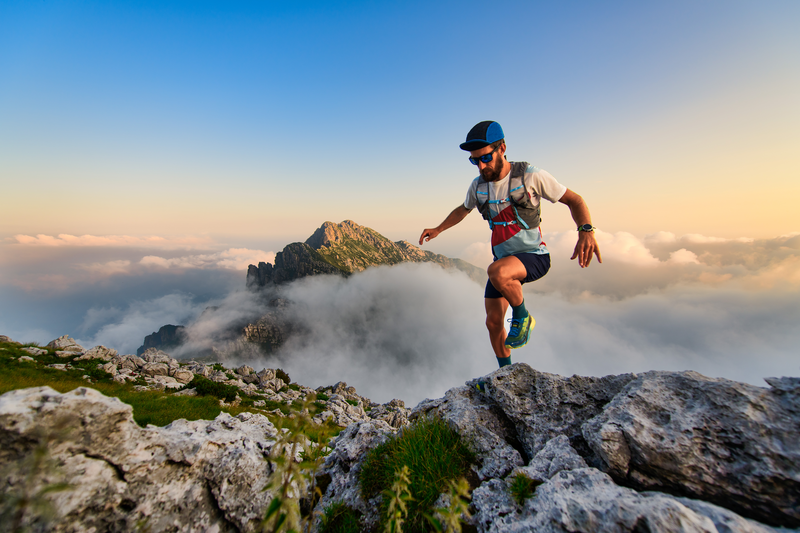
94% of researchers rate our articles as excellent or good
Learn more about the work of our research integrity team to safeguard the quality of each article we publish.
Find out more
ORIGINAL RESEARCH article
Front. Cardiovasc. Med. , 10 February 2023
Sec. Atherosclerosis and Vascular Medicine
Volume 10 - 2023 | https://doi.org/10.3389/fcvm.2023.1042272
This article is part of the Research Topic Revolutions in Human Biospecimen Study: Leveraging new technologies to explore the pathology, therapeutics and biomarkers for atherosclerotic disease View all 10 articles
Introduction: Autoantibodies against inflammatory cytokines may be used for the prevention of atherosclerosis. Preclinical studies consider colony-stimulating factor 2 (CSF2) as an essential cytokine with a causal relationship to atherosclerosis and cancer. We examined the serum anti-CSF2 antibody levels in patients with atherosclerosis or solid cancer.
Methods: We measured the serum anti-CSF2 antibody levels via amplified luminescent proximity homogeneous assay-linked immunosorbent assay based on the recognition of recombinant glutathione S-transferase-fused CSF2 protein or a CSF2-derived peptide as the antigen.
Results: The serum anti-CSF2 antibody (s-CSF2-Ab) levels were significantly higher in patients with acute ischemic stroke (AIS), acute myocardial infarction (AMI), diabetes mellitus (DM), and chronic kidney disease (CKD) compared with healthy donors (HDs). In addition, the s-CSF2-Ab levels were associated with intima-media thickness and hypertension. The analyzes of samples obtained from a Japan Public Health Center-based prospective study suggested the utility of s-CSF2-Ab as a risk factor for AIS. Furthermore, the s-CSF2-Ab levels were higher in patients with esophageal, colorectal, gastric, and lung cancer than in HDs but not in those with mammary cancer. In addition, the s-CSF2-Ab levels were associated with unfavorable postoperative prognosis in colorectal cancer (CRC). In CRC, the s-CSF2-Ab levels were more closely associated with poor prognosis in patients with p53-Ab-negative CRC despite the lack of significant association of the anti-p53 antibody (p53-Ab) levels with the overall survival.
Conclusion: S-CSF2-Ab was useful for the diagnosis of atherosclerosis-related AIS, AMI, DM, and CKD and could discriminate poor prognosis, especially in p53-Ab-negative CRC.
Cytokines, such as interleukins, tumor necrosis factor, interferons, and colony-stimulating factors, contribute to the presence and development of a variety of diseases (1, 2), including cancer (3), acute ischemic stroke (AIS; 4), and acute myocardial infarction (AMI; 5). As a cytokine, colony-stimulating factor 2 (CSF2, also known as granulocyte-macrophage colony-stimulating factor) deficiency has been reported to be associated with increased atherogenesis under hypercholesterolemic conditions in mouse models (6), and the administration of CSF2 can prevent the progression of atherosclerosis through changes in the composition of atherosclerotic lesions (7). Atherosclerosis is intimately linked to and accompanied by diabetes mellitus (DM) and chronic kidney disease (CKD) (8). Several reports have demonstrated that atherosclerosis mainly contributes to the emergence of AIS and AMI (9, 10).
Intriguingly, growing evidence supports that atherosclerosis and cancer are closely related based on the shared pathophysiology of inflammation as a disease promoter (11, 12). Furthermore, CSF2 has been shown to exert antitumor activity in gastrointestinal tract cancers, such as esophageal cancer (EC; 13), colorectal cancer (CRC) (14), and gastric cancer (GC; 15). CSF2 upregulation was associated with increased aggressiveness of various tumor types, including head and neck cancers (16), glioblastomas (17), and bladder cancer (18). The utility of widely used serum biomarkers, such as carcinoembryonic antigen (CEA) (19), carbohydrate antigen 19–9 (CA19-9) (20), and anti-p53 antibody (p53-Ab; 21–23), for the early diagnosis and prognosis prediction of gastrointestinal tract cancers is limited (24, 25). The discovery of novel biomarkers in gastrointestinal tract cancers is required for the prognostic prediction and development of targeted therapeutics.
Recent studies have reported the production of serum autoantibodies against secreted proteins (25–32). Autoantibodies produced against cytokines circulating in the peripheral blood can potentially impact their binding to target membrane receptors and the subsequent disease progression. We have previously identified autoantibodies against CSF2 in the sera of patients with acute cardiac syndrome (33). In this study, we examined the serum anti-CSF2 antibody levels in patients with AIS, AMI, DM, CKD, and cancer.
The sera of patients with AIS and transient ischemic attack (TIA), which were collected within 2 weeks after disease onset, were obtained from Chiba Prefectural Sawara Hospital and Chiba Rosai Hospital. The stroke subtypes were determined according to the criteria of the Trial of ORG 10172 in Acute Stroke Treatment classification system (34), and large-artery atherosclerosis and small-arterial occlusion (lacuna) were included as AIS or ischemic stroke. The sera of patients with DM and AMI were obtained from Chiba University Hospital and Kyoto University Hospital, respectively. The sera of patients with CKD were obtained from the Kumamoto cohort (35, 36). The Department of Surgery, Toho University Hospital, collected sera from patients with EC, GC, CRC, lung cancer (LC), and mammary cancer (MC; 37) between June 2010 and February 2016, and all patients were followed up until July 2018 or death. EC was analyzed in 91 cases, GC was analyzed in 57 cases and CRC was analyzed in 113 cases, of which all cases were underwent radical surgery. Patients who underwent neoadjuvant chemotherapy and had a double cancer were excluded from the study. According to the Japanese Classification of Colorectal, Appendiceal, and Anal Carcinoma, 3d English Edition (Secondary Publication; 38), the numbers of patients with colorectal cancer were as follows: five patients in stage 0, 29 in stage I, 32 in stage II, 31 in stage III, and 16 in stage IV. In addition, the sera of healthy donors (HDs) were selected from people who underwent a medical checkup at Chiba University, Port Square Kashiwado Clinic, and Chiba Prefectural Sawara Hospital. Clinicopathological characteristics and prognoses were obtained retrospectively. Individuals with no history of cancer, autoimmune disease, or cerebrovascular disease and those without any abnormalities on cranial MRI were enrolled as HDs.
Serum samples of AIS, TIA, DM, CKD, and HD were collected at the time of hospital admission. Serum samples of the cancers were collected before treatment. All serum samples were centrifuged at 3,000 g for 10 min, and the supernatants were stored at −80°C until use. To preserve sample integrity, repeated freezing/thawing of serum samples was avoided.
Clinical data of all patients; e.g., maximum intima-media thickness (max-IMT; 39), were the maximum values of the intima-media thickness for both left and right sides of the carotid ultrasound results performed using a high-frequency line array probe. The plaque score was computed by summing the maximum thickness (millimeters) of the plaques in each segment on both sides (40, 41). The cardio-ankle vascular index (CAVI; 42), which is independent of blood pressure, was developed using CAVI = a{(2ρ/ΔP) x ln(Ps/Pd)PWV2} + b, where Ps is the systolic blood pressure, Pd is the diastolic blood pressure, PWV is the pulse wave velocity, ΔP is Ps–Pd, ρ is blood density, and a and b are scale conversion constants. Scale conversion constants were determined to match CAVI with PWV (Hasegawa’s method). All these measurements and calculations were made at the same time automatically in VaSera (Fukuda Denshi Co. LTD, Tokyo). All data were collected by Japanese clinical professionals and were written in the patient’s clinical record. Data regarding the risk factors for atherosclerosis, including hypertension, diabetes, hyperlipidemia, cardiovascular disease (CVD), smoking, and alcohol usage were defined as previously described (43, 44) and the levels of serum p53 antibodies (p53-Abs; 22), serum CEA (45) and CA19-9 (46) were also evaluated as previously described. The cutoff values for serum p53-Ab, CEA, and CA19-9 levels were set at 1.3 IU/ml, 10 ng/ml, and 37.0 ng/ml, respectively.
The cDNA containing the amino-terminal (first 145 amino-acid residues) of CSF2 (NM_000758.4) was inserted into the BamHI/NotI site of pGEX-4 T-1 to obtain the pGEX-4 T-1-CSF2 plasmid (GenScript, Piscataway, NJ). The expression of the cDNA product was induced by treating pGEX-4 T-1-CSF2-transformed Escherichia coli BL-21 with 0.1-mM isopropyl-β-D-thiogalactoside (Wako Pure Chemicals, Osaka, Japan) for 4 h at 37°C. The cells were subsequently lysed in BugBuster Master Mix (Merck KGaA, Darmstadt, Germany). Glutathione S-transferase (GST)-tagged CSF2 protein was purified using Glutathione-Sepharose® (Cytiva, Pittsburgh, PA column chromatography according to the manufacturer’s protocols, as previously described) (43, 47).
The potential antigenic epitopes in CSF2 were predicted through the examination of the full-length CSF2 protein using an online tool1, as previously described (48, 49). An N-terminal biotinylated 18-mer peptide encompassing the amino-acid residues between 77 and 94 of CSF2 was designed (33), and the synthetic peptide with a purity >95.93% was manufactured by Eurofins Genomics K.K. (Tokyo, Japan). The amino-acid sequence of the peptide, designated as bCSF2-77, is as follows: biotin-LYKQGLRGSLTKLKGPLT-COOH.
Purified GST-CSF2 and control GST proteins were electrophoresed using 11% sodium dodecyl-sulfate (SDS)–polyacrylamide gels, followed by transfer to nitrocellulose membranes (Advantec, Tokyo, Japan). The membranes were blocked with 0.1% dry milk in Tris-buffered saline [150 mM NaCl, 20 mM Tris–HCl (pH 7.6), and 0.1% Tween-20; TBS-T] and subsequently incubated with anti-GST antibodies (goat, ab6613, Abcam, Cambridgeshire, United Kingdom), anti-CSF2 antibodies (rabbit, sc-37,753, Santa Cruz Biotechnology, Dallas, TX), or HD and patient sera diluted at 1:1000. Thereafter, the membranes were washed five times with TBS-T and incubated with horseradish peroxidase-conjugated second antibodies (anti-goat IgG, anti-rabbit IgG, and antihuman IgG; Santa Cruz Biotechnology, Santa Cruz, CA) for 20 min. After washing with TBS-T five times, Immobilon Western Chemiluminescent Substrate (Merck KGaA) was added to the membranes, and luminescence was detected using LuminoGraph II (Atto, Tokyo, Japan), as previously described (30, 44, 50).
Amplified luminescence proximity homogeneous assay-linked immunosorbent assay (AlphaLISA) was employed to measure the serum anti-CSF2 antibody (s-CSF2-Ab) and serum anti-bCSF2-77-peptide antibody (s-CSF2pep-Ab) levels. The reaction mixture containing 2.5 μL of serum samples diluted at 1:100 in AlphaLISA buffer (25 mM HEPES pH 7.4, 0.1% casein, 0.5% Triton X-100, 1 mg/mL of dextran-500, and 0.05% Proclin-300) and 2.5 μL of GST or GST-CSF2 proteins (10 μg/ml) or 400 ng/mL of bCSF2-77 was incubated in 384-well white opaque OptiPlate microtiter plates (PerkinElmer, Beaconsfield, United Kingdom) at room temperature for 6–8 h. Next, 2.5 μL of anti-human immunoglobulin G (IgG)-conjugated acceptor beads (40 μg/ml) and 2.5-μL glutathione-conjugated donor beads (40 μg/ml) or 2.5 μL of streptavidin-conjugated donor beads (40 μg/ml) were added. After incubation in the dark for 7–28 days at room temperature, chemical emission was measured using an EnSpire Alpha microplate reader (PerkinElmer), as previously described (48, 51, 52). Specific reactions were calculated by subtracting the emitted alpha photon counts of the GST and buffer controls from those of the GST-CSF2 protein and the bCSF2-77 peptide, respectively.
The longitudinal correlation between plasma s-CSF2pep-Ab levels and AIS in the Japan Public Health Center (JPHC)-based prospective study was investigated using AlphaLISA. The study nested within the JPHC cohort (53, 54) included approximately 30,000 Japanese individuals aged 40–69 years at the baseline period of 1990–1994 with stored plasma samples. The s-CSF2pep-Ab levels were measured in 202 cases who were included in the cohort between the baseline period and 2008 and in 202 controls whose sex, age (within 2 years), date of blood sampling (within 3 months), time since the last meal (within 4 h), and study location were matched with the cases. We used conditional logistic regression model to estimate the odds ratios (ORs) with 95% confidence intervals (CIs) for estimating the risk of AIS based on s-CSF2pep-Ab levels.
LoVo cells, a human CRC line expressing wild-type p53 (55), and DLD1 cells, a human CRC line expressing mutant p53 (56), were cultured at 37°C in a humidified atmosphere containing 5% CO2. LoVo cells were cultured in Ham’s F-12 (Ham’s F-12, NACALAI TESQUE, Inc., Kyoto, Japan) supplemented with 10% heat-inactivated fetal bovine serum (Thermo Fisher Scientific, Waltham, MA) and 100 μg/ml of kanamycin (Meiji Pharmaceutical, Kyoto, Japan). DLD1 cells were cultured in Dulbecco’s Modified Eagle’s Medium (NACALAI TESQUE) and supplemented with 10% fetal bovine serum and 100 μg/ml of kanamycin.
The effects of CSF2 and s-CSF2-Ab on the proliferation and viability of LoVo and DLD1 cells were evaluated using 3-(4,5-dimethylthiazol-2-yl)-5-(3-carboxymethoxyphenyl)-2-(4-sulfophenyl)-2H-tetrazolium (MTS, Promega, Madison, WI) assay, as previously reported (57, 58). Briefly, the cells were seeded into 96-well plates at a density of 2 × 103 cells/well. Next, rabbit anti-CSF2 antibody (10 μg/ml; Cusabio Technology, Houston, TX), CSF2 (1 μg/ml; PeproTech, Rocky Hill, NJ), or anti-CSF2 antibody (10 μg/ml) plus CSF2 (1 μg/ml) was added, and the plates were incubated for 72 h at 37°C in a humidified atmosphere containing 5% CO2. For the MTS assay, 20 μl of the 20:1 MTS (1.9 mg/ml)-PMS (phenazine methosulfate, Promega; 44 μg/ml) dye solution (CellTiter 96 AQueous Nonradioactive Cell Proliferation Assay, Promega) was directly added to each well, and the cells were incubated for 3–4 h. The absorbance at 490 nm was measured using a microplate reader (Emax, Molecular Devices, Sunnyvale, CA), and the data were expressed as relative cell viability ratios, i.e., absorbance of each experimental group versus the drug-free control.
LoVo cells (1 × 105 cells/well) seeded into 24-well plates were transfected with pCMV-p53WT plasmid (0.5 μg), a plasmid expressing wild-type p53, together with pGL3-p21-Luc (0.1 μg), a firefly luciferase reporter plasmid containing the promoter region of p21/Cip1, and pRL-SV40 (0.01 μg; Promega), a control Renilla luciferase reporter plasmid, using Lipofectamine Plus (Thermo Fisher Scientific). pCMV-p53WT was generously provided by Dr. Bert Vogelstein (Howard Hughes Medical Institute), and pGL3-p21-Luc (59) was kindly provided by Dr. Mian Wu (University of Science and Technology of China; 60). After 24 h of incubation, ultrapure water, rabbit anti-CSF2 antibody (10 μg/ml), or CSF2 (1 μg/ml) was added to each well. Two days after the transfection, firefly and Renilla luciferase activities were determined using the Dual-Luciferase Assay System (Promega) and a luminescence reader (Atto, Tokyo, Japan), as previously reported (49, 60). The firefly luciferase activity was normalized to the luciferase activity of the Renilla control plasmid.
LoVo cells were treated with 1 μg/ml of CSF2 or 10 μg/ml of anti-CSF2 antibody for 4 h, followed by washing with phosphate-buffered saline. The cells were lysed in 2% SDS, 10% glycerol, 50-mM Tris-Cl (pH 6.8), 0.01% bromophenol blue, and 1% β-mercaptoethanol. The lysates were incubated at 100°C for 3 min, sonicated for 10 min, separated on 9% SDS–polyacrylamide gels, and transferred to nitrocellulose membranes. The following primary antibodies were used with 1:1000 dilution in TBS-T: rabbit anti-Ser-46 phospho-specific p53 antibody (sc-101,764, Santa Cruz Biotechnology), rabbit anti-Ser-392 phospho-specific p53 antibody (sc-7,997-R, Santa Cruz Biotechnology), mouse anti-Ser-315 phospho-specific p53 antibody (K0059-3, Medical & Biological Laboratories, Nagoya, Japan), rabbit anti-Thr-81 phospho-p53 antibody (2,676, Cell Signaling Technology, Danvers, MA), and rabbit anti-β-actin antibody (sc-8,432, Santa Cruz Biotechnology).
The Mann–Whitney U test and Student’s t-test (two-sided) were employed to determine significant differences between the two groups, and the Kruskal–Wallis test (Mann–Whitney U with Bonferroni’s correction applied) was employed to evaluate the differences among three or more groups. Correlations were determined using Spearman’s correlation and logistic regression analyzes. All statistical analyzes were conducted using GraphPad Prism 5 (GraphPad, San Diego, CA) and the EZR software (61). Western blotting analysis was conducted using the ImageJ software (NIH, Bethesda, MD). A repeated-measured analysis of variance (ANOVA) with Tukey’s post hoc comparisons was performed on the MTS assay results and luciferase reporter assay results. The predictive values of the putative disease markers were evaluated using receiver operating characteristic (ROC) curve analysis, and the cutoff values were set to maximize the sums of sensitivity plus specificity. Furthermore, the analyzes of patient survival were evaluated using the Kaplan–Meier method and compared using the log-rank test. All tests were two-tailed, and P values <0.05 were considered to indicate statistically significant differences.
We performed western blotting to confirm the presence of serum autoantibodies against CSF2 in the sera of patients with AIS, DM, EC, and CRC. As presented in Figure 1, the recombinant GST-CSF2 protein reacted with the commercial anti-GST and anti-CSF2 antibodies, whereas the control GST protein reacted with the anti-GST but not with the anti-CSF2 antibody. The GST-CSF2 protein was also recognized by serum IgG antibodies from patients with AIS (#07544 and #07684), DM (#22226), EC (#EC-6), and CRC (#Co-58) but not by the sera of the HD (#09101). To avoid contingency, we performed western blotting for more serum samples. The GST-CSF2 protein was also recognized by serum IgG antibodies from patients with AIS (#007303, #07344, and #07684), TIA (#02291, #07278, and #07642), DM (#22297, #22370, and #22375), EC (#EC-3, #EC-4, and #EC-6), and CRC (#Co-3, #Co-5, and #Co-12), but not by the sera of the HDs (#09101, #07547, and #07572; Supplementary Figure S2). And the GST protein alone did not demonstrate observable reactivity with any of these serum samples (data not shown).
Figure 1. Detection of serum antibodies against CSF2. Presence of antibodies against CSF2 in the serum samples of patients with acute ischemic stroke (AIS), diabetes mellitus (DM), esophageal cancer (EC), and colorectal cancer (CRC). Purified GST-CSF2 (lane 1) and GST (lane 2) proteins were separated on sodium dodecyl sulfate–polyacrylamide gels, followed by staining with Coomassie Brilliant Blue (CBB) or western blotting using anti-GST (αGST), anti-CSF2 (αCSF2), and serum samples from patients with AIS (07544, 07684), DM (22226), EC (EC-6), and CRC (Co-58) and from a healthy donor (HD)(09101). The full, non-adjusted image of Figure 1 is shown in the Supplementary Figure S1. Molecular weights are presented in the left lane. Arrows indicate the positions of GST-CSF2 (44.6 kDa) and GST (26 kDa). CSF2, colony-stimulating factor 2.
Next, we examined the s-CSF2-Ab levels in HDs and patients with AIS and TIA using AlphaLISA. As presented in Figure 2A, the s-CSF2-Ab levels were significantly higher in patients with AIS than in HDs (Figure 2A). Similarly, the s-CSF2pep-Ab levels were significantly higher in patients with AIS and TIA than in HDs (Figure 2B). Based on the cutoff s-CSF2-Ab level, defined as two standard deviations (SDs) above the average s-CSF2-Ab level in HDs, the s-CSF2-Ab positivity rates were 5.5% in HDs and 12.2% in patients with AIS (Table 1). Using the same approach to define the cutoff s-CSF2pep-Ab level, the rates of s-CSF2pep-Ab positivity were 5.6, 18.1, and 10.9% in HDs and in patients with AIS and TIA, respectively. Furthermore, the ROC analysis revealed that the areas under the curve (AUCs) for s-CSF2-Ab vs. AIS, s-CSF2pep-Ab vs. AIS, and s-CSF2pep-Ab vs. TIA were 0.576, 0.658, and 0.647, respectively (Figures 2C–E), suggesting that the s-CSF2pep-Ab level was more closely associated with AIS than the s-CSF2-Ab level.
Figure 2. Comparison of the s-CSF2-Ab and s-CSF2pep-Ab levels in patients with AIS and TIA and in HDs. (A–B) Levels of serum antibodies against (A) s-CSF2-Ab and (B) s-CSF2pep-Ab determined using amplified luminescence proximity homogeneous assay-linked immunosorbent assay (AlphaLISA), calculated by subtracting the levels of antibodies against the control GST. Scatter dot plots of the s-CSF2-Ab and s-CSF2pep-Ab levels are also presented. Bars represent averages ± standard deviation (SD). **P < 0.05; *P < 0.01; ns, not significant, Kruskal–Wallis test. (C–E) A receiver operating characteristic (ROC) curve analysis was conducted to assess the ability of s-CSF2-Ab to detect AIS (C) and that of s-CSF2pep-Ab to detect AIS (D) and TIA (E). The numbers indicate the cutoff values for the indicated markers, and the numbers in parentheses indicate sensitivity (left) and specificity (right). The areas under the ROC curve (AUC) and 95% confidence intervals (CIs) are also shown. AIS, acute ischemic stroke; HD, healthy donor; CSF2-Ab, CSF2 antibody; CSF2pep-Ab, CSF2 peptide antibody; TIA, transient ischemic attack.
Table 1. Comparison of the s-CSF2-Ab and s-CSF2pep-Ab levels in HDs versus those in patients with AIS and TIA.
Next, we measured the s-CSF2-Ab and s-CSF2pep-Ab levels in patients with AMI and DM. The average (±SD) ages of HDs and patients with AMI and DM were 58.29 ± 5.63, 58.20 ± 8.50, and 58.48 ± 9.17 years, respectively (Table 2). The s-CSF2-Ab and s-CSF2pep-Ab levels were significantly higher in patients with AMI and DM than in HDs (Figures 3A,B). Based on the cutoff s-CSF2-Ab and s-CSF2pep-Ab levels, defined as two SDs above the average levels in HDs, the rates of s-CSF2-Ab and s-CSF2pep-Ab positivity were 2.3 and 1.6% in HDs, 10.2 and 11.7% in patients with AMI, and 13.3 and 10.2% in patients with DM, respectively (Table 2). The ROC analysis revealed that the AUCs for s-CSF2-Ab were 0.650 and 0.638 for AMI and DM, respectively. Similarly, the ROC analysis revealed that the AUCs for s-CSF2pep-Ab were 0.785 and 0.740 for AMI and DM, respectively. The higher AUCs for both s-CSF2-Ab and s-CSF2pep-Ab in AMI and DM than in AIS and TIA (Figures 3D–G) suggested that s-CSF2-Ab and s-CSF2pep-Ab might be more closely associated with AMI and DM than with AIS and TIA.
Figure 3. Comparison of the s-CSF2-Ab and s-CSF2pep-Ab levels between HDs and patients with AMI and DM. (A,B) The levels of s-CSF2-Ab and s-CSF2pep-Ab in HDs and patients with acute myocardial infarction (AMI) (A) and diabetes mellitus (DM) (B) were determined using AlphaLISA. The bars represent averages ± SD. *** P < 0.001, Mann–Whitney U test. (D,E) The ROC curves to assess the ability of s-CSF2-Ab to predict AMI and DM are presented in (D) and (E), respectively. (F,G) The ability of s-CSF2pep-Ab to predict AMI (E) and DM (F) was also evaluated via ROC analysis. Comparison of the overall survival in patients with DM between the s-CSF2-Ab positive (s-CSF2-Ab+) and s-CSF2-Ab negative (s-CSF2-Ab−) groups (P = 0.019; C) and between the s-CSF2pep-Ab positive (s-CSF2-Ab+) and s-CSF2pep-Ab negative (s-CSF2-Ab−) groups (P = 0.034; C). Statistical analyzes were performed by the Log-Rank test between two groups.
The patients with DM, who were followed up for 100 months after serum collection, were categorized into positive and negative groups based on the cutoff s-CSF2-Ab and s-CSF2pep-Ab levels determined using the ROC analysis (Figures 3E,G). Albeit not correlated with age, sex, obesity, and the onset of cerebrovascular events or cancer, the s-CSF2pep-Ab positivity was significantly correlated with the onset of cardiovascular events (Supplementary Table S1). Furthermore, both the s-CSF2-Ab-and s-CSF2pep-Ab-positive groups had poor DM prognosis compared with the antibody-negative groups (P = 0.019 and P = 0.034, respectively; Figures 3C,H). Importantly, the difference in the overall survival between the antibody-positive and antibody-negative groups was most striking after 80 months.
We also examined the s-CSF2-Ab and s-CSF2pep-Ab levels in the sera of 82 HDs and 300 patients with CKD, including 145 patients with diabetic kidney disease (CKD type 1), 32 patients with nephrosclerosis (CKD type 2), and 123 patients with glomerulonephritis (CKD type 3). The s-CSF2-Ab and s-CSF2pep-Ab levels were significantly higher in patients with CKD types 1, 2, and 3 than in HDs (Figures 4A,B). Based on the cutoff antibody levels, defined as two SDs above the average levels in HDs, the s-CSF2-Ab positivity rates were 4.8, 22.1, 28.1, and 22%, and the s-CSF2pep-Ab positivity rates were 1.2, 18.6, 21.9, and 14.6% in HDs and patients with CKD types 1, 2, and 3, respectively (Table 3). The ROC analysis revealed that the AUCs for s-CSF2-Ab were 0.644, 0.683, and 0.593 (Figures 4C–E), and the AUCs for s-CSF2pep-Ab were 0.657, 0.669, and 0.631 (Figures 4F–H) in patients with CKD types 1, 2, and 3, respectively. The AUC for the s-CSF2-Ab level was higher in patients with CKD type 2 than in those with CKD type 1.
Figure 4. Association of the s-CSF2-Ab and s-CSF2pep-Ab levels with CKD. The levels of s-CSF-Ab (A) and s-CSF2pep-Ab (B) in HDs and in patients with diabetic CKD (CKD type 1), nephrosclerosis (CKD type 2), and glomerulonephritis (CKD type 3) were examined using AlphaLISA. The bars represent averages ± SD. *P < 0.05; **P < 0.01; ***P < 0.001, Kruskal–Wallis test. The data are summarized in Table 3. (C–E) ROC analysis to determine the ability of s-CSF2-Ab to predict CKD type 1 (C), type 2 (D), and type 3 (E). (F–H) ROC analysis to determine the ability of s-CSF2pep-Ab to predict CKD type 1 (F), type 2 (G), and type 3 (H). The numbers in the graphs are the same as those presented Figure 2. CKD, chronic kidney disease.
We conducted a comparative analysis of the s-CSF2-Ab levels and participant characteristics in a cohort of 384 participants from Chiba Prefectural Sawara Hospital, including 196 patients with AIS, 79 patients with TIA, and 109 HDs. The baseline characteristics of the cohort are summarized in Supplementary Table S2. The Mann–Whitney U test was employed to compare the s-CSF2-Ab levels between males and females; obese or not (body mass index, < or ≥ 25 kg/m2); those with (+) or without (−) another disease including DM, hypertension, CVD, and dyslipidemia; and those with or without smoking and alcohol intake habits. Significantly higher s-CSF2-Ab levels were observed in patients with hypertension than in those without hypertension (P = 0.020; Table 4). Contrarily, the s-CSF2-Ab levels were inversely associated with dyslipidemia (P = 0.027) possibly because the patients but not the HDs were taking cholesterol-lowering drugs, such as statins. However, s-CSF2-Ab showed lower levels in participants with CVD, probably because only 15 positive cases with CVD complications were compared with 369 negative cases with CVD complications (P = 0.976).
Table 4. Association between s-CSF2-Ab levels and the data from participants in the Sawara Hospital cohort.
Furthermore, Spearman’s rank-order correlation analysis was conducted to determine the correlation between the serum antibody levels and participant characteristics, including general information (e.g., age, body height, weight, body mass index, and degree of artery stenosis) and blood tests (sodium, chloride, white and red blood cell counts, hematocrit, etc.). The s-CSF2-Ab levels were significantly correlated with age, blood pressure (BP), smoking duration, and max-IMT (Table 5). However, the s-CSF2-Ab levels were inversely correlated with the levels of total cholesterol, cholinesterase, and albumin. The observed correlation with max-IMT suggested that s-CSF2-Ab levels were associated with early atherosclerosis prior to stenosis (62–64).
Table 5. Correlation analysis of s-CSF2-Ab levels with data from subjects in the Sawara Hospital cohort.
Spearman’s rank-order correlation analysis of the CKD cohort consisting of 300 participants also revealed a significant correlation with plaque score, max-IMT, and cardio-ankle vascular index (left and right; Supplementary Table S3), which reflect the degree of atherosclerosis (65). Furthermore, C-reactive protein was associated with the s-CSF2-Ab levels in the CKD cohort, supporting the role of s-CSF2-Ab in inflammation in CKD.
Logistic regression analysis was conducted to determine the predictors of AIS in the Chiba Prefectural Sawara Hospital cohort (Supplementary Table S2). The univariate logistic regression analysis revealed that elevated s-CSF2-Ab levels were associated with increased risk of AIS (P < 0.0001). Moreover, the multivariate logistic regression analysis demonstrated that age, hypertension, and s-CSF2-Ab level were independent predictors of AIS (Supplementary Table S4).
To determine whether s-CSF2pep-Ab can be used as a marker to predict the onset of AIS, we examined the JPHC cohort samples, which were obtained as previously described (30). The s-CSF2pep-Ab levels were strongly associated with the risk of AIS. The ORs (95% CI) were 1.72 (0.91–3.24), 2.47 (1.31–4.65), and 3.03 (1.58–5.80) for the samples with the second, third, and fourth quartiles of the s-CSF2pep-Ab levels, respectively, compared with the first quartile (Table 6). Consistently, the s-CSF2pep-Ab levels were significantly higher in patients with TIA (Figure 2E), which can be a prodromal stage of AIS (66), compared with HDs. These results indicated that s-CSF2pep-Ab might be a useful marker in predicting the onset of AIS.
Based on previous reports suggesting that CSF2 could inhibit the development of cancer (13–15, 67), we further investigated the serum samples from patients with EC, CRC, GC, LC, and MC. The s-CSF2-Ab levels were significantly higher in patients with EC, CRC, GC, and LC, but not in those with MC, compared with HDs (Figure 5; Table 7). The highest rates of s-CSF2-Ab positivity were observed in patients with EC and CRC (Table 7). The AUCs for s-CSF2-Ab were 0.816 and 0.714 versus EC and CRC, respectively (Figures 5B,D), which were much higher than versus AIS (0.576), AMI (0.650), DM (0.638), type-1 CKD (0.644), type-2 CKD (0.683), type-3 CKD (0.593), GC (0.659), and LC (0.636; Figures 2C, 3D,E, 4C–E, 5B–E). This may indicate that s-CSF2-Ab levels are more closely associated with EC and CRC compared to others.
Figure 5. Comparison of the s-CSF2-Ab levels between HDs and patients with cancers. (A) Scatter dot plots of the s-CSF2-Ab levels in HDs and in patients with EC, gastric cancer (GC), CRC, lung cancer (LC), and mammary cancer (MC). The results are presented as described in the legend of Figure 2. **P < 0.01; ***P < 0.001; ns, not significant. ROC analysis was conducted to assess the ability of s-CSF2-Abs to detect EC (B), GC (C), CRC (D), and LC (E).
The patients with CRC were divided into the positive and negative groups based on the cutoff s-CSF2-Ab levels determined using the ROC analysis (Figure 5D) to evaluate the association of the s-CSF2-Ab levels with postoperative survival and all of whom underwent radical surgery. The overall survival was significantly different between the s-CSF2-Ab-positive and s-CSF2-Ab-negative patients with CRC (P = 0.011; Figure 6A). Moreover, we analyzed the correlation between s-CSF2-Ab and the age or the stage in patients with CRC. The result revealed that the s-CSF2-Ab was independent of the age or the stage (Supplementary Table S5). The comparison of the overall survival in patients with CRC categorized according to serum p53-Ab positivity (i.e., serum p53-Ab ≥1.3 IU/ml) did not reveal a significant difference (P = 0.952; Figure 6B).
Figure 6. Comparison of survival according to s-CSF2-Ab positivity in patients with CRC after surgery. (A) Comparison of the postoperative survival between the s-CSF2-Ab+ and s-CSF2-Ab− patients with CRC (P = 0.011). (B) Comparison of the overall survival between the p53-Ab+ (≥1.3 U/ml) and p53-Ab− (<1.3 U/ml) patients with CRC (P = 0.952). (C) Comparison of the overall survival between the s-CSF2-Ab+ and s-CSF2-Ab− patients with CRC among those with p53-Ab ≥1.3 U/ml (P = 0.463). (D) Comparison of the overall survival between the s-CSF2-Ab+ and s-CSF2-Ab− patients with CRC among those with p53-Ab <1.3 U/ml (P = 0.004). The cutoff values in patients with CRC were determined via the ROC analysis presented in Figure 5D. Statistical analyzes were performed by the Log-Rank test between two groups.
The correlation analysis of patients with CRC indicated that the s-CSF2-Ab levels were associated with the serum p53-Ab level (P = 0.039) but not with the serum CEA or CA19-9 levels (Table 8), suggesting a degree of functional relevance between the serum CSF2 and p53 levels. Next, we categorized the patients with CRC into p53-Ab-positive and p53-Ab-negative populations. Within the p53-Ab-negative group, the prognosis of the s-CSF2-Ab-positive patients was significantly worse than that of the s-CSF2-Ab-negative patients (P = 0.004), which was not observed within the p53-Ab-positive group (Figures 6C,D). Serum p53-Ab-negative cancers may be maintaining functional wild-type p53; therefore, these results raised the possibility that s-CSF2-Ab and CSF2 could influence the development of CRC via wild-type p53. Of note, the overall survival based on the s-CSF2-Ab levels did not exhibit significant differences in patients with EC and GC (Supplementary Figures 3A,B).
Table 8. Association between s-CSF2-Ab levels and data from participants in the Toho University Hospital cohort.
We evaluated the effects of the CSF2 and anti-CSF2 antibodies on cell replication using LoVo cells, a human CRC line harboring wild-type p53 (55). LoVo cells were treated with CSF2 and/or a commercial rabbit polyclonal anti-CSF2 antibody (Cusabio Technology) to examine their impact on cell replication using the MTS assay. As presented in Supplementary Figure S4, treatment with CSF2 for 72 h led to a slight decrease in cell replication, whereas the presence of the anti-CSF2 antibody significantly promoted the proliferation of LoVo cells. Moreover, the replication-promoting effect of the anti-CSF2 antibody was reversed by the simultaneous addition of an excess quantity of antigenic CSF2 (PeproTech), suggesting that the stimulation of proliferation by the anti-CSF2 antibody was attributed mainly to the suppression of CSF2 in the medium and not to other effects due to impurities. Contrarily, none of the effects of CSF2 and the anti-CSF2 antibody observed in LoVo cells were detected in DLD1 harboring mutant p53 (Supplementary Figure S4).
Next, we used pGL3-p21-Luc containing the promoter sequence of p21/Cip1, a p53-target gene, to evaluate the p53 activity after the addition of CSF2 and/or the anti-CSF2 antibody using a luciferase reporter assay. In LoVo cells, p21-Luc, which was partially preactivated by cotransfection of wild-type p53, was further activated by treatment with CSF2 but significantly attenuated by treatment with the anti-CSF2 antibody (P < 0.01; Supplementary Figure S5). The decrease in the activation of p21-Luc by the anti-CSF2 antibody was reversed by the concurrent addition of excess antigenic CSF2, indicating that the reduction of p21-Luc activation by the anti-CSF2 antibody was caused by the suppression of CSF2 in the medium rather than its effects related to other impurities in the CSF2 antibody preparation. Therefore, these results indicate that CSF2 might induce the p53 transactivation ability, leading to the inhibition of tumor cell proliferation.
To determine p53-mediated transactivation, we measured the phosphorylation of p53 at Ser-46 using western blotting (68). The levels of Ser-46-phosphorylated p53 were reduced after treatment with the rabbit anti-CSF2 antibody and increased after treatment with CSF2 (Supplementary Figure S6). No apparent changes were observed in the levels of p53 phosphorylated at other sites, including Ser-392, Ser-315, and Thr-81 (data not shown). Thus, these results indicated that the rabbit anti-CSF2 antibody and the serum autoantibodies against CSF2 promoted tumor progression by suppressing p53 activity, which was preactivated by endogenous CSF2.
In the present study, we investigated the relationship between CSF2 and atherosclerosis-related diseases, including AIS, AMI, DM, CKD, and cancer based on data from previous studies. We confirmed the presence of anti-CSF antibodies in patient sera using western blotting with recombinant full-length GST-tagged CSF2 (Figure 1). Subsequently, we measured the serum antibody levels using GST-CSF2 and the N-terminal biotinylated 18-mer peptide, bCSF2-77, as antigens in AlphaLISA. We found that both the s-CSF2-Ab and s-CSF2pep-Ab levels were higher in patients with AIS, AMI, DM, and CKD than those with HDs (Figures 2, 3, 5; Tables 1–3). The s-CSF2-Ab levels were closely correlated with max-IMT, plaque score, and cardio-ankle vascular index (Table 5; Supplementary Table S3), which are typical indices of atherosclerosis leading to AIS and AMI (62–65). CSF2 has been demonstrated to have a protective impact on atherogenesis (6), and the administration of CSF2 has been reported to prevent the progression of atherosclerosis via changes in the composition of atherosclerotic lesions (7). We observed that both the s-CSF2-Ab and s-CSF2pep-Ab levels were highly correlated with atherosclerosis-related diseases, such as AIS, AMI, CKD, and DM. Intriguingly, we also found that the levels of s-CSF2pep-Ab but not s-CSF2-Ab were associated with TIA. It is possible that the CSF2 peptide with a single epitope can be specifically recognized by anti-CSF2 antibodies, whereas the CSF2 protein with multiple epitopes can also cross-react with other non-specific antibodies in addition to being recognized by specific anti-CSF2 antibodies. Furthermore, we found that the AUC of s-CSF2pep-Ab in patients with AIS, AMI, DM, and CKD were all higher than the AUC of s-CSF2-Ab in patients, except for type-2 CKD. Consistent with this, it was reported that using peptide epitopes would be beneficial with respect to assay specificity, while recombinant proteins also included many cross-reactive epitopes that would react with low specificity antibodies, leading to a lower specificity of the test (48, 69). Therefore, the use of s-CSF2pep-Ab as an antibody marker may be more accurate for the diagnosis of diseases. The s-CSF2-Ab levels were correlated well with hypertension (Table 4) and weakly with BP (Table 5) but were not correlated with glycated hemoglobin and blood glucose, which are typical DM markers. Patients with diabetic CKD (CKD type 1) and those with nephrosclerosis (CKD type 2) exhibited equally higher s-CSF2-Ab and s-CSF2pep-Ab levels than the HDs (Figures 4A, B). The AUCs of CKD type 2 were higher than those of CKD type 1. These results indicate that the s-CSF2-Ab levels do not directly reflect DM but are associated with DM-induced atherosclerotic disorders. Furthermore, using multivariate logistic regression analysis, we identified age, hypertension, and s-CSF2-Ab as independent predictors of AIS (Supplementary Table S4). Thus, s-CSF2-Ab may be able to discriminate atherosclerosis caused by hypertension, which leads to the onset of AIS and AMI. These findings, subsequently validated by the analysis of the JPHC cohort (Table 6), indicate that s-CSF2-Ab should be considered as a risk factor for AIS.
In addition, the s-CSF2-Ab levels were associated with several cancers, including EC, CRC, GC, and LC (Figure 5; Table 7). As presented in Table 8, the s-CSF2-Ab and p53-Ab levels were correlated. Among the patients with CRC whose serum p53-Ab levels were below the cutoff, the prognosis was worse in those with high s-CSF2-Ab levels than in those with low s-CSF2-Ab levels. Furthermore, the P value of Figure 6D was even lower than that of Figure 6A in the survival analysis of patients with CRC. After transactivation by the wild-type p53, the protein product of mouse double minute 2 homolog (MDM2) preferentially polyubiquitinates p53 protein, leading to its degradation (68, 70). Contrarily, in the presence of mutant p53, the MDM2 levels are maintained at low levels, and the degradation of p53 is limited, leading to the high p53 expression (71). Therefore, most of the anti-p53 autoantibodies in patients with CRC are formed in response to p53 mutations (72), and patients without p53-Ab harbor wild-type p53. Based on the observed effect of p53-Ab status, we used wild-type p53-harboring LoVo cells to investigate the relationship of CSF2 and CSF2-Ab with p53 (Supplementary Figures S4, S5). We found that anti-CSF2-Ab reduced the p53 transactivation ability, leading to the increased proliferation of LoVo cells (Supplementary Figures S4, S5). Consistently, the levels of Ser-46-phosphorylated p53 were increased by treatment with CSF2 and reduced by treatment with anti-CSF2-Ab (Supplementary Figure S6). Interestingly, it was previously reported that the anti-CSF2 antibodies decreased CSF2 cytokine bioavailability, possibly by disrupting their binding to receptors (73–75), and the expression of p53 was significantly increased in neutrophils cultured with CSF2 (76). These results indicate that the high levels of CSF2-Ab may reduce p53 activity via adsorption of CSF2 in the circulation, leading to an increase in the proliferation of cancer cells and unfavorable prognosis in patients with CRC (Figure 6D). Gene therapy using wild-type p53, delivered using physical methods or viral vectors, was reported to significantly suppress CRC (77), but not MC (78), in preclinical and clinical models. Therefore, CRC might be more sensitive to the tumor suppressor p53 than MC, which might partially explain our findings that the s-CSF2-Ab levels were not associated with MC (Figure 5; Table 7).
Numerous lines of evidence support the presence of a relationship between atherosclerosis and cancer, which share inflammation as an underlying pathophysiology (11, 12). p53 deficiency not only leads to accelerated atherosclerosis (79–81) but also increases BP, heart rate, and sympathetic activity of the heart and blood vessels, thus contributing to the development of hypertension (82). In the present study, we found that the s-CSF2-Ab levels were significantly associated with hypertension, which is a major risk factor for not only atherosclerosis (9, 83–85) but also AIS and AMI (35, 86–88). Therefore, based on the current study findings, we hypothesize that the s-CSF2-Ab levels may contribute to atherosclerosis through the elevated hypertension caused by reduced p53 activity. Therefore, based on the current study findings, we hypothesize that the s-CSF2-Ab may directly contribute to the development of cancer by reducing p53 activity, and indirectly contribute to the onset of atherosclerosis-related AIS, AMI, and CKD through p53-mediated regulation of hypertension. This may explain the overall higher positive rate of s-CSF2-Ab in patients with solid cancer than those in patients with atherosclerosis-related AIS, AMI, DM, and CKD (Tables 1–3, 7). The overview diagram (Figure 7) summarizes the relationship between s-CSF2-Ab and wild-type p53 in cancer and atherosclerosis-related diseases. Consistently, the high s-CSF2-Ab levels in patients with DM indicated unfavorable prognosis, which was especially pronounced after 80 months (Figures 3C,H). Thus, s-CSF2-Ab might have a causal relationship with the development of atherosclerosis and cancer.
Figure 7. Diagram of the relationship between s-CSF2-Ab and p53 in cancer and atherosclerosis-related diseases. Arrows represent the downstream activation, and blocked arrows represent inhibitory action. Created with BioRender.com. MDM2, mouse double minute 2 homolog.
Results of this study suggest that the measurement of serum s-CSF2-Ab levels can provide valuable information for diagnosing atherosclerosis-related AIS and solid cancers. The serum s-CSF2pep-Ab levels can be used for diagnosing TIA. Investigations showed that approximately 15% of patients with ischemic stroke experience a TIA before the onset of AIS (89), and approximately 50% of the patients with TIA visited medical facilities after their symptoms completely disappeared (90, 91). However, it is sometimes difficult for physicians to diagnose a TIA only by taking the patient’s history into consideration, as half of the TIA patients visit the hospital after the symptoms have completely disappeared. Although tests such as MRI and carotid artery angiography can be used to improve diagnoses, they are generally expensive, time consuming, and inconvenient. In the case of solid cancers, early diagnoses can greatly improve the survival time of patients and reduce the financial burden caused by medical treatments. Therefore, if s-CSF2pep-Ab can be applied as a biomarker in combination with other risk factors and biomarkers for AIS, TIA or solid cancers to diagnose the occurrence of the disease, this will greatly facilitate clinical practice and contribute to the medical economy. Cytokines and cytokine antibodies in the serum are the ideal targets for the development of preventative and therapeutic approaches due to drug accessibility. The administration of CSF2 has been demonstrated to prevent the progression of atherosclerosis (7). Alternatively, the development decoy molecules that can bind to and adsorb s-CSF2-Ab might be effective for disease prevention.
We acknowledge that the current study has several limitations. First, atherosclerosis risk factors, such as hypercholesterolemia, were not associated with the elevated levels of s-CSF2-Ab in the present study. Antihypertensive drugs, cholesterol-lowering statins, and antiplatelet agents are considered to influence the pathogenesis of atherosclerosis (92–95). Because detailed statistics on medications taken by patients prior to hospital admission are not available, we were unable to study the specific effects of drugs on s-CSF2-Ab levels, and further studies are needed in patients who were not taking drugs that could affect atherosclerosis and cancer. In future studies, the potential modulating effects of these drugs on the s-CSF2-Ab levels should be considered. Second, the blood samples were collected at the time of hospital admission and the period of stroke onset and admission differed depending on the patient, which is another limitation. Third, as we used specimens obtained from hospitals and universities in Japan, it is unclear whether our conclusions are applicable to other populations. Thus, it is essential for general practical use in the world to conduct more international collaborative research using specimens from many countries. Fourth, the cutoff values we used were average+2SD and Youden index of ROC analysis. However, other methods should also be considered to optimize the cutoff values, improving the ability of s-CSF2-Ab as a biomarker to discriminate normal individuals from patients and favorable prognosis from unfavorable prognosis.
It is possible to predict the onset of atherosclerotic AIS and AMI by using s-CSF2-Ab as a marker. Moreover, high s-CSF2-Ab levels exhibited poor overall survival in patients with CRC who may be harboring wild-type p53. Taken together, these results indicate s-CSF2-Ab as a potentially valuable therapeutic target for the prevention of atherosclerosis and solid cancers.
The original contributions presented in the study are included in the article/Supplementary material, further inquiries can be directed to the corresponding authors.
The studies involving human participants were reviewed and approved by Local Ethics Review Board of Chiba University, Graduate School of Medicine in Chiba, Japan (no. 2018–320), Toho University Graduate School of Medicine in Tokyo, Japan (no. A19033). The patients/participants provided their written informed consent to participate in this study.
SYL, TMac, YI, and TH conceived and designed the study. SYL, MK, TMac, KYa, MO, MT, and KI conducted the experiments and acquired the data. YY, SM, MI, KYos, AH, KYok, HI, KK, NS, ST, and HT contributed to the reagents, materials, analysis tools, or the patient data. YY, SY, MS, EN, and IK analyzed and interpreted the data. BSZ, TMat, MI, HS, and MT performed the statistical analyzes. SYL, YI, and TH drafted the manuscript. All authors contributed to the article and approved the submitted version.
This work was supported, in part, by research grants from the Japan Science and Technology Agency (JST), JSPS KAKENHI Grant Number 20 K17953, 19 K09451, 17 K19810, 20 K07810, 16 K10520, 21 K08695, and 15 K10117. The Japan Public Health Center-based Prospective Study was supported by (National Cancer Center Research and Development Fund since 2011) and a Grant-in-Aid for Cancer Research from the Ministry of Health, Labour and Welfare of Japan (from 1989 to 2010).
The authors would like to thank Masaki Takiguchi (Department of Biochemistry and Genetics, Graduate School of Medicine, Chiba University) for valuable discussions. We are grateful to Bert Vogelstein (Howard Hughes Medical Institute) and Mian Wu (University of Science and Technology of China) for providing expression and reporter plasmids.
The authors declare that the research was conducted in the absence of any commercial or financial relationships that could be construed as a potential conflict of interest.
All claims expressed in this article are solely those of the authors and do not necessarily represent those of their affiliated organizations, or those of the publisher, the editors and the reviewers. Any product that may be evaluated in this article, or claim that may be made by its manufacturer, is not guaranteed or endorsed by the publisher.
The Supplementary material for this article can be found online at: https://www.frontiersin.org/articles/10.3389/fcvm.2023.1042272/full#supplementary-material
1. Jiang, YX, Yang, SW, Li, PA, Luo, X, Li, ZY, Hao, YX, et al. The promotion of the transformation of quiescent gastric cancer stem cells by IL-17 and the underlying mechanisms. Oncogene. (2017) 36:1256–64. doi: 10.1038/onc.2016.291
2. Matsubara, T, Furukawa, S, and Yabuta, K. Serum levels of tumor necrosis factor, interleukin 2 receptor, and interferon-gamma in Kawasaki disease involved coronary-artery lesions. Clin Immunol Immunopathol. (1990) 56:29–36. doi: 10.1016/0090-1229(90)90166-n
3. Tong, Y, Song, Y, and Deng, S. Combined analysis and validation for DNA methylation and gene expression profiles associated with prostate cancer. Cancer Cell Int. (2019) 19:50. doi: 10.1186/s12935-019-0753-x
4. Qin, C, Liu, Q, Hu, ZW, Zhou, LQ, Shang, K, Bosco, DB, et al. Microglial TLR4-dependent autophagy induces ischemic white matter damage via STAT1/6 pathway. Theranostics. (2018) 8:5434–51. doi: 10.7150/thno.27882
5. Lebedeva, A, Fitzgerald, W, Molodtsov, I, Shpektor, A, Vasilieva, E, and Margolis, L. Differential clusterization of soluble and extracellular vesicle-associated cytokines in myocardial infarction. Sci Rep. (2020) 10:21114. doi: 10.1038/s41598-020-78004-y
6. Ditiatkovski, M, Toh, BH, and Bobik, A. GM-CSF deficiency reduces macrophage PPAR-gamma expression and aggravates atherosclerosis in ApoE-deficient mice. Arterioscler Thromb Vasc Biol. (2006) 26:2337–44. doi: 10.1161/01.Atv.0000238357.60338.90
7. Shindo, J, Ishibashi, T, Yokoyama, K, Nakazato, K, Ohwada, T, Shiomi, M, et al. Granulocyte-macrophage colony–stimulating factor prevents the progression of atherosclerosis via changes in the cellular and extracellular composition of atherosclerotic lesions in watanabe heritable hyperlipidemic rabbits. Circulation. (1999) 99:2150–6. doi: 10.1161/01.CIR.99.16.2150
8. Valdivielso, JM, Rodríguez-Puyol, D, Pascual, J, Barrios, C, Bermúdez-López, M, Sánchez-Niño, MD, et al. Atherosclerosis in chronic kidney disease: more, kess, or just different? Arterioscler Thromb Vasc Biol. (2019) 39:1938–66. doi: 10.1161/atvbaha.119.312705
9. Libby, P, Buring, JE, Badimon, L, Hansson, GK, Deanfield, J, Bittencourt, MS, et al. Atheroscler Nat Rev Dis Primers. (2019) 5:56. doi: 10.1038/s41572-019-0106-z
10. Agarwal, S, Sud, K, Thakkar, B, Menon, V, Jaber, WA, and Kapadia, SR. Changing trends of atherosclerotic risk factors among patients with acute myocardial infarction and acute ischemic stroke. Am J Cardiol. (2017) 119:1532–41. doi: 10.1016/j.amjcard.2017.02.027
11. Barrera, G, Pizzimenti, S, and Dianzani, MU. Lipid peroxidation: control of cell proliferation, cell differentiation and cell death. Mol Asp Med. (2008) 29:1–8. doi: 10.1016/j.mam.2007.09.012
12. Thanan, R, Oikawa, S, Hiraku, Y, Ohnishi, S, Ma, N, Pinlaor, S, et al. Oxidative stress and its significant roles in neurodegenerative diseases and cancer. Int J Mol Sci. (2014) 16:193–217. doi: 10.3390/ijms16010193
13. Zhang, J, Liu, Q, Qiao, L, Hu, P, Deng, G, Liang, N, et al. Novel role of granulocyte-macrophage colony-stimulating factor: antitumor effects through inhibition of epithelial-to-mesenchymal transition in esophageal cancer. Onco Targets Ther. (2017) 10:2227–37. doi: 10.2147/ott.S133504
14. Urdinguio, RG, Fernandez, AF, Moncada-Pazos, A, Huidobro, C, Rodriguez, RM, Ferrero, C, et al. Immune-dependent and independent antitumor activity of GM-CSF aberrantly expressed by mouse and human colorectal tumors. Cancer Res. (2013) 73:395–405. doi: 10.1158/0008-5472.Can-12-0806
15. Liu, H, Lin, C, Shen, Z, Zhang, H, He, H, Li, H, et al. Decreased expression of granulocyte-macrophage colony-stimulating factor is associated with adverse clinical outcome in patients with gastric cancer undergoing gastrectomy. Oncol Lett. (2017) 14:4701–7. doi: 10.3892/ol.2017.6738
16. Hong, IS. Stimulatory versus suppressive effects of GM-CSF on tumor progression in multiple cancer types. Exp Mol Med. (2016) 48:e242. doi: 10.1038/emm.2016.64
17. Albulescu, R, Codrici, E, Popescu, ID, Mihai, S, Necula, LG, Petrescu, D, et al. Cytokine patterns in brain tumour progression. Mediat Inflamm. (2013) 2013:979748. doi: 10.1155/2013/979748
18. Perez, FA, Fligner, CL, and Yu, EY. Rapid clinical deterioration and leukemoid reaction after treatment of urothelial carcinoma of the bladder: possible effect of granulocyte colony-stimulating factor. J Clin Oncol. (2009) 27:e215–7. doi: 10.1200/jco.2009.22.4931
19. Thomas, DS, Fourkala, EO, Apostolidou, S, Gunu, R, Ryan, A, Jacobs, I, et al. Evaluation of serum CEA, CYFRA21-1 and CA125 for the early detection of colorectal cancer using longitudinal preclinical samples. Br J Cancer. (2015) 113:268–74. doi: 10.1038/bjc.2015.202
20. Amoura, Z, Duhaut, P, Huong, DL, Wechsler, B, Costedoat-Chalumeau, N, Francès, C, et al. Tumor antigen markers for the detection of solid cancers in inflammatory myopathies. Cancer Epidemiol Biomark Prev. (2005) 14:1279–82. doi: 10.1158/1055-9965.Epi-04-0624
21. Shimada, H, Arima, M, Nakajima, K, Koide, Y, Okazumi, S, Matsubara, H, et al. Detection of serum p53 antibodies in mucosal esophageal cancer and negative conversion after treatment. Am J Gastroenterol. (1998) 93:1388–9. doi: 10.1111/j.1572-0241.1998.1388a.x
22. Shimada, H, Ochiai, T, and Nomura, F. Titration of serum p53 antibodies in 1,085 patients with various types of malignant tumors: a multiinstitutional analysis by the Japan p53 antibody research group. Cancer. (2003) 97:682–9. doi: 10.1002/cncr.11092
23. Mattioni, M, Soddu, S, Porrello, A, D'Alessandro, R, Spila, A, and Guadagni, F. Serum anti-p53 antibodies as a useful marker for prognosis of gastric carcinoma. Int J Biol Markers. (2007) 22:302–6. doi: 10.5301/jbm.2008.1415
24. Chu, DZ, Erickson, CA, Russell, MP, Thompson, C, Lang, NP, Broadwater, RJ, et al. Prognostic significance of carcinoembryonic antigen in colorectal carcinoma. Serum levels before and after resection and before recurrence. Arch Surg. (1991) 126:314–6. doi: 10.1001/archsurg.1991.01410270054010
25. Zhang, G, Xu, Q, Wang, Z, Sun, L, Lv, Z, Liu, J, et al. p53 protein expression affected by TP53 polymorphism is associated with the biological behavior and prognosis of low rectal cancer. Oncol Lett. (2019) 18:6807–21. doi: 10.3892/ol.2019.10999
26. Browne, SK. Anticytokine autoantibody–associated immunodeficiency. Annu Rev Immunol. (2014) 32:635–57. doi: 10.1146/annurev-immunol-032713-120222
27. Browne, SK, and Holland, SM. Immunodeficiency secondary to anticytokine autoantibodies. Curr Opin Allergy Clin Immunol. (2010) 10:534–41. doi: 10.1097/ACI.0b013e3283402b41
28. Palmer, JP, Asplin, CM, Clemons, P, Lyen, K, Tatpati, O, Raghu, PK, et al. Insulin antibodies in insulin-dependent diabetics before insulin treatment. Science. (1983) 222:1337–9. doi: 10.1126/science.6362005
29. Matsumura, T, Terada, J, Kinoshita, T, Sakurai, Y, Yahaba, M, Tsushima, K, et al. Circulating autoantibodies against neuroblastoma suppressor of tumorigenicity 1 (NBL1): a potential biomarker for coronary artery disease in patients with obstructive sleep apnea. PLoS One. (2018) 13:e0195015. doi: 10.1371/journal.pone.0195015
30. Hiwasa, T, Wang, H, Goto, K-i, Mine, S, Machida, T, Kobayashi, E, et al. Serum anti-DIDO1, anti-CPSF2, and anti-FOXJ2 antibodies as predictive risk markers for acute ischemic stroke. BMC Med. (2021) 19:131. doi: 10.1186/s12916-021-02001-9
31. Hiwasa, T, Machida, T, Zhang, X-M, Kimura, R, Wang, H, Iwase, K, et al. Elevated levels of autoantibodies against ATP2B4 and BMP-1 in sera of patients with atherosclerosis-related diseases. Immunome Res. (2015) 11:097. doi: 10.4172/1745-7580.1000097
32. Kubota, M, Yoshida, Y, Kobayashi, E, Matsutani, T, Li, SY, Zhang, BS, et al. Serum anti-SERPINE1 antibody as a potential biomarker of acute cerebral infarction. Sci Rep. (2021) 11:21772. doi: 10.1038/s41598-021-01176-8
33. Chen, PM, Ohno, M, Hiwasa, T, Nishi, K, Saijo, S, Sakamoto, J, et al. Nardilysin is a promising biomarker for the early diagnosis of acute coronary syndrome. Int J Cardiol. (2017) 243:1–8. doi: 10.1016/j.ijcard.2017.04.047
34. Adams, HP, Bendixen, BH, Kappelle, LJ, Biller, J, Love, BB, Gordon, DL, et al. Classification of subtype of acute ischemic stroke. Definitions for use in a multicenter clinical trial. TOAST. Trial of org 10172 in acute stroke treatment. Stroke. (1993) 24:35–41. doi: 10.1161/01.STR.24.1.35
35. Nishiura, R, Fujimoto, S, Sato, Y, Yamada, K, Hisanaga, S, Hara, S, et al. Elevated osteoprotegerin levels predict cardiovascular events in new hemodialysis patients. Am J Nephrol. (2009) 29:257–63. doi: 10.1159/000157629
36. Komatsu, H, Fujimoto, S, Hara, S, Fukuda, A, Fukudome, K, Yamada, K, et al. Recent therapeutic strategies improve renal outcome in patients with IgA nephropathy. Am J Nephrol. (2009) 30:19–25. doi: 10.1159/000197116
37. Ito, M, Hiwasa, T, Oshima, Y, Yajima, S, Suzuki, T, Nanami, T, et al. Identification of serum anti-striatin 4 antibodies as a common marker for esophageal cancer and other solid cancers. Mol Clin Oncol. (2021) 15:237. doi: 10.3892/mco.2021.2399
38. Japanese Classification of Colorectal. Appendiceal, and anal carcinoma: the 3d English edition secondary publication. J Anus Rectum Colon. (2019) 3:175–95. doi: 10.23922/jarc.2019-018. PMID:31768468
39. Terminology and Diagnostic Criteria Committee, Japan Society of Ultrasonics in Medicine. Standard method for ultrasound evaluation of carotid artery lesions. J Med Ultrason (2001). (2009) 36:219–26. doi: 10.1007/s10396-009-0238-y
40. Nakahashi, T, Tada, H, Sakata, K, Nomura, A, Ohira, M, Mori, M, et al. Additive prognostic value of carotid plaque score to enhance the age, creatinine, and ejection fraction score in patients with acute coronary syndrome. J Atheroscler Thromb. (2018) 25:709–19. doi: 10.5551/jat.42317
41. Handa, N, Matsumoto, M, Maeda, H, Hougaku, H, Ogawa, S, Fukunaga, R, et al. Ultrasonic evaluation of early carotid atherosclerosis. Stroke. (1990) 21:1567–72. doi: 10.1161/01.str.21.11.1567
42. Shirai, K, Utino, J, Otsuka, K, and Takata, M. A novel blood pressure-independent arterial wall stiffness parameter; cardio-ankle vascular index (CAVI). J Atheroscler Thromb. (2006) 13:101–7. doi: 10.5551/jat.13.101
43. Yoshida, Y, Wang, H, Hiwasa, T, Machida, T, Kobayashi, E, Mine, S, et al. Elevation of autoantibody level against PDCD11 in patients with transient ischemic attack. Oncotarget. (2018) 9:8836–48. doi: 10.18632/oncotarget.23653
44. Wang, H, Zhang, XM, Tomiyoshi, G, Nakamura, R, Shinmen, N, Kuroda, H, et al. Association of serum levels of antibodies against MMP1, CBX1, and CBX5 with transient ischemic attack and cerebral infarction. Oncotarget. (2018) 9:5600–13. doi: 10.18632/oncotarget.23789
45. Hashiguchi, Y, Kasai, M, Fukuda, T, Ichimura, T, Yasui, T, and Sumi, T. Serum carcinoembryonic antigen as a tumour marker in patients with endometrial cancer. Curr Oncol. (2016) 23:e439–42. doi: 10.3747/co.23.3153
46. Kambara, Y, Miyake, H, Nagai, H, Yoshioka, Y, Shibata, K, Asai, S, et al. CA19-9 is a significant prognostic marker of patients with stage III gastric cancer. Eur J Surg Oncol. (2020) 46:1918–24. doi: 10.1016/j.ejso.2020.05.003
47. Sugimoto, K, Hiwasa, T, Shibuya, K, Hirano, S, Beppu, M, Isose, S, et al. Novel autoantibodies against the proteasome subunit PSMA7 in amyotrophic lateral sclerosis. J Neuroimmunol. (2018) 325:54–60. doi: 10.1016/j.jneuroim.2018.09.013
48. Hiwasa, T, Tomiyoshi, G, Nakamura, R, Shinmen, N, Kuroda, H, Kunimatsu, M, et al. Serum SH3BP5-specific antibody level is a biomarker of atherosclerosis. Immunome Res. (2017) 9:18559–69. doi: 10.18632/oncotarget.24963
49. Liu, TL, Shimada, H, Ochiai, T, Shiratori, T, Lin, SE, Kitagawa, M, et al. Enhancement of chemosensitivity toward peplomycin by calpastatin-stabilized NF-kappaB p65 in esophageal carcinoma cells: possible involvement of Fas/Fas-L synergism. Apoptosis. (2006) 11:1025–37. doi: 10.1007/s10495-006-6353-y
50. Yoshida, Y, Zhang, XM, Wang, H, Machida, T, Mine, S, Kobayashi, E, et al. Elevated levels of autoantibodies against DNAJC2 in sera of patients with atherosclerotic diseases. Heliyon. (2020) 6:e04661. doi: 10.1016/j.heliyon.2020.e04661
51. Li, SY, Yoshida, Y, Kobayashi, E, Adachi, A, Hirono, S, Matsutani, T, et al. Association between serum anti-ASXL2 antibody levels and acute ischemic stroke, acute myocardial infarction, diabetes mellitus, chronic kidney disease and digestive organ cancer, and their possible association with atherosclerosis and hypertension. Int J Mol Med. (2020) 46:1274–88. doi: 10.3892/ijmm.2020.4690
52. Li, SY, Yoshida, Y, Kobayashi, E, Kubota, M, Matsutani, T, Mine, S, et al. Serum anti-AP3D1 antibodies are risk factors for acute ischemic stroke related with atherosclerosis. Sci Rep. (2021) 11:13450. doi: 10.1038/s41598-021-92786-9
53. Tsugane, S, and Sawada, N. The JPHC study: design and some findings on the typical Japanese diet. Jpn J Clin Oncol. (2014) 44:777–82. doi: 10.1093/jjco/hyu096
54. Ikeda, A, Iso, H, Sasazuki, S, Inoue, M, and Tsugane, S. The combination of helicobacter pylori-and cytotoxin-associated gene-a seropositivity in relation to the risk of myocardial infarction in middle-aged Japanese: the Japan public health center-based study. Atherosclerosis. (2013) 230:67–72. doi: 10.1016/j.atherosclerosis.2013.06.013
55. Ahmed, D, Eide, PW, Eilertsen, IA, Danielsen, SA, Eknæs, M, Hektoen, M, et al. Epigenetic and genetic features of 24 colon cancer cell lines. Oncogenesis. (2013) 2:e71. doi: 10.1038/oncsis.2013.35
56. Nita, ME, Nagawa, H, Tominaga, O, Tsuno, N, Fujii, S, Sasaki, S, et al. 5-fluorouracil induces apoptosis in human colon cancer cell lines with modulation of Bcl-2 family proteins. Br J Cancer. (1998) 78:986–92. doi: 10.1038/bjc.1998.617
57. Couture, C, Desjardins, P, Zaniolo, K, Germain, L, and Guérin, SL. Enhanced wound healing of tissue-engineered human corneas through altered phosphorylation of the CREB and AKT signal transduction pathways. Acta Biomater. (2018) 73:312–25. doi: 10.1016/j.actbio.2018.04.021
58. Zhu, Y, Zhang, X, Qi, L, Cai, Y, Yang, P, Xuan, G, et al. HULC long noncoding RNA silencing suppresses angiogenesis by regulating ESM-1 via the PI3K/Akt/mTOR signaling pathway in human gliomas. Oncotarget. (2016) 7:14429–40. doi: 10.18632/oncotarget.7418
59. Jiang, P, Du, W, Heese, K, and Wu, M. The bad guy cooperates with good cop p53: bad is transcriptionally up-regulated by p53 and forms a bad/p53 complex at the mitochondria to induce apoptosis. Mol Cell Biol. (2006) 26:9071–82. doi: 10.1128/mcb.01025-06
60. Shinmen, N, Koshida, T, Kumazawa, T, Sato, K, Shimada, H, Matsutani, T, et al. Activation of NFAT signal by p53-K120R mutant. FEBS Lett. (2009) 583:1916–22. doi: 10.1016/j.febslet.2009.04.041
61. Kanda, Y. Investigation of the freely available easy-to-use software 'EZR' for medical statistics. Bone Marrow Transplant. (2013) 48:452–8. doi: 10.1038/bmt.2012.244
62. Tran, LT, Park, HJ, and Kim, HD. Is the carotid intima-media thickness really a good surrogate marker of atherosclerosis? J Atheroscler Thromb. (2012) 19:680–90. doi: 10.5551/jat.11767
63. Zureik, M, Ducimetière, P, Touboul, PJ, Courbon, D, Bonithon-Kopp, C, Berr, C, et al. Common carotid intima-media thickness predicts occurrence of carotid atherosclerotic plaques: longitudinal results from the aging vascular study (EVA) study. Arterioscler Thromb Vasc Biol. (2000) 20:1622–9. doi: 10.1161/01.atv.20.6.1622
64. Koivistoinen, T, Virtanen, M, Hutri-Kähönen, N, Lehtimäki, T, Jula, A, Juonala, M, et al. Arterial pulse wave velocity in relation to carotid intima-media thickness, brachial flow-mediated dilation and carotid artery distensibility: the cardiovascular risk in young finns study and the health 2000 survey. Atherosclerosis. (2012) 220:387–93. doi: 10.1016/j.atherosclerosis.2011.08.007
65. Nakamura, K, Tomaru, T, Yamamura, S, Miyashita, Y, Shirai, K, and Noike, H. Cardio-ankle vascular index is a candidate predictor of coronary atherosclerosis. Circ J. (2008) 72:598–604. doi: 10.1253/circj.72.598
66. Rothwell, PM, and Warlow, CP. Timing of TIAs preceding stroke: time window for prevention is very short. Neurology. (2005) 64:817–20. doi: 10.1212/01.Wnl.0000152985.32732.Ee
67. Selvarajan, V, Bidkar, AP, Shome, R, Banerjee, A, Chaubey, N, Ghosh, SS, et al. Studying in vitro phagocytosis of apoptotic cancer cells by recombinant GMCSF-treated RAW 264.7 macrophages. Int J Biol Macromol. (2017) 102:1138–45. doi: 10.1016/j.ijbiomac.2017.05.003
68. Levine, AJ. p53 and the immune response: 40 years of exploration-a plan for the future. Int J Mol Sci. (2020) 21:21. doi: 10.3390/ijms21020541
69. Pandey, S, Malviya, G, and Chottova, DM. Role of peptides in diagnostics. Int J Mol Sci. (2021) 22:22. doi: 10.3390/ijms22168828
70. Wu, X, Bayle, JH, Olson, D, and Levine, AJ. The p53-mdm-2 autoregulatory feedback loop. Genes Dev. (1993) 7:1126–32. doi: 10.1101/gad.7.7a.1126
71. Lukashchuk, N, and Vousden, KH. Ubiquitination and degradation of mutant p53. Mol Cell Biol. (2007) 27:8284–95. doi: 10.1128/mcb.00050-07
72. Levine, AJ, Momand, J, and Finlay, CA. The p53 tumour suppressor gene. Nature. (1991) 351:453–6. doi: 10.1038/351453a0
73. Piccoli, L, Campo, I, Fregni, CS, Rodriguez, BM, Minola, A, Sallusto, F, et al. Neutralization and clearance of GM-CSF by autoantibodies in pulmonary alveolar proteinosis. Nat Commun. (2015) 6:7375. doi: 10.1038/ncomms8375
74. Shibata, Y, Berclaz, PY, Chroneos, ZC, Yoshida, M, Whitsett, JA, and Trapnell, BC. GM-CSF regulates alveolar macrophage differentiation and innate immunity in the lung through PU.1. Immunity. (2001) 15:557–67. doi: 10.1016/s1074-7613(01)00218-7
75. Jarrell, JA, Baker, MC, Perugino, CA, Liu, H, Bloom, MS, Maehara, T, et al. Neutralizing anti-IL-1 receptor antagonist autoantibodies induce inflammatory and fibrotic mediators in IgG4-related disease. J Allergy Clin Immunol. (2022) 149:358–68. doi: 10.1016/j.jaci.2021.05.002
76. Zahran, N, Sabry, O, and Raafat, M. Granulocyte macrophage colony stimulating factor as an adjuvant in ESRD at high risk of bacterial infection. J Med Sci. (2019) 19:17–23. doi: 10.3923/jms.2019.17.23
77. Baek, JH, Agarwal, ML, Tubbs, RR, Vladisavljevic, A, Tomita, H, Bukowski, RM, et al. In vivo recombinant adenovirus-mediated p53 gene therapy in a syngeneic rat model for colorectal cancer. J Korean Med Sci. (2004) 19:834–41. doi: 10.3346/jkms.2004.19.6.834
78. Parker, LP, Wolf, JK, and Price, JE. Adenoviral-mediated gene therapy with Ad5CMVp53 and Ad5CMVp21 in combination with standard therapies in human breast cancer cell lines. Ann Clin Lab Sci. (2000) 30:395–405.
79. Guevara, NV, Kim, HS, Antonova, EI, and Chan, L. The absence of p53 accelerates atherosclerosis by increasing cell proliferation in vivo. Nat Med. (1999) 5:335–9. doi: 10.1038/6585
80. Merched, AJ, Williams, E, and Chan, L. Macrophage-specific p53 expression plays a crucial role in atherosclerosis development and plaque remodeling. Arterioscler Thromb Vasc Biol. (2003) 23:1608–14. doi: 10.1161/01.Atv.0000084825.88022.53
81. van Vlijmen, BJ, Gerritsen, G, Franken, AL, Boesten, LS, Kockx, MM, Gijbels, MJ, et al. Macrophage p53 deficiency leads to enhanced atherosclerosis in APOE*3-Leiden transgenic mice. Circ Res. (2001) 88:780–6. doi: 10.1161/hh0801.089261
82. Liu, J, Li, W, Li, Y, Chen, T, El-Dahr, S, Feng, Y, et al. Abstract 15: loss of tumor suppressor protein p53 is linked to high blood pressure. Hypertension. (2013) 62:A15. doi: 10.1161/hyp.62.suppl_1.A15
83. Hollander, W. Role of hypertension in atherosclerosis and cardiovascular disease. Am J Cardiol. (1976) 38:786–800. doi: 10.1016/0002-9149(76)90357-x
84. Kannel, WB, Neaton, JD, Wentworth, D, Thomas, HE, Stamler, J, Hulley, SB, et al. Overall and coronary heart disease mortality rates in relation to major risk factors in 325,348 men screened for the MRFIT. Multiple risk factor intervention trial. Am Heart J. (1986) 112:825–36. doi: 10.1016/0002-8703(86)90481-3
85. Stamler, J, Neaton, JD, and Wentworth, DN. Blood pressure (systolic and diastolic) and risk of fatal coronary heart disease. Hypertension. (1989) 13:I2–I12. doi: 10.1161/01.hyp.13.5_suppl.i2
86. Goldstein, LB, Bushnell, CD, Adams, RJ, Appel, LJ, Braun, LT, Chaturvedi, S, et al. Guidelines for the primary prevention of stroke: a guideline for healthcare professionals from the American Heart Association/American Stroke Association. Stroke. (2011) 42:517–84. doi: 10.1161/STR.0b013e3181fcb238
87. Alloubani, A, Saleh, A, and Abdelhafiz, I. Hypertension and diabetes mellitus as a predictive risk factors for stroke. Diabetes Metab Syndr. (2018) 12:577–84. doi: 10.1016/j.dsx.2018.03.009
88. Atabek, ME, Akyürek, N, Eklioglu, BS, and Alp, H. Impaired systolic blood dipping and nocturnal hypertension: an independent predictor of carotid intima-media thickness in type 1 diabetic patients. J Diabetes Complicat. (2014) 28:51–5. doi: 10.1016/j.jdiacomp.2013.09.007
89. Johnston, SC, Fayad, PB, Gorelick, PB, Hanley, DF, Shwayder, P, van Husen, D, et al. Prevalence and knowledge of transient ischemic attack among US adults. Neurology. (2003) 60:1429–34. doi: 10.1212/01.wnl.0000063309.41867.0f
90. Rothwell, PM, Giles, MF, Chandratheva, A, Marquardt, L, Geraghty, O, Redgrave, JN, et al. Effect of urgent treatment of transient ischaemic attack and minor stroke on early recurrent stroke (EXPRESS study): a prospective population-based sequential comparison. Lancet. (2007) 370:1432–42. doi: 10.1016/s0140-6736(07)61448-2
91. Zock, E, Kerkhoff, H, and Kleyweg, RP. Initial reactions of patients after a stroke: more than half undertake no action. Ned Tijdschr Geneeskd. (2012) 156:A4336.
92. Kang, BY, Wang, W, Palade, P, Sharma, SG, and Mehta, JL. Cardiac hypertrophy during hypercholesterolemia and its amelioration with rosuvastatin and amlodipine. J Cardiovasc Pharmacol. (2009) 54:327–34. doi: 10.1097/FJC.0b013e3181b76713
93. Aude, YW, and Mehta, JL. Nonplatelet-mediated effects of aspirin. Drugs Today (Barc). (2002) 38:501–7. doi: 10.1358/dot.2002.38.7.820117
94. Hörl, G, Froehlich, H, Ferstl, U, Ledinski, G, Binder, J, Cvirn, G, et al. Simvastatin efficiently lowers small LDL-IgG immune complex levels: a therapeutic quality beyond the lipid-lowering effect. PLoS One. (2016) 11:e0148210. doi: 10.1371/journal.pone.0148210
Keywords: colony-stimulating factor 2, acute ischemic stroke, colorectal cancer, antibody biomarker, atherosclerosis
Citation: Li S-Y, Yoshida Y, Kubota M, Zhang B-S, Matsutani T, Ito M, Yajima S, Yoshida K, Mine S, Machida T, Hayashi A, Takemoto M, Yokote K, Ohno M, Nishi E, Kitamura K, Kamitsukasa I, Takizawa H, Sata M, Yamagishi K, Iso H, Sawada N, Tsugane S, Iwase K, Shimada H, Iwadate Y and Hiwasa T (2023) Utility of atherosclerosis-associated serum antibodies against colony-stimulating factor 2 in predicting the onset of acute ischemic stroke and prognosis of colorectal cancer. Front. Cardiovasc. Med. 10:1042272. doi: 10.3389/fcvm.2023.1042272
Received: 12 September 2022; Accepted: 11 January 2023;
Published: 10 February 2023.
Edited by:
Ying Wang, University of British Columbia, CanadaCopyright © 2023 Li, Yoshida, Kubota, Zhang, Matsutani, Ito, Yajima, Yoshida, Mine, Machida, Hayashi, Takemoto, Yokote, Ohno, Nishi, Kitamura, Kamitsukasa, Takizawa, Sata, Yamagishi, Iso, Sawada, Tsugane, Iwase, Shimada, Iwadate and Hiwasa. This is an open-access article distributed under the terms of the Creative Commons Attribution License (CC BY). The use, distribution or reproduction in other forums is permitted, provided the original author(s) and the copyright owner(s) are credited and that the original publication in this journal is cited, in accordance with accepted academic practice. No use, distribution or reproduction is permitted which does not comply with these terms.
*Correspondence: Shu-Yang Li, bGlzaHV5YW5nMTk5MEBob3RtYWlsLmNvbQ==; Takaki Hiwasa, aGl3YXNhX3Rha2FraUBmYWN1bHR5LmNoaWJhLXUuanA=
Disclaimer: All claims expressed in this article are solely those of the authors and do not necessarily represent those of their affiliated organizations, or those of the publisher, the editors and the reviewers. Any product that may be evaluated in this article or claim that may be made by its manufacturer is not guaranteed or endorsed by the publisher.
Research integrity at Frontiers
Learn more about the work of our research integrity team to safeguard the quality of each article we publish.