- Department of Cardiovascular Medicine, Osaka Metropolitan University Graduate School, Osaka, Japan
Chronic coronary syndrome (CCS) is a progressive disease, which often first manifests as acute coronary syndrome (ACS). Imaging modalities are clinically useful in making decisions about the management of patients with CCS. Accumulating evidence has demonstrated that myocardial ischemia is a surrogate marker for CCS management; however, its ability to predict cardiovascular death or nonfatal myocardial infarction is limited. Herein, we present a review that highlights the latest knowledge available on coronary syndromes and discuss the role and limitations of imaging modalities in the diagnosis and management of patients with coronary artery disease. This review covers the essential aspects of the role of imaging in assessing myocardial ischemia and coronary plaque burden and composition. Furthermore, recent clinical trials on lipid-lowering and anti-inflammatory therapies have been discussed. Additionally, it provides a comprehensive overview of intracoronary and noninvasive cardiovascular imaging modalities and an understanding of ACS and CCS, with a focus on histopathology and pathophysiology.
1. Introduction
Coronary artery disease (CAD) is a progressive disease that often first manifests as acute coronary syndrome (ACS). ACS is a life-threatening disease that affects approximately 1,045,000 people per year in the United States, leading to hospitalization and contributing to a remarkable economic and health care burden (1). In 2019, the European Society of Cardiology proposed the term chronic coronary syndrome (CCS) in the revised guidelines for stable CAD (2). It emphasizes that CCS is a chronic condition rather than the conventional “stable” angina or “stable” CAD. This paradigm shift calls for the early diagnosis, intervention, and continuous treatment of risk factors in patients with CCS to prevent cardiovascular events, including sudden death, ACS, and heart failure.
Clinical symptoms are an important aspect in diagnosing CCS (2). Although ACS often occurs as the first manifestation, noninvasive tests such as electrocardiography, echocardiography and elevated cardiac troponin levels can help in the diagnosis of ACS (3). Imaging modalities are highly useful for assessing the presence of CAD in patients with and without clinical symptoms based on clinical risk factors. The therapeutic goals of CCS include the lifelong prevention of ACS development and symptom reduction (2). While the primary percutaneous coronary intervention (PCI) strategy reportedly improves outcomes in patients with ST-segment elevation myocardial infarction (STEMI) (2), the evaluation of myocardial ischemia, a surrogate marker of the disease, is central to determining indications for coronary revascularization in patients with CCS. The COURAGE (the Clinical Outcomes Utilizing Revascularization and Aggressive Drug Evaluation) trial demonstrated that PCI did not reduce the risk of death, nonfatal myocardial infarction (MI), or other major cardiovascular events (4). Subsequently, a sub-analysis demonstrated that a greater ischemic burden is associated with PCI benefits (5). To determine whether PCI is superior to optimized medical therapy (OMT) in patients with stable angina and a high ischemic burden the International Study of Comparative Health Effectiveness With Medical and Invasive Approaches (ISCHEMIA) trial was conducted at 320 institutions in 37 countries (n = 5,179). The ISCHEMIA trial compared the invasive (OMT + coronary revascularization) and conservative (OMT + invasive strategy, if necessary) strategies in patients with stable angina pectoris and moderate-to-severe myocardial ischemia (>10%), while excluding patients with heart failure, left main coronary lesions, and chronic kidney disease (6). There was no statistically significant difference in the primary endpoint between the two groups during follow-up. The observations from these key clinical trials indicate the importance of OMT and coronary revascularization with their appropriate timing and indications.
The identification of underlying factors, such as coronary plaque burden, high-risk plaques, and coronary microvascular disease (CMD), is crucial for the management of patients with CCS beyond the stage of myocardial ischemia. Figure 1 illustrates the role of imaging and therapeutic targets in the management of patients with CAD. Imaging modalities are clinically useful in deciding the appropriate management of patients with CAD (2, 7–11). The development of noninvasive imaging technologies, such as coronary computed tomography angiography (CCTA) and cardiac magnetic resonance (CMR), has propelled our understanding of the features that accelerate subclinical CAD leading to ACS (12, 13). Intracoronary imaging has deepened our understanding of the mechanisms underlying coronary lesion destabilization in ACS (14, 15). Accurate prediction of coronary lesions leading to ACS requires a comprehensive assessment of plaque vulnerability, including plaque burden (16, 17), inflammatory status (18), coronary plaque mechanical stress (19, 20), and coronary microvascular function (21). These in turn determine the fate of coronary plaque rupture/erosion. In this study, we present a review highlighting the latest CAD knowledge and investigate the role and future perspective of coronary plaque imaging in the diagnosis and management of patients with CAD and clinical trials for lipid-lowering and anti-inflammatory therapies.
2. Pathophysiology of ACS
Understanding the pathogenesis of ACS largely relies on careful autopsy studies of sudden cardiac death. Fuster et al. proposed the concept of ACS, including unstable angina, acute MI, and sudden cardiac death, and determined that plaque rupture-induced thrombus formation is linked to occlusion and severe stenosis of the coronary arteries (3, 22). The most common pathological cause of ACS is plaque rupture; postmortem studies conducted in the 1980s demonstrated that plaque rupture is found in the most fatal MIs (23, 24), indicating the presence of vulnerable plaques. Vulnerable plaques are typically characterized by a large central lipid core which is covered by a thin inflamed fibrous cap with few smooth muscle cells (25); chronic inflammation weakens the collagen structure of the fibrous caps (19, 26). In recent decades, attempts have been made to use imaging to identify precursor lesions that progress to ACS (16, 27–30) on the hypothesis that local therapeutic interventions may prevent plaque rupture-induced thrombosis. Increased plaque volume (31), thin fibrous caps (32), and microcalcifications (27, 33) detected on imaging modalities reportedly serve as independent predictors of ACS; however, ACS prediction remains challenging (34).
Plaque erosion is the second leading cause of ACS, is reportedly more common in women, and has fewer inflammatory cells and proteoglycan-rich lesions than a ruptured plaque (35–38). Superficial erosion-induced plaque thrombosis involves less macrophage-mediated inflammation, just as in the case of fibrous cap rupture. Superficial erosion is complicated by lesions with different epidemiologies and morphologies and involves a pathophysiological mechanism different from that of a fibrous cap rupture. Factors other than plaques, such as endothelial shear stress and neutrophil extracellular traps (NETosis) (37, 38), are thought to play a vital role in plaque erosion-induced thrombus formation. Furthermore, recent clinical studies have demonstrated that intimal healing following a silent plaque rupture/erosion plays a vital role in plaque progression (39, 40).
Following plaque rupture and erosion, calcified nodules are reportedly the least common cause of ACS (≤5%). Calcified nodules are characterized by the eruption of calcified nodules with an underlying fibrocalcific plaque and minimal or no necrosis (25). ACS caused by plaque erosion or calcified nodules can cause non-occlusive thrombosis in the culprit lesion; however, plaque rupture-induced ACS is often accompanied by occlusive thrombosis.
3. Role of imaging in nonobstructive CAD
Approximately one-fifth of ACS cases occur despite the absence of coronary thrombi, suggesting that functional changes other than thrombus formation may contribute to its development. MI with nonobstructive coronary artery disease (MINOCA) is a condition in which MI is diagnosed based on elevated levels of cardiac enzymes and symptoms without evidence of obstructive coronary artery disease on invasive coronary angiography (ICA). The following three diagnostic criteria for MINOCA were proposed in a position paper by the European Society of Cardiology: (1) AMI criteria as defined by the Third Universal Definition of Myocardial Infarction with clinical evidence of ischemic symptoms; (2) absence of a > 50% stenotic lesion in the major epicardial vessels; and (3) absence of other specific causes for the acute clinical symptoms (41). Various mechanisms have been postulated for the development of MINOCA, including plaque disruption, epicardial coronary vasospasm, coronary microvascular dysfunction, coronary embolism/thrombosis, CAD, spontaneous coronary artery dissection, and supply-demand mismatch (42). MINOCA occurs in 6%–8% of patients diagnosed with acute MI. MINOCA is more common in women and often presents as non-STEMI. Imaging modalities such as CMR and intravascular imaging are reportedly useful in investigating the underlying cause of MINOCA (42). Simultaneous assessment of CFR or coronary microvascular resistance and detection of ischemia is recommended during catheter laboratory testing in patients with ischemia with nonobstructive coronary arteries (INOCA) (43). Comprehensive assessment of the structure, ischemia, and CMD will help provide better CCS management (21, 44).
There is a growing interest in INOCA (12, 45, 46), a condition in which there is no significant stenosis in the epicardial coronary artery on ICA despite anginal symptoms and ischemic findings on noninvasive testing. Figure 2 illustrates the diagnostic strategy for INOCA (47). The pathogenesis of vasospastic angina (VSA) involves abnormal endothelial function, that is, decreased production of nitric oxide by endothelial cells, which is a scenario encountered in CCS (38). VSA treatment includes smoking cessation and administration of calcium channel blockers and vasodilators. Another condition of INOCA is CMD, which is more common in women than in men and involves organic microvascular narrowing and coronary spasm (21). The diagnostic criteria of CMD are defined by the Coronary Vasomotor Disorders International Study (COVADIS) group (44): exertional chest pain or dyspnea, absence of obstructive CAD, objective evidence of myocardial ischemia on functional imaging, and CMD as determined by measuring CFR or coronary microvascular resistance (21). Coronary microcirculatory function can be indirectly assessed using noninvasive imaging modalities, including transthoracic doppler echocardiography (48, 49), CMR (50), and positron emission tomography (PET)/computed tomography (CT) (51, 52). Moreover, a comprehensive assessment of CFR and coronary microvascular resistance, in addition to ischemia detection, is recommended in the catheter laboratory when diagnosing INOCA (43).
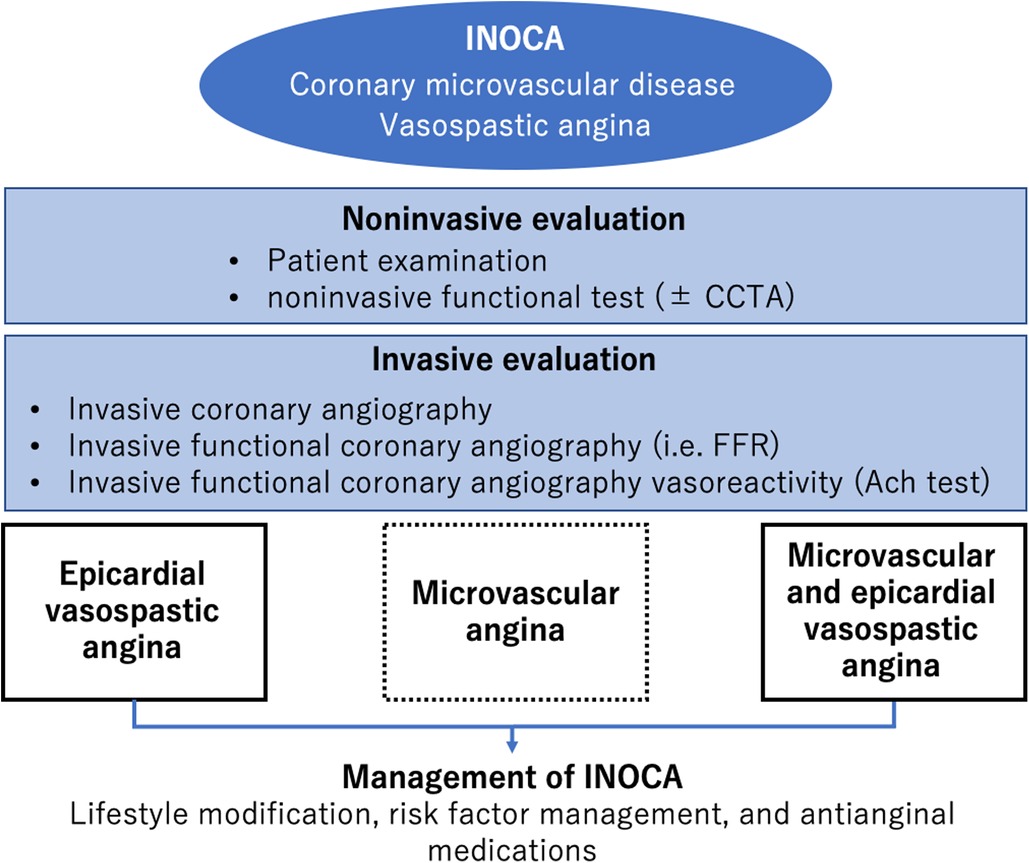
Figure 2. Diagnosis and management of INOCA. Modified from reference (47).
4. Functional or structural imaging
Patients with a history of MI and anginal symptoms are reportedly at high risk of cardiovascular events and may require more intensive treatments (53). For patients with a high pretest probability, evaluation of myocardial ischemia is important for patient management. For several decades, physicians have largely depended on the identification of patients with myocardial ischemia to perform coronary revascularization (54). Functional imaging for identifying myocardial ischemia includes single photon emission computed tomography (SPECT), PET/CT, stress echocardiography, or stress CMR. Although these imaging modalities are highly useful with high diagnostic accuracy for obstructive CAD in clinical practice, their ability to diagnose diffuse nonobstructive CAD is limited (55, 56).
CCTA is a noninvasive imaging technique that enables visualization of cardiac structures, coronary plaque structure and composition, and functional stenosis severity. Table 1 summarizes the recent clinical trials investigating the utility of CCTA in patients with stable chest pain. CCTA reportedly offers a high negative predictive value for significant obstructive CAD and has become a first-line test for symptomatic patients with suspected CAD (59), asymptomatic patients with low-to-intermediate cardiovascular risk (59), and those with low pretest probability (2). The strength of CCTA is that it provides direct visualization of the entire coronary artery and determines the presence of nonobstructive CAD. In the PROMISE (A Randomized Comparison of Anatomic vs. Functional Diagnostic Testing Strategies in Symptomatic Patients with Suspected Coronary Artery Disease) trial which investigated stable symptomatic outpatients referred for non-invasive evaluation of suspected CAD (n = 10,003), there was no significant difference between the two groups (randomized to anatomical testing with CCTA or functional testing) in the primary outcome (7). Further investigation demonstrated that CCTA had a higher discriminatory ability to predict outcomes than functional testing (57). This finding may be explained by the fact that CCTA enables the identification of nonobstructive diseases that develop into ACS and obstructive CAD requiring coronary revascularization (55, 56).
To test the hypothesis that an early invasive treatment strategy would reduce events in patients with moderate or greater ischemia rather than a conservative pharmacotherapeutic treatment strategy, the ISCHEMIA trial included cases of stable angina with documented moderate-to-severe (≥10%) ischemia (6). Blinded CCTA was performed, and patients with left main coronary artery lesions were excluded. A total of 5,179 patients from a total of 8,518 were randomized, with 2,588 patients in the invasive treatment group and 2,591 in the conservative treatment group (mean age, 64 years; 40% had diabetes and 90% had anginal symptoms). The invasive treatment group consisted of patients who underwent diagnostic catheterization within approximately one month if the core laboratory demonstrated ≥10% ischemia with exclusion of left main CAD on CCTA; PCI or CABG was performed within three months, if necessary. The conservative treatment group did not require imaging tests or invasive treatment but was continued on OMT, with a primary focus of controlling the patients’ symptoms. The follow-up period for both groups was 3.3 years, with very high follow-up rates of 99.4% and 99.7%, respectively. These results suggest that OMT may be an appropriate option for patients with CCS who meet the inclusion criteria of the ISCHEMIA trial with SPECT and CCTA. Extended analysis will provide further insights into the appropriate management of patients with CCS (60).
5. Non-invasive plaque imaging
The utility of CCTA-guided management has been well-documented. The SCOT-HEART (Scottish Computed Tomography of the Heart) trial demonstrated that CCTA-guided therapy provides better clinical outcomes than standard therapy does in patients with CCS (8). This can be explained by the effects of aspirin, statins, coronary revascularization, and lifestyle modifications through the identification of the presence of coronary atherosclerotic plaques on CCTA. Figure 3 illustrates a representative CCTA image of a patient with chest pain, showing high-risk plaque features, which led to ACS. The ROMICAT-II (Multicenter Study to Rule Out Myocardial Infarction by Cardiac Computed Tomography) trial demonstrated that high-risk coronary plaque features, including positive remodeling, low-attenuation plaques (LAP), spotty calcification, and napkin-ring sign, were independent predictors of ACS; these serve as useful diagnostic tools to rule out ACS in clinical practice (62). Although the predictive value of high-risk plaque features is not high enough to predict long-term ACS prognosis, a recent clinical trial demonstrated that a LAP volume of >4% is the strongest predictor of clinical risk factors, plaque volume, and stenosis severity (17). CCTA-derived high-risk plaques are associated with an increased incidence of ACS even in patients without myocardial ischemia (55). The combination of fraction flow reserve-CT and coronary structural features may provide a comprehensive assessment of patients requiring coronary revascularization and future ACS events (63).
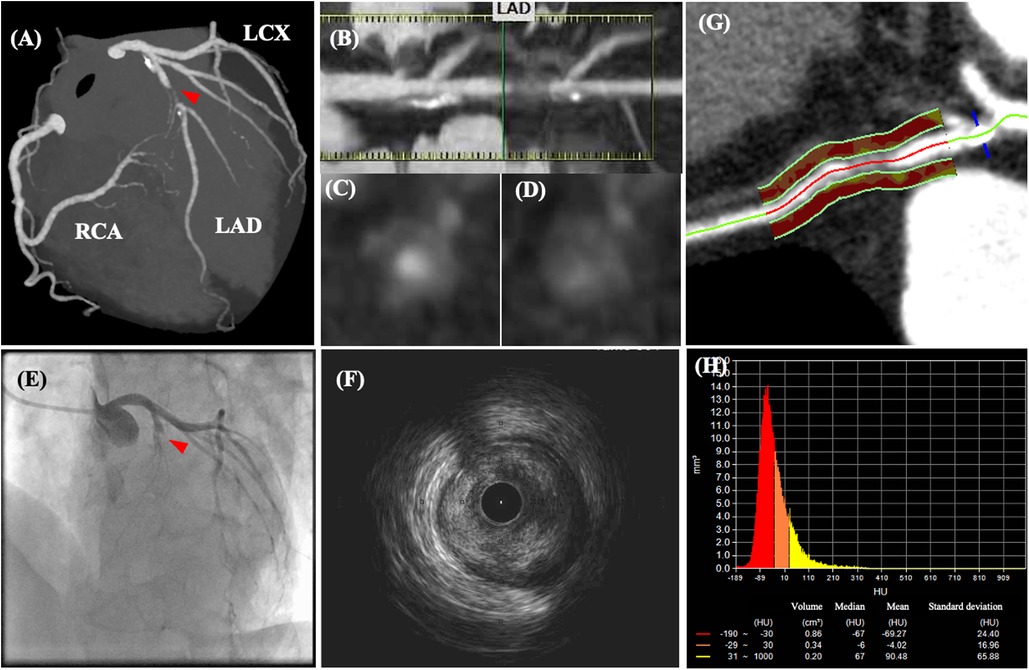
Figure 3. Coronary computed tomography angiography and angiographic images of a patient with acute chest pain. CCTA images of a patient with acute chest pain without ST-segment changes on electrocardiography. CCTA images (A–D) indicate 70–99% luminal stenosis with high-risk plaque features (positive remodeling, low-attenuation plaque, napkin ring sign, and spotty calcification) in the proximal left ascending coronary artery (LAD). After CCTA examination, the patient’s chest pain worsened, and ST-segment elevation was detected. (E) Emergent coronary angiography revealed total occlusion of the LAD, which corresponded to the location of the high-risk plaque visualized on CCTA. (F) Intravascular ultrasonography revealed an intraluminal thrombus secondary to plaque rupture. (G,H) Post-hoc analysis demonstrated an increased pericoronary artery attenuation of >−70 HU. Modified from reference (61).
CMR can assess perfusion and wall motion abnormalities of the left ventricle and cardiac structures, serving as the gold standard noninvasive imaging technique for diagnosing cardiomyopathy (64, 65), myocarditis (66), MI, and mechanical complications of MI (67). CMR with late gadolinium enhancement also allows the visualization of scar tissue and enables differentiation of recent MI from prior MI (68). CMR with T1 mapping is an alternative method for assessing myocardial edema (69, 70). CMR is widely used to differentiate other diseases mimicking ACS, including Takotsubo cardiomyopathy (65, 71) and MI with MINOCA (42). CMR also allows visualization of coronary plaques by assessing the signal intensity (72, 73). Hyperintense plaques, defined as a plaque-to-myocardium signal intensity ratio of >1.4, reportedly predicts ACS events in patients with suspected or known CAD (72). Daniel et al. demonstrated an association of intraplaque hemorrhage-related unstable carotid plaque features with stroke and myocardial infarction in 1,349 patients without a history of stroke or CAD with subclinical atherosclerosis. This indicates that the presence of intraplaque hemorrhage in the carotid arteries is associated with stroke and CAD development, independent of plaque size or cardiovascular risk factors (74, 75).
PET/CT imaging with a variety of radioactive tracer probes is used to identify and characterize arterial plaque burden at high risk for rupture and subsequent thromboembolic vessel occlusion (76). Peripheral vascular and coronary inflammation can be detected and quantified using 18-F-fluorodeoxyglucose (18F-FDG)-PET/CT (77). However, in approximately 50% of patients with ACS or MI, no local increase in coronary 18F-FDG uptake is observed. Thus, a less inflammatory but lipid-rich coronary plaque burden may account for a significant portion of the coronary plaque ruptures. Additionally, 18F-FDG-PET/CT scans may be negative in lipid-rich plaques because inflammation-induced macrophage infiltration, a major substrate for 18F-FDG uptake, is less pronounced in the surviving hypoxic cells. 18F-fluoromisonidazole (FMISO) changes to a more reactive form and remains intracellular by covalently binding to intracellular molecules. Thus, while using 18F-FMISO to signal hypoxia, PET in combination with 18F-sodium fluoride (18F-NaF) can determine active calcification in the CAD process and microcalcifications (78, 79).
6. Intracoronary plaque imaging
ICA, the gold standard for assessing CAD severity, enables visualization of the coronary arterial lumen; however, its ability to assess the outer vessel walls is limited. Intravascular ultrasound (IVUS), optical coherence tomography (OCT), and angioscopy are used to elucidate the pathogenesis of ACS and progression of coronary atherosclerosis. The use of intracoronary imaging in PCI guidance is increasing (80), thereby revealing the post-interventional mechanisms of stent failure, including thrombosis and restenosis (81, 82). Dual antiplatelet therapy (DAPT) effectively prevents post-implantation stent thrombosis; however, bleeding is the primary, major complication ofcoronary revascularization (83). Complex PCI involves intervention in patients with left main disease, multiple stent implantations, and severely calcified lesions, often requiring a longer duration of DAPT (84, 85). Clinical guidelines recommend the potential benefit of an antiplatelet-anticoagulation combination therapy or anticoagulation monotherapy (86). Furthermore, recent clinical studies have demonstrated that intracoronary imaging may beneficially affect the outcomes of patients who undergo PCI (87). Whether antiplatelet or anticoagulation therapy is effective in preventing device-oriented complications in patients undergoing complex PCI remains controversial.
IVUS assesses the coronary plaque morphology, arterial lumen, and vessel wall size, which improves our understanding of the pathogenesis of ACS (88–90). In addition to advances in the faster pullback of grayscale IVUS, virtual histology (VH-), integrated backscatter (IB-), and near-infrared spectroscopy (NIRS-) IVUSs allow the evaluation of atherosclerotic tissue characteristics. In the PROSPECT trial using VH-IVUS, 697 patients with ACS were studied; plaque volume of >70%, VH-thin-capped fibroatheroma (TCFA), and minimal luminal area of <4 mm2 were predictors of lesions associated with a 3-year incidence of major cardiovascular adverse events (MACE) (16). However, IVUS’s (resolution: 100–200 µm) ability to detect fibrous cap thickness in TCFA (<65 µm) is limited. NIRS–IVUS is an imaging technique based on near-infrared spectroscopy that assesses the probability of lipids being present as a chemogram (91). A multicenter prospective study investigating 1,563 patients showed that NIRS–IVUS enabled the identification of patients with a high probability of developing MACE (92). Over a mean follow-up of 732 days, the incidence of MACE in patients with maxLCBI4mm ≥400 without significant stenosis was 13%, which was twice as high as that in patients without maxLCBI4mm ≥400 (6%). These clinical studies indicate that the lipid/necrotic core burden may be a potential therapeutic target.
OCT uses near-infrared light to image the structures of the coronary vessel walls with a high resolution of 0–15 µm (93) (Figure 4). OCT can investigate tissue response and stent expansion or apposition during PCI; however, it requires blood clearing during procedures. Recent clinical trials have demonstrated the non-inferiority of OCT compared to IVUS and its superiority over ICA alone (94). This indicates that intravascular imaging-guided PCI has advantages over angiography-guided PCI in patients with ACS (95) and CCS (96). OCT aids in visualizing the microstructures of the coronary arterial walls, including the fibrous cap (97), lipid content (98, 99), calcification (100), macrophages (101, 102), cholesterol crystals (103, 104) and neovascularization (105–107). In the CLIMA study (108), Prati et al. investigated the prognostic value of OCT findings of lesions in the left descending coronary artery. In a total of 1,776 lipid plaques, the presence of MLA <3.5 mm2, fibrous cap thickness <75 µm, lipid arc circumferential extension of >180°, and OCT-defined macrophages were associated with an increased risk of the primary endpoint. Despite the substantial association between the presence of TCFA and MACE, OCT-TCFAs do not necessarily lead to ACS (99). Most thrombus formation associated with plaque rupture or erosion without clinical events is thought to be remodeled by vessel healing, followed by progression of the stenosis grade (40, 109). These findings have motivated the introduction of novel intracoronary imaging modalities, such as near-infrared autofluorescence and near-infrared fluorescence OCT (110), dual-modality OCT-IVUS (111), and polarization-sensitive (PS-) OCT (112, 113) to investigate the biological tissue components and comprehensive structures of coronary atherosclerosis (114, 115).
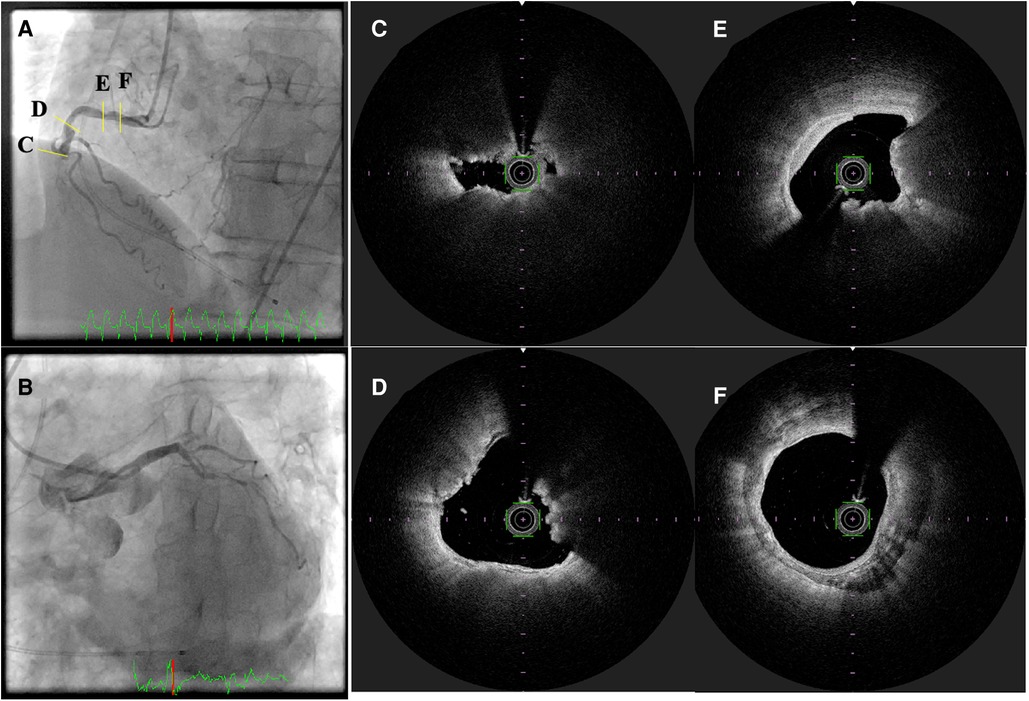
Figure 4. Optical frequency domain imaging of ST-segment elevation myocardial infarction. (A,B) Invasive coronary angiography revealed total occlusion of the mid-portion of the right coronary artery with collateral flow to the left descending coronary artery. (C–F) optical frequency domain imaging (OFDI) images after thrombectomy. (C) Thin capped fibroatheroma, (D,E) calcified nodules (yellow arrows), and (F) calcification.
7. Lipid-lowering therapy in CAD
A meta-analysis of randomized clinical trials (RCT) investigating the effects of intensive lipid-lowering therapy found that statin therapy caused regression of the atherosclerotic disease burden (116). Other RCTs have also demonstrated that for patients at a higher risk of atherosclerosis, lower LDL cholesterol levels were better for plaque regression and CAD prognosis (117–119). Ezetimibe, a commonly used non-statin lipid-lowering drug, reduces LDL cholesterol levels by 13%–20%, with a low incidence of side effects. The IMPROVE-IT (Improved Reduction of Outcomes: Vytorin Efficacy International Trial) trial demonstrated that the addition of ezetimibe to statin therapy resulted in a progressive reduction in LDL cholesterol levels and improved cardiovascular outcomes (118).
The role of imaging in the investigation of the effects of lipid-lowering therapies in patients with CAD is being increasingly recognized. In the FOURIER trials, Sabatine et al. demonstrated that PCSK9 inhibition with evolocumab, a monoclonal antibody, lowers the LDL cholesterol levels, leading to a reduced risk of cardiovascular events. The effect of alirocumab, a PCSK9 inhibitor, on the plaque volume and characteristics were evaluated in the PACMAN-AMI (Effects of the PCSK9 Antibody Alirocumab on Coronary Atherosclerosis in Patients With Acute Myocardial Infarction) randomized trial (15). The mean change in atherosclerotic volume assessed using IVUS was −2.13% in the alirocumab group and −0.92% in the placebo group. The change in the minimal fibrous capsule thickness was also more in the alirocumab group than in the placebo group, proving its plaque-reducing effect.
CCTA is an imaging modality widely used to study the pharmacological effects of changes in the plaque volume and composition. Budoff et al. demonstrated that icosapent ethyl reduced LAP volume (<50 HU) on CCTA over 18 months (58). Furthermore, in 857 patients undergoing serial CCTA imaging, van Rosendael et al. investigated the compositional changes in the untreated, progressed coronary lesions, including low-attenuation (−30 to 75 HU), fibro-fatty (76–130 HU), fibrous (131–350 HU), low-density calcium (351–700 HU), high-density calcium (701–1,000), and 1 K (1,000 HU) plaques (11). Serial CCTA imaging demonstrated that statin therapy is associated with a decrease in low-attenuation and fibrofatty plaques and a greater progression of high-density calcium and 1 K plaques. Taken together, CCTA is a useful imaging modality that assesses coronary structures and disease burden and its changes in response to OMT and lifestyle modifications.
8. Anti-inflammatory therapy and future perspectives of imaging
Although experimental studies have demonstrated a causal relationship between vascular inflammation and atherosclerosis, until recently, no robust evidence has suggested that anti-inflammatory therapy can prevent adverse cardiovascular outcomes (120). Table 2 summarizes the recent clinical trials that have investigated the effects of anti-inflammatory therapy in patients with CAD. In the CANTOS (Canakinumab Antiinflammatory Thrombosis Outcome Study) trial which evaluated the effects of inflammation-targeted therapy in patients with stable CAD at residual inflammatory risk, high doses of canakinumab produced a 15% reduction in MACE and a 17% reduction in cardiovascular events (121). The CIRT trial, a prospective RCT consisting of 4,786 patients with stable atherosclerosis and diabetes or metabolic syndrome, demonstrated that low-dose methotrexate did not reduce MACE (122). However, low-dose methotrexate reduces plasma IL-1β, IL-6, and CRP levels, supporting the concept that adequate inhibition of the innate immune pathway is necessary to ensure long-term cardiovascular benefits.
COLCOT and LoDoCo2 are two large clinical trials that provided and confirmed the hypothesis that repurposed colchicine is an effective anti-inflammatory agent in atherosclerosis (123, 125). Colchicine is an antimitotic agent that inhibits tubulin polymerization and microfibrinolysis. Part of its anti-inflammatory effect is due to its inhibition of NLRP3 inflammasome formation, which indirectly suppresses IL-1β activation and decreases the downstream IL-6 and CRP levels. The COLCOT trial included approximately 5,000 patients with ACS who either received colchicine at 0.5 mg/day or placebo. During the 2-year follow-up, the colchicine group showed a 23% reduction in cardiovascular events. These clinical trials provided evidence for the addition of anti-inflammatory therapy to standard medical regimens, and suggested the importance of imaging techniques to assess vascular inflammation and plaque stabilization (10, 126, 127).
Imaging of the inflammation for the risk stratification can determine patients at a higher risk of atherosclerotic cardiovascular disease (ASCVD). Although PET/CT enables assessment of the inflammatory status of the large aorta, pericoronary arteries, and carotid arteries, its clinical application remains limited (128–131). CCTA enables the measurement of epicardial adipose tissue volume and composition (132, 133). Recent software developments have enabled pericoronary adipose tissue attenuation analysis (Figure 3D), which serves as a predictor of patient outcomes (18). In addition, degradation of collagen, a major component of fibrous caps, plays a pivotal role in coronary plaque healing (134). Microscopic polarization-sensitive (PS) OCT (112, 113) is used to assess plaque structure and tissue polarization. Catheter-based PS-OCT enables quantitative assessment of plaque characteristics, such as collagen, vascular smooth muscle cells, and macrophages, by measuring polarization properties (birefringence and depolarization) (135–137). Otsuka et al. demonstrated that fibrous caps of plaques in patients with ACS had lower birefringence than those of plaques in patients with stable CAD (138). These findings indicate fibrous cap integrity, which could be weakened by matrix metalloproteases-induced collagen degradation. Further studies are warranted to determine whether polarimetric signatures provide additional value for diagnosing plaque stability beyond that provided by the coronary plaque structural features (82, 137–139).
9. Conclusions
The identification of patients with myocardial ischemia can guide the management of stable CAD, which contributes to appropriate coronary revascularization. Systemic and vascular inflammation are potential imaging targets to assess plaque vulnerability in patients at a higher risk of ASCVD events. Novel imaging technologies will open up new avenues for the assessment of plaque vulnerability beyond the stage of ischemia.
Author contributions
RK and KO drafted the manuscript. DF supervised this study and drafted the manuscript. All authors contributed to the article and approved the submitted version.
Funding
This work was partially supported by research grants from the Japanese Heart Foundation, the Konica Minolta Science and Technology Foundation, and KAKENHI (Nos: 20K22923 and 22K08109).
Acknowledgments
We would like to thank Editage (www.editage.com) for the English language editing.
Conflict of interest
The authors declare that the research was conducted in the absence of any commercial or financial relationships that could be construed as a potential conflict of interest.
Publisher's note
All claims expressed in this article are solely those of the authors and do not necessarily represent those of their affiliated organizations, or those of the publisher, the editors and the reviewers. Any product that may be evaluated in this article, or claim that may be made by its manufacturer, is not guaranteed or endorsed by the publisher.
References
1. Virani SS, Alonso A, Benjamin EJ, Bittencourt MS, Callaway CW, Carson AP, et al. Heart disease and stroke statistics-2020 update: a report from the American Heart Association. Circulation. (2020) 141:e139–e596. doi: 10.1161/CIR.0000000000000757
2. Knuuti J, Wijns W, Saraste A, Capodanno D, Barbato E, Funck-Brentano C, et al. 2019 ESC guidelines for the diagnosis and management of chronic coronary syndromes. Eur Heart J. (2020) 41:407–77. doi: 10.1093/eurheartj/ehz425
3. Fuster V, Kovacic JC. Acute coronary syndromes: pathology, diagnosis, genetics, prevention, and treatment. Circ Res. (2014) 114:1847–51. doi: 10.1161/CIRCRESAHA.114.302806
4. Boden WE, O’Rourke RA, Teo KK, Hartigan PM, Maron DJ, Kostuk WJ, et al. Optimal medical therapy with or without PCI for stable coronary disease. N Engl J Med. (2007) 356:1503–16. doi: 10.1056/NEJMoa070829
5. Shaw LJ, Berman DS, Maron DJ, Mancini GBJ, Hayes SW, Hartigan PM, et al. Optimal medical therapy with or without percutaneous coronary intervention to reduce ischemic burden: results from the clinical outcomes utilizing revascularization and aggressive drug evaluation (COURAGE) trial nuclear substudy. Circulation. (2008) 117:1283–91. doi: 10.1161/CIRCULATIONAHA.107.743963
6. Maron DJ, Hochman JS, Reynolds HR, Bangalore S, O’Brien SM, Boden WE, et al. Initial invasive or conservative strategy for stable coronary disease. N Engl J Med. (2020) 382:1395–407. doi: 10.1056/NEJMoa1915922
7. Douglas PS, Hoffmann U, Patel MR, Mark DB, Al-Khalidi HR, Cavanaugh B, et al. Outcomes of anatomical versus functional testing for coronary artery disease. N Engl J Med. (2015) 372:1291–300. doi: 10.1056/NEJMoa1415516
8. SCOT-HEART Investigators, Newby DE, Adamson PD, Berry C, Boon NA, Dweck MR, et al. Coronary CT angiography and 5-year risk of myocardial infarction. N Engl J Med. (2018) 379:924–33. doi: 10.1056/NEJMoa1805971
9. Hecht HS, Blaha MJ, Kazerooni EA, Cury RC, Budoff M, Leipsic J, et al. CAC-DRS: coronary artery calcium data and reporting system. An expert consensus document of the society of cardiovascular computed tomography (SCCT). J Cardiovasc Comput Tomogr. (2018) 12:185–91. doi: 10.1016/j.jcct.2018.03.008
10. Vaidya K, Arnott C, Martínez GJ, Ng B, McCormack S, Sullivan DR, et al. Colchicine therapy and plaque stabilization in patients with acute coronary syndrome: a CT coronary angiography study. JACC Cardiovasc Imaging. (2018) 11:305–16. doi: 10.1016/j.jcmg.2017.08.013
11. van Rosendael AR, van den Hoogen IJ, Gianni U, Ma X, Tantawy SW, Bax AM, et al. Association of statin treatment with progression of coronary atherosclerotic plaque composition. JAMA Cardiol. (2021) 6:1257–66. doi: 10.1001/jamacardio.2021.3055
12. Pasupathy S, Air T, Dreyer RP, Tavella R, Beltrame JF. Systematic review of patients presenting with suspected myocardial infarction and nonobstructive coronary arteries. Circulation. (2015) 131:861–70. doi: 10.1161/CIRCULATIONAHA.114.011201
13. Abdelrahman KM, Chen MY, Dey AK, Virmani R, Finn AV, Khamis RY, et al. Coronary computed tomography angiography from clinical uses to emerging technologies: JACC state-of-the-art review. J Am Coll Cardiol. (2020) 76:1226–43. doi: 10.1016/j.jacc.2020.06.076
14. Araki M, Park SJ, Dauerman HL, Uemura S, Kim JS, Di Mario C, et al. Optical coherence tomography in coronary atherosclerosis assessment and intervention. Nat Rev Cardiol. (2022) 19:684–703. doi: 10.1038/s41569-022-00687-9
15. Räber L, Ueki Y, Otsuka T, Losdat S, Häner JD, Lonborg J, et al. Effect of alirocumab added to high-intensity statin therapy on coronary atherosclerosis in patients with acute myocardial infarction: the PacMan-AMI randomized clinical trial. JAMA. (2022) 327:1771–81. doi: 10.1001/jama.2022.5218
16. Stone GW, Maehara A, Lansky AJ, de Bruyne B, Cristea E, Mintz GS, et al. A prospective natural-history study of coronary atherosclerosis. N Engl J Med. (2011) 364:226–35. doi: 10.1056/NEJMoa1002358
17. Williams MC, Kwiecinski J, Doris M, McElhinney P, D’Souza MS, Cadet S, et al. Low-attenuation noncalcified plaque on coronary computed tomography angiography predicts myocardial infarction: results from the multicenter SCOT-HEART trial (Scottish computed tomography of the HEART). Circulation. (2020) 141:1452–62. doi: 10.1161/CIRCULATIONAHA.119.044720
18. Antonopoulos AS, Sanna F, Sabharwal N, Thomas S, Oikonomou EK, Herdman L, et al. Detecting human coronary inflammation by imaging perivascular fat. Sci Transl Med. (2017) (398):eaal2658. doi: 10.1126/scitranslmed.aal2658
19. Doradla P, Otsuka K, Nadkarni A, Villiger M, Karanasos A, Zandvoort LJCV, et al. Biomechanical stress profiling of coronary atherosclerosis: identifying a multifactorial metric to evaluate plaque rupture risk. JACC Cardiovasc Imaging. (2020) 13:804–16. doi: 10.1016/j.jcmg.2019.01.033
20. Adriaenssens T, Allard-Ratick MP, Thondapu V, Sugiyama T, Raffel OC, Barlis P, et al. Optical coherence tomography of coronary plaque progression and destabilization: JACC focus seminar Part 3/3. J Am Coll Cardiol. (2021) 78:1275–87. doi: 10.1016/j.jacc.2021.07.032
21. Camici PG, d’Amati G, Rimoldi O. Coronary microvascular dysfunction: mechanisms and functional assessment. Nat Rev Cardiol. (2015) 12:48–62. doi: 10.1038/nrcardio.2014.160
22. Fuster V, Badimon L, Badimon JJ, Chesebro JH. The pathogenesis of coronary artery disease and the acute coronary syndromes (1). N Engl J Med. (1992) 326:242–50. doi: 10.1056/NEJM199201233260406
23. Burke AP, Farb A, Malcom GT, Liang YH, Smialek J, Virmani R. Coronary risk factors and plaque morphology in men with coronary disease who died suddenly. N Engl J Med. (1997) 336:1276–82. doi: 10.1056/NEJM199705013361802
24. Virmani R, Kolodgie FD, Burke AP, Farb A, Schwartz SM. Lessons from sudden coronary death: a comprehensive morphological classification scheme for atherosclerotic lesions. Arterioscler Thromb Vasc Biol. (2000) 20:1262–75. doi: 10.1161/01.ATV.20.5.1262
25. Yahagi K, Kolodgie FD, Otsuka F, Finn AV, Davis HR, Joner M, et al. Pathophysiology of native coronary, vein graft, and in-stent atherosclerosis. Nat Rev Cardiol. (2016) 13:79–98. doi: 10.1038/nrcardio.2015.164
26. Burke AP, Kolodgie FD, Farb A, Weber DK, Malcom GT, Smialek J, et al. Healed plaque ruptures and sudden coronary death: evidence that subclinical rupture has a role in plaque progression. Circulation. (2001) 103:934–40. doi: 10.1161/01.CIR.103.7.934
27. Motoyama S, Sarai M, Harigaya H, Anno H, Inoue K, Hara T, et al. Computed tomographic angiography characteristics of atherosclerotic plaques subsequently resulting in acute coronary syndrome. J Am Coll Cardiol. (2009) 54:49–57. doi: 10.1016/j.jacc.2009.02.068
28. Ozaki Y, Okumura M, Ismail TF, Motoyama S, Naruse H, Hattori K, et al. Coronary CT angiographic characteristics of culprit lesions in acute coronary syndromes not related to plaque rupture as defined by optical coherence tomography and angioscopy. Eur Heart J. (2011) 32:2814–23. doi: 10.1093/eurheartj/ehr189
29. Otsuka K, Fukuda S, Tanaka A, Nakanishi K, Taguchi H, Yoshikawa J, et al. Napkin-ring sign on coronary CT angiography for the prediction of acute coronary syndrome. JACC Cardiovasc Imaging. (2013) 6:448–57. doi: 10.1016/j.jcmg.2012.09.016
30. Ahmadi A, Leipsic J, Blankstein R, Taylor C, Hecht H, Stone GW, et al. Do plaques rapidly progress prior to myocardial infarction? The interplay between plaque vulnerability and progression. Circ Res. (2015) 117:99–104. doi: 10.1161/CIRCRESAHA.117.305637
31. Stone GW, Mintz GS, Virmani R. Vulnerable plaques, vulnerable patients, and intravascular imaging. J Am Coll Cardiol. (2018) 72:2022–6. doi: 10.1016/j.jacc.2018.09.010
32. Kume T, Akasaka T, Kawamoto T, Okura H, Watanabe N, Toyota E, et al. Measurement of the thickness of the fibrous cap by optical coherence tomography. Am Heart J. (2006) 152:755.e1–e4. doi: 10.1016/j.ahj.2006.06.030
33. Ehara S, Kobayashi Y, Yoshiyama M, Shimada K, Shimada Y, Fukuda D, et al. Spotty calcification typifies the culprit plaque in patients with acute myocardial infarction: an intravascular ultrasound study. Circulation. (2004) 110:3424–9. doi: 10.1161/01.CIR.0000148131.41425.E9
34. Arbab-Zadeh A, Fuster V. The myth of the “vulnerable plaque”: transitioning from a focus on individual lesions to atherosclerotic disease burden for coronary artery disease risk assessment. J Am Coll Cardiol. (2015) 65:846–55. doi: 10.1016/j.jacc.2014.11.041
35. Arbab-Zadeh A, Fuster V. From detecting the vulnerable plaque to managing the vulnerable patient: JACC state-of-the-art review. J Am Coll Cardiol. (2019) 74:1582–93. doi: 10.1016/j.jacc.2019.07.062
36. Kolodgie FD, Burke AP, Farb A, Weber DK, Kutys R, Wight TN, et al. Differential accumulation of proteoglycans and hyaluronan in culprit lesions: insights into plaque erosion. Arterioscler Thromb Vasc Biol. (2002) 22:1642–8. doi: 10.1161/01.ATV.0000034021.92658.4C
37. Quillard T, Araújo HA, Franck G, Shvartz E, Sukhova G, Libby P. TLR2 And neutrophils potentiate endothelial stress, apoptosis and detachment: implications for superficial erosion. Eur Heart J. (2015) 36:1394–404. doi: 10.1093/eurheartj/ehv044
38. Crea F, Libby P. Acute coronary syndromes: the way forward from mechanisms to precision treatment. Circulation. (2017) 136:1155–66. doi: 10.1161/CIRCULATIONAHA.117.029870
39. Kubo T, Maehara A, Mintz GS, Doi H, Tsujita K, Choi SY, et al. The dynamic nature of coronary artery lesion morphology assessed by serial virtual histology intravascular ultrasound tissue characterization. J Am Coll Cardiol. (2010) 55:1590–7. doi: 10.1016/j.jacc.2009.07.078
40. Yamamoto MH, Yamashita K, Matsumura M, Fujino A, Ishida M, Ebara S, et al. Serial 3-vessel optical coherence tomography and intravascular ultrasound analysis of changing morphologies associated with lesion progression in patients with stable angina pectoris. Circ Cardiovasc Imaging. (2017) 10:1–9. doi: 10.1161/CIRCIMAGING.117.006347
41. Agewall S, Beltrame JF, Reynolds HR, Niessner A, Rosano G, Caforio ALP, et al. ESC Working group position paper on myocardial infarction with non-obstructive coronary arteries. Eur Heart J. (2017) 38:143–53. doi: 10.1093/eurheartj/ehw149
42. Tamis-Holland JE, Jneid H, Reynolds HR, Agewall S, Brilakis ES, Brown TM, et al. Contemporary diagnosis and management of patients with myocardial infarction in the absence of obstructive coronary artery disease: a scientific statement from the American Heart Association. Circulation. (2019) 139:e891–e908. doi: 10.1161/CIR.0000000000000670
43. Marinescu MA, Löffler AI, Ouellette M, Smith L, Kramer CM, Bourque JM. Coronary microvascular dysfunction, microvascular angina, and treatment strategies. JACC Cardiovasc Imaging. (2015) 8:210–20. doi: 10.1016/j.jcmg.2014.12.008
44. Shimokawa H, Suda A, Takahashi J, Berry C, Camici PG, Crea F, et al. Clinical characteristics and prognosis of patients with microvascular angina: an international and prospective cohort study by the coronary vasomotor disorders international study (COVADIS) group. Eur Heart J. (2021) 42:4592–600. doi: 10.1093/eurheartj/ehab282
45. Suda A, Takahashi J, Hao K, Kikuchi Y, Shindo T, Ikeda S, et al. Coronary functional abnormalities in patients with angina and nonobstructive coronary artery disease. J Am Coll Cardiol. (2019) 74:2350–60. doi: 10.1016/j.jacc.2019.08.1056
46. Reynolds HR, Picard MH, Spertus JA, Peteiro J, Lopez Sendon JL, Senior R, et al. Natural history of patients with ischemia and no obstructive coronary artery disease: the CIAO-ischemia study. Circulation. (2021) 144:1008–23. doi: 10.1161/CIRCULATIONAHA.120.046791
47. Kunadian V, Chieffo A, Camici PG, Berry C, Escaned J, Maas AHEM, et al. An EAPCI expert consensus document on ischaemia with non-obstructive coronary arteries in collaboration with European society of cardiology working group on coronary pathophysiology & microcirculation endorsed by coronary vasomotor disorders international study group. Eur Heart J. (2020) 41:3504–20. doi: 10.1093/eurheartj/ehaa503
48. Nakanishi K, Fukuda S, Shimada K, Miyazaki C, Otsuka K, Kawarabayashi T, et al. Prognostic value of coronary flow reserve on long-term cardiovascular outcomes in patients with chronic kidney disease. Am J Cardiol. (2013) 112:928–32. doi: 10.1016/j.amjcard.2013.05.025
49. Kubo T, Fukuda S, Hirata K, Shimada K, Maeda K, Komukai K, et al. Comparison of coronary microcirculation in female nurses after day-time versus night-time shifts. Am J Cardiol. (2011) 108:1665–8. doi: 10.1016/j.amjcard.2011.07.028
50. Zhou W, Lee JCY, Leung ST, Lai A, Lee T-F, Chiang JB, et al. Long-term prognosis of patients with coronary microvascular disease using stress perfusion cardiac magnetic resonance. JACC Cardiovasc Imaging. (2021) 14:602–11. doi: 10.1016/j.jcmg.2020.09.034
51. Naya M, Murthy VL, Foster CR, Gaber M, Klein J, Hainer J, et al. Prognostic interplay of coronary artery calcification and underlying vascular dysfunction in patients with suspected coronary artery disease. J Am Coll Cardiol. (2013) 61:2098–106. doi: 10.1016/j.jacc.2013.02.029
52. Gdowski MA, Murthy VL, Doering M, Monroy-Gonzalez AG, Slart R, Brown DL. Association of isolated coronary microvascular dysfunction with mortality and major adverse cardiac events: a systematic review and meta-analysis of aggregate data. J Am Heart Assoc. (2020) 9:e014954. doi: 10.1161/JAHA.119.014954
53. Sorbets E, Fox KM, Elbez Y, Danchin N, Dorian P, Ferrari R, et al. Long-term outcomes of chronic coronary syndrome worldwide: insights from the international CLARIFY registry. Eur Heart J. (2020) 41:347–56. doi: 10.1093/eurheartj/ehz660
54. Task Force on Myocardial Revascularization of the European Society of Cardiology (ESC) and the European Association for Cardio-Thoracic Surgery (EACTS), European Association for Percutaneous Cardiovascular Interventions (EAPCI), Wijns W, Kolh P, Danchin N, Di Mario C, Falk V, Folliguet T, et al. Guidelines on myocardial revascularization. Eur Heart J. (2010) 31:2501–55. doi: 10.1093/eurheartj/ehq277
55. Otsuka K, Fukuda S, Tanaka A, Nakanishi K, Taguchi H, Yoshiyama M, et al. Prognosis of vulnerable plaque on computed tomographic coronary angiography with normal myocardial perfusion image. Eur Heart J Cardiovasc Imaging. (2014) 15:332–40. doi: 10.1093/ehjci/jet232
56. Lee JM, Choi KH, Koo BK, Park J, Kim J, Hwang D, et al. Prognostic implications of plaque characteristics and stenosis severity in patients with coronary artery disease. J Am Coll Cardiol. (2019) 73:2413–24. doi: 10.1016/j.jacc.2019.02.060
57. Hoffmann U, Ferencik M, Udelson JE, Picard MH, Truong QA, Patel MR, et al. Prognostic value of noninvasive cardiovascular testing in patients with stable chest pain: insights from the PROMISE trial (prospective multicenter imaging study for evaluation of chest pain). Circulation. (2017) 135:2320–32. doi: 10.1161/CIRCULATIONAHA.116.024360
58. Budoff MJ, Bhatt DL, Kinninger A, Lakshmanan S, Muhlestein JB, Le VT, et al. Effect of icosapent ethyl on progression of coronary atherosclerosis in patients with elevated triglycerides on statin therapy: final results of the EVAPORATE trial. Eur Heart J. (2020) 41:3925–32. doi: 10.1093/eurheartj/ehaa652
59. Narula J, Chandrashekhar Y, Ahmadi A, Abbara S, Berman DS, Blankstein R, et al. SCCT 2021 Expert consensus document on coronary computed tomographic angiography: a report of the society of cardiovascular computed tomography. J Cardiovasc Comput Tomogr. (2021) 15:192–217. doi: 10.1016/j.jcct.2020.11.001
60. Anthopolos R, Maron DJ, Bangalore S, Reynolds HR, Xu Y, O’Brien SM, et al. Ischemia-EXTEND studies: rationale and design. Am Heart J. (2022) 254:228–33. doi: 10.1016/j.ahj.2022.09.009
61. Hojo K, Otsuka K, Kasayuki N. Plaque rupture after coronary CT angiography. Eur Heart J. (2022):ehac743. in press. doi: 10.1093/eurheartj/ehac743
62. Puchner SB, Liu T, Mayrhofer T, Truong QA, Lee H, Fleg JL, et al. High-risk plaque detected on coronary CT angiography predicts acute coronary syndromes independent of significant stenosis in acute chest pain: results from the ROMICAT-II trial. J Am Coll Cardiol. (2014) 64:684–92. doi: 10.1016/j.jacc.2014.05.039
63. Lee JM, Choi KH, Koo BK, Park J, Kim J, Hwang D, et al. Prognostic implications of plaque characteristics and stenosis severity in patients with coronary artery disease. J Am Coll Cardiol. (2019) 73:2413–24. doi: 10.1016/j.jacc.2019.02.060
64. Ingkanisorn WP, Kwong RY, Bohme NS, Geller NL, Rhoads KL, Dyke CK, et al. Prognosis of negative adenosine stress magnetic resonance in patients presenting to an emergency department with chest pain. J Am Coll Cardiol. (2006) 47:1427–32. doi: 10.1016/j.jacc.2005.11.059
65. Yamaura H, Ishikawa H, Otsuka K, Kasayuki N. Reverse takotsubo cardiomyopathy as a cause of acute chest pain in a young woman following COVID-19 vaccination. Circ Cardiovasc Imaging. (2022) 15:e013661. doi: 10.1161/CIRCIMAGING.121.013661
66. Ferreira VM, Schulz-Menger J, Holmvang G, Kramer CM, Carbone I, Sechtem U, et al. Cardiovascular magnetic resonance in nonischemic myocardial inflammation: expert recommendations. J Am Coll Cardiol. (2018) 72:3158–76. doi: 10.1016/j.jacc.2018.09.072
67. Tanimoto T, Imanishi T, Kitabata H, Nakamura N, Kimura K, Yamano T, et al. Prevalence and clinical significance of papillary muscle infarction detected by late gadolinium-enhanced magnetic resonance imaging in patients with ST-segment elevation myocardial infarction. Circulation. (2010) 122:2281–7. doi: 10.1161/CIRCULATIONAHA.109.935338
68. Kwong RY, Schussheim AE, Rekhraj S, Aletras AH, Geller N, Davis J, et al. Detecting acute coronary syndrome in the emergency department with cardiac magnetic resonance imaging. Circulation. (2003) 107:531–7. doi: 10.1161/01.cir.0000047527.11221.29
69. Taylor AJ, Salerno M, Dharmakumar R, Jerosch-Herold M. T1 mapping: basic techniques and clinical applications. JACC Cardiovasc Imaging. (2016) 9:67–81. doi: 10.1016/j.jcmg.2015.11.005
70. Kim HW, Jenista ER, Wendell DC, Azevedo CF, Campbell MJ, Darty SN, et al. Patients with acute myocarditis following mRNA COVID-19 vaccination. JAMA Cardiol. (2021) 6:1196–201. doi: 10.1001/jamacardio.2021.2828
71. Bossone E, Lyon A, Citro R, Athanasiadis A, Meimoun P, Parodi G, et al. Takotsubo cardiomyopathy: an integrated multi-imaging approach. Eur Heart J Cardiovasc Imaging. (2014) 15:366–77. doi: 10.1093/ehjci/jet167
72. Noguchi T, Kawasaki T, Tanaka A, Yasuda S, Goto Y, Ishihara M, et al. High-intensity signals in coronary plaques on noncontrast T1-weighted magnetic resonance imaging as a novel determinant of coronary events. J Am Coll Cardiol. (2014) 63:989–99. doi: 10.1016/j.jacc.2013.11.034
73. Matsumoto K, Ehara S, Hasegawa T, Sakaguchi M, Otsuka K, Yoshikawa J, et al. Localization of coronary high-intensity signals on T1-weighted MR imaging: relation to plaque morphology and clinical severity of angina pectoris. JACC Cardiovasc Imaging. (2015) 8:1143–52. doi: 10.1016/j.jcmg.2015.06.013
74. Bos D, Arshi B, van den Bouwhuijsen QJA, Ikram MK, Selwaness M, Vernooij MW, et al. Atherosclerotic carotid plaque composition and incident stroke and coronary events. J Am Coll Cardiol. (2021) 77:1426–35. doi: 10.1016/j.jacc.2021.01.038
75. van der Toorn JE, Bos D, Ikram MK, Verwoert GC, van der Lugt A, Ikram MA, et al. Carotid plaque composition and prediction of incident atherosclerotic cardiovascular disease. Circ Cardiovasc Imaging. (2022) 15:e013602. doi: 10.1161/CIRCIMAGING.121.013602
76. Tarkin JM, Joshi FR, Rudd JHF. PET Imaging of inflammation in atherosclerosis. Nat Rev Cardiol. (2014) 11:443–57. doi: 10.1038/nrcardio.2014.80
77. Rudd JHF, Narula J, Strauss HW, Virmani R, Machac J, Klimas M, et al. Imaging atherosclerotic plaque inflammation by fluorodeoxyglucose with positron emission tomography: ready for prime time? J Am Coll Cardiol. (2010) 55:2527–35. doi: 10.1016/j.jacc.2009.12.061
78. Weck MR, Chow MWL, Joshi NV, Williams MC, Jones C, Fletcher AM, et al. Coronary arterial 18F-sodium fluoride uptake: a novel marker of plaque biology. J Am Coll Cardiol. (2012) 59:1539–48. doi: 10.1016/j.jacc.2011.12.037
79. Joshi NV, Vesey AT, Williams MC, Shah ASV, Calvert PA, Craighead FHM, et al. 18F-fluoride Positron emission tomography for identification of ruptured and high-risk coronary atherosclerotic plaques: a prospective clinical trial. Lancet. (2014) 383:705–13. doi: 10.1016/S0140-6736(13)61754-7
80. Maehara A, Matsumura M, Ali ZA, Mintz GS, Stone GW. IVUS-guided versus OCT-guided coronary stent implantation: a critical appraisal. JACC Cardiovasc Imaging. (2017) 10:1487–503. doi: 10.1016/j.jcmg.2017.09.008
81. Yamaji K, Ueki Y, Souteyrand G, Daemen J, Wiebe J, Nef H, et al. Mechanisms of very late bioresorbable scaffold thrombosis: the INVEST registry. J Am Coll Cardiol. (2017) 70:2330–44. doi: 10.1016/j.jacc.2017.09.014
82. Otsuka K, Villiger M, van Zandvoort LJC, Neleman T, Karanasos A, Dijkstra J, et al. Polarimetric signatures of vascular tissue response to drug-eluting stent implantation in patients. JACC Cardiovasc Imaging. (2020) 13:2695–6. doi: 10.1016/j.jcmg.2020.07.009
83. Valgimigli M, Frigoli E, Heg D, Tijssen J, Jüni P, Vranckx P, et al. Dual antiplatelet therapy after PCI in patients at high bleeding risk. N Engl J Med. (2021) 385:1643–55. doi: 10.1056/NEJMoa2108749
84. Giustino G, Chieffo A, Palmerini T, Valgimigli M, Feres F, Abizaid A, et al. Efficacy and safety of dual antiplatelet therapy after complex PCI. J Am Coll Cardiol. (2016) 68:1851–64. doi: 10.1016/j.jacc.2016.07.760
85. Otsuka K. Newer-generation drug-eluting stents in patients undergoing complex percutaneous coronary intervention. JACC Asia. (2021) 1:342–4. doi: 10.1016/j.jacasi.2021.09.001
86. Valgimigli M, Bueno H, Byrne RA, Collet JP, Costa F, Jeppsson A, et al. 2017 ESC focused update on dual antiplatelet therapy in coronary artery disease developed in collaboration with EACTS: the task force for dual antiplatelet therapy in coronary artery disease of the European society of cardiology (ESC) and of the European association for cardio-thoracic surgery (EACTS). Eur Heart J. (2018) 39:213–60. doi: 10.1093/eurheartj/ehx419
87. Groenland FTW, Neleman T, Kakar H, Scoccia A, des Plantes AC Z, Clephas PRD, et al. Intravascular ultrasound-guided versus coronary angiography-guided percutaneous coronary intervention in patients with acute myocardial infarction: a systematic review and meta-analysis. Int J Cardiol. (2022) 353:35–42. doi: 10.1016/j.ijcard.2022.01.021
88. Tanaka A, Kawarabayashi T, Nishibori Y, Sano T, Nishida Y, Fukuda D, et al. No-reflow phenomenon and lesion morphology in patients with acute myocardial infarction. Circulation. (2002) 105:2148–52. doi: 10.1161/01.cir.0000015697.59592.07
89. Fukuda D, Shimada K, Tanaka A, Kusuyama T, Yamashita H, Ehara S, et al. Comparison of levels of serum matrix metalloproteinase-9 in patients with acute myocardial infarction versus unstable angina pectoris versus stable angina pectoris. Am J Cardiol. (2006) 97:175–80. doi: 10.1016/j.amjcard.2005.08.020
90. Hong MK, Mintz GS, Lee CW, Kim YH, Lee SW, Song JM, et al. Comparison of coronary plaque rupture between stable angina and acute myocardial infarction: a three-vessel intravascular ultrasound study in 235 patients. Circulation. (2004) 110:928–33. doi: 10.1161/01.CIR.0000139858.69915.2E
91. Terada K, Kubo T, Kameyama T, Matsuo Y, Ino Y, Emori H, et al. NIRS-IVUS for differentiating coronary plaque rupture, erosion, and calcified nodule in acute myocardial infarction. JACC Cardiovasc Imaging. (2021) 14:1440–50. doi: 10.1016/j.jcmg.2020.08.030
92. Waksman R, Di Mario C, Torguson R, Ali ZA, Singh V, Skinner WH, et al. Identification of patients and plaques vulnerable to future coronary events with near-infrared spectroscopy intravascular ultrasound imaging: a prospective, cohort study. Lancet. (2019) 394:1629–37. doi: 10.1016/S0140-6736(19)31794-5
93. Bouma BE, Villiger M, Otsuka K, Oh WY. Intravascular optical coherence tomography [invited]. Biomed Opt Express. (2017) 8:2660–86. doi: 10.1364/BOE.8.002660
94. Kubo T, Shinke T, Okamura T, Hibi K, Nakazawa G, Morino Y, et al. Optical frequency domain imaging vs. intravascular ultrasound in percutaneous coronary intervention (OPINION trial): one-year angiographic and clinical results. Eur Heart J. (2017) 38:3139–47. doi: 10.1093/eurheartj/ehx351
95. Meneveau N, Souteyrand G, Motreff P, Caussin C, Amabile N, Ohlmann P, et al. Optical coherence tomography to optimize results of percutaneous coronary intervention in patients with non-ST-elevation acute coronary syndrome: results of the multicenter, randomized DOCTORS study (does optical coherence tomography optimize results of stenting). Circulation. (2016) 134:906–17. doi: 10.1161/CIRCULATIONAHA.116.024393
96. Ali ZA, Maehara A, Généreux P, Shlofmitz RA, Fabbiocchi F, Nazif TM, et al. Optical coherence tomography compared with intravascular ultrasound and with angiography to guide coronary stent implantation (ILUMIEN III: OPTIMIZE PCI): a randomised controlled trial. Lancet. (2016) 388:2618–28. doi: 10.1016/S0140-6736(16)31922-5
97. Yonetsu T, Kakuta T, Lee T, Takahashi K, Kawaguchi N, Yamamoto G, et al. In vivo critical fibrous cap thickness for rupture-prone coronary plaques assessed by optical coherence tomography. Eur Heart J. (2011) 32:1251–9. doi: 10.1093/eurheartj/ehq518
98. Tanaka A, Imanishi T, Kitabata H, Kubo T, Takarada S, Tanimoto T, et al. Lipid-rich plaque and myocardial perfusion after successful stenting in patients with non-ST-segment elevation acute coronary syndrome: an optical coherence tomography study. Eur Heart J. (2009) 30:1348–55. doi: 10.1093/eurheartj/ehp122
99. Xing L, Higuma T, Wang Z, Aguirre AD, Mizuno K, Takano M, et al. Clinical significance of lipid-rich plaque detected by optical coherence tomography: a 4-year follow-up study. J Am Coll Cardiol. (2017) 69:2502–13. doi: 10.1016/j.jacc.2017.03.556
100. Sugiyama T, Yamamoto E, Fracassi F, Lee H, Yonetsu T, Kakuta T, et al. Calcified plaques in patients with acute coronary syndromes. JACC Cardiovasc Interv. (2019) 12:531–40. doi: 10.1016/j.jcin.2018.12.013
101. Tearney GJ, Yabushita H, Houser SL, Aretz HT, Jang IK, Schlendorf KH, et al. Quantification of macrophage content in atherosclerotic plaques by optical coherence tomography. Circulation. (2003) 107:113–9. doi: 10.1161/01.CIR.0000044384.41037.43
102. Phipps JE, Vela D, Hoyt T, Halaney DL, Mancuso JJ, Buja LM, et al. Macrophages and intravascular OCT bright spots: a quantitative study. JACC Cardiovasc Imaging. (2015) 8:63–72. doi: 10.1016/j.jcmg.2014.07.027
103. Otsuka K, Shimada K, Ishikawa H, Nakamura H, Katayama H, Takeda H, et al. Usefulness of pre- and post-stent optical frequency domain imaging findings in the prediction of periprocedural cardiac troponin elevation in patients with coronary artery disease. Heart Vessels. (2020) 35:451–62. doi: 10.1007/s00380-019-01512-z
104. Katayama Y, Tanaka A, Taruya A, Kashiwagi M, Nishiguchi T, Ozaki Y, et al. Feasibility and clinical significance of in vivo cholesterol crystal detection using optical coherence tomography. Arterioscler Thromb Vasc Biol. (2020) 40:220–9. doi: 10.1161/ATVBAHA.119.312934
105. Hasegawa T, Otsuka K, Iguchi T, Matsumoto K, Ehara S, Nakata S, et al. Serum n-3 to n-6 polyunsaturated fatty acids ratio correlates with coronary plaque vulnerability: an optical coherence tomography study. Heart Vessels. (2014) 29:596–602. doi: 10.1007/s00380-013-0404-4
106. Iguchi T, Hasegawa T, Otsuka K, Matsumoto K, Yamazaki T, Nishimura S, et al. Insulin resistance is associated with coronary plaque vulnerability: insight from optical coherence tomography analysis. Eur Heart J Cardiovasc Imaging. (2014) 15:284–91. doi: 10.1093/ehjci/jet158
107. Taruya A, Tanaka A, Nishiguchi T, Matsuo Y, Ozaki Y, Kashiwagi M, et al. Vasa vasorum restructuring in human atherosclerotic plaque vulnerability: a clinical optical coherence tomography study. J Am Coll Cardiol. (2015) 65:2469–77. doi: 10.1016/j.jacc.2015.04.020
108. Prati F, Romagnoli E, Gatto L, La Manna A, Burzotta F, Ozaki Y, et al. Relationship between coronary plaque morphology of the left anterior descending artery and 12 months clinical outcome: the CLIMA study. Eur Heart J. (2020) 41:383–91. doi: 10.1093/eurheartj/ehz520
109. Fracassi F, Crea F, Sugiyama T, Yamamoto E, Uemura S, Vergallo R, et al. Healed culprit plaques in patients with acute coronary syndromes. J Am Coll Cardiol. (2019) 73:2253–63. doi: 10.1016/j.jacc.2018.10.093
110. Ughi GJ, Wang H, Gerbaud E, Gardecki JA, Fard AM, Hamidi E, et al. Clinical characterization of coronary atherosclerosis with dual-modality OCT and near-infrared autofluorescence imaging. JACC Cardiovasc Imaging. (2016) 9:1304–14. doi: 10.1016/j.jcmg.2015.11.020
111. Ren J, Shishkov M, Villiger ML, Otsuka K, Nadkarni SK, Bouma BE. Single-catheter dual-modality intravascular imaging combining IVUS and OFDI: a holistic structural visualisation of coronary arteries. EuroIntervention. (2021) 17:e919–22. doi: 10.4244/EIJ-D-20-00990
112. de Boer JF, Hitzenberger CK, Yasuno Y. Polarization sensitive optical coherence tomography – A review [invited]. Biomed Opt Express. (2017) 8:1838–73. doi: 10.1364/BOE.8.001838
113. Nadkarni SK, Pierce MC, Park BH, de Boer JF, Whittaker P, Bouma BE, et al. Measurement of collagen and smooth muscle cell content in atherosclerotic plaques using polarization-sensitive optical coherence tomography. J Am Coll Cardiol. (2007) 49:1474–81. doi: 10.1016/j.jacc.2006.11.040
114. Bourantas CV, Jaffer FA, Gijsen FJ, Van Soest G, Madden SP, Courtney BK, et al. Hybrid intravascular imaging: recent advances, technical considerations, and current applications in the study of plaque pathophysiology. Eur Heart J. (2017) 38:400–12. doi: 10.1093/eurheartj/ehw097
115. Otsuka K, Villiger M, Nadkarni SK, Bouma BE. Intravascular polarimetry: clinical translation and future applications of catheter-based polarization sensitive optical frequency domain imaging. Front Cardiovasc Med. (2020) 7:146. doi: 10.3389/fcvm.2020.00146
116. Cholesterol Treatment Trialists’ (CTT) Collaboration, Baigent C, Blackwell L, Emberson J, Holland LE, Reith C, et al. Efficacy and safety of more intensive lowering of LDL cholesterol: a meta-analysis of data from 170,000 participants in 26 randomised trials. Lancet. (2010) 376:1670–81. doi: 10.1016/S0140-6736(10)61350-5
117. Boekholdt SM, Hovingh GK, Mora S, Arsenault BJ, Amarenco P, Pedersen TR, et al. Very low levels of atherogenic lipoproteins and the risk for cardiovascular events: a meta-analysis of statin trials. J Am Coll Cardiol. (2014) 64:485–94. doi: 10.1016/j.jacc.2014.02.615
118. Cannon CP, Blazing MA, Giugliano RP, McCagg A, White JA, Theroux P, et al. Ezetimibe added to statin therapy after acute coronary syndromes. N Engl J Med. (2015) 372:2387–97. doi: 10.1056/NEJMoa1410489
119. Sabatine MS, Giugliano RP, Keech AC, Honarpour N, Wiviott SD, Murphy SA, et al. Evolocumab and clinical outcomes in patients with cardiovascular disease. N Engl J Med. (2017) 376:1713–22. doi: 10.1056/NEJMoa1615664
120. Libby P. The changing landscape of atherosclerosis. Nature. (2021) 592:524–33. doi: 10.1038/s41586-021-03392-8
121. Ridker PM, Everett BM, Thuren T, MacFadyen JG, Chang WH, Ballantyne C, et al. Antiinflammatory therapy with canakinumab for atherosclerotic disease. N Engl J Med. (2017) 377:1119–31. doi: 10.1056/NEJMoa1707914
122. Ridker PM, Everett BM, Pradhan A, MacFadyen JG, Solomon DH, Zaharris E, et al. Low-dose methotrexate for the prevention of atherosclerotic events. N Engl J Med. (2019) 380:752–62. doi: 10.1056/NEJMoa1809798
123. Tardif JC, Kouz S, Waters DD, Bertrand OF, Diaz R, Maggioni AP, et al. Efficacy and safety of low-dose colchicine after myocardial infarction. N Engl J Med. (2019) 381:2497–505. doi: 10.1056/NEJMoa1912388
124. Nidorf SM, Eikelboom JW, Budgeon CA, Thompson PL. Low-dose colchicine for secondary prevention of cardiovascular disease. J Am Coll Cardiol. (2013) 61:404–10. doi: 10.1016/j.jacc.2012.10.02
125. Nidorf SM, Fiolet ATL, Mosterd A, Eikelboom JW, Schut A, Opstal TSJ, et al. Colchicine in patients with chronic coronary disease. N Engl J Med. (2020) 383:1838–47. doi: 10.1056/NEJMoa2021372
126. Cecconi A, Vilchez-Tschischke JP, Mateo J, Sanchez-Gonzalez J, España S, Fernandez-Jimenez R, et al. Effects of colchicine on atherosclerotic plaque stabilization: a multimodality imaging study in an animal model. J Cardiovasc Transl Res. (2021) 14:150–60. doi: 10.1007/s12265-020-09974-7
127. Montarello NJ, Singh K, Sinhal A, Wong DTL, Alcock R, Rajendran S, et al. Assessing the impact of colchicine on coronary plaque phenotype after myocardial infarction with optical coherence tomography: rationale and design of the COCOMO-ACS study. Cardiovasc Drugs Ther. (2022) 36:1175–86. doi: 10.1007/s10557-021-07240-9
128. Vesey AT, Jenkins WS, Irkle A, Moss A, Sng G, Forsythe RO, et al. 18F-fluoride and 18F-fluorodeoxyglucose positron emission tomography after transient ischemic attack or Minor ischemic stroke: case-control study. Circ Cardiovasc Imaging. (2017) 10:e004976. doi: 10.1161/CIRCIMAGING.116.004976
129. Fletcher AJ, Tew YY, Tzolos E, Joshi SS, Kaczynski J, Nash J, et al. Thoracic aortic 18F-sodium fluoride activity and ischemic stroke in patients with established cardiovascular disease. JACC Cardiovasc Imaging. (2022) 15:1274–88. doi: 10.1016/j.jcmg.2021.12.013
130. Ohyama K, Matsumoto Y, Shimokawa H. Coronary artery spasm and perivascular adipose tissue inflammation: insights from translational imaging research. Eur Cardiol. (2019) 14:6–9. doi: 10.15420/ecr.2019.3.2
131. Piri R, Gerke O, Høilund-Carlsen PF. Molecular imaging of carotid artery atherosclerosis with PET: a systematic review. Eur J Nucl Med Mol Imaging. (2020) 47:2016–25. doi: 10.1007/s00259-019-04622-y
132. Mahabadi AA, Berg MH, Lehmann N, Kälsch H, Bauer M, Kara K, et al. Association of epicardial fat with cardiovascular risk factors and incident myocardial infarction in the general population: the Heinz Nixdorf Recall Study. J Am Coll Cardiol. (2013) 61:1388–95. doi: 10.1016/j.jacc.2012.11.062
133. Hwang IC, Park HE, Choi SY. Epicardial adipose tissue contributes to the development of non-calcified coronary plaque: a 5-year computed tomography follow-up study. J Atheroscler Thromb. (2017) 24:262–74. doi: 10.5551/jat.36467
134. Vergallo R, Crea F. Atherosclerotic plaque healing. N Engl J Med. (2020) 383:846–57. doi: 10.1056/NEJMra2000317
135. Villiger M, Otsuka K, Karanasos A, Doradla P, Ren J, Lippok N, et al. Coronary plaque microstructure and composition modify optical polarization: a new endogenous contrast mechanism for optical frequency domain imaging. JACC Cardiovasc Imaging. (2018) 11:1666–76. doi: 10.1016/j.jcmg.2017.09.023
136. Villiger M, Otsuka K, Karanasos A, Doradla P, Ren J, Lippok N, et al. Repeatability assessment of intravascular polarimetry in patients. IEEE Trans Med Imaging. (2018) 37:1618–25. doi: 10.1109/TMI.2018.2815979
137. van Zandvoort LJC, Otsuka K, Villiger M, Neleman T, Dijkstra J, Zijlstra F, et al. Polarimetric signatures of coronary thrombus in patients with acute coronary syndrome. Circ J. (2021) 85:1806–13. doi: 10.1253/circj.CJ-20-0862
138. Otsuka K, Villiger M, Karanasos A, van Zandvoort LJC, Doradla P, Ren J, et al. Intravascular polarimetry in patients with coronary artery disease. JACC Cardiovasc Imaging. (2020) 13:790–801. doi: 10.1016/j.jcmg.2019.06.015
Keywords: chronic coronary syndrome, acute coronary syndrome, imaging, ischemia, atherosclerosis, plaque vulnerability, coronary microvascular dysfunction, inflammation
Citation: Kitada R, Otsuka K and Fukuda D (2023) Role of plaque imaging for identification of vulnerable patients beyond the stage of myocardial ischemia. Front. Cardiovasc. Med. 10:1095806. doi: 10.3389/fcvm.2023.1095806
Received: 11 November 2022; Accepted: 21 February 2023;
Published: 17 March 2023.
Edited by:
Masanori Aikawa, Harvard Medical School, United States© 2023 Kitada, Otsuka and Fukuda. This is an open-access article distributed under the terms of the Creative Commons Attribution License (CC BY). The use, distribution or reproduction in other forums is permitted, provided the original author(s) and the copyright owner(s) are credited and that the original publication in this journal is cited, in accordance with accepted academic practice. No use, distribution or reproduction is permitted which does not comply with these terms.
*Correspondence: Kenichiro Otsuka b3RzdWthLmtlbmljaGlyb0BvbXUuYWN1Lmpw
†These authors have contributed equally to this work
Specialty Section: This article was submitted to Atherosclerosis and Vascular Medicine, a section of the journal Frontiers in Cardiovascular Medicine