- 1Laboratory of Vascular Pathology, IIS-Fundación Jiménez Díaz, Madrid, Spain
- 2Biomedicine Department, Alfonso X El Sabio University, Madrid, Spain
- 3CIBERCV, Madrid, Spain
- 4Medicine Department, Autonoma University of Madrid, Madrid, Spain
Aortic aneurysms, including abdominal aortic aneurysms (AAAs), is the second most prevalent aortic disease and represents an important cause of death worldwide. AAA is a permanent dilation of the aorta on its infrarenal portion, pathologically associated with oxidative stress, proteolysis, vascular smooth muscle cell loss, immune-inflammation, and extracellular matrix remodeling and degradation. Most epidemiological studies have shown a potential protective role of diabetes mellitus (DM) on the prevalence and incidence of AAA. The effect of DM on AAA might be explained mainly by two factors: hyperglycemia [or other DM-related factors such as insulin resistance (IR)] and/or by the effect of prescribed DM drugs, which may have a direct or indirect effect on the formation and progression of AAAs. However, recent studies further support that the protective role of DM in AAA may be attributable to antidiabetic therapies (i.e.: metformin or SGLT-2 inhibitors). This review summarizes current literature on the relationship between DM and the incidence, progression, and rupture of AAAs, and discusses the potential cellular and molecular pathways that may be involved in its vascular effects. Besides, we provide a summary of current antidiabetic therapies which use could be beneficial for AAA.
1. AAA and DM
Aortic aneurysms, including abdominal aortic aneurysms (AAA), is the second most prevalent aortic disease following atherosclerosis, affecting approximately 1%–2% of all 65-year-old men and therefore, representing an important cause of death worldwide (1). AAA is a local dilatation of the abdominal aorta larger than 3 cm or exceeding by 50 per cent the normal aortic diameter (2) and is pathologically associated with oxidative stress, proteolysis, extracellular matrix (ECM) remodeling and degradation, vascular smooth muscle cell (VSMC) loss and immune-inflammatory responses (3, 4) (Figure 1). A significant change in the management of patients with AAA has occurred during the last 15–20 years, which has improved mortality rates after surgery treatment (5). In addition, screening programs and changes in sociodemographic and social trends, is helping reducing rupture incidence and mortality rates (1, 6–8). AAAs are often asymptomatic until rupture and incidentally detected by ultrasound, x-rays, or CT scans when patients are examined for a non-related reason. Several risk factors for AAA have been identified including aging, male sex, hypertension, dyslipidemia, and smoking (9). Prior studies have failed to identify specific treatments for AAA and randomized clinical trials have supported surgery (open or endovascular surgery) only in patients with large (aortic diameter > 5–5.5 cm) or symptomatic AAAs (2, 10).
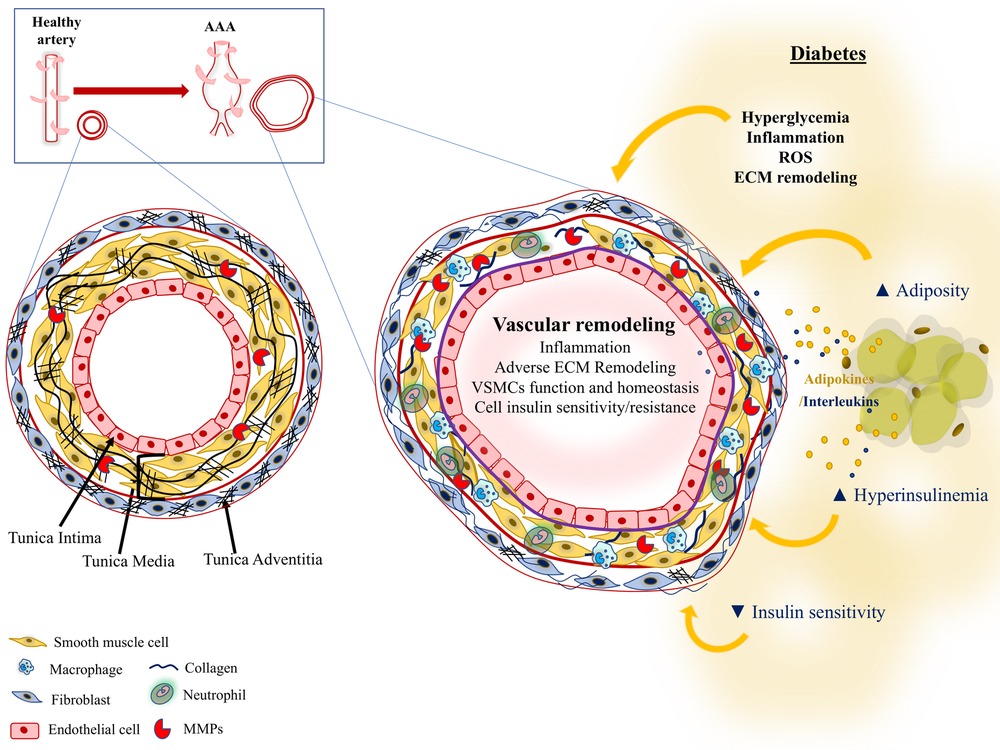
Figure 1. Pathological processes in type 1 and/or type 2 diabetes potentially affecting AAA progression.
Diabetes Mellitus (DM), a disease which is characterized by chronic hyperglycemia, induces nephropathy and microvascular disease, and represents a major risk factor for cardiovascular disease (CVD) (80 percent of deaths among those with DM) (11, 12). Type 1 DM (T1DM) and Type 2 DM (T2DM) have abnormally elevated blood glucose levels as main characteristic of the disease but differ in many other aspects. While T1DM is primarily the result of autoimmune destruction of pancreatic beta cells, T2DM is characterized by the loss of insulin sensitivity, deficiency in insulin secretion and is frequently linked to obesity (13). Although DM is one of the main risk factors for CVD, most epidemiological studies have described its potential protective role on the prevalence and incidence of AAA (14–18).
For non-post-operative data, most studies suggest the protective role of DM in AAA (14). Meta-analysis of prospective studies demonstrate that people with DM have lower prevalence of AAA (19–22) and that people with DM and small AAAs (aortic diameter between 3 and 5 cm) have slower AAA progression (22–24). The VIVA trial showed an inverse relationship between HbA1c blood levels and AAA growth rate (24). A recent study in 250 subjects showed that patients with DM have more than a 35 percent reduction in the median AAA growth rates despite having more severe concomitant vascular comorbidities (25). A retrospective study on the relationship between DM and AAA rupture showed that patients with DM and AAA are significantly less likely to present AAA rupture or to die from AAA rupture when compared to nondiabetic patients with AAA (26). However, the authors of this study suggested that the protective effect on AAA could be due to DM itself or to the pharmacological treatments of DM. According to these results, a meta-analysis of 9 studies of AAA rupture and 2 studies of non-rupture symptomatic AAA demonstrated that DM was associated with lower prevalence of AAA rupture (18). Although DM and/or antidiabetic drugs seems to exert a protective effect on the vascular wall, their effect on the post-operative cardiovascular outcomes after open surgery in patients undergoing AAA repair is more controversial. In this sense, a recent nationwide study in France identified T1DM as a risk factor of post-operative mortality in patients undergoing AAA repair (27). Some reports show increased post-operative mortality in patients with DM compared to controls (22, 28). However, some others report lower mortality (19). The adjusted analysis in a Swedish nationwide observational study showed that patients with DM have significantly lower risk of total and cardiovascular mortality after acute aortic repair, whereas rates of cardiovascular events, acute myocardial infarction and stroke did not differ between groups (29). There are also some studies which show no differences in post-operative mortality between patients with and without DM (17, 30–32). One of those studies showed that patients with DM had higher rates of acute myocardial infarction and major adverse cardiovascular events after elective open AAA repair than those without DM, despite not finding differences in post-operative mortality (31).
The research on the protective role of DM in AAA has been mainly tested on animal models. To our knowledge, these studies have been mainly performed in models of T1DM (15). Streptozotocin-induced T1DM shows a protective effect in AAA induced by either by elastase infusion in the abdominal aorta of C57BL/6 mice or by Angiotensin II (Ang II) infusion in apolipoprotein E knock-out (ApoE−/−) mice (33, 34). Dr. Zhonglin Chai's group demonstrated that cell division autoantigen 1 (CDA1) is upregulated in DM and enhances TGF-β signaling, including the vasculature (35). In this study, the authors demonstrated that deletion of CDA1 in mice with DM decreased TGF-β signaling and reduced ECM accumulation, which would revert the protective effect of DM and then, contribute to aneurysm formation (36, 37). Importantly, stimulation of TGF-β signaling prevents AAA (38, 39) and blockade of TGF-β accelerates AAA development (39, 40). Thus, the protective effect of CDA1 could be explained by its effect on TGF-β signaling. A recent study in mice has suggested the potential contribution of dysregulated prolyl hydroxylase domain (PHD) containing proteins to DM mediated AAA suppression (41). The authors proposed that AAA attenuation in the setting of DM was derived from enhanced aneurysmal angiogenesis as a consequence of dysregulated PHD activity. On the other hand, mice models of T2DM present hyperglycemia and insulin resistance (IR), often associated with obesity (42), which fairly recapitulates human disease. However, to our knowledge, although Tanaka et al. studied the effect of T2DM on carotid aneurysm (by using KK-Ay mice), no mouse experiments have been performed to study the effect of T2DM on AAA, which implies a lack of information on the potential mechanisms by which DM would exert protective effect on AAA.
In spite of the commented clinical and experimental evidence suggesting a protective role of DM in AAA, a Mendelian randomized analysis recently demonstrated that lifelong genetic predisposition to T2DM does not protect against AAA (43). Moreover, human diabetic arteries have intrinsic properties potentially related to AAA development, such as increased stiffness (44), endothelial dysfunction (45, 46) and calcifications (47). In this respect, DM due to the combination of hyperglycemia (T1 and T2DM) and obesity and IR (T2DM) could lead to pathological vascular remodeling through mechanisms including oxidative stress, inflammation and ECM degradation (Figure 1). In the next sections, we will show the discrepancy between some studies showing a protective role of DM in AAA with others supporting the potential contribution of DM-related factors to pathological mechanisms involved in AAA development.
1.1. Hyperglycemia and AAA
Hyperglycemia, a common risk factor for T1DM and T2DM, drives inflammation and reactive oxygen species (ROS) production, impairing endothelial cell and VSMC function (48–52). In addition, hyperglycemia accelerates senescence in endothelial cells (52, 53), VSMCs (54, 55), endothelial progenitor cells (56) and mesenchymal stem cells (57), which contributes to vascular dysfunction. Hyperglycemia induces changes in VSMC responses to vascular injury (55), which have been described to be mediated, at least in part, by β3 integrin signaling (55) and by suppression of insulin receptor substrate-1-mediated p53/KLF4 complex stabilization (58). DM and chronic hyperglycemia drive advanced glycation end products (AGE) production, activates glucose autooxidation and inhibits the production of antioxidant agents, all together influencing the activation of circulating and resident cells of the vascular wall. Thus, diabetes would be expected to be a risk factor for the development of AAA. However, hyperglycemia has been shown to stabilize the collagen network by generating a thicker aortic wall which exert a protective effect on the wall stress in the abdominal aorta of patients with DM (23, 59). In this regard, accumulation of collagen IV is commonly observed in human diabetic arteries (60, 61), while its deficiency is associated with AAA development (62). Moreover, circulating collagen IV degradation fragments correlated with AAA progression in the VIVA cohort (62). Furthermore, Golledge et al. suggested that the progression of AAA is slower in patients with diabetes through changes in monocyte-ECM interactions (23). Moreover, an study in AAA biopsies obtained from diabetic and nondiabetic patients suggested that cross-linking AGEs play a protective role in AAA progression in diabetic patients (63). Besides, Miyama et al. showed that hyperglycemia reduces progression of AAA disease in two models of elastase and Ang II infusion in mice (34). This study demonstrated that insulin treatment attenuates this protective effect. Identifying the mechanisms by which hyperglycemia exert a protective role against AAA formation and progression would contribute to the development of novel clinical therapies for AAA disease.
1.2. Obesity and AAA
Observational studies, interventional studies and post-hot analysis of clinical trials demonstrate that T2DM long-term glucose fluctuation is correlated with an increased risk of micro- and macro-vascular complications (64–71). However, it is conceivable that long-term variability of other DM-related risk factors (insulin levels, blood pressure, dyslipidemia, heart rate, body weight, and serum uric acid) may be involved in the development of AAA.
Obesity is a major health problem worldwide and is a major risk factor of the pathophysiology of T2DM and weight gain, promotes diabetes progression and is associated with worse glycemic control. Obesity is a risk factor for human AAA (72–74). The Physician's Health Study showed that, relative to men with body mass index (BMI) < 25, overweight (BMI = 25–30) and obese (BMI ≥ 30) men had 30%–70% higher risk of developing AAA (75). The same prospective analysis in the Physicians' Health Study showed that despite obesity was associated with a higher risk of AAA, the history of DM tended to associate with a lower risk of diagnosed AAA, particularly over longer follow-up. Increased adipose tissue mass has been shown to promote the two main defects of diabetes: IR and beta-cell dysfunction. A study on a Swedish population found that the risk of AAA was 30% higher in individuals with increased waist circumference (abdominal adiposity) (76). Moreover, patients with metabolic syndrome have a more than two fold increased risk for the development of T2DM, cardiovascular morbidity and mortality (77, 78). A recent study in 354 patients concluded that metabolic syndrome proportionally aggravates the progression of AAA (79). Interestingly, although the rupture rate was significantly higher and the survival rate significantly lower in patients with metabolic syndrome, the size of AAA was significantly smaller in patients with DM compared to patients with no DM (despite being metabolic syndrome more prevalent in the DM group) (79).
Regional differences in periaortic adipocytes and their differential ability to promote chemokine release, macrophage infiltration, and proinflammatory cytokine expression was related to enhanced AAA risk in obesity. The presence of adipocytes in the vascular wall is associated with AAA development and/or rupture (74). One of the main features in AAA pathogenesis is inflammation, which is often associated with the excess of PVAT. The amount of PVAT is higher in the aortas of patients with AAA (80). However, the molecular influence of PVAT on AAA has not been well described. Adipose tissue depots produce proinflammatory adipokines (leptin, TNFα and resistin) which have been suggested to have a role on AAA development. On the other hand, adiponectin, an adipokine which is positively involved in glucose and lipid systemic homeostasis, is decreased in the context of obesity (81). Interestingly, experimental AAA models have shown that adiponectin infusion reduces vascular inflammation, prevents vascular infiltration of macrophage and attenuates AAA development (82, 83). A recent systematic review and meta-analysis of animal and human observational studies, concluded that, although leptin and adiponectin upregulation do not affect AAA in animal models, studies in humans showed that resistin and leptin serum levels together with the amount of PVAT were higher in patients with AAA compared to control (84).
Besides autocrine and paracrine effect of fat tissue, adipocyte metabolism and molecular signaling might influence AAA development. It has been shown that genetic deletion of Ang II type 1a receptor in PVAT reduces AAA formation in ApoE−/− mice (85). In 3T3-L1 adipocytes, Ang II inhibits insulin-stimulated IR and IRS-S tyrosine-phosphorylation, Akt activation, and glucose uptake (86). However, not only adipocytes but also other types of cells within the adipose tissue have been suggested to have a role on the progression of AAA. In this sense, macrophages within the adipose tissue are key in the inflammatory process of AAA due to their role on the degradation of the ECM (72). Diet-induced obesity as well as the leptin-deficient (ob/ob) genetic mouse model of obesity promotes macrophage infiltration in PVAT surrounding abdominal aortas and further increases Ang II-induced AAAs (72).
Although obesity is known to be one of the main risk factor of T2DM, the inverse correlation of DM with AAA could suggest a protective role of obesity against AAA. However, as mentioned before, adiposity has been implicated in other pathogenic mechanisms of relevance to AAA, suggesting that the relationship between obesity and AAA may be complex. Specific studies on the effect of DM with or without DM are needed to elucidate the contribution of both diseases on the pathophysiology of AAA.
1.3. Insulin resistance and AAA
Multiple studies have highlighted the association between DM and AAA. However, no conclusive studies have been done to elucidate the link between IR, one of the most important characteristics of T2DM, and the incidence and progression of AAA.
Systemic IR can cause vascular dysfunction and accelerate vascular disease in people with DM and metabolic syndrome (87–89). A clinical study shows an association of AAA diameter with IR (90). In this study, C-peptide, insulin concentrations and IR index (HOMA2 IR) were significantly higher in patients with AAA > 5 cm compared to those with AAA < 5 cm. IR might promote incidence and AAA progression by systemic factors (dyslipidemia, hyperglycemia, inflammation) as well as by the disruption of insulin signaling in endothelial cells, VSMCs and/or macrophages. Interestingly, insulin receptor-mediated signaling pathways in these cells are down-regulated in hyperinsulinemic environment (88, 91). In this sense, altered insulin signaling might be due to down regulation of insulin receptor signaling or by the excessive insulin receptor signaling (87). Studies in endothelial cells suggest that downregulation of insulin receptor may contribute to vascular remodeling in an insulin resistant environment (91, 92). A recent study focused on macrophages as potential responsible of the potential protective effect of DM on AAA showed that stimulation of macrophages with serum from patients with DM and AAA increase macrophage metabolism (based on differences in extracellular acidification and the expression of genes involved in glycolysis and lipid oxidation), which is accompanied by a shift towards an anti-inflammatory state (93). IR is accompanied by hyperinsulinemia and literature suggests that insulin modulates the inflammatory response in macrophages. In this regard, most of the studies show that insulin enhances inflammatory response and secretion of inflammatory cytokines (94, 95). However, there are also studies showing no effect on cytokine production by the macrophages in response to insulin, or even demonstrate the potential anti-inflammatory effect of insulin (96–98). Although there are several published studies on the mechanism of IR vascular remodeling, it remains unexplored whether endothelial, VSMC and macrophage IR affects the incidence and progression of AAA.
2. Oral antidiabetic drugs and AAA
Treatment of patients with DM includes lifestyle modifications (mainly healthy diet and exercise), together with a proper pharmacologic therapy (99–101) (Table 1). Drugs used to treat patients with T2DM have a variety of mechanisms by which they lower glucose levels (102), with different molecular targets and potential uses for other diseases. Recent available literature shows protective effects of some antidiabetic drugs on the incidence and progression of AAA (Figure 2).
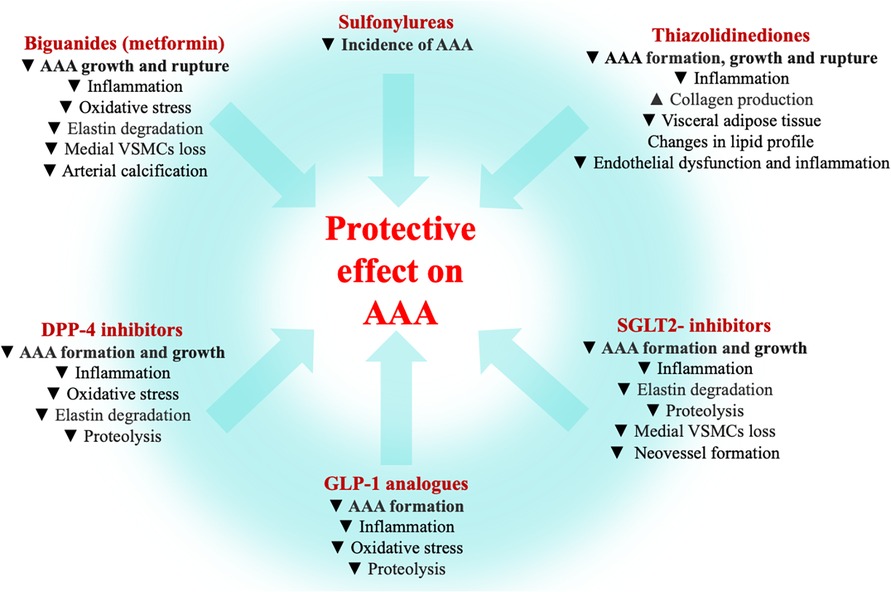
Figure 2. Antidiabetic agents and AAA. Summary of the mechanisms by which biguanides (103–124), sulfonylureas (131), thiazolidinediones (125–130), dipeptidyl peptidase inhibitors (DDP-4) inhibitors (149, 151–155, 158), glucagon-like peptide-1 (GLP-1) analogues (145–149, 156–158) and type-2 sodium-glucose co-transporter (SGLT2)-inhibitors (133–144) exert a protective effect on AAA (data from experimental and clinical studies).
2.1. Metformin and AAA
Studies on metformin treatment suggest that this drug may reduce CVD independently on its effect on improving glucose control (103–107). One of the mechanisms that have been proposed for this protective effect of metformin is through its action via phosphorylation of AMP-activated protein kinase (AMPK) (108), which affects several glucose-activated lipogenesis genes and GLUT4 transporter. Experimental models have shown that metformin is effective in limiting AAA progression in both DM and normoglycemic conditions. Two experimental studies showed a protective effect of metformin on AAA formation through a decrease in proinflammatory cells in the vascular wall and a reduction in proinflammatory cytokines (109, 110). A recent study in an experimental AAA model in mice showed that metformin reduced autophagy in AAA through Atg7, suggesting this molecule as a potential mediator of the protective effect of metformin in AAA (107). Besides, vascular calcification is associated with T2DM and increases the risk of cardiovascular morbidity and mortality. Pharmacological administration of metformin alleviate arterial calcification through AMPK-activated autophagy (111). In fact, it has been previously suggested that the epidemiologic evidence of the protective role of DM in AAA may be attributable to antidiabetic therapy with metformin (110). Metformin suppresses experimental AAAs and reduces enlargement rate of clinical AAAs (110, 112–115). The findings of association between metformin prescription and AAA growth rates showed that treatment with metformin in T2DM patients was associated with a reduced AAA growth in three different cohorts of approximately 1,700 patients that were analyzed by different imaging protocols (116). A systemic review and meta-analysis of 10 studies showed that patients with T2DM that were treated with metformin had slower annual AAA growth rate compared with T2DM patients without metformin. In a nationwide analysis of diabetic Veterans Affairs patients, prescription for metformin was associated with decreased AAA enlargement (112). The mechanism by which metformin may exert a protective effect on AAA may be related to its anti-inflammatory and antioxidant effect on the vasculature (109, 110, 114, 117–119) and/or to its effect on the ECM remodeling (120). Metformin treatment also reduced the frequency of rupture of AAA (121). Therefore, the translational potential use for metformin therapy is beyond the treatment of DM. Interestingly, Golledge et al. recently started a clinical trial [Metformin Aneurysm Trial (MAT)] to elucidate whether metformin reduces the risk of serious complications of AAA (122). The primary outcome of the MAT trial is the proportion of AAA events: rupture-related mortality or need for surgical repair. The secondary outcomes include AAA growth, major adverse cardiovascular events, and health-related quality of life. Another recent clinical trial (The Metformin for Abdominal Aneurysm Growth Inhibition [MAAAGI] trial started on February 2020, and had to be paused during the COVID-19 pandemia (123). In this trial, primary efficacy will be assessed by difference in AAA diameter determined by computed tomography after five years vs. at baseline. Secondary outcomes will be AAA volume and ultrasound diameter growth, rupture and elective repair AAA events, quality of life, and health economic assessment. The Limiting AAA with Metformin trial (LIMIT) started on February 2022 (124). The primary outcome will be the change in maximal orthogonal diameter of the infrarenal aorta after two years vs. at baseline and secondary outcomes will include profile of adverse cardiovascular events, all-cause mortality, change in serological markers of hepatic, hematopoietic and renal function within others. Thus, there will be very valuable data about metformin effect on AAA within the next years.
2.2. Thiazolidinediones and AAA
Several studies with pioglitazone have suggested a beneficial effect on CVD. These antidiabetic agents exhibit anti-inflammatory effects by reducing the levels of TNF-α (125, 126). Jones et al. demonstrated in an experimental model of Ang II-induced AAA that rosiglitazone treatment reduce inflammation and increase the aortic wall thickness by increasing collagen production. Besides, administration of rosiglitazone in mice inhibited Ang-II-mediated activation of JNK, thereby reducing the formation of aneurysms (127). It has been shown that pioglitazone decreases visceral adipose tissue, reduces cholesterol and triglycerides blood levels, increases HDL cholesterol, reduces hyperinsulinemia and IR, improves endothelial dysfunction and inflammation, risk factors for AAA development and progression (128). Thus, pioglitazone may have direct or indirect effect on AAA disease. A model of Ang II-induced AAAs in ApoE-deficient mice showed that suprarenal aortic expansion was significantly reduced by the treatment with pioglitazone compared to the control group (129). A study with rosiglitazone, another peroxisome proliferator-activated receptor-gamma agonist, showed that pretreatment or posttreatment with rosiglitazone reduced aortic expansion and rupture in the same animal model of AAA (130).
2.3. α-Glucosidase inhibitors and AAA
Few studies with α-glucosidase inhibitors have demonstrated protective cardiovascular effects of these hypoglycemic drugs. A nested case–control analysis using the database extracted from Taiwan's national health insurance research database showed that alpha-glucosidase inhibitors was not associated with aneurysm events (131). In this study, incidence of AAA was lower in those receiving metformin, sulfonylurea, and TZD, but not dipeptidil peptidase-4 (DPP4) inhibitors and alpha-glucosidase inhibitors. Further studies on acarbose effects on AAA disease in patients with and without DM are needed.
2.4. SLGT2 inhibitors and AAA
SGLT2 is a glucose transporter located in the proximal tubules of the kidneys, which is responsible for the reabsorption of the majority of the filtered glucose entering the tubules (132). Clinical and experimental data suggest that SGLT-2 inhibitors have beneficial effects in cardiovascular and metabolic diseases such as nonalcoholic steatohepatitis (133, 134), obesity (135–137), heart failure (138), and atherosclerosis; however, little is known about the effect of SGLT-2 inhibition on AAA. A recent study evaluates the effect of oral chronic treatment with empagliflozin, an SGLT-2 inhibitor, on AAA induced by Ang II infusion in mice (141). This study shows that empaglifozin treatment reduced the suprarenal aortic diameter independently of blood pressure effects. Besides, empagliflozin diminished elastin degradation, neovessel formation, macrophage infiltration and expression of CCL-2 [chemokine (C-C motif) ligand 2] and CCL-5 [chemokine (C-C motif) ligand 5], VEGF (vascular endothelial growth factor), MMP-2 and MMP-9 at the AAA lesion. In vitro studies with empaglifozin shows that this inhibitor reduces leukocyte-endothelial cell interactions and chemokine release induced by Ang II in human aortic endothelial cells (141). A more recent study on the effect of dapaglifozin on AAAs in a elastase-induced AAA experimental model (142) demonstrated that the daily treatment with dapagliflozin beginning one day prior to elastase infusion and for 14 days results in a significantly reduced aneurysmal aortic expansion. Besides, dapagliflozin reduced aortic accumulation of macrophages, CD4+ T cells, and B cells, attenuated medial VSMCs loss and reduced neovessel density. An experimental study demonstrated that administration of empagliflozin reverts angiotensin II-induced dissecting AAA in mice (143) possibly by reducing the expression of inflammatory chemokines, VEGF, MMP-2 and MMP9, and by reducing macrophage infiltration into the aortic wall. Empagliflozin reduces the activation of vascular p38 MAPK and NF-κB, which have been implicated in the development of AAA (143, 144).
2.5. GLP-1 analogs and AAA
The incretin hormone, GLP-1 is an intestinal hormone and neuronal peptide which is involved in glucose-induced insulin secretion and participates, together with GIP, in regulating glucose metabolism, energy homeostasis, control of appetite, gastrointestinal motility and trophicity. However, experimental and clinical studies have shown beneficial effects of these hormones in other pathologies and GLP-1-based therapy have been shown to prevents aneurysm formation in vivo (145, 146). While there is a wide knowledge of the role of GLP-1 in the context of ischemic cardiac disease, very little is known about its effect in AAA. The beneficial effect of GLP-1 in AAA might be due to its effect on the mechanisms implicated in AAA formation/progression, such as inflammation, oxidative stress, and proteolytic activity. A recent study speculates that the proteoglycan syndecan-1 (Sdc-1), whose expression is regulated by intracellular targets of the GLP-1 receptor (GLP-1R), modulates pro-inflammatory processes, and has a protective role in AAA (147). In this sense, GLP-1R activation in aortic VSMCs reverts Ang II induced reduction of Sdc-1 expression. Besides, experimental studies in both elastase and calcium chloride and Ang II-induced AAA, treatment with the GLP-1 agonist (lixisenatide and sitagliptin respectively) reduced AAA development, decreased macrophage infiltration and TNFα mRNA and MMP-9 expression in the aorta (148, 149).
2.6. DPP-4 inhibitors and AAA
DPP-4 inhibitors inhibits the proteolytic enzyme DPP-4 which degrades GLP-1, therefore prolonging the action of GLP-1 and resulting in enhanced glycemic control (150). Several recent studies in humans have aimed to investigate the role of DPP-4 in AAA pathogenesis. Lu et al. showed that plasma levels of DPP-4 were increased in patients with AAA and the increase was correlated with the diameter of the AAA. Besides, these authors studied the role of sitagliptin, a DPP-4 inhibitor, in a model of AAA induced by Ang II-infusion in mice. The incidence of AAA formation was significantly lower in mice treated with sitagliptin. Specifically, administered sitagliptin in Ang II-infused mice exhibited decreased expansion of the suprarenal aorta, reduced elastin lamina degradation of the aorta, and diminished vascular inflammation by macrophage infiltration (149). Noda et al. demonstrated that vildagliptin, another DPP-4 inhibitor, significantly diminished the formation of AAA and reduced expression of MMP-2, MMP-9, and IL-6 an experimental model of calcium chloride-induced AAA (151). An experimental study with teneligtip showed that AAA formation was significantly reduced in the treated group compared with the control group. Teneligtip also retarded AAA growth, reduced elastin degradation and reduced macrophage infiltration (152). Similarly, a rat model of AAA showed that alogliptin attenuates aneurysm formation and aortic dilatation ratio via an antioxidative effect (reduction of ROS formation), and reduced MMP2 and MMP-9 expression levels in the aortic tissue (153). Two different studies with the non-selective DPP-4 inhibitor MK0626 in murine models of AAA demonstrated a reduced AAA formation in the treated group compared to the control group that was related with changes in the ECM (154, 155).
A decreased infiltration of macrophages in the aortic wall may be, at least in part, responsible of the protective effect of GLP-1 analogs on AAA formation and progression (156). Studies in mice have shown that TNFα expression levels are increased in aneurysm tissue compared with healthy aortic tissues. Pharmacological and genetic inhibition of TNFα expression decreases AAA and, therefore, the protective effect of GLP-1R agonists limiting AAA development might be due to its effect on TNFα expression (157). Similarly, administration of GLP-1 agonist or DPP-4 inhibitors reduces the expression of MMP-2 and MMP-9 in the aorta. This effect may account for the protective effect of these drugs on preserving the ECM and therefore, on reducing the progression of AAA (158).
3. Conclusion
Although some DM-related factors might protect against AAA formation and progression (e.g., hyperglycemia-modulation of ECM), most features of DM disease are part of the risk factors which are associated with CVD and, most likely, contribute in some degree to the development of AAA. Most published reviews on the association of DM and AAA do not emphasize differences between T1DM and T2DM. In fact, experimental investigation on the study of T2DM and AAA in T2DM mouse models are lacking. Analyzing the differences between the effect of T1DM and T2DM on the formation and progression of AAA may help elucidating the question of whether DM is protective for AAA. Besides, the relationship between AAA and insulin resistance and/or the pre-diabetic state is poorly understood and needs further investigation. Furthermore, the ability of adipose tissue and its secreted adipokines to modulate the vascular environment may require more attention since increased adiposity is one of the main features in T2DM. At the same time, some of the clinical studies linking a protective role of DM in the incidence and progression of AAA do not specify whether those patients are receiving antidiabetic treatments. In addition, controversial results between non-postoperative and postoperative data reveal non-conclusive evidence of the protective effect of DM per se in AAA. The main challenge in these studies in humans is the availability of patients with AAA with no previous drug prescription.
It is being established that the protective effect against AAA is, at least in part, dependent on the pharmacological treatments used for DM. In this regard, recent available literature shows clear protective effects of antidiabetic drugs (metformin, SGLT2-inhibitors, incretin-based therapies, among others) on the incidence and progression of AAA. Although some of these drugs have recently been introduced and cannot explain old observations, other antidiabetic agents such as metformin have been used before and may explain, at least in part, the AAA progression in patients with DM. In addition, new data from specific clinical trials will be available within the next years that will help elucidating the mechanisms by which these drugs protect against AAA.
Author contributions
BP write the review, IC revise the content and help in the figures, LB and JM supervised and corrected the final version. All authors contributed to the article and approved the submitted version.
Funding
This study was funded by the Spanish MINECO (PID2019-106814RB-I00), “la Caixa” Banking Foundation (HR22-00253) and Comunidad de Madrid (P2022/BMD-7333, INMUNOVAR-CM).
Conflict of interest
The authors declare that the research was conducted in the absence of any commercial or financial relationships that could be construed as a potential conflict of interest.
Publisher's note
All claims expressed in this article are solely those of the authors and do not necessarily represent those of their affiliated organizations, or those of the publisher, the editors and the reviewers. Any product that may be evaluated in this article, or claim that may be made by its manufacturer, is not guaranteed or endorsed by the publisher.
References
1. Wanhainen A, Hultgren R, Linné A, Holst J, Gottsäter A, Langenskiöld M, et al. Outcome of the Swedish nationwide abdominal aortic aneurysm screening program. Circulation. (2016) 134(16):1141–8. doi: 10.1161/CIRCULATIONAHA.116.022305
2. Erbel R, Aboyans V, Boileau C, Bossone E, Bartolomeo RD, Eggebrecht H, et al. 2014 ESC guidelines on the diagnosis and treatment of aortic diseases: document covering acute and chronic aortic diseases of the thoracic and abdominal aorta of the adult. The task force for the diagnosis and treatment of aortic diseases of the European society of cardiology (ESC). Eur Heart J. (2014) 35(41):2873–926. doi: 10.1093/eurheartj/ehu281
3. Michel JB, Martin-Ventura JL, Egido J, Sakalihasan N, Treska V, Lindholt J, et al. Novel aspects of the pathogenesis of aneurysms of the abdominal aorta in humans. Cardiovasc Res. (2011) 90(1):18–27. doi: 10.1093/cvr/cvq337
4. Torres-Fonseca M, Galan M, Martinez-Lopez D, Cañes L, Roldan-Montero R, Alonso J, et al. Pathophisiology of abdominal aortic aneurysm: biomarkers and novel therapeutic targets. Clin E Investig En Arterioscler Publicacion of Soc Espanola Arterioscler. (2019) 31(4):166–77. doi: 10.1016/j.arteri.2018.10.002
5. Kessler V, Klopf J, Eilenberg W, Neumayer C, Brostjan C. AAA Revisited: a comprehensive review of risk factors, management, and hallmarks of pathogenesis. Biomedicines. (2022) 10(1):94. doi: 10.3390/biomedicines10010094
6. Anjum A, von Allmen R, Greenhalgh R, Powell JT. Explaining the decrease in mortality from abdominal aortic aneurysm rupture. Br J Surg. (2012) 99(5):637–45. doi: 10.1002/bjs.8698
7. Laine MT, Laukontaus SJ, Sund R, Aho PS, Kantonen I, Albäck A, et al. A population-based study of abdominal aortic aneurysm treatment in Finland 2000 to 2014. Circulation. (2017) 136(18):1726–34. doi: 10.1161/CIRCULATIONAHA.117.028259
8. Zommorodi S, Leander K, Roy J, Steuer J, Hultgren R. Understanding abdominal aortic aneurysm epidemiology: socioeconomic position affects outcome. J Epidemiol Community Health. (2018) 72(10):904–10. doi: 10.1136/jech-2018-210644
9. Kubota Y, Folsom AR, Pankow JS, Wagenknecht LE, Tang W. Diabetes-related factors and abdominal aortic aneurysm events: the atherosclerotic risk in communities study. Ann Epidemiol. (2018) 28(2):102–106.e1. doi: 10.1016/j.annepidem.2017.12.003
10. Chaikof EL, Brewster DC, Dalman RL, Makaroun MS, Illig KA, Sicard GA, et al. SVS Practice guidelines for the care of patients with an abdominal aortic aneurysm: executive summary. J Vasc Surg. (2009) 50(4):880–96. doi: 10.1016/j.jvs.2009.07.001
11. Ma CX, Ma XN, Guan CH, Li YD, Mauricio D, Fu SB. Cardiovascular disease in type 2 diabetes mellitus: progress toward personalized management. Cardiovasc Diabetol. (2022) 21(1):74. doi: 10.1186/s12933-022-01516-6
12. Triplitt C, Alvarez CA. Best practices for lowering the risk of cardiovascular disease in diabetes. Diabetes Spectr. (2008) 21(3):177–89. doi: 10.2337/diaspect.21.3.177
13. Tan SY, Mei Wong JL, Sim YJ, Wong SS, Mohamed Elhassan SA, Tan SH, et al. Type 1 and 2 diabetes mellitus: a review on current treatment approach and gene therapy as potential intervention. Diabetes Metab Syndr. (2019) 13(1):364–72. doi: 10.1016/j.dsx.2018.10.008
14. Climent E, Benaiges D, Chillarón JJ, Flores-Le Roux JA, Pedro-Botet J. Diabetes mellitus as a protective factor of abdominal aortic aneurysm: possible mechanisms. Clin E Investig En Arterioscler Publicacion of Soc Espanola Arterioscler. (2018) 30(4):181–7. doi: 10.1016/j.arteri.2018.01.002
15. Raffort J, Lareyre F, Clément M, Hassen-Khodja R, Chinetti G, Mallat Z. Diabetes and aortic aneurysm: current state of the art. Cardiovasc Res. (2018) 114(13):1702–13. doi: 10.1093/cvr/cvy174
16. Pafili K, Gouni-Berthold I, Papanas N, Mikhailidis DP. Abdominal aortic aneurysms and diabetes mellitus. J Diabetes Complications. (2015) 29(8):1330–6. doi: 10.1016/j.jdiacomp.2015.08.011
17. Weiss JS, Sumpio BE. Review of prevalence and outcome of vascular disease in patients with diabetes mellitus. Eur J Vasc Endovasc Surg Off J Eur Soc Vasc Surg. (2006) 31(2):143–50. doi: 10.1016/j.ejvs.2005.08.015
18. Takagi H, Umemoto T. ALICE (All-Literature investigation of cardiovascular evidence) group. Negative association of diabetes with rupture of abdominal aortic aneurysm. Diab Vasc Dis Res. (2016) 13(5):341–7. doi: 10.1177/1479164116651389
19. Lopez-de-Andrés A, Jiménez-Trujillo I, Jiménez-García R, Hernández-Barrera V, de Miguel-Yanes JM, Méndez-Bailón M, et al. National trends in incidence and outcomes of abdominal aortic aneurysm among elderly type 2 diabetic and non-diabetic patients in Spain (2003-2012). Cardiovasc Diabetol. (2015) 14:48. doi: 10.1186/s12933-015-0216-1
20. Dinesh Shah A, Langenberg C, Rapsomaniki E, Denaxas S, Pujades-Rodriguez M, Gale CP, et al. Type 2 diabetes and incidence of a wide range of cardiovascular diseases: a cohort study in 1·9 million people. Lancet Lond Engl. (2015) 385(Suppl 1):S86. doi: 10.1016/S0140-6736(15)60401-9
21. Xiong J, Wu Z, Chen C, Wei Y, Guo W. Association between diabetes and prevalence and growth rate of abdominal aortic aneurysms: a meta-analysis. Int J Cardiol. (2016) 221:484–95. doi: 10.1016/j.ijcard.2016.07.016
22. De Rango P, Farchioni L, Fiorucci B, Lenti M. Diabetes and abdominal aortic aneurysms. Eur J Vasc Endovasc Surg Off J Eur Soc Vasc Surg. (2014) 47(3):243–61. doi: 10.1016/j.ejvs.2013.12.007
23. Golledge J, Karan M, Moran CS, Muller J, Clancy P, Dear AE, et al. Reduced expansion rate of abdominal aortic aneurysms in patients with diabetes may be related to aberrant monocyte-matrix interactions. Eur Heart J. (2008) 29(5):665–72. doi: 10.1093/eurheartj/ehm557
24. Kristensen KL, Dahl M, Rasmussen LM, Lindholt JS. Glycated hemoglobin is associated with the growth rate of abdominal aortic aneurysms: a substudy from the VIVA (viborg vascular) randomized screening trial. Arterioscler Thromb Vasc Biol. (2017) 37(4):730–6. doi: 10.1161/ATVBAHA.116.308874
25. Nordness MJ, Baxter BT, Matsumura J, Terrin M, Zhang K, Ye F, et al. The effect of diabetes on abdominal aortic aneurysm growth over 2 years. J Vasc Surg. (2022) 75(4):1211–1222.e1. doi: 10.1016/j.jvs.2021.10.019
26. Theivacumar NS, Stephenson MA, Mistry H, Valenti D. Diabetes mellitus and aortic aneurysm rupture: a favorable association? Vasc Endovascular Surg. (2014) 48(1):45–50. doi: 10.1177/1538574413505921
27. Raffort J, Lareyre F, Fabre R, Mallat Z, Pradier C, Bailly L. Nationwide study in France investigating the impact of diabetes on mortality in patients undergoing abdominal aortic aneurysm repair. Sci Rep. (2021) 11(1):19395. doi: 10.1038/s41598-021-98893-x
28. Leurs LJ, Laheij RJF, Buth J, EUROSTAR Collaborators. Influence of diabetes mellitus on the endovascular treatment of abdominal aortic aneurysms. J Endovasc Ther Off J Int Soc Endovasc Spec. (2005) 12(3):288–96. doi: 10.1583/04-1260MR.1
29. Taimour S, Franzén S, Zarrouk M, Acosta S, Nilsson P, Miftaraj M, et al. Nationwide comparison of long-term survival and cardiovascular morbidity after acute aortic aneurysm repair in patients with and without type 2 diabetes. J Vasc Surg. (2020) 71(1):30–38.e3. doi: 10.1016/j.jvs.2019.01.063
30. Hughes K, Jackson JD, Prendergast TI, Rose DA, Bolorunduro O, Obirieze A, et al. Diabetes mellitus is not associated with major morbidity following open abdominal aortic aneurysm repair. J Surg Res. (2013) 184(2):751–4. doi: 10.1016/j.jss.2013.04.025
31. Zarrouk M, Franzén S, Acosta S, Nilsson P, Miftaraj M, Eliasson B, et al. Long-term survival and cardiovascular morbidity after elective open aortic aneurysm repair in patients with and without type 2 diabetes: a nationwide propensity-adjusted analysis. Ann Vasc Surg. (2019) 59:110–8. doi: 10.1016/j.avsg.2019.01.011
32. Taimour S, Avdic T, Franzén S, Zarrouk M, Acosta S, Nilsson P, et al. Survival, cardiovascular morbidity, and reinterventions after elective endovascular aortic aneurysm repair in patients with and without diabetes: a nationwide propensity-adjusted analysis. Vasc Med Lond Engl. (2019) 24(6):539–46. doi: 10.1177/1358863X19870243
33. Dua MM, Miyama N, Azuma J, Schultz GM, Sho M, Morser J, et al. Hyperglycemia modulates plasminogen activator inhibitor-1 expression and aortic diameter in experimental aortic aneurysm disease. Surgery. (2010) 148(2):429–35. doi: 10.1016/j.surg.2010.05.014
34. Miyama N, Dua MM, Yeung JJ, Schultz GM, Asagami T, Sho E, et al. Hyperglycemia limits experimental aortic aneurysm progression. J Vasc Surg. (2010) 52(4):975–83. doi: 10.1016/j.jvs.2010.05.086
35. Pham Y, Tu Y, Wu T, Allen TJ, Calkin AC, Watson AM, et al. Cell division autoantigen 1 plays a profibrotic role by modulating downstream signalling of TGF-beta in a murine diabetic model of atherosclerosis. Diabetologia. (2010) 53(1):170–9. doi: 10.1007/s00125-009-1555-9
36. Chai Z, Dai A, Tu Y, Li J, Wu T, Wang Y, et al. Genetic deletion of cell division autoantigen 1 retards diabetes-associated renal injury. J Am Soc Nephrol JASN. (2013) 24(11):1782–92. doi: 10.1681/ASN.2013010060
37. Li J, Huynh P, Dai A, Wu T, Tu Y, Chow B, et al. Diabetes reduces severity of aortic aneurysms depending on the presence of cell division autoantigen 1 (CDA1). Diabetes. (2018) 67(4):755–68. doi: 10.2337/db17-0134
38. Dai J, Losy F, Guinault AM, Pages C, Anegon I, Desgranges P, et al. Overexpression of transforming growth factor-beta1 stabilizes already-formed aortic aneurysms: a first approach to induction of functional healing by endovascular gene therapy. Circulation. (2005) 112(7):1008–15. doi: 10.1161/CIRCULATIONAHA.104.523357
39. Wang Y, Ait-Oufella H, Herbin O, Bonnin P, Ramkhelawon B, Taleb S, et al. TGF-beta activity protects against inflammatory aortic aneurysm progression and complications in angiotensin II-infused mice. J Clin Invest. (2010) 120(2):422–32. doi: 10.1172/JCI38136
40. Chen X, Rateri DL, Howatt DA, Balakrishnan A, Moorleghen JJ, Cassis LA, et al. TGF-β Neutralization enhances AngII-induced aortic rupture and aneurysm in both thoracic and abdominal regions. PloS One. (2016) 11(4):e0153811. doi: 10.1371/journal.pone.0153811
41. Guo J, Shoji T, Ge Y, Zheng X, Li Y, Zhao S, et al. Treatment with the prolyl hydroxylase inhibitor JNJ promotes abdominal aortic aneurysm progression in diabetic mice. Eur J Vasc Endovasc Surg Off J Eur Soc Vasc Surg. (2022) 63(3):484–94. doi: 10.1016/j.ejvs.2021.10.030
42. King AJ. The use of animal models in diabetes research. Br J Pharmacol. (2012) 166(3):877–94. doi: 10.1111/j.1476-5381.2012.01911.x
43. Morris DR, Jones GT, Holmes MV, Bown MJ, Bulbulia R, Singh TP, et al. Genetic predisposition to diabetes and abdominal aortic aneurysm: a two stage Mendelian randomisation study. Eur J Vasc Endovasc Surg Off J Eur Soc Vasc Surg. (2022) 63(3):512–9. doi: 10.1016/j.ejvs.2021.10.038
44. Åström Malm I, De Basso R, Blomstrand P, Bjarnegård N. Increased arterial stiffness in males with abdominal aortic aneurysm. Clin Physiol Funct Imaging. (2021) 41(1):68–75. doi: 10.1111/cpf.12667
45. Sun J, Deng H, Zhou Z, Xiong X, Gao L. Endothelium as a potential target for treatment of abdominal aortic aneurysm. Oxid Med Cell Longev. (2018) 2018:6306542. doi: 10.1155/2018/6306542
46. DeRoo E, Stranz A, Yang H, Hsieh M, Se C, Zhou T. Endothelial dysfunction in the pathogenesis of abdominal aortic aneurysm. Biomolecules. (2022) 12(4):509. doi: 10.3390/biom12040509
47. Iwakawa N, Tanaka A, Ishii H, Kataoka T, Niwa K, Hitora Y, et al. Impact of diabetes Mellitus on the aortic wall changes as atherosclerosis progresses: aortic dilatation and calcification. J Atheroscler Thromb. (2020) 27(6):509–15. doi: 10.5551/jat.50930
48. Natarajan R. Epigenetic mechanisms in diabetic vascular complications and metabolic memory: the 2020 edwin bierman award lecture. Diabetes. (2021) 70(2):328–37. doi: 10.2337/dbi20-0030
49. Russell NDF, Cooper ME. 50 Years forward: mechanisms of hyperglycaemia-driven diabetic complications. Diabetologia. (2015) 58(8):1708–14. doi: 10.1007/s00125-015-3600-1
50. Papatheodorou K, Banach M, Bekiari E, Rizzo M, Edmonds M. Complications of diabetes 2017. J Diabetes Res. (2018) 2018:3086167. doi: 10.1155/2018/3086167
51. Zhang ZY, Miao LF, Qian LL, Wang N, Qi MM, Zhang YM, et al. Molecular mechanisms of glucose fluctuations on diabetic complications. Front Endocrinol. (2019) 10:640. doi: 10.3389/fendo.2019.00640
52. Senthil KKJ, Gokila VM, Wang SY. Activation of Nrf2-mediated anti-oxidant genes by antrodin C prevents hyperglycemia-induced senescence and apoptosis in human endothelial cells. Oncotarget. (2017) 8(57):96568–87. doi: 10.18632/oncotarget.19951
53. Wan Y, Liu Z, Wu A, Khan AH, Zhu Y, Ding S, et al. Hyperglycemia promotes endothelial cell senescence through AQR/PLAU signaling axis. Int J Mol Sci. (2022) 23(5):2879. doi: 10.3390/ijms23052879
54. Li S, Zhan JK, Wang YJ, Lin X, Zhong JY, Wang Y, et al. Exosomes from hyperglycemia-stimulated vascular endothelial cells contain versican that regulate calcification/senescence in vascular smooth muscle cells. Cell Biosci. (2019) 9:1. doi: 10.1186/s13578-018-0263-x
55. Panchatcharam M, Miriyala S, Yang F, Leitges M, Chrzanowska-Wodnicka M, Quilliam LA, et al. Enhanced proliferation and migration of vascular smooth muscle cells in response to vascular injury under hyperglycemic conditions is controlled by β3 integrin signaling. Int J Biochem Cell Biol. (2010) 42(6):965–74. doi: 10.1016/j.biocel.2010.02.009
56. Kuki S, Imanishi T, Kobayashi K, Matsuo Y, Obana M, Akasaka T. Hyperglycemia accelerated endothelial progenitor cell senescence via the activation of p38 mitogen-activated protein kinase. Circ J Off J Jpn Circ Soc. (2006) 70(8):1076–81. doi: 10.1253/circj.70.1076
57. Yin M, Zhang Y, Yu H, Li X. Role of hyperglycemia in the senescence of mesenchymal stem cells. Front Cell Dev Biol. (2021) 9:665412. doi: 10.3389/fcell.2021.665412
58. Xi G, Shen X, Wai C, White MF, Clemmons DR. Hyperglycemia induces vascular smooth muscle cell dedifferentiation by suppressing insulin receptor substrate-1–mediated p53/KLF4 complex stabilization. J Biol Chem. (2019) 294(7):2407–21. doi: 10.1074/jbc.RA118.005398
59. Astrand H, Rydén-Ahlgren A, Sundkvist G, Sandgren T, Länne T. Reduced aortic wall stress in diabetes mellitus. Eur J Vasc Endovasc Surg Off J Eur Soc Vasc Surg. (2007) 33(5):592–8. doi: 10.1016/j.ejvs.2006.11.011
60. Preil SAR, Kristensen LP, Beck HC, Jensen PS, Nielsen PS, Steiniche T, et al. Quantitative proteome analysis reveals increased content of basement membrane proteins in arteries from patients with type 2 diabetes Mellitus and lower levels among metformin users. Circ Cardiovasc Genet. (2015) 8(5):727–35. doi: 10.1161/CIRCGENETICS.115.001165
61. Steffensen LB, Iversen XES, Hansen RS, Jensen PS, Thorsen ASF, Lindholt JS, et al. Basement membrane proteins in various arterial beds from individuals with and without type 2 diabetes mellitus: a proteome study. Cardiovasc Diabetol. (2021) 20(1):182. doi: 10.1186/s12933-021-01375-7
62. Steffensen LB, Stubbe J, Lindholt JS, Beck HC, Overgaard M, Bloksgaard M, et al. Basement membrane collagen IV deficiency promotes abdominal aortic aneurysm formation. Sci Rep. (2021) 11(1):12903. doi: 10.1038/s41598-021-92303-y
63. Koole D, van Herwaarden JA, Schalkwijk CG, Lafeber FPJG, Vink A, Smeets MB, et al. A potential role for glycated cross-links in abdominal aortic aneurysm disease. J Vasc Surg. (2017) 65(5):1493–1503.e3. doi: 10.1016/j.jvs.2016.04.028
64. Ceriello A, Monnier L, Owens D. Glycaemic variability in diabetes: clinical and therapeutic implications. Lancet Diabetes Endocrinol. (2019) 7(3):221–30. doi: 10.1016/S2213-8587(18)30136-0
65. Ranjan AG, Rosenlund SV, Hansen TW, Rossing P, Andersen S, Nørgaard K. Improved time in range over 1 year is associated with reduced albuminuria in individuals with sensor-augmented insulin pump-treated type 1 diabetes. Diabetes Care. (2020) 43(11):2882–5. doi: 10.2337/dc20-0909
66. Hirakawa Y, Arima H, Zoungas S, Ninomiya T, Cooper M, Hamet P, et al. Impact of visit-to-visit glycemic variability on the risks of macrovascular and microvascular events and all-cause mortality in type 2 diabetes: the ADVANCE trial. Diabetes Care. (2014) 37(8):2359–65. doi: 10.2337/dc14-0199
67. Zinman B, Marso SP, Poulter NR, Emerson SS, Pieber TR, Pratley RE, et al. Day-to-day fasting glycaemic variability in DEVOTE: associations with severe hypoglycaemia and cardiovascular outcomes (DEVOTE 2). Diabetologia. (2018) 61(1):48–57. doi: 10.1007/s00125-017-4423-z
68. Zhou JJ, Schwenke DC, Bahn G, Reaven P, Investigators VADT. Glycemic variation and cardiovascular risk in the veterans affairs diabetes trial. Diabetes Care. (2018) 41(10):2187–94. doi: 10.2337/dc18-0548
69. Echouffo-Tcheugui JB, Zhao S, Brock G, Matsouaka RA, Kline D, Joseph JJ. Visit-to-Visit glycemic variability and risks of cardiovascular events and all-cause mortality: the ALLHAT study. Diabetes Care. (2019) 42(3):486–93. doi: 10.2337/dc18-1430
70. Sheng CS, Tian J, Miao Y, Cheng Y, Yang Y, Reaven PD, et al. Prognostic significance of long-term HbA1c variability for all-cause mortality in the ACCORD trial. Diabetes Care. (2020) 43(6):1185–90. doi: 10.2337/dc19-2589
71. Ceriello A, Ofstad AP, Zwiener I, Kaspers S, George J, Nicolucci A. Empagliflozin reduced long-term HbA1c variability and cardiovascular death: insights from the EMPA-REG OUTCOME trial. Cardiovasc Diabetol. (2020) 19(1):176. doi: 10.1186/s12933-020-01147-9
72. Police SB, Thatcher SE, Charnigo R, Daugherty A, Cassis LA. Obesity promotes inflammation in periaortic adipose tissue and angiotensin II-induced abdominal aortic aneurysm formation. Arterioscler Thromb Vasc Biol. (2009) 29(10):1458–64. doi: 10.1161/ATVBAHA.109.192658
73. Henriques T, Zhang X, Yiannikouris FB, Daugherty A, Cassis LA. Androgen increases AT1a receptor expression in abdominal aortas to promote angiotensin II-induced AAAs in apolipoprotein E-deficient mice. Arterioscler Thromb Vasc Biol. (2008) 28(7):1251–6. doi: 10.1161/ATVBAHA.107.160382
74. Kugo H, Tanaka H, Moriyama T, Zaima N. Pathological implication of adipocytes in AAA development and the rupture. Ann Vasc Dis. (2018) 11(2):159–68. doi: 10.3400/avd.ra.17-00130
75. Wang L, Djousse L, Song Y, Akinkuolie AO, Matsumoto C, Manson JE, et al. Associations of diabetes and obesity with risk of abdominal aortic aneurysm in men. J Obes. (2017) 2017:3521649. doi: 10.1155/2017/3521649
76. Stackelberg O, Björck M, Sadr-Azodi O, Larsson SC, Orsini N, Wolk A. Obesity and abdominal aortic aneurysm. Br J Surg. (2013) 100(3):360–6. doi: 10.1002/bjs.8983
77. Wassink AMJ, van der Graaf Y, Olijhoek JK, Visseren FLJ, SMART Study Group. Metabolic syndrome and the risk of new vascular events and all-cause mortality in patients with coronary artery disease, cerebrovascular disease, peripheral arterial disease or abdominal aortic aneurysm. Eur Heart J. (2008) 29(2):213–23. doi: 10.1093/eurheartj/ehm582
78. Gorter PM, Olijhoek JK, van der Graaf Y, Algra A, Rabelink TJ, Visseren FLJ, et al. Prevalence of the metabolic syndrome in patients with coronary heart disease, cerebrovascular disease, peripheral arterial disease or abdominal aortic aneurysm. Atherosclerosis. (2004) 173(2):363–9. doi: 10.1016/j.atherosclerosis.2003.12.033
79. Lee JS, Park SC, Kim SD. The relation between metabolic syndrome and aspects of abdominal aortic aneurysm. Asian J Surg. (2022) 45(1):307–14. doi: 10.1016/j.asjsur.2021.05.046
80. Folkesson M, Vorkapic E, Gulbins E, Japtok L, Kleuser B, Welander M, et al. Inflammatory cells, ceramides, and expression of proteases in perivascular adipose tissue adjacent to human abdominal aortic aneurysms. J Vasc Surg. (2017) 65(4):1171–1179.e1. doi: 10.1016/j.jvs.2015.12.056
81. Yang WS, Lee WJ, Funahashi T, Tanaka S, Matsuzawa Y, Chao CL, et al. Weight reduction increases plasma levels of an adipose-derived anti-inflammatory protein, adiponectin. J Clin Endocrinol Metab. (2001) 86(8):3815–9. doi: 10.1210/jcem.86.8.7741
82. Yoshida S, Fuster JJ, Walsh K. Adiponectin attenuates abdominal aortic aneurysm formation in hyperlipidemic mice. Atherosclerosis. (2014) 235(2):339–46. doi: 10.1016/j.atherosclerosis.2014.05.923
83. Wågsäter D, Vorkapic E, van Stijn CMW, Kim J, Lusis AJ, Eriksson P, et al. Elevated adiponectin levels suppress perivascular and aortic inflammation and prevent AngII-induced advanced abdominal aortic aneurysms. Sci Rep. (2016) 6:31414. doi: 10.1038/srep31414
84. Thanigaimani S, Golledge J. Role of adipokines and perivascular adipose tissue in abdominal aortic aneurysm: a systematic review and meta-analysis of animal and human observational studies. Front Endocrinol. (2021) 12:618434. doi: 10.3389/fendo.2021.618434
85. Sakaue T, Suzuki J, Hamaguchi M, Suehiro C, Tanino A, Nagao T, et al. Perivascular adipose tissue angiotensin II type 1 receptor promotes vascular inflammation and aneurysm formation. Hypertens Dallas Tex. (2017) 70(4):780–9. doi: 10.1161/HYPERTENSIONAHA.117.09512
86. Gutierrez-Rodelo C, Arellano-Plancarte A, Hernandez-Aranda J, Landa-Galvan HV, Parra-Mercado GK, Moreno-Licona NJ, et al. Angiotensin II inhibits insulin receptor signaling in adipose cells. Int J Mol Sci. (2022) 23(11):6048. doi: 10.3390/ijms23116048
87. Bornfeldt KE, Tabas I. Insulin resistance, hyperglycemia, and atherosclerosis. Cell Metab. (2011) 14(5):575–85. doi: 10.1016/j.cmet.2011.07.015
88. Rask-Madsen C, King GL. Vascular complications of diabetes: mechanisms of injury and protective factors. Cell Metab. (2013) 17(1):20–33. doi: 10.1016/j.cmet.2012.11.012
89. Steinberg HO, Chaker H, Leaming R, Johnson A, Brechtel G, Baron AD. Obesity/insulin resistance is associated with endothelial dysfunction. Implications for the syndrome of insulin resistance. J Clin Invest. (1996) 97(11):2601–10. doi: 10.1172/JCI118709
90. Lareyre F, Moratal C, Zereg E, Carboni J, Panaïa-Ferrari P, Bayer P, et al. Association of abdominal aortic aneurysm diameter with insulin resistance index. Biochem Medica. (2018) 28(3):030702. doi: 10.11613/BM.2018.030702
91. Rask-Madsen C, Li Q, Freund B, Feather D, Abramov R, Wu IH, et al. Loss of insulin signaling in vascular endothelial cells accelerates atherosclerosis in apolipoprotein E null mice. Cell Metab. (2010) 11(5):379–89. doi: 10.1016/j.cmet.2010.03.013
92. Vicent D, Ilany J, Kondo T, Naruse K, Fisher SJ, Kisanuki YY, et al. The role of endothelial insulin signaling in the regulation of vascular tone and insulin resistance. J Clin Invest. (2003) 111(9):1373–80. doi: 10.1172/JCI15211
93. Chinetti G, Carboni J, Murdaca J, Moratal C, Sibille B, Raffort J, et al. Diabetes-Induced changes in macrophage biology might lead to reduced risk for abdominal aortic aneurysm development. Metabolites. (2022) 12(2):128. doi: 10.3390/metabo12020128
94. Costa Rosa LF, Safi DA, Cury Y, Curi R. The effect of insulin on macrophage metabolism and function. Cell Biochem Funct. (1996) 14(1):33–42. doi: 10.1002/(SICI)1099-0844(199603)14:1%3C33::AID-CBF637%3E3.3.CO;2-U
95. Iida KT, Shimano H, Kawakami Y, Sone H, Toyoshima H, Suzuki S, et al. Insulin up-regulates tumor necrosis factor-alpha production in macrophages through an extracellular-regulated kinase-dependent pathway. J Biol Chem. (2001) 276(35):32531–7. doi: 10.1074/jbc.M009894200
96. Senokuchi T, Liang CP, Seimon TA, Han S, Matsumoto M, Banks AS, et al. Forkhead transcription factors (FoxOs) promote apoptosis of insulin-resistant macrophages during cholesterol-induced endoplasmic reticulum stress. Diabetes. (2008) 57(11):2967–76. doi: 10.2337/db08-0520
97. Su D, Coudriet GM, Hyun Kim D, Lu Y, Perdomo G, Qu S, et al. Foxo1 links insulin resistance to proinflammatory cytokine IL-1beta production in macrophages. Diabetes. (2009) 58(11):2624–33. doi: 10.2337/db09-0232
98. Iida KT, Suzuki H, Sone H, Shimano H, Toyoshima H, Yatoh S, et al. Insulin inhibits apoptosis of macrophage cell line, THP-1 cells, via phosphatidylinositol-3-kinase-dependent pathway. Arterioscler Thromb Vasc Biol. (2002) 22(3):380–6. doi: 10.1161/hq0302.105272
99. Ganesan K, Rana MBM, Sultan S. Oral hypoglycemic medications. In: Statpearls. Treasure island (FL): StatPearls Publishing (2022). p. 1–14. Available at: http://www.ncbi.nlm.nih.gov/books/NBK482386/ (Cited September 20, 2022).
100. American Diabetes Association. Standards of medical care in diabetes—2022 abridged for primary care providers. Clin Diabetes. (2022) 40(1):10–38. doi: 10.2337/cd22-as01
101. NPS MedicineWise. Medicines for type 2 diabetes: 2022 update [Internet]. NPS MedicineWise. (2022). Available at: https://www.nps.org.au/type-2-diabetes-meds-2022-update (Cited February 15, 2023).
102. Solis-Herrera C, Triplitt C, Cersosimo E, DeFronzo RA. Pathogenesis of type 2 diabetes Mellitus. In: Feingold KR, Anawalt B, Boyce A, Chrousos G, de Herder WW, Dhatariya K, et al., editors. Endotext. South dartmouth (MA): MDText.com, Inc. (2000). p. 1–161. Available at: http://www.ncbi.nlm.nih.gov/books/NBK279115/ (Cited September 21, 2022).
103. Holman RR, Paul SK, Bethel MA, Matthews DR, Neil HAW. 10-year follow-up of intensive glucose control in type 2 diabetes. N Engl J Med. (2008) 359(15):1577–89. doi: 10.1056/NEJMoa0806470
104. Kooy A, de Jager J, Lehert P, Bets D, Wulffelé MG, Donker AJM, et al. Long-term effects of metformin on metabolism and microvascular and macrovascular disease in patients with type 2 diabetes mellitus. Arch Intern Med. (2009) 169(6):616–25. doi: 10.1001/archinternmed.2009.20
105. Hong J, Zhang Y, Lai S, Lv A, Su Q, Dong Y, et al. Effects of metformin versus glipizide on cardiovascular outcomes in patients with type 2 diabetes and coronary artery disease. Diabetes Care. (2013) 36(5):1304–11. doi: 10.2337/dc12-0719
106. UK Prospective Diabetes Study (UKPDS) Group. Effect of intensive blood-glucose control with metformin on complications in overweight patients with type 2 diabetes (UKPDS 34). Lancet Lond Engl. (1998) 352(9131):854–65. doi: 10.1016/S0140-6736(98)07037-8
107. Guo J, Wang Z, Xue M, Mi L, Zhao M, Ma C, et al. Metformin protects against abdominal aortic aneurysm by Atg7-induced autophagy. Adv Clin Exp Med Off Organ Wroclaw Med Univ. (2022) 31(1):59–69. doi: 10.17219/acem/142026
108. Zhou G, Myers R, Li Y, Chen Y, Shen X, Fenyk-Melody J, et al. Role of AMP-activated protein kinase in mechanism of metformin action. J Clin Invest. (2001) 108(8):1167–74. doi: 10.1172/JCI13505
109. Vasamsetti SB, Karnewar S, Kanugula AK, Thatipalli AR, Kumar JM, Kotamraju S. Metformin inhibits monocyte-to-macrophage differentiation via AMPK-mediated inhibition of STAT3 activation: potential role in atherosclerosis. Diabetes. (2015) 64(6):2028–41. doi: 10.2337/db14-1225
110. Fujimura N, Xiong J, Kettler EB, Xuan H, Glover KJ, Mell MW, et al. Metformin treatment status and abdominal aortic aneurysm disease progression. J Vasc Surg. (2016) 64(1):46–54.e8. doi: 10.1016/j.jvs.2016.02.020
111. Phadwal K, Koo E, Jones RA, Forsythe RO, Tang K, Tang Q, et al. Metformin protects against vascular calcification through the selective degradation of Runx2 by the p62 autophagy receptor. J Cell Physiol. (2022) 237(11):4303–16. doi: 10.1002/jcp.30887
112. Itoga NK, Rothenberg KA, Suarez P, Ho TV, Mell MW, Xu B, et al. Metformin prescription status and abdominal aortic aneurysm disease progression in the U.S. Veteran population. J Vasc Surg. (2019) 69(3):710–716.e3. doi: 10.1016/j.jvs.2018.06.194
113. Raffort J, Hassen-Khodja R, Jean-Baptiste E, Lareyre F. Relationship between metformin and abdominal aortic aneurysm. J Vasc Surg. (2020) 71(3):1056–62. doi: 10.1016/j.jvs.2019.08.270
114. He J, Li N, Fan Y, Zhao X, Liu C, Hu X. Metformin inhibits abdominal aortic aneurysm formation through the activation of the AMPK/mTOR signaling pathway. J Vasc Res. (2021) 58(3):148–58. doi: 10.1159/000513465
115. Unosson J, Wågsäter D, Bjarnegård N, De Basso R, Welander M, Mani K, et al. Metformin prescription associated with reduced abdominal aortic aneurysm growth rate and reduced chemokine expression in a Swedish cohort. Ann Vasc Surg. (2021) 70:425–33. doi: 10.1016/j.avsg.2020.06.039
116. Golledge J, Moxon J, Pinchbeck J, Anderson G, Rowbotham S, Jenkins J, et al. Association between metformin prescription and growth rates of abdominal aortic aneurysms. Br J Surg. (2017) 104(11):1486–93. doi: 10.1002/bjs.10587
117. Isoda K, Young JL, Zirlik A, MacFarlane LA, Tsuboi N, Gerdes N, et al. Metformin inhibits proinflammatory responses and nuclear factor-kappaB in human vascular wall cells. Arterioscler Thromb Vasc Biol. (2006) 26(3):611–7. doi: 10.1161/01.ATV.0000201938.78044.75
118. Li Y, Gappy S, Liu X, Sassalos T, Zhou T, Hsu A, et al. Metformin suppresses pro-inflammatory cytokines in vitreous of diabetes patients and human retinal vascular endothelium. PloS One. (2022) 17(7):e0268451. doi: 10.1371/journal.pone.0268451
119. An H, Wei R, Ke J, Yang J, Liu Y, Wang X, et al. Metformin attenuates fluctuating glucose-induced endothelial dysfunction through enhancing GTPCH1-mediated eNOS recoupling and inhibiting NADPH oxidase. J Diabetes Complications. (2016) 30(6):1017–24. doi: 10.1016/j.jdiacomp.2016.04.018
120. Esfahanian N, Shakiba Y, Nikbin B, Soraya H, Maleki-Dizaji N, Ghazi-Khansari M, et al. Effect of metformin on the proliferation, migration, and MMP-2 and -9 expression of human umbilical vein endothelial cells. Mol Med Rep. (2012) 5(4):1068–74. doi: 10.3892/mmr.2012.753
121. Yuan Z, Heng Z, Lu Y, Wei J, Cai Z. The protective effect of metformin on abdominal aortic aneurysm: a systematic review and meta-analysis. Front Endocrinol. (2021) 12:1–9. doi: 10.3389/fendo.2021.721213
122. Golledge J, Arnott C, Moxon J, Monaghan H, Norman R, Morris D, et al. Protocol for the metformin aneurysm trial (MAT): a placebo-controlled randomised trial testing whether metformin reduces the risk of serious complications of abdominal aortic aneurysm. Trials. (2021) 22(1):962. doi: 10.1186/s13063-021-05915-0
123. Wanhainen A, Unosson J, Mani K, Gottsäter A, Olsson KW, Björck M, et al. The metformin for abdominal aortic aneurysm growth inhibition (MAAAGI) trial. Eur J Vasc Endovasc Surg. (2021) 61(4):710–1. doi: 10.1016/j.ejvs.2020.11.048
124. MD RLD. LIMItIng AAA With meTformin (LIMIT) Trial. clinicaltrials.gov (2022 Mar). Report No.: NCT04500756. Available at: https://clinicaltrials.gov/ct2/show/NCT04500756 [Cited Feb 13, 2023).
125. Motoki T, Kurobe H, Hirata Y, Nakayama T, Kinoshita H, Rocco KA, et al. PPAR-γ agonist attenuates inflammation in aortic aneurysm patients. Gen Thorac Cardiovasc Surg. (2015) 63(10):565–71. doi: 10.1007/s11748-015-0576-1
126. Quinn CE, Hamilton PK, Lockhart CJ, McVeigh GE. Thiazolidinediones: effects on insulin resistance and the cardiovascular system. Br J Pharmacol. (2008) 153(4):636–45. doi: 10.1038/sj.bjp.0707452
127. Pirianov G, Torsney E, Howe F, Cockerill GW. Rosiglitazone negatively regulates c-jun N-terminal kinase and toll-like receptor 4 proinflammatory signalling during initiation of experimental aortic aneurysms. Atherosclerosis. (2012) 225(1):69–75. doi: 10.1016/j.atherosclerosis.2012.07.034
128. Feingold KR Oral and injectable (non-insulin) pharmacological agents for the treatment of type 2 diabetes. In: Feingold KR, Anawalt B, Boyce A, Chrousos G, de Herder WW, Dhatariya K, et al., editors. Endotext. South dartmouth (MA): MDText.com, Inc. (2000). p. 1–262. Available at: http://www.ncbi.nlm.nih.gov/books/NBK279141/ [Cited September 21, 2022).
129. Golledge J, Cullen B, Rush C, Moran CS, Secomb E, Wood F, et al. Peroxisome proliferator-activated receptor ligands reduce aortic dilatation in a mouse model of aortic aneurysm. Atherosclerosis. (2010) 210(1):51–6. doi: 10.1016/j.atherosclerosis.2009.10.027
130. Jones A, Deb R, Torsney E, Howe F, Dunkley M, Gnaneswaran Y, et al. Rosiglitazone reduces the development and rupture of experimental aortic aneurysms. Circulation. (2009) 119(24):3125–32. doi: 10.1161/CIRCULATIONAHA.109.852467
131. Hsu CY, Su YW, Chen YT, Tsai SH, Chang CC, Li SY, et al. Association between use of oral-antidiabetic drugs and the risk of aortic aneurysm: a nested case–control analysis. Cardiovasc Diabetol. (2016) 15(1):125. doi: 10.1186/s12933-016-0447-9
132. Thomas MC, Cherney DZI. The actions of SGLT2 inhibitors on metabolism, renal function and blood pressure. Diabetologia. (2018) 61(10):2098–107. doi: 10.1007/s00125-018-4669-0
133. Honda Y, Imajo K, Kato T, Kessoku T, Ogawa Y, Tomeno W, et al. The selective SGLT2 inhibitor ipragliflozin has a therapeutic effect on nonalcoholic steatohepatitis in mice. PloS One. (2016) 11(1):e0146337. doi: 10.1371/journal.pone.0146337
134. Ohki T, Isogawa A, Toda N, Tagawa K. Effectiveness of ipragliflozin, a sodium-glucose co-transporter 2 inhibitor, as a second-line treatment for non-alcoholic fatty liver disease patients with type 2 diabetes Mellitus who do not respond to incretin-based therapies including glucagon-like peptide-1 analogs and dipeptidyl peptidase-4 inhibitors. Clin Drug Investig. (2016) 36(4):313–9. doi: 10.1007/s40261-016-0383-1
135. Xu L, Ota T. Emerging roles of SGLT2 inhibitors in obesity and insulin resistance: focus on fat browning and macrophage polarization. Adipocyte. (2018) 7(2):121–8. doi: 10.1080/21623945.2017.1413516
136. Ji W, Zhao M, Wang M, Yan W, Liu Y, Ren S, et al. Effects of canagliflozin on weight loss in high-fat diet-induced obese mice. PloS One. (2017) 12(6):e0179960. doi: 10.1371/journal.pone.0179960
137. Bolinder J, Ljunggren Ö, Kullberg J, Johansson L, Wilding J, Langkilde AM, et al. Effects of dapagliflozin on body weight, total fat mass, and regional adipose tissue distribution in patients with type 2 diabetes mellitus with inadequate glycemic control on metformin. J Clin Endocrinol Metab. (2012) 97(3):1020–31. doi: 10.1210/jc.2011-2260
138. Kosiborod M, Cavender MA, Fu AZ, Wilding JP, Khunti K, Holl RW, et al. Lower risk of heart failure and death in patients initiated on sodium-glucose cotransporter-2 inhibitors versus other glucose-lowering drugs: the CVD-REAL study (comparative effectiveness of cardiovascular outcomes in new users of sodium-glucose cotransporter-2 inhibitors). Circulation. (2017) 136(3):249–59. doi: 10.1161/CIRCULATIONAHA.117.029190
139. Han JH, Oh TJ, Lee G, Maeng HJ, Lee DH, Kim KM, et al. The beneficial effects of empagliflozin, an SGLT2 inhibitor, on atherosclerosis in ApoE -/- mice fed a western diet. Diabetologia. (2017) 60(2):364–76. doi: 10.1007/s00125-016-4158-2
140. Nakatsu Y, Kokubo H, Bumdelger B, Yoshizumi M, Yamamotoya T, Matsunaga Y, et al. The SGLT2 inhibitor luseogliflozin rapidly normalizes aortic mRNA levels of inflammation-related but not lipid-metabolism-related genes and suppresses atherosclerosis in diabetic ApoE KO mice. Int J Mol Sci. (2017) 18(8):E1704. doi: 10.3390/ijms18081704
141. Ortega R, Collado A, Selles F, Gonzalez-Navarro H, Sanz MJ, Real JT, et al. SGLT-2 (Sodium-Glucose cotransporter 2) inhibition reduces ang II (angiotensin II)-induced dissecting abdominal aortic aneurysm in ApoE (apolipoprotein E) knockout mice. Arterioscler Thromb Vasc Biol. (2019) 39(8):1614–28. doi: 10.1161/ATVBAHA.119.312659
142. Liu H, Wei P, Fu W, Xia C, Li Y, Tian K, et al. Dapagliflozin ameliorates the formation and progression of experimental abdominal aortic aneurysms by reducing aortic inflammation in mice. Oxid Med Cell Longev. (2022) 2022:8502059. doi: 10.1155/2022/8502059
143. Martorell S, Hueso L, Gonzalez-Navarro H, Collado A, Sanz MJ, Piqueras L. Vitamin D receptor activation reduces angiotensin-II-induced dissecting abdominal aortic aneurysm in apolipoprotein E-knockout mice. Arterioscler Thromb Vasc Biol. (2016) 36(8):1587–97. doi: 10.1161/ATVBAHA.116.307530
144. Saito T, Hasegawa Y, Ishigaki Y, Yamada T, Gao J, Imai J, et al. Importance of endothelial NF-κB signalling in vascular remodelling and aortic aneurysm formation. Cardiovasc Res. (2013) 97(1):106–14. doi: 10.1093/cvr/cvs298
145. Raffort J, Chinetti G, Lareyre F. Glucagon-Like peptide-1: a new therapeutic target to treat abdominal aortic aneurysm? Biochimie. (2018) 152:149–54. doi: 10.1016/j.biochi.2018.06.026
146. Ngetich E, Lapolla P, Chandrashekar A, Handa A, Lee R. The role of dipeptidyl peptidase-IV in abdominal aortic aneurysm pathogenesis: a systematic review. Vasc Med Lond Engl. (2022) 27(1):77–87. doi: 10.1177/1358863X211034574
147. Ntika S, Tracy LM, Franco-Cereceda A, Björck HM, Krizhanovskii C. Syndecan-1 expression is increased in the aortic wall of patients with type 2 diabetes but is unrelated to elevated fasting plasma glucagon-like peptide-1. Biomedicines. (2021) 9(6):697. doi: 10.3390/biomedicines9060697
148. Yu J, Morimoto K, Bao W, Yu Z, Okita Y, Okada K. Glucagon-like peptide-1 prevented abdominal aortic aneurysm development in rats. Surg Today. (2016) 46(9):1099–107. doi: 10.1007/s00595-015-1287-z
149. Lu HY, Huang CY, Shih CM, Chang WH, Tsai CS, Lin FY, et al. Dipeptidyl peptidase-4 inhibitor decreases abdominal aortic aneurysm formation through GLP-1-dependent monocytic activity in mice. PloS One. (2015) 10(4):e0121077. doi: 10.1371/journal.pone.0121077
150. Chinda K, Palee S, Surinkaew S, Phornphutkul M, Chattipakorn S, Chattipakorn N. Cardioprotective effect of dipeptidyl peptidase-4 inhibitor during ischemia-reperfusion injury. Int J Cardiol. (2013) 167(2):451–7. doi: 10.1016/j.ijcard.2012.01.011
151. Noda Y, Miyoshi T, Yonezawa T, Oe H, Toh N, Oono Y, et al. Vildagliptin, a dipeptidyl peptidase-4 inhibitor, ameliorates the development of CaCl2-induced abdominal aortic aneurysm in mice via anti-inflammatory effects. Eur Heart J. (2013) 34(suppl_1):P603. doi: 10.1093/eurheartj/eht307.P603
152. Takahara Y, Tokunou T, Ichiki T. Suppression of abdominal aortic aneurysm formation in mice by teneligliptin, a dipeptidyl peptidase-4 inhibitor. J Atheroscler Thromb. (2018) 25(8):698–708. doi: 10.5551/jat.42481
153. Bao W, Morimoto K, Hasegawa T, Sasaki N, Yamashita T, Hirata K, et al. Orally administered dipeptidyl peptidase-4 inhibitor (alogliptin) prevents abdominal aortic aneurysm formation through an antioxidant effect in rats. J Vasc Surg. (2014) 59(4):1098–108. doi: 10.1016/j.jvs.2013.04.048
154. Byrne JS, Chen E, Husain M, Ouzounian M, Robbins C, Lindsay T. Abstract 101: dipeptidylpeptidase-iv inhibition using MK626 attenuates BAPB/AT2 induced murine aneurysm. Arterioscler Thromb Vasc Biol. (2017) 37(suppl_1):A101.
155. A Dipeptidyl Peptidase-4 Inhibitor but not Incretins Suppresses Abdominal Aortic Aneurysms in Angiotensin II-Infused Apolipoprotein E-Null Mice. Available at: https://www.jstage.jst.go.jp/article/jat/23/4/23_31997/_article (Cited October 14, 2022).
156. Raffort J, Lareyre F, Clément M, Hassen-Khodja R, Chinetti G, Mallat Z. Monocytes and macrophages in abdominal aortic aneurysm. Nat Rev Cardiol. (2017) 14(8):457–71. doi: 10.1038/nrcardio.2017.52
157. Xiong W, MacTaggart J, Knispel R, Worth J, Persidsky Y, Baxter BT. Blocking TNF-alpha attenuates aneurysm formation in a murine model. J Immunol Baltim Md. (2009) 183(4):2741–6. doi: 10.4049/jimmunol.0803164
Keywords: abdominal aortic aneursym, diabet mellitus, insulin resistance, obesity, antidiabetic drugs
Citation: Picatoste B, Cerro-Pardo I, Blanco-Colio LM and Martín-Ventura JL (2023) Protection of diabetes in aortic abdominal aneurysm: Are antidiabetics the real effectors?. Front. Cardiovasc. Med. 10:1112430. doi: 10.3389/fcvm.2023.1112430
Received: 30 November 2022; Accepted: 6 March 2023;
Published: 23 March 2023.
Edited by:
Haruhito A. Uchida, Okayama University, JapanReviewed by:
Anders Gottsäter, Lund University, SwedenLars Melholt Rasmussen, Odense University Hospital, Denmark
© 2023 Picatoste B, Cerro-Pardo, Blanco-Colio and Martín-Ventura. This is an open-access article distributed under the terms of the Creative Commons Attribution License (CC BY). The use, distribution or reproduction in other forums is permitted, provided the original author(s) and the copyright owner(s) are credited and that the original publication in this journal is cited, in accordance with accepted academic practice. No use, distribution or reproduction is permitted which does not comply with these terms.
*Correspondence: Belén Picatoste bWFyaWEucGljYXRvc3RlQHF1aXJvbnNhbHVkLmVz, YnBpY2F0b3N0ZUBnbWFpbC5jb20=
Specialty Section: This article was submitted to Atherosclerosis and Vascular Medicine, a section of the journal Frontiers in Cardiovascular Medicine