- 1Department of Cardiovascular and Thoracic Sciences, Catholic University of the Sacred Heart, Rome, Italy
- 2Maria Cecilia Hospital, Gruppo Villa Maria (GVM) Care and Research, Cotignola, Italy
- 3Department of Cardiovascular and Thoracic Sciences, Fondazione Policlinico Universitario Agostino Gemelli Istituto di Ricovero e Cura a Carattere Scientifico (IRCCS), Rome, Italy
- 4Sports Medicine Unit, Fondazione Policlinico Universitario Agostino Gemelli Istituto di Ricovero e Cura a Carattere Scientifico (IRCCS), Rome, Italy
Myocardial bridging (MB) is the most frequent congenital coronary anomaly in which a segment of an epicardial coronary artery takes a tunneled course under a bridge of the myocardium. This segment is compressed during systole, resulting in the so-called “milking effect” at coronary angiography. As coronary blood flow occurs primarily during diastole, the clinical relevance of MB is heterogeneous, being usually considered an asymptomatic bystander. However, many studies have suggested its association with myocardial ischemia, anginal symptoms, and adverse cardiac events. The advent of contemporary non-invasive and invasive imaging modalities and the standardization of intracoronary functional assessment tools have remarkably improved our understanding of MB-related ischemia, suggesting the role of atherosclerotic lesions proximal to MB, vasomotor disorders and microvascular dysfunction as possible pathophysiological substrates. The aim of this review is to provide a contemporary overview of the pathophysiology and of the non-invasive and invasive assessment of MB, in the attempt to implement a case-by-case therapeutic approach according to the specific endotype of MB-related ischemia.
Introduction
Myocardial Bridging (MB) is the most common inborn coronary artery variant in which a segment of an epicardial coronary artery, most frequently (70–98%) the left anterior descending (LAD) coronary artery, takes an intramural course under a bridge of myocardium (1).
Coronary angiography (CA) has been typically considered the gold standard for the diagnosis of MB, detecting the “milking effect” induced by the systolic compression of the intramural artery in addition to its delayed diastolic relaxation (2–4). Nevertheless, CA may underestimate the presence of MB, whose incidence depends on the modality used to identify the tunneled segment. Indeed, MB has been documented from 5 to 8% of invasive angiographic series, but from 18 to 25% or 30 to 55% when using coronary computed tomography angiography (CCTA) or autopsy reports, respectively (5).
As coronary blood flow occurs primarily during diastole, the clinical relevance of MB is still a matter of debate, being typically considered as an innocent bystander (6). However, several studies documented that patients with MB experienced a high burden of anginal symptoms, angina-equivalents (i.e., dyspnea) and, less frequently, palpitations and/or ventricular arrhythmias (7). Although this anomaly is present at birth, the onset of symptoms usually does not occur before the third decade of age. Several factors, such as concomitant coronary artery disease (CAD), tachycardia, and the rise in left ventricular (LV) pressures, usually associated with aging, diastolic dysfunction and LV hypertrophy, may worsen the supply-demand mismatch imposed by MB and unmask or exacerbate the hemodynamic impact of MB (8, 9).
Interestingly, MB patients may also present with acute coronary syndrome (ACS), as a result of coronary artery spasm (CAS), coronary artery dissection, or coronary artery thrombosis (10).
Nowadays, a growing body of evidence suggests a direct association between MB and myocardial ischemia. Moreover, MB has been recently recognized as a cause of ischemia with non-obstructive coronary artery disease (INOCA) (11), and several mechanisms have been described as possible pathophysiological substrates of MB-related ischemia (8, 10, 12, 13; Figure 1).
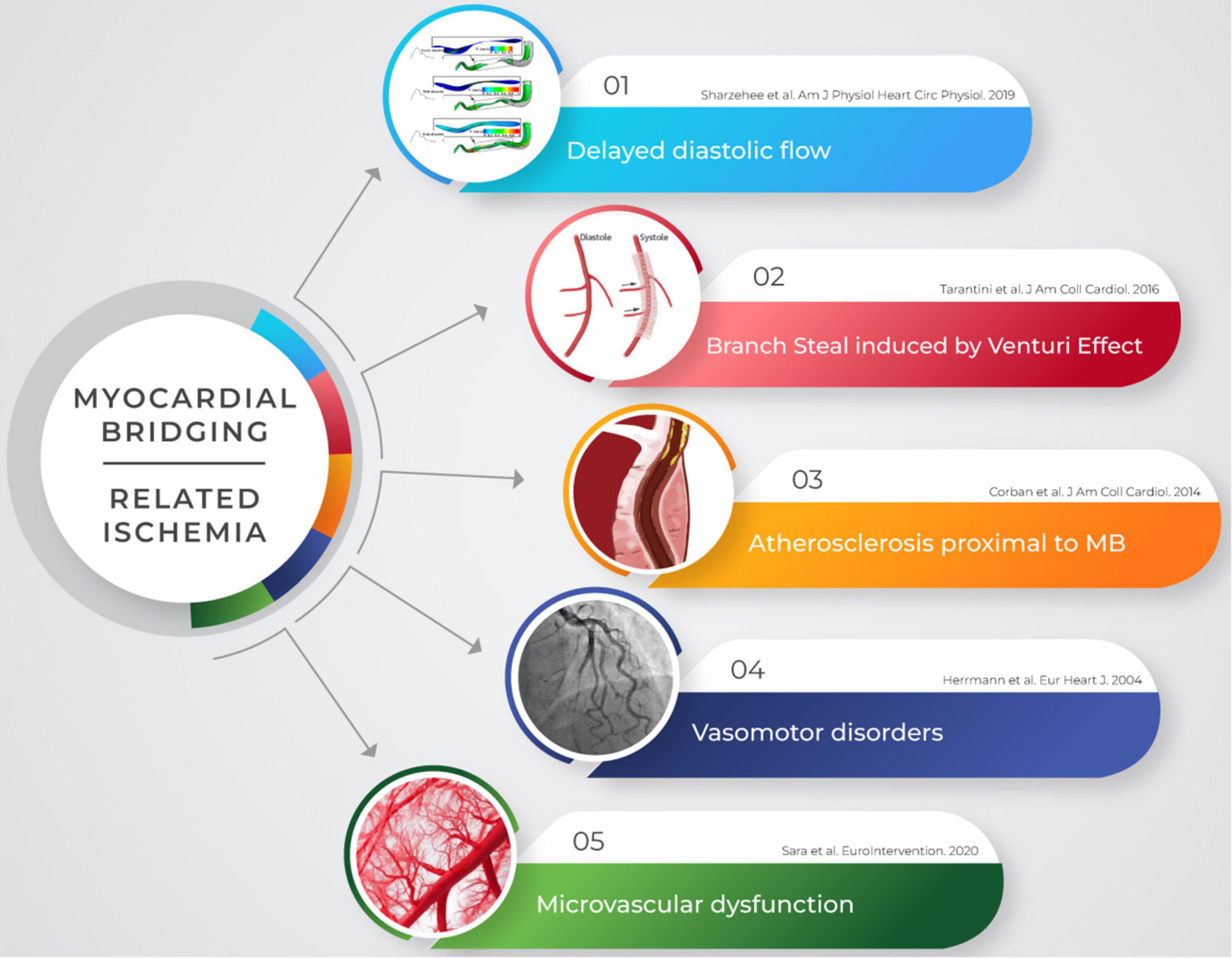
Figure 1. Myocardial ischemia is not purely related to vessel systolic compression in patients with MB. Several mechanisms may account for the occurrence of symptoms and may be detected though invasive and non-invasive modalities. MB, myocardial bridging.
Nevertheless, these multiple mechanisms cannot be unmasked by a single diagnostic modality (Table 1).
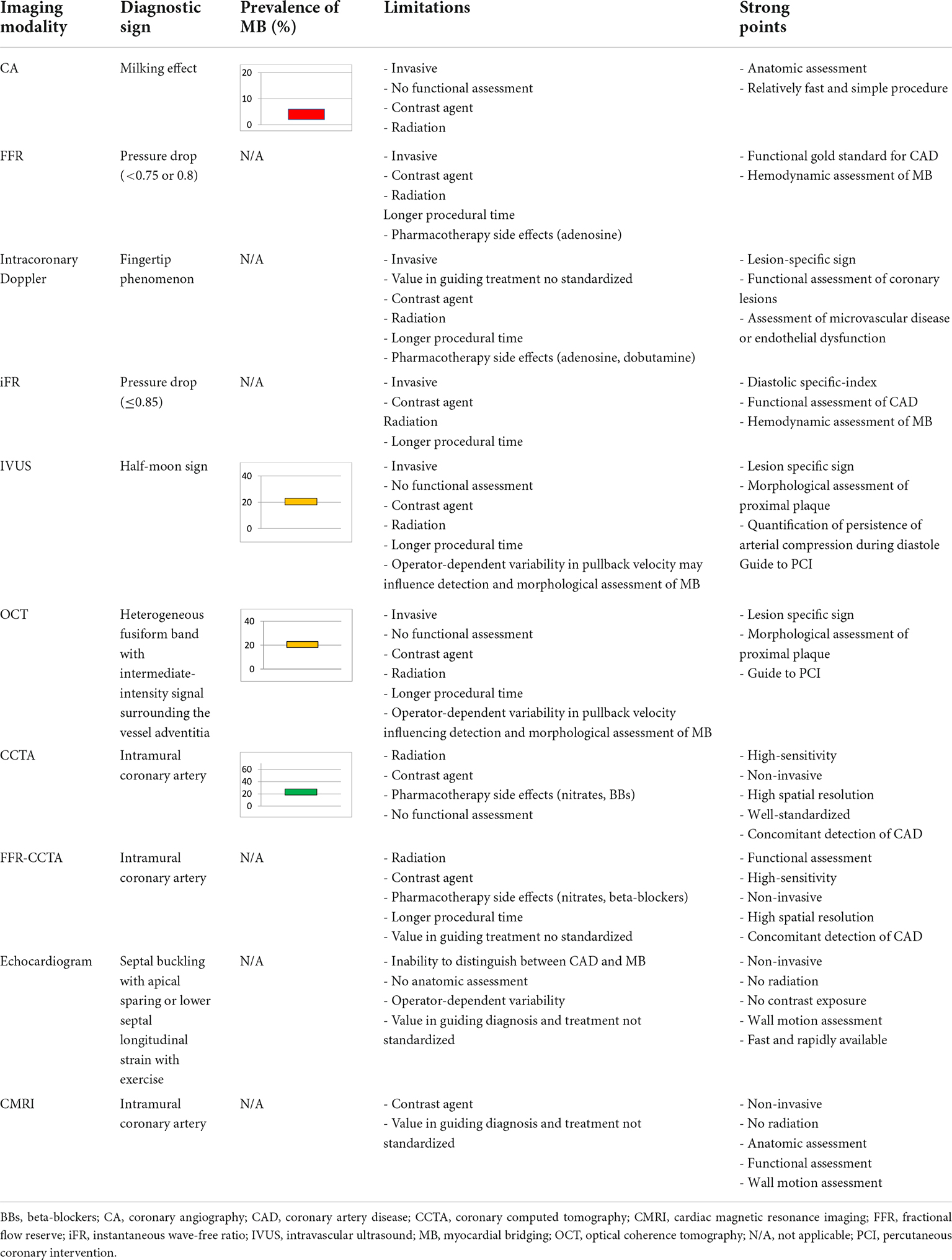
Table 1. Invasive and non-invasive diagnostic modalities for the detection, anatomic and functional assessment of MB.
The aim of our review is to show the role of different diagnostic strategies (both non-invasive and invasive) in the anatomical and functional assessment of MB. A comprehensive approach might play a key role in implementing personalized medical or invasive therapeutic strategies, ultimately yielding a benefit on symptoms and reducing the occurrence of adverse cardiac events (13, 14).
Mechanisms of myocardial ischemia and clinical relevance in patients with myocardial bridging
The systolic phase of the myocardial cycle is only marginally involved in the myocardial perfusion (∼15%) (1). Nonetheless, an increasing body of evidence supports the involvement of different mechanisms of ischemia in patients with MB: delayed early diastolic artery relaxation, development of atherosclerotic stenosis proximal to MB, functional disorders of the coronary circulation (i.e., impaired endothelium-dependent vasodilatation and microvascular dysfunction) and the “branch steal” phenomenon.
The assumption that MB affects coronary blood flow during systole has been overcome since the diastolic lumen gain may be late and incomplete once the systolic compression of the tunneled artery ends (15–18). In 1993, Erbel et al. were the first to describe the delayed early diastolic artery relaxation within the tunneled segment through angiography and IVUS (19). Subsequently, other IVUS studies confirmed the same phenomenon, showing the concordance between delayed diastolic relaxation, increased intracoronary Doppler flow velocity, and ischemic symptoms and signs (20). Furthermore, stress-echocardiography (SE) studies also suggested that slow and incomplete MB decompression may underlie stress-induced ischemia in MB patients (18).
Myocardial bridging-related ischemia is not to be exclusively sought in the systo-diastolic hemodynamic modifications imposed by MB, but also in the anatomical and/or functional anomalies of the coronary circulation that may coexist or be favored by the MB itself. In this regard, an association between MB and CAD has long been described (8, 13). A local systolic retrograde flow phenomenon proximal to MB has been detected, predisposing to the development of atherosclerosis (21). Furthermore, the compression-relaxation of the intramural segment induces changes in wall shear stress (WSS), producing an area of low WSS proximal to MB (21). Low WSS induces the release of inflammatory mediators and endothelial vasoactive agents, whose levels were found to be significantly higher in the proximal segment compared with the intramural segment (2, 22). Using a parametric finite element model, Nikoliæ et al. found a correlation between the position of plaque near MB with WSS and oscillatory shear index. This finding reinforces the notion that plaque progression may be favored by hemodynamic disturbances provoked by MB (23).
On the contrary, the intramural tract is typically spared from atherosclerosis, probably because of the “separation” of the tunneled segment from epicardial adipose tissue and its pro-inflammatory signals (i.e., cytokines and adipokines) (24–26) as shown in preclinical models by the lack of foam cells and modified smooth muscle cells in the intramural artery (27).
The presence of atherosclerotic plaques proximal to MB is a potential cause of chronic coronary syndrome (CCS) (10, 15–17), as well as ACS due to plaque erosion/rupture or vasospasm and coronary dissection (10, 28–31).
Myocardial bridging has also been associated with impaired endothelium-dependent vasodilatation (32–36). Endothelial dysfunction, together with the hyper-reactivity of vascular smooth muscle cells, represent the two pathophysiological mechanisms implicated in the occurrence of CAS (37). Previous studies, using a provocative test with incremental acetylcholine (ACH) dose infusion, demonstrated that CAS is more common in patients with MB (32–36). Furthermore, anatomical properties of MB, such as length and percentage of systolic compression, were demonstrated to predict the occurrence of provoked LAD spasm (32, 34).
It has been proposed that the turbulent flow and changes in WSS, due to MB, may promote direct injury to the endothelium and endothelial cell apoptosis (33, 35). Moreover, the expression of endothelial nitric oxide synthase (eNOS), a marker of preserved endothelial function, is significantly lower in the MB segment than in proximal and distal segments (36). These mechanisms ultimately converge to a paradoxical response to ACH and an increased risk of CAS (36). The occurrence of CAS in MB patients may have significant clinical relevance: Nam et al. reported that patients with MB and CAS experienced a higher rate of recurrent angina and a more frequent prescription of anti-anginal medication (32). Furthermore, according to the correlation between MB and myocardial infarction risk (38), CAS may also represent a mechanism underlying the development of ACS (39).
Endothelial dysfunction is, however, not confined to the epicardial coronary artery but may extend to the coronary microcirculation (11). Indeed, it is not unlikely that impaired endothelium-dependent vasodilatation affects also distal arterioles, resulting in microvascular spasm as well as spasm of the epicardial tract strictly close to MB (40).
Nowadays, little data is available on the link between MB and coronary microvascular dysfunction (CMD). CMD is prevalent across several cardiovascular conditions, emerging as an increasing cause of INOCA (41, 42). Two different endotypes of microvascular dysfunction currently exists: structural microvascular remodeling and functional arteriolar dysregulation (11). The former is caused by an increase in wall to lumen ratio and a loss of myocardial capillary density, and it is characterized by an impaired endothelium-independent vasodilatation, represented by a reduced coronary flow reserve (CFR) and an increased index of microcirculatory resistance (IMR) (11). The latter is caused by endothelial dysfunction of medium and large size arterioles, with a pathological response to ACH test (impaired endothelium-dependent vasodilatation) (11).
Only two studies investigated the presence of CMD in patients with MB (40, 43). Among patients with chest pain and non-obstructive CAD, Sara et al. showed that those with angiographic evidence of MB had a higher frequency of microvascular endothelial dysfunction compared to patients without MB (57.7 vs. 51.0%, p = 0.075). However, this reached statistical significance among patients aged ≤ 50 years (57.3 vs. 44.2%, p = 0.010) (40). The second study showed a high rate of CMD (22.1%) among patients with persistent angina, non-obstructive CAD and angiographic evidence of MB (43). On the basis of these results, CMD may represent an additional mechanism of ischemia in patients with MB. Therefore, a comprehensive invasive assessment of MB patients that includes the evaluation of coronary microcirculation (i.e., IMR and CFR) might provide additional information. However, further data are needed to suspect a more marked involvement of microvascular dysfunction in these patients.
Lastly, the “branch steal effect” is an additional ischemic mechanism in MB patients: the crossing of blood through the constrict segment in the end systole/early diastole leads to an increase in diastolic flow velocity (“Venturi effect”) that results in a depressurization at the ostium of side branches within the MB (44, 45). This phenomenon is more evident in the case of septal perforator arteries of the LAD coronary artery (7). Interestingly, Lin et al. proposed this mechanism to explain the echocardiographic finding of “septal wall motion abnormality” during dobutamine stress test as sign of focal ischemia. Conversely, the recovery of perfusion pressure distal to the LAD was not associated with ischemic signs (i.e., echocardiographic finding of “apical sparing”) (44).
In summary, several mechanisms suggest the ischemic relevance of MB: exertion-induced angina may occur for a delay in early diastolic artery relaxation, “branch steal,” hemodynamically significant proximal CAD and CMD. On the other hand, epicardial spasm, coronary artery dissection, and coronary artery thrombosis may underlie the development of ACS.
Non-invasive anatomical and functional assessment
Although CA may detect the presence of MB, there are specific patient-dependent and procedure-related complications that are inherent to the invasive procedure (46). Some non-invasive imaging modalities may be useful to detect MB and to investigate myocardial ischemia in MB patients, since this cohort of patients is typically younger, has a lower rate of cardiovascular risk factors and, therefore, a low pre-test probability of CAD (47).
Among non-invasive imaging tests, CCTA plays a prominent role. Its strength is represented by a high spatial resolution, accounting for a higher detection of MB compared with CA (48). CCTA enables an accurate evaluation of the vessel wall and lumen, as well as the surrounding myocardium, allowing to classify MB of the LAD coronary artery in terms of depth and length, suitable only for MB located in the LAD coronary artery (49). In this regard, MB is classifiable into superficial (<2 mm), deep (≥2 mm), and very deep (≥5 mm) according to the depth; and into short (<25 mm) or long (≥25 mm) according to the length of the tunneled segment (49, 50). Kim et al. proposed an alternative classification of LAD MB into three types: the type I, characterized by a partial muscular encasement; the type II, characterized by a full encasement of the vessel by myocardium but without measurable overlying myocardium; the type III, characterized by a measurable overlying myocardium > 0.7 mm (48).
These anatomical evaluations have relevant clinical, prognostic and therapeutical implications: indeed, the deeper variant is more commonly associated with symptoms and adverse cardiac events; moreover, CCTA may be helpful to guide the choice of surgical treatment (myotomy vs. CABG) (13, 51).
The pivotal limitation of CCTA is the low temporal resolution, making difficult to assess the hemodynamic significance of MB. Moreover, the use of a “cocktail” of drugs before scanning, such as beta-blockers and nitroglycerin that prolong diastolic time and induce vasodilation, respectively, results in an underestimation of the impairment in blood flow imposed by MB (13).
Among non-invasive tests, echocardiogram plays a role in the functional assessment of MB patients. Lin et al. described an echocardiographic finding characterized by a focal abnormality in the end-systolic to early-diastolic septal wall motion with apical sparing (44). Eighteen patients with angina and this sign were prospectively enrolled for invasive assessment (CA, IVUS, and intracoronary pressure measurements): all these patients were found to have MB of the LAD coronary artery and an abnormal diastolic-fractional flow reserve (dFFR ≤ 0.75) (44). In addition, patients with hemodynamically significant LAD MB present lower septal longitudinal strain compared to controls during stress echocardiographic strain imaging (52). “Septal buckling with apical sparing” has a diagnostic accuracy similar to SE in identifying significant CAD through a new wall motion abnormality (52). However, even though SE is a well-established diagnostic tool in detecting myocardial ischemia, its key limitation is related to the impossibility to understand if the wall motion abnormalities are attributable to MB, CAD or other pathological conditions, if an anatomical assessment is not performed.
The evaluation of coronary flow velocity reserve (CFVR) measured by transthoracic Doppler echocardiography (TTDE) has been found to represent another modality to hemodynamically assess LAD MB. In this regard, Aleksandric et al. showed that non-invasive CFVR-TTDE during dobutamine infusion was a predictor of functional significant MB, found to be more accurate than CFVR during adenosine infusion (18, 53). A cut-off value of ≤2.1 was found to have the best accuracy for identifying MB associated with stress-induced myocardial ischemia with a sensitivity (Sn), specificity (Sp), positive (PPV), and negative predictive value (NPV) of 96, 95, 88, and 98%, respectively (area under curve AUC 0.986) (53). Inotropic stimulation with dobutamine, compared to vasodilatation with adenosine, provides better significance of MB in relation to stress-induced myocardial ischemia in the invasive setting too (4). Interestingly, the cut-off value of ≤2.1 is similar to the cut-off value for TTDE-CFVR during adenosine as well as during dobutamine provocation in the functional assessment of fixed coronary stenosis (Sn 92 vs. 92%; Sp 90 vs. 86%; PPV 85 vs. 73%; NPV 95 vs. 96%) (54, 55).
Among non-invasive diagnostic modalities, cardiovascular magnetic resonance imaging (CMRI), single-photon emission computed tomography (SPECT), and positron emission tomography (PET) provide the quantification of myocardial blood flow at rest and during induced-hyperemia, representing possible tools in the assessment of inducible-ischemia and CMD in MB patients (41).
However, little data supported the role of these modalities in detecting ischemia in patients with MB and anginal symptoms, and there are no standardized diagnostic criteria for functional evaluation of MB with non-invasive stress testing or myocardial perfusion imaging because previous studies were based on small number of patients (56). Further studies are needed to better validate these tools.
Invasive anatomical and functional assessment
Intracoronary imaging
If we consider the gap in incidence rate between CA and other imaging modalities, routine CA is not completely sensitive for the detection of MB (5). The use of intracoronary vasodilators (i.e., nitrates) can increase, through a reflex rise of the adrenergic drive, the systolic narrowing of the tunneled artery and the angiographic sensitivity in diagnosing MB (57). Nevertheless, in patients with thin MB, the “milking effect” may be missed and invasive intracoronary imaging techniques may be required to limit the underdiagnose of MB.
Intravascular ultrasound (IVUS) has been used throughout a multitude of studies for the morphological assessment of MB. It allows for accurate measurement of the lumen diameter and evaluation of vessel wall morphology (58). IVUS is able to detect the systolic compression of the bridged segment, but the peculiar finding is an echolucent area between the tunneled artery and the epicardial tissue, persisting throughout the cardiac cycle. This phenomenon is called “half-moon” sign and represents a muscle band overlying the tunneled segment (1). This sign is highly specific because it is detectable only in the segment with systolic compression (59). Moreover, IVUS confirms that vessel compression within MB is not exclusively a systolic event but it extends to early diastole (59). Additionally, IVUS is a useful tool for the quantitative and qualitative assessment of atherosclerosis proximal to MB. In this regard, Yamada et al. found that the percentage of arterial compression was directly related to the atherosclerotic burden located proximally to MB (60).
Similarly, optical coherence tomography (OCT) may represent an adjuvant tool in the diagnosis of MB, detecting angiographically undetectable MB (61). OCT is a light-based technique that provides in vivo high-resolution (∼10 μm) imaging of coronary artery (62). Although its resolution is higher than IVUS, the role of OCT in detecting MB has not been completely elucidated. Some studies proposed the main OCT features of MB, describing a heterogeneous fusiform band with sharp borders and low/intermediate-intensity signal, similar to tunica media, surrounding the vessel adventitia. Obviously, the fusiform band detected by OCT, corresponds to the “half-moon” sign using IVUS (63–67). OCT provides the detection and anatomic characterization of atherosclerotic lesions proximal to MB too (64). Furthermore, in cases of ACS and concomitant presence of MB, OCT helps in differentiating between different pathophysiological subtypes, such as plaque rupture/erosion, thrombosis or spontaneous coronary artery dissection (SCAD) (30). Finally, despite weak evidence, the use of OCT might help in guiding percutaneous revascularization, with the aim of minimizing peri- and post-procedural complications during stent implantation in a MB segment (63).
The key limitation of IVUS and OCT in the assessment of MB is related to the lack of functional and hemodynamic information on both MB and proximal atherosclerotic lesions. Furthermore, they increased procedural cost and the risk of underestimating the length of MB when rapid pullback is performed (Table 1).
In conclusion, the use of invasive anatomical assessment with imaging tools such as IVUS and OCT allows to maximize the diagnosis of MB, quantify arterial compression, characterize the coexisting proximal CAD, and identify the mechanism underlying the occurrence of ACS.
Physiological assessment of myocardial bridging
Functional assessment tools should be considered complementary to imaging tests. In this regard, the Doppler flow wire and pressure wire methods represent useful modalities to define the hemodynamic impact of MB (13; Figure 2).
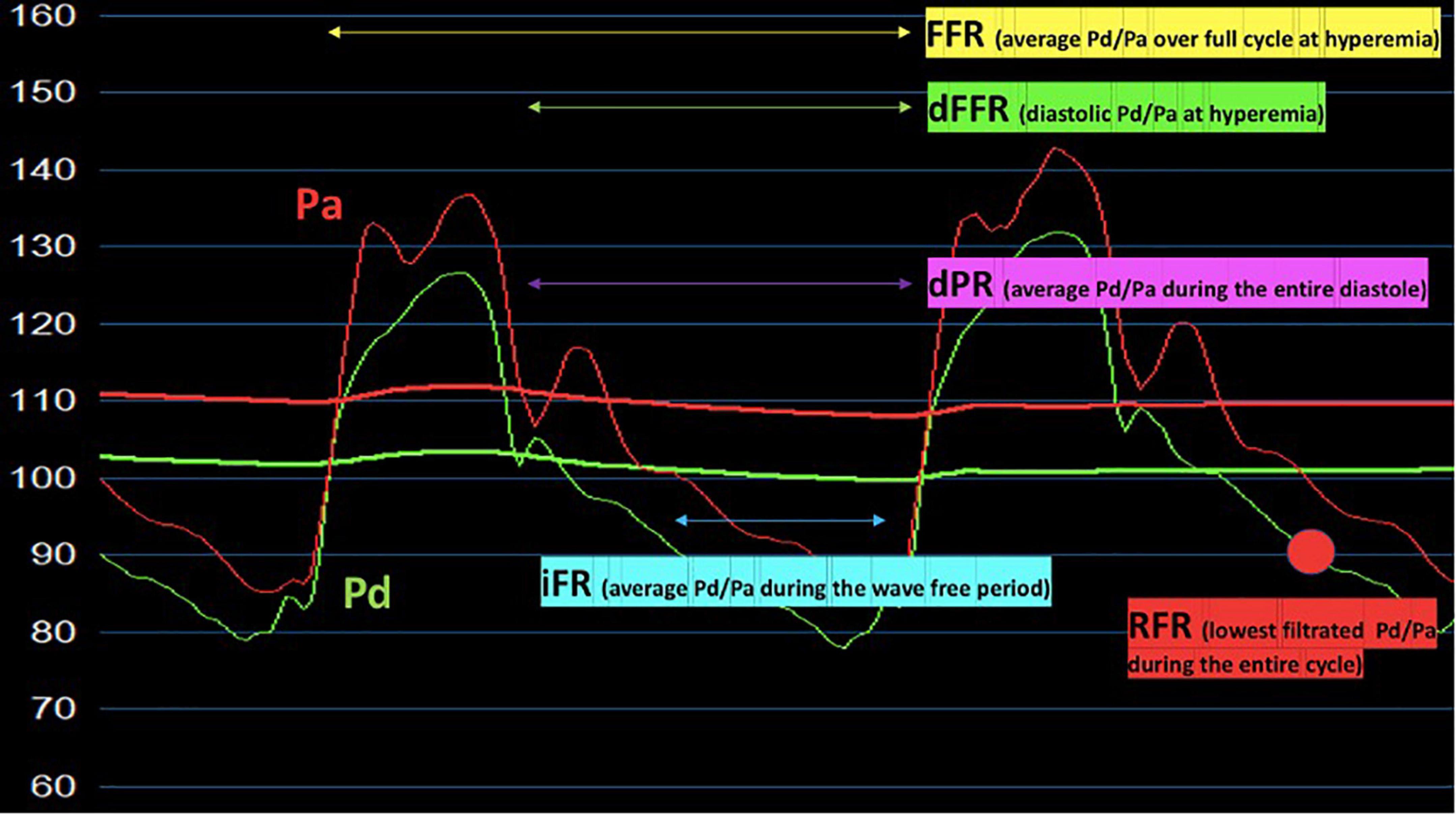
Figure 2. Hyperemic and non-hyperemic pressure ratios (FFR, dFFR, iFR, RFR, dPR) proposed for the invasive functional assessment of patients with myocardial bridging. Pa, aortic pressure; Pd, distal pressure; FFR, fractional flow reserve; dFFR, diastolic-fractional flow reserve; iFR, instantaneous wave-free pressure ratio; RFR, resting full-cycle ratio dPR, diastolic pressure ratio.
Fractional flow reserve (FFR) is a pressure wire-based index that, through the measurement of the trans-stenotic pressure gradient during maximal hyperemia (achieved by adenosine administration), is recommended to assess the hemodynamic relevance of intermediate-grade stenosis when evidence of ischemia is not available (68, 69). To date, 0.80 is the accepted FFR threshold for defining hemodynamically relevant fixed stenosis (68, 69).
Although FFR is generally considered the gold standard for the physiological invasive assessment of atherosclerotic plaque, it may fail to identify the hemodynamic impact of dynamic coronary obstructions such as MB (14). FFR is based on the assumption that the difference between mean and diastolic pressure gradient values across the lesion is not significant: this is true for fixed stenosis, but it might not be valid for dynamic flow obstruction (7, 14). MB reduces systolic pressure gradients, as a consequence of distal pressure overshooting during myocardial contraction. This leads to an overestimation of the mean pressure (evaluated by FFR) and to an underestimation of the hemodynamic significance of MB (7). Therefore, specific diastolic functional indices have been developed to overcome the limits of FFR (13), such as dFFR which has proven to be more sensitive than conventional FFR for functional assessment of MB (70).
Moreover, as MB is a dynamic stenosis deeply influenced by the degree of extravascular compression and intra-myocardial tension, the assessment during rest may underestimate the hemodynamic relevance of a significant proportion of MBs. Dobutamine represents the drug of choice for inotropic stimulation in MB patients (18). In this regard, Escaned et al. evaluated the usefulness and safety of combining dobutamine challenge with FFR and dFFR in the presence of MB. This investigation revealed that dobutamine challenge enhanced diagnostic sensitivity of both FFR and dFFR. Interestingly, dobutamine challenge increased the discrepancy between FFR and dFFR (70). This may be explained by the decreased and negativization of systolic pressure gradient across MB segment during dobutamine provocation, leading to an artificial and paradoxical elevation in the mean pressure gradient used by traditional FFR.
The diagnostic superiority of dFFR during dobutamine provocation, compared to conventional FFR during adenosine provocation, has been recently confirmed by Aleksandric et al. using exercise-induced myocardial ischemia (4). They proposed the cut-off value of ≤0.76 for dFFR during dobutamine provocation to identify stress-induced myocardial ischemia in MB patients with a Sn, Sp, PPV and NPV of 95, 95, 90, and 98%, respectively (AUC 0.927). Curiously, this value is the same as the cut-off value used for dFFR during adenosine provocation in the functional assessment of fixed coronary stenosis (Sn 96%; Sp 100%) (71).
However, despite the promising diagnostic value, dFFR is not routinely performed in clinical practice, due to the difficulty of execution and time-consuming.
Other non-hyperemic pressure indices, including instantaneous wave-free ratio (iFR), diastolic pressure ratio (dPR), and resting full-cycle ratio (RFR) have been developed. They are obtained without the administration of vasodilators, resulting easier to perform than dFFR.
Instantaneous wave-free ratio is a pressure-derived index, recently validated for the assessment of coronary artery stenosis. It is a specific-diastolic index, calculated during a portion of diastole when vascular resistance is low and stable, the so-called “wave-free period” (72). In a prospective study by Tarantini et al., among 20 patients with angina and/or positive non-invasive stress test, absence of CAD and angiographic evidence of MB, iFR was found to be more consistent with angina and/or inducible ischemia compared with FFR. Indeed, iFR at rest was abnormal (≤0.89) in 7 patients, while no MB was hemodynamically significant according to FFR. During inotropic challenge, median FFR did not change significantly, whereas dobutamine-induced iFR resulted to be remarkable lower compared to iFR at rest (73).
Collectively, diastolic indices may identify a proportion of hemodynamically relevant MBs that are not unmasked by conventional FFR. The superiority of diastolic indices over systo-diastolic indices (i.e., FFR) in the evaluation of ischemic burden related to MB reflects the fundamental involvement of diastole in the pathophysiology of MB-related ischemia (70, 74).
Nevertheless, the evaluation of iFR depends on the assumption that maximal flow and minimal microcirculatory resistance occur during a period within diastole (the “wave-free period”), while the hemodynamic modifications imposed by MB and concomitant CAD may be not completely predictable. In this regard, two alternative non-hyperaemic pressure indices might be proposed: dPR and RFR.
Diastolic pressure ratio is the ratio between mean distal coronary pressure averaged over the entire diastolic period and the mean aortic pressure (75). Instead, RFR is calculated from the lowest value of distal pressure (Pd) and aortic pressure (Pa) over the entire cardiac cycle (76). These indices may potentially unmask significant or multiple occlusions that would be missed by an assessment dedicated only to specific periods of the cardiac cycle (75, 76).
Unfortunately, ischemic cut-off values for the above-discussed indices, at rest and during inotropic provocation, are not available for MB patients. Further studies are needed for the validation of these tools and for the definition of reliable cut-off values.
The Doppler guide wire, through selective catheterization and measuring phasic flow velocity of MB segment, reveals a typical velocity pattern termed “fingertip” phenomenon, characterized by an abrupt early-diastolic acceleration, a rapid mid-diastolic deceleration and a mid-to-late diastolic plateau (10, 13). However, the absence of the “fingertip” was found in 13% of MB patients, probably because the systolic compression sometimes is not strong enough to induce the hemodynamic changes that lead to this sign (10). Further studies are needed to confirm these results and to determine whether this phenomenon, as a marker of MB severity, predicts adverse cardiovascular events.
Provocative test
It is well-known that endothelial dysfunction is a pathophysiological hallmark of MB-related ischemia, promoting the development of vasomotor disorders such as epicardial and microvascular CAS. In order to unmask endothelial dysfunction and CAS, three provocative tests (with ACH, ergonovine and hyperventilation) can be performed in the catheterization laboratory (77). ACH test is preferred over ergonovine and hyperventilation, because it is associated with a lower rate of complications compared to ergonovine, and it is more standardized and reproducible than hyperventilation (78). On the other hand, non-invasive provocative tests (i.e., ergonovine and hyperventilation) have been associated with significant adverse events including death, because detection and alleviation of the induced spasm may be delayed (79).
The 2019 ESC CCS guidelines support the use of intracoronary ACH test in patients with normal findings or non-obstructive lesions on coronary arteriography and clinical suspicion of CAS for the assessment of epicardial spasm (IIa recommendation) and/or microvascular spasm (IIb recommendation) (80–82). Therefore, interventional cardiologists should not refrain from performing provocative test in patients with MB and clinical picture of vasospastic angina.
Several studies investigated the relationship between CAS and MB, demonstrating that MB patients present a high rate of CAS. Furthermore, MB patients with a positive response to ACH test have a worse prognosis compared with those without spasm (10, 32–36, 83–88). However, the location of provoked spasm was not always the same: most of studies found epicardial spasm of the intramural segment. On the contrary, Saito et al. found that epicardial CAS was more frequently provoked in the proximal segment of MB (34). Moreover, heterogeneity in dose-response relationship has been noted in patients with MB and ACH-induced spasm. MB patients who responded to lower ACH doses (20 μg) have higher incidence of severe, diffuse and long (>30 mm) spasm. This results in a higher occurrence of adverse events at 12-month follow-up, compared to MB patients reacting to higher ACH doses (50 and 100 μg) (88).
Therefore, the use of ACH provocative test may be useful in the identification and quantification of a pathophysiological mechanism (impaired endothelium-dependent vasodilatation) of MB-related ischemia, with relevant prognostic and therapeutic implications.
Therapeutic management of patients with myocardial bridging
The therapeutic management of MB patients remains a relevant challenge. Treatment options should be considered according to clinical presentation, evidence and degree of inducible ischemia, coronary and cardiac anatomy, comorbidities and patient preference (13). Nevertheless, no guidelines or expert consensus are currently available.
As discussed, the ischemic burden related to MB is supported by various pathophysiological mechanisms. In this regard, invasive and non-invasive physiological assessment, intracoronary imaging, and provocative test may unmask the dominant mechanism of myocardial ischemia, allowing to guide the treatment according to specific pathophysiological endotypes of myocardial ischemia (Figure 3).
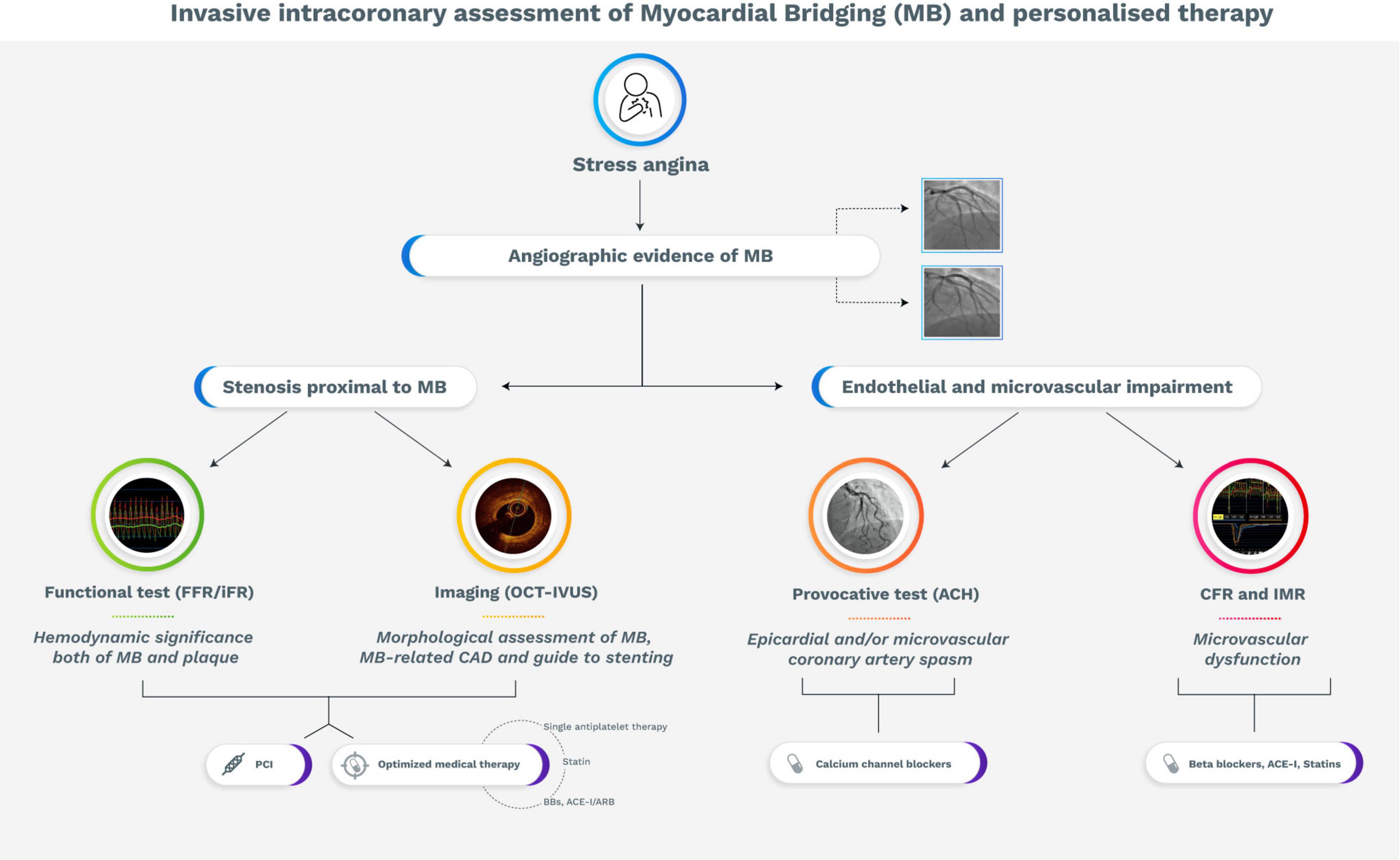
Figure 3. Flow diagram with proposed strategy for the management of symptomatic patients with myocardial bridging. ACE-I, Angiotensin-converting enzyme-inhibitors; Ach, acetylcholine; ARB, Angiotensin receptor blocker; BBs, beta-blockers; CAD, coronary artery disease; CFR, coronary flow reserve; FFR, fractional flow reserve; iFR, instantaneous wave-free ratio; IMR, index of microvascular resistance IVUS, intravascular ultrasound; MB, myocardial bridging; OCT, optical coherence tomography; PCI, percutaneous coronary intervention.
Pharmacologic therapy is considered the first strategy for most of symptomatic MB patients (8). Beta-blockers (BBs) represent the mainstay of treatment in patients with MB and ischemic symptoms or signs of inducible ischemia. Due to their negative chronotropic effect, BBs allow to increase diastolic perfusion and diastolic filling time. In addition, they reduce compression of the tunneled vessel by their negative inotropic effect (7). Similarly, non-dihydropyridine calcium channel blockers (CCBs) may satisfy the same target, and they should be preferred in patients with contraindications to betablockers (89).
However, BBs, especially non-selective ones, may be detrimental in MB patients with concomitant CAS. In fact, blockade of the beta-2 receptors, which mediate vasodilation, increases the risk of CAS and, supposedly, adverse cardiac events (90). On the contrary, CCBs, by combining their negative ino-chronotropic effect and vasodilatory effect, may represent the treatment of choice in MB patients with concomitant vasomotor disorders unmasked by a positive ACH test (13, 91). Conversely, nitrates, although powerful vasodilators, should be avoided since they worsen systolic narrowing and symptoms through a reflex rise of the adrenergic drive.
Since MB has been associated with an increased prevalence of CAD proximal to the bridged segment, optimized medical therapy of cardiovascular risk factors should be considered once atherosclerosis is detected (13).
If symptoms persist despite maximally tolerated medical therapy, a “revascularization” strategy should be considered. The choice among percutaneous coronary intervention (PCI), coronary artery bypass graft (CABG) or supra-arterial myotomy should be guided by anatomical features of MB (i.e., length and depth), age, concomitant CAD, and patient preference.
Randomized data are lacking for the use of PCI in the treatment of MB, and this approach was historically reserved to patients with anginal symptoms refractory to maximal medical therapy (92). Any rationale for PCI in this setting would be to treat stenosis proximal to MB as well as the dynamic obstruction within the tunneled segment, aiming at protecting it from systolic compression (8). However, PCI with stenting in MB patients may lead to a high rate of complications such as in-stent restenosis (ISR), very late stent thrombosis, and coronary perforation, due to the sustained stress over time within the intramural tract. These complications were described mainly in studies using bare-metal stent (BMS) and first-generation drug eluting stent (DES) (8, 63, 93–96). Probably, the use of second-generation DES or future bioabsorbable scaffolds may potentially limit these complications (13). Furthermore, the use of intracoronary imaging tools may provide accurate information about MB sizing, guiding the percutaneous treatment. In this regard, a recent retrospective study showed that the guidance of OCT limited the incidence of perforation and ISR in the MB segment covered with DES (63).
Given the MB stenting-related complications, surgery is an effective therapeutic alternative for symptomatic MB refractory to maximally tolerated medical therapy. Myotomy is the treatment of choice for MB with favorable anatomy (i.e., non-tortuous artery, short and superficial intra-myocardial course), especially in pediatric population (97). Conversely, myotomy presents a high rate of failure in adult patients, probably because abnormal flow related to MB causes damage to coronary circulation (i.e., endothelial dysfunction), persisting and impairing coronary flow after surgical intervention (5). CABG is superior to myotomy in case of complex anatomy (i.e., deep and/or long MB) and adult patients (51).
Unfortunately, studies assessing short- and long-term effect of antianginal drugs vs. PCI or surgical treatment in MB patients are lacking. Large and prospective randomized clinical trials are needed in this regard.
Conclusion
Myocardial bridging has long been considered an accidental finding. However, a growing body of evidence suggested that it is associated with impaired quality of life and might lead to adverse cardiac events.
Invasive intracoronary assessment, via imaging techniques (i.e., OCT and IVUS), or full invasive physiological evaluation (i.e., FFR, iFR, CFR, IMR), together with the utilization of provocative test (i.e., ACH test), improved the ability to evaluate the hemodynamic relevance of MB, to understand the underlying pathophysiological mechanisms, leading to an optimization of the therapy according to each specific endotypes.
Nevertheless, the uncertainties we are currently facing in the diagnosis, characterization, and treatment of patients presenting with MB and evidence of ischemia are remarkable, and related, at least in part, to the skepticism and the paucity of evidence that support the clinical and prognostic relevance of MB in the setting of CCS and ACS. Therefore, there is an unmet need in defining unequivocally the diagnostic tools and the related cut-offs to be used, guiding the therapeutic management of patients with MB. The present review, systematically addressing the current shreds of evidence in the field, set the stage for considering MB as a novel and interesting therapeutic target, aiming to launch in the near future reliable and compelling efficacy endpoints to be utilized in proof-of-concept clinical trials.
Author contributions
RL and GC had a leading role in writing the manuscript. DD’A had a leading role in manuscript revision. FCr, PZ, AR, GR, MD, MG, AZ, FCa, RV, GP, AL, FB, CT, and VP had a supporting role in manuscript revision. All authors have read and agreed to the content of the manuscript.
Conflict of interest
Author MG declares that he has received consulting fees or honoraria from Terumo, outside the present work.
The remaining authors declare that the research was conducted in the absence of any commercial or financial relationships that could be construed as a potential conflict of interest.
Publisher’s note
All claims expressed in this article are solely those of the authors and do not necessarily represent those of their affiliated organizations, or those of the publisher, the editors and the reviewers. Any product that may be evaluated in this article, or claim that may be made by its manufacturer, is not guaranteed or endorsed by the publisher.
References
1. Alegria JR, Herrmann J, Holmes DR Jr, Lerman A, Rihal CS. Myocardial bridging. Eur Heart J. (2005) 26:1159–68. doi: 10.1093/eurheartj/ehi203
2. Möhlenkamp S, Hort W, Ge J, Erbel R. Update on myocardial bridging. Circulation. (2002) 106:2616–22. doi: 10.1161/01.CIR.0000038420.14867.7A
3. Sharzehee M, Chang Y, Song JP, Han HC. Hemodynamic effects of myocardial bridging in patients with hypertrophic cardiomyopathy. Am J Physiol Heart Circ Physiol. (2019) 317:H1282–91. doi: 10.1152/ajpheart.00466.2019
4. Aleksandric SB, Djordjevic-Dikic AD, Dobric MR, Giga VL, Soldatovic IA, Vukcevic V, et al. Functional assessment of myocardial bridging with conventional and diastolic fractional flow reserve: vasodilator versus inotropic provocation. J Am Heart Assoc. (2021) 10:e020597. doi: 10.1161/JAHA.120.020597
5. Hostiuc S, Negoi I, Rusu MC, Hostiuc M. Myocardial bridging: a meta-analysis of prevalence. J Forensic Sci. (2018) 63:1176–85. doi: 10.1111/1556-4029.13665
6. Hayashi T, Ishikawa K. Myocardial bridge: harmless or harmful. Intern Med. (2004) 43:1097–8. doi: 10.2169/internalmedicine.43.1097
7. Tarantini G, Migliore F, Cademartiri F, Fraccaro C, Iliceto S. Left anterior descending artery myocardial bridging: a clinical approach. J Am Coll Cardiol. (2016) 68:2887–99. doi: 10.1016/j.jacc.2016.09.973
8. Corban MT, Hung OY, Eshtehardi P, Rasoul-Arzrumly E, McDaniel M, Mekonnen G, et al. Myocardial bridging: contemporary understanding of pathophysiology with implications for diagnostic and therapeutic strategies. J Am Coll Cardiol. (2014) 63:2346–55. doi: 10.1016/j.jacc.2014.01.049
9. Cappannoli L, Ciliberti G, Restivo A, Palumbo P, D’Alò F, Sanna T, et al. ‘Here comes the story of the Hurricane’: a case report of AL cardiac amyloidosis and myocardial bridging. Eur Heart J Case Rep. (2022) 6:ytac225. doi: 10.1093/ehjcr/ytac225
10. Teragawa H, Oshita C, Ueda T. The myocardial bridge: potential influences on the coronary artery vasculature. Clin Med Insights Cardiol. (2019) 1:1179546819846493. doi: 10.1177/1179546819846493
11. Kunadian V, Chieffo A, Camici PG, Berry C, Escaned J, Maas AHEM, et al. An EAPCI expert consensus document on ischaemia with non-obstructive coronary arteries in collaboration with european society of cardiology working group on coronary pathophysiology & microcirculation endorsed by coronary vasomotor disorders international study group. Eur Heart J. (2020) 41:3504–20. doi: 10.1093/eurheartj/ehaa503
12. Yuan SM. Myocardial bridging. Braz J Cardiovasc Surg. (2016) 31:60–2. doi: 10.5935/1678-9741.20150082
13. Sternheim D, Power DA, Samtani R, Kini A, Fuster V, Sharma S, et al. Myocardial bridging: diagnosis, functional assessment, and management: JACC state-of-the-art review. J Am Coll Cardiol. (2021) 78:2196–212. doi: 10.1016/j.jacc.2021.09.859
14. Gould KL, Johnson NP. Myocardial bridges: lessons in clinical coronary pathophysiology. JACC Cardiovasc Imaging. (2015) 8:705–9. doi: 10.1016/j.jcmg.2015.02.013
15. Ge J, Erbel R, Rupprecht HJ, Koch L, Kearney P, Görge G, et al. Comparison of intravascular ultrasound and angiography in the assessment of myocardial bridging. Circulation. (1994) 89:1725–32. doi: 10.1161/01.CIR.89.4.1725
16. Schwarz ER, Klues HG, vom Dahl J, Klein I, Krebs W, Hanrath P. Functional characteristics of myocardial bridging. a combined angiographic and intracoronary Doppler flow study. Eur Heart J. (1997) 18:434–42. doi: 10.1093/oxfordjournals.eurheartj.a015263
17. Ge J, Jeremias A, Rupp A, Abels M, Baumgart D, Liu F, et al. New signs characteristic of myocardial bridging demonstrated by intracoronary ultrasound and Doppler. Eur Heart J. (1999) 20:1707–16. doi: 10.1053/euhj.1999.1661
18. Aleksandric S, Djordjevic-Dikic A, Beleslin B, Parapid B, Teofilovski-Parapid G, Stepanovic J, et al. Noninvasive assessment of myocardial bridging by coronary flow velocity reserve with transthoracic Doppler echocardiography: vasodilator vs. inotropic stimulation. Int J Cardiol. (2016) 225:37–45. doi: 10.1016/j.ijcard.2016.09.101
19. Erbel R, Rupprecht HJ, Ge J, Gerber T, Gunter G, Meyer J. Coronary artery shape and flow changes induced by myocardial bridging. Echocardiography. (1993) 10:71–7. doi: 10.1111/j.1540-8175.1993.tb00013.x
20. Schwarz ER, Gupta R, Haager PK, vom Dahl J, Klues HG, Minartz J, et al. Myocardial bridging in absence of coronary artery disease: proposal of a new classification based on clinical-angiographic data and long-term follow-up. Cardiology. (2009) 112:13–21. doi: 10.1159/000137693
21. Corban MT, Hung OY, Timmins LH, Rasoul-Arzrumly E, McDaniel M, Mekonnen G, et al. Myocardial bridging. J Am Coll Cardiol. (2014) 64:2179–81.
22. Malek AM, Alper SL, Izumo S. Hemodynamic shear stress and its role in atherosclerosis. JAMA. (1999) 282:2035–42. doi: 10.1001/jama.282.21.2035
23. Nikoliæ D, Radoviæ M, Aleksandriæ S, Tomaševiæ M, Filipoviæ N. Prediction of coronary plaque location on arteries having myocardial bridge, using finite element models. Comput Methods Programs Biomed. (2014) 117:137–44. doi: 10.1016/j.cmpb.2014.07.012
24. Chatzizisis YS, Giannoglou GD. Myocardial bridges spared from atherosclerosis: overview of the underlying mechanisms. Can J Cardiol. (2009) 25:219–22. doi: 10.1016/S0828-282X(09)70065-0
25. Verhagen SN, Rutten A, Meijs MF, Isgum I, Cramer MJ, van der Graaf Y, et al. Relationship between myocardial bridges and reduced coronary atherosclerosis in patients with angina pectoris. Int J Cardiol. (2013) 167:883–8. doi: 10.1016/j.ijcard.2012.01.091
26. Ishii T, Asuwa N, Masuda S. The effects of a myocardial bridge on coronary atherosclerosis and ischaemia. J Pathol. (1998) 185:4–9. doi: 10.1002/(SICI)1096-9896(199805)185:1<4::AID-PATH50>3.0.CO;2-3
27. Ishikawa Y, Ishii T, Asuwa N. Absence of atherosclerosis evolution in the coronary arterial segment covered by myocardial tissue in cholesterol-fed rabbits. Virchows Arch. (1997) 430:163–71. doi: 10.1007/BF01008038
28. Ishikawa Y, Akasaka Y, Suzuki K, Fujiwara M, Ogawa T, Yamazaki K, et al. Anatomic properties of myocardial bridge predisposing to myocardial infarction. Circulation. (2009) 120:376–83. doi: 10.1161/CIRCULATIONAHA.108.820720
29. Ishikawa Y, Akasaka Y, Akishima-Fukasawa Y, Iuchi A, Suzuki K, Uno M, et al. Histopathologic profiles of coronary atherosclerosis by myocardial bridge underlying myocardial infarction. Atherosclerosis. (2013) 226:118–23. doi: 10.1016/j.atherosclerosis.2012.10.037
30. Wu S, Liu W, Zhou Y. Spontaneous coronary artery dissection in the presence of myocardial bridge causing myocardial infarction: an insight into mechanism. Int J Cardiol. (2016) 206:77–8. doi: 10.1016/j.ijcard.2016.01.085
31. Fujibayashi D, Morino Y, Ikari Y. A case of acute myocardial infarction due to coronary spasm in the myocardial bridge. J Invasive Cardiol. (2008) 20:E217–9.
32. Nam P, Choi BG, Choi SY, Byun JK, Mashaly A, Park Y, et al. The impact of myocardial bridge on coronary artery spasm and long-term clinical outcomes in patients without significant atherosclerotic stenosis. Atherosclerosis. (2018) 270:8–12. doi: 10.1016/j.atherosclerosis.2018.01.026
33. Kim JW, Park CG, Suh SY, Choi CU, Kim EJ, Rha SW, et al. Comparison of frequency of coronary spasm in Korean patients with versus without myocardial bridging. Am J Cardiol. (2007) 100:1083–6. doi: 10.1016/j.amjcard.2007.05.030
34. Saito Y, Kitahara H, Shoji T, Tokimasa S, Nakayama T, Sugimoto K, et al. Relation between severity of myocardial bridge and vasospasm. Int J Cardiol. (2017) 248:34–8. doi: 10.1016/j.ijcard.2017.07.002
35. Teragawa H, Fukuda Y, Matsuda K, Hirao H, Higashi Y, Yamagata T, et al. Myocardial bridging increases the risk of coronary spasm. Clin Cardiol. (2003) 26:377–83. doi: 10.1002/clc.4950260806
36. Masuda T, Ishikawa Y, Akasaka Y, Itoh K, Kiguchi H, Ishii T, et al. The effect of myocardial bridging of the coronary artery on vasoactive agents and atherosclerosis localization. J Pathol. (2001) 193:408–14. doi: 10.1002/1096-9896(2000)9999:9999<::AID-PATH792>3.0.CO;2-R
37. Lanza GA, Careri G, Crea F. Mechanisms of coronary artery spasm. Circulation. (2011) 124:1774–82. doi: 10.1161/CIRCULATIONAHA.111.037283
38. Thygesen K, Alpert JS, Jaffe AS, Chaitman BR, Bax JJ, Morrow DA, et al. Executive Group on behalf of the Joint European Society of Cardiology (ESC)/American College of Cardiology (ACC)/American Heart Association (AHA)/World Heart Federation (WHF) Task Force for the Universal Definition of Myocardial Infarction. Fourth Universal Definition of Myocardial Infarction (2018). J Am Coll Cardiol. (2018) 72:2231–64. doi: 10.1161/CIR.0000000000000617
39. Hong L, Liu J, Luo S, Li J. Relation of myocardial bridge to myocardial infarction: a meta-analysis. Chin Med J. (2014) 127:945–50.
40. Sara JDS, Corban MT, Prasad M, Prasad A, Gulati R, Lerman LO, et al. Prevalence of myocardial bridging associated with coronary endothelial dysfunction in patients with chest pain and non-obstructive coronary artery disease. EuroIntervention. (2020) 15:1262–8. doi: 10.4244/EIJ-D-18-00920
41. D’Amario D, Borovac JA, Crea F. Coronary microvascular dysfunction in heart failure with preserved ejection fraction: not the end but the end of the beginning. Eur J Heart Fail. (2021) 23:773–5. doi: 10.1002/ejhf.2069
42. D’Amario D, Migliaro S, Borovac JA, Restivo A, Vergallo R, Galli M, et al. Microvascular dysfunction in heart failure with preserved ejection fraction. Front Physiol. (2019) 10:1347. doi: 10.3389/fphys.2019.01347
43. Pargaonkar VS, Kimura T, Kameda R, Tanaka S, Yamada R, Schwartz JG, et al. Invasive assessment of myocardial bridging in patients with angina and no obstructive coronary artery disease. EuroIntervention. (2021) 16:1070–8. doi: 10.4244/EIJ-D-20-00779
44. Lin S, Tremmel JA, Yamada R, Rogers IS, Yong CM, Turcott R, et al. A novel stress echocardiography pattern for myocardial bridge with invasive structural and hemodynamic correlation. J Am Heart Assoc. (2013) 2:e000097. doi: 10.1161/JAHA.113.000097
45. Gould KL, Kirkeeide R, Johnson NP. Coronary branch steal: experimental validation and clinical implications of interacting stenosis in branching coronary arteries. Circ Cardiovasc Imaging. (2010) 3:701–9. doi: 10.1161/CIRCIMAGING.110.937656
46. Tavakol M, Ashraf S, Brener SJ. Risks and complications of coronary angiography: a comprehensive review. Glob J Health Sci. (2012) 4:65–93. doi: 10.5539/gjhs.v4n1p65
47. Podolec J, Wiewiórka Ł, Siudak Z, Malinowski K, Dudek D, Gackowski A. Prevalence and clinical presentation of myocardial bridge on the basis of the National Polish Percutaneous Interventions Registry and the Classification of Rare Cardiovascular Diseases. Kardiol Pol. (2018) 77:465–70. doi: 10.5603/KP.a2019.0041
48. Kim PJ, Hur G, Kim SY, Namgung J, Hong SW, Kim YH, et al. Frequency of myocardial bridges and dynamic compression of epicardial coronary arteries: a comparison between computed tomography and invasive coronary angiography. Circulation. (2009) 119:1408–16. doi: 10.1161/CIRCULATIONAHA.108.788901
49. Konen E, Goitein O, Sternik L, Eshet Y, Shemesh J, Di Segni E. The prevalence and anatomical patterns of intramuscular coronary arteries: a coronary computed tomography angiographic study. J Am Coll Cardiol. (2007) 49:587–93. doi: 10.1016/j.jacc.2006.09.039
50. Forsdahl SH, Rogers IS, Schnittger I, Tanaka S, Kimura T, Pargaonkar VS, et al. Myocardial bridges on coronary computed tomography angiography – correlation with intravascular ultrasound and fractional flow reserve. Circ J. (2017) 81:1894–900. doi: 10.1253/circj.CJ-17-0284
51. Zhang JZ, Zhu GY, Zhang Y, Bai LJ, Wang Z. Myocardial bridge bypass graft: a novel surgical procedure for extensive myocardial bridges. Ann Thorac Surg. (2021) 112:e115–7. doi: 10.1016/j.athoracsur.2020.11.055
52. Pargaonkar VS, Rogers IS, Su J, Forsdahl SH, Kameda R, Schreiber D, et al. Accuracy of a novel stress echocardiography pattern for myocardial bridging in patients with angina and no obstructive coronary artery disease - A retrospective and prospective cohort study. Int J Cardiol. (2020) 311:107–13. doi: 10.1016/j.ijcard.2020.02.006
53. Aleksandric SB, Djordjevic-Dikic AD, Giga VL, Tesic MB, Soldatovic IA, Banovic MD, et al. Coronary flow velocity reserve using dobutamine test for noninvasive functional assessment of myocardial bridging. J Clin Med. (2021) 11:204. doi: 10.3390/jcm11010204
54. Daimon M, Watanabe H, Yamagishi H, Muro T, Akioka K, Hirata K, et al. Physiologic assessment of coronary artery stenosis by coronary flow reserve measurements with transthoracic Doppler echocardiography: comparison with exercise thallium-201 single piston emission computed tomography. J Am Coll Cardiol. (2001) 37:1310–5. doi: 10.1016/S0735-1097(01)01167-6
55. Takeuchi M, Miyazaki C, Yoshitani H, Otani S, Sakamoto K, Yoshikawa J. Assessment of coronary flow velocity with transthoracic Doppler echocardiography during dobutamine stress echocardiography. J Am Coll Cardiol. (2001) 38:117–23. doi: 10.1016/S0735-1097(01)01322-5
56. Abadie B, Jaber W. Dobutamine stress PET/CT for assessment of hemodynamic significance of coronary myocardial bridges. J Nucl Cardiol. (2021) doi: 10.1007/s12350-021-02759-8 [Epub ahead of print].
57. Hongo Y, Tada H, Ito K, Yasumura Y, Miyatake K, Yamagishi M. Augmentation of vessel squeezing at coronary-myocardial bridge by nitroglycerin: study by quantitative coronary angiography and intravascular ultrasound. Am Heart J. (1999) 138:345–50. doi: 10.1016/S0002-8703(99)70123-7
58. Bangalore S, Bhatt DL. Coronary intravascular ultrasound. Circulation. (2013) 127:e868–74. doi: 10.1161/CIRCULATIONAHA.113.003534
59. Ge J, Jeremias A, Rupp A, Abels M, Abels M, Baumgart D, et al. New signs characteristic of myocardial bridging demonstrated by intracoronary ultrasound and Doppler. Eur Heart J. (1999) 20:1707–16. doi: 10.1053/euhj.1999.1661
60. Yamada R, Tremmel JA, Tanaka S, Lin S, Kobayashi Y, Hollak MB, et al. Functional versus anatomic assessment of myocardial bridging by intravascular ultrasound: impact of arterial compression on proximal atherosclerotic plaque. J Am Heart Assoc. (2016) 5:e001735. doi: 10.1161/JAHA.114.001735
61. Shimamura K, Kubo T, Akasaka T. Evaluation of coronary plaques and atherosclerosis using optical coherence tomography. Expert Rev Cardiovasc Ther. (2021) 19:379–86. doi: 10.1080/14779072.2021.1914588
62. Triantafyllis AS, de Ridder S, Teeuwen K, Otterspoor LC. Myocardial bridging, a trigger for Takotsubo syndrome. Neth Heart J. (2018) 26:573–4. doi: 10.1007/s12471-018-1142-0
63. Xu T, You W, Wu Z, Meng P, Ye F, Wu X, et al. Retrospective analysis of OCT on MB characteristics and 1-year follow-up of the ISR incidence after the DES implantation in patients with MB. Sci Rep. (2022) 12:534. doi: 10.1038/s41598-021-04579-9
64. Cao HM, Jiang JF, Deng B, Xu JH, Xu WJ. Evaluation of myocardial bridges with optical coherence tomography. J Int Med Res. (2010) 38:681–5. doi: 10.1177/147323001003800232
65. Ye Z, Lai Y, Mintz GS, Yao Y, Tang J, Luo Y, et al. Fusiform appearance of myocardial bridging detected by OCT. JACC Cardiovasc Imaging. (2016) 9:892–4. doi: 10.1016/j.jcmg.2015.05.006
66. Okamura A, Okura H, Iwai S, Kyodo A, Kamon D, Hashimoto Y, et al. Detection of myocardial bridge by optical coherence tomography. Int J Cardiovasc Imaging. (2022) doi: 10.1007/s10554-021-02497-5 [Epub ahead of print].
67. Ye Z, Lai Y, Yao Y, Mintz GS, Liu X. Optical coherence tomography and intravascular ultrasound assessment of the anatomic size and wall thickness of a muscle bridge segment. Catheter Cardiovasc Interv. (2019) 93:772–8. doi: 10.1002/ccd.28094
68. Elgendy IY, Conti CR, Bavry AA. Fractional flow reserve: an updated review. Clin Cardiol. (2014) 37:371–80. doi: 10.1002/clc.22273
69. Neumann FJ, Sousa-Uva M, Ahlsson A, Alfonso F, Banning AP, Benedetto U, et al. ESC Scientific Document Group. 2018 ESC/EACTS Guidelines on myocardial revascularization. Eur Heart J. (2019) 40:87–165. doi: 10.1093/eurheartj/ehy855
70. Escaned J, Cortés J, Flores A, Goicolea J, Alfonso F, Hernández R, et al. Importance of diastolic fractional flow reserve and dobutamine challenge in physiologic assessment of myocardial bridging. J Am Coll Cardiol. (2003) 42:226–33. doi: 10.1016/S0735-1097(03)00588-6
71. Abe M, Tomiyama H, Yoshida H, Doba N. Diastolic fractional flow reserve to assess the functional severity of moderate coronary artery stenoses: comparison with fractional flow reserve and coronary flow velocity reserve. Circulation. (2000) 102:2365–70. doi: 10.1161/01.CIR.102.19.2365
72. Davies JE, Sen S, Dehbi HM, Al-Lamee R, Petraco R, Nijjer SS, et al. Use of the instantaneous wave-free ratio or fractional flow reserve in PCI. N Engl J Med. (2017) 376:1824–34.
73. Tarantini G, Barioli A, Nai Fovino L, Fraccaro C, Masiero G, Iliceto S, et al. Unmasking myocardial bridge-related ischemia by intracoronary functional evaluation. Circ Cardiovasc Interv. (2018) 11:e006247. doi: 10.1161/CIRCINTERVENTIONS.117.006247
74. Kumar G, Desai R, Gore A, Rahim H, Maehara A, Matsumura M, et al. Real world validation of the nonhyperemic index of coronary artery stenosis severity-Resting full-cycle ratio-RE-VALIDATE. Catheter Cardiovasc Interv. (2020) 96:E53–8. doi: 10.1002/ccd.28523
75. Kern MJ, Seto AH. dPR, Another diastolic resting pressure ratio. Circ Cardiovasc Interv. (2018) 11:e007540. doi: 10.1161/CIRCINTERVENTIONS.118.007540
76. Svanerud J, Ahn JM, Jeremias A, van ’t Veer M, Gore A, Maehara A, et al. Validation of a novel non-hyperaemic index of coronary artery stenosis severity: the Resting Full-cycle Ratio (VALIDATE RFR) study. EuroIntervention. (2018) 14:806–14. doi: 10.4244/EIJ-D-18-00342
77. Previtali M, Ardissino D, Barberis P, Panciroli C, Chimienti M, Salerno JA, et al. Hyperventilation and ergonovine tests in Prinzmetal’s variant angina pectoris in men. Am J Cardiol. (1989) 63:17–20. doi: 10.1016/0002-9149(89)91068-0
78. Ong P, Athanasiadis A, Borgulya G, Vokshi I, Bastiaenen R, Kubik S, et al. Clinical usefulness, angiographic characteristics, and safety evaluation of intracoronary acetylcholine provocation testing among 921 consecutive white patients with unobstructed coronary arteries. Circulation. (2014) 129:1723–30. doi: 10.1161/CIRCULATIONAHA.113.004096
79. Buxton A, Goldberg S, Hirshfeld JW, Wilson J, Mann T, Williams DO, et al. Refractory ergonovine-induced coronary vasospasm: importance of intracoronary nitroglycerin. Am J Cardiol. (1980) 46:329–34. doi: 10.1016/0002-9149(80)90080-6
80. Knuuti J, Wijns W, Saraste A, Capodanno D, Barbato E, Funck-Brentano C, et al. 2019 ESC Guidelines for the diagnosis and management of chronic coronary syndromes. Eur Heart J. (2020) 41:407–77.
81. Beltrame JF, Crea F, Kaski JC, Ogawa H, Ong P, Sechtem U, et al. International standardization of diagnostic criteria for vasospastic angina. Eur Heart J. (2017) 38:2565–8.
82. Ong P, Camici PG, Beltrame JF, Crea F, Shimokawa H, Sechtem U, et al. International standardization of diagnostic criteria for microvascular angina. Int J Cardiol. (2018) 250:16–20. doi: 10.1016/j.ijcard.2017.08.068
83. Shiode N, Kato M, Teragawa H, Yamada T, Hirao H, Nomura K, et al. Vasomotility and nitric oxide bioactivity of the bridging segments of the left anterior descending coronary artery. Am J Cardiol. (1998) 81:341–3. doi: 10.1016/S0002-9149(97)00912-0
84. Herrmann J, Higano ST, Lenon RJ, Rihal CS, Lerman A. Myocardial bridging is associated with alteration in coronary vasoreactivity. Eur Heart J. (2004) 25:2134–42. doi: 10.1016/j.ehj.2004.08.015
85. Montone RA, Gurgoglione FL, Del Buono MG, Rinaldi R, Meucci MC, Iannaccone G, et al. Interplay between myocardial bridging and coronary spasm in patients with myocardial ischemia and non-obstructive coronary arteries: pathogenic and prognostic implications. J Am Heart Assoc. (2021) 10:e020535. doi: 10.1093/ehjacc/zuab020.139
86. Kim JW, Seo HS, Na JO, Na JO, Suh SY, Choi CU, et al. Myocardial bridging is related to endothelial dysfunction but not to plaque as assessed by intracoronary ultrasound. Heart. (2008) 94:765–9. doi: 10.1136/hrt.2007.115980
87. Arai R, Kano H, Suzuki S, Semba H, Arita T, Yagi N, et al. Myocardial bridging is an independent predictor of positive spasm provocation testing by intracoronary ergonovine injections: a retrospective observational study. Heart Vessels. (2020) 35:474–86. doi: 10.1007/s00380-019-01518-7
88. Im SI, Rha SW, Choi BG, Choi SY, Kim SW, Na JO, et al. Angiographic and clinical characteristics according to intracoronary acetylcholine dose in patients with myocardial bridge. Cardiology. (2013) 125:250–7. doi: 10.1159/000351181
89. Lee MS, Chen CH. Myocardial bridging: an up-to-date review. J Invasive Cardiol. (2015) 27:521–8.
90. Kern MJ, Ganz P, Horowitz JD, Gaspar J, Barry WH, Lorell BH, et al. Potentiation of coronary vasoconstriction by beta-adrenergic blockade in patients with coronary artery disease. Circulation. (1983) 67:1178–85. doi: 10.1161/01.CIR.67.6.1178
91. Beltrame JF, Crea F, Kaski JC, Ogawa H, Ong P, Sechtem U, et al. The Who, What, Why, When, How and Where of Vasospastic Angina. Circ J. (2016) 80:289–98. doi: 10.1253/circj.CJ-15-1202
92. Klues HG, Schwarz ER, vom Dahl J, Reffelmann T, Reul H, Potthast K, et al. Disturbed intracoronary hemodynamics in myocardial bridging: early normalization by intracoronary stent placement. Circulation. (1997) 96:2905. doi: 10.1161/01.CIR.96.9.2905
93. Pourhoseini S, Bakhtiari M, Babaee A, Ostovan MA, Eftekhar-Vaghefi SH, Ostovan N, et al. Increased risk of coronary perforation durings percutaneous intervention of myocardial bridge: What histopathology says. J Cardiovasc Thorac Res. (2017) 9:108–12. doi: 10.15171/jcvtr.2017.18
94. Li W, Li Y, Sheng L, Gong Y. Myocardial bridge: is the risk of perforation increased? Can J Cardiol. (2008) 24:e80–1. doi: 10.1016/S0828-282X(08)70198-3
95. Borovac JA, D’Amario D, Vergallo R, Porto I, Bisignani A, Galli M, et al. Neoatherosclerosis after drug-eluting stent implantation: a novel clinical and therapeutic challenge. Eur Heart J Cardiovasc Pharmacother. (2019) 5:105–16. doi: 10.1093/ehjcvp/pvy036
96. Jiang Q, Liang C, Wu Z. Myocardial bridging is a potential risk factor of very late stent thrombosis of drug eluting stent. Med Sci Monit. (2012) 18:HY9–12. doi: 10.12659/MSM.882717
Keywords: myocardial bridging, myocardial ischemia, invasive intracoronary assessment, intracoronary physiology, intracoronary imaging, non-invasive tests, tailored therapy
Citation: Ciliberti G, Laborante R, Di Francesco M, Restivo A, Rizzo G, Galli M, Canonico F, Zito A, Princi G, Vergallo R, Leone AM, Burzotta F, Trani C, Palmieri V, Zeppilli P, Crea F and D’Amario D (2022) Comprehensive functional and anatomic assessment of myocardial bridging: Unlocking the Gordian Knot. Front. Cardiovasc. Med. 9:970422. doi: 10.3389/fcvm.2022.970422
Received: 15 June 2022; Accepted: 21 October 2022;
Published: 08 November 2022.
Edited by:
Srdjan Aleksandric, Clinical Center of Serbia, University of Belgrade, SerbiaReviewed by:
Giulio Russo, Policlinico Tor Vergata, ItalyEugenio Picano, National Research Council (CNR), Italy
Copyright © 2022 Ciliberti, Laborante, Di Francesco, Restivo, Rizzo, Galli, Canonico, Zito, Princi, Vergallo, Leone, Burzotta, Trani, Palmieri, Zeppilli, Crea and D’Amario. This is an open-access article distributed under the terms of the Creative Commons Attribution License (CC BY). The use, distribution or reproduction in other forums is permitted, provided the original author(s) and the copyright owner(s) are credited and that the original publication in this journal is cited, in accordance with accepted academic practice. No use, distribution or reproduction is permitted which does not comply with these terms.
*Correspondence: Domenico D’Amario, ZG9tZW5pY28uZGFtYXJpb0BnbWFpbC5jb20=
†These authors have contributed equally to this work and share first authorship