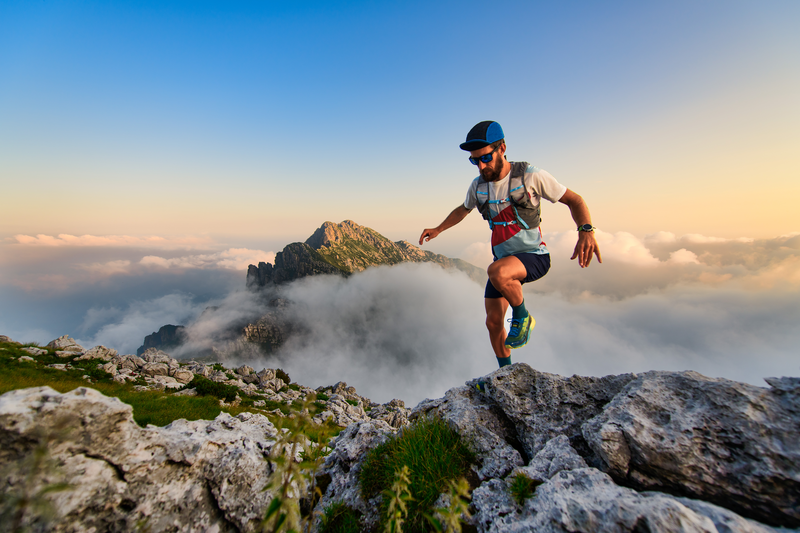
94% of researchers rate our articles as excellent or good
Learn more about the work of our research integrity team to safeguard the quality of each article we publish.
Find out more
OPINION article
Front. Cardiovasc. Med. , 18 April 2023
Sec. Heart Failure and Transplantation
Volume 10 - 2023 | https://doi.org/10.3389/fcvm.2023.1120487
This article is part of the Research Topic Insights in Heart Failure and Transplantation: 2022 View all 13 articles
Despite the best available therapies, heart failure with reduced ejection fraction (HFrEF) remains one of the leading causes of morbidity and mortality worldwide (1). Dilated cardiomyopathy (DCM) is one of the leading cause of HFrEF (2). DCM is characterized by progressive heart enlargement with a rEF that is caused by genetic, ischemic, and other disorders (3). Neurohumoral imbalances of the sympathetic nervous system, renin-angiotensin-aldosterone systems (RAAS) and the natriuretic peptide system, are associated with maladaptive cardiac remodeling in HFrEF (4–8). Corin, a cardiac type II transmembrane protease, activates pro-atrial natriuretic peptide (pro-ANP) to biologically active ANP by proteolytic cleavage during pro-ANP secretion from cardiomyocytes (9–12). Through production of biologically active ANP, corin appears to slow the progression of DCM to HFrEF and death, which makes it an attractive therapeutic target in HF management (13–23). Reduced levels of circulating and cardiac corin in patients with symptomatic HFrEF were reported in numerous studies (14, 15, 24–31). The biologically active corin-ANP axis blocked the development of systolic/diastolic dysfunction, low cardiac output, pulmonary and/or systemic fluid retention (edema), dyspnea and elevated blood HF biomarkers (ANP and B-type natriuretic peptide, BNP) (15–18, 23, 30, 31). Pre-clinical studies revealed that the biologically active corin-ANP axis also reduces the development of chronic adverse fibrotic ventricular remodeling (cardiac fibrosis, diffuse accumulation of collagen I/III fibers) (17, 19, 20, 22). Although the protective role of pro-fibrotic angiotensin II (Ang II)-AT1 axis blockage in reverse remodeling in HFrEF is widely accepted, the therapeutic potential of the corin-ANP axis in preventing fibrosis, are less appreciated. Herein, we present and discuss pre-clinical and clinical evidence supporting the targeted restoration of biological activity of the corin-ANP axis as a valuable anti-fibrotic therapeutic strategy in DCM-HFrEF.
Under physiological conditions, corin is expressed by atrial and ventricular cardiomyocytes on the external membrane surface as a zymogen and proteolytically active enzyme (9, 10, 17, 21). In atrial cardiomyocytes, corin is co-expressed with its biological substrate pro-ANP- (10, 32). Upon secretion, pro-ANP is proteolytically cleaved by corin and released into circulation as biologically active ANP (11, 12). Circulating biologically active ANP acts locally in the heart and remotely in the kidneys and vasculature by preferentially stimulating the transmembrane natriuretic peptide-A receptor, which generates the intracellular cyclic guanosine monophosphate (cGMP) and stimulates protein kinase G-driven signaling pathways (33, 34). Remotely, the ANP-cGMP axis triggers natriuresis and vasodilation and inhibits renal renin secretion; this decreases cardiac volume overload, aldosterone synthesis and Ang II production in the circulation (11, 35–37). In the heart, the ANP-cGMP pathway counters hypertrophy and fibrosis through autocrine/paracrine regulatory mechanisms leading to inhibition of fibroblast-mediated collagen synthesis (33, 38, 39). Specifically, by stimulation of cGMP production and protein kinase G activation, biologically active ANP may transmit extracellular signals and modulate downstream effector molecules into the same cardiomyocytes it was secreted from (an autocrine mechanism) or on neighboring cardiac myocyte and fibroblast cells (a paracrine mechanism) (33, 39).
Dysregulation of ANP-cGMP axis by blunted corin has been shown to contribute to systolic dysfunction, maladaptive cardiac remodeling and edema, leading to HFrEF development (15–19, 21, 22, 30, 40, 41). In DCM, the balance between cardiac anti-fibrotic/pro-fibrotic processes are under control of hemodynamic and humoral modulators such as corin-ANP-cGMP axis and the RAAS. The dysregulation of this balance, its pathological shift and contribution to HFrEF development in DCM are schematically illustrated in Figure 1 and described below.
Figure 1. Schematic representation of the pathological shift of the cardiac corin-atrial natriuretic peptide (ANP) axis and classical RAAS [renin-angiotensin II (Ang II)—aldosterone] axis in dilated cardiomyopathy, which promotes cardiac fibrosis, edema and accelerates HFrEF progression.
In DCM at pre-HF stage, RAAS plays an adaptive protective role compensating for impaired cardiac function and structural changes by stimulating sodium-water retention by the kidney and increasing arterial vasoconstriction. However, prolonged, persistent RAAS activation stimulates DCM progression (4–6, 8, 40, 42–44). In DCM at pre-HF stage, the corin-ANP-cGMP axis, when biologically functional, counters the outcomes of the pathologically activated systemic and cardiac classical RAAS by maintaining cardio-renal homeostasis promoting diuresis, natriuresis, and vasodilation and anti-fibrotic action (4, 6, 40, 45, 46). However, as DCM progress in human and mice, cardiac corin expression and activity are reduced leading to impairment of biological activity of the corin-ANP-cGMP axis (21, 30). Declines in corin levels indicate systolic dysfunction as it happened even before the increases in plasma ANP and BNP levels and the onset of edema (21, 23, 26, 30), which is a major hallmark of HF and a key driver of symptoms (3, 47). Consequently, as the natriuretic peptide system is impaired and becomes insufficient to properly balance RAAS activity, pathologically active RAAS further promotes cardiac dilation, fibrotic ventricular remodeling, salt-water retention (edema), and HFrEF development in humans and pre-clinical models (4, 6, 40, 42, 44, 46, 48, 49). Although HFrEF (stages C-D) is associated with a boost of pro-ANP expression by the ventricle's cardiomyocytes (21, 37), pro-ANP cleavage and production of biologically active ANP are compromised as the level of corin is significantly reduced (21).
As DCM progresses to HFrEF (stages C-D), renin is over-secreted by the kidneys into circulation. It triggers Ang II activation pathways (systemic and locally within the heart), cardiac Ang II-independent signaling and stimulates aldosterone secretion from the adrenal glands, which fosters fibrotic remodeling (6, 40, 43, 49, 50). Systemic (circulating) Ang II and aldosterone play an important role in cardiac fibrosis development, as increased local production of Ang II in the heart is not enough to induce ventricular hypertrophy or fibrosis (51).
Converging evidence from human and pre-clinical mouse studies indicate that, as DCM progresses to HF Stages C and D, the protective action of the corin-ANP-cGMP axis is impaired as the coordinated relationship between cardiac pro-ANP expression and enzymes responsible for pro-ANP activation (corin) and ANP degradation (neprilysin) become imbalanced. In particular, levels of the ANP degrading enzyme neprilysin begin to rise (30, 34) while levels of ANP activating enzyme corin fall (14, 15–17, 19, 21– 23, 25, 28, 30, 31). Consequently, the blunted ANP homeostasis contributes to the relative cGMP deficiency in HFrEF.
HFrEF is characterized by elevated pro-ANP expression, which is due to increased expression by the atria and reprogramming of cardiac left ventricular gene expression with induction of pro-ANP. However, levels of cardiac and circulating corin significantly decline in patients and preclinical models with DCM and HFrEF (15, 17, 19, 21, 25, 28, 30, 31, 41). In patients with HFrEF, decreases in circulating corin lead to impaired cleavage/activation of pro-ANP and dysregulated relationships between pro-ANP, ANP and cGMP levels (15, 30). At the same time, neprilysin levels progressively increase with severity of clinical HF assessed by Framingham criteria and are negatively correlated with corin levels (23, 30). In a pre-clinical DCM-HFrEF model, restoration of suppressed cardiac corin was associated with normalization of circulating neprilysin and suppression of renin activity and aldosterone in circulation (41). Low plasma corin was associated with poor HF-related clinical outcomes: lower NYHA functional status (increased functional class), increased cardiovascular mortality and major adverse cardiac events. Depressed cardiac and plasma corin reflects the progression of systolic dysfunction (severity of cardiomyopathy), left ventricular remodeling and fibrosis; it promoted the development of symptomatic HFrEF (17, 21, 30).
In experimental DCM, ANP was a critical protective modulator of aldosterone-Ang II-induced interstitial/perivascular fibrosis in the left atrium and ventricle (38). ANP also protected against systolic dysfunction, symptomatic HF, and survival in mice with normal renal function (38). Cardiac pro-ANP deficiency in mice with DCM was associated with significant reduction of cGMP levels in circulation. In these mice, cardiac pro-ANP deficiency was not compensated by cardiac expression of pro-BNP, but was associated with a decline in cardiac transcripts for pro-C-type NP (38), a potent anti-fibrotic modulator that inhibits cardiac fibroblast proliferation and collagen synthesis (34, 38). Consistent with these findings, the survival benefits of neprilysin inhibitors within ARNI therapy (combined Ang II receptor, AT1 and neprilysin inhibitors sacubitril/valsartan) have been attributed in part to its effect on blunting cardiac ventricular remodeling and fibrosis (a risk factor for sudden cardiac death), by preserving biologically active levels of ANP. Thus, ANP circulating levels were elevated after treatment with ARNI therapy, the difference in BNP levels was inconsistent, NT-pro-BNP levels decreased and CNP levels were not affected by treatment (34, 52–54). Increases in ANP plasma levels in patients with ARNI therapy for chronic HFrEF were associated with increased urinary cGMP levels (55). Another study demonstrated that in patients with acute decompensated HFrEF, ARNI therapy was associated with higher urinary cGMP levels (56). However, in both these studies (55, 56), corin levels were not analyzed.
Similar to ANP, genetic restoration of both proteolytically active or inactive cardiac corin in mice with DCM improved systolic function, delayed symptomatic HFrEF progression and prolonged survival (17, 18, 41). However, only proteolytically active (ANP-cleaving) cardiac corin has protective anti-fibrotic action (17, 41). Cardiac restoration of proteolytically active corin led to a significant reduction in cardiac collagen I/III transcripts and a trend towards reduction of TGFβ transcripts, and overall suppression of interstitial and perivascular ventricular fibrosis (17). Restoration of cardiac corin significantly increased pro-ANP cleavage to ANP and cGMP production, both of which are potent inhibitors of cardiac fibroblast proliferation and collagen synthesis (17). Cardiac-specific overexpression of proteolytically active corin reduced myocardial infarct size 24 h post-experimental myocardial infarction (MI) induced by left coronary artery ligation in mice. Corin overexpression prevented these mice from development of severe systolic dysfunction, cardiac remodeling and edema 4 weeks post-MI (57). However, this study did not assess the impact of cardiac corin overexpression on the pro-ANP-cGMP axis and cardiac fibrosis. In mouse HF models induced by left coronary artery ligation and transverse aortic constriction, intraperitoneal injection of a recombinant extracellular fragment of human corin with an engineered activation site lowered Ang II and aldosterone plasma levels, boosted cGMP levels, improved cardiac function and attenuated cardiac remodeling and fibrosis (22). The analysis of pro-ANP metabolism in the plasma of patients with stable chronic HFrEF, indicated that ARNI therapy increased pro-ANP cleavage, which was linked to an increase in corin activity (58).
Considering the above knowledge, we hypothesize that enhancing cardiac corin expression by ARNI therapy might contribute to improved cardiac remodeling in HFrEF. Thus, ARNI therapy could provide beneficial antifibrotic outcomes by suppressing the profibrotic action of angiotensin II and boosting antifibrotic ANP activity. Increased ANP activity may be achieved not only through reduced degradation of biologically active ANP by neprilysin, but also through a feedback mechanism of improved systolic function stimulating cardiac corin expression, which in turn improves pro-ANP cleavage and increases biologically active ANP levels. It is worth testing the hypothesis that in HFrEF patients, ARNI therapy is associated with increased corin levels in circulation and cardiac left ventricle and reduced impairment of pro-ANP cleavage, which contribute to reverse cardiac remodeling.
Available experimental and clinical evidence suggests that in DCM, dysregulation of the biological effects of ANP, at least in part by insufficient corin expression and/or activity, promotes cardiac fibrosis associated with relative cGMP deficiency and contributes to the progression of systolic dysfunction and symptomatic HFrEF. These insights may suggest a new therapeutic paradigm to prevent DCM from becoming a relentless, progressive and fatal form of HFrEF. Preserving or boosting the biological activity of the corin-ANP-cGMP axis by corin targeted interventions may offer potential therapeutic strategies for preventing or blocking progressive cardiac fibrosis in DCM-HFrEF.
IPG: contributed to the conceptualization, writing original draft, illustration and editing. RDS: contributed to editing and Figure generation. GLR: contributed to the conceptualization, editing and illustration of the manuscript. All authors contributed to the article and approved the submitted version.
This study was in part supported by the National Institutes of Health grants HL115036 (IPG) and NS089707 (GLR).
The Scheme presented as a Figure 1 was created using BioRender.com.
The authors declare that the research was conducted in the absence of any commercial or financial relationships that could be construed as a potential conflict of interest.
All claims expressed in this article are solely those of the authors and do not necessarily represent those of their affiliated organizations, or those of the publisher, the editors and the reviewers. Any product that may be evaluated in this article, or claim that may be made by its manufacturer, is not guaranteed or endorsed by the publisher.
1. Savarese G, Becher PM, Lund LH, Seferovic P, Rosano GMC, Coats A. Global burden of heart failure: a comprehensive and updated review of epidemiology. Cardiovasc Res. (2022) 118(17):3272–87. doi: 10.1093/cvr/cvac013
2. Rosenbaum AN, Agre KE, Pereira NL. Genetics of dilated cardiomyopathy: practical implications for heart failure management. Nat Rev Cardiol. (2020) 17(5):286–97. doi: 10.1038/s41569-019-0284-0
3. Bozkurt B, Hershberger RE, Butler J, Grady KL, Heidenreich PA, Isler ML, et al. 2021 ACC/AHA key data elements and definitions for heart failure: a report of the American college of cardiology/American heart association task force on clinical data standards (writing committee to develop clinical data standards for heart failure). J Am Coll Cardiol. (2021) 77(16):2053–150. doi: 10.1016/j.jacc.2020.11.012
4. Schrier RW, Abraham WT. Hormones and hemodynamics in heart failure. N Engl J Med. (1999) 341(8):577–85. doi: 10.1056/NEJM199908193410806
5. Hartupee J, Mann DL. Neurohormonal activation in heart failure with reduced ejection fraction. Nat Rev Cardiol. (2017) 14(1):30–8. doi: 10.1038/nrcardio.2016.163
6. Sullivan RD, Mehta RM, Tripathi R, Reed GL, Gladysheva IP. Renin activity in heart failure with reduced systolic function-new insights. Int J Mol Sci. (2019) 20(13):3182. doi: 10.3390/ijms20133182
7. Aimo A, Gaggin HK, Barison A, Emdin M, Januzzi JL Jr. Imaging, biomarker, and clinical predictors of cardiac remodeling in heart failure with reduced ejection fraction. JACC Heart Fail. (2019) 7(9):782–94. doi: 10.1016/j.jchf.2019.06.004
8. Mann DL, Felker GM. Mechanisms and models in heart failure: a translational approach. Circ Res. (2021) 128(10):1435–50. doi: 10.1161/CIRCRESAHA.121.318158
9. Hooper JD, Scarman AL, Clarke BE, Normyle JF, Antalis TM. Localization of the mosaic transmembrane serine protease corin to heart myocytes. Eur J Biochem. (2000) 267(23):6931–7. doi: 10.1046/j.1432-1033.2000.01806.x
10. Gladysheva IP, Robinson BR, Houng AK, Kovats T, King SM. Corin is co-expressed with pro-ANP and localized on the cardiomyocyte surface in both zymogen and catalytically active forms. J Mol Cell Cardiol. (2008) 44(1):131–42. doi: 10.1016/j.yjmcc.2007.10.002
11. Armaly Z, Assady S, Abassi Z. Corin: a new player in the regulation of salt-water balance and blood pressure. Curr Opin Nephrol Hypertens. (2013) 22(6):713–22. doi: 10.1097/01.mnh.0000435609.35789.32
12. Zhang X, Gu X, Zhang Y, Dong N, Wu Q. Corin: a key mediator in sodium homeostasis, vascular remodeling, and heart failure. Biology. (2022) 11(5):717. doi: 10.3390/biology11050717
13. Langenickel TH, Pagel I, Buttgereit J, Tenner K, Lindner M, Dietz R, et al. Rat corin gene: molecular cloning and reduced expression in experimental heart failure. Am J Physiol Heart Circ Physiol. (2004) 287(4):H1516–21. doi: 10.1152/ajpheart.00947.2003
14. Ibebuogu UN, Gladysheva IP, Reed GL. Is heart failure due to impaired cleavage and activation of atrial natriuretic peptide? J Am Coll Cardiol. (2009) 53:A467–A8. doi: 10.1016/j.jacc.2009.01.024
15. Ibebuogu UN, Gladysheva IP, Houng AK, Reed GL. Decompensated heart failure is associated with reduced corin levels and decreased cleavage of pro-atrial natriuretic peptide. Circ Heart Fail. (2011) 4(2):114–20. doi: 10.1161/CIRCHEARTFAILURE.109.895581
16. Dries DL. Process matters: emerging concepts underlying impaired natriuretic peptide system function in heart failure. Circ Heart Fail. (2011) 4(2):107–10. doi: 10.1161/CIRCHEARTFAILURE.111.960948
17. Gladysheva IP, Wang D, McNamee RA, Houng AK, Mohamad AA, Fan TM, et al. Corin overexpression improves cardiac function, heart failure, and survival in mice with dilated cardiomyopathy. Hypertension. (2013) 61(2):327–32. doi: 10.1161/HYPERTENSIONAHA.112.193631
18. Ngo DT, Horowitz JD, Sverdlov AL. Heart failure: a corin-deficient state? Hypertension. (2013) 61(2):284–5. doi: 10.1161/HYPERTENSIONAHA.112.196253
19. Ichiki T, Boerrigter G, Huntley BK, Sangaralingham SJ, McKie PM, Harty GJ, et al. Differential expression of the pro-natriuretic peptide convertases corin and furin in experimental heart failure and atrial fibrosis. Am J Physiol Regul Integr Comp Physiol. (2013) 304(2):R102–9. doi: 10.1152/ajpregu.00233.2012
20. Pang A, Hu Y, Zhou P, Long G, Tian X, Men L, et al. Corin is down-regulated and exerts cardioprotective action via activating pro-atrial natriuretic peptide pathway in diabetic cardiomyopathy. Cardiovasc Diabetol. (2015) 14:134. doi: 10.1186/s12933-015-0298-9
21. Tripathi R, Wang D, Sullivan R, Fan TH, Gladysheva IP, Reed GL. Depressed corin levels indicate early systolic dysfunction before increases of atrial natriuretic peptide/B-type natriuretic peptide and heart failure development. Hypertension. (2016) 67(2):362–7. doi: 10.1161/HYPERTENSIONAHA.115.06300
22. Niu Y, Zhang S, Gu X, Zhou T, Li F, Liu M, et al. Recombinant soluble corin improves cardiac function in mouse models of heart failure. J Am Heart Assoc. (2021) 10(7):e019961. doi: 10.1161/JAHA.120.019961
23. Gladysheva IP, Sullivan RD, Reed GL. Neprilysin and corin in HF: does combining 2 biomarkers double our insights? JACC Heart Fail. (2021) 9(5):406. doi: 10.1016/j.jchf.2021.01.016
24. Barth AS, Kuner R, Buness A, Ruschhaupt M, Merk S, Zwermann L, et al. Identification of a common gene expression signature in dilated cardiomyopathy across independent microarray studies. J Am Coll Cardiol. (2006) 48(8):1610–7. doi: 10.1016/j.jacc.2006.07.026
25. Dong N, Chen S, Yang J, He L, Liu P, Zheng D, et al. Plasma soluble corin in patients with heart failure. Circ Heart Fail. (2010) 3(2):207–11. doi: 10.1161/CIRCHEARTFAILURE.109.903849
26. Ward RD, Zaidi SS, Ramanathan K, Yu X, Gladysheva IP, Reed GL. Corin levels are linked to systolic function and Serum sodium. J Card Fail. (2013) 19(8):S12. doi: 10.1016/j.cardfail.2013.06.037
27. Barnet CS, Liu X, Body SC, Collard CD, Shernan SK, Muehlschlegel JD, et al. Plasma corin decreases after coronary artery bypass graft surgery and is associated with postoperative heart failure: a pilot study. J Cardiothorac Vasc Anesth. (2015) 29(2):374–81. doi: 10.1053/j.jvca.2014.11.001
28. Zhou X, Chen JC, Liu Y, Yang H, Du K, Kong Y, et al. Plasma corin as a predictor of cardiovascular events in patients with chronic heart failure. JACC Heart Fail. (2016) 4(8):664–9. doi: 10.1016/j.jchf.2016.03.006
29. Yu Z, Lu X, Xu W, Jin M, Tao Y, Zhou X. Serum corin is associated with the risk of chronic heart failure. Oncotarget. (2017) 8(59):100353–7. doi: 10.18632/oncotarget.22227
30. Zaidi SS, Ward RD, Ramanathan K, Yu X, Gladysheva IP, Reed GL. Possible enzymatic downregulation of the natriuretic peptide system in patients with reduced systolic function and heart failure: a pilot study. Biomed Res Int. (2018) 2018:7279036. doi: 10.1155/2018/7279036
31. Verstreken S, Delrue L, Goethals M, Bartunek J, Vanderheyden M. Natriuretic peptide processing in patients with and without left ventricular dysfunction. Int Heart J. (2019) 60(1):115–20. doi: 10.1536/ihj.18-012
32. Gladysheva IP, King SM, Houng AK. N-glycosylation modulates the cell-surface expression and catalytic activity of corin. Biochem Biophys Res Commun. (2008) 373(1):130–5. doi: 10.1016/j.bbrc.2008.05.181
33. Kong Q, Blanton RM. Protein kinase G I and heart failure: shifting focus from vascular unloading to direct myocardial antiremodeling effects. Circ Heart Fail. (2013) 6(6):1268–83. doi: 10.1161/CIRCHEARTFAILURE.113.000575
34. Goetze JP, Bruneau BG, Ramos HR, Ogawa T, de Bold MK, de Bold AJ. Cardiac natriuretic peptides. Nat Rev Cardiol. (2020) 17(11):698–717. doi: 10.1038/s41569-020-0381-0
35. Burnett JC Jr., Granger JP, Opgenorth TJ. Effects of synthetic atrial natriuretic factor on renal function and renin release. Am J Physiol. (1984) 247(5 Pt 2):F863–6. doi: 10.1152/ajprenal.1984.247.5.F863
36. Johnston CI, Hodsman PG, Kohzuki M, Casley DJ, Fabris B, Phillips PA. Interaction between atrial natriuretic peptide and the renin angiotensin aldosterone system. Endogenous antagonists. Am J Med. (1989) 87(6B):24S–8S. doi: 10.1016/0002-9343(89)90087-9
37. Volpe M, Carnovali M, Mastromarino V. The natriuretic peptides system in the pathophysiology of heart failure: from molecular basis to treatment. Clin Sci. (2016) 130(2):57–77. doi: 10.1042/CS20150469
38. Wang D, Gladysheva IP, Fan TH, Sullivan R, Houng AK, Reed GL. Atrial natriuretic peptide affects cardiac remodeling, function, heart failure, and survival in a mouse model of dilated cardiomyopathy. Hypertension. (2014) 63(3):514–9. doi: 10.1161/HYPERTENSIONAHA.113.02164
39. Blanton RM. cGMP signaling and modulation in heart failure. J Cardiovasc Pharmacol. (2020) 75(5):385–98. doi: 10.1097/FJC.0000000000000749
40. Tripathi R, Sullivan R, Fan TM, Wang D, Sun Y, Reed GL, et al. Enhanced heart failure, mortality and renin activation in female mice with experimental dilated cardiomyopathy. PLoS One. (2017) 12(12):e0189315. doi: 10.1371/journal.pone.0189315
41. Tripathi R, Sullivan RD, Fan TM, Houng AK, Mehta RM, Reed GL, et al. Cardiac-specific overexpression of catalytically inactive corin reduces edema, contractile dysfunction, and death in mice with dilated cardiomyopathy. Int J Mol Sci. (2020) 21(1):203. doi: 10.3390/ijms21010203
42. Dzau VJ, Colucci WS, Hollenberg NK, Williams GH. Relation of the renin-angiotensin-aldosterone system to clinical state in congestive heart failure. Circulation. (1981) 63(3):645–51. doi: 10.1161/01.CIR.63.3.645
43. Weber KT. Aldosterone in congestive heart failure. N Engl J Med. (2001) 345(23):1689–97. doi: 10.1056/NEJMra000050
44. Sullivan RD, Mehta RM, Tripathi R, Gladysheva IP, Reed GL. Normalizing plasma renin activity in experimental dilated cardiomyopathy: effects on edema, cachexia, and survival. Int J Mol Sci. (2019) 20(16):3886. doi: 10.3390/ijms20163886
45. Lopez B, Ravassa S, Moreno MU, Jose GS, Beaumont J, Gonzalez A, et al. Diffuse myocardial fibrosis: mechanisms, diagnosis and therapeutic approaches. Nat Rev Cardiol. (2021) 18(7):479–98. doi: 10.1038/s41569-020-00504-1
46. Abassi Z, Khoury EE, Karram T, Aronson D. Edema formation in congestive heart failure and the underlying mechanisms. Front Cardiovasc Med. (2022) 9:933215. doi: 10.3389/fcvm.2022.933215
47. Gladysheva IP, Sullivan RD, Pellicori P. Editorial: edema in heart failure with reduced ejection fraction. Front Cardiovasc Med. (2023) 10:1141937. doi: 10.3389/fcvm.2023.1141937
48. Tripathi R, Sullivan RD, Fan TM, Mehta RM, Gladysheva IP, Reed GL. A low-sodium diet boosts ang (1–7) production and NO-cGMP bioavailability to reduce edema and enhance survival in experimental heart failure. Int J Mol Sci. (2021) 22(8):4035. doi: 10.3390/ijms22084035
49. Gladysheva IP, Sullivan RD, Ramanathan K, Reed GL. Soluble (Pro)Renin receptor levels are regulated by plasma renin activity and correlated with edema in mice and humans with HFrEF. Biomedicines. (2022) 10(8):1874. doi: 10.3390/biomedicines10081874
50. Buffolo F, Tetti M, Mulatero P, Monticone S. Aldosterone as a mediator of cardiovascular damage. Hypertension. (2022) 79(9):1899–911. doi: 10.1161/HYPERTENSIONAHA.122.17964
51. Xiao HD, Fuchs S, Campbell DJ, Lewis W, Dudley SC Jr., Kasi VS, et al. Mice with cardiac-restricted angiotensin-converting enzyme (ACE) have atrial enlargement, cardiac arrhythmia, and sudden death. Am J Pathol. (2004) 165(3):1019–32. doi: 10.1016/S0002-9440(10)63363-9
52. Ibrahim NE, McCarthy CP, Shrestha S, Gaggin HK, Mukai R, Szymonifka J, et al. Effect of neprilysin inhibition on Various natriuretic peptide assays. J Am Coll Cardiol. (2019) 73(11):1273–84. doi: 10.1016/j.jacc.2018.12.063
53. Abboud A, Januzzi JL. Reverse cardiac remodeling and ARNI therapy. Curr Heart Fail Rep. (2021) 18(2):71–83. doi: 10.1007/s11897-021-00501-6
54. Murphy SP, Prescott MF, Maisel AS, Butler J, Pina IL, Felker GM, et al. Association between angiotensin receptor-neprilysin inhibition, cardiovascular biomarkers, and cardiac remodeling in heart failure with reduced ejection fraction. Circ Heart Fail. (2021) 14(6):e008410. doi: 10.1161/CIRCHEARTFAILURE.120.008410
55. Murphy SP, Prescott MF, Camacho A, Iyer SR, Maisel AS, Felker GM, et al. Atrial natriuretic peptide and treatment with sacubitril/valsartan in heart failure with reduced ejection fraction. JACC Heart Fail. (2021) 9(2):127–36. doi: 10.1016/j.jchf.2020.09.013
56. Morrow DA, Velazquez EJ, DeVore AD, Prescott MF, Duffy CI, Gurmu Y, et al. Cardiovascular biomarkers in patients with acute decompensated heart failure randomized to sacubitril-valsartan or enalapril in the PIONEER-HF trial. Eur Heart J. (2019) 40(40):3345–52. doi: 10.1093/eurheartj/ehz240
57. Sullivan RD, Houng AK, Gladysheva IP, Fan TM, Tripathi R, Reed GL, et al. Corin overexpression reduces myocardial infarct size and modulates cardiomyocyte apoptotic cell death. Int J Mol Sci. (2020) 21(10):3456. doi: 10.3390/ijms21103456
Keywords: corin, atrial natriuretic peptide, fibrosis, dilated cardiomyopathy, edema
Citation: Gladysheva IP, Sullivan RD and Reed GL (2023) Falling corin and ANP activity levels accelerate development of heart failure and cardiac fibrosis. Front. Cardiovasc. Med. 10:1120487. doi: 10.3389/fcvm.2023.1120487
Received: 10 December 2022; Accepted: 3 April 2023;
Published: 18 April 2023.
Edited by:
Matteo Cameli, University of Siena, ItalyReviewed by:
Nicolò Ghionzoli, University of Siena, Italy© 2023 Gladysheva, Sullivan and Reed. This is an open-access article distributed under the terms of the Creative Commons Attribution License (CC BY). The use, distribution or reproduction in other forums is permitted, provided the original author(s) and the copyright owner(s) are credited and that the original publication in this journal is cited, in accordance with accepted academic practice. No use, distribution or reproduction is permitted which does not comply with these terms.
*Correspondence: Inna P. Gladysheva aW5uYWdsYWR5c2hldmFAYXJpem9uYS5lZHU= Guy L. Reed Z3V5cmVlZEBhcml6b25hLmVkdQ==
Disclaimer: All claims expressed in this article are solely those of the authors and do not necessarily represent those of their affiliated organizations, or those of the publisher, the editors and the reviewers. Any product that may be evaluated in this article or claim that may be made by its manufacturer is not guaranteed or endorsed by the publisher.
Research integrity at Frontiers
Learn more about the work of our research integrity team to safeguard the quality of each article we publish.