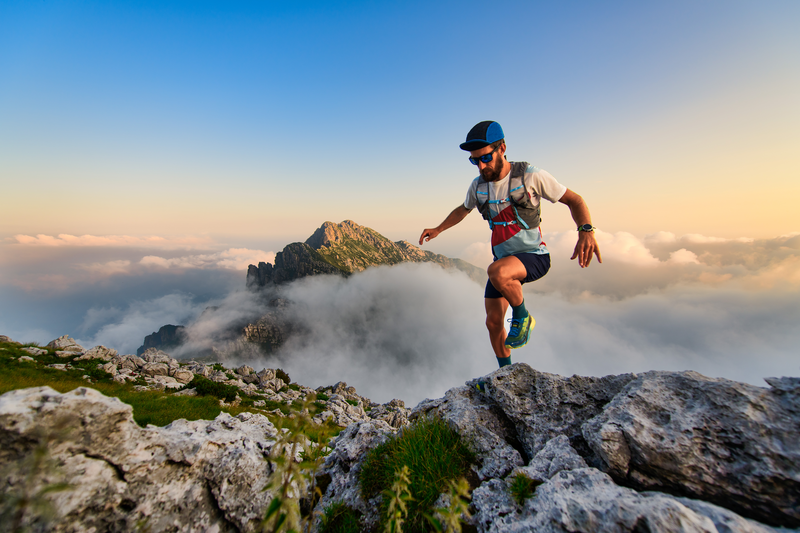
95% of researchers rate our articles as excellent or good
Learn more about the work of our research integrity team to safeguard the quality of each article we publish.
Find out more
MINI REVIEW article
Front. Cardiovasc. Med. , 29 August 2022
Sec. Lipids in Cardiovascular Disease
Volume 9 - 2022 | https://doi.org/10.3389/fcvm.2022.957897
This article is part of the Research Topic Implications of Lipids and Modified Lipoproteins in Atherogenesis: From mechanisms towards novel diagnostic and therapeutic targets View all 16 articles
Atherosclerosis is a major cause of acute coronary syndrome and stroke. Foam cell formation in macrophages is involved in controlling plaque stability and the pathogenesis of atherosclerosis. Accordingly, many studies have examined the processes of lipid incorporation, such as scavenger receptor-mediated uptake of oxidized low-density lipoprotein, in cells. In addition to receptor-mediated machinery, growing evidence has suggested that pinocytosis, which is a receptor-independent endocytic pathway, is associated with foam cell formation when a sufficient number of lipoproteins is accumulated around cells. Pinocytotic engulfment of nanoparticles is initiated by plasma membrane ruffling in a phosphatidylinositol-3 kinase-dependent manner. Subsequent to pinosome closure, the majority of pinosomes are internalized through endocytic processes, and they can be recycled into the plasma membrane. These pinocytotic processes are modulated by small GTPases and their cytoskeletal rearrangement. Moreover, pinocytotic abilities may vary between immunological subsets in cells. Accordingly, macrophages may show diverse pinocytotic abilities depending on the surrounding microenvironment. This review summarizes the current understanding of pinocytotic engulfment of lipoprotein in macrophages, and discusses how this endocytic process is governed under hypercholesterolemic conditions.
Ischemic heart disease is the leading cause of death globally. Atherosclerosis is a major risk factor for coronary disease because expansion of such eccentric thickening of the arterial wall can lead to thromboembolism and thrombotic occlusion. Numerous basic and epidemiological studies have shown that an abnormality of cholesterol handling, excessive adaptive response to vascular insults, and inflammation burden are associated with the pathogenesis of atherosclerosis (1, 2). Among these events, cholesterol accumulation in macrophages is an essential process to expand atherosclerotic plaques and is a major factor for defining plaque stability (3, 4). Several endocytic pathways for internalizing low-density lipoprotein (LDL) cholesterol have been reported. Scavenger receptors, such as scavenger receptor-A, CD36, and lectin-like oxidized LDL receptor 1 (5, 6), in macrophages recognize oxidized LDL, but not native LDL, to internalize LDL through the conventional endocytic process. In addition to the receptor-mediated pathways, macrophages enable the incorporation of nanoparticles through pinocytosis. Pinocytosis is a fundamental cellular process that engulfs extracellular fluids. Pinocytosis is categorized into micropinocytosis and macropinocytosis, which are endocytic processes that engulf small particles (typically < 0.1 μm) and large particles (typically > 0.2 μm), respectively (7). Such pinocytotic engulfment in macrophages, which occurs spontaneously and can be further modified by environmental factors, are related to the innate immune system to monitor surrounding antigens and microbial-associated molecules. Growing evidence has suggested that pinocytosis is mediated through incorporation of native LDL, thereby inducing formation of foamy macrophages (8). This mini review summarizes the recent understanding of internalization and trafficking of pinosomes and their regulatory mechanisms.
The pinocytotic deposition of native LDL is sufficient to convert cultured macrophages into foam cells (9). Pinocytotic uptake is independent of the degree of LDL oxidation and does not saturate (7), and the molecular mechanisms underlying pinocytotic LDL uptake are distinct from those of receptor-mediated pathways. Indeed, targeted deletion of the macrophage scavenger receptors scavenger receptor-A and CD36 does not inhibit pinocytotic LDL uptake in macrophage-colony stimulating factor (M-CSF)-differentiated macrophages (10). Generally, macropinocytotic internalization of nanoparticles is divided into plasma membrane ruffling and pinosome closure, which are regulated by submembranous actin organization. Small GTPases have central roles in this cytoskeletal regulation. Ras-GTP drives the activation of phosphoinositide 3-kinases (PI3Ks), which generates patches of PtdIns(3,4,5)P3 (11). The Rho family GTPase Rac and actin-nucleation-promoting factors, such as SCAR/WAVE complex, enable binding to these patches, which nucleates actin filaments. In addition to actin-regulating factors, phospholipase Cγ can be activated by PtdIns(3,4,5)P3, thereby generating diacylglycerol and subsequent activation of protein kinase C, which is involved in the positive regulation of pinocytosis (12). Kruth and colleagues investigated mechanisms underlying pinocytosis using pharmacological approaches in M-CSF-differentiated macrophages, and found that pinocytosis was inhibited by a broad-range PI3K inhibitors (10). In contrast, inhibition of the class I PI3K isoforms β, γ, or δ did not affect micropinocytosis in M-CSF-stimulated macrophages. Similarly, macrophages from mice expressing dominant-negative class I PI3K β, γ, or δ isoforms had no inhibitory effects. Therefore, PI3Ks, excluding class I isoforms, drive macropinocytosis in macrophages. Pharmacological screening of signaling pathways has shown that dynamin, microtubules, actin, and vacuolar type H(+)-ATPase appear to be associated with pinocytotic uptake (10). Accordingly, phosphoinositide metabolism accompanied by cytoskeletal regulation are indispensable even for pinocytotic LDL uptake in macrophages (Figure 1).
Figure 1. Overview of pinocytotic deposition of low-density lipoprotein cholesterol in macrophages. Phosphoinositide 3-kinase drives pinocytotic plasma membrane ruffling. Small GTPases are associated with trafficking and recycling of pinosomes, as well as with pinocytotic plasma membrane regulation, and have diverse actions in pinocytotic low-density lipoprotein cholesterol uptake in macrophages. While pinocytosis appears to be optimized in an alternative (M2) macrophage subset, certain elements, such as Toll-like receptor 4 (TLR4)-mediated signaling, enable the restoration of pinocytosis, even in an inflammatory (M1) subset. TLR4 driver lipopolysaccharide (LPS) can polarize macrophage differentiation toward M1 subset, and activates pinocytotic activation through unknown mechanisms. Chemokine (C-C motif) ligand 19 reportedly possesses similar pinocytotic effects in the cells. In the case of mmLDL-differentiated macrophages, cytoskeletal rearrangement appears to be driven through TLR4/Ras/Raf/ERK/MEK axis independently of PI3K signaling. Furthermore, pro-inflammatory cytokine TNF-α upregulates calpain-6, a non-proteolytic isoform of calpain protease family, to inhibit CWC22-mediated Rac1 splicing. This interferes with endosomal recycling pathway, and in turn increases lysosomal processing of endosome-derived lipoprotein cholesterol to generate cytosolic lipid droplets. In contrast to M1 subset, macrophage-colony stimulating factor (M-CSF)-differentiated M2 macrophages exhibits phosphatidylinositol 3-kinase (PI3K)-dependent cytoskeletal rearrangement and membrane ruffling. Similarly, thrombospndin-1 enables to elicit CD47-mediated activation of PI3K and subsequent pinocytotic uptake of native LDL. In this case, it is likely that Akt3 negatively regulates Cdc42 and acyl-CoA cholesterol acyltransferase 1 to decelerate pinocytotic cholesterol deposition in the cells. ACAT1, acyl-CoA cholesterol acyltransferase 1; CCL19, Chemokine (C-C motif) ligand 19; EE, early endosome; LE, late endosome; LPS, lipopolysaccharide; M-CSF, macrophage-colony stimulating factor; mmLDL, minimally modified low density lipoprotein; PI3K, phosphatidylinositol 3-kinase; PIP3, phosphatidyl inositol 3-phosphate; Rac1, Rac family small GTPase 1; RE, recycling endosome; TLR4, Toll-like receptor 4; TNF-α, tumor necrosis factor-α; TSP-1, thrombospndin-1.
In addition to the molecules noted above, the contribution of Rho GTPases to pinocytotic regulation has been well–documented. Anzinger et al. reported that the Rho GTPase inhibitor toxin B substantially inhibited pinocytotic LDL uptake in M-CSF-differentiated human macrophages (13). Using time-lapse microscopy, they found that this inhibitor almost completely inhibited macropinocytosis, although cholesterol deposition in cells was not completely inhibited. Their findings suggest the contribution of another endocytic process, such as micropinocytosis, to LDL cholesterol uptake. In contrast, pharmacological inhibition of Rac1 failed to inhibit pinocytotic LDL uptake in M-CSF-differentiated macrophages (10). Our previous study showed that expression of the Rho GTPases RhoA and Rac1 in bone marrow cells was imperceptible, while it was dramatically induced during the differentiation of cells into macrophages in the presence of M-CSF (14). Treatment of bone marrow cells with tumor necrosis factor (TNF)-α suppressed maturation of Rac1 mRNA and potentiated macropinocytosis. Deficiency of calpain-6, which is a non-proteolytic isoform of the calpain protease family, in TNF-α-stimulated murine macrophages showed interrupted macropinocytosis concomitantly with the normalization of Rac1 splicing. Macropinocytosis in calpain-6-deficient macrophages was restored by small interfering RNA-based silencing of Rac1. Therefore, aberrant Rac1 mRNA regulation exerts inhibitory actions in pinocytotic LDL uptake in TNF-α-stimulated macrophages. While the mechanisms by which Rac1 or its splice variants interrupt LDL uptake are unclear, Rac1-mediated regulation of the endosomal recycling pathway may contribute to the inhibitory actions. Indeed, pinosomes in Rac1-expressing macrophages frequently express the recycling endosome marker Rab11. Moreover, macropinosome velocity in cells is decelerated by pharmacological inhibition of Rac1. Therefore, pinocytotic LDL uptake is likely to be due to Rac1-dependent vesicle trafficking rather than Rac1-dependent membrane ruffling. Rho GTPases, such as RhoD, Rac1, Cdc42, TCL, and TC10, are thought to be essential factors for endocytic trafficking (15). Indeed, overexpression of dominant active forms of Rac1 accelerate macropinocytosis activity in rat fibroblasts (16). However, notably, prolonged Rac1 activity impairs the maturation of Rab21-positive pinosomes in macrophages (17), suggesting that optimal Rac1 regulation can maximize pinocytosis. However, Ding et al. showed positive regulation of pinocytotic LDL uptake by Cdc42, which is a small GTPase, in human and murine macrophages (18). Indeed, the loss of Akt3 in murine and human M-CSF-differentiated macrophages upregulates with-no-lysine kinase 1 and subsequently activates serum and glucocorticoid-inducible kinase 1. Serum and glucocorticoid-inducible kinase 1 promotes expression of the Rho family GTPase Cdc42, thereby accelerating cytoskeletal rearrangement and pinocytosis. Similarly, Akt3-dependent negative regulation of pinocytosis is detectable in murine peritoneal macrophages. This regulation is accompanied by limited receptor-dependent uptake of acetylated LDL and downregulation of acyl-CoA cholesterol acyltransferase (19). Acyl-CoA cholesterol acyltransferase converts free cholesterol into cholesterol ester to form cytosolic lipid droplets in peritoneal macropahges (20). Therefore, Akt3 has a protective role in foam cell formation in macrophages and atherogenesis. How Akt3 simultaneously downregulates receptor-dependent and receptor-independent pathways is currently unclear, but Akt3 might interfere with a common signaling pathway, such as endocytic vesicle trafficking or the exocytotic process. This possibility is consistent with a previous study, which showed that overexpression of dominant-negative Cdc42 counteracted macropinocytosis in vascular endothelial cells (21). Collectively, small GTPases are associated with vesicle trafficking and pinocytotic plasma membrane regulation (Figure 1), and have diverse actions regarding pinocytotic LDL uptake in macrophages. Therefore, defining pinocytotic activity by expression levels of small GTPases alone is difficult.
Macrophages can be divided into a variety of subpopulations, which comprise pro-inflammatory, immunosuppressive, and tissue-repairing types, and they are dynamically interconverted depending on the tissue microenvironment (22). During bacterial infection, monocyte-macrophages can be activated by inflammatory elements, such as Toll-like receptor (TLR) ligands and interferon-γ, which facilitate the skewing of macrophages into the M1 subset (classically activated macrophages). M1 macrophages produce inflammatory cytokines, such as TNF-α, interleukin (IL)-6, and IL-12, reactive oxygen species, and reactive nitrogen species, and induce a Th1-type immune response (23). Furthermore, M1 macrophages exert strong antibacterial or antiviral activity and antitumor effects. In contrast to the M1 subset, macrophages activated by IL-4 and IL-13 produced by Th2 cells, basophils, mast cells, and innate lymphoid cells are converted into M2 macrophages (alternatively activated macrophages) (23). This activation exerts host defense against parasites, tissue repair, angiogenesis, and tumor growth, and becomes immunosuppressive. M2 macrophages strongly express arginase and mannose receptors (24). Tumor-associated macrophages that are infiltrated into tumor tissue are thought to be converted from M1 to M2 subsets, thereby accelerating tumor progression (25). Tumor-associated macrophages showing low IL-12 expression levels and high IL-10 expression levels, possess weak antitumor activity, and drive matrix remodeling and angiogenesis (26). In addition to the progression of cancer, aberrant regulation of macrophage subsets can be involved in various diseases. Therefore, phenotypic regulation of macrophage subsets can be regarded as a therapeutic target. Arteriosclerosis, which is a Th1-dominant vascular disorder, can be treated by skewing M1 to M2 macrophages (27). In contrast, bronchial asthma, which is a Th2-dominant disease, might be targeted by the opposite strategy (28).
M2 macrophages show constitutive pinocytotic activity and can be further stimulated with related cytokines such as M-CSF. Notably, the majority of the above-mentioned observations on pinocytotic LDL uptake were from investigations using M-CSF-differentiated human monocyte-derived macrophages. Redka et al. showed that M-CSF/IL-4-differentiated M2 macrophages had robust micropinocytosis activity, while that in granulocyte M-CSF/interferon-γ/lipopolysaccharide-differentiated M1 macrophages was negligible (29). They found that Rho GTPase expression levels in M1 macrophages were lower than those in the M2 subset. In addition to small GTPases, insufficient PI3K activity is likely responsible for modest pinocytotic ability in the M1 subset (29). Calcium-sensing receptors appear to be necessary for sustaining small GTPases and PI3K in M2 macrophages. However, notably, M1 macrophages show robust pinocytotic activity when the cells are stimulated with certain pro-inflammatory substances. Indeed, acute treatment of pro-inflammatory macrophages with lipopolysaccharide or CC chemokine ligand 19 markedly accelerates macropinosome formation in human monocyte-derived macrophages (29). Moreover, minimally oxidized low-density lipoprotein (mmLDL), which is an alternative TLR4 ligand, and cholesteryl ester hydroperoxide, an active component of mmLDL, induce TLR4-dependent macropinocytotic incorporation of oxidized LDL and native LDL (30). In this case, mmLDL elicits an association of spleen tyrosine kinase with a TLR4 complex, TLR4 phosphorylation, activation of the Vav1-Ras-Raf-MEK-ERK1/2 axis, phosphorylation of paxillin, and activation of Rac, Cdc42, and Rho in murine peritoneal macrophages. These findings suggest that mmLDL-induced TLR4 signaling normalizes the disparity of small GTPases. Collectively, the status of small GTPases is likely to depend on types of extracellular stimuli rather than synchronizing with the phenotypic status of M1/M2 subsets. Accordingly, the master regulator of pinocytosis is currently unclear.
While the regulatory mechanisms underlying pinocytosis are poorly understood, several studies have focused on the upstream modulator of this process (Figure 1). Agonists of liver X receptors (LXRs) are involved in the downregulation of pinocytotic uptake of native LDL in M-CSF-differentiated macrophages (31). LXRs are ligand-activated transcription factors involved in the control of lipid metabolism and inflammation. Because targeting LXRs in bone marrow cells facilitates atherosclerosis (32), LXRs may interfere with pinocytotic cholesterol deposition in macrophages and subsequent pathogenesis of atherosclerosis. Csányi et al. found that thrombospndin-1 (TSP-1) and its cytoskeletal regulation potentiated pinocytosis (33). Indeed, treatment of TSP-1 with human and murine M-CSF-differentiated macrophages stimulated membrane ruffle formation and pericellular solute internalization by macropinocytosis. The TSP1 cognate receptor CD47, NADPH oxidase 1 (Nox1) signaling, PI3K, and myotubularin-related protein 6 appear to be associated with TSP1-induced macropinocytosis. Our previous study showed that CWC22, which is an essential loading factor of exon junction complex, was associated with pinocytotic incorporation of native LDL in murine bone marrow-derived macrophages (14). CWC22 in macrophages shows nuclear localization in human mild atherosclerotic lesions, while it shows cytosolic localization in advanced atherosclerotic lesions. Macrophages in advanced lesions simultaneously express calpain-6, which potentiates formation of a calpain-6/CWC22 complex in the cytoplasm and inhibits nuclear localization of CWC22. Because CWC22 has a direct role in Rac1 mRNA splicing, calpain-6 counteracts CWC22-mediated maturation of Rac1 mRNA. Indeed, calpain-6-deficient macrophages show low Rac1 protein expression levels and insufficient macropinocytosis activity. Knockdown of Cwc22 in calpain-6-deficient macrophages leads to the recovery macropinocytosis, concomitantly with restoring Rac1 mRNA maturation. As noted above, Rac1 facilitates the dynamics of recycling endosomes. Therefore, CWC22 and related splicing factors are thought to be negative regulators of the pinocytotic process.
While fluid phase pinocytosis is a constitutive process in macrophages and other cells under the physiological conditions, this process is reportedly potentiated in former cell type when they are localized in atherosclerotic lesions. In vivo experiments using Apoe−/− hypercholesterolemic mice have indicated that fluorescent nanobeads, which is similar in size to LDL and were injected into blood circulation, were massively accumulated in CD68-positive macrophages in atherosclerotic lesions, but not in non-atheroma regions in arteries (34). We also investigated the uptake of nanobeads using Ldlr−/− hypercholesterolemic mice. As a result, similar deposition of nanoparticle was reproduced in foamy macrophages in atherosclerotic lesions (14). These observations suggest that macrophages in atherosclerotic lesions preferentially engulf environmental LDL-like particles under hypercholesterolemia. Interestingly, targeting calpain-6 suppressed nanobeads incorporation in foamy macrophages as well as atherogenesis in hypercholesterolemic mice without altering plasma lipid profiles. Notably, overexpression of calpain-6 can upregulate pinocytotic incorporation of LDL in bone marrow-derived macrophages without modifying receptor-mediated uptake of oxidative LDL and phagocytic uptake of aggregated LDL. Considering that calpain-6 is exclusively expressed in macrophages in atherosclerotic lesions, it is interpreted that the pinocytotic incorporation of LDL in lesional macrophages, at least of their calpain-6-mediated portion, may be responsible for the pathogenesis of atherosclerosis. Nevertheless, since calpain-6 contribute to the other atherogenic processes in macrophages such as cellular motility (14), the pathophysiological importance of this endocytic process in the pathogenesis of atherosclerosis has not been fully determined.
As noted above, the contribution of pinocytosis to the pathogenesis of atherosclerosis is currently sketchy. This is because of the lack of responsible regulatory element(s) of this processes. Cell-based experiments suggest the dominant roles of small GTPases in pinocytotic membrane ruffling, while they also contribute to the other fundamental processes of cells, including mitosis and cell motility. Identification of the pinocytotic master regulator enables to perform intervention study in animal models to determine the pathogenic significance of this processes. It was reported that targeted deletion of scavenger receptors, CD36, and scavenger receptor-A in hypercholesterolemic mice inhibits the pathogenesis of atherosclerosis without altering oxidized LDL uptake in macrophages (35). Therefore, pinocytotic uptake of LDL in the cells is worthy to be further investigated. To identify the master regulator of pinocytotic LDL uptake, comprehensive studies evaluating membrane regulation, vesicle trafficking, exocytosis/efflux, and subsequent cholesterol deposition are necessary.
TM conceived and designed the review, appraised the literature, and wrote the manuscript.
This study was supported in part by the Japan Society for the Promotion of Science KAKENHI grants [grant nos.: JP19K08590 and JP22H03520 (to TM)], a research grant from Bristol-Myers Squibb (to TM), a research grant from the Mochida Memorial Foundation for Medical and Pharmaceutical Research (to TM), and a research grant from the Naito Memorial Foundation (to TM).
We thank Ellen Knapp, PhD, from Edanz (https://jp.edanz.com/ac) for editing a draft of this manuscript.
The author declares that the research was conducted in the absence of any commercial or financial relationships that could be construed as a potential conflict of interest.
All claims expressed in this article are solely those of the authors and do not necessarily represent those of their affiliated organizations, or those of the publisher, the editors and the reviewers. Any product that may be evaluated in this article, or claim that may be made by its manufacturer, is not guaranteed or endorsed by the publisher.
1. Miyazaki T, Miyazaki A. Dysregulation of calpain proteolytic systems underlies degenerative vascular disorders. J Atheroscler Thromb. (2018) 25:1–15. doi: 10.5551/jat.RV17008
2. Ross R. Atherosclerosis–an inflammatory disease. N Engl J Med. (1999) 340:115–26. doi: 10.1056/NEJM199901143400207
3. Miyazaki T, Miyazaki A. Impact of dysfunctional protein catabolism on macrophage cholesterol handling. Curr Med Chem. (2019) 26:1631–43. doi: 10.2174/0929867325666180326165234
4. Miyazaki T, Miyazaki A. Emerging roles of calpain proteolytic systems in macrophage cholesterol handling. Cell Mol Life Sci. (2017) 74:3011–21. doi: 10.1007/s00018-017-2528-7
5. Tian K, Ogura S, Little PJ, Xu SA-O, Sawamura T. Targeting LOX-1 in atherosclerosis and vasculopathy: current knowledge and future perspectives. Ann N Y Acad Sci. (2019) 1443:34–53. doi: 10.1111/nyas.13984
6. Chistiakov DA, Bobryshev YV, Orekhov AN. Macrophage-mediated cholesterol handling in atherosclerosis. J Cell Mol Med. (2016) 20:17–28. doi: 10.1111/jcmm.12689
7. Kruth HS. Fluid-phase pinocytosis of LDL by macrophages: a novel target to reduce macrophage cholesterol accumulation in atherosclerotic lesions. Curr Pharm Des. (2013) 19:5865–72. doi: 10.2174/1381612811319330005
8. Kruth HS. Receptor-independent fluid-phase pinocytosis mechanisms for induction of foam cell formation with native low-density lipoprotein particles. Curr Opin Lipidol. (2011) 22:386–93. doi: 10.1097/MOL.0b013e32834adadb
9. Kruth HS, Jones NL, Huang W, Zhao B, Ishii I, Chang J, et al. Macropinocytosis is the endocytic pathway that mediates macrophage foam cell formation with native low density lipoprotein. J Biol Chem. (2005) 280:2352–60. doi: 10.1074/jbc.M407167200
10. Barthwal MJ, Anzinger JJ, Xu Q, Bohnacker T, Wymann MP, Kruth HS. Fluid-phase pinocytosis of native low density lipoprotein promotes murine M-CSF differentiated macrophage foam cell formation. PLoS ONE. (2013) 8:e58054. doi: 10.1371/journal.pone.0058054
11. Hoeller O, Bolourani P, Clark J, Stephens LR, Hawkins PT, Weiner OD, et al. Two distinct functions for PI3-kinases in macropinocytosis. J Cell Sci. (2013) 126:4296–307. doi: 10.1242/jcs.134015
12. Singla B, Ghoshal P, Lin H, Wei Q, Dong Z, Csányi G. PKCδ-Mediated Nox2 activation promotes fluid-phase pinocytosis of antigens by immature dendritic cells. Front Immunol. (2018) 9:537. doi: 10.3389/fimmu.2018.00537
13. Anzinger JJ, Chang J, Xu Q, Buono C, Li Y, Leyva FJ, et al. Native low-density lipoprotein uptake by macrophage colony-stimulating factor-differentiated human macrophages is mediated by macropinocytosis and micropinocytosis. Arterioscler Thromb Vasc Biol. (2010) 30:2022–31. doi: 10.1161/ATVBAHA.110.210849
14. Miyazaki T, Tonami K, Hata S, Aiuchi T, Ohnishi K, Lei XF, et al. Calpain-6 confers atherogenicity to macrophages by dysregulating pre-mRNA splicing. J Clin Invest. (2016) 126:3417–32. doi: 10.1172/JCI85880
15. Phuyal S, Farhan H. Multifaceted Rho GTPase Signaling at the Endomembranes. Front Cell Dev Biol. (2019) 7:127. doi: 10.3389/fcell.2019.00127
16. Ahram M, Sameni M, Qiu RG, Linebaugh B, Kirn D, Sloane BF. Rac1-induced endocytosis is associated with intracellular proteolysis during migration through a three-dimensional matrix. Exp Cell Res. (2000) 260:292–303. doi: 10.1006/excr.2000.5031
17. Fujii M, Kawai K, Egami Y, Araki N. Dissecting the roles of Rac1 activation and deactivation in macropinocytosis using microscopic photo-manipulation. Sci Rep. (2013) 3:2385. doi: 10.1038/srep02385
18. Ding L, Zhang L, Kim M, Byzova T, Podrez E. Akt3 kinase suppresses pinocytosis of low-density lipoprotein by macrophages via a novel WNK/SGK1/Cdc42 protein pathway. J Biol Chem. (2017) 292:9283–93. doi: 10.1074/jbc.M116.773739
19. Ding L, Biswas S, Morton RE, Smith JD, Hay N, Byzova TV, et al. Akt3 deficiency in macrophages promotes foam cell formation and atherosclerosis in mice. Cell Metab. (2012) 15:861–71. doi: 10.1016/j.cmet.2012.04.020
20. Huang L-H, Melton EM, Li H, Sohn P, Rogers MA, Mulligan-Kehoe MJ, et al. Myeloid Acyl-CoA:cholesterol acyltransferase 1 deficiency reduces lesion macrophage content and suppresses atherosclerosis progression. J Biol Chem. (2016) 291:6232–44. doi: 10.1074/jbc.M116.713818
21. Tkachenko E, Lutgens E, Stan RV, Simons M. Fibroblast growth factor 2 endocytosis in endothelial cells proceed via syndecan-4-dependent activation of Rac1 and a Cdc42-dependent macropinocytic pathway. J Cell Sci. (2004) 117:3189–99. doi: 10.1242/jcs.01190
22. Murray PJ, Allen JE, Biswas SK, Fisher EA, Gilroy DW, Goerdt S, et al. Macrophage activation and polarization: nomenclature and experimental guidelines. Immunity. (2014) 41:14–20. doi: 10.1016/j.immuni.2014.06.008
23. Shapouri-Moghaddam A, Mohammadian S, Vazini H, Taghadosi M, Esmaeili SA, Mardani F, et al. Macrophage plasticity, polarization, and function in health and disease. J Cell Physiol. (2018) 233:6425–40. doi: 10.1002/jcp.26429
24. Gensel JC, Zhang B. Macrophage activation and its role in repair and pathology after spinal cord injury. Brain Res. (2015) 1619:1–11. doi: 10.1016/j.brainres.2014.12.045
25. Miyazaki T, Akasu R, Miyazaki A. Calpain-associated proteolytic regulation of the stromal microenvironment in cancer. Curr Pharm Des. (2021) 27:3128–38. doi: 10.2174/1381612827666210311143053
26. Yamaguchi T, Fushida S, Yamamoto Y, Tsukada T, Kinoshita J, Oyama K, et al. Tumor-associated macrophages of the M2 phenotype contribute to progression in gastric cancer with peritoneal dissemination. Gastric Cancer. (2016) 19:1052–65. doi: 10.1007/s10120-015-0579-8
27. de Gaetano M, Crean D, Barry M, Belton O. M1- and M2-type macrophage responses are predictive of adverse outcomes in human atherosclerosis. Front Immunol. (2016) 7:275. doi: 10.3389/fimmu.2016.00275
28. Ogulur I, Pat Y, Ardicli O, Barletta E, Cevhertas L, Fernandez-Santamaria R, et al. Advances and highlights in biomarkers of allergic diseases. Allergy. (2021) 76:3659–86. doi: 10.1111/all.15089
29. Redka DS, Gütschow M, Grinstein S, Canton J. Differential ability of proinflammatory and anti-inflammatory macrophages to perform macropinocytosis. Mol Biol Cell. (2018) 29:53–65. doi: 10.1091/mbc.E17-06-0419
30. Choi S-H, Harkewicz R, Lee JH, Boullier A, Almazan F, Li AC, et al. Lipoprotein accumulation in macrophages via toll-like receptor-4-dependent fluid phase uptake. Circ Res. (2009) 104:1355–63. doi: 10.1161/CIRCRESAHA.108.192880
31. Buono C, Li Y, Waldo SW, Kruth HS. Liver X receptors inhibit human monocyte-derived macrophage foam cell formation by inhibiting fluid-phase pinocytosis of LDL. J Lipid Res. (2007) 48:2411–8. doi: 10.1194/jlr.M700170-JLR200
32. Tangirala RK, Bischoff ED, Joseph SB, Wagner BL, Walczak R, Laffitte BA, et al. Identification of macrophage liver X receptors as inhibitors of atherosclerosis. Proc Natl Acad Sci U S A. (2002) 99:11896–901. doi: 10.1073/pnas.182199799
33. Csányi G, Feck DM, Ghoshal P, Singla B, Lin H, Nagarajan S, et al. CD47 and Nox1 mediate dynamic fluid-phase macropinocytosis of native LDL. Antioxid Redox Signal. (2017) 26:886–901. doi: 10.1089/ars.2016.6834
34. Buono C, Anzinger JJ, Amar M, Kruth HS. Fluorescent pegylated nanoparticles demonstrate fluid-phase pinocytosis by macrophages in mouse atherosclerotic lesions. J Clin Invest. (2009) 119:1373–81. doi: 10.1172/JCI35548
35. Manning-Tobin JJ, Moore KJ, Seimon TA, Bell SA, Sharuk M, Alvarez-Leite JI, et al. Loss of SR-A and CD36 activity reduces atherosclerotic lesion complexity without abrogating foam cell formation in hyperlipidemic mice. Arterioscler Thromb Vasc Biol. (2009) 29:19–26. doi: 10.1161/ATVBAHA.108.176644
Keywords: CWC22, calpain-6, Rac1, Cdc42, Akt, liver X receptors, macrophage-colony stimulating factor
Citation: Miyazaki T (2022) Pinocytotic engulfment of lipoproteins by macrophages. Front. Cardiovasc. Med. 9:957897. doi: 10.3389/fcvm.2022.957897
Received: 31 May 2022; Accepted: 11 August 2022;
Published: 29 August 2022.
Edited by:
Tatsuya Sawamura, Shinshu University, JapanReviewed by:
Masayuki Yoshida, Tokyo Medical and Dental University, JapanCopyright © 2022 Miyazaki. This is an open-access article distributed under the terms of the Creative Commons Attribution License (CC BY). The use, distribution or reproduction in other forums is permitted, provided the original author(s) and the copyright owner(s) are credited and that the original publication in this journal is cited, in accordance with accepted academic practice. No use, distribution or reproduction is permitted which does not comply with these terms.
*Correspondence: Takuro Miyazaki, dGFrdUBwaGFybS5zaG93YS11LmFjLmpw
Disclaimer: All claims expressed in this article are solely those of the authors and do not necessarily represent those of their affiliated organizations, or those of the publisher, the editors and the reviewers. Any product that may be evaluated in this article or claim that may be made by its manufacturer is not guaranteed or endorsed by the publisher.
Research integrity at Frontiers
Learn more about the work of our research integrity team to safeguard the quality of each article we publish.