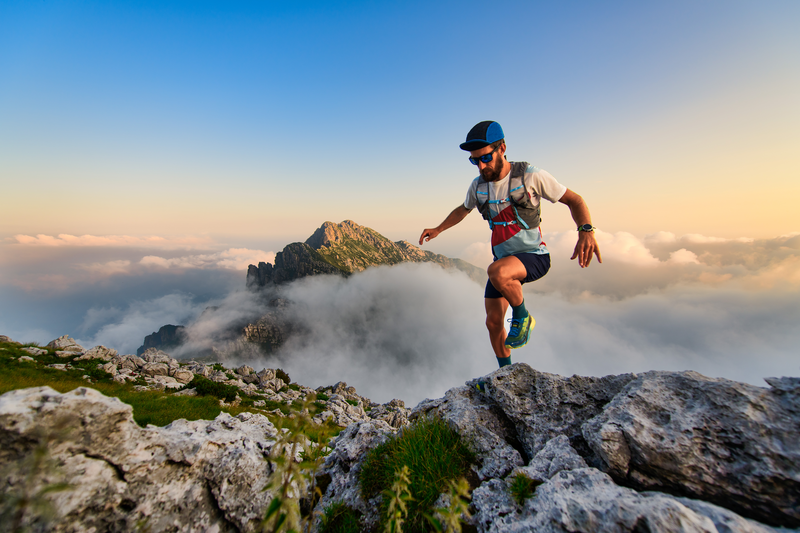
94% of researchers rate our articles as excellent or good
Learn more about the work of our research integrity team to safeguard the quality of each article we publish.
Find out more
REVIEW article
Front. Cardiovasc. Med. , 26 April 2023
Sec. Cardiac Rhythmology
Volume 10 - 2023 | https://doi.org/10.3389/fcvm.2023.1142429
This article is part of the Research Topic Frontiers in Cardiovascular Medicine: Rising Stars 2022 View all 75 articles
Cardiac electrophysiology is a constantly evolving speciality that has benefited from technological innovation and refinements over the past several decades. Despite the potential of these technologies to reshape patient care, their upfront costs pose a challenge to health policymakers who are responsible for the assessment of the novel technology in the context of increasingly limited resources. In this context, it is critical for new therapies or technologies to demonstrate that the measured improvement in patients' outcomes for the cost of achieving that improvement is within conventional benchmarks for acceptable health care value. The field of Health Economics, specifically economic evaluation methods, facilitates this assessment of value in health care. In this review, we provide an overview of the basic principles of economic evaluation and provide historical applications within the field of cardiac electrophysiology. Specifically, the cost-effectiveness of catheter ablation for both atrial fibrillation (AF) and ventricular tachycardia, novel oral anticoagulants for stroke prevention in AF, left atrial appendage occlusion devices, implantable cardioverter defibrillators, and cardiac resynchronization therapy will be reviewed.
Cardiac electrophysiology has undergone substantial innovation over the past several decades. From the advent of leadless pacemakers and physiologic pacing (1–3) to newer catheter ablation techniques that use cryoablation and electroporation (4), electrophysiology is expanding rapidly with cutting-edge clinical technologies. Despite the potential of these technologies to reshape patient care, their upfront costs garner criticism from health policymakers who are responsible for the assessment of the novel technology in the context of increasingly limited resources. For instance, in the United States, health care costs as a proportion of the economy have risen dramatically over time. They now represent 19.7% of gross domestic product (GDP), up from 5.0% of GDP in 1960 (5). The European Union has seen healthcare costs per capita rise between 2012 and 2019, exceeding growth rates of gross domestic product per capita (6).
Figure 1. Cost-effectiveness plane. The horizontal axis represents the difference in benefits between the two therapies (e.g., difference in quality-adjusted life expectancy) and the vertical axis represents the difference in the cost. Quadrant II of the plane represents a situation where a new treatment “dominates” or is always acceptable than the comparator treatment; it reflects a situation where the new therapy is less costly and more effective. The opposite occurs in Quadrant IV, where the existing therapy dominates the new treatment. Quadrant I is where a new therapy is both more effective and most costly. If the incremental benefits are attained for acceptable incremental costs (this relationship is represented by the slope of the dotted line), then the new treatment is acceptable. [Reproduced from EP Europace 2011; 13(Suppl_2):ii3–ii8 with permission].
In this context, it is critical for new therapies or technologies to demonstrate that the measured improvement in patients' outcomes for the cost of achieving that improvement is within conventional benchmarks for acceptable health care value. The field of Health Economics, specifically economic evaluation methods, facilitates this assessment of value in health care.
This review will provide an overview of economic evaluation to assess value in healthcare and discuss selected historical applications within cardiac electrophysiology. Specifically, catheter ablation for both atrial fibrillation (AF) and ventricular tachycardia (VT), novel oral anticoagulant agents for stroke prevention in AF, left atrial appendage occlusion (LAAO) devices, implantable cardioverter defibrillators (ICD), and cardiac resynchronization therapy (CRT) will be reviewed.
Health policymakers are faced with decisions that pertain to which new therapies or technologies to adopt. In the field of health economics, these challenges are related to the fundamental principle of “opportunity cost,” which is defined as the potential benefits lost or forgone from other alternatives by choosing one alternative (7). Using leadless pacemakers as an example in the Norwegian Health System, Fagerlund and colleagues estimated that adoption of the Micra pacemaker over traditional transvenous pacemakers in 80 patients at high risk for complication from transvenous pacemaker implantation would require approximate 5 million Norwegian Krone per year (approximately 0.5 million US Dollars). If holding the health budget constant, adoption of the Micra pacemaker would require decreased funding elsewhere in the health budget, or more broadly, decreasing the budget elsewhere in the social sector to increase healthcare sector spending (8).
To help facilitate health policy decisions, economic evaluation is a field that assesses the “value” of a new technology or therapy. That is, such an evaluation poses the question: does a new technology represent good value for money? Does a new therapy provide additional benefits compared to conventional care for a reasonable price? The relationship between benefits and costs can be summarized as an incremental cost effectiveness ratio (ICER) (9, 10).
For value comparisons across medicine, the ICER is most commonly assigned the units of a cost ($) per quality-adjusted life year (QALY) gained. QALYs represent patient life expectancy adjusted by quality-of-life. That is, each year of life expectancy is scaled by the quality of life experienced by the patient for a given disease, e.g., heart failure. This scaling factor, also known as a utility, can range from 0 to 1, where 1 denotes perfect health and 0 denotes death (11).
The relationship between incremental costs (Cost A − Cost B) and incremental clinical benefits (Effect A − Effect B) is shown above. An ideal scenario would be to adopt a new therapy, which is both more effective than its comparator and less costly. However, few technologies in cardiac sciences are truly cost-savings. Most new therapies provide additional clinical benefits for increased costs (Figure 1). A recent example in the area of heart failure is dapagliflozin, which conferred clinical benefits (i.e., reduced risk of HF hospitalization and death from cardiovascular causes) in symptomatic heart failure (HF) patients (12, 13). In a cost-effectiveness analysis of the landmark DAPA-HF (Dapagliflozin and Prevention of Adverse Outcomes in Heart Failure) trial, patients in the dapagliflozin arm accrued an additional 0.46 QALYs compared to placebo for an additional cost of $38,212, which included $35,708 in dapagliflozin costs over a patient's lifetime horizon (14). The “time horizon” describes the timeframe over which these cost and benefits are calculated. Economic evaluation in cardiology often adopts a lifetime horizon, given the chronic nature of the disease processes, to ensure that all relevant costs and benefits are captured.
Whether a therapy is considered cost-effective depends on country-specific thresholds for value in healthcare. ICERs that fall below these willingness-to-pay thresholds are considered economically attractive. The World Health Organization has suggested an approximate benchmark of 3 times the gross domestic product per capita as an upper threshold for acceptable cost-effectiveness for a given country (15, 16). However, this definition is not routinely used by national health technology assessment agencies. In Canada and Europe, conventional thresholds range from $50,000 to $100,000 per QALY gained or £20,000–30,000, respectively (17, 18). In the United States, a value taxonomy has been proposed by the American College of Cardiology and American Heart Association where high-value represents either cost-savings or an ICER <$50,000 per QALY gained, intermediate value is represented by ICERs between $50,000 to <$150,000 per QALY gained, and low value is described by ICERs ≥$150,000 per QALY gained (19).
Economic evaluation can take several forms (Table 1). Fundamentally, all these types of economic evaluation assess how a particular intervention influences costs and benefits. Cost-effectiveness (CEA) and cost-utility (CUA) analyses are the most common types of economic evaluation, which are used to derive an ICER comparing two therapies. In CEA, benefits are commonly expressed in natural units such as life expectancy or life-years (LYs) gained. The “natural units” may also be expressed as disease-specific, clinically relevant endpoints. For example, in a CEA of an antibacterial eluting envelop to reduce post-operative infections associated with cardiac implantable electronic device implantation, the ICER was expressed as a cost per infection prevented (20). However, the use of natural units other than life expectancy limits value comparison across diseases.
A cost-utility analysis is similar to a CEA, but also incorporates quality of life into the measure of clinical benefit. CUAs facilitate comparisons across different interventions and disease states by using a common standard of clinical benefits (i.e., QALYs). Other forms of economic evaluation include cost-minimization analysis, which considers the least costly alternative and assumes that the benefits/outcomes of two therapies are identical, and cost-benefit analysis, where both the costs and benefits of a therapy are expressed in monetary terms (21).
Catheter ablation is an established therapy for patients with symptomatic atrial fibrillation. It has been shown to reduce AF recurrences, provide durable improvements in quality of life compared to medical therapy alone, and attenuate progression of the natural history of AF (22–25). Furthermore, among patients with impaired left ventricular function, catheter ablation improves survival and reduces HF hospitalization (26).
However, in patients where a rhythm control strategy is clinically appropriate, the upfront costs of catheter ablation are noticeably greater than an antiarrhythmic medication approach, due to the human and material costs of the procedure, as well as the outpatient diagnostic testing for follow-up and peri-operative imaging that may be required. The cost of catheter ablation procedure varies depending on the country and regional context; the average cost per patient is estimated to be approximately $27,000–$38,000 (2021 USD) in the United States (27, 28), $15,000 in Canada (2021 CAD) (29–31), and £7,000 (2021 GBP) (32). In comparison, medical therapy is estimated to cost less than a quarter of overall ablation costs annually (30).
While these upfront costs are substantive, cost-effectiveness analyses have attempted to provide a more comprehensive assessment of costs relative to clinical benefits projected over a longer follow up duration. That is, after ablation there may be cost offsets from the reduction in symptomatic AF burden and potentially averted HF hospitalizations. Further, there may be fewer drug complications after discontinuation of long-term antiarrhythmic medications (33). These favourable outcomes may contribute to a sustained improvement in quality of life for people with AF.
Early cost effectiveness analyses using modelling-based approaches showed that catheter ablation of AF was economically attractive compared to drug therapy alone (Table 2). For example, a Canadian analysis demonstrated an ICER of $59,194 per QALY gained for catheter ablation compared to amiodarone using a 5-year time horizon in a population of symptomatic patients on first-line anti-arrhythmic drug therapy (35). A British analysis in a similar population also demonstrated an ICER of £7,763 per QALY gained (34). However, early analyses were limited due to modelling assumptions that catheter ablation reduced stroke risk, based on the prevailing assumption at the time where rhythm control conferred reduced stroke risk (39). Additionally, these analyses did not have the benefit of longer-term clinical effectiveness data reporting hard cardiovascular outcomes.
More recently, the CABANA (Catheter ABlation vs. ANtiarrhythmic Drug Therapy for Atrial Fibrillation) study, the largest clinical trial on catheter ablation for AF vs. pharmacotherapy, did not find a difference in the primary composite endpoint of death, stroke, serious bleeding and cardiac arrest hazard ratio (HR) 0.86 [95% confidence interval (CI) 0.65–1.15] (22). However, the trial demonstrated improved quality of life and less AF recurrence with catheter ablation.
In the economic substudy of CABANA conducted from the U.S. healthcare perspective, catheter ablation was associated with an ICER of $57,893 per QALY gained compared to drug therapy alone, which falls within conventional U.S. societal thresholds for good value in health care. Generally speaking, cost-utility analyses summarize clinical benefits as a QALY. Thus, the clinical benefits that may drive cost-effectiveness are either survival, quality of life or both in some combination. Since CABANA did not demonstrate improved survival among individuals with AF randomized to catheter ablation in the intention-to-treat analysis, cost-effectiveness was contingent solely on quality-of-life gains. Note, that without quality-of-life adjustments, the ICER was $183,318 per LY gained (28).
However, emerging evidence suggests that certain subgroups with AF may also derive mortality benefit from catheter ablation compared to medical therapy in addition to increased quality of life. In these subgroups, one would anticipate an even more attractive value proposition as cost-effectiveness would be motivated by increases in life expectancy as well as quality of life. For example, in the CASTLE AF (Catheter Ablation for Atrial Fibrillation with Heart Failure) trial, catheter ablation prevented all-cause mortality relative to pharmacotherapy with a HR of 0.53 (95% CI: 0.32–0.86) (26) in patients with HF and reduced ejection fraction (EF ≤35%). In the HF subgroup of CABANA, which included 778 patients, demonstrated a 43% reduction in mortality in addition to quality-of-life improvements and freedom from AF recurrence. Of note, most patients in this analysis had heart failure with preserved ejection fraction (EF >50%) (40).
Accordingly, several cost-effectiveness analyses have noted catheter ablation to be economically attractive across a several country settings and in patients with either HF and reduced or preserved ejection fraction. For individuals with concomitant AF with HF reduced ejection fraction, the estimated ICERs were $35,360 per QALY gained in Canada (30), $35,020 per LY in Australia (37) and $38,496 per QALY gained in the United States (38). Among those with HF and preserved ejection fraction, there also appears to be an economic benefit conferred by catheter ablation of AF ($54,135 per QALY in the United States) (28).
In summary, catheter ablation for AF appears to be good “value” for money by improving quality of life and in some subsets, such as heart failure, offering mortality benefit in addition to quality-of-life gains. However, these benefits are accrued at increased costs over a patient's lifetime; despite studies suggesting reduced health resource use post-ablation, catheter ablation is not cost-savings overall (41, 42). Given an aging population and the rising prevalence of AF, increasing demand for catheter ablation highlights another important consideration in policy decision making—affordability. This concept is distinct from the economic concept of efficiency, or value for money, which is the focus of cost-effectiveness analyses. Nonetheless, the landscape of catheter ablation continues to evolve. Future work will be required to understand the economics of newer catheter technology, such as pulse field/electroporation and cryoballoon therapy (43), or providing catheter ablation of selected patient subgroups such as those early in the AF disease course, as suggested by EAST-AFNET-6 (Early Treatment of Atrial Fibrillation for Stroke Prevention Trial) and ablation trials such as EARLY AF (Early Aggressive Invasive Intervention for Atrial Fibrillation) and PROGRESSIVE AF (Impact of First-Line Rhythm Therapy on AF Progression) (25, 44, 45).
Anticoagulation is the mainstay for stroke and thromboembolism prevention in atrial fibrillation. Historically, despite the advantages over placebo, aspirin monotherapy, and combination antiplatelet therapy, clinical practice rates of warfarin among eligible patients remained suboptimal at below 60% (46, 47). Possible barriers to warfarin use in clinical practice include its narrow therapeutic window and requirement for ongoing dose adjustment and monitoring. The approval of direct oral anticoagulants (DOACs) over the past decade has offered a safe effective alternative, which represents a significant evolution in stroke prevention therapy (48, 49).
Since the cost of warfarin was much less expensive at the time of DOAC approval, DOACs required compelling additional clinical benefits to be considered cost-effective by conventional benchmarks for good value in health care. Indeed, an individual patient-level meta-analysis of the landmark DOAC trials showed that standard-dose DOACs, compared to warfarin, were associated with a significantly lower hazard of stroke or systemic embolism (HR: 0.81; 95% CI: 0.74–0.89), all-cause death (HR: 0.92; 95% CI: 0.87–0.97), and intracranial bleeding (HR: 0.45; 95% CI: 0.37–0.56) (50).
Accordingly, the majority of cost-effectiveness studies comparing individual DOACs to warfarin have estimated favorable ICERs that fall below country-specific willingness-to-pay thresholds (Table 3) (51–53). For example, in the trial-based economic evaluation of the ARISTOTLE (Apixaban for Reduction in Stroke and Other Thromboembolic Events in Atrial Fibrillation) trial, comparing apixaban to warfarin, the ICER was $53,825 per QALY gained from the US healthcare perspective (58). From the Belgian healthcare payer perspective, rivaroxaban was economically attractive compared to warfarin for stroke prevention in patients with atrial fibrillation. Using cohort level data from the ROCKET AF (Rivaroxaban Once daily oral direct factor Xa inhibition Compared with vitamin K antagonism for prevention of stroke and Embolism Trial in AF) trial to inform a Markov model, the estimated ICER was €8,809 per QALY and the probability of cost-effectiveness was 87% at a threshold of €35,000 per QALY gained (54).
Table 3. Selected economic evaluations of anticoagulation for stroke prevention in atrial fibrillation.
A recurring theme in the sensitivity analyses of these economic evaluations is the price of DOAC as a determinant of the ICER. That is, the greatest variation in cost-effectiveness is due to the initial DOAC price used to estimate the ICER. For example, in the cost-effectiveness analysis of the ARISTOTLE trial comparing apixaban to warfarin, the ICER decreased from $53,825 to $26,927 per QALY gained with a 50% reduction in apixaban price (58). With the imminent arrival of generic formulations of several DOACs, the value proposition is expected to improve. Nevertheless, even at current prices, DOACs are considered the standard of care from both an economic and clinical perspective across the major cardiovascular societies in North America and Europe (60–62).
In some health jurisdictions where health technology assessment is used to guide funding decisions, such as the United Kingdom, it will be particularly important that any new anticoagulant that comes to market demonstrates improved value compared to the existing standard of care (i.e., DOACs). This may be accomplished in several ways: (a) an improved safety profile, which may translate to less decrement in quality of life from bleeding events; (b) improved clinical effectiveness with additional stroke reduction, which would improve quality of life and possibly survival; and (c) comparable or lower price than DOACs, which is unlikely due to the impacts on return on investment. Recently, an oral factor Xia inhibitor has shown promise in a phase 2 dose finding study; the PACIFIC-AF (Safety of the oral factor XIa inhibitor asundexian compared with apixaban in patients with atrial fibrillation) trial recently showed that asundexian had a two-thirds reduction in bleeding risk of apixaban in patients with AF and stroke risk based on CHA2DS2-VASc score risk (63). Work is ongoing to confirm these findings in Phase III trials.
Left atrial appendage occlusion has been proposed as a non-pharmacologic strategy for stroke prevention in AF. The majority of clinical data supporting left atrial appendage closure comes from two seminal trials, PREVAIL (Evaluation of the WATCHMAN LAA Closure Device in Patients With Atrial Fibrillation Versus Long Term Warfarin Therapy) and PROTECT-AF (WATCHMAN Left Atrial Appendage System for Embolic Protection in Patients With Atrial Fibrillation) (64, 65). In patient-level meta-analysis that pooled the five-year outcome data from both trials, there were significant reductions in hemorrhagic stroke, cardiovascular death, all-cause death and post-procedure bleeding with LAA closure compared to warfarin anticoagulation (66).
However, there are ongoing concerns regarding the effectiveness of LAA closure regarding ischemic stroke and systemic embolism prevention. That is, the rate of ischemic stroke and systemic embolism was numerically higher with LAAO compared to warfarin in the meta-analysis, albeit these results were not statistically significant (hazard ratio 1.71; p = 0.080). Additionally, the PREVAIL trial failed to demonstrate non-inferiority of their coprimary composite endpoint of stroke, systemic embolism, or cardiovascular/unexplained death (65).
Nevertheless, an advantage to health economics methods is the ability to quantify the uncertainty in estimates of projected benefit, costs, and cost-effectiveness. Using probabilistic sensitivity analysis, each model input incorporates the surrounding confidence interval. Each time the economic model is executed, a single estimated ICER is generated by sampling inputs from their respective distributions rather than using mean parameter value. The model is then repeated many times (e.g., 1,000 or 10,000 simulations) to estimate the probability that the ICER meets benchmarks for cost-effectiveness (Figure 2).
Figure 2. Incremental cost-effectiveness plane for comparing ablation with drug therapy for the entire CABANA cohort (catheter ablation vs. Antiarrhythmic Drug Therapy for Atrial Fibrillation; N = 2204; A) and the heart failure subgroup with New York Heart Association class ≥II symptoms (n = 778; B). Estimates of incremental costs and quality-adjusted life-years (QALYs) are shown (1 blue circle for each of 5,000 bootstrap samples). Quadrant I represents scenarios where ablation is more costly and less effective, Quadrant II represents scenarios where ablation is more costly and effective, Quadrant III represents scenarios where ablation is less costly and less effective, and Quadrant IV represents scenarios where ablation is less costly and more effective. The willingness-to-pay thresholds of $50,000 and $100,000 per QALY gained are represented as the slope of the green and red lines, respectively. Scenarios that fall below these willingness-to-pay-thresholds are considered economically attractive. (Reproduced from Circulation. 2022;146:535–547 with permission).
Applying probabilistic sensitivity analyses in the cost-effectiveness analysis of the pooled PREVAIL and PROTECT AF trials, Reddy and colleagues found that LAAO was economically attractive compared to warfarin in the US setting with an estimated ICER of $48,674 per QALY by year 7 post implant, and cost-savings by year 10 (67). In the probabilistic sensitivity analysis with 10,000 simulations, the probability of LAAO cost-effectiveness was 98% when compared to warfarin.
One important caveat is that majority of these LAAO cost-effectiveness studies rely on clinical effectiveness data that compare a single LAAO device, Watchman (Boston Scientific) to warfarin. There is limited generalizability to other LAAO devices, such as the Amplatzer Amulet (Abbott), due to differences in upfront device costs, complications, and long-term effectiveness. Additionally, with improved safety profile of DOACs relative to warfarin, the relative advantage of LAAOs on bleeding risk becomes less certain (68). One analysis in the Canadian context suggested that DOACs were more cost-effective than LAAO occlusion with apixaban dominating dabigatran, LAA occlusion and rivaroxaban (69). However, there is limited data on the comparative efficacy of DOACs vs. LAAO to inform the inputs of current modelling studies, limiting their application to health policy decision making.
Future cost-effectiveness studies will benefit from upcoming clinical trials comparing LAAO to DOACs including CATALYST (Clinical Trial of Atrial Fibrillation Patients Comparing Left Atrial Appendage Occlusion Therapy to Non-vitamin K Antagonist Oral Anticoagulants; ClinicalTrials.gov NCT04226547), CHAMPION-AF (WATCHMAN FLX Versus NOAC for Embolic Protection in in the Management of Patients With Non-Valvular Atrial Fibrillation; ClinicalTrials.gov NCT04394546), and Occlusion-AF (Left Atrial Appendage Occlusion Versus Novel Oral Anticoagulation for Stroke Prevention in Atrial Fibrillation; ClinicalTrials.gov NCT03642509).
Sudden cardiac death is estimated to account for up to 20% of global mortality, and prevention and treatment of sudden cardiac death remains a significant public health challenge (70). In the 1990s, ICDs emerged as a disruptive technology for prevention of sudden death. ICDs were initially used in a relatively limited setting for secondary prevention among patients who had been resuscitated from malignant ventricular arrhythmias. However, the majority of sudden cardiac deaths are sustained among patients without prior episodes of sustained VT or ventricular fibrillation (70).
The evidence for ICD use in primary prevention populations (i.e., patients at risk for sudden death, but no history of sustained ventricular arrhythmias) were largely informed by two large randomized trials, SCD-HeFT (Sudden Cardiac Death in Heart Failure Trial) and MADIT II (Multicenter Automatic Defibrillator Implantation Trial II), which showed substantial improvements in all-cause survival with the ICD therapy (71, 72). These expanded indications for ICDs posed a challenged to health policymakers due to the opportunity cost associated with offering ICD implantation in a larger pool of eligibility patients (73, 74). An estimated 100,000 patients receive an ICD annually in the United States (75), although this number is an underestimate of the eligible population; <50% of the eligible individuals actually receive an ICD (76, 77). Although the reasons are multifactorial and complex, the low ICD uptake was initially thought in part due to the high cost of ICDs and anticipated economic burden on healthcare budgets (73). In the 2000s, US acquisition prices for the device alone ranged from $22,000 to $52,000 (reported in 2022 USD) (73)—not including the human and infrastructural resources required to implant the device and follow up patients in the long-term (78).
High-quality cost-effectiveness analyses of ICDs conducted in a variety of geographic settings estimate ICERs comparable with other well-accepted therapies despite high lifetime costs associated with ICD therapy (Table 4) (79–82). A notable feature of these cost-effectiveness studies is that the majority of lifetime costs occur upfront at the time of ICD implantation, while benefits take years to accrue. The influence of delayed clinical benefit is best demonstrated in the economic sub-study of the SCD-HeFT trial. Mark et al. found that primary prevention ICD was economically attractive compared to amiodarone (ICER of $38,389 per LY gained), but this finding was dependent on survival past the five-year follow-up of the SCD-HeFT trial. Indeed, at 5 years post-implantation, the ICER for primary prevention ICD was $127,503/LY. This later fell to $88,657/LY at 8 years of follow-up (79). Current best practice guidelines for economic evaluation recommend choosing a time horizon where all relevant costs and benefits are captured (87). In the case of primary prevention ICD implantation, these benefits are expected to accrue over a patient's lifetime, in which case a lifetime horizon or follow up is appropriate.
Table 4. Selected economic evaluations of implantable cardioverter defibrillator and cardiac resynchronization therapy devices.
Following the development of CRT systems, there were similar concerns among healthcare administrators regarding increasing costs of medical technology as a proportion of healthcare spending (88). These financial concerns, such as budget limitations and differences in reimbursement, may in part explain higher CRT implantation rates in the US compared to Europe, and the variation among European countries themselves (88). Nevertheless, the use of CRT plus an ICD among patients with severe LV systolic dysfunction and evidence of dyssynchrony [i.e., left bundle branch block (LBBB)] is cost-effective beyond ICD therapy alone. That is, the addition of a coronary sinus lead provides additional mortality and morbidity benefits, as well as improved quality of life, for additional costs within conventional thresholds for healthcare value (85, 86). For example, Mealing and colleagues performed a complex decision analytic model from the perspective of the United Kingdom National Health System comparing ICDs, CRT-pacemakers and CRT-defibrillators (CRT-D) informed by pooled individual patient data from 13 randomized clinical trials (89). Among patients with EF ≤35% and LBBB, CRT-D was considered cost-effective compared to ICD therapy alone at a willingness to pay threshold of £30,000 per QALY gained. However, with more severe heart failure symptoms [i.e., New York Heart Association (NYHA) class III or IV], CRT-P was also considered cost-effective relative to either CRT-D therapy or ICD/medical therapy alone.
There are several factors that may influence the cost-effectiveness of device therapy therapy in the contemporary context. For example, the cost of an CRT ± ICD has decreased with free market competition (90) and improved technology, specifically improved battery life has reduced the frequency of generator replacements (91). However, efficiencies gained through lower incremental costs are tempered by possible attenuation of clinical benefit in among subgroups of candidate patients due to the evolution in prerequisite guideline-directed medical therapy. For example, the absolute risk, and thereby the absolute risk reduction in mortality conferred by ICD therapy, is decreased with improvements in medical therapy for left ventricular dysfunction. An analysis of randomized clinical trials enrolling patients with heart failure and reduced ejection fraction between 1995 and 2014 showed there was a 44% decline in the rate of sudden death over time (92). Furthermore, the benefit of primary prevention ICDs among patients with non-ischemic etiology has become more controversial upon publication of the DANISH (Danish Study to Assess the Efficacy of ICDs in Patients with Non-ischemic Systolic Heart Failure on Mortality) trial, which found no benefit to ICD therapy compared to standard medical therapy in patients with an EF less than 35% and no coronary disease (HR: 0.87; 95% CI: 0.68–1.12) (93). Compared to previous trials which also enrolled patients with non-ischemic cardiomyopathy (94), DANISH enrolled a patient cohort from a decade later, who were optimized on more contemporary medical therapy (i.e., higher use of mineralocorticoid antagonists) and who had a higher proportion of CRT device use (93).
In summary, the value proposition of medical devices is not static. Updated cost-effectiveness analyses are necessary to account for changing conditions that influence the value of a given therapy. In the case of an ICD, future cost-effectiveness analysis should account for decreased ICD acquisition costs, decreased costs from less frequent generator replacements with improved ICD battery technology, contemporary device programming, and the influence of the current guideline-directed medical therapies on baseline sudden cardiac death risk. Furthermore, economic studies are required to evaluate newer, more expensive ICD technology, such as subcutaneous ICDs, compared to the current standard of transvenous ICDs.
Similar to ICDs, there may be a shift in the value proposition of CRTs. The relatively recent development of physiologic pacing techniques, such as His bundle or left bundle branch area pacing, offers the potential for similar resynchronization benefits to CRTs for decreased implantation costs (95, 96). Large clinical trials are currently underway assessing the clinical effectiveness of traditional CRT to physiologic pacing, such as the Left vs. Left trial (Cardiac Resynchronization Therapy Using His/Left Bundle Pacing vs. Left Ventricular Epicardial Pacing in Patients with Heart Failure; ClinicalTrials.gov NCT05650658), which aim to enroll 2,139 participants to compare His or Left bundle branch area pacing vs. biventricular pacing in patients with heart failure due to left ventricular systolic dysfunction (EF ≤50%) and with either a wide QRS (≥130 ms) or >40% pacing optimized on guideline-directed medical therapy.
Multiple randomized clinical trials have shown that catheter ablation is an important treatment for VT particularly in the context of ischemic cardiomyopathy. A recent meta-analysis of nine trials comparing ablation to antiarrhythmic therapy in patients with structural heart disease and VT concluded that ablation reduced the risk of VT recurrence and ICD therapies, but had no effect on heart failure hospitalization, cardiovascular mortality or all-cause mortality (97).
VT catheter ablation can be a time intensive and complex procedure associated with substantial upfront costs. Unlike catheter ablation for AF, foci causing VT in the context of cardiomyopathy can be endocardial or epicardial, and in many different anatomical locations. Substrate mapping is often long and requires highly specialized operators and equipment. Thus, a focus on cost-effectiveness is of increasing importance for this procedure to assess the relative balance between upfront costs, variable effect on patient outcomes (i.e., improved quality of life, reduced ICD shocks but no difference in mortality), and downstream cost reduction from decreased medical resource use associated with recurrent VT events and ICD shocks (98).
There is very limited data on the cost-effectiveness of VT ablation in cardiomyopathy (Table 5). One cost effectiveness analysis conducted from the UK perspective compared VT catheter ablation to anti-arrhythmic therapy among patients with ischemic cardiomyopathy and an ICD (100). The study found that catheter ablation was unlike to be cost-effective with an estimated ICER of £144,150 per quality-adjusted life-year gained, over a 5-year time horizon, which falls outside the UK's willingness to pay thresholds for value in healthcare. Consistent with the available clinical trials at the time, the benefit of ablation was driven by small gains in quality of life, but not mortality. However, a limitation noted by the authors was the lack of robust trial data reporting quality of life; only three of six trials that informed the analysis infrequently measured health-related quality of life (100).
Table 5. Selected economic evaluations of catheter ablation for ventricular tachycardia in the setting of structural heart disease.
A trial-based economic evaluation was conducted alongside the VANISH (Ventricular Tachycardia Ablation vs. Escalated Antiarrhythmic Drug Therapy in Ischemic Heart Disease) trial, which is currently the largest trial comparing escalation of anti-arrhythmic therapy (with either amiodarone or mexiletine) to VT ablation in patients with ICDs and ischemic cardiomyopathy who failed initial anti-arrhythmic therapy (102). VANISH found that ablation was more effective at reducing the incidence of the primary composite endpoint of VT storm, death or ICD shock (HR: 0.72, 95% CI: 0.58–0.98), although there was no difference in all-cause death compared to escalated anti-arrhythmic therapy.
The economic analysis was notable for several reasons. In addition to prospective collection of health resource use to inform the economic analysis, VANISH also systematically collected health-related quality of life. From the perspective of the Canadian healthcare system, catheter ablation was economically attractive compared to antiarrhythmic therapy with an estimated ICER of $34,057 (2015 CAD) per QALY gained (99). However, the finding of cost-effectiveness varied based on the findings of a pre-specified subgroup analysis that demonstrated an interaction between baseline antiarrhythmic drug prior to trial enrollment (i.e., amiodarone or sotalol) and the primary clinical composite endpoint (102). That is, for patients who had VT refractory to amiodarone therapy, catheter ablation “dominated” escalated therapy by providing additional QALYs and a cost-savings of $769 (95% CI: −$27,092 to $35,330) over a 3-year time horizon. For sotalol-refractory VT, there was no difference in QALYs and increased total costs with ablation (99).
The well-conducted, trial-based economic evaluation of VANISH provides evidence for cost-effectiveness of ablation in drug-refractory VT particularly among the subgroup of patients with ischemic cardiomyopathy and amiodarone-refractory VT. However, there is limited data to extrapolate these economic findings to the broader patient population with VT. That is, the cost-effectiveness of VT ablation among patients with non-ischemic cardiomyopathy is unknown. Furthermore, in the context of recent trials demonstrating the effectiveness of earlier VT ablation (103–105), cost-effectiveness studies are still required to assess the value proposition of VT ablation in patients naïve to antiarrhythmic therapy. Finally, there is limited data regarding the value proposition of less complex VT ablation for other indications such as those without structural heart disease (i.e., outflow tract VT ablation, Belhassen VT), other cardiomyopathies such as arrhythmogenic cardiomyopathy, and bundle branch re-entry VT.
While cost-effectiveness analyses are useful tools to facilitate health policy decisions, these methods only consider costs and clinical effectiveness as key factors that inform the decision-making process. In reality, health decisions are more nuanced and influenced by additional factors (Figure 3). For example, even if adopting a new therapy is deemed cost-effective, it may not be fiscally sustainable for a given health system's finite budget due to associated opportunity costs (i.e., if a particularly large number of people are eligible for a therapy and absolute cost become exceedingly high and requiring reallocation of resources). This is especially germane to health systems which are publicly financed and those with a global budget approach to resource allocation. In addition, when considered on a global health scale, other considerations may dominate the value equation.
Figure 3. Considerations that may influence health policy decisions in addition to cost-effectiveness.
Societal values also are key aspects in the decision-making process. Immediately life-saving interventions (i.e., rule of rescue) and treatments for vulnerable groups (e.g., children) may be more influential than costs and clinical effectiveness alone, where there may be greater weight placed on these societal values over cost-effectiveness (106, 107). For example, an alternate funding model and process of appraisal was established for oncology therapies in England in 2010 with an update in 2016 (108). The purpose of a separate funding and appraisal mechanism was to provide patients more timely access to promising cancer therapies that would be potentially rejected on basis of conventional cost-effectiveness (108). Additionally, treatments should also be distributed equally throughout society with fair access for vulnerable and marginalized populations (109). As therapeutic options continue to expand for a number of diseases in the context of limited healthcare resources, funding decisions will continue to become increasingly complex and difficult. More comprehensive methodologies, such as multi-criteria decision analysis, are starting to gain traction among health technology agencies to help inform health decisions that take into account these additional considerations of affordability, equity, access, and other societal values (110, 111).
Cardiac electrophysiology encompasses many growing technologies with important clinical applications. Many of these applications will come at a cost for increased clinical benefit. Decisions about whether or not to fund these therapies within a constrained health care budget is challenging. Cost-effectiveness analyses inform health policy decisions through the exploration of the complex relationship between costs and clinical outcomes. These analyses also assess directly the clinical benefits accrued over time from the adoption of a new technology and compare those apparent benefits to the known additional costs. In this way, an estimation of an individual intervention's value is presented for the consideration of health care policymakers. The role of formal economic evaluation is increasingly important as the rate of innovation in both drug and device development outpaces available health care expenditure. Tailored adoption of novel device and drug technology on the basis of their societal value will help facilitate a fiscally sustainable health care system.
WH and DC contributed to conception and design of the study. WH wrote the first draft of the manuscript. WH, DC, AQ, GS and ER contributed to sections of the manuscript. All authors contributed to the article and approved the submitted version.
The authors declare that the research was conducted in the absence of any commercial or financial relationships that could be construed as a potential conflict of interest.
All claims expressed in this article are solely those of the authors and do not necessarily represent those of their affiliated organizations, or those of the publisher, the editors and the reviewers. Any product that may be evaluated in this article, or claim that may be made by its manufacturer, is not guaranteed or endorsed by the publisher.
1. Tjong FV, Reddy VY. Permanent leadless cardiac pacemaker therapy: a comprehensive review. Circulation. (2017) 135(15):1458–70. doi: 10.1161/CIRCULATIONAHA.116.025037
2. Zhu K, Sun Y, Lin M, Deng Y, Li L, Li G, et al. The physiologic mechanisms of paced QRS narrowing during left bundle branch pacing in right bundle branch block patients. Front Cardiovasc Med. (2022) 9:835493. doi: 10.3389/fcvm.2022.835493
3. Moss AJ, Hall WJ, Cannom DS, Klein H, Brown MW, Daubert JP, et al. Cardiac-resynchronization therapy for the prevention of heart-failure events. N Engl J Med. (2009) 361(14):1329–38. doi: 10.1056/NEJMoa0906431
4. Wazni OM, Dandamudi G, Sood N, Hoyt R, Tyler J, Durrani S, et al. Cryoballoon ablation as initial therapy for atrial fibrillation. N Engl J Med. (2021) 384(4):316–24. doi: 10.1056/NEJMoa2029554
5. National Health Expenditure Data: Historical United States: Centers for Medicare and Medicaid Services (2021). Available at: https://www.cms.gov/Research-Statistics-Data-and-Systems/Statistics-Trends-and-Reports/NationalHealthExpendData/NationalHealthAccountsHistorical (Cited October 1, 2022).
7. Palmer S, Raftery J. Economic notes: opportunity cost. Br Med J. (1999) 318(7197):1551–2. doi: 10.1136/bmj.318.7197.1551
8. Fagerlund BC, Tjelle TE, Harboe I, Giske L, Movik E, Ørjasæter IK, et al. The MicraTM transcatheter pacing system, a leadless pacemaker, in patients indi- cated for single-chamber ventricular pacemaker implantation: A single technology assessment. Oslo, Norway: Norwegian Institute of Public Health (2018) (Updated June 2018).
9. Scott RD II, Solomon SL, McGowan JE Jr. Applying economic principles to health care. Emerg Infect Dis. (2001) 7(2):282–5. doi: 10.3201/eid0702.010227
10. Mark DB, Hlatky MA. Medical economics and the assessment of value in cardiovascular medicine: part I. Circulation. (2002) 106(4):516–20. doi: 10.1161/01.CIR.0000021407.93752.7B
11. Whitehead SJ, Ali S. Health outcomes in economic evaluation: the QALY and utilities. Br Med Bull. (2010) 96:5–21. doi: 10.1093/bmb/ldq033
12. McMurray JJV, Solomon SD, Inzucchi SE, Kober L, Kosiborod MN, Martinez FA, et al. Dapagliflozin in patients with heart failure and reduced ejection fraction. N Engl J Med. (2019) 381(21):1995–2008. doi: 10.1056/NEJMoa1911303
13. Anker SD, Butler J, Filippatos G, Ferreira JP, Bocchi E, Bohm M, et al. Empagliflozin in heart failure with a preserved ejection fraction. N Engl J Med. (2021) 385(16):1451–61. doi: 10.1056/NEJMoa2107038
14. Parizo JT, Goldhaber-Fiebert JD, Salomon JA, Khush KK, Spertus JA, Heidenreich PA, et al. Cost-effectiveness of dapagliflozin for treatment of patients with heart failure with reduced ejection fraction. JAMA Cardiol. (2021) 6(8):926–35. doi: 10.1001/jamacardio.2021.1437
15. Bertram MY, Lauer JA, Stenberg K, Edejer TTT. Methods for the economic evaluation of health care interventions for priority setting in the health system: an update from WHO CHOICE. Int J Health Policy Manag. (2021) 10(11):673–7. doi: 10.34172/ijhpm.2020.244
16. Marseille E, Larson B, Kazi DS, Kahn JG, Rosen S. Thresholds for the cost-effectiveness of interventions: alternative approaches. Bull World Health Organ. (2015) 93(2):118–24. doi: 10.2471/BLT.14.138206
17. Balijepalli C, Gullapalli L, Druyts E, Yan K, Desai K, Barakat S, et al. Can standard health technology assessment approaches help guide the price of orphan drugs in Canada? A review of submissions to the Canadian agency for drugs and technologies in health common drug review. Clinicoecon Outcomes Res. (2020) 12:445–57. doi: 10.2147/CEOR.S264589
18. McCabe C, Claxton K, Culyer AJ. The NICE cost-effectiveness threshold: what it is and what that means. Pharmacoeconomics. (2008) 26(9):733–44. doi: 10.2165/00019053-200826090-00004
19. Anderson JL, Heidenreich PA, Barnett PG, Creager MA, Fonarow GC, Gibbons RJ, et al. ACC/AHA statement on cost/value methodology in clinical practice guidelines and performance measures: a report of the American college of cardiology/American heart association task force on performance measures and task force on practice guidelines. J Am Coll Cardiol. (2014) 63(21):2304–22. doi: 10.1016/j.jacc.2014.03.016
20. Rennert-May E, Raj SR, Leal J, Exner DV, Manns BJ, Chew DS. Economic evaluation of an absorbable antibiotic envelope for prevention of cardiac implantable electronic device infection. Europace. (2021) 23(5):767–74. doi: 10.1093/europace/euaa291
21. Rennert-May E, Conly J, Leal J, Smith S, Manns B. Economic evaluations and their use in infection prevention and control: a narrative review. Antimicrob Resist Infect Control. (2018) 7:31. doi: 10.1186/s13756-018-0327-z
22. Mark DB, Anstrom KJ, Sheng S, Piccini JP, Baloch KN, Monahan KH, et al. Effect of catheter ablation vs. Medical therapy on quality of life among patients with atrial fibrillation: the CABANA randomized clinical trial. JAMA. (2019) 321(13):1275–85. doi: 10.1001/jama.2019.0692
23. Imberti JF, Ding WY, Kotalczyk A, Zhang J, Boriani G, Lip G, et al. Catheter ablation as first-line treatment for paroxysmal atrial fibrillation: a systematic review and meta-analysis. Heart. (2021) 107(20):1630–6. doi: 10.1136/heartjnl-2021-319496
24. Poole JE, Bahnson TD, Monahan KH, Johnson G, Rostami H, Silverstein AP, et al. Recurrence of atrial fibrillation after catheter ablation or antiarrhythmic drug therapy in the CABANA trial. J Am Coll Cardiol. (2020) 75(25):3105–18. doi: 10.1016/j.jacc.2020.04.065
25. Andrade JG, Deyell MW, Macle L, Wells GA, Bennett M, Essebag V, et al. Progression of atrial fibrillation after cryoablation or drug therapy. N Engl J Med. (2022) 388:105–16. doi: 10.1056/NEJMoa2212540
26. Marrouche NF, Kheirkhahan M, Brachmann J. Catheter ablation for atrial fibrillation with heart failure. N Engl J Med. (2018) 379(5):492. doi: 10.1056/NEJMoa1707855
27. Perino AC, Fan J, Schmitt SK, Kaiser DW, Heidenreich PA, Narayan SM, et al. Patient and facility variation in costs of catheter ablation for atrial fibrillation. J Cardiovasc Electrophysiol. (2018) 29(8):1081–8. doi: 10.1111/jce.13655
28. Chew DS, Li Y, Cowper PA, Anstrom KJ, Piccini JP, Poole JE, et al. Cost-effectiveness of catheter ablation versus antiarrhythmic drug therapy in atrial fibrillation: the CABANA randomized clinical trial. Circulation. (2022) 146(7):535–47. doi: 10.1161/CIRCULATIONAHA.122.058575
29. Assasi N, Blackhouse G, Xie F, Gaebel K, Robertson D, Hopkins R, et al. Ablation procedures for rhythm control in patients with atrial fibrillation: clinical and cost-effectiveness analyses. CADTH Technol Overv. (2012) 2(1):e2101. https://www.ncbi.nlm.nih.gov/pmc/articles/PMC3442612/23002373
30. Lau D, Sandhu RK, Andrade JG, Ezekowitz J, So H, Klarenbach S. Cost-utility of catheter ablation for atrial fibrillation in patients with heart failure: an economic evaluation. J Am Heart Assoc. (2021) 10(14):e019599. doi: 10.1161/JAHA.120.019599
31. Khaykin Y, Morillo CA, Skanes AC, McCracken A, Humphries K, Kerr CR. Cost comparison of catheter ablation and medical therapy in atrial fibrillation. J Cardiovasc Electrophysiol. (2007) 18(9):907–13. doi: 10.1111/j.1540-8167.2007.00902.x
32. Leung LWM, Imhoff RJ, Marshall HJ, Frame D, Mallow PJ, Goldstein L, et al. Cost-effectiveness of catheter ablation versus medical therapy for the treatment of atrial fibrillation in the United Kingdom. J Cardiovasc Electrophysiol. (2022) 33(2):164–75. doi: 10.1111/jce.15317
33. Friedman DJ, Field ME, Rahman M, Goldstein L, Sha Q, Sidharth M, et al. Catheter ablation and healthcare utilization and cost among patients with paroxysmal versus persistent atrial fibrillation. Heart Rhythm O2. (2021) 2(1):28–36. doi: 10.1016/j.hroo.2020.12.017
34. McKenna C, Palmer S, Rodgers M, Chambers D, Hawkins N, Golder S, et al. Cost-effectiveness of radiofrequency catheter ablation for the treatment of atrial fibrillation in the United Kingdom. Heart. (2009) 95(7):542–9. doi: 10.1136/hrt.2008.147165
35. Blackhouse G, Assasi N, Xie F, Gaebel K, Campbell K, Healey JS, et al. Cost-effectiveness of catheter ablation for rhythm control of atrial fibrillation. Int J Vasc Med. (2013) 2013:262809. doi: 10.1155/2013/262809
36. Aronsson M, Walfridsson H, Janzon M, Walfridsson U, Nielsen JC, Hansen PS, et al. The cost-effectiveness of radiofrequency catheter ablation as first-line treatment for paroxysmal atrial fibrillation: results from a MANTRA-PAF substudy. Europace. (2015) 17(1):48–55. doi: 10.1093/europace/euu188
37. Gao L, Moodie M. Modelling the lifetime cost-effectiveness of catheter ablation for atrial fibrillation with heart failure. BMJ Open. (2019) 9(9):e031033. doi: 10.1136/bmjopen-2019-031033
38. Chew DS, Loring Z, Anand J, Fudim M, Lowenstern A, Rymer JA, et al. Economic evaluation of catheter ablation of atrial fibrillation in patients with heart failure with reduced ejection fraction. Circ Cardiovasc Qual Outcomes. (2020) 13(12):e007094. doi: 10.1161/CIRCOUTCOMES.120.007094
39. Sherman DG. Stroke prevention in atrial fibrillation: pharmacological rate versus rhythm control. Stroke. (2007) 38(2 Suppl):615–7. doi: 10.1161/01.STR.0000254719.26536.a9
40. Packer DL, Piccini JP, Monahan KH, Al-Khalidi HR, Silverstein AP, Noseworthy PA, et al. Ablation versus drug therapy for atrial fibrillation in heart failure: results from the CABANA trial. Circulation. (2021) 143(14):1377–90. doi: 10.1161/CIRCULATIONAHA.120.050991
41. Jarman JWE, Hussain W, Wong T, Markides V, March J, Goldstein L, et al. Resource use and clinical outcomes in patients with atrial fibrillation with ablation versus antiarrhythmic drug treatment. BMC Cardiovasc Disord. (2018) 18(1):211. doi: 10.1186/s12872-018-0946-6
42. Andrade JG, Macle L, Verma A, Deyell MW, Champagne J, Dubuc M, et al. Quality of life and health care utilization in the CIRCA-DOSE study. JACC Clin Electrophysiol. (2020) 6(8):935–44. doi: 10.1016/j.jacep.2020.04.017
43. Parameswaran R, Al-Kaisey AM, Kalman JM. Catheter ablation for atrial fibrillation: current indications and evolving technologies. Nat Rev Cardiol. (2021) 18(3):210–25. doi: 10.1038/s41569-020-00451-x
44. Andrade JG, Wells GA, Deyell MW, Bennett M, Essebag V, Champagne J, et al. Cryoablation or drug therapy for initial treatment of atrial fibrillation. N Engl J Med. (2021) 384(4):305–15. doi: 10.1056/NEJMoa2029980
45. Kirchhof P, Camm AJ, Goette A, Brandes A, Eckardt L, Elvan A, et al. Early rhythm-control therapy in patients with atrial fibrillation. N Engl J Med. (2020) 383(14):1305–16. doi: 10.1056/NEJMoa2019422
46. Ogilvie IM, Newton N, Welner SA, Cowell W, Lip GY. Underuse of oral anticoagulants in atrial fibrillation: a systematic review. Am J Med. (2010) 123(7):638–45.e4. doi: 10.1016/j.amjmed.2009.11.025
47. Chew DS, Au F, Xu Y, Manns BJ, Tonelli M, Wilton SB, et al. Geographic and temporal variation in the treatment and outcomes of atrial fibrillation: a population-based analysis of national quality indicators. CMAJ Open. (2022) 10(3):E702–13. doi: 10.9778/cmajo.20210246
48. Wadhera RK, Russell CE, Piazza G. Cardiology patient page. Warfarin versus novel oral anticoagulants: how to choose? Circulation. (2014) 130(22):e191–3. doi: 10.1161/CIRCULATIONAHA.114.010426
49. Mercaldi CJ, Ciarametaro M, Hahn B, Chalissery G, Reynolds MW, Sander SD, et al. Cost efficiency of anticoagulation with warfarin to prevent stroke in medicare beneficiaries with nonvalvular atrial fibrillation. Stroke. (2011) 42(1):112–8. doi: 10.1161/STROKEAHA.110.592907
50. Carnicelli AP, Hong H, Connolly SJ, Eikelboom J, Giugliano RP, Morrow DA, et al. Direct oral anticoagulants versus warfarin in patients with atrial fibrillation: patient-level network meta-analyses of randomized clinical trials with interaction testing by age and sex. Circulation. (2022) 145(4):242–55. doi: 10.1161/CIRCULATIONAHA.121.056355
51. Freeman JV, Zhu RP, Owens DK, Garber AM, Hutton DW, Go AS, et al. Cost-effectiveness of dabigatran compared with warfarin for stroke prevention in atrial fibrillation. Ann Intern Med. (2011) 154(1):1–11. doi: 10.7326/0003-4819-154-1-201101040-00289
52. Lopez-Lopez JA, Sterne JAC, Thom HHZ, Higgins JPT, Hingorani AD, Okoli GN, et al. Oral anticoagulants for prevention of stroke in atrial fibrillation: systematic review, network meta-analysis, and cost effectiveness analysis. Br Med J. (2017) 359:j5058. doi: 10.1136/bmj.j5058
53. Ferreira J, Mirco A. Systematic review of cost-effectiveness analyses of novel oral anticoagulants for stroke prevention in atrial fibrillation. Rev Port Cardiol. (2015) 34(3):179–91. doi: 10.1016/j.repc.2014.08.008
54. Kleintjens J, Li X, Simoens S, Thijs V, Goethals M, Rietzschel ER, et al. Cost-effectiveness of rivaroxaban versus warfarin for stroke prevention in atrial fibrillation in the Belgian healthcare setting. Pharmacoeconomics. (2013) 31(10):909–18. doi: 10.1007/s40273-013-0087-9
55. Canestaro WJ, Patrick AR, Avorn J, Ito K, Matlin OS, Brennan TA, et al. Cost-effectiveness of oral anticoagulants for treatment of atrial fibrillation. Circ Cardiovasc Qual Outcomes. (2013) 6(6):724–31. doi: 10.1161/CIRCOUTCOMES.113.000661
56. You JH. Novel oral anticoagulants versus warfarin therapy at various levels of anticoagulation control in atrial fibrillation–a cost-effectiveness analysis. J Gen Intern Med. (2014) 29(3):438–46. doi: 10.1007/s11606-013-2639-2
57. Shah A, Shewale A, Hayes CJ, Martin BC. Cost-effectiveness of oral anticoagulants for ischemic stroke prophylaxis among nonvalvular atrial fibrillation patients. Stroke. (2016) 47(6):1555–61. doi: 10.1161/STROKEAHA.115.012325
58. Cowper PA, Sheng S, Lopes RD, Anstrom KJ, Stafford JA, Davidson-Ray L, et al. Economic analysis of apixaban therapy for patients with atrial fibrillation from a US perspective: results from the ARISTOTLE randomized clinical trial. JAMA Cardiol. (2017) 2(5):525–34. doi: 10.1001/jamacardio.2017.0065
59. Wu Y, Zhang C, Gu ZC. Cost-effectiveness analysis of direct oral anticoagulants vs. vitamin K antagonists in the elderly with atrial fibrillation: insights from the evidence in a real-world setting. Front Cardiovasc Med. (2021) 8:675200. doi: 10.3389/fcvm.2021.675200
60. Andrade JG, Aguilar M, Atzema C, Bell A, Cairns JA, Cheung CC, et al. The 2020 Canadian cardiovascular society/Canadian heart rhythm society comprehensive guidelines for the management of atrial fibrillation. Can J Cardiol. (2020) 36(12):1847–948. doi: 10.1016/j.cjca.2020.09.001
61. Hindricks G, Potpara T, Dagres N, Arbelo E, Bax JJ, Blomstrom-Lundqvist C, et al. Corrigendum to: 2020 ESC guidelines for the diagnosis and management of atrial fibrillation developed in collaboration with the European association for cardio-thoracic surgery (EACTS): the task force for the diagnosis and management of atrial fibrillation of the European society of cardiology (ESC) developed with the special contribution of the European heart rhythm association (EHRA) of the ESC. Eur Heart J. (2021) 42(40):4194. doi: 10.1093/eurheartj/ehab648
62. January CT, Wann LS, Calkins H, Chen LY, Cigarroa JE, Cleveland JC Jr, et al. 2019 AHA/ACC/HRS focused update of the 2014 AHA/ACC/HRS guideline for the management of patients with atrial fibrillation: a report of the American college of cardiology/American heart association task force on clinical practice guidelines and the heart rhythm society. J Am Coll Cardiol. (2019) 74(1):104–32. doi: 10.1016/j.jacc.2019.01.011
63. Piccini JP, Caso V, Connolly SJ, Fox KAA, Oldgren J, Jones WS, et al. Safety of the oral factor XIa inhibitor asundexian compared with apixaban in patients with atrial fibrillation (PACIFIC-AF): a multicentre, randomised, double-blind, double-dummy, dose-finding phase 2 study. Lancet. (2022) 399(10333):1383–90. doi: 10.1016/S0140-6736(22)00456-1
64. Reddy VY, Sievert H, Halperin J, Doshi SK, Buchbinder M, Neuzil P, et al. Percutaneous left atrial appendage closure vs. warfarin for atrial fibrillation: a randomized clinical trial. Jama. (2014) 312(19):1988–98. doi: 10.1001/jama.2014.15192
65. Holmes DR J, Kar S, Price MJ, Whisenant B, Sievert H, Doshi SK, et al. Prospective randomized evaluation of the watchman left atrial appendage closure device in patients with atrial fibrillation versus long-term warfarin therapy: the PREVAIL trial. J Am Coll Cardiol. (2014) 64(1):1–12. doi: 10.1016/j.jacc.2014.04.029
66. Reddy VY, Doshi SK, Kar S, Gibson DN, Price MJ, Huber K, et al. 5-year outcomes after left atrial appendage closure: from the PREVAIL and PROTECT AF trials. J Am Coll Cardiol. (2017) 70(24):2964–75. doi: 10.1016/j.jacc.2017.10.021
67. Reddy VY, Akehurst RL, Gavaghan MB, Amorosi SL, Holmes DR Jr. Cost-effectiveness of left atrial appendage closure for stroke reduction in atrial fibrillation: analysis of pooled, 5-year, long-term data. J Am Heart Assoc. (2019) 8(13):e011577. doi: 10.1161/JAHA.118.011577
68. Chew DS, Zhou K, Pokorney SD, Matchar DB, Vemulapalli S, Allen LA, et al. Left atrial appendage occlusion versus oral anticoagulation in atrial fibrillation: a decision analysis. Ann Intern Med. (2022) 175(9):1230–9. doi: 10.7326/M21-4653
69. Micieli A, Wijeysundera HC, Qiu F, Atzema CL, Singh SM. A decision analysis of percutaneous left atrial appendage occlusion relative to novel and traditional oral anticoagulation for stroke prevention in patients with new-onset atrial fibrillation. Med Decis Making. (2016) 36(3):366–74. doi: 10.1177/0272989X15593083
70. Hayashi M, Shimizu W, Albert CM. The spectrum of epidemiology underlying sudden cardiac death. Circ Res. (2015) 116(12):1887–906. doi: 10.1161/CIRCRESAHA.116.304521
71. Bardy GH, Lee KL, Mark DB, Poole JE, Packer DL, Boineau R, et al. Amiodarone or an implantable cardioverter-defibrillator for congestive heart failure. N Engl J Med. (2005) 352(3):225–37. doi: 10.1056/NEJMoa043399
72. Moss AJ, Zareba W, Hall WJ, Klein H, Wilber DJ, Cannom DS, et al. Prophylactic implantation of a defibrillator in patients with myocardial infarction and reduced ejection fraction. N Engl J Med. (2002) 346(12):877–83. doi: 10.1056/NEJMoa013474
73. Hlatky MA, Mark DB. The high cost of implantable defibrillators. Eur Heart J. (2007) 28(4):388–91. doi: 10.1093/eurheartj/ehl311
74. Camm J, Klein H, Nisam S. The cost of implantable defibrillators: perceptions and reality. Eur Heart J. (2007) 28(4):392–7. doi: 10.1093/eurheartj/ehl166
75. Patel NJ, Edla S, Deshmukh A, Nalluri N, Patel N, Agnihotri K, et al. Gender, racial, and health insurance differences in the trend of implantable cardioverter-defibrillator (ICD) utilization: a United States experience over the last decade. Clin Cardiol. (2016) 39(2):63–71. doi: 10.1002/clc.22496
76. Narayanan K, Reinier K, Uy-Evanado A, Teodorescu C, Chugh H, Marijon E, et al. Frequency and determinants of implantable cardioverter defibrillator deployment among primary prevention candidates with subsequent sudden cardiac arrest in the community. Circulation. (2013) 128(16):1733–8. doi: 10.1161/CIRCULATIONAHA.113.002539
77. Al-Khatib SM, Hellkamp AS, Hernandez AF, Fonarow GC, Thomas KL, Al-Khalidi HR, et al. Trends in use of implantable cardioverter-defibrillator therapy among patients hospitalized for heart failure: have the previously observed sex and racial disparities changed over time? Circulation. (2012) 125(9):1094–101. doi: 10.1161/CIRCULATIONAHA.111.066605
78. O'Brien BJ, Connolly SJ, Goeree R, Blackhouse G, Willan A, Yee R, et al. Cost-effectiveness of the implantable cardioverter-defibrillator: results from the Canadian implantable defibrillator study (CIDS). Circulation. (2001) 103(10):1416–21. doi: 10.1161/01.CIR.103.10.1416
79. Mark DB, Nelson CL, Anstrom KJ, Al-Khatib SM, Tsiatis AA, Cowper PA, et al. Cost-effectiveness of defibrillator therapy or amiodarone in chronic stable heart failure: results from the sudden cardiac death in heart failure trial (SCD-HeFT). Circulation. (2006) 114(2):135–42. doi: 10.1161/CIRCULATIONAHA.105.581884
80. Sanders GD, Hlatky MA, Owens DK. Cost-effectiveness of implantable cardioverter-defibrillators. N Engl J Med. (2005) 353(14):1471–80. doi: 10.1056/NEJMsa051989
81. Zwanziger J, Hall WJ, Dick AW, Zhao H, Mushlin AI, Hahn RM, et al. The cost effectiveness of implantable cardioverter-defibrillators: results from the multicenter automatic defibrillator implantation trial (MADIT)-II. J Am Coll Cardiol. (2006) 47(11):2310–8. doi: 10.1016/j.jacc.2006.03.032
82. Smith T, Jordaens L, Theuns DA, van Dessel PF, Wilde AA, Hunink MG. The cost-effectiveness of primary prophylactic implantable defibrillator therapy in patients with ischaemic or non-ischaemic heart disease: a European analysis. Eur Heart J. (2013) 34(3):211–9. doi: 10.1093/eurheartj/ehs090
83. Larsen G, Hallstrom A, McAnulty J, Pinski S, Olarte A, Sullivan S, et al. Cost-effectiveness of the implantable cardioverter-defibrillator versus antiarrhythmic drugs in survivors of serious ventricular tachyarrhythmias: results of the antiarrhythmics versus implantable defibrillators (AVID) economic analysis substudy. Circulation. (2002) 105(17):2049–57. doi: 10.1161/01.CIR.0000015504.57641.D0
84. Feldman AM, de Lissovoy G, Bristow MR, Saxon LA, De Marco T, Kass DA, et al. Cost effectiveness of cardiac resynchronization therapy in the comparison of medical therapy, pacing, and defibrillation in heart failure (COMPANION) trial. J Am Coll Cardiol. (2005) 46(12):2311–21. doi: 10.1016/j.jacc.2005.08.033
85. Noyes K, Veazie P, Hall WJ, Zhao H, Buttaccio A, Thevenet-Morrison K, et al. Cost-effectiveness of cardiac resynchronization therapy in the MADIT-CRT trial. J Cardiovasc Electrophysiol. (2013) 24(1):66–74. doi: 10.1111/j.1540-8167.2012.02413.x
86. Woo CY, Strandberg EJ, Schmiegelow MD, Pitt AL, Hlatky MA, Owens DK, et al. Cost-effectiveness of adding cardiac resynchronization therapy to an implantable cardioverter-defibrillator among patients with mild heart failure. Ann Intern Med. (2015) 163(6):417–26. doi: 10.7326/M14-1804
87. Ramsey SD, Willke RJ, Glick H, Reed SD, Augustovski F, Jonsson B, et al. Cost-effectiveness analysis alongside clinical trials II-an ISPOR good research practices task force report. Value Health. (2015) 18(2):161–72. doi: 10.1016/j.jval.2015.02.001
88. Boriani G, Biffi M, Martignani C, Valzania C, Diemberger I, Bertini M, et al. Is cardiac resynchronization therapy cost-effective? Europace. (2009) 11(Suppl 5):v93–7. doi: 10.1093/europace/eup274
89. Mealing S, Woods B, Hawkins N, Cowie MR, Plummer CJ, Abraham WT, et al. Cost-effectiveness of implantable cardiac devices in patients with systolic heart failure. Heart. (2016) 102(21):1742–9. doi: 10.1136/heartjnl-2015-308883
90. Koski R. Medications associated with implantable cardiac devices. US Pharm. (2016) 41(2):30–4. https://www.uspharmacist.com/article/medications-associated-with-implantable-cardiac-devices
91. Thijssen J, Borleffs CJ, van Rees JB, Man S, de Bie MK, Venlet J, et al. Implantable cardioverter-defibrillator longevity under clinical circumstances: an analysis according to device type, generation, and manufacturer. Heart Rhythm. (2012) 9(4):513–9. doi: 10.1016/j.hrthm.2011.11.022
92. Shen L, Jhund PS, Petrie MC, Claggett BL, Barlera S, Cleland JGF, et al. Declining risk of sudden death in heart failure. N Engl J Med. (2017) 377(1):41–51. doi: 10.1056/NEJMoa1609758
93. Kober L, Thune JJ, Nielsen JC, Haarbo J, Videbaek L, Korup E, et al. Defibrillator implantation in patients with nonischemic systolic heart failure. N Engl J Med. (2016) 375(13):1221–30. doi: 10.1056/NEJMoa1608029
94. Al-Khatib SM, Fonarow GC, Joglar JA, Inoue LYT, Mark DB, Lee KL, et al. Primary prevention implantable cardioverter defibrillators in patients with nonischemic cardiomyopathy: a meta-analysis. JAMA Cardiol. (2017) 2(6):685–8. doi: 10.1001/jamacardio.2017.0630
95. Wang Y, Zhu H, Hou X, Wang Z, Zou F, Qian Z, et al. Randomized trial of left bundle branch vs. biventricular pacing for cardiac resynchronization therapy. J Am Coll Cardiol. (2022) 80(13):1205–16. doi: 10.1016/j.jacc.2022.07.019
96. Cheng Y, Wang Z, Li Y, Qi J, Liu J. Left bundle branch pacing in heart failure patients with left bundle branch block: a systematic review and meta-analysis. Pacing Clin Electrophysiol. (2022) 45(2):212–8. doi: 10.1111/pace.14405
97. Virk SA, Kumar S. Catheter ablation of ventricular tachycardia in patients with structural heart disease: a meta-analysis. JACC Clin Electrophysiol. (2022) 2:255–7. doi: 10.1016/j.jacep.2022.09.002
98. Al-Khatib SM, Stevenson WG, Ackerman MJ, Bryant WJ, Callans DJ, Curtis AB, et al. 2017 AHA/ACC/HRS guideline for management of patients with ventricular arrhythmias and the prevention of sudden cardiac death: executive summary: a report of the American college of cardiology/American heart association task force on clinical practice guidelines and the heart rhythm society. Heart Rhythm. (2018) 15(10):e190–252. doi: 10.1016/j.hrthm.2017.10.035
99. Coyle K, Coyle D, Nault I, Parkash R, Healey JS, Gray CJ, et al. Cost effectiveness of ventricular tachycardia ablation versus escalation of antiarrhythmic drug therapy: the VANISH trial. JACC Clin Electrophysiol. (2018) 4(5):660–8. doi: 10.1016/j.jacep.2018.01.007
100. Chen Y, Gomes M, Garcia JV, Hunter RJ, Chow AW, Dhinoja M, et al. Cost-effectiveness of ablation of ventricular tachycardia in ischaemic cardiomyopathy: limitations in the trial evidence base. Open Heart. (2020) 7(1):e001155. doi: 10.1136/openhrt-2019-001155
101. Calkins H, Bigger JT Jr, Ackerman SJ, Duff SB, Wilber D, Kerr RA, et al. Cost-effectiveness of catheter ablation in patients with ventricular tachycardia. Circulation. (2000) 101(3):280–8. doi: 10.1161/01.CIR.101.3.280
102. Sapp JL, Wells GA, Parkash R, Stevenson WG, Blier L, Sarrazin JF, et al. Ventricular tachycardia ablation versus escalation of antiarrhythmic drugs. N Engl J Med. (2016) 375(2):111–21. doi: 10.1056/NEJMoa1513614
103. Della Bella P, Baratto F, Vergara P, Bertocchi P, Santamaria M, Notarstefano P, et al. Does timing of ventricular tachycardia ablation affect prognosis in patients with an implantable cardioverter defibrillator? Results from the multicenter randomized PARTITA trial. Circulation. (2022) 145(25):1829–38. doi: 10.1161/CIRCULATIONAHA.122.059598
104. Arenal A, Avila P, Jimenez-Candil J, Tercedor L, Calvo D, Arribas F, et al. Substrate ablation vs. antiarrhythmic drug therapy for symptomatic ventricular tachycardia. J Am Coll Cardiol. (2022) 79(15):1441–53. doi: 10.1016/j.jacc.2022.01.050
105. Tung R, Xue Y, Chen M, Jiang C, Shatz DY, Besser SA, et al. First-line catheter ablation of monomorphic ventricular tachycardia in cardiomyopathy concurrent with defibrillator implantation: the PAUSE-SCD randomized trial. Circulation. (2022) 145(25):1839–49. doi: 10.1161/CIRCULATIONAHA.122.060039
106. Dolan P, Cookson R. A qualitative study of the extent to which health gain matters when choosing between groups of patients. Health Policy. (2000) 51(1):19–30. doi: 10.1016/S0168-8510(99)00079-2
107. Schwappach DL. Resource allocation, social values and the QALY: a review of the debate and empirical evidence. Health Expect. (2002) 5(3):210–22. doi: 10.1046/j.1369-6513.2002.00182.x
108. Morrell L, Wordsworth S, Schuh A, Middleton MR, Rees S, Barker RW. Will the reformed cancer drugs fund address the most common types of uncertainty? An analysis of NICE cancer drug appraisals. BMC Health Serv Res. (2018) 18(1):393. doi: 10.1186/s12913-018-3162-2
109. Chew D, Clement F. Open access budget impact assessment tools: a welcome step in supporting evidence-informed policy decisions. Can J Cardiol. (2022) 38(10):1485–7. doi: 10.1016/j.cjca.2022.07.003
110. Baran-Kooiker A, Czech M, Kooiker C. Multi-criteria decision analysis (MCDA) models in health technology assessment of orphan drugs-a systematic literature review. Next steps in methodology development? Front Public Health. (2018) 6(287). doi: 10.3389/fpubh.2018.00287
Keywords: cost effectiveness analysis (CEA), economic evaluation (cost effectiveness), cardiac electrophysiogy, atrial fibrilation (AF), ICD (implantable cardioverter-defibrillator), CRT-D, cardiac resynchronization therapy–defibrillator, anticoagulation (AC), ablation < electrophysiology
Citation: Hijazi W, Vandenberk B, Rennert-May E, Quinn A, Sumner G and Chew DS (2023) Economic evaluation in cardiac electrophysiology: Determining the value of emerging technologies. Front. Cardiovasc. Med. 10:1142429. doi: 10.3389/fcvm.2023.1142429
Received: 11 January 2023; Accepted: 3 April 2023;
Published: 26 April 2023.
Edited by:
Robert Hatala, National Institute of Cardiovascular Diseases, SlovakiaReviewed by:
Pramesh Kovoor, Westmead Hospital, Australia© 2023 Hijazi, Vandenberk, Rennert-May, Quinn, Sumner and Chew. This is an open-access article distributed under the terms of the Creative Commons Attribution License (CC BY). The use, distribution or reproduction in other forums is permitted, provided the original author(s) and the copyright owner(s) are credited and that the original publication in this journal is cited, in accordance with accepted academic practice. No use, distribution or reproduction is permitted which does not comply with these terms.
*Correspondence: Derek S. Chew ZGNoZXdAdWNhbGdhcnkuY2E=
Specialty Section: This article was submitted to Cardiac Rhythmology, a section of the journal Frontiers in Cardiovascular Medicine
Abbreviations AF, atrial fibrillation; ARISTOTLE, Apixaban for Reduction in Stroke and Other Thromboembolic Events in Atrial Fibrillation; CABANA, Catheter ABlation vs. ANtiarrhythmic Drug Therapy for Atrial Fibrillation; CASTLE AF, Catheter Ablation for Atrial Fibrillation with Heart Failure; CATALYST, Clinical Trial of Atrial Fibrillation Patients Comparing Left Atrial Appendage Occlusion Therapy to Non-vitamin K Antagonist Oral Anticoagulants; CEA, cost-effectiveness analysis; CHAMPION-AF, WATCHMAN FLX Versus NOAC for Embolic Protection in in the Management of Patients With Non-Valvular Atrial Fibrillation; CI, confidence interval; CUA, cost-utility analysis; CRT, cardiac resynchronization therapy; DANISH, Danish Study to Assess the Efficacy of ICDs in Patients with Non-ischemic Systolic Heart Failure on Mortality; DOAC, direct oral anticoagulant; EARLY AF, Early Aggressive Invasive Intervention for Atrial Fibrillation; EAST-AFNET-6, Early Treatment of Atrial Fibrillation for Stroke Prevention Trial; EF, ejection fraction; GDP, gross domestic product; HF, heart failure; HR, hazard ratio; ICD, implantable cardioverter defibrillators; ICER, incremental cost effectiveness ratio; LAAO, left atrial appendage occlusion; LBBB, left bundle branch block; LY, life year; MADIT II, Multicenter Automatic Defibrillator Implantation Trial II; NYHA, New York Heart Association; Occlusion-AF, Left Atrial Appendage Occlusion Versus Novel Oral Anticoagulation for Stroke Prevention in Atrial Fibrillation; PACIFIC-AF, Safety of the oral factor XIa inhibitor asundexian compared with apixaban in patients with atrial fibrillation; PREVAIL, Evaluation of the WATCHMAN LAA Closure Device in Patients With Atrial Fibrillation Versus Long Term Warfarin Therapy; PROGRESSIVE AF, Impact of “First-Line” Rhythm Therapy on AF Progression; PROTECT-AF, WATCHMAN Left Atrial Appendage System for Embolic Protection in Patients With Atrial Fibrillation; QALY, quality-adjusted life year; ROCKET AF, Rivaroxaban Once daily oral direct factor Xa inhibition Compared with vitamin K antagonism for prevention of stroke and Embolism Trial in AF; SCD-HeFT, Sudden Cardiac Death in Heart Failure Trial; VANISH, Ventricular Tachycardia Ablation vs. Escalated Antiarrhythmic Drug Therapy in Ischemic Heart Disease; VT, ventricular tachycardia.
Disclaimer: All claims expressed in this article are solely those of the authors and do not necessarily represent those of their affiliated organizations, or those of the publisher, the editors and the reviewers. Any product that may be evaluated in this article or claim that may be made by its manufacturer is not guaranteed or endorsed by the publisher.
Research integrity at Frontiers
Learn more about the work of our research integrity team to safeguard the quality of each article we publish.