- 1Division of Cardiology, Cardiovascular Medical Center, Far Eastern Memorial Hospital, New Taipei City, Taiwan
- 2School of Medicine, National Yang Ming Chiao Tung University, Taipei City, Taiwan
- 3Department of Nuclear Medicine Center, Far Eastern Memorial Hospital, New Taipei City, Taiwan
- 4Division of Radiation Oncology, Department of Radiology, Far Eastern Memorial Hospital, New Taipei City, Taiwan
- 5Department of Biomedical Imaging and Radiological Sciences, National Yang Ming Chiao Tung University, Taipei City, Taiwan
- 6Division of Radiology, Department of Radiology, Far Eastern Memorial Hospital, New Taipei City, Taiwan
- 7Department of Electronic Engineering, Asia Eastern University of Science and Technology, New Taipei City, Taiwan
- 8School of Medicine, Institute of Traditional Medicine, National Yang Ming Chiao Tung University, Taipei City, Taiwan
- 9Head and Neck Cancer Surveillance and Research Group, Far Eastern Memorial Hospital, New Taipei City, Taiwan
Background: Encouraging results have been reported for the treatment of ventricular tachycardia (VT) with stereotactic body radiation therapy (SBRT) with 25 Gy. SBRT with 12 Gy for refractory VT was designed to reduce long-term cardiac toxicity.
Methods: Stereotactic body radiation therapy-VT simulation, planning, and treatment were performed using standard techniques. A patient was treated with a marginal dose of 12 Gy in a single fraction to the planning target volume (PTV). The goal was for at least ≥ 95% of the PTV to be covered by at least 95% of 12 Gy radiation.
Results: From April 2021 through June 2022, a patient with refractory VT underwent treatment. The volume for PTV was 65.8 cm3. The mean radiation dose administered to the heart (the heart volume excluding the PTV) was 2.2 Gy. No acute or late toxicity was observed after SBRT. Six months after SBRT, the patient experienced new monomorphic right ventricular outflow tract (RVOT) VT. Interestingly, the substrate of the left ventricular basal to middle posteroseptal wall before SBRT was turned into scar zones with a local voltage < 0.5 mV. Catheter ablation to treat RVOT VT was performed, and the situation remains stable to date.
Conclusion: This study reports the first patient with refractory VT successfully treated with 12.0 Gy SBRT, suggesting that 12 Gy is a potential dose to treat refractory VT. Further investigations and enrollment of more patients are warranted to assess the long-term efficacy and side effects of this treatment.
Introduction
Ventricular tachycardia (VT) is a life-threatening disease that is caused by electric reentry within and around patches of myocardial scarring (1), especially in patients with postmyocardial infarction (post-MI) heart failure. The implantation of a cardioverter-defibrillator (ICD) prevents sudden cardiac death, but ICD shocks are also associated with mortality (2, 3). Recently, cardiac imaging and electroanatomic mapping (EAM) have improved in guiding the ablation procedure to identify the VT substrate and to ablate these regions, with encouraging results (4, 5). However, following such procedures, the recurrence rate after a first VT ablation is 12.0–62.0% (6, 7), so patients must undergo repeated catheter ablation procedures (8).
Stereotactic body radiation therapy (SBRT) is an advanced external beam radiotherapy technique that precisely delivers a high radiation dose with an image-guided technique to targets in a few fractions (9, 10). Recently, encouraging results were reported for patients with VT who were treated with SBRT (11, 12). Cuculich et al. (11) documented that all awake patients treated with SBRT in a single fraction of 25 Gy exhibited a 99.9% decrease in the VT burden compared to the baseline. In a subsequent phase I/II trial, the frequency of VT episodes or premature ventricular contraction (PVC) burden was reduced by 75.0% in 89.0% of patients, as reported by Robinson et al. (12).
However, 10.5% of patients in that study experienced grade 3 treatment-related adverse effects, 11.1% developed grade 2 radiation pneumonitis, and 28% exhibited pericardial effusions (12). Calculation of the late radiation response of the heart by equivalent dose in 2-Gy fractions (EQD2, corrected for fractionation with alpha/beta ratio = 2.5) showed that the administration of 25 Gy in one fraction might actually elicit the same response as 152 Gy (13). Interestingly, radiosurgery with 12 Gy provides a high rate of control of acoustic neuroma (14), which provides insight into abnormal nerve behavior that may be reversed by radiosurgery with marginal doses of 12 Gy.
A stereotactic ablative radiosurgery (SARS) for refractory VT (SARS-VT) trial was established at our institution after obtaining approval from the Human Experimentation Committee of Far Eastern Memorial Hospital (FEMH108074-F) to reduce long-term cardiac toxicity, improve quality of life, and search for the optimal radiation ablation dose. Here, we report the first patient in the world with refractory VT who was treated with SBRT using 12 Gy radiation and was followed for more than 1 year. This patient had a favorable outcome with a satisfactory quality of life.
Case report
Patient history
A 63-year-old man had a past history of type 2 diabetes mellitus, hypertension, hyperlipidemia, chronic kidney disease and congenital bicuspid aortic valve stenosis with coronary artery disease. Coronary artery angioplasty and stenting to the right coronary artery and left circumflex artery had been performed more than 10 years prior. The patient had developed congestive heart failure (New York Heart Association, functional class II to III) with a reduced ejection fraction and a left ventricular ejection fraction (LVEF) of less than 35% in the past 3 years. Two years before this presentation, he received xenograft aortic valve replacement due to rapidly progressive aortic valve stenosis and coronary artery bypass graft with saphenous vein graft (SVG) anastomosis to the left anterior descending artery. A dual-chamber implanted cardioverter-defibrillator (ICD) was also implanted for subsequent sustained monomorphic VT.
One year ago, the patient had a recurrent VT storm with ICD shock. The twelve-lead electrocardiogram revealed two different morphologies of clinical VT (Figures 1A,B). Both VT morphologies indicated right bundle branch block morphology; one involved the superior axis, and the other involved the inferior axis, which suggested an origin of VT from the left ventricular septal wall. During the electrophysiology study, bipolar voltage mapping with a three-dimensional (3D) CARTO mapping system (Biosense, Diamond Bar, CA) suggested the presence of a large, uneven, mixed scar and viable zone in the area of the left ventricular basal to middle posteroseptal wall (Figures 2A,C,E). We defined the border zone as where the voltage was <1.5 mV. Local abnormal ventricular activity (LAVA) was identified as a substrate using a PENTARAY® NAV ECO high-density mapping catheter near the scar margin. Endocardial ablation with LAVA elimination was performed with an acute end point of non-inducibility under ventricular extrastimuli. However, recurrent VT storms still occurred 5 months after catheter ablation, with a total of 21 episodes of ICD therapy (15 events of antitachycardia pacing and 6 events of shock). The patient received a detailed explanation of the treatment that would be given in the SARS-VT trial from both the treating electrophysiologist (the first author) and the radiation oncologist (the last author). After discussion, he agreed to receive ablated cardiac radiotherapy at a dose of 12 Gy. The patient provided written informed consent to receive treatment. All the authors participated in data collection and analysis.
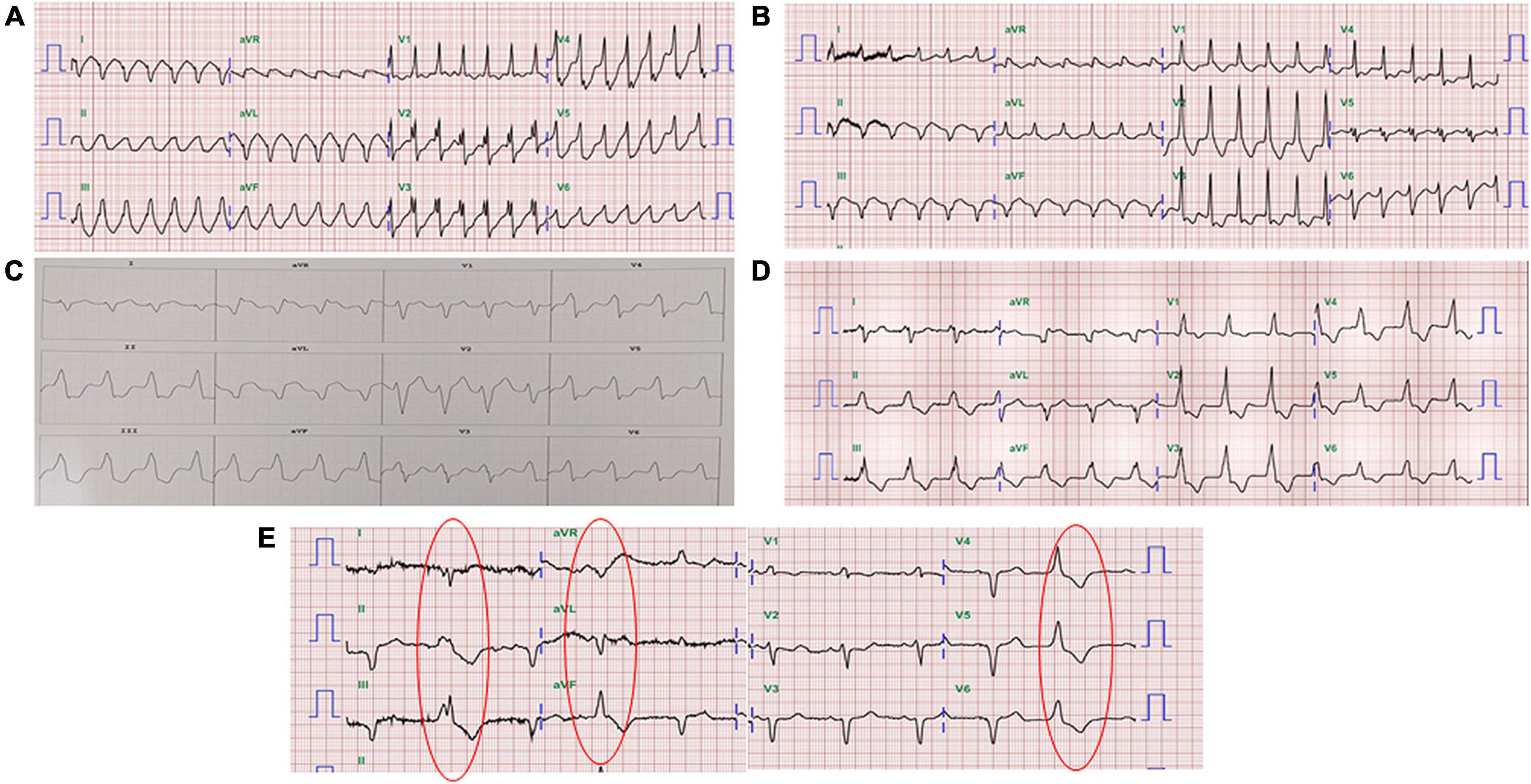
Figure 1. Twelve-lead electrocardiography of the patient. (A) VT with right bundle branch block morphology and an inferior axis. (B) VT with right bundle branch block morphology and a superior axis. (C) VT with the origin in the RVOT. (D) Slow VT with all positive concordance in the precordial leads and an inferior axis suggested at a different location from the initial therapy zone. (E) The morphology of ventricular premature beats was the same as the morphology of the slow VT.
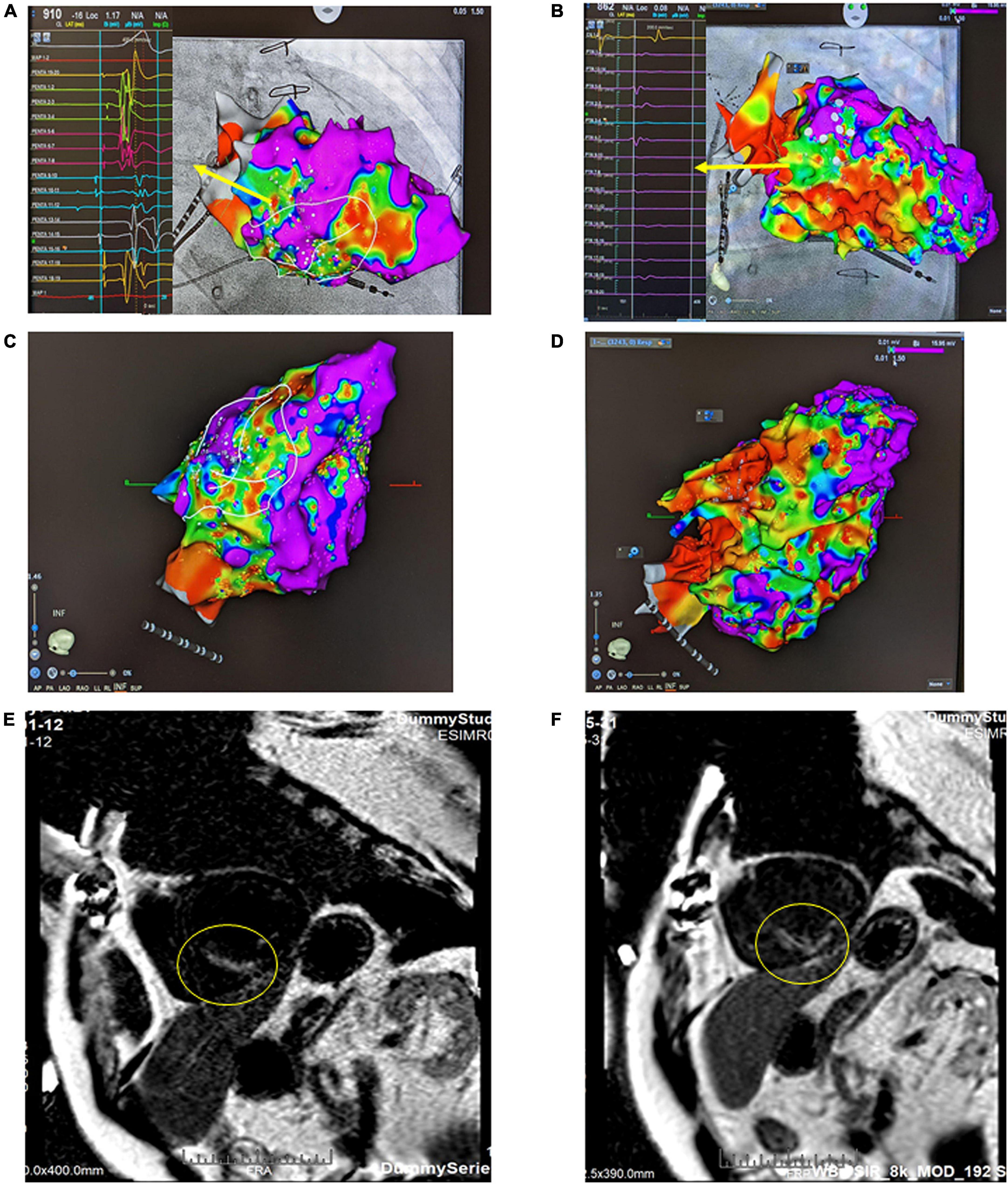
Figure 2. Bipolar voltage mapping with a 3D CARTO mapping system (Biosense, Diamond Bar, CA). (A) The presence of a large, uneven, mixed scar and viable zone in the area of the left ventricular basal to the middle posteroseptal wall from the right anterior oblique (RAO) view. The arrow indicates LAVA, the substrate, as the target ablation zone. (B) After SBRT, the therapy zone became a scar zone. The arrow indicates local voltage < 0.5 mV. (C) Inferior view of the left ventricle (LV) before SARS. (D) Inferior view of the left ventricle (LV) after SBRT. (E) Cardiac MRI using a wide-band sequence before SBRT. A delay in the enhancement of the mid-basal septal wall and mid-inferior wall was noted, which indicated scar formation in this area. (F) Cardiac MRI after SBRT. The previously shown delay in enhancement of the mid-basal septal wall and mid inferior wall was still present; however, the affected area was slightly larger than that on the previous MRI.
Stereotactic body radiation therapy planning and delivery
The procedural workflow is shown in Figure 3. Multimodal imaging combining scar imaging, including the 3D CARTO mapping system (Biosense, Diamond Bar, CA), respiratory phase-correlated 4-dimensional computed tomography (4D-CT) simulation with a 2.5 mm slice thickness (Discovery CT590 RT, GE Healthcare, Chicago, IL, USA), cardiac magnetic resonance imaging (MRI) and 99mTc-sestamibi myocardial perfusion imaging was done offline to delineate a target for ablation. The substrate was targeted at the clinical target volume (CTV) and was segmented through corroboration of all previously acquired imaging and electrocardiographic imaging data by the radiation oncologist and electrophysiologist. An internal target volume (ITV) was created from the phased-binned 4D-CT to account for the maximum range of motion that was assessed by reviewing the playback of all the phases of the 4D-CT overlaid with the reference CT scan. The amplitude for the target was approximately 5 mm. This result was similar to previous reports (12, 15, 16). The data reported that cardiac motion was variable depending on the specific substructure of the heart but was within 5 mm (15, 16). The planning target volume (PTV) was generated as a 3-mm volumetric expansion from the ITV. A plan was developed in the Pinnacle3 planning system (version 9.8.1, Philips Medical Systems, Madison, WI, USA).
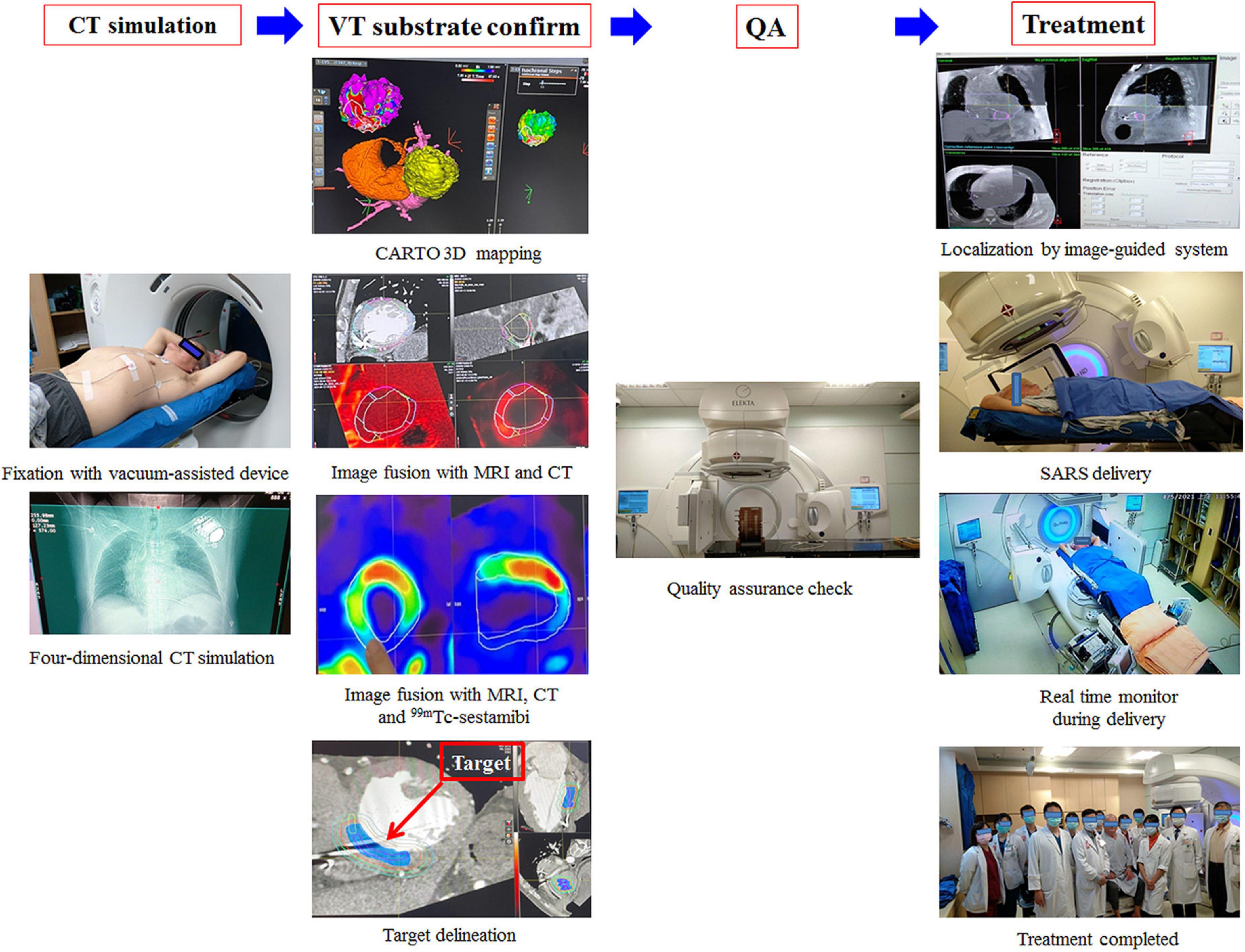
Figure 3. Multimodal imaging combined with scar imaging, including the CARTO mapping system (Biosense, Diamond Bar, CA), CT, MRI and 99mTc-sestamibi MPI, was used offline to delineate a target for ablation. A plan was developed in the Pinnacle3 planning system (version 9.8.1, Philips Medical Systems, Madison, WI, USA). One day before treatment, quality assurance was performed. On the day of treatment, the patient was immobilized on the vacuum device, and cone-beam computed tomography (CBCT) was used for patient setup and target localization. The treatment unit was aligned with the patient, and a dose of 12 Gy was delivered precisely by volumetric-modulated arc therapy (VMAT) through a linear accelerator machine (Versa HDTM, Elekta, Crawley, West Sussex, UK) with 6 MV photons.
A dose of 12 Gy in a single fraction was prescribed to the PTV with the goal of achieving maximal dose coverage while avoiding a dose in excess of the calculated dose constraints for surrounding organs, including the heart and coronary arteries. The goal was for at least ≥ 95% of the PTV to be covered by at least 95% of the prescribed dose (12 Gy). Beam geometry was optimized to avoid the ICD geometrically. Optimization was used to place prescription hotspots (areas receiving > 100% of the prescription dose) within the ITV and CTV, enabling the ITV and CTV to be covered by up to 125 and 150% of the prescribed dose, respectively (Figure 4).
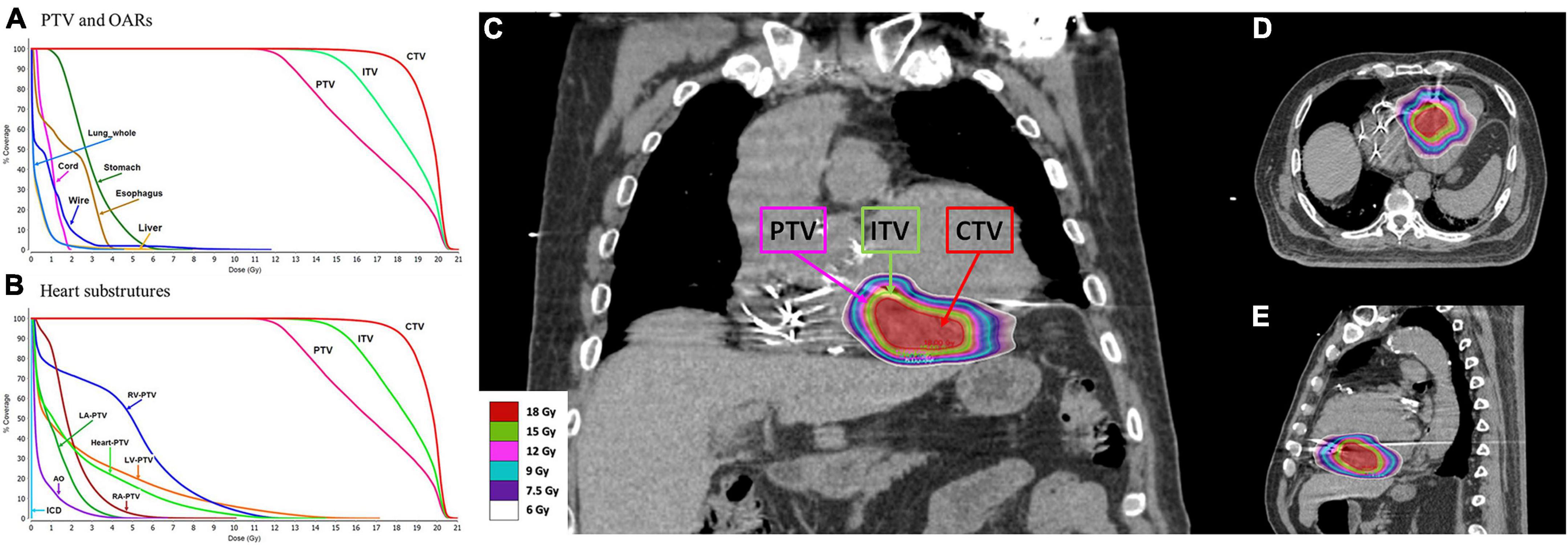
Figure 4. The substrate was targeted at the clinical target volume (CTV). An internal target volume (ITV) was created from the phased-binned 4D CT images to account for the maximum range of motion that was assessed by reviewing the playback of all the phases of the 4D-CT overlaid with the reference CT scan. The planning target volume (PTV) was generated as a 3-mm volumetric expansion from the ITV. The goal was for at least ≥ 95% of the PTV to be covered by at least 95% of the prescribed dose (12 Gy). Optimization was performed to place prescription hotspots (areas receiving > 100% of the prescribed dose) within the ITV and CTV, enabling the ITV and CTV to be covered by up to 125% and 150% of the prescribed dose, respectively. (A) Dose volume histogram (DVH) for the PTV and organs at risk. (B) DVH for the PTV and heart substructures. RV, right ventricle; LV, left ventricle; RA, right atrium; LA, left atrium; AO, aorta; ICD, cardioverter-defibrillator. (C) Coronal view of the plan with color wash. (D) Transverse view of the plan with color wash. (E) Sagittal view of the plan with color wash.
The criteria for the arteries, esophagus, stomach, lungs, and spinal cord were based on those used in a previous report (16). The plan was subjected to and passed standard internal physics quality assurance on a calibrated phantom 1 day before delivery. On the day of treatment, the patient was immobilized on the vacuum device (Klarity, R7515NL-44NL). We used cone-beam computed tomography (CBCT) for patient setup and target localization. The treatment unit was aligned with the patient, and SBRT of 12 Gy was delivered precisely without sedation or anesthesia by an image-guided radiotherapy-equipped linear accelerator (volumetric-modulated arc therapy, VMAT, Versa HDTM, Elekta, Crawley, West Sussex, UK).
Results
Patient and treatment
The CTV, ITV, and PTV were recorded. The target volumes for the CTV, ITV, and PTV were 23.23, 42.45, and 65.75 cm3, respectively. The conformal index was 1.03. The treatment dose rate was 1,400 MU/min for a total marginal dose of 12 Gy with 6 MV flattening-filter-free beams, and the duration of the treatment session was 24 min. The mean radiation dose administered to the heart (the heart volume excluding the PTV) was 2.2 Gy. The maximal doses (<0.03 cc) administered to the left and right coronary arteries were 4.2 and 6.9 Gy, respectively. The maximal dose administered to the mitral valve was 6.5 Gy. The medium doses to the left and right ventricles were 0.8 and 5.0 Gy, respectively. The maximal dose administered to the spinal cord and maximal esophageal dose were 2.0 and 4.4 Gy, respectively. The dosimetry details are shown in Table 1.
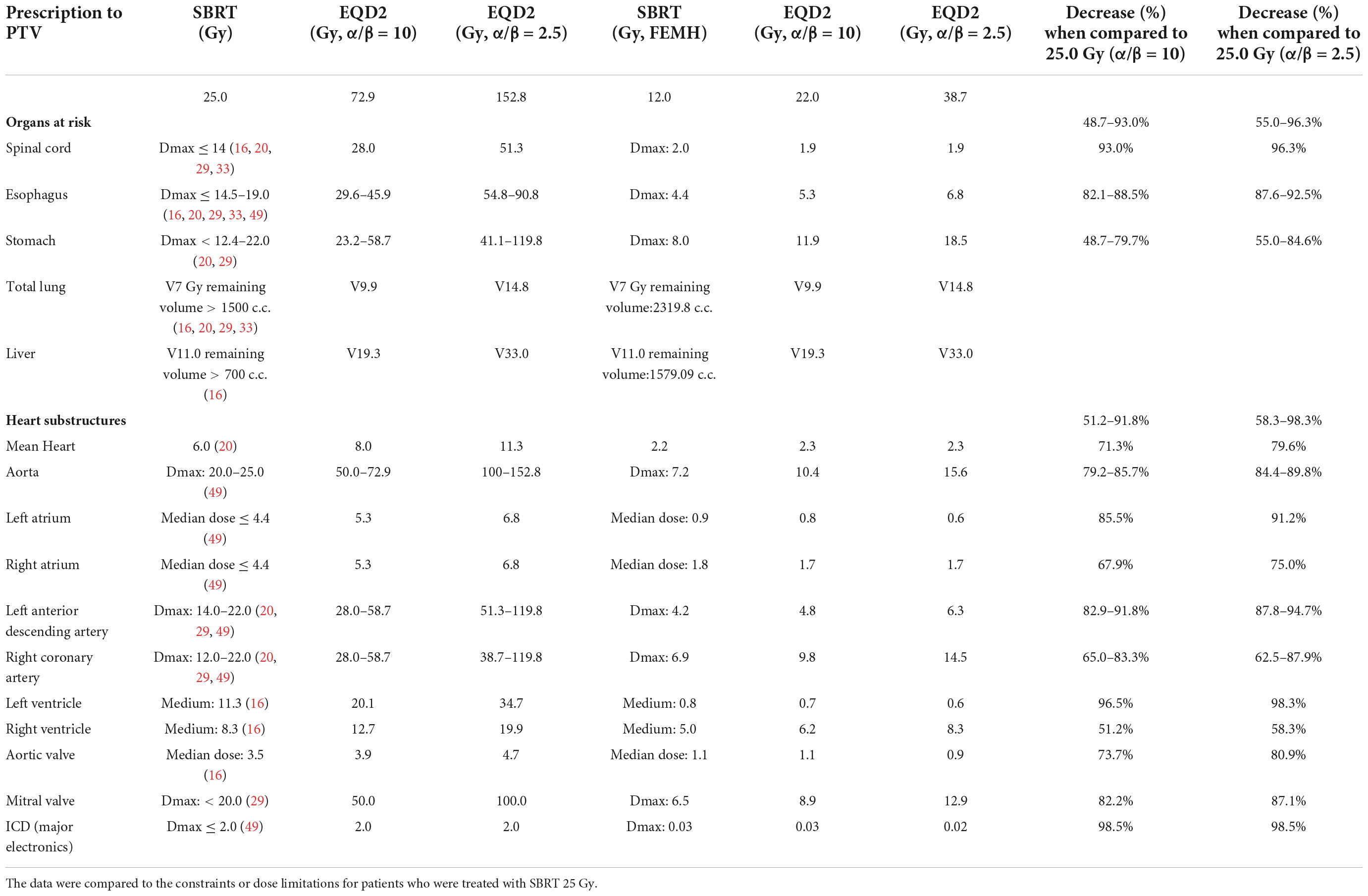
Table 1. The calculated doses to organs at risk and heart substructures for patients treated with stereotactic body radiation therapy (SBRT) 12 Gy.
Safety
No acute toxicity was observed during or immediately after SBRT. No adverse effects were observed from ICD during or after SBRT. No adverse events were related to SBRT during the whole 1-year observation period.
Efficacy
Before SBRT, the patient received two types of antiarrhythmic agents (amiodarone and mexiletine), a maximal tolerated dose of bisoprolol, sacubitril/valsartan and empagliflozin (25 mg daily), which have been suggested to reduce the combined risk of cardiovascular death or hospitalization for heart failure in patients with heart failure. After SBRT and the second electrophysiology study, antiarrhythmic agents and optimal medical treatments for heart failure remained unchanged. Six months after SBRT, 16 episodes of antitachycardia pacing (ATP) plus ICD shock occurred in 24 h. Based on the protocol, a second SBRT needed to be delivered. The patient underwent a second electrophysiology study to confirm the original therapy zone. Interestingly, the substrate of the left ventricular basal to middle posteroseptal wall before SBRT had turned into scar zones with a local voltage < 0.5 mV (Figures 2B,D,F). One monomorphic right ventricular outflow tract (RVOT) VT was easily inducible (Figure 1C). No low-voltage zone or LAVA was observed in this zone through high-density voltage mapping. Catheter ablation to the RVOT VT was performed with an acute end point of non-inducibility under ventricular extrastimuli. During follow-up, another morphology of sustained slow VT with a rate of 92 bpm was noted, without hemodynamic compromise. The morphology of slow VT was all positively concordant in the precordial leads and inferior axis, which indicated a different origin of VT (Figure 1D). Due to the wider QRS and electric replacement interval of the ICD, we upgraded the ICD to a cardiac resynchronization therapy defibrillator (CRT-D). The upgrade to CRT-D may also significantly reduce the frequency of VT. The burden of ventricular arrhythmia has been markedly reduced since then. The morphology of the ventricular premature complex was the same as that of slow VT (Figure 1E). Figure 5 describes the New York Heart Association (NYHA) functional classification, blood pressure, left ventricular ejection fraction (LVEF) and laboratory data with a timeline for the patient before, during and after treatment.
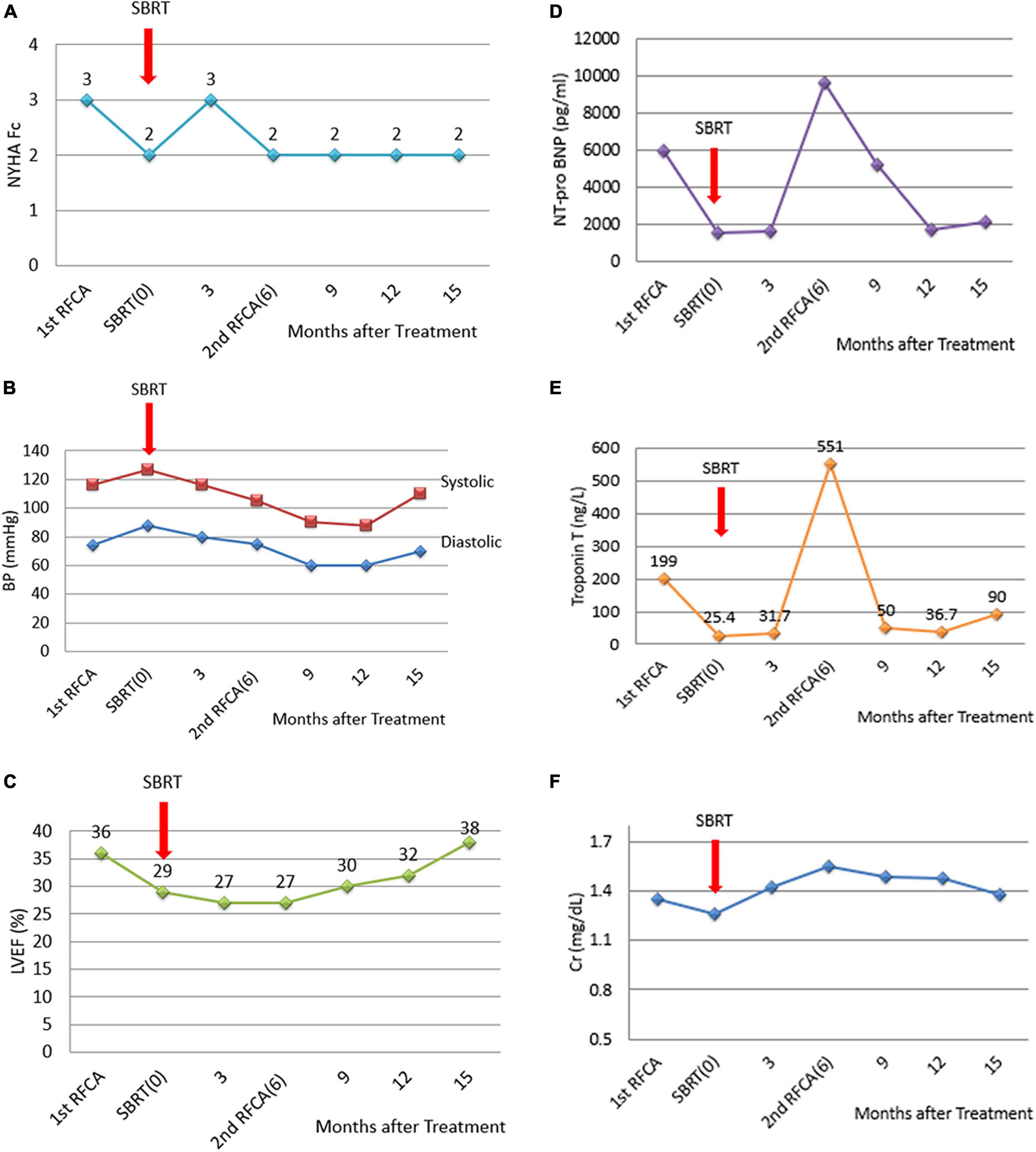
Figure 5. Diagrams of the NYHA functional classification, blood pressure, LVEF and laboratory data with a timeline for the patient before, during and after the treatment. (A) NYHA functional classification, explaining the severity of heart failure. (B) Blood pressure. (C) LVEF improved slightly after catheter ablation to the RVOT VT. The second electrophysiological study documented successful local treatment with SBRT using 12 Gy at the left ventricular basal to middle posterior septal wall. However, (D) NT-pro-BNP and (E) Troponin T levels increased 6 months after SBRT because of recurrent electric storms derived from the RVOT VT. After catheter ablation of the RVOT VT, serum NT-proBNP and troponin T levels decreased. (F) Serum creatinine levels remained unchanged.
Discussion
This report is the first to describe SBRT with a PTV dose of 12 Gy performed in a patient with recurrent VT. The target volume for SBRT was delineated using the CARTO mapping system, with subsequent matching by MRI, 99mTc-sestamibi myocardial perfusion imaging and 4D-CT imaging. SBRT with 12 Gy silenced the abnormal electrical activity of the substrate and successfully reduced the VT burden during the follow-up period.
Cardiac SBRT with 25 Gy in one fraction is a new and innovative treatment for patients with refractory VT (Table 2). To date, eight case reports (17–24) and 11 case series (11, 12, 25–32) have been published. Ninety-one patients were treated with a radiation dose of 25 Gy, and one patient was treated with 24 Gy. The rate of reduction in the VT burden by SBRT was approximately 69–90% (11, 12, 26, 27, 31). However, the rate of recurrent VT after SBRT was 20–100%. Treatment with 25 Gy in one fraction, when corrected for the late radiation effect for fractionation with alpha/beta ratio = 2.5, was equivalent to 152 Gy (13). During the ENCORE VT study (12), two patients experienced grade 3 heart failure exacerbation and pericarditis. Additionally, five patients presented with grade 2 pericardial effusions, and two patients experienced grade 2 radiation pneumonitis. Similarly, Neuwirth et al. (26) reported that one patient exhibited grade 3 late radiation-related progression of mitral regurgitation and changes in valvular morphology 17 months after radiosurgery. Qian et al. (33) reported that 3 of six patients experienced adverse effects after SBRT with 25 Gy: one suffered from pneumonia 1 month later; another had severe left ventricular dysfunction, mitral regurgitation and tricuspid regurgitation and developed heart failure decompensation 104 days later; and the other presented with moderate pericardial effusion 396 days later. Therefore, the potential risks for late radiotherapy-related effects of cardiac toxicity should still be considered with caution.
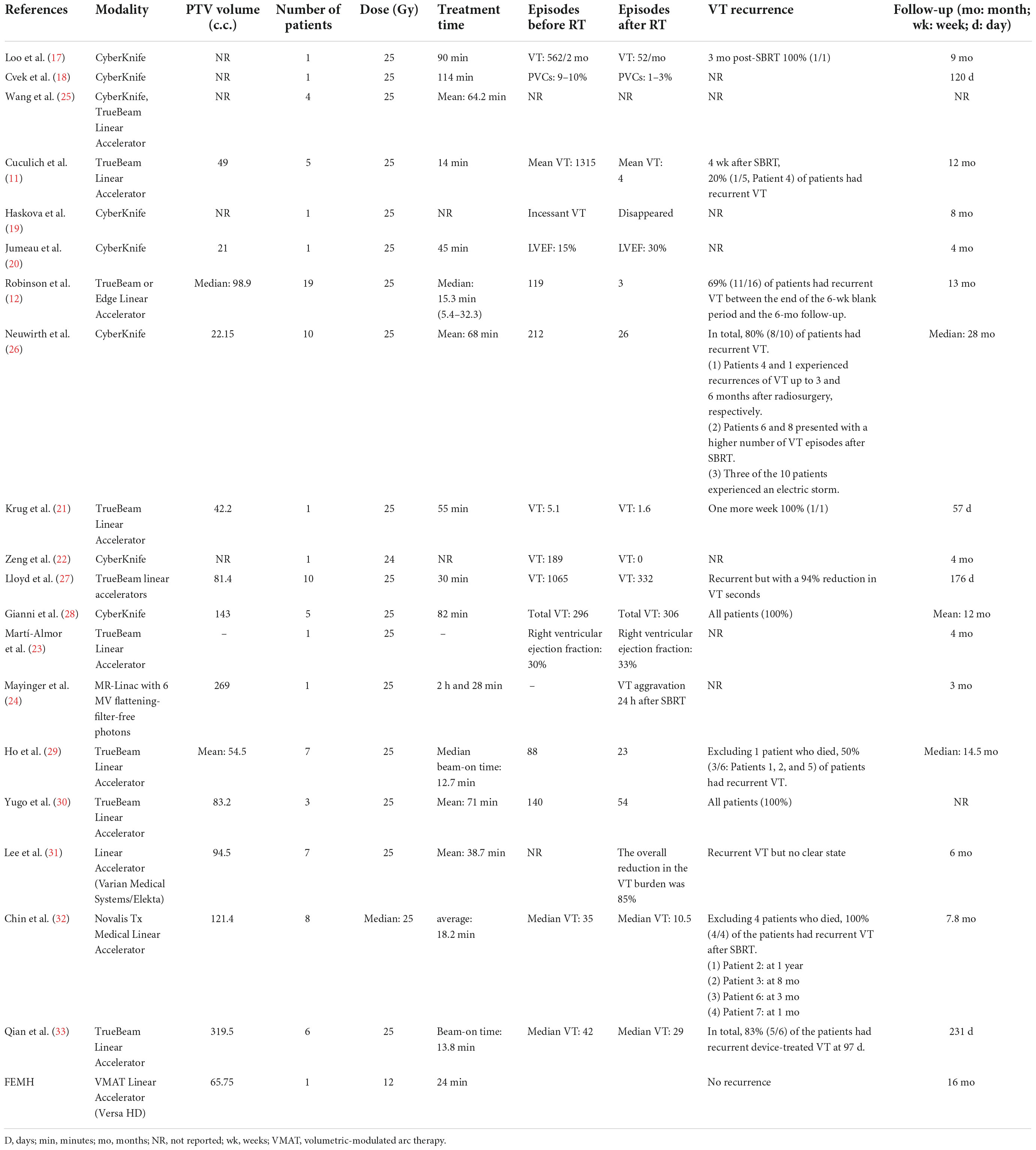
Table 2. Comparison of a patient with refractory VT treated with SBRT using a dose of 12 Gy at the Far Eastern Memorial Hospital (FEMH) with a selected published series of patients treated with SBRT.
SBRT (20 Gy or higher) is associated with reduced capillary density, myocardial degeneration and fibrosis (34, 35). Endothelial vacuolization and disruption of gap junctions on electron microscopy specimens is noted after SBRT as relatively acute effects that occur well before cellular DNA damage–related apoptosis and fibrosis begin (27). Additionally, severe architectural disruption, inflammatory cell infiltration, fibrin deposition and necrosis in myocardial tissue caused by high-dose irradiation in swine have been reported (22, 30, 31). Notably, irradiation with 16 Gy to the mouse heart induced mitochondrial damage and metabolic alterations of the cardiac tissue and led to structural remodeling, functional injury and fibrotic alterations (36). Interestingly, the severity and extent of myocardial injury, such that it reduced resting myocardial perfusion in rats, became more evident at the end of 6 months after 20 Gy heart irradiation in a single fraction (37). Similarly, when 13N-ammonia PET/CT myocardial perfusion imaging (MPI) was used to detect changes in myocardial perfusion induced by the administration of 20 Gy in one fraction to beagle dogs, the data showed a 10% reduction in LVEF at 6 and 12 months after irradiation (38). Similar findings have also been recently documented in human myocardium after SBRT, consistent with the preclinical models (19). Considering all these published observations, high-dose irradiation of the heart potentially results in cardiac damage.
More importantly, the published data show that the potential rate of recurrent VT after SBRT is 20–100% (Table 1). In contrast to those who received 25 Gy, three patients who received lower SBRT doses (median dose of 20 Gy) achieved clinically positive outcomes, as reported by Qian et al. (33). Ho et al. (29) delivered 20 Gy to the PTV with effective results that were lower than those of other reports. Lehmann et al. (39) suggested that ablated RT doses to create a complete atrioventricular conduction block and point doses to the coronary arteries should be < 10 Gy to remain safe. Radiosurgery with 12 Gy provides a high control rate for acoustic neuromas (14). Additionally, for patients with benign tumors adjacent to the anterior visual pathway who were treated by single-fraction stereotactic radiosurgery with less than 12 Gy vs. 12 Gy, the probability of radiation-induced optic neuropathy was 0% vs. 10% (40). Sharma et al. (41) demonstrated that 12 Gy caused mitochondrial degeneration. Mitochondria play an important role in cellular stress signals activated for acute, chronic nerve cell injury and nerve cell death (42) and maintain homeostasis and cellular integrity (43). Dysfunctional mitochondria affect neuronal trafficking across neurons. As mentioned above, mitochondrial damage in cardiac tissue could lead to structural remodeling, functional injury and fibrotic alterations (36). Moreover, 12 Gy irradiation has upregulated matrix metalloproteinase (MMP)-2 and MMP-9 activity. MMP-2 and MMP-9 degrade collagen IV of basement membranes, weakening the structural integrity (44). Notably, in the study of the late radiation response of canines, the median effective dose (ED50) for pericardial fibrosis in 2 Gy fractions was 46.1 Gy (13). However, for 12 Gy in one fraction corrected for fractionation with alpha/beta ratio = 2.5, the EQD2 was 38.7 Gy. Therefore, abnormal nerve reentry may be silenced by degenerating or dysfunctional mitochondria related to SBRT of 12 Gy, which could affect the neuronal trafficking process, structural remodeling and cellular integrity but also decrease the risk of pericardial fibrosis.
The Radiation Therapy Oncology Group (RTOG) 90-05 suggested that 15, 18, and 24 Gy could eradicate brain tumors of 31–40, 21–30, and less than 20 mm, respectively (45). Cardiac SBRT 25 Gy exhibited robust clinical efficacy in humans and shortened the QRS interval to electrophysiologic reprogramming (46). However, conduction velocity reprogramming could be achieved by treatment with as little as 15 Gy of radiation as well as 25 Gy does, suggesting that 15 Gy of RT may be a good dose to achieve electrical conduction reprogramming without transmural fibrosis (46). Interestingly, the RT dose that caused the formation of connective tissue in the myocardium of the left ventricle and septum significantly in the canine study by 2 Gy fractions was 68.0 Gy, and myocytolysis was 70.4 Gy given in 2 Gy fractions (13). Corrected for fractionation with alpha/beta ratio = 2.5, the EQD2 values of 15 and 18 Gy in one fraction were 58.3 and 82.0 Gy, respectively. In other words, conduction velocity reprogramming may be induced by 15 Gy, and 15 Gy does not cause myocardium fibrosis because its EQD2 is less than 68.0 Gy. The EQD2 of 18 Gy equal to 82.0 Gy (i.e., over 68 Gy) means it has a greater chance of causing transmural fibrosis. These lines of evidence support the potential strategy of giving 12 Gy of SBRT to the PTV, and the dose grading in the ITV and CTV to substate was 15 and 18 Gy, respectively. The results for the patient reported here were efficient, as confirmed by high-density mapping, MRI and EKG (Figures 2B,D,F).
Darby et al. (47) reported that rates of major coronary events increased linearly with the mean dose to the heart by 7.4% per gray. Hohmann et al. (48) noted that 3 months after VT was treated by SBRT, the left ventricular ejection fraction (LVEF) decline was correlated with mean dose and V20Gy. Considering the respective EQD2 with alpha/beta ratios = 10 and 2.5 for acute and late effect calculations, the mean heart dose was decreased by 6 Gy (71%) and 9 Gy (80%), respectively. On the other hand, the respective risk of acute and late toxicity of coronary events or heart toxicity could be reduced by 42 and 67%, respectively. Similarly, the acute and late effect doses to the heart substructures were decreased by 40–93 and 58–98%, respectively, when compared with treatment with 25 Gy. All together, the evidence supports the hypothesis that the administration of a lower radiosurgery dose to stop VT recycling and prevent damage to cardiac tissue in the long term may be a feasible idea, but more cases and longer follow-up times are needed to ensure efficacy. Additionally, the patient experienced recurrent VT, and a repeat bipolar voltage mapping study with the 3D CARTO mapping system confirmed a new lesion located at the RVOT, which was explained using the CARTO mapping system to check the electric reentry activity before retreatment. Because a lower radiosurgery dose provides a second chance to re-irradiate patients safely when they experience recurrent VT, relocalization of recurrent VT becomes more important for secondary irradiation.
To the best of our knowledge, this case report is the first in the world of a patient with refractory VT treated with SBRT using 12 Gy, and successful local treatment was confirmed by the 3D CARTO system, cardiac MRI and serial clinical EKG, suggesting that 12 Gy is a good dose for refractory VT. However, this study only describes the results from the first patient who was successfully treated with SBRT at this dose. Further study of more patients is warranted to assess the long-term efficacy and side effects of this treatment, as well as its mechanism of action and cost-effectiveness.
Data availability statement
The original contributions presented in this study are included in the article/supplementary material, further inquiries can be directed to the corresponding author.
Ethics statement
The studies involving human participants were reviewed and approved by the Human Experimentation Committee of Far Eastern Memorial Hospital (FEMH108074-F). The patients/participants provided their written informed consent to participate in this study. Written informed consent was obtained from the participant/s for the publication of this case report.
Author contributions
C-HH, S-HH, Y-WW, and P-WS contributed to conception and design of the study. S-YW, M-CT, H-JT, and H-PY performed the image-fusion localization. Y-HL, W-PC, and H-HL take care of patient. C-HH and S-HH wrote the first draft of the manuscript. All authors contributed to manuscript revision, read, and approved the submitted version.
Funding
This study was supported by grant PI20190001 from Far Eastern Memorial Hospital.
Conflict of interest
The authors declare that the research was conducted in the absence of any commercial or financial relationships that could be construed as a potential conflict of interest.
Publisher’s note
All claims expressed in this article are solely those of the authors and do not necessarily represent those of their affiliated organizations, or those of the publisher, the editors and the reviewers. Any product that may be evaluated in this article, or claim that may be made by its manufacturer, is not guaranteed or endorsed by the publisher.
References
1. Al-Khatib SM, Stevenson WG, Ackerman MJ, Bryant WJ, Callans DJ, Curtis AB, et al. 2017 AHA/ACC/HRS guideline for management of patients with ventricular arrhythmias and the prevention of sudden cardiac death: a report of the American College of Cardiology/American Heart Association task force on clinical practice guidelines and the heart rhythm society. Circulation. (2018) 138:e272–391. doi: 10.1016/j.hrthm.2017.10.036
2. Poole JE, Johnson GW, Hellkamp AS, Anderson J, Callans DJ, Raitt MH, et al. Prognostic importance of defibrillator shocks in patients with heart failure. N Engl J Med. (2008) 359:1009–17.
3. Proietti R, Labos C, Davis M, Thanassoulis G, Santangeli P, Russo V, et al. A systematic review and meta-analysis of the association between implantable cardioverter-defibrillator shocks and long-term mortality. Can J Cardiol. (2015) 31:270–7.
4. Cochet H, Komatsu Y, Sacher F, Jadidi AS, Scherr D, Riffaud M, et al. Integration of merged delayed-enhanced magnetic resonance imaging and multidetector computed tomography for the guidance of ventricular tachycardia ablation: a pilot study. J Cardiovasc Electrophysiol. (2013) 24:419–26. doi: 10.1111/jce.12052
5. Dickfeld T, Tian J, Ahmad G, Jimenez A, Turgeman A, Kuk R, et al. MRI-Guided ventricular tachycardia ablation: integration of late gadolinium-enhanced 3D scar in patients with implantable cardioverter-defibrillators. Circ Arrhythm Electrophysiol. (2011) 4:172–84. doi: 10.1161/CIRCEP.110.958744
6. Liang JJ, Santangeli P, Callans DJ. Long-term outcomes of ventricular tachycardia ablation in different types of structural heart disease. Arrhythm Electrophysiol Rev. (2015) 4:177–83.
7. Sapp JL, Wells GA, Parkash R, Stevenson WG, Blier L, Sarrazin JF, et al. Ventricular tachycardia ablation versus escalation of antiarrhythmic drugs. N Engl J Med. (2016) 375:111–21.
8. Aliot EM, Stevenson WG, Almendral-Garrote JM, Bogun F, Calkins CH, Delacretaz E, et al. EHRA/HRS expert consensus on catheter ablation of ventricular arrhythmias: developed in a partnership with the European Heart Rhythm Association (EHRA), a registered branch of the European Society of Cardiology (ESC), and the Heart Rhythm Society (HRS); in collaboration with the American College of Cardiology (ACC) and the American Heart Association (AHA). Heart Rhythm. (2009) 6:886–933.
9. Potters L, Kavanagh B, Galvin JM, Hevezi JM, Janjan NA, Larson DA, et al. American society for therapeutic radiology and oncology (ASTRO) and American College of Radiology (ACR) practice guideline for the performance of stereotactic body radiation therapy. Int J Radiat Oncol Biol Phys. (2010) 76:326–32. doi: 10.1016/j.ijrobp.2009.09.042
10. Benedict SH, Yenice KM, Followill D, Galvin JM, Hinson W, Kavanagh B, et al. Stereotactic body radiation therapy: the report of AAPM task group 101. Med Phys. (2010) 37:4078–101.
11. Cuculich PS, Schill MR, Kashani R, Mutic S, Lang A, Cooper D, et al. Noninvasive cardiac radiation for ablation of ventricular tachycardia. N Engl J Med. (2017) 377:2325–36.
12. Robinson CG, Samson PP, Moore KMS, Hugo GD, Knutson N, Mutic S, et al. Phase I/II trial of electrophysiology-guided noninvasive cardiac radioablation for ventricular tachycardia. Circulation. (2019) 139:313–21.
13. Gillette SM, Gillette EL, Shida T, Boon J, Miller CW, Powers BE. Late radiation response of canine mediastinal tissues. Radiother Oncol. (1992) 23:41–52.
14. Chopra R, Kondziolka D, Niranjan A, Lunsford LD, Flickinger JC. Long-term follow-up of acoustic schwannoma radiosurgery with marginal tumor doses of 12 to 13 Gy. Int J Radiat Oncol Biol Phys. (2007) 68:845–51.
15. Ouyang Z, Schoenhagen P, Wazni O, Tchou P, Saliba WI, Suh JH, et al. Analysis of cardiac motion without respiratory motion for cardiac stereotactic body radiation therapy. J Appl Clin Med Phys. (2020) 21:48–55.
16. Knutson NC, Samson PP, Hugo GD, Goddu SM, Reynoso FJ, Kavanaugh JA, et al. Radiation therapy workflow and dosimetric analysis from a phase 1/2 Trial of noninvasive cardiac radioablation for ventricular tachycardia. Int J Radiat Oncol Biol Phys. (2019) 104:1114–23. doi: 10.1016/j.ijrobp.2019.04.005
17. Loo BW Jr., Soltys SG, Wang L, Lo A, Fahimian BP, Iagaru A, et al. Stereotactic ablative radiotherapy for the treatment of refractory cardiac ventricular arrhythmia. Circ Arrhythm Electrophysiol. (2015) 8:748–50.
18. Cvek J, Neuwirth R, Knybel L, Molenda L, Otahal B, Pindor J, et al. Cardiac radiosurgery for malignant ventricular tachycardia. Cureus. (2014) 6:e190.
19. Haskova J, Peichl P, Pirk J, Cvek J, Neuwirth R, Kautzner J. Stereotactic radiosurgery as a treatment for recurrent ventricular tachycardia associated with cardiac fibroma. HeartRhythm Case Rep. (2019) 5:44–7. doi: 10.1016/j.hrcr.2018.10.007
20. Jumeau R, Ozsahin M, Schwitter J, Vallet V, Duclos F, Zeverino M, et al. Rescue procedure for an electrical storm using robotic non-invasive cardiac radio-ablation. Radiother Oncol. (2018) 128:189–91. doi: 10.1016/j.radonc.2018.04.025
21. Krug D, Blanck O, Demming T, Dottermusch M, Koch K, Hirt M, et al. Stereotactic body radiotherapy for ventricular tachycardia (cardiac radiosurgery) : First-in-patient treatment in Germany. Strahlenther Onkol. (2020) 196:23–30. doi: 10.1007/s00066-019-01530-w
22. Zeng LJ, Huang LH, Tan H, Zhang HC, Mei J, Shi HF, et al. Stereotactic body radiation therapy for refractory ventricular tachycardia secondary to cardiac lipoma: a case report. Pacing Clin Electrophysiol. (2019) 42:1276–9. doi: 10.1111/pace.13731
23. Marti-Almor J, Jimenez-Lopez J, Rodriguez de Dios N, Tizon H, Valles E, Algara M. Noninvasive ablation of ventricular tachycardia with stereotactic radiotherapy in a patient with arrhythmogenic right ventricular cardiomyopathy. Rev Esp Cardiol (Engl Ed). (2020) 73:97–9. doi: 10.1016/j.rec.2019.06.004
24. Mayinger M, Kovacs B, Tanadini-Lang S, Ehrbar S, Wilke L, Chamberlain M, et al. First magnetic resonance imaging-guided cardiac radioablation of sustained ventricular tachycardia. Radiother Oncol. (2020) 152:203–7. doi: 10.1016/j.radonc.2020.01.008
25. Wang L, Fahimian B, Soltys SG, Zei P, Lo A, Gardner EA, et al. Stereotactic arrhythmia radioablation (STAR) of ventricular tachycardia: a treatment planning study. Cureus. (2016) 8:e694.
26. Neuwirth R, Cvek J, Knybel L, Jiravsky O, Molenda L, Kodaj M, et al. Stereotactic radiosurgery for ablation of ventricular tachycardia. Europace. (2019) 21:1088–95.
27. Lloyd MS, Wight J, Schneider F, Hoskins M, Attia T, Escott C, et al. Clinical experience of stereotactic body radiation for refractory ventricular tachycardia in advanced heart failure patients. Heart Rhythm. (2020) 17:415–22. doi: 10.1016/j.hrthm.2019.09.028
28. Gianni C, Rivera D, Burkhardt JD, Pollard B, Gardner E, Maguire P, et al. Stereotactic arrhythmia radioablation for refractory scar-related ventricular tachycardia. Heart Rhythm. (2020) 17:1241–8.
29. Ho LT, Chen JL, Chan HM, Huang YC, Su MY, Kuo SH, et al. First Asian population study of stereotactic body radiation therapy for ventricular arrhythmias. Sci Rep. (2021) 11:10360. doi: 10.1038/s41598-021-89857-2
30. Yugo D, Lo LW, Wu YH, Chung FP, Lin YJ, Chang SL, et al. Case series on stereotactic body radiation therapy in non-ischemic cardiomyopathy patients with recurrent ventricular tachycardia. Pacing Clin Electrophysiol. (2021) 44:1085–93. doi: 10.1111/pace.14254
31. Lee J, Bates M, Shepherd E, Riley S, Henshaw M, Metherall P, et al. Cardiac stereotactic ablative radiotherapy for control of refractory ventricular tachycardia: initial UK multicentre experience. Open Heart. (2021) 8:e001770. doi: 10.1136/openhrt-2021-001770
32. Chin R, Hayase J, Hu P, Cao M, Deng J, Ajijola O, et al. Non-invasive stereotactic body radiation therapy for refractory ventricular arrhythmias: an institutional experience. J Interv Card Electrophysiol. (2021) 61:535–43. doi: 10.1007/s10840-020-00849-0
33. Qian PC, Quadros K, Aguilar M, Wei C, Boeck M, Bredfeldt J, et al. Substrate modification using stereotactic radioablation to treat refractory ventricular tachycardia in patients with ischemic cardiomyopathy. JACC Clin Electrophysiol. (2022) 8:49–58. doi: 10.1016/j.jacep.2021.06.016
34. Boerma M, Sridharan V, Mao XW, Nelson GA, Cheema AK, Koturbash I, et al. Effects of ionizing radiation on the heart. Mutat Res Rev Mutat Res. (2016) 770(Pt B):319–27.
35. Sharma A, Wong D, Weidlich G, Fogarty T, Jack A, Sumanaweera T, et al. Noninvasive stereotactic radiosurgery (CyberHeart) for creation of ablation lesions in the atrium. Heart Rhythm. (2010) 7:802–10. doi: 10.1016/j.hrthm.2010.02.010
36. Xu P, Yi Y, Luo Y, Liu Z, Xu Y, Cai J, et al. Radiationinduced dysfunction of energy metabolism in the heart results in the fibrosis of cardiac tissues. Mol Med Rep. (2021) 24:842. doi: 10.3892/mmr.2021.12482
37. Dogan I, Sezen O, Sonmez B, Zengin AY, Yenilmez E, Yulug E, et al. Myocardial perfusion alterations observed months after radiotherapy are related to the cellular damage. Nuklearmedizin. (2010) 49:209–15. doi: 10.3413/nukmed-0315-10-05
38. Song J, Yan R, Wu Z, Li J, Yan M, Hao X, et al. (13)N-Ammonia PET/CT detection of myocardial perfusion abnormalities in beagle dogs after local heart irradiation. J Nucl Med. (2017) 58:605–10.
39. Lehmann HI, Deisher AJ, Takami M, Kruse JJ, Song L, Anderson SE, et al. External arrhythmia ablation using photon beams: ablation of the atrioventricular junction in an intact animal model. Circ Arrhythm Electrophysiol. (2017) 10:e004304. doi: 10.1161/CIRCEP.116.004304
40. Leavitt JA, Stafford SL, Link MJ, Pollock BE. Long-term evaluation of radiation-induced optic neuropathy after single-fraction stereotactic radiosurgery. Int J Radiat Oncol Biol Phys. (2013) 87:524–7. doi: 10.1016/j.ijrobp.2013.06.2047
41. Sharma NK, Stone S, Kumar VP, Biswas S, Aghdam SY, Holmes-Hampton GP, et al. Mitochondrial degeneration and autophagy associated with delayed effects of radiation in the mouse brain. Front Aging Neurosci. (2019) 11:357. doi: 10.3389/fnagi.2019.00357
42. Jordan J, Cena V, Prehn JH. Mitochondrial control of neuron death and its role in neurodegenerative disorders. J Physiol Biochem. (2003) 59:129–41.
43. Jing CH, Wang L, Liu PP, Wu C, Ruan D, Chen G. Autophagy activation is associated with neuroprotection against apoptosis via a mitochondrial pathway in a rat model of subarachnoid hemorrhage. Neuroscience. (2012) 213:144–53. doi: 10.1016/j.neuroscience.2012.03.055
44. Yang K, Palm J, Konig J, Seeland U, Rosenkranz S, Feiden W, et al. Matrix-Metallo-Proteinases and their tissue inhibitors in radiation-induced lung injury. Int J Radiat Biol. (2007) 83:665–76. doi: 10.1080/09553000701558977
45. Shaw E, Scott C, Souhami L, Dinapoli R, Kline R, Loeffler J, et al. Single dose radiosurgical treatment of recurrent previously irradiated primary brain tumors and brain metastases: final report of RTOG protocol 90-05. Int J Radiat Oncol Biol Phys. (2000) 47:291–8. doi: 10.1016/s0360-3016(99)00507-6
46. Zhang DM, Navara R, Yin T, Szymanski J, Goldsztejn U, Kenkel C, et al. Cardiac radiotherapy induces electrical conduction reprogramming in the absence of transmural fibrosis. Nat Commun. (2021) 12:5558. doi: 10.1038/s41467-021-25730-0
47. Darby SC, Ewertz M, McGale P, Bennet AM, Blom-Goldman U, Bronnum D, et al. Risk of ischemic heart disease in women after radiotherapy for breast cancer. N Engl J Med. (2013) 368:987–98.
48. Hohmann S, Deisher AJ, Suzuki A, Konishi H, Rettmann ME, Merrell KW, et al. Left ventricular function after noninvasive cardiac ablation using proton beam therapy in a porcine model. Heart Rhythm. (2019) 16:1710–9. doi: 10.1016/j.hrthm.2019.04.030
49. Blanck O, Buergy D, Vens M, Eidinger L, Zaman A, Krug D, et al. Radiosurgery for ventricular tachycardia: preclinical and clinical evidence and study design for a German multi-center multi-platform feasibility trial (RAVENTA). Clin Res Cardiol. (2020) 109:1319–32. doi: 10.1007/s00392-020-01650-9
Keywords: refractory, ablative, radiosurgery, stereotactic body radiation therapy, ventricular tachycardia, volumetric-modulated arc therapy
Citation: Huang S-H, Wu Y-W, Shueng P-W, Wang S-Y, Tsai M-C, Liu Y-H, Chuang W-P, Lin H-H, Tien H-J, Yeh H-P and Hsieh C-H (2022) Case report: Stereotactic body radiation therapy with 12 Gy for silencing refractory ventricular tachycardia. Front. Cardiovasc. Med. 9:973105. doi: 10.3389/fcvm.2022.973105
Received: 28 June 2022; Accepted: 17 October 2022;
Published: 03 November 2022.
Edited by:
Simone Savastano, San Matteo Hospital Foundation (IRCCS), ItalyReviewed by:
Yu-ling Ma, University of Oxford, United KingdomChristian Graeff, Helmholtz Association of German Research Centres (HZ), Germany
Copyright © 2022 Huang, Wu, Shueng, Wang, Tsai, Liu, Chuang, Lin, Tien, Yeh and Hsieh. This is an open-access article distributed under the terms of the Creative Commons Attribution License (CC BY). The use, distribution or reproduction in other forums is permitted, provided the original author(s) and the copyright owner(s) are credited and that the original publication in this journal is cited, in accordance with accepted academic practice. No use, distribution or reproduction is permitted which does not comply with these terms.
*Correspondence: Chen-Hsi Hsieh, Y2hlbmljYWJAZ21haWwuY29t, Y2hlbmNpMjhAeW0uZWR1LnR3