacotherapy. Recently published phase II trials reported the beneficial effects of CD34 + cell therapy on CMD, suggesting that CD34 + cell therapy may be a promising new treatment strategy for CMD patients with advanced structural microvascular alterations potentially through angiogenesis (46–48). In addition to CMD subtypes, sex differences should also be considered. Women with angina and no obstructive CAD were shown to have lower CFR due to higher resting coronary flow compared to men, implicating the sex difference in coronary physiology (49). A recent study that performed sex-specific proteomic profiling associated with CMD in patients with HFpEF from PROMIS-HFpEF trial demonstrated that CMD was related to the inflammation-mediated chemokine and cytokine signaling pathway among men with HFpEF, and the P13-kinase and transforming growth factor-beta signaling pathway among women with HFpEF. Thus, reduction of inflammation can potentially be more important than neurohormonal modulation in men, while neurohormonal blockade may be more effective in women to prevent left ventricular remodeling and myocardial fibrosis (50). The previous observation that treatment with sacubitril-valsartan more significantly reduces heart failure hospitalizations in women than in men with HFpEF supports this hypothesis (51). A recent finding related to inflammation is the interesting association between clonal hematopoiesis of indeterminate potential (CHIP), particularly in TET2 and DNMT3A, and an increased risk of cardiovascular disease, possibly via the interleukin-6 (IL-6) signaling pathway (52, 53). Another study evaluating the association between CMD and CHIP reported that CHIP in TET2 and DNMT3A is an independent risk factor for CMD, with CHIP mediating 32% of the increased risk of major adverse cardiovascular events in CMD patients (54). Higher levels of IL-6 were associated with all-cause or cardiovascular death and heart failure hospitalization in patients recently hospitalized with HFpEF, even after adjustment for other risk factors (55). These data may highlight anti-inflammatory therapy as a potential therapeutic target for HFpEF and CMD. Finally, as a non-pharmacologic therapy, there is growing evidence regarding exercise therapy for patients with HFpEF (56). Although exercise training is associated with improved peak oxygen uptake and exercise tolerance in patients with HFpEF, a meta-analysis of randomized controlled trials showed that improvements in cardiorespiratory function and quality of life with exercise training were not accompanied by improvements in left ventricular systolic and diastolic dysfunction in HFpEF patients (57). A recent study examining the effects of exercise therapy showed no change in peripheral vascular function or endothelial repair capacity despite improvement in peak oxygen uptake with aerobic exercise training (58). Further studies are needed to determine the effects of exercise training on coronary microvascular function.
CMD and HFrEF
There is scarce evidence for an association between CMD and HFrEF in comparison to HFpEF. Given the systemic nature of microvascular disease (59–64), the fact that diabetic microvascular complications (neuropathy, nephropathy, and retinopathy) were associated with worse clinical outcomes in patients with HFpEF or HFrEF may indicate the pathophysiologic roles of CMD on both HFpEF and HFrEF (65). Notably, the accumulation of microvascular complications has been associated with an increased incidence of cardiac hypertrophy in patients with HFpEF, while it has been associated with a decreased incidence of cardiac hypertrophy in patients with HFrEF, indicating the differential impact of CMD on HFpEF and HFrEF (65). In fact, a previous study assessing the correlation between regional coronary flow reserve (CFR) and contractile reserve in patients with dilated cardiomyopathy revealed a significant positive correlation between CFR and contractile reserve in the left anterior descending and left circumflex territories. Furthermore, decreased CFR was correlated with increased left ventricular end-diastolic pressure in the same regions, highlighting the importance of microvascular reserve in maintaining myocardial contractile function (66). Small studies have evaluated the impact of CFR on clinical outcomes in patients with HFrEF and have consistently shown that abnormal CFR predicts worse clinical outcomes (67, 68). A retrospective study including 510 HFrEF patients who underwent rest/stress myocardial perfusion positron emission tomography to quantify CFR found that lower CFR (≤1.65) was associated with a two-fold increased risk of major adverse cardiovascular events compared to those with higher CFR (>1.65), supporting previous observations in a larger cohort (69). When factors contributing to decreased CFR are divided into structural CMD due to decreased hyperemic flow and functional CMD due to increased resting flow, it has been reported that decreased hyperemic flow increases the relative risk of death and heart failure onset and progression by 3.5 times (70). However, a recent report from a large registry data demonstrated that structural and functional CMD contributed equally to worsening clinical outcomes. Notably, CFR was lower in patients with reduced LVEF than those with preserved LVEF, primarily due to increased resting flow. The mechanism of increased resting flow in patients with reduced LVEF is unclear; however, it may be attributed to increased left ventricular end-diastolic pressure and increased myocardial oxygen demand due to elevated heart rate and left ventricular mass (71).
Summary and future perspectives
Coronary microvascular dysfunction can often coexist in heart failure patients with preserved and reduced ejection fractions. Although the underlying pathophysiology of functional or structural CMD may differ, both may equally contribute to worse clinical outcomes. There is a paucity of available data on whether comorbid CMD is a potential target for heart failure therapy. Furthermore, it needs to be clarified whether different CMD subtypes respond differently to a treatment. However, further phenotypic assessment of heart failure patients may pave the way to a clearer understanding of the pathogenesis of heart failure and the unmet clinical needs of heart failure patients.
Author contributions
TT drafted and revised manuscript. The other authors made critical revisions. All authors contributed to the article and approved the submitted version.
Conflict of interest
The authors declare that the research was conducted in the absence of any commercial or financial relationships that could be construed as a potential conflict of interest.
Publisher's note
All claims expressed in this article are solely those of the authors and do not necessarily represent those of their affiliated organizations, or those of the publisher, the editors and the reviewers. Any product that may be evaluated in this article, or claim that may be made by its manufacturer, is not guaranteed or endorsed by the publisher.
Abbreviations
AF, atrial fibrillation; CAD, coronary artery disease; CBF, coronary blood flow; CHIP, clonal hematopoiesis of indeterminate potential; CMD, coronary microvascular dysfunction; CFR, coronary flow reserve; HFpEF, heart failure with preserved ejection fraction; HFrEF, heart failure with reduced ejection fraction; LVEF, left ventricular ejection fraction; PCI, percutaneous coronary intervention; PAWP, pulmonary artery wedge pressure.
References
1. Maron DJ, Hochman JS, Reynolds HR, Bangalore S, O’Brien SM, Boden WE, et al. Initial invasive or conservative strategy for stable coronary disease. N Engl J Med. (2020) 382:1395–407. doi: 10.1056/NEJMoa1915922
2. Perera D, Clayton T, O’Kane PD, Greenwood JP, Weerackody R, Ryan M, et al. REVIVED-BCIS2 Investigators. Percutaneous revascularization for ischemic left ventricular dysfunction. N Engl J Med. (2022) 387:1351–60. doi: 10.1056/NEJMoa2206606
3. Duncker DJ, Bache RJ. Regulation of coronary blood flow during exercise. Physiol Rev. (2008) 88:1009–86. doi: 10.1152/physrev.00045.2006
4. Lombardo TA, Rose L, Taeschler M, Tuluy S, Bing RJ. The effect of exercise on coronary blood flow, myocardial oxygen consumption and cardiac efficiency in man. Circulation. (1953) 7:71–8. doi: 10.1161/01.CIR.7.1.71
5. Sara JD, Widmer RJ, Matsuzawa Y, Lennon RJ, Lerman LO, Lerman A. Prevalence of coronary microvascular dysfunction among patients with chest pain and nonobstructive coronary artery disease. JACC Cardiovasc Interv. (2015) 8:1445–53. doi: 10.1016/j.jcin.2015.06.017
6. Toya T, Ahmad A, Corban MT, Ozcan I, Sara JD, Sebaali F, et al. Risk stratification of patients with NonObstructive coronary artery disease using resistive reserve ratio. J Am Heart Assoc. (2021) 10:e020464. doi: 10.1161/JAHA.120.020464
7. Toya T, Corban MT, Park JY, Ahmad A, Ozcan I, Sebaali F, et al. Prognostic impact and clinical outcomes of coronary flow reserve and hyperaemic microvascular resistance. EuroIntervention. (2021) 17:569–75. doi: 10.4244/EIJ-D-20-00853
8. Sinha A, Rahman H, Webb A, Shah AM, Perera D. Untangling the pathophysiologic link between coronary microvascular dysfunction and heart failure with preserved ejection fraction. Eur Heart J. (2021) 42:4431–41. doi: 10.1093/eurheartj/ehab653
9. Shah SJ, Lam CSP, Svedlund S, Saraste A, Hage C, Tan R-S, et al. Prevalence and correlates of coronary microvascular dysfunction in heart failure with preserved ejection fraction: PROMIS-HFpEF. Eur Heart J. (2018) 39:3439–50. doi: 10.1093/eurheartj/ehy531
10. Hage C, Svedlund S, Saraste A, Faxén UL, Benson L, Fermer ML, et al. Association of coronary microvascular dysfunction with heart failure hospitalizations and mortality in heart failure with preserved ejection fraction: a follow-up in the PROMIS-HFpEF study. J Card Fail. (2020) 26:1016–21. doi: 10.1016/j.cardfail.2020.08.010
11. Yang JH, Obokata M, Reddy YN, Redfield MM, Lerman A, Borlaug BA. Endothelium-dependent and independent coronary microvascular dysfunction in patients with heart failure with preserved ejection fraction. Eur J Heart Fail. (2020) 22:432–41. doi: 10.1002/ejhf.1671
12. Ahmad A, Corban MT, Toya T, Verbrugge FH, Sara JD, Lerman LO, et al. Coronary microvascular dysfunction is associated with exertional haemodynamic abnormalities in patients with heart failure with preserved ejection fraction. Eur J Heart Fail. (2021) 23:765–72. doi: 10.1002/ejhf.2010
13. Taqueti VR, Solomon SD, Shah AM, Desai AS, Groarke JD, Osborne MT, et al. Coronary microvascular dysfunction and future risk of heart failure with preserved ejection fraction. Eur Heart J. (2018) 39:840–9. doi: 10.1093/eurheartj/ehx721
14. Obokata M, Reddy YNV, Melenovsky V, Kane GC, Olson TP, Jarolim P, et al. Myocardial injury and cardiac reserve in patients with heart failure and preserved ejection fraction. J Am Coll Cardiol. (2018) 72:29–40. doi: 10.1016/j.jacc.2018.04.039
15. Arnold JR, Kanagala P, Budgeon CA, Jerosch-Herold M, Gulsin GS, Singh A, et al. Prevalence and prognostic significance of microvascular dysfunction in heart failure with preserved ejection fraction. JACC Cardiovasc Imaging. (2022) 15:1001–11. doi: 10.1016/j.jcmg.2021.11.022
16. Patel RB, Greene SJ, Xu H, Alhanti B, Peterson P, Yancy CW, et al. Intersection of atrial fibrillation and heart failure with mildly reduced and preserved ejection fraction in >400 000 participants in the get with the guidelines-heart failure registry. Eur J Heart Fail. (2022) 25:63–73. doi: 10.1002/ejhf.2729
17. Cikes M, Planinc I, Claggett B, Cunningham J, Milicic D, Sweitzer N, et al. Atrial fibrillation in heart failure with preserved ejection fraction: the PARAGON-HF trial. JACC Heart Fail. (2022) 10:336–46. doi: 10.1016/j.jchf.2022.01.018
18. Santhanakrishnan R, Wang N, Larson MG, Magnani JW, McManus DD, Lubitz SA, et al. Atrial fibrillation begets heart failure and vice versa: temporal associations and differences in preserved versus reduced ejection fraction. Circulation. (2016) 133:484–92. doi: 10.1161/CIRCULATIONAHA.115.018614
19. Reddy YNV, Obokata M, Verbrugge FH, Lin G, Borlaug BA. Atrial dysfunction in patients with heart failure with preserved ejection fraction and atrial fibrillation. J Am Coll Cardiol. (2020) 76:1051–64. doi: 10.1016/j.jacc.2020.07.009
20. Corban MT, Toya T, Ahmad A, Lerman LO, Lee H-C, Lerman A. Atrial fibrillation and endothelial dysfunction: a potential link? Mayo Clin Proc. (2021) 96:1609–21. doi: 10.1016/j.mayocp.2020.11.005
21. Corban MT, Godo S, Burczak DR, Noseworthy PA, Toya T, Lewis BR, et al. Coronary endothelial dysfunction is associated with increased risk of incident atrial fibrillation. J Am Heart Assoc. (2020) 9:e014850. doi: 10.1161/JAHA.119.014850
22. Paulus WJ, Tschöpe C. A novel paradigm for heart failure with preserved ejection fraction: comorbidities drive myocardial dysfunction and remodeling through coronary microvascular endothelial inflammation. J Am Coll Cardiol. (2013) 62:263–71. doi: 10.1016/j.jacc.2013.02.092
23. Franssen C, Chen S, Unger A, Korkmaz HI, De Keulenaer GW, Tschöpe C, et al. Myocardial microvascular inflammatory endothelial activation in heart failure with preserved ejection fraction. JACC Heart Fail. (2016) 4:312–24. doi: 10.1016/j.jchf.2015.10.007
24. Hu Y-F, Chen Y-J, Lin Y-J, Chen S-A. Inflammation and the pathogenesis of atrial fibrillation. Nat Rev Cardiol. (2015) 12:230–43. doi: 10.1038/nrcardio.2015.2
25. Haas AV, Rosner BA, Kwong RY, Rao AD, Garg R, Di Carli MF, et al. Sex differences in coronary microvascular function in individuals with type 2 diabetes. Diabetes. (2019) 68:631–6. doi: 10.2337/db18-0650
26. Picchi A, Limbruno U, Focardi M, Cortese B, Micheli A, Boschi L, et al. Increased basal coronary blood flow as a cause of reduced coronary flow reserve in diabetic patients. Am J Physiol Heart Circ Physiol. 2011;301:H2279–2284. doi: 10.1152/ajpheart.00615.2011
27. van de Wouw J, Sorop O, van Drie RWA, van Duin RWB, Nguyen ITN, Joles JA, et al. Perturbations in myocardial perfusion and oxygen balance in swine with multiple risk factors: a novel model of ischemia and no obstructive coronary artery disease. Basic Res Cardiol. (2020) 115:21. doi: 10.1007/s00395-020-0778-2
28. Sorop O, Heinonen I, van Kranenburg M, van de Wouw BV, Nguyen ITN, Octavia Y, et al. Multiple common comorbidities produce left ventricular diastolic dysfunction associated with coronary microvascular dysfunction, oxidative stress, and myocardial stiffening. Cardiovasc Res. (2018) 114:954–64. doi: 10.1093/cvr/cvy038
29. Heinonen I, Sorop O, van Dalen BM, Wüst RCI, van de Wouw J, de Beer VJ, et al. Cellular, mitochondrial and molecular alterations associate with early left ventricular diastolic dysfunction in a porcine model of diabetic metabolic derangement. Sci Rep. (2020) 10:13173. doi: 10.1038/s41598-020-68637-4
30. Leccisotti L, Cinti F, Sorice GP, D’Amario D, Lorusso M, Guzzardi MA, et al. Dapagliflozin improves myocardial flow reserve in patients with type 2 diabetes: the DAPAHEART trial: a preliminary report. Cardiovasc Diabetol. (2022) 21:173. doi: 10.1186/s12933-022-01607-4
31. Jürgens M, Schou M, Hasbak P, Kjær A, Wolsk E, Zerahn B, et al. Effects of empagliflozin on myocardial flow reserve in patients with type 2 diabetes Mellitus: the SIMPLE trial. J Am Heart Assoc. (2021) 10:e020418. doi: 10.1161/JAHA.120.020418
32. Sezer M, Kocaaga M, Aslanger E, Atici A, Demirkiran A, Bugra Z, et al. Bimodal pattern of coronary microvascular involvement in diabetes Mellitus. J Am Heart Assoc. (2016) 5:e003995. doi: 10.1161/JAHA.116.003995
33. Boerhout CKM, de Waard GA, Lee JM, Mejia-Renteria H, Lee SH, Jung J-H, et al. Prognostic value of structural and functional coronary microvascular dysfunction in patients with non-obstructive coronary artery disease; from the multicentre international ILIAS registry. EuroIntervention. (2022) 18:719–28. doi: 10.4244/EIJ-D-22-00043
34. Sorop O, Olver TD, van de Wouw J, Heinonen I, van Duin RW, Duncker DJ, et al. The microcirculation: a key player in obesity-associated cardiovascular disease. Cardiovasc Res. (2017) 113:1035–45. doi: 10.1093/cvr/cvx093
35. Berwick ZC, Dick GM, Tune JD. Heart of the matter: coronary dysfunction in metabolic syndrome. J Mol Cell Cardiol. (2012) 52:848–56. doi: 10.1016/j.yjmcc.2011.06.025
36. Campbell DJ, Somaratne JB, Prior DL, Yii M, Kenny JF, Newcomb AE, et al. Obesity is associated with lower coronary microvascular density. PLoS One. (2013) 8:e81798. doi: 10.1371/journal.pone.0081798
37. Gruber C, Kohlstedt K, Loot AE, Fleming I, Kummer W, Mühlfeld C. Stereological characterization of left ventricular cardiomyocytes, capillaries, and innervation in the nondiabetic, obese mouse. Cardiovasc Pathol. (2012) 21:346–54. doi: 10.1016/j.carpath.2011.11.003
38. Sorop O, van den Heuvel M, van Ditzhuijzen NS, de Beer VJ, Heinonen I, van Duin RWB, et al. Coronary microvascular dysfunction after long-term diabetes and hypercholesterolemia. Am J Physiol Heart Circ Physiol. (2016) 311:H1339–51. doi: 10.1152/ajpheart.00458.2015
39. Brouwers FP, de Boer RA, van der Harst P, Voors AA, Gansevoort RT, Bakker SJ, et al. Incidence and epidemiology of new onset heart failure with preserved vs. Reduced ejection fraction in a community-based cohort: 11-year follow-up of PREVEND. Eur Heart J. (2013) 34:1424–31. doi: 10.1093/eurheartj/eht066
40. Ter Maaten JM, Damman K, Verhaar MC, Paulus WJ, Duncker DJ, Cheng C, et al. Connecting heart failure with preserved ejection fraction and renal dysfunction: the role of endothelial dysfunction and inflammation. Eur J Heart Fail. (2016) 18:588–98. doi: 10.1002/ejhf.497
41. Paulus WJ. Paracrine coronary endothelial modulation of diastolic left ventricular function in man: implications for diastolic heart failure. J Card Fail. (1996) 2:S155–164. doi: 10.1016/S1071-9164(96)80072-8
42. Ford TJ, Stanley B, Good R, Rocchiccioli P, McEntegart M, Watkins S, et al. Stratified medical therapy using invasive coronary function testing in angina: the CorMicA trial. J Am Coll Cardiol. (2018) 72:2841–55. doi: 10.1016/j.jacc.2018.09.006
43. Ford TJ, Ong P, Sechtem U, Beltrame J, Camici PG, Crea F, et al. Assessment of vascular dysfunction in patients without obstructive coronary artery disease. JACC Cardiovasc Interv. (2020) 13:1847–64. doi: 10.1016/j.jcin.2020.05.052
44. Berry C, Sykes R. Microvascular dysfunction in heart failure with preserved ejection fraction: a modifiable therapeutic target? JACC Cardiovasc Imaging. (2022) 15:1012–4. doi: 10.1016/j.jcmg.2022.04.008
45. Mohammed SF, Hussain S, Mirzoyev SA, Edwards WD, Maleszewski JJ, Redfield MM. Coronary microvascular rarefaction and myocardial fibrosis in heart failure with preserved ejection fraction. Circulation. (2015) 131:550–9. doi: 10.1161/CIRCULATIONAHA.114.009625
46. Henry TD, Bairey Merz CN, Wei J, Corban MT, Quesada O, Joung S, et al. Autologous CD34+ stem cell therapy increases coronary flow reserve and reduces angina in patients with coronary microvascular dysfunction. Circ Cardiovasc Interv. (2022) 15:e010802. doi: 10.1161/CIRCINTERVENTIONS.122.012528
47. Corban MT, Toya T, Albers D, Sebaali F, Lewis BR, Bois J, et al. IMPROvE-CED trial: intracoronary autologous CD34+ cell therapy for treatment of coronary endothelial dysfunction in patients with angina and nonobstructive coronary arteries. Circ Res. (2022) 130:326–38. doi: 10.1161/CIRCRESAHA.121.319644
48. Bairey Merz CN, Marbán E. Stem cell therapy targets: repêchage!. Circ Res. (2022) 130:339–42. doi: 10.1161/CIRCRESAHA.121.320663
49. Corban MT, Prasad A, Gulati R, Lerman LO, Lerman A. Sex-specific differences in coronary blood flow and flow velocity reserve in symptomatic patients with non-obstructive disease. EuroIntervention. (2021) 16:1079–84. doi: 10.4244/EIJ-D-19-00520
50. Chandramouli C, Ting TW, Tromp J, Agarwal A, Svedlund S, Saraste A, et al. Sex differences in proteomic correlates of coronary microvascular dysfunction among patients with heart failure and preserved ejection fraction. Eur J Heart Fail. (2022) 24:681–4. doi: 10.1002/ejhf.2435
51. McMurray JJV, Jackson AM, Lam CSP, Redfield MM, Anand IS, Ge J, et al. Effects of sacubitril-valsartan versus valsartan in women compared with men with heart failure and preserved ejection fraction. Circulation. (2020) 141:338–51. doi: 10.1161/CIRCULATIONAHA.119.044491
52. Libby P, Sidlow R, Lin AE, Gupta D, Jones LW, Moslehi J, et al. Clonal hematopoiesis: crossroads of aging, cardiovascular disease, and cancer: jACC review topic of the week. J Am Coll Cardiol. (2019) 74:567–77. doi: 10.1016/j.jacc.2019.06.007
53. Bick AG, Pirruccello JP, Griffin GK, Gupta N, Gabriel S, Saleheen D, et al. Genetic interleukin 6 signaling deficiency attenuates cardiovascular risk in clonal hematopoiesis. Circulation. (2020) 141:124–31. doi: 10.1161/CIRCULATIONAHA.119.044362
54. Akhiyat N, Lasho TL, Ganji M, Toya T, Shi C-X, Chen X, et al. Clonal hematopoiesis of indeterminate potential is associated with coronary microvascular dysfunction in early nonobstructive coronary artery disease. Arterioscler Thromb Vasc Biol. (2023) 43(5):774–83. doi: 10.1161/ATVBAHA.122.318928
55. Mooney L, Jackson CE, Adamson C, McConnachie A, Welsh P, Myles RC, et al. Adverse outcomes associated with interleukin-6 in patients recently hospitalized for heart failure with preserved ejection fraction. Circ Heart Fail. (2023) 16(4):e010051. doi: 10.1161/CIRCHEARTFAILURE.122.010051
56. Heinonen I, Sorop O, de Beer VJ, Duncker DJ, Merkus D. What can we learn about treating heart failure from the heart’s response to acute exercise? Focus on the coronary microcirculation. J Appl Physiol (1985). (2015) 119:934–43. doi: 10.1152/japplphysiol.00053.2015
57. Pandey A, Parashar A, Kumbhani D, Agarwal S, Garg J, Kitzman D, et al. Exercise training in patients with heart failure and preserved ejection fraction: meta-analysis of randomized control trials. Circ Heart Fail. (2015) 8:33–40. doi: 10.1161/CIRCHEARTFAILURE.114.001615
58. Gevaert AB, Böhm B, Hartmann H, Goovaerts I, Stoop T, Van De Heyning CM, et al. Effect of training on vascular function and repair in heart failure with preserved ejection fraction. JACC: Heart Fail. (2023) 11(4):454–64. doi: 10.1016/j.jchf.2022.12.011
59. Toya T, Sara JD, Corban MT, Taher R, Godo S, Herrmann J, et al. Assessment of peripheral endothelial function predicts future risk of solid-tumor cancer. Eur J Prev Cardiol. (2020) 27:608–18. doi: 10.1177/2047487319884246
60. Toya T, Sara JD, Ahmad A, Nardi V, Taher R, Lerman LO, et al. Incremental prognostic impact of peripheral microvascular endothelial dysfunction on the development of ischemic stroke. J Am Heart Assoc. (2020) 9:e015703. doi: 10.1161/JAHA.119.015703
61. Toya T, Sara JD, Scharf EL, Ahmad A, Nardi V, Ozcan I, et al. Impact of peripheral microvascular endothelial dysfunction on white matter hyperintensity. J Am Heart Assoc. (2021) 10:e021066. doi: 10.1161/JAHA.121.021722
62. Toya T, Ahmad A, Attia Z, Cohen-Shelly M, Ozcan I, Noseworthy PA, et al. Vascular aging detected by peripheral endothelial dysfunction is associated with ECG-derived physiological aging. J Am Heart Assoc. (2021) 10:e018656. doi: 10.1161/JAHA.120.018656
63. Rajai N, Ahmad A, Toya T, Sara JD, Herrmann J, Lerman LO, et al. Coronary microvascular dysfunction is an independent predictor of developing cancer in patients with non-obstructive coronary artery disease (NOCAD). Eur J Prev Cardiol. (2022) 30:209–16. doi: 10.1093/eurjpc/zwac184
64. Bonetti PO, Pumper GM, Higano ST, Holmes DR, Kuvin JT, Lerman A. Noninvasive identification of patients with early coronary atherosclerosis by assessment of digital reactive hyperemia. J Am Coll Cardiol. (2004) 44:2137–41. doi: 10.1016/j.jacc.2004.08.062
65. Tromp J, Lim SL, Tay WT, Teng TK, Chandramouli C, Ouwerkerk W, et al. Microvascular disease in patients with diabetes with heart failure and reduced ejection versus preserved ejection fraction. Diabetes Care. (2019) 42:1792–9. doi: 10.2337/dc18-2515
66. Skalidis EI, Parthenakis FI, Patrianakos AP, Hamilos MI, Vardas PE. Regional coronary flow and contractile reserve in patients with idiopathic dilated cardiomyopathy. J Am Coll Cardiol. (2004) 44:2027–32. doi: 10.1016/j.jacc.2004.08.051
67. Rigo F, Gherardi S, Galderisi M, Sicari R, Picano E. The independent prognostic value of contractile and coronary flow reserve determined by dipyridamole stress echocardiography in patients with idiopathic dilated cardiomyopathy. Am J Cardiol. (2007) 99:1154–8. doi: 10.1016/j.amjcard.2006.11.049
68. Anantharam B, Janardhanan R, Hayat S, Hickman M, Chahal N, Bassett P, et al. Coronary flow reserve assessed by myocardial contrast echocardiography predicts mortality in patients with heart failure. Eur J Echocardiogr. (2011) 12:69–75. doi: 10.1093/ejechocard/jeq109
69. Majmudar MD, Murthy VL, Shah RV, Kolli S, Mousavi N, Foster CR, et al. Quantification of coronary flow reserve in patients with ischaemic and non-ischaemic cardiomyopathy and its association with clinical outcomes. Eur Heart J Cardiovasc Imaging. (2015) 16:900–9. doi: 10.1093/ehjci/jev012
70. Neglia D, Michelassi C, Trivieri MG, Sambuceti G, Giorgetti A, Pratali L, et al. Prognostic role of myocardial blood flow impairment in idiopathic left ventricular dysfunction. Circulation. (2002) 105:186–93. doi: 10.1161/hc0202.102119
Keywords: coronary physiology, coronary microvascular dysfunction, heart failure with reduced ejection fraction, heart failure with preserved ejection fraction, coronary flow reserve
Citation: Toya T, Nagatomo Y, Ikegami Y, Masaki N and Adachi T (2023) Coronary microvascular dysfunction in heart failure patients. Front. Cardiovasc. Med. 10:1153994. doi: 10.3389/fcvm.2023.1153994
Received: 30 January 2023; Accepted: 23 May 2023;
Published: 2 June 2023.
Edited by:
Branko Dusan Beleslin, Institute for Cardiovascular Diseases, Clinical Center of Serbia, SerbiaReviewed by:
Ilkka HA Heinonen, University of Turku, Finland© 2023 Toya, Nagatomo, Ikegami, Masaki and Adachi. This is an open-access article distributed under the terms of the Creative Commons Attribution License (CC BY). The use, distribution or reproduction in other forums is permitted, provided the original author(s) and the copyright owner(s) are credited and that the original publication in this journal is cited, in accordance with accepted academic practice. No use, distribution or reproduction is permitted which does not comply with these terms.
*Correspondence: Takumi Toya con367@ndmc.ac.jp
†ORCID Takumi Toya orcid.org/0000-0002-4681-2798