- 1Department of Cardiology, Life Science Center, The First Affiliated Hospital of Xinxiang Medical University, Weihui, China
- 2Key Laboratory of Cardiovascular Injury and Repair Medicine of Henan, Weihui, China
- 3Henan Normal University, Xinxiang, China
Long non-coding RNA (lncRNAs) are longer than 200 nucleotides and cannot encode proteins but can regulate the expression of genes through epigenetic, transcriptional, and post-transcriptional modifications. The pathophysiology of smooth muscle cells can lead to many vascular diseases, and studies have shown that lncRNAs can regulate the phenotypic conversion of smooth muscle cells so that smooth muscle cells proliferate, migrate, and undergo apoptosis, thereby affecting the development and prognosis of vascular diseases. This review discusses the molecular mechanisms of lncRNA as a signal, bait, stent, guide, and other functions to regulate the phenotypic conversion of vascular smooth muscle cells, and summarizes the role of lncRNAs in regulating vascular smooth muscle cells in atherosclerosis, hypertension, aortic dissection, vascular restenosis, and aneurysms, providing new ideas for the diagnosis and treatment of vascular diseases.
Introduction
Phenotype transformation of vascular smooth muscle cells (VSMCs) is an important cause of vascular dysfunction, capable of inducing vascular diseases, such as atherosclerosis (AS), hypertension, vascular stenosis, and diabetic vascular complications (1–6), and mature smooth muscle cells are widely distributed in the walls of blood vessels and internal organs, and normal VSMCs have no significant function in proliferating, migrating, and secreting the extracellular matrix, called constrictive VSMCs, which maintain vascular elasticity and ensure vasoconstriction (7). VSMCs exhibit significant proliferation and migration under immature or pathological conditions, such as inflammation, hypertension, and diabetes, and they synthesize large amounts of extracellular matrix, which are called synthetic VSMCs (8). After the phenotypic transformation occurs in smooth muscle cells, they change from “contractile type” to “synthetic type,” causing changes in vascular function and playing an important role in the development of vascular remodeling, and increasing evidence is emerging that the phenotype of VSMCs can develop fibroblastic, osteoblastic, and even macrophage-like cell characteristics (9, 10). Thus, understanding the pathophysiological changes in muscle cells is essential for diagnosing and treating vascular diseases. For a better understanding, we have made a graph, as shown in Figure 1.
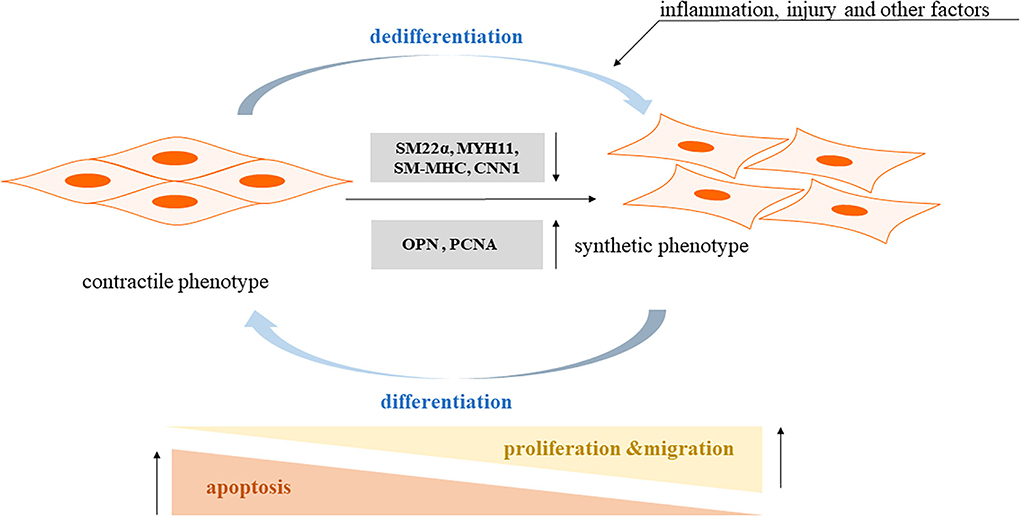
Figure 1. The pathophysiological mechanism of smooth muscle cells phenotypic transformation. Contractile phenotype markers: SM22a: smooth muscle 22a; SM-MHC: smooth muscle myosin heavy chain; MYH11: myosin heavy chain 11; CNN1: calponin 1. Synthetic phenotype markers: OPN: osteopontin; PCNA: proliferating cell nuclear antigen.
Long non-coding RNAs (lncRNAs) are longer than 200 nucleotides and cannot code for proteins (11); according to the genome and the location relationship between adjacent genes, lncRNAs can be divided into sense, antisense, bidirectional, intronic, and intergenic lncRNA (12), and lncRNA expression has the spatial specificity of tissue expression. They play important roles in disease development, such as regulating transcription, epigenetic modifications, protein, and RNA stability, and translation and post-translational modifications, by interacting with DNA, RNA, and proteins, which are closely related to their intracellular localization, lncRNAs localized in the nucleus play several roles: (1) regulate chromatin remodeling, induce histone modifications to regulate downstream gene expression; (2) act as enhancer RNAs to regulate transcription; and (3) interfere with pre-mRNA processing to regulate mRNA splicing. LncRNAs localized in the cytoplasm can play several roles: (1) act as decoys that can regulate specific transcription factors and inhibit their function (13, 14); (2) act as sponges to adsorb miRNAs, regulate their stability and reduce their bioavailability (15); (3) act as molecular scaffolds that can bring two or more proteins into complex transcriptional or post-transcriptional complexes regulating gene expression; and (4) binding to specific proteins to affect protein translation and post-translational modifications, or as a precursor molecule for small molecule RNAs (16). The specific functions are shown in Figure 2 (17).
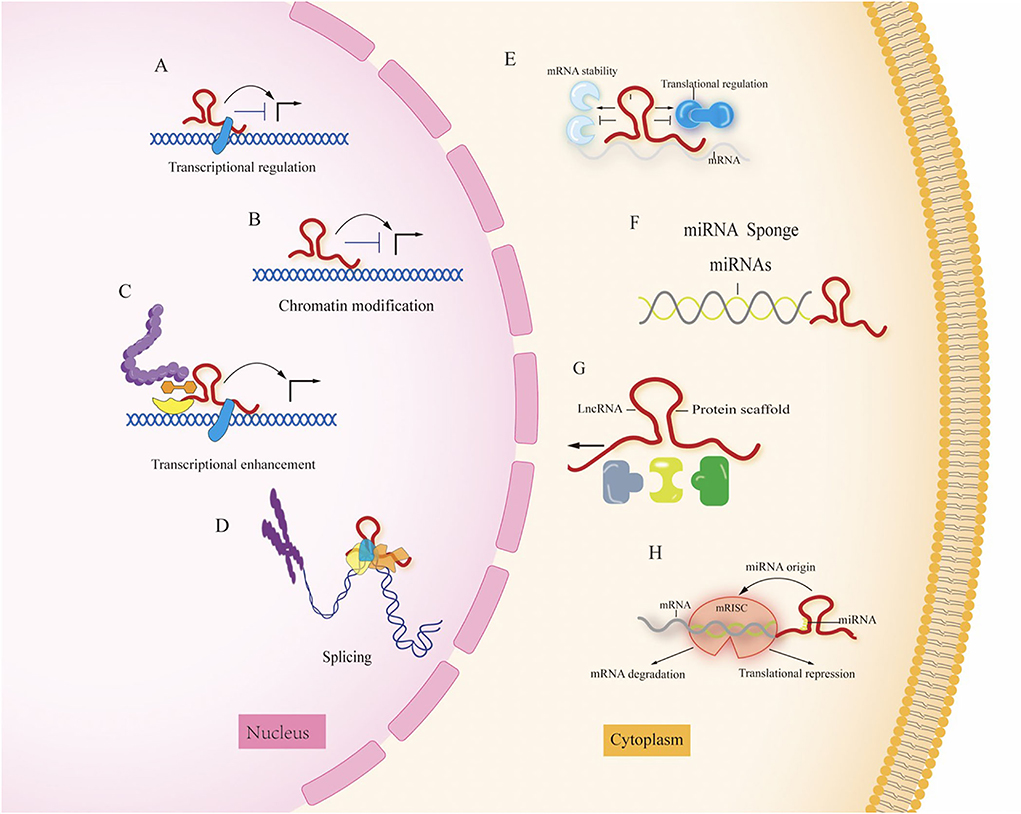
Figure 2. The different roles of LncRNA in the nucleus and cytoplasm. (A) Transcriptional regulation; (B) chromation modification; (C) transcriptional enhancement; (D) splicing; (E) regulation of mRNA stability; (F) miRNA sponge; (G) protein scaffold; (H) LncRNAs can function as miRNA host transcripts (miRNA origin). Mature single-stranded miRNA is derived from lncRNA transcripts and loaded into RNA induced silencing complex (RISC) by repressing translation or inducing mRNA degradation.
LncRNAs regulate smooth muscle cell phenotypic transition (18) and play a key role in related diseases, but the molecular mechanisms are not fully understood. In this review, we briefly outline the effect of the regulation of lncRNAs on differentiation and phenotypic transition in VSMCs during pathological remodeling. We also focus on how lncRNAs play a regulatory role in various conditions and their contribution to vascular diseases.
Role of LncRNA in smooth muscle cell phenotypic transformation
This review focuses on the mechanisms by which LncRNAs are known to play a regulatory role in various conditions and their contribution to vascular diseases. Table 1 shows the lncRNAs implicated in the regulation of VSMC phenotype and their validated targets.
LncRNA and atherosclerosis
Dysfunction of smooth muscle cells can trigger plaque formation, which is an important link in the pathogenesis of atherosclerosis, namely, the phenotypic transformation of proliferation, migration, and apoptosis. Platelet-derived growth factor-BB (PDGF-BB), oxidized low-density lipoprotein (ox-LDL), interleukin-6 (IL-6), and tumor necrosis factor-α (TNF-α) can change the phenotypic transformation of VSMCs from a contractile to a synthetic phenotype, promote smooth muscle cell proliferation and migration, and inhibit apoptosis. Matrix metalloprotein 2 (MMP-2) and matrix metalloprotein 9 (MMP-9) are important VSMC migration regulators. B cell lymphoma-2 (Bcl-2) and BCL2-Associated X (Bax) are apoptosis-related proteins. LncRNAs play various roles in regulating smooth muscle cell phenotypic transformation, as well as cell proliferation and migration. Detection of smooth muscle cell phenotypic transformation-related markers, cell proliferation, apoptosis-related proteins, and other clear regulatory roles implies that the effective control of smooth muscle cell phenotypic transformation may be an important therapeutic measure to prevent and treat AS.
The lncRNA SMILR can act as an enhancer or molecular scaffold to promote the proliferation of VSMCs by interacting with the promoter region of hyaluronidase 2 (HAS2), an important component of the extracellular matrix deposited in AS lesions, which promotes vessel wall thickening and reflects the degree of AS disease progression (19). Animal studies show that VSMC-specific HAS2 overexpression in transgenic mice increases susceptibility to AS and promoted vessel wall thickening. The investigators found increased expression of lncRNA SMILR in unstable AS plaques, which was detectable in the plasma of patients. These results confirmed that lncRNA SMILR is a driver of VSMC proliferation (20). However, it has been shown that lincRNA-p21 can also act as an enhancer partially bound to mouse double minutes2 (MDM2), enhancing the transcriptional activity of p53 and enabling p53 to interact with protein 300 (p300) and bind to the promoter/enhancer of its target gene, thereby inhibiting cell proliferation and inducing apoptosis in VSMCs (21). Another study showed that under ox-LDL stimulation, lncRNA antisense non-coding RNA in the INK4 locus (ANRIL) can act as a molecular scaffold to promote the binding of WD-40 repeat-containing protein 5(WDR5) and histone deacetylase 3 (HDAC3), thus, forming a WDR5/HDAC3 complex that regulates the expression of the target gene NADPH oxidase 1 (NOX1) through histone modifications, upregulates reactive oxygen species (ROS) levels, promotes phenotypic transition in HASMCs, and is a potential scaffolding protein (23).
LncRNAs can exert a role by directly binding to proteins and participating in protein phosphorylation and the activation of signaling pathways. lncRNAs are required to localize specific protein complexes, which can interact with DNA or mRNA and inhibit their expression or translation through methylation. In AS plaques, lncRNA ZNF800 expression is upregulated by directly binding to phosphatase and tensin homolog deleted on chromosome 10 (PTEN), thereby blocking the AKT (also known as protein kinase B PKB)/mammalian target of rapamycin (mTOR) pathway to inhibit PDGF-BB-mediated proliferation and migration of VSMCs. MMP1 promotes cell migration by degrading ECM components, and vascular endothelial growth factor-α (VEGF-α) can also lead to cell proliferation and migration. LncRNA ZNF800 regulates the hypoxia-inducible factor-1α (HIF-1α)-mediated VEGF-α or MMP1 pathway through the PTEN-activated AKT/mTOR signaling pathway to inhibit VSMC proliferation and migration (26). Similarly, the inhibition of lncRNA myocardial infarction-associated transcript (MIAT) limits the phosphorylation of extracellular signal-regulated kinase (p-ERK), increases the phosphorylation of ETS transcription factor (p-ELK1) accumulation in the nucleus, and subsequently decreases early growth response 1 (EGR1) expression, thereby regulating the proliferation of smooth muscle cells (SMCs) through the EGR1-ELK1-ERK pathway. The lncRNA MIAT also binds to the promoter region of Krüppel-like factor 4 (KLF4) and enhances its transcription, participating in the phenotypic transformation of SMCs to pro-inflammatory macrophage-like cells. In vivo studies have shown that SMCs in mouse and minipig models of AS display changes similar to those of HASMCs, thus confirming that lncRNA MIAT plays a regulatory role in advanced AS lesion formation by inducing the differentiation and dedifferentiation of SMCs (27). Another study showed that lncRNA 430945 is highly expressed in human AS tissues, which in turn promotes the angiotensin II (AngII)-induced proliferation of VSMCs. The upregulation of lncRNA 430945 expression activates signaling pathways associated with receptor tyrosine kinase-like orphan receptor 2 (ROR2) and Ras homolog gene family member A (RhoA), promoting AngII-induced proliferation and migration of VSMCs (29). In contrast, metformin exerts an anti-AS effect by activating AMP-activated protein kinase (AMPK), increasing the expression of lncRNA ANRIL, enhancing the affinity of lncRNA ANRIL to the AMPKγ subunit, increasing the catalytic activity of AMPK, and increasing its phosphorylation level, thereby inhibiting the phenotypic transition of VSMCs (88). The downregulation of miR-34c expression may be owing to the demethylation associated with lncRNA BRAF-activated non-coding RNA (BANCR). High mobility group protein B1 (HMGB1) is a pro-inflammatory mediator that upregulates the expression of cytokines, chemokines, and adhesion molecules, thereby enhancing macrophage infiltration, leading to AS. miR-34c overexpression inhibits the expression of HMGB1, TNF-α, and Bcl-2. LncRNA BANCR overexpression induces HASMC proliferation by downregulating miR-34c methylation and reversing the effect of miR-34c on HMGB1, TNF-α, and Bcl-2 expression, thereby promoting HASMCs proliferation and inhibiting apoptosis (30). The expression of LncRNA RP11-531A24.3 is reduced in advanced AS lesions; in cells overexpressing it, lncRNA RP11-531A24.3 inhibits the migration and proliferation of HA-VSMCs by binding directly to the RNA-binding protein annexin 2 (ANXA2) in the cytoplasm to reduce its expression at the mRNA and protein levels (31).
The cardiac mesoderm enhancer-associated non-coding RNA (CARMN) regulates specific transcription factors, and serum response factor (SRF), a transcription factor that binds to CArG elements, plays an important role in regulating the VSMC phenotype by interacting with multiple cofactors (32). Myocardin (MYOCD) is a specific transcriptional co-activator involved in the differentiation of cardiomyocytes and VSMCs. MYOCD enhances the binding of SRF to the CArG box and transcriptionally activates a variety of downstream VSMC contractile genes, representing the contractile and differentiated VSMC phenotype (33). The lncRNA CARMN enhances trans-MYOCD function by directly binding to MYOCD to maintain the contractile phenotype of VSMCs in healthy arteries. In contrast, in diseased arteries, lncRNA CARMN expression is downregulated, thereby attenuating the trans-activating activity of the MYOCD/SRF complex on SMC-specific gene expression and triggering the dedifferentiation of VSMCs, leading to increased neointimal formation (34). Another transcriptional regulator, cyclin-dependent kinase 9 (CDK9), was shown to be a direct target of lncRNA PEBP1P2, and overexpression of lncRNA PEBP1P2 significantly inhibited proliferation, migration, and dedifferentiation during PDGF-BB-induced phenotypic transformation of VSMCs by directly binding to CDK9 to downregulate its expression. This idea was similarly validated in animal experiments, where lncRNA PEBP1P2 overexpression attenuated neointima formation and VSMC phenotypic transformation induced in a balloon-injured carotid artery model (35). LncRNA LIPCAR accelerates the cell cycle by inhibiting the expression of the anti-proliferative gene P21 and activating the transcriptional regulator CDK2, decreasing the expression of α-SMA, and increasing the expression of MMP-2 and MMP-9 to promote VSMC proliferation, migration, and ultimately endothelial hyperplasia and AS plaque formation (36, 37). In another study, lncRNA H19 was expressed in the neoplastic endothelium of a mouse balloon injury model as well as in the VSMCs of human plaques (38), and knockdown of lncRNA H19 enhanced the interaction between Bax and p53 proteins by increasing p53-regulated transcription, leading to the proliferation of VSMCs and a reduction in plaque size, and mediated VSMC apoptosis to delay the development of AS (39). In AS plaques, lncRNA AC105942.1 expression was downregulated and hnRNPA2/B1 expression was upregulated, whereas hnRNPA2/B1 functions in the cell cycle by regulating the transcriptional levels of cell cycle protein kinase (CDK4) and p27. When lncRNA AC105942.1 expression was upregulated, the proliferation of AngII-treated VSMCs was reduced, CDK4 expression was decreased, and p27 was upregulated, whereas heterogeneous nuclear ribonucleoprotein A2/B1 (hnRNPA2/B1) expression was reduced. hnRNPA2/B1 knockdown also significantly reduced CDK4 expression and upregulated p27 levels, and the results suggest that lncRNA AC105942.1 acts by downregulating hnRNPA2/B1 expression to regulate the transcriptional levels of CDK4 and p27, thereby inhibiting the proliferative effects of AngII on VSMCs (44).
However, in ox-LDL-induced HA-VSMCs, knockdown of lncRNA H19 also acts as a sponge to adsorb miR-599 to reduce pappalysin 1 (PAPPA) to inhibit the increase in cyclin D1 and N-cadherin in HA-VSMCs and decrease E-cadherin to promote proliferation, migration, and invasion of HA-VSMCs (40). H19 also acts as a competitive endogenous RNA (ceRNA) for miR-148b to enhance the expression of wnt family member 1 (WNT1). Moreover, miR-148 inhibitors exert their pro-proliferative and anti-apoptotic effects by activating ox-LDL-stimulated Wnt/β-catenin signaling in HA-VSMCs (41). LncRNA taurine upregulated gene 1 (TUG1) expression was also upregulated in VSMCs induced by hypoxia or TNF-α in patients with AS. In established injury models, lncRNA TUG1 promotes VSMC proliferation and AS by targeting miRNA-21 to downregulate PTEN expression, decrease PTEN activity, and increase cyclin D1 expression (45). Another lncRNA, ANRIL, also called cyclin-dependent kinase inhibitor 2B antisense RNA 1 (CDKN2B-AS1), acts as a ceRNA to competitively bind miR-126-5p to upregulate protein tyrosine phosphatase non-receptor type 7 (PTPN7) expression and inhibit the phosphatidylinositide 3-kinases (PI3K)-AKT pathway, thereby hindering ox-LDL-induced proliferation and accelerating apoptosis (24). LncRNA forkhead box protein C2-AS1 (FOXC2-AS1) expression was significantly upregulated in VSMCs induced by ox-LDL and IL-6. LncRNA FOXC2-AS1 binds to miR-1253 as a ceRNA, causing miR-1253 to target forkhead box protein F1 (FOXF1), increasing the levels of Bcl-2 and significantly decreasing Bax and caspase-3, thereby regulating cell proliferation and the development of AS (46). Similarly, overexpression of lncRNA HLA complex group 11 (HCG11) can act as a sponge to negatively regulate miR-144 while increasing FOXF1 expression, resulting in increased Bcl-2 and decreased Bax expression, thereby promoting proliferation and inhibiting apoptosis in VSMCs (47). Silencing the lncRNA MIAT acts as a sponge for miR-641, induces stromal interaction molecule 1 (STIM1), attenuates the protein expression of proliferating cell nuclear antigen (PCNA), and Ki-67, and thus inhibits ox-LDL-induced proliferation, migration, and invasion (28). Likewise, overexpression of lncRNA C-terminal binding protein 1-antisense RNA 2 (CTBP1-AS2) acts as a ceRNA for miR-195-5p to promote autophagy-related 14 (ATG14) expression and decrease PCNA and Ki-67 expression levels, thereby inhibiting HAVSMC proliferation (48). Downregulation of lncRNA X-inactive-specific transcript (XIST), as a competitive endogenous RNA for miR-539-5p to enhance the expression of secreted phosphoprotein 1, inhibited the upregulation of PCNA and Ki-67 expression, as well as the expression of MMP-2 and MMP-9, thereby suppressing the proliferation, migration, and invasion of VSMCs by ox-LDL stimulation (89). Similarly, overexpression of another lncRNA, cancer susceptibility candidate 2 (CASC2), can act as a sponge to negatively regulate the expression of miR-532-3p, upregulate the expression of non-canonical poly (A) polymerase 5 (PAPD5), and inhibit the expression of PCNA, α-SMA, MMP-2, and MMP-9. LncRNA CASC2 inhibits the proliferation of VSMCs and promotes apoptosis by regulating the miR-532-3p/PAPD5 axis (50). miR-361-5p targets the 3′-UTR of ATP-binding cassette transporter A1 (ABCA1) mRNA and downregulates lncRNA maternally expressed gene 3 (MEG3), possibly by binding miR-361-5p to act as an endogenous “sponge,” thereby abolishing the miRNA-mediated inhibitory activity on the 3′-UTR of ABCA1 and promoting the proliferation and slowing the apoptosis of VSMCs (52). Likewise, overexpression of lncRNA maternally expressed gene 8 (MEG8) indirectly targets peroxisome proliferator-activated receptor α (PPARα) by adsorbing miR-181a-5p at the 3′-UTR and positively regulating its expression, thereby inhibiting the proliferation and migration of VSMCs and promoting their apoptosis (53). Another study indicated that lncRNA MEG8 as a ceRNA targeting the miR-195-5p/RECK (reversion inducing cysteine-rich protein with kazal motifs) axis attenuated the hypoxia-induced overproliferation, inflammation, and migration of VSMCs (54). However, overexpression of metastasis associated with lung adenocarcinoma transcript 1 (MALAT1) can also sponge miRNA-124-3p to positively regulate PPARα levels, inhibit proliferation, and promote apoptosis of VSMCs (55). Another study showed that the expression of p53, lincRNA-p21, and sirtuin 7(SIRT7)was downregulated, whereas that of miR-17-5p was upregulated in carotid tissue from AS mice and peripheral blood from patients with AS. p53-dependent lincRNA-p21 could increase SIRT7 expression by binding to miR-17-5p, thereby inhibiting VSMC proliferation and promoting apoptosis, while reducing AS-vulnerable plaques and lipid accumulation in mice (22). Similarly, overexpression of lncRNA small nucleolar RNA host gene 7-003 (SNHG7-003) inhibited the proliferation, migration, and invasion of VSMCs by suppressing miR-1306-5p, which directly binds SIRT7, upregulates its expression, and downregulates the contractile marker α-SMA in VSMCs (56). In contrast, overexpression of lncRNA CAMK2D associated transcript 1 (C2dat1) promoted the proliferation and migration of VSMCs by repressing miR-34a, another member of the SIRT family, by detecting the expression of PCNA, and by wound healing to detect migration (57). LncRNA small nucleolar RNA host gene 12 (SNHG12) was significantly upregulated in ox-LDL-treated hVSMCs. Moreover, SNHG12 acts as a sponge for miR-7665p. Eukaryotic translation initiation factor 5A(EIF5A)is a direct target gene of miR-766-5, and EIF5A promotes the proliferation and migration of ox-LDL-induced hVSMCs. However, silencing lncRNA SNHG12 counteracts the effect of EIF5A. This demonstrates that silencing lncRNA SNHG12 blocks the proliferation and migration of hVSMCs by targeting the miR-766-5p/EIF5A axis (58). In another study, knockdown of lncRNA SHNG12 targeting miR-199a-5p/HIF-1α was shown to be involved in the pathophysiological process of AS by regulating the phenotype of VSMCs (59). LncRNA lymph enhancer-binding factor 1-antisense RNA 1 (LEF1-AS1) regulates the PTEN/PI3K/AKT signaling pathway in VSMCs by targeting miR-544 (60). LINC01123 is highly expressed in patients with carotid atherosclerosis and promotes cell proliferation and migration by regulating the miR-1277-5p/KLF5 axis in ox-LDL-induced VSMCs (61). Similarly, investigators found that in ox-LDL-induced VSMCs, LINC00341 expression was increased, whereas miR-214 expression was significantly decreased. LINC00341 promoted FOXO4 protein expression by adsorbing miR-214, and forkhead box O4 (FOXO4) protein could counteract the promoter region of LINC00341 binding to promote its transcription, and LINC00341 promoted the proliferation and migration of VSMCs by regulating the miR-214/FOXO4 axis (62).
LncRNA and diabetes
It is well known that diabetes can cause extensive damage to the macrovascular and microvascular systems in different organs and tissues, resulting in macrovascular complications like atherosclerosis, hypertension and stroke, and microvascular complications like diabetic nephropathy, diabetic retinopathy, and diabetic neuropathy (90). Therefore, it is important to understand that diabetes-related LncRNAs affect the development of diabetes by regulating smooth muscle cell phenotypic transition.
VSMCs are the main cells involved in the process of medial membrane vascular calcification. Calcified vascular smooth muscle cells can change from a contractile phenotype to a bone/chondrogenic phenotype (91). High glucose induces severe calcification/senescence in HA-VSMCs, a process that is exacerbated by lncRNA-ES3 (LINC00458) expression. Investigators found that lncRNA-ES3 acts as a ceRNA for miR-95-5p, miR-6776-5p, miR-3620-5p, and miR-4747-5p, exacerbating calcification/senescence in HA-VSMCs. Basic helix-loop-helix family member e40 (Bhlhe40) attenuates high glucose-induced calcification/senescence in HA-VSMCs by binding to the promoter region of the lncRNA-ES3 and subsequently regulating its expression in HA-VSMCs (63). Another study showed that lncRNA erythroid pro-survival (lincRNA EPS) could regulate the Wnt/β-catenin pathway by promoting TGF-β expression and interfering with Wnt3 and β-catenin expression, thereby inhibiting the differentiation to osteogenesis and migration of VSMCs and thereby reducing diabetes-related vascular calcification (64).
A study showed that lncRNA urothelial carcinoma associated 1 (UCA1) was significantly downregulated and miR-582-5p was upregulated in VSMCs and serum exosomes of patients with T2DM. There was a negative correlation between them and miR582-5p was a direct target of lncRNA UCA1. Downregulation of lncRNA UCA1 attenuated the proliferation and invasion of VSMCs induced by increasing glucose dose. However, these inhibited trends were partially abolished by co-transfection of miR582-5p inhibitor; therefore, the authors concluded that miR-582-5p was engaged in the repair of VSMCs induced by lncRNA UCA1 in the hyperglycemic state (65).
LncRNA and hypertension
AngII is an active downstream peptide of the renin–angiotensin system that promotes the proliferation and migration of VSMCs by binding to its receptor (92). VSMCs are the major cellular components of the arterial mesothelium, and their proliferation promotes vascular remodeling in hypertension (93). Vascular remodeling caused by essential hypertension is a major cause of death in patients. Therefore, inhibiting cellular dysfunction and phenotypic transition in VSMCs may be a novel therapeutic strategy for essential hypertension.
The expression of serum lncRNA HLA complex group 18 (HCG18) was reduced in hypertensive patients and PDGF-BB-treated VSMCs. After knockdown of HCG18, the expression levels of contractile phenotypic markers, α-SMA, SM22α, and smoothelin, were significantly reduced in VSMCs, whereas synthetic markers, such as OPN, were increased; that is, knockdown of lncRNA HCG18 promoted the proliferation of VSMCs (67). In PDGF-BB-treated VSMCs, lncRNA growth arrest-specific transcript 5 (GAS5) blocked the PDGF-BB-induced proliferation and migration of VSMCs by competitively binding to miR-21, thereby attenuating its inhibitory effect on programmed cell death 4 (PDCD4). Thus, the lncRNA GAS5/miR-21/PDCD4 axis may be a potential target for hypertension treatment (68).
AngII-treated VSMCs were very close to the in vitro hypertensive state, and the apoptosis rate of VSMCs increased significantly after H2O2 treatment. Knockdown of lncRNA MRAK048635_P1 reversed this change. α-SMA, SM22α, and calponin expression levels were significantly reduced, while OPN expression levels were enhanced. VSMC proliferation and migration also increased. Knockdown of lncRNA MRAK048635_P1 in VSMCs also resulted in the overexpression of cyclin D1, cyclin E, CDK2, and CDK4. In the G1 phase, CDK phosphorylates the Rb protein and activates transcription factor E2F, which regulates the cell cycle and thus promotes the transcription of proliferation-related genes. Therefore, downregulation of lncRNA MRAK048635_P1 expression induces a phenotypic shift from contractile to synthetic VSMCs, promotes VSMC proliferation and migration, and inhibits apoptosis (70).
lncRNA AK098656 is mainly expressed in HASMCs, and lncRNA AK098656 overexpression promotes the proliferation of HASMCs by downregulating α-SMA and upregulating the expression of OPN and collagen-I. LncRNA AK098656 binds directly to both MYH11/FN1 (fibronectin-1) proteins, which can act as a scaffold to drag MYH11 closer to the proteasome to promote its degradation, and it can also mediate MYH11/FN1 degradation through the lysosomal pathway. Expression of MYH11, FN1, and α-SMA was also lower in the thoracic aorta, left renal artery, and superior mesenteric artery of rats overexpressing the lncRNA AK098656 gene, while collagen-I deposition increased, arterial lumen narrowing increased intima-media thickness and intima-media/lumen ratio and reduced vasodilation, which induced resistance to vascular arterial remodeling. LncRNA AK098656 can promote hypertension by accelerating contractile protein degradation, increasing VSMC synthetic markers, and, ultimately, antiatherogenic narrowing (71).
LncRNA and revascularization, vascular remodeling
In a rat balloon injury model of restenosis, the expression of lncRNA H19 and miR-675 increased significantly in the neoplastic endothelium. The lncRNA H19-derived miR-675 was found to regulate PTEN and promote the proliferation of VSMCs by directly targeting the 3′-UTR of PTEN (42). In in-stent restenosis patient sera, lncRNA cyclin-dependent protein kinase inhibitors antisense RNA 1 (CDKN2B-AS1) levels were elevated, and miR-143-3p levels were decreased. In human carotid artery smooth muscle cells (hHCtASMCs), knockdown of lncRNA CDKN2B-AS1 resulted in the inhibition of cell proliferation and migration. miR-143-3p is a target of lncRNA CDKN2B-AS1. The results of in vitro studies suggest that the lncRNA CDKN2B-AS1/miR-143-3p axis may regulate the proliferation and migration of hHCtASMCs (72). Similarly, knockdown of lncRNA colorectal neoplasia differentially expressed (CRNDE] significantly inhibited PDGF-BB-induced proliferation and migration of VSMCs (73).
LncRNA GAS5 expression was reduced in PDGF-BB-induced VSMCs, but lncRNA GAS5 overexpression inhibited VSMC proliferation, blocked the cell cycle in the G1/G0 phase, and enhanced caspase-3 cleavage, promoting cell cycle arrest and apoptosis. Overexpression of lncRNA GAS5 increases the expression of the transcriptional regulator p53 and its downstream genes NOXA and p21. This hypothesis was supported by animal experiments. LncRNA GAS5 inhibited neoplastic endothelial formation by increasing the expression of p53 and its downstream genes NOXA and p21 to suppress VSMC proliferation and induce their apoptosis (69). LncRNA nuclear paraspeckle assembly transcript 1 (NEAT1) expression was elevated in PDGF-BB-induced VSMCs, and knockdown of lncRNA NEAT1 decreased the proliferation and migration ability of VSMCs and significantly reduced neoplastic endosomes, with similar changes in the proliferation markers Ki-67 and SM α-actin. PDGF-BB can also promote the binding of lncRNA NEAT1 to WDR5 to activate gene transcription and shift the SM-specific gene promoter from “open” to “closed” to suppress the expression of specific genes in SMCs, thereby regulating their phenotypic transition (75).
LncRNA and pulmonary arterial hypertension
Pulmonary arterial hypertension (PAH) is a refractory cardiovascular disease characterized mainly by increased pulmonary vascular resistance and pulmonary artery pressure, resulting in vascular remodeling, leading to right ventricular hypertrophy, and eventually right heart failure. Hypoxia is a major factor in PAH pathogenesis. During hypoxic exposure, pulmonary artery smooth muscle cells (PASMCs) undergo excessive proliferation and migration, leading to hypertrophy of PASMCs and narrowing of the pulmonary vascular lumen, resulting in pulmonary hypertension (94).
In hypoxic PASMCs, lncRNA hoxa cluster antisense RNA 3 (Hoxaas3) affects transcriptional regulation by regulating histone H3K9 acetylation, which activates Hoxaas3 upregulation in PASMCs and increases the percentage of cells in the S + G2/M phase. In contrast, knockdown of Hoxaas3 reduces the number of cells in the S + G2/M phase and downregulates PCNA, Ki-67, cyclin A, D, and E expression, thereby inhibiting the proliferation of PASMCs under hypoxic conditions. Overexpression of Hoxa3 can reverse these changes. These results suggest that under hypoxia, Hoxaas3 regulates cell cycle changes by interacting with Homeobox a3 (Hoxa3) to allow PASMCs to proliferate (77). In hypoxia-induced PASMCs, the expression of another lncRNA, ANRIL, is significantly downregulated. The downregulation of lncRNA ANRIL expression caused more PASMCs to move from the G0/G1 phase into the G2/M+S phase, with increased expression of the cell cycle-related proteins, cyclin A, D, and E, and enhanced cell proliferation. In addition, the Transwell migration assay confirmed that the downregulation of ANRIL expression increased the migration of PASMCs under hypoxic conditions (25). A novel LncRNA TCONS_00034812 expression was significantly downregulated in PAH rats and hypoxic pulmonary artery SMCs. LncRNA TCONS_00034812 knockdown similarly increased the percentage of G2/M+S phase cells in PASMCs, ultimately leading to thickening of the pulmonary vascular mesoderm. Storkhead box 1 (STOX1) factor is a downstream lncRNA TCONS_00034812 target, and lncRNA TCONS_00034812 negatively regulates STOX1 to affect the proliferation of PASMCs (78). LncRNA Rps4l expression was downregulated in both hypoxia-induced PH tissues and PASMCs, and hypoxia increased the proportion of cells in the G2/M+S phase. In contrast, overexpression of lncRNA Rps4l inhibited the proliferation of PASMCs and attenuated hypoxia-induced cell cycle progression, causing PASMCs to stagnate in the G0/G1 phase. The increased expression levels of cyclins A, D, and E under hypoxic conditions were reversed by overexpression of lncRNA Rps4l. Upregulation of lncRNA Rps4l expression results in a significant reduction in the migratory capacity of PASMCs under hypoxic conditions by regulating the interleukin enhancer-binding factor 3 (ILF3)/HIF-1α axis (79). The expression of LncRNA AC068039.4, which functions in the same way, is significantly upregulated in hypoxia-induced PASMCs, and knockdown of lncRNA AC068039.4 reduced hypoxia-induced G2/M and S-phase cell percentages and attenuated PASMCs proliferation and migration. LncRNA AC068039.4 also binds miR-26a-5p through the ceRNA pattern to regulate the downstream target gene transient receptor potential canonical 6 (TRPC6) to promote PASMCs proliferation, migration, and cell cycle progression, thereby promoting pulmonary vascular remodeling (80).
The smad pathway is important for the vascular development and differentiation of VSMCs, which requires the phosphorylation of smad transcription factors for its subsequent nuclear translocation, DNA binding, and eventual transcriptional activation. Investigators found that the downregulation of lncRNA MYOSLID attenuates TGF-β1-induced Smad2 phosphorylation, disrupts F-actin formation, and blocks TGF-β1-induced megakaryoblastic leukemia 1 [MKL1) nuclear translocation, suggesting that lncRNA MYOSLID plays a key role in SMAD activation and subsequent transcription of VSMCs, and this study shows that lncRNA MYOSLID promotes the expression of contractile markers by inhibiting the proliferation and migration of VSMCs, but its effect on contractile gene expression in VSMCs is cellular context-dependent and may be restricted to VSMCs (81).
In hypoxia-treated PASMCs and PAH patient sera, investigators found a higher expression level of lncRNA NEAT1, which targets miR-34a-5p, while miR-34a-5p targets KLF4. Hypoxia significantly decreased αSMA and caspase-3 expression and increased PCNA and MMP-2 levels. In contrast, the knockdown of lncRNA NEAT1 reversed these alterations by the adsorption of miR-34a-5p and downregulation of KLF4, thereby slowing the progression of PAH (76).
LncRNA UCA1 was overexpressed in hypoxic HPASMCs, and overexpression of the inhibitor of growth proteins5 (ING5) reduced PCNA expression, inhibited cell viability, and promoted apoptosis in hypoxic HPASMCs, which was reversed by lncRNA UCA1 overexpression. LncRNA UCA1 competes with ING5 for heterogeneous nuclear ribonucleoprotein I, a protein that binds RNA and splice mRNA, and promotes proliferation and inhibit apoptosis (66). In PASMCs of hypoxia-induced rats, the expression of lncRNA CASC2 was significantly reduced and the expression of phenotypic transition markers troponin and α-SMA was reduced, while the amount of syndecan-1 and PCNA was significantly increased, and overexpression of lncRNA CASC2 resulted in opposite changes in the above markers. Therefore, overexpression of lncRNA CASC2 alleviated hypoxia-induced cell proliferation and migration, thereby regulating phenotype transition in PASMCs to partially restore hypoxia (51).
LncRNA and aneurysm
Aortic aneurysms are usually defined as localized dilatations larger than 50% of the normal diameter and can occur in the thorax, but have the highest incidence in the abdominal aorta (95). Many inflammatory factors, such as CC chemokine ligand 2 (CCL2), IL-6, IL-1β, and TNFα, induce a chronic inflammatory response, inflammatory cell infiltration accompanied by elastin disruption and degeneration, and loss of mesangial SMCs. The pathophysiological process of aortic aneurysms is characterized by inflammatory cell infiltration, elastic and collagen fiber degradation, smooth muscle cell death, arterial wall defects, and increased oxidative stress (96). There is growing evidence that lncRNA promotes the proliferation of VSMCs or inhibiting apoptosis can prevent aneurysm progression.
In the thoracic aortic tissue of patients with aortic dissection (AD), lncRNA H19 was highly expressed, which competitively bound and inhibited the expression of miR-193b-3p. Upon PDGF-BB induction, the expression of lncRNA H19, MMP-2, and MMP-9 was upregulated; the expression of miR-193b-3p, α-SMA, and SM22α was downregulated; and the proliferation and migration rates of HASMCs were increased. However, silencing lncRNA H19 reversed the change induced by PDGF-BB. These results were consistently validated in animal experiments, indicating that silencing lncRNA H19 significantly attenuated PDGF-BB-induced proliferation and migration of HASMCs through the upregulation of miR-193b-3p, thereby reducing pathological injury in the thoracic aorta of AD mice (43). LncRNA X-inactive-specific transcript (XIST] is upregulated in the aortic wall tissue of patients with Stanford type A aortic dissection (TAAD) and correlates with the prognosis of TAAD. Knockdown of lncRNA XIST regulates downstream PTEN by inhibiting miR-17, which increases PCNA expression, accelerates Bcl-2 expression, and suppresses the levels of Bax and caspase-3, thereby promoting VSMC proliferation and inhibiting apoptosis to slow TAAD progression (49). Tissues near endothelial tears in patients with AD were proliferating; the expression of linc01278 and ACTG2 was downregulated; miR-500b-5p expression was upregulated; VSMC differentiation markers SMA, SM22α, calponin, and MYH11 were decreased. Silencing linc01278 targeted miR-500b-5p and ACTG2 in the three untranslated regions decreased the expression of SMA, SM22α, calponin, and MYH1; promoted the phenotypic conversion of aortic VSMCs from contractile to synthetic phenotypes; and promoted VSMC proliferation and migration. Thus, the linc01278/miR-500b-5p/ACTG2 axis may provide novel molecular mechanisms for diagnostic markers and therapeutic targets of AD (82). In AD, another lncRNA, PVT1 expression was upregulated, while the downregulation of lncRNA plasmacytoma variant translocation 1 (PVT1) expression led to an increase in α-SMA and SM22α expression and decreased MMP-2 and MMP-9 expression by targeting miR-27b-3p, which inhibited phenotypic transition and suppressed proliferation and migration in PDGF-BB-treated HASMCs (83).
In SMCs, the lncRNA lung cancer-associated transcript 1 (LUCAT1) exhibits anti-proliferative and pro-apoptotic effects, and knockdown of LUCAT1 leads to decreased caspase-3 activity and recovery after myelin regulatory factor (MYRF) overexpression. LUCAT1 acts as a decoy for miR-199a-5p and promotes MYRF expression, and lncRNA LUCAT1/miR-199a-5p/MYRF regulates the proliferation and apoptosis of SMCs in abdominal aortic aneurysms (85). In abdominal aortic aneurysm (AAA) tissues, lncRNA PVT1, and NCK-associated protein 1-like (NCKAP1L) expression was elevated and induced in vitro in AAA models, while miR-3127-5p showed the opposite trend, and lncRNA PVT1 acted as a sponge for miR-3127-5p to regulate NCKAP1L expression, inhibit VSMC proliferation, and induce apoptosis (84). In contrast, in AAA tissue, lncRNA SNHG5 was downregulated; overexpression of lncRNA SNHG5 could act as a molecular sponge for miR-205-5p and downregulate its expression, but upregulate the expression of SMAD4, thus increasing proliferation and migration and decreasing apoptosis in abdominal aortic aneurysm VSMCs (86). Another study found that H2O2 inhibited the activity of VSMCs, thus mimicking the AAA model. After H2O2 treatment, LINC00473 expression was upregulated, Bax expression was enhanced, and Bcl-2 expression was decreased. In AAA, brain acid-soluble protein 1 (BASP1) expression was inversely correlated with miR-212-5p expression but positively correlated with LINC00473 levels. These results suggest that LINC00473 competitively interacts with miR-212-5p to promote BASP1 expression and VSMC apoptosis, ultimately leading to AAA exacerbation (87). In AAA tissues and AngII-stimulated VSMCs, the expression of lncRNA CRNDE was downregulated, and the data suggest that overexpression of lncRNA CRNDE can promote VSMC proliferation and inhibit apoptosis by upregulating Bcl-3 ubiquitination of Smad3 protein and upregulating smad3 expression, thereby inhibiting mouse AAA growth (74).
Conclusion and perspectives
LncRNAs are relatively newly discovered RNA molecules with important regulatory functions. These findings suggest that lncRNAs may have profound effects on the regulation of VSMCs and are regulators of gene expression and vascular function. Although our knowledge of lncRNAs is limited, their emergence may further our understanding of the complex regulatory network of cellular function in clinical vascular diseases. Targeting lncRNAs may be an extremely promising modality of governance not only in tumors but also in cardiac or vascular diseases, and thus, they are regulators of smooth muscle cell phenotypic transition.
Author contributions
B-HL and H-BL: original draft writing and manuscript revision. D-XL and Z-GC: manuscript revision. S-XG and JZ: graphic design. G-AZ and FL: manuscript design and revision.
Funding
This work was supported by the Research Projects of Higher Education Institutions in Henan Province of China (Nos: 21A320012 and 22A360017), Key Specialized Research and Development Breakthrough in Henan Province of China (Nos: 212102310350, 222102310442, and 222102310631), Graduate Student Research Innovation Support Program of Xinxiang Medical University in Henan Province of China (YJSCX202174Y), and The First Affiliated Hospital of Xinxiang Medical University Youth Foundation (Grant Nos. QN-2017-B026 and QN-2021-B15).
Acknowledgments
We would like to thank Editage (www.editage.cn) for English language editing.
Conflict of interest
The authors declare that the research was conducted in the absence of any commercial or financial relationships that could be construed as a potential conflict of interest.
Publisher's note
All claims expressed in this article are solely those of the authors and do not necessarily represent those of their affiliated organizations, or those of the publisher, the editors and the reviewers. Any product that may be evaluated in this article, or claim that may be made by its manufacturer, is not guaranteed or endorsed by the publisher.
References
1. Brunner AL, Beck AH, Edris B, Sweeney RT, Zhu SX, Li R, et al. Transcriptional profiling of long non-coding RNAs and novel transcribed regions across a diverse panel of archived human cancers. Genome Biol. (2012) 13:1–13. doi: 10.1186/gb-2012-13-8-r75
2. Scheuermann JC, Boyer LA. Getting to the heart of the matter: long non-coding RNAs in cardiac development and disease. EMBO J. (2013) 32:1805–16. doi: 10.1038/emboj.2013.134
3. Herring BP, Hoggatt AM, Burlak C, Offermanns S. Previously differentiated medial vascular smooth muscle cells contribute to neointima formation following vascular injury. Vasc Cell. (2014) 6:21. doi: 10.1186/2045-824X-6-21
4. Shi N, Chen SY. Mechanisms simultaneously regulate smooth muscle proliferation and differentiation. J Biomed Res. (2014) 28:40–46. doi: 10.7555/JBR.28.20130130
5. Uchida S, Dimmeler S. Long non-coding RNAs in cardiovascular diseases. Circ Res. (2015) 116:737–50. doi: 10.1161/CIRCRESAHA.116.302521
6. Leeper NJ, Maegdefessel L. Non-coding RNAs: key regulators of smooth muscle cell fate in vascular disease. Cardiovasc Res. (2018) 114:611–21. doi: 10.1093/cvr/cvx249
7. Basatemur GL, Jørgensen HF, Clarke MCH, Bennett MR, Mallat Z. Vascular smooth muscle cells in atherosclerosis. Nat Rev Cardiol. (2019) 16:727–44. doi: 10.1038/s41569-019-0227-9
8. Dobnikar L, Taylor AL, Chappell J, Oldach P, Harman JL, Oerton E, et al. Disease-relevant transcriptional signatures identified in individual smooth muscle cells from healthy mouse vessels. Nat Commun. (2018) 9:4567. doi: 10.1038/s41467-018-06891-x
9. Wang G, Jacquet L, Karamariti E, Xu Q. Origin and differentiation of vascular smooth muscle cells. J Physiol. (2015) 593:3013–30. doi: 10.1113/JP270033
10. Allahverdian S, Chaabane C, Boukais K, Francis GA, Bochaton-Piallat ML. Smooth muscle cell fate and plasticity in atherosclerosis. Cardiovasc Res. (2018)0.114:540–50. doi: 10.1093/cvr/cvy022
11. Wang KC, Chang HY. Molecular mechanisms of long noncoding RNAs. Mol Cell. (2011) 43:904–14. doi: 10.1016/j.molcel.2011.08.018
12. Jian L, Jian D, Chen Q, Zhang L. Long Noncoding RNAs in Atherosclerosis. J Atheroscler Thromb. (2016) 23:376–84. doi: 10.5551/jat.33167
13. Kallen AN, Zhou XB, Xu J, Qiao C, Ma J, Yan L, et al. The imprinted H19 lncRNA antagonizes let-7 microRNAs. Mol Cell. (2013) 52:101–12. doi: 10.1016/j.molcel.2013.08.027
14. Ferre F, Colantoni A, Helmer-Citterich M. Revealing protein-lncRNA interaction. Brief Bioinform. (2016)17:106–16. doi: 10.1093/bib/bbv031
15. Imig J, Brunschweiger A, Brümmer A, Guennewig B, Mittal N, Kishore S, et al. miR-CLIP capture of a miRNA targetome uncovers a lincRNA H19-miR-106a interaction. Nat Chem Biol. (2015) 11:107–14. doi: 10.1038/nchembio.1713
16. Kung JTY, Colognori D, Lee JT. Long noncoding RNAs: past, present, and future. Genetics. (2013) 193:651–69. doi: 10.1534/genetics.112.146704
17. Deng L, Bradshaw AC, Baker AH. Role of non-coding RNA in vascular remodelling. Curr Opin Lipidol. (2016) 27:439–48. doi: 10.1097/MOL.0000000000000336
18. Miano JM, Long X. The short and long of noncoding sequences in the control of vascular cell phenotypes. Cell Mol Life Sci. (2015) 72:3457–88. doi: 10.1007/s00018-015-1936-9
19. Zhang M, He J, Jiang C, Zhang W, Yang Y, Wang Z, et al. Plaque-hyaluronidase-responsive high-density-lipoprotein-mimetic nanoparticles for multistage intimal-macrophage-targeted drug delivery and enhanced anti-atherosclerotic therapy. Int J Nanomed. (2017) 12:533–58. doi: 10.2147/IJN.S124252
20. Ballantyne MD, Pinel K, Dakin R, Vesey AT, Diver L, Mackenzie R, et al. Smooth Muscle Enriched Long Non-coding RNA (SMILR) regulates cell proliferation. Circulation. (2016) 133:2050–65. doi: 10.1161/CIRCULATIONAHA.115.021019
21. Wu G, Cai J, Han Y, Chen J, Huang ZP, Chen C, et al. LincRNA-p21 regulates neointima formation, vascular smooth muscle cell proliferation, apoptosis, and atherosclerosis by enhancing p53 activity. Circulation. (2014) 130:1452–65. doi: 10.1161/res.115.suppl_1.32
22. Wang H, He F, Liang B, Jing Y, Zhang P, Liu W, et al. p53-dependent LincRNA-p21 protects against proliferation and anti-apoptosis of vascular smooth muscle cells in atherosclerosis by upregulating SIRT7 via microRNA-17-5p. J Cardiovasc Transl Res. (2021) 14:426–40. doi: 10.1007/s12265-020-10074-9
23. Zhang C, Ge S, Gong W, Xu J, Guo Z, Liu Z, et al. LncRNA ANRIL acts as a modular scaffold of WDR5 and HDAC3 complexes and promotes alteration of the vascular smooth muscle cell phenotype. Cell Death Dis. (2020) 11:435. doi: 10.1038/s41419-020-2645-3
24. Li J, Chen J, Zhang F, Li J, An S, Cheng M, et al. LncRNA CDKN2B-AS1 hinders the proliferation and facilitates apoptosis of ox-LDL-induced vascular smooth muscle cells via the ceRNA network of CDKN2B-AS1/miR-126-5p/PTPN7. Int J Cardiol. (2021) 340:79–87. doi: 10.1016/j.ijcard.2021.08.009
25. Wang S, Zhang C, Zhang X. Downregulation of long non-coding RNA ANRIL promotes proliferation and migration in hypoxic human pulmonary artery smooth muscle cells. Mol Med Rep. (2020) 21:589–96. doi: 10.3892/mmr.2019.10887
26. Lu YB, Shi C, Yang B, Lu ZF, Wu YL, Zhang RY, et al. Long non-coding RNA ZNF800 suppresses proliferation and migration of vascular smooth muscle cells by upregulating PTEN and inhibiting AKT/mTOR/HIF-1α signaling. Atherosclerosis. (2020) 312:43–53. doi: 10.1016/j.atherosclerosis.2020.09.007
27. Fasolo F, Jin H, Winski G, Chernogubova E, Pauli J, Winter H, et al. Long non-coding rna controls advanced atherosclerotic lesion formation and plaque destabilization. Circulation. (2021) 144:1567–83. doi: 10.1161/CIRCULATIONAHA.120.052023
28. Ma G, Bi S, Zhang P. Long non-coding RNA MIAT regulates ox-LDL-induced cell proliferation, migration and invasion by miR-641/STIM1 axis in human vascular smooth muscle cells. BMC Cardiovasc Disord. (2021) 21:248. doi: 10.1186/s12872-021-02048-9
29. Cui C, Wang X, Shang XM, Li L, Ma Y, Zhao GY, et al. lncRNA 430945 promotes the proliferation and migration of vascular smooth muscle cells via the ROR2/RhoA signaling pathway in atherosclerosis. Mol Med Rep. (2019) 19:4663–72. doi: 10.3892/mmr.2019.10137
30. Jiang X, Liu Z, Qi X. LncRNA BANCR induced vascular smooth muscle cell proliferation by downregulating miR-34c methylation in atherosclerosis. J Thromb Thrombolysis. (2021) 51:924–32. doi: 10.1007/s11239-020-02314-1
31. Wu Y, Cai F, Lu Y, Hu Y, Wang Q. lncRNA RP11-531A243 inhibits the migration and proliferation of vascular smooth muscle cells by downregulating ANXA2 expression. Exp Ther Med. (2021) 22:1439. doi: 10.3892/etm.2021.10874
32. Onuh JO, Qiu H. Serum response factor-cofactor interactions and their implications in disease. FEBS J. (2021) 288:3120–34. doi: 10.1111/febs.15544
33. Miano JM. Myocardin in biology and disease. J Biomed Res. (2015) 29:3–19. doi: 10.7555/JBR.29.20140151
34. Dong K, Shen J, He X, Hu G, Wang L, Osman I, et al. Is an Evolutionarily Conserved Smooth Muscle Cell-Specific LncRNA That Maintains Contractile Phenotype by Binding Myocardin. Circulation. (2021) 144:1856–75. doi: 10.1161/CIRCULATIONAHA.121.055949
35. He X, Lian Z, Yang Y, Wang Z, Fu X, Liu Y, et al. Long non-coding RNA PEBP1P2 Suppresses Proliferative VSMCs Phenotypic Switching and Proliferation in Atherosclerosis. Mol Ther Nucleic Acids. (2020) 22:84–98. doi: 10.1016/j.omtn.2020.08.013
36. Hung J, Miscianinov V, Sluimer JC, Newby DE, Baker AH. Targeting non-coding RNA in vascular biology and disease. Front Physiol. (2018) 9:1655. doi: 10.3389/fphys.2018.01655
37. Wang X, Li D, Chen H, Wei X, Xu X. Expression of long non-coding RNA LIPCAR promotes cell proliferation, cell migration, and change in phenotype of vascular smooth muscle cells. Med Sci Monit. (2019) 25:7645–51. doi: 10.12659/MSM.915681
38. Cai X, Cullen BR. The imprinted H19 non-coding RNA is a primary microRNA precursor. RNA. (2007) 13:313–6. doi: 10.1261/rna.351707
39. Sun H, Jiang Q, Sheng L, Cui K. Downregulation of lncRNA H19 alleviates atherosclerosis through inducing the apoptosis of vascular smooth muscle cells. Mol Med Rep. (2020) 22:3095–102. doi: 10.3892/mmr.2020.11394
40. Lu G, Chu Y, Tian P. Knockdown of H19 Attenuates Ox-LDL-induced vascular smooth muscle cell proliferation, migration, and invasion by regulating miR-599/PAPPA axis. J Cardiovasc Pharmacol. (2021) 77:386–96. doi: 10.1097/FJC.0000000000000959
41. Zhang L, Cheng H, Yue Y, Li S, Zhang D, He R, et al. H19 knockdown suppresses proliferation and induces apoptosis by regulating miR-148b/WNT/β-catenin in ox-LDL -stimulated vascular smooth muscle cells. J Biomed Sci. (2018) 25:11. doi: 10.1186/s12929-018-0418-4
42. Lv J, Wang L, Zhang J, Lin R, Wang L, Sun W, et al. Long noncoding RNA H19-derived miR-675 aggravates restenosis by targeting PTEN. Biochem Biophys Res Commun. (2018) 497:1154–61. doi: 10.1016/j.bbrc.2017.01.011
43. Ren M, Wang T, Wei X, Wang Y, Ouyang C, Xie Y, et al. LncRNA H19 regulates smooth muscle cell functions and participates in the development of aortic dissection through sponging miR-193b-3p. Biosci Rep. (2021) 41. doi: 10.1042/BSR20202298
44. Zhang RY, Wu CM, Hu XM, Lin XM, Hua YN, Chen JJ, et al. LncRNA AC105942.1 downregulates hnRNPA2/B1 to attenuate vascular smooth muscle cells proliferation. DNA Cell Biol. (2021) 40:652–61. doi: 10.1089/dna.2020.6451
45. Li FP, Lin DQ, Gao LY. LncRNA TUG1 promotes proliferation of vascular smooth muscle cell and atherosclerosis through regulating miRNA-21/PTEN axis. Eur Rev Med Pharmacol Sci. (2018) 22:7439–47. doi: 10.26355/eurrev_201811_16284
46. Wang YQ, Xu ZM, Wang XL, Zheng JK, Du Q, Yang JX, et al. LncRNA FOXC2-AS1 regulated proliferation and apoptosis of vascular smooth muscle cell through targeting miR-1253/FOXF1 axis in atherosclerosis. Eur Rev Med Pharmacol Sci. (2020) 24:3302–14.
47. Liu Y, Cui X, Wang C, Zhao S. LncRNA HCG11 regulates proliferation and apoptosis of vascular smooth muscle cell through targeting miR-144-3p/FOXF1 axis in atherosclerosis. Biol Res. (2020) 53:44. doi: 10.1186/s40659-020-00306-2
48. Wang Y, Zhang CX, Ge SL, Gong WH. CTBP1-AS2 inhibits proliferation and induces autophagy in ox-LDL-stimulated vascular smooth muscle cells by regulating miR-195-5p/ATG14. Int J Mol Med. (2020) 46:839–48. doi: 10.3892/ijmm.2020.4624
49. Zhang X, Wu H, Mai C, Qi Y. Long non-coding RNA XIST/miR-17/PTEN axis modulates the proliferation and apoptosis of vascular smooth muscle cells to affect stanford Type A aortic dissection. J Cardiovasc Pharmacol. (2020) 76:53–62. doi: 10.1097/FJC.0000000000000835
50. Wang C, Zhao J, Nan X, Guo Z, Huang S, Wang X, et al. Long noncoding RNA CASC2 inhibits ox-LDL-mediated vascular smooth muscle cells proliferation and migration via the regulation of miR-532-3p/PAPD5. Mol Med. (2020) 26:74. doi: 10.1186/s10020-020-00200-3
51. Gong J, Chen Z, Chen Y, Lv H, Lu H, Yan F, et al. Long non-coding RNA CASC2 suppresses pulmonary artery smooth muscle cell proliferation and phenotypic switch in hypoxia-induced pulmonary hypertension. Respir Res. (2019) 20:53. doi: 10.1186/s12931-019-1018-x
52. Wang M, Li C, Zhang Y, Zhou X, Liu Y, Lu C, et al. LncRNA MEG3-derived miR-361-5p regulate vascular smooth muscle cells proliferation and apoptosis by targeting ABCA1. Am J Transl Res. (2019) 11:3600–9.
53. Zhang B, Dong Y, Zhao Z. LncRNA MEG8 regulates vascular smooth muscle cell proliferation, migration and apoptosis by targeting PPARα. Biochem Biophys Res Commun. (2019) 510:171–6. doi: 10.1016/j.bbrc.2019.01.074
54. Xu D, Dai R, Chi H, Ge W, Rong J. Long non-coding RNA MEG8 suppresses hypoxia-induced excessive proliferation, migration and inflammation of vascular smooth muscle cells by regulation of the miR-195-5p/RECK axis. Front Mol Biosci. (2021) 8:697273. doi: 10.3389/fmolb.2021.697273
55. Cheng C, Xu BL, Sheng JL, He F, Yang T, Shen SC, et al. LncRNA MALAT1 regulates proliferation and apoptosis of vascular smooth muscle cells by targeting miRNA-124-3p/PPARα axis. Eur Rev Med Pharmacol Sci. (2019) 23:9025–32.
56. Zheng J, Tan Q, Chen H, Chen K, Wang H, Chen Z, et al. lncRNA-SNHG7-003 inhibits the proliferation, migration and invasion of vascular smooth muscle cells by targeting the miR-1306-5p/SIRT7 signaling pathway. Int J Mol Med. (2021) 47:741–50. doi: 10.3892/ijmm.2020.4821
57. Wang H, Jin Z, Pei T, Song W, Gong Y, Chen D, et al. Long non-coding RNAs C2dat1 enhances vascular smooth muscle cell proliferation and migration by targeting MiR-34a-5p. J Cell Biochem. (2019) 120:3001–8. doi: 10.1002/jcb.27070
58. Liu W, Che J, Gu Y, Song L, Jiao Y, Yu S, et al. Silencing of lncRNA SNHG12 inhibits proliferation and migration of vascular smooth muscle cells via targeting miR-766-5p/EIF5A axis. Adv Clin Exp Med. (2021) 30:591–8. doi: 10.1007/978-981-16-0267-2
59. Sun Y, Zhao JT, Chi BJ, Wang KF. Long non-coding RNA SNHG12 promotes vascular smooth muscle cell proliferation and migration via regulating miR-199a-5p/HIF-1α. Cell Biol Int. (2020) 44:1714–26. doi: 10.1002/cbin.11365
60. Zhang L, Zhou C, Qin Q, Liu Z, Li P. LncRNA LEF1-AS1 regulates the migration and proliferation of vascular smooth muscle cells by targeting miR-544a/PTEN axis. J Cell Biochem. (2019) 120:14670–8. doi: 10.1002/jcb.28728
61. Weng G, Gu M, Zhang Y, Zhao G, Gu Y. LINC01123 promotes cell proliferation and migration via regulating miR-1277-5p/KLF5 axis in ox-LDL-induced vascular smooth muscle cells. J Mol Histol. (2021) 52:943–53. doi: 10.1007/s10735-021-10010-4
62. Liu X, Ma BD, Liu S, Liu J, Ma BX. Long noncoding RNA LINC00341 promotes the vascular smooth muscle cells proliferation and migration via miR-214/FOXO4 feedback loop. Am J Transl Res. (2019) 11:1835–42.
63. Zhong JY, Cui XJ, Zhan JK, Wang YJ, Li S, Lin X, et al. LncRNA-ES3 inhibition by Bhlhe40 is involved in high glucose-induced calcification/senescence of vascular smooth muscle cells. Ann N Y Acad Sci U.S.A. (2020) 1474:61–72. doi: 10.1111/nyas.14381
64. Li Y, Xi Z, Yu Z, Yang C, Tan C. LincRNA-EPS increases TGF-β expression to inhibit the Wnt/β-catenin pathway, VSMC osteoblastic differentiation and vascular calcification in diabetic mice. Exp Ther Med. (2022) 23:425. doi: 10.3892/etm.2022.11352
65. Yang JL, Han NH. LncRNA UCA1 stimulates the repair of hyperglycemic vascular smooth muscle cells through targeting miR-582-5p. Eur Rev Med Pharmacol Sci. (2020) 24:12859–66. doi: 10.26355/eurrev_202012_24188
66. Zhu TT, Sun RL, Yin YL, Quan JP, Song P, Xu J, et al. Long noncoding RNA UCA1 promotes the proliferation of hypoxic human pulmonary artery smooth muscle cells. Pflugers Arch. (2019) 471:347–55. doi: 10.1007/s00424-018-2219-8
67. Lu Y, Guo J, Zhu S, Zhang H, Zhu Q, Li Y, et al. LncRNA HCG18 is critical for vascular smooth muscle cell proliferation and phenotypic switching. Hum Cell. (2020) 33:537–44. doi: 10.1007/s13577-020-00366-2
68. Liu K, Liu C, Zhang Z. lncRNA GAS5 acts as a ceRNA for miR-21 in suppressing PDGF-bb-induced proliferation and migration in vascular smooth muscle cells. J Cell Biochem. (2019) 120:15233–40. doi: 10.1002/jcb.28789
69. Tang R, Mei X, Wang YC, Cui XB, Zhang G, Li W, et al. LncRNA GAS5 regulates vascular smooth muscle cell cycle arrest and apoptosis via p53 pathway. Biochim Biophys Acta Mol Basis Dis. (2019) 1865:2516–25. doi: 10.1016/j.bbadis.2019.05.022
70. Fang G, Qi J, Huang L, Zhao X. LncRNA MRAK048635_P1 is critical for vascular smooth muscle cell function and phenotypic switching in essential hypertension. Biosci Rep. (2019) 39. doi: 10.1042/BSR20182229
71. Jin L, Lin X, Yang L, Fan X, Wang W, Li S, et al. AK098656 a novel vascular smooth muscle cell-dominant long non-coding RNA, promotes hypertension. Hypertension. (2018) 71:262–72. doi: 10.1161/HYPERTENSIONAHA.117.09651
72. Ma H, Dong A. Long non-coding RNA cyclin-dependent kinase inhibitor 2B antisense ribonucleic acid 1 is associated with in-stent restenosis and promotes human carotid artery smooth muscle cell proliferation and migration by sponging miR-143-3p. Exp Ther Med. (2021) 21:234. doi: 10.3892/etm.2021.9665
73. Zhou Y, He X, Liu R, Qin Y, Wang S, Yao X, et al. LncRNA CRNDE regulates the proliferation and migration of vascular smooth muscle cells. J Cell Physiol. (2019) 23:16205-14 doi: 10.1002/jcp.28284
74. Li K, Cui M, Zhang K, Wang G, Zhai S. LncRNA CRNDE affects the proliferation and apoptosis of vascular smooth muscle cells in abdominal aortic aneurysms by regulating the expression of Smad3 by Bcl-3. Cell Cycle. (2020) 19:1036–47. doi: 10.1080/15384101.2020.1743915
75. Ahmed ASI, Dong K, Liu J, Wen T, Yu L, Xu F, et al. Long noncoding RNA (nuclear paraspeckle assembly transcript 1) is critical for phenotypic switching of vascular smooth muscle cells. Proc Natl Acad Sci U.S.A. (2018) 115:E8660–7. doi: 10.1073/pnas.1803725115
76. Dou X, Ma Y, Qin Y, Dong Q, Zhang S, Tian R, et al. NEAT1 silencing alleviates pulmonary arterial smooth muscle cell migration and proliferation under hypoxia through regulation of miR-34a-5p/KLF4. Mol Med Rep. (2021) 24. doi: 10.3892/mmr.2021.12389
77. Zhang H, Liu Y, Yan L, Wang S, Zhang M, Ma C, et al. Long noncoding RNA Hoxaas3 contributes to hypoxia-induced pulmonary artery smooth muscle cell proliferation. Cardiovasc Res. (2019) 115:647–57. doi: 10.1093/cvr/cvy250
78. Liu Y, Sun Z, Zhu J, Xiao B, Dong J, Li X, et al. LncRNA-TCONS_00034812 in cell proliferation and apoptosis of pulmonary artery smooth muscle cells and its mechanism. J Cell Physiol. (2018) 233:4801–14. doi: 10.1002/jcp.26279
79. Liu Y, Zhang H, Li Y, Yan L, Du W, Wang S, et al. Long non-coding RNA Rps4l mediates the proliferation of hypoxic pulmonary artery smooth muscle cells. Hypertension. (2020) 76:1124–33. doi: 10.1161/HYPERTENSIONAHA.120.14644
80. Qin Y, Zhu B, Li L, Wang D, Qiao Y, Liu B, et al. Overexpressed lncRNA AC068039.4 contributes to proliferation and cell cycle progression of pulmonary artery smooth muscle cells via sponging miR-26a-5p/TRPC6 in hypoxic pulmonary arterial hypertension. Shock. (2021) 55:244–55. doi: 10.1097/SHK.0000000000001606
81. Zhao J, Zhang W, Lin M, Wu W, Jiang P, Tou E, et al. MYOSLID Is a Novel Serum Response Factor-Dependent Long Noncoding RNA That Amplifies the Vascular Smooth Muscle Differentiation Program. Arterioscler Thromb Vasc Biol. (2016) 36:2088–99. doi: 10.1161/ATVBAHA.116.307879
82. Wang W, Liu Q, Wang Y, Piao H, Zhu Z, Li D, et al. LINC01278 Sponges miR-500b-5p to regulate the expression of ACTG2 to control phenotypic switching in human vascular smooth muscle cells during aortic dissection. J Am Heart Assoc. (2021) 10:e018062. doi: 10.1161/JAHA.120.018062
83. Li S, Zhao X, Cheng S, Li J, Bai X, Meng X, et al. Downregulating long non-coding RNA PVT1 expression inhibited the viability, migration and phenotypic switch of PDGF-BB-treated human aortic smooth muscle cells via targeting miR-27b-3p. Hum Cell. (2021) 34:335–48. doi: 10.1007/s13577-020-00452-5
84. Huang Y, Ren L, Li J, Zou H. Long non-coding RNA PVT1/microRNA miR-3127-5p/NCK-associated protein 1-like axis participates in the pathogenesis of abdominal aortic aneurysm by regulating vascular smooth muscle cells. Bioengineered. (2021) 12:12583–96. doi: 10.1080/21655979.2021.2010384
85. Xia Q, Zhang L, Yan H, Yu L, Shan W, Jiang H, et al. LUCAT1 contributes to MYRF-dependent smooth muscle cell apoptosis and may facilitate aneurysm formation via the sequestration of miR-199a-5p. Cell Biol Int. (2020) 44:755–63. doi: 10.1002/cbin.11270
86. Nie H, Zhao W, Wang S, Zhou W. Based on bioinformatics analysis lncrna SNHG5 modulates the function of vascular smooth muscle cells through mir-205-5p/SMAD4 in abdominal aortic aneurysm. Immun Inflamm Dis. (2021) 9:1306–20. doi: 10.1002/iid3.478
87. Tian Z, Sun Y, Sun X, Wang J, Jiang T. LINC00473 inhibits vascular smooth muscle cell viability to promote aneurysm formation via miR-212-5p/BASP1 axis. Eur J Pharmacol. (2020) 873:172935. doi: 10.1016/j.ejphar.2020.172935
88. Hu DJ, Li ZY, Zhu YT, Li CC. Overexpression of long noncoding RNA ANRIL inhibits phenotypic switching of vascular smooth muscle cells to prevent atherosclerotic plaque development. Aging. (2020) 13:4299–316. doi: 10.18632/aging.202392
89. Zhang Y, Tang Y, Yan J. LncRNA-XIST Promotes Proliferation and Migration in ox-LDL Stimulated Vascular Smooth Muscle Cells through miR-539-5p/SPP1 Axis. Oxid Med Cell Longev. (2022) 2022:9911982. doi: 10.1155/2022/9911982
90. Feng SD, Yang JH, Yao CH, Yang SS, Zhu ZM, Wu D, et al. Potential regulatory mechanisms of lncRNA in diabetes and its complications. Biochem Cell Biol. (2017) 95:361–7. doi: 10.1139/bcb-2016-0110
91. Shi J, Yang Y, Cheng A, Xu G, He F. Metabolism of vascular smooth muscle cells in vascular diseases. Am J Physiol Heart Circ Physiol. (2020) 319:H613–31. doi: 10.1152/ajpheart.00220.2020
92. Montezano AC, Nguyen Dinh Cat A, Rios FJ, Touyz RM. Angiotensin II and vascular injury. Curr Hypertens Rep. (2014) 16:431. doi: 10.1007/s11906-014-0431-2
93. Brown IA, Diederich L, Good ME, DeLalio LJ, Murphy SA, Cortese-Krott MM, et al. Vascular smooth muscle remodeling in conductive and resistance arteries in hypertension. Arterioscler Thromb Vasc Biol. (2018) 38:1969–85. doi: 10.1161/ATVBAHA.118.311229
94. Galie N, Hoeper MM, Humbert M, Torbicki A, Vachiery JL, Barbera JA, et al. 2015 ESC/ERS Guidelines for the diagnosis and treatment of pulmonary hypertension: the joint task force for the diagnosis and treatment of pulmonary hypertension of the European Society of Cardiology (ESC) and the European Respiratory Society (ERS): Endorsed by: association for European Paediatric and Congenital Cardiology (AEPC), International Society for Heart and Lung Transplantation (ISHLT). Eur Heart J. 37 doi: 10.1093/eurheartj/ehv317
95. Kuivaniemi H, Elmore JR. Opportunities in abdominal aortic aneurysm research: epidemiology, genetics, and pathophysiology. Ann Vasc Surg. (2012) 26:862–70. doi: 10.1016/j.avsg.2012.02.005
Keywords: long non-coding RNAs, vascular smooth muscle cells, phenotypic transformation, vascular disease, atherosclerosis
Citation: Lu B-H, Liu H-B, Guo S-X, Zhang J, Li D-X, Chen Z-G, Lin F and Zhao G-A (2022) Long non-coding RNAs: Modulators of phenotypic transformation in vascular smooth muscle cells. Front. Cardiovasc. Med. 9:959955. doi: 10.3389/fcvm.2022.959955
Received: 02 June 2022; Accepted: 01 August 2022;
Published: 26 August 2022.
Edited by:
Wen-Jun Tu, Chinese Academy of Medical Sciences and Peking Union Medical College, ChinaReviewed by:
Beatrice Charreau, Université de Nantes, FranceMadeline Nieves-Cintron, University of California, Davis, United States
Copyright © 2022 Lu, Liu, Guo, Zhang, Li, Chen, Lin and Zhao. This is an open-access article distributed under the terms of the Creative Commons Attribution License (CC BY). The use, distribution or reproduction in other forums is permitted, provided the original author(s) and the copyright owner(s) are credited and that the original publication in this journal is cited, in accordance with accepted academic practice. No use, distribution or reproduction is permitted which does not comply with these terms.
*Correspondence: Fei Lin, bGluZmVpeGl4aUBhbGl5dW4uY29t; Guo-An Zhao, Z3VvYW56aGFvQHh4bXUuZWR1LmNu
†These authors share first authorship