- Department of Cardiology, The Affiliated Hospital of Southwest Jiaotong University, The Third People’s Hospital of Chengdu, Chengdu, Sichuan, China
Background: The relationship between abnormal lipid levels and atherosclerotic cardiovascular diseases is well established, but the association between remnant cholesterol (RC) and coronary heart disease (CHD) remains uncertain. The aim of this meta-analysis is to systematically evaluate the prognostic value of RC concentration in patients with CHD.
Methods: PubMed, EMBASE, Cochrane, and Web of Science databases were reviewed to identify relevant observational cohort studies published in English up to December 2021. Random-effects meta-analysis compared the highest and lowest RC concentration. The primary outcome was a composite of major adverse cardiovascular events (MACEs) and all-cause mortality in patients with CHD.
Results: A total of 10 studies recruiting 30,605 patients with CHD were selected to be included in this meta-analysis. Patients with CHD with elevated RC concentration had an increased risk of the composite endpoint events (RR = 1.54, 95% CI: 1.26–1.87) and MACEs (RR = 1.70, 95% CI: 1.54–1.88), but the risk of all-cause mortality was not statistically significant (RR = 1.16, 95% CI: 0.79–1.69, P = 0.44). Subgroup analysis showed consistent results.
Conclusion: Our results suggest that elevated concentration RC may independently predict MACEs in patients with CHD. Determination of RC concentration may improve risk stratification of prognosis in patients with CHD. However, more high-quality studies are necessary to confirm this association.
Introduction
Coronary heart disease (CHD) is the most common type of organ disease caused by atherosclerosis, which is seriously threatening people’s life and health (1, 2). The prevalence of CHD in the world is approximately 4.6–9.2%, and in 2019, the disease caused 9.14 million deaths worldwide (1). Dyslipidemia is one of the important risk factors for CHD. Currently, lowering low-density lipoprotein cholesterol (LDL-C) is one of the main intervention targets in the treatment of CHD. However, previous studies found that even after reducing LDL-C to an appropriate level and controlling other risk factors, there may be significant differences in the prognosis of patients with CHD (3). Therefore, it is important to find more reliable prognostic indicators to evaluate the long-term prognosis of CHD and formulate the best treatment plan.
In recent years, remnant cholesterol (RC) has been reported to have a critical role in atherosclerosis and CHD, which might indicate it may also be a critical component of the residual risk of patients with CHD (3, 4). RC is the cholesterol content of all non-low-density lipoprotein (LDL) and non-high-density lipoprotein (HDL). Compared with LDL-C, RC had a stronger atherogenic ability because it possesses a larger quantity and volume, carries more cholesterol, and does not need oxidative modification (5, 6). Some observational cohort studies have linked high RC concentrations with an increased risk of CHD (7, 8). Furthermore, RC was found to be causally associated with CHD development in previously healthy individuals (9). However, the prognostic value of plasma RC levels in secondary prevention settings is still undefined because previous studies showed inconsistent and controversial results (10–12). Meanwhile, it is yet to be established whether the prognostic value of RC varies among populations, ages, or the classification of CHDs. In particular, there is still a lack of a standardized method for RC measurement, with strikingly different RC concentrations across studies (10, 12, 13), which may also have contributed to the discrepancy in the outcomes (14–16).
Therefore, our study aims to systematically review and compile meta-analyses of the evidence on the relationship between RC concentration and CHD outcome, by identifying the potential confounders and investigating the prognostic value, which may provide a novel perspective for risk assessment and treatment in patients with CHD.
Materials and methods
Our meta-analysis was performed according to the recommendation of the meta-analysis of Observational Studies in Epidemiology (MOOSE) (17) and the Preferred Reporting Items for Systematic Reviews and Meta-Analyses (PRISMA) statement (18).
Search strategy
We comprehensively searched four medical databases, including PubMed, EMBASE, Cochrane, and Web of Science, to identify cohort studies assessing the relationship between RC concentration and cardiovascular outcomes published in English from inception up to 25 December 2021. The query syntax was set using Medical Subject Headings (MeSH) and thesaurus search terms, including (“remnant cholesterol” OR “remnant-like particle cholesterol” OR “triglyceride-rich lipoprotein cholesterol”) AND (“coronary heart disease” OR “coronary disease” OR “coronary artery disease”). The detailed search strategy was presented in the Supplementary material. References retrieved from the studies, as well as relevant reports, were also hand-searched to reduce the likelihood of missing any publications.
Inclusion and exclusion criteria
The inclusion criteria for this study were presented as follows: (1) cohort studies; (2) an inception cohort involving adults with CHD; CHD included stable or unstable angina, and myocardial infarction (MI); (3) the exposure factor was RC concentration; (4) the endpoint was major adverse cardiovascular events (MACEs) and all-cause mortality. MACEs included cardiac death, MI, ischemic stroke, myocardial ischemia, heart failure, unstable angina requiring readmission, and coronary revascularization; and (5) the highest and lowest RC concentration groups of multivariate-adjusted relative risks (RRs), odds ratios (ORs), or hazard ratios (HRs) and their 95% confidence intervals (95% CI) or the above indicators could be calculated with the complete data (19).
Exclusion criteria included (1) case reports, commentary, and conference abstracts; (2) animal, cross-sectional studies, or randomized clinical trials; (3) studies carried out among pregnant women or children; and (4) examined non-relevant outcomes.
YT did the screening of the titles and abstracts of the identified articles, and pertinent articles were independently reviewed in full text by two investigators (YT and WW). Thus, disagreement was resolved through consensus.
Data collection and quality assessment
Data extraction was in a standardized style. Two investigators (YT and LQ) independently extracted the following data: the first author, publication year, population, study design, type of CHD, sample size, percentage of women, age, exposure assessment method, fasting status, follow-up duration, outcome assessment, categorical or continuous, adjusted risk estimates, and adjustment for variables.
The quality of observational cohort studies was assessed using the Newcastle–Ottawa Scale (NOS) (20), which was ranked as poor (score 1–3), fair (score 4–6), or good (score 7–9) according to the quality of study participant selection, comparability, and outcome. Studies with NOS ≥7 points were considered high quality. Any disagreements were discussed and resolved by a chief investigator (HW), and a consensus was reached in all cases.
Statistical analysis
Review Manager 5.4 software (The Cochrane Collaboration, Oxford, UK) and Stata 16.0 (Stata Corporation, College Station, TX, USA) were employed for statistical analysis. The I2 statistics and chi-square Cochran’s Q-test were used to assess the heterogeneity across studies. If P ≥ 0.05 and I2 < 50%, suggesting that no significant heterogeneity could be found, a fixed-effect model was also applied. In addition, if P < 0.05 and I2 ≥ 50%, a random-effect model was cautiously applied, and then subgroup analysis was used to explore the source of heterogeneity (21). The elimination of individual studies one by one was also performed for sensitivity analysis in order to explore the heterogeneity and assess the stability of the meta-analysis. A funnel plot combined with Egger’s test was employed to investigate the potential publication bias of the involved studies. Finally, the RR with 95% CI was employed for the effect estimation metric, and HR and OR were converted into RR (22–24). The p-value of < 0.05 meant the difference was statistically significant.
Results
Literature search results
A total of 2,308 articles were retrieved, and after 381 duplicates were removed, 1,927 unique records remained. After screening the titles and abstracts of the articles, 38 records were considered for a detailed full-text screening. Of these studies, 28 articles were excluded: studies with a non-CHD population (n = 11) (16, 25–34), those with no interest outcome (n = 5) (35–39), those with potential patients’ duplication with other articles (n = 5) (8, 9, 40–42), and studies with ineligible study design (n = 7) (43–49). Finally, 10 articles (4, 10–13, 50–54) covering 12 cohorts enrolling 30,605 subjects met the selection criteria. Figure 1 depicts the literature screening process and results in detail.
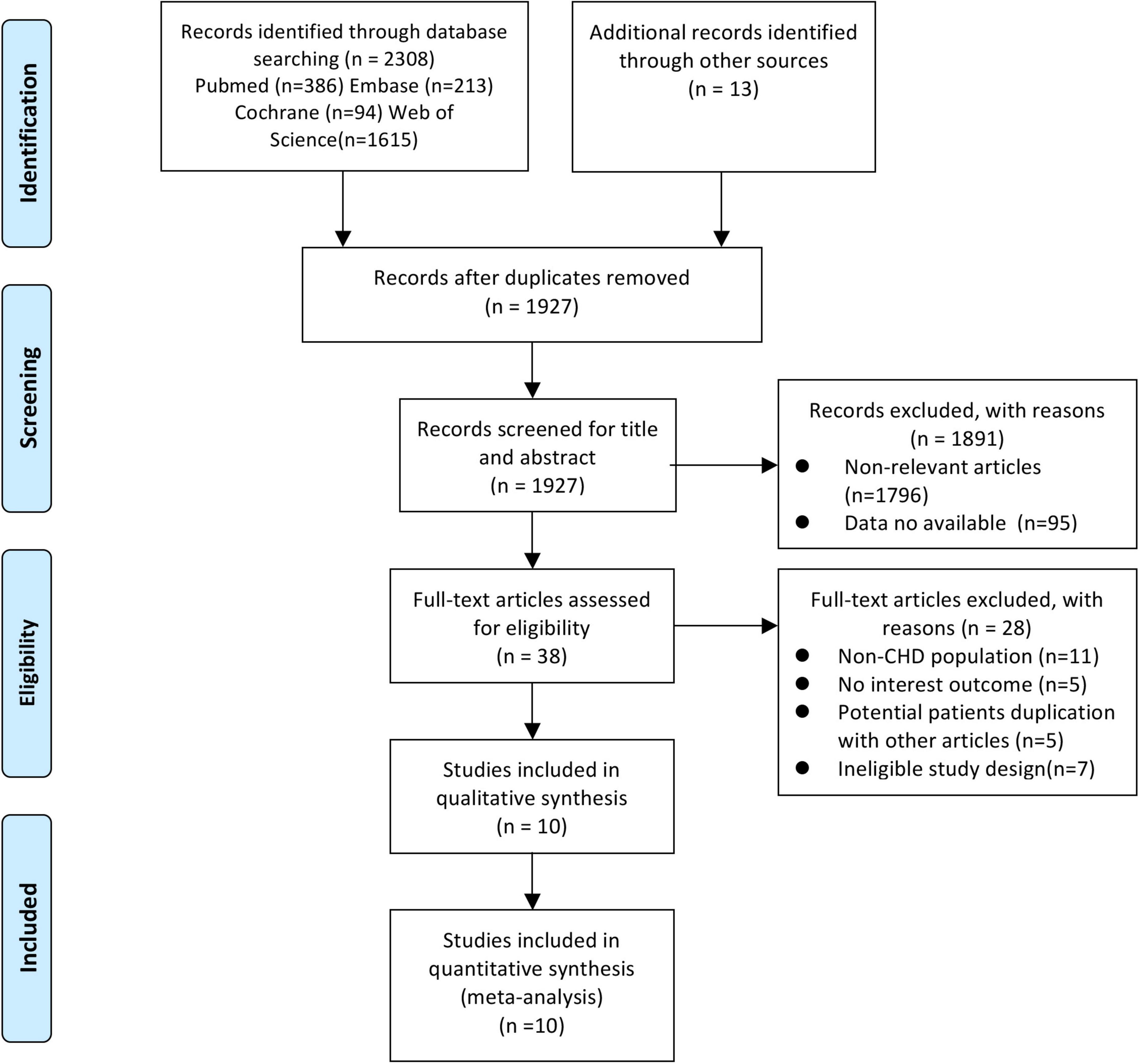
Figure 1. Flow diagram of the study search and selection process. From Moher et al. (65).
Study characteristics and quality assessment
The main characteristics of the included studies are summarized in Table 1. Of the 10 included studies, three were performed in China (11, 50, 54), three in Japan (4, 13, 51), two in Denmark (52, 53), one in the United States (12), and one collaborative study involving multiple countries (10). The studies were published from 1999 (13) to 2021 (11), of which nine were prospective cohort designs (4, 11–13, 50–54) and one was retrospective cohort design (10). In addition, two studies included two cohorts (50, 52). The sample size ranged from 120 (51) to 6723 (11). The follow-up time ranged from 1.7 (51) to 7.0 (52) years, and participants’ age varied from 57.7 (11) to 68.0 (53) years. The average NOS scores for these studies included were 7.3, demonstrating that the quality of the cohort study was good.
Meta-analysis results
The results demonstrated that elevated RC concentration was related to an increased risk of composite endpoint events (MACEs and all-cause death) (RR = 1.54, 95% CI: 1.26–1.87, P < 0.0001) in a random-effect model (Figure 2). Significant heterogeneity between studies was observed (I2 = 85%, P < 0.0001), and sensitivity analysis indicated that the total combined effect size did not change significantly in each step, demonstrating that the meta-analysis results were relatively stable. However, if the study conducted by Martin et al. (12) was eliminated, the heterogeneity decreased significantly (I2 = 44%, RR = 1.61, 95% CI: 1.43–1.80).
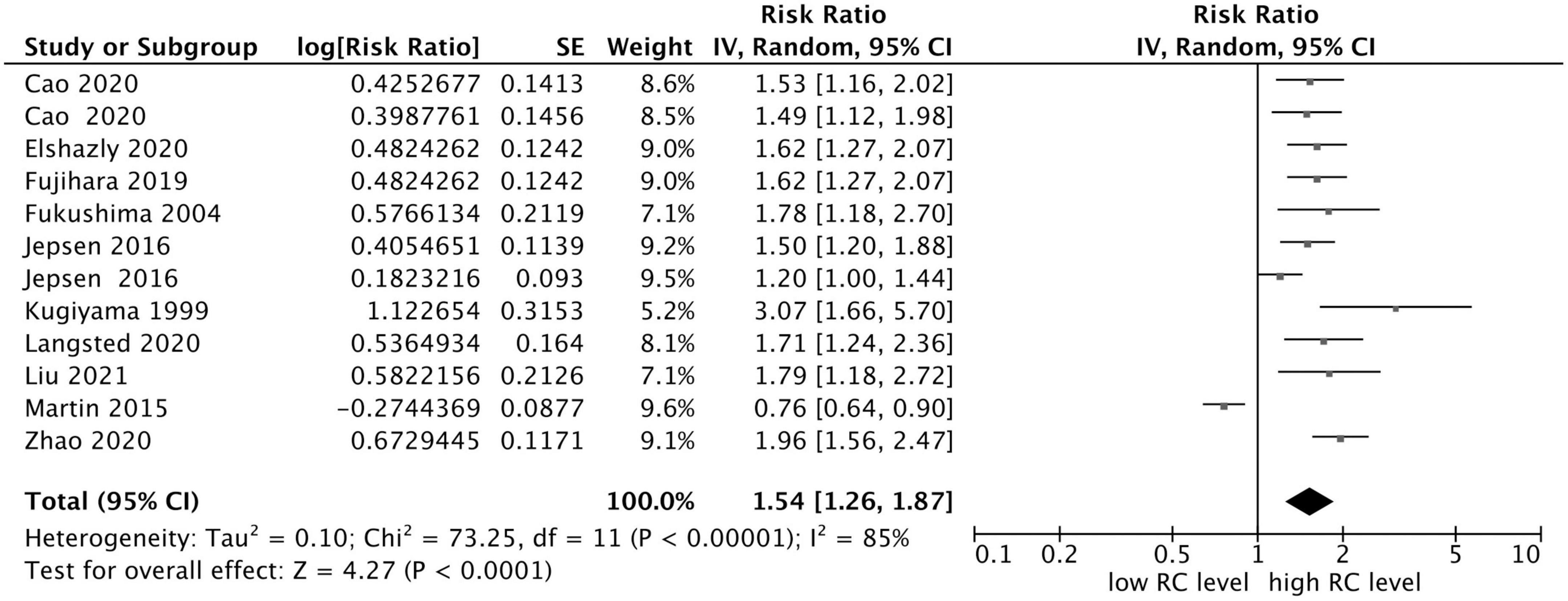
Figure 2. Forest plots showing the pooled RR with 95% CI of composite endpoint events for the highest versus lowest remnant cholesterol concentration.
In addition, as shown in Figure 3, eight of 10 studies reported the MACEs as an outcome, and the other two studies addressed the all-cause mortality outcome, in which one study reported both MACEs risk and all-cause mortality risk. Furthermore, patients with CHD with elevated RC concentration had an increased risk of MACEs (RR = 1.70, 95% CI: 1.54–1.88, P < 0.0001) without significant heterogeneity (I2 = 0%, P = 0.56) in a fixed-effect model. However, the risk of all-cause mortality was not statistically significant (RR = 1.16, 95% CI: 0.79–1.69, P = 0.44), with significant heterogeneity (I2 = 89%, P < 0.0001) in a random effect model. In addition, sensitivity analysis showed that after removing the study of Martin et al. (12), the heterogeneity decreased significantly, and the total combined effect size changed as well (I2 = 32%, RR = 1.34, 95% CI: 1.10–1.63, P = 0.003).
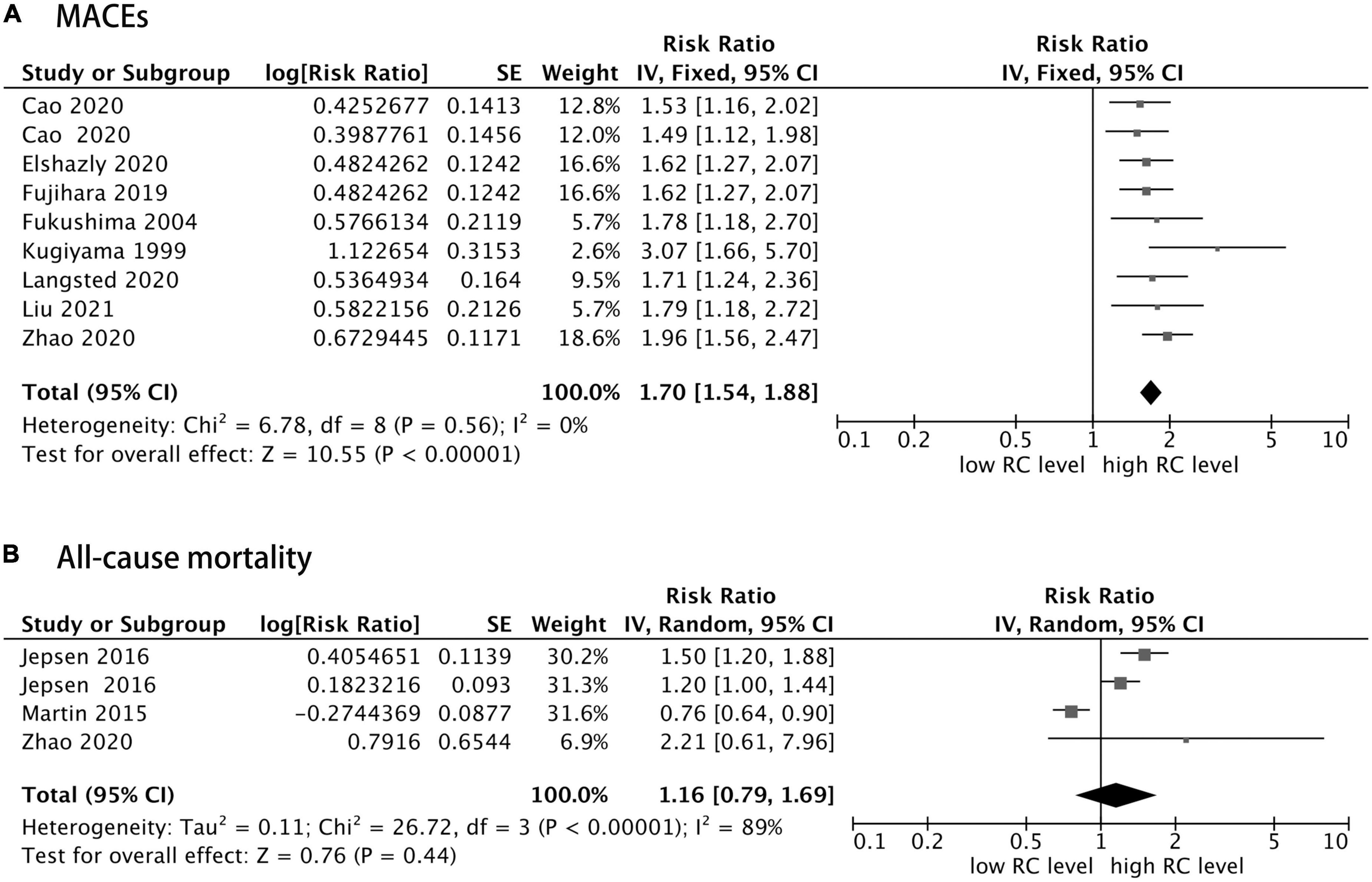
Figure 3. Forest plots showing the pooled RR with 95% CI of major adverse cardiovascular events (MACEs) (A) and all-cause mortality (B) for the highest versus lowest remnant cholesterol concentration.
Subgroup analysis
In the subgroup analysis, the association remained constant, suggesting a positive association between RC concentration and CHD risks in studies conducted in the Asian region (RR = 1.72, 95% CI: 1.53–1.95, P < 0.0001) for those with the diagnosis of MI (RR = 1.64, 95% CI: 1.46–1.85, P < 0.0001) and with older age (≥65 years old) (RR = 1.78, 95% CI: 1.46–2.15, P < 0.0001). In addition, this constant association continued in selected studies for those publications with sample size <1,000 cases (RR = 1.88, 95% CI: 1.38–2.55, P < 0.0001), those that used the immunoseparation method (RR = 1.72, 95% CI: 1.39–2.12, P < 0.0001) and fasting status test (RR = 1.70, 95% CI: 1.51–1.91, P < 0.0001) for RC assessment, and studies with a long follow-up time (≥3 years) (RR = 1.54, 95% CI: 1.35–1.76, P < 0.0001) (Table 2).
Publication bias test
As shown in Figure 4, the funnel plot was asymmetrical, and for further quantitative analysis using Egger’s test, a publication bias was suggested (P < 0.05).
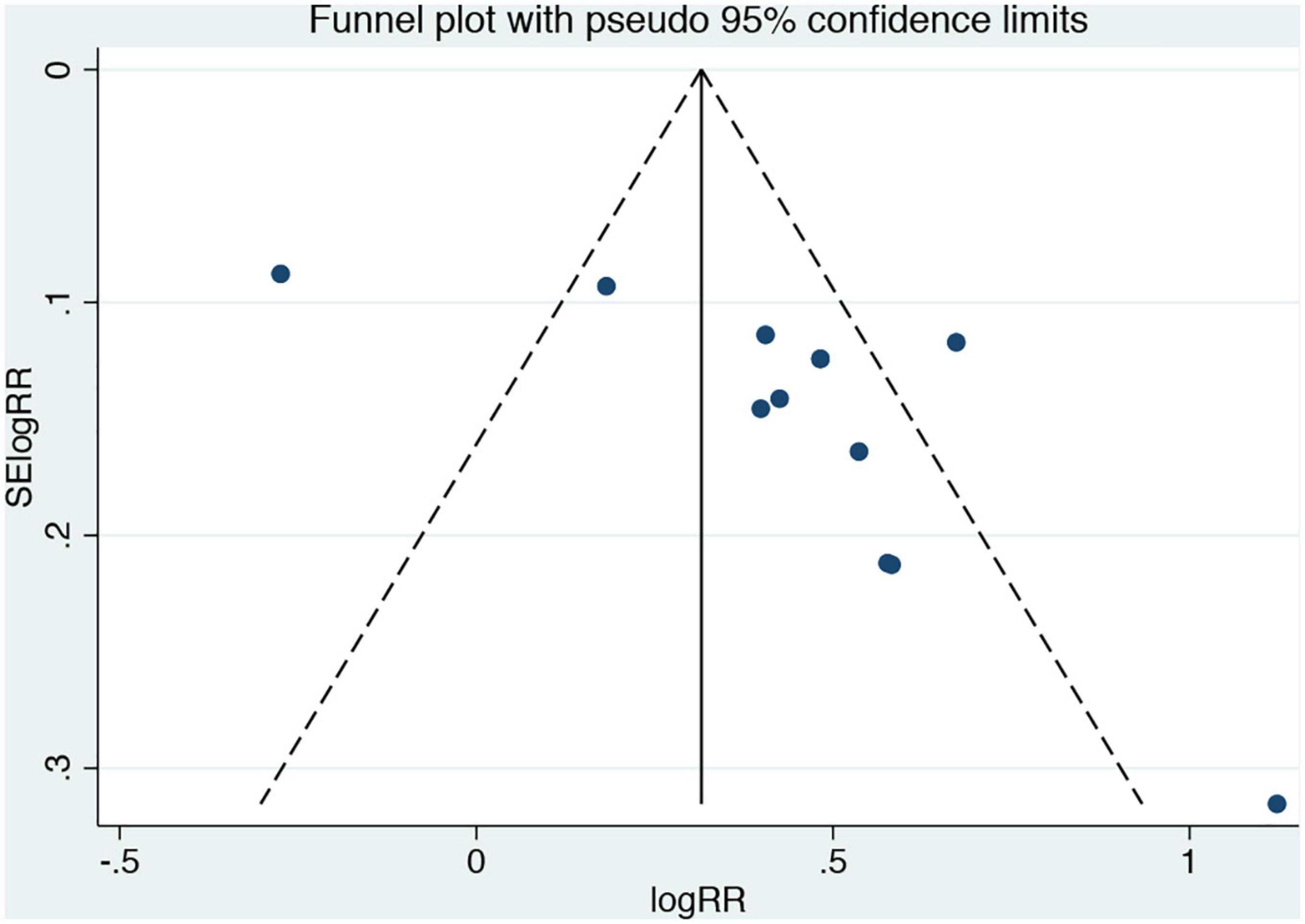
Figure 4. Funnel plots for the analysis of remnant cholesterol concentration and composite endpoint events. Results compare participants in the highest versus lowest remnant cholesterol concentration.
Discussion
In the present study, the relationship between RC concentration and the prognosis of the patients with CHD was evaluated by meta-analysis for the first time. The results illustrated that elevated RC concentration was significantly correlated with an increased risk of the composite endpoint events and MACEs in patients with CHD, but the risk of all-cause mortality was not statistically significant. In addition, the prognostic significance of higher RC concentration on CHD risks was also confirmed in the subgroup analysis. This meta-analysis contributes to the increasing evidence that higher RC concentration may be an independent predictor of poor cardiovascular outcomes in patients with CHD.
Remnant cholesterol, also known as triglyceride-rich lipoprotein cholesterol, is the cholesterol content of all non-LDL and non-HDL. In the fasting state, RC is composed of liver-derived very low-density lipoprotein (VLDL) and intermediate-density lipoprotein (IDL) in the fasting state, as well as intestinal-derived chylomicron remnants (CM) (27). Recently, an increasing number of studies have demonstrated that RC concentration had a relationship to the occurrence and development of atherosclerosis (27, 53). Particularly, when LDL-C was controlled at an appropriate level, RC was assumed to be the main reason for mediating residual risks in the patients with CHD and even a better predictor of risk than LDL-C (52). Unlike LDL-C, RC could easily penetrate the vessel wall and is directly taken up by the scavenging receptors on macrophages without oxidative modification, leading to forming foam cells and promoting atherosclerotic plaque formation (55, 56). In addition, it could also increase the production of reactive oxygen species free radicals, cause endothelial cell dysfunction (57), and induce the expression of pro-inflammatory mediators, as well as the production of cytokines, interleukin, and atherosclerotic adhesion molecules (58). All of the earlier mechanisms can lead to plaque formation and progressive rupture and promote the occurrence of MACEs, which in turn influences the prognosis of the patients.
The previous clinical studies demonstrated similar conclusions between RC concentration and prognosis in the general population but not in patients with CHD. The latest study by Wadstrom et al. (8) revealed that in the Copenhagen General Population Study, during the 15-year follow-up of 106,937 people, elevated RC concentration was relevant to an increased risk of MI up to multivariable-adjusted HR of 4.2, as well as corresponding HRs were 1.8 for ischemic stroke, and 4.8 for peripheral artery disease (PAD). In addition, in the Copenhagen City Heart Study, corresponding HRs were 2.6 for MI, 2.1 for ischemic stroke, and 4.9 for PAD (8). Castañer et al. (27) also reported that in the PREDIMED cohort study of high cardiovascular-risk groups, every 10 mg/dl increase in RC concentration would increase the risk of cardiovascular events by 21%. After multivariate-adjusted analysis, it was concluded that the levels of triglyceride and RC rather than LDL-C were related to the occurrence of MACEs in the population who were overweight or obese and had a high risk of cardiovascular diseases, which were independent of lifestyle and other risk factors. A few other studies have also reached a similar conclusion (32, 33, 59). However, in our study, RC concentration had no effect on the risk of all-cause mortality, possibly due to the available small sample size and high heterogeneity. Furthermore, it seems the results were consistent across the populations and ages in our subgroup analysis. Wang et al. (31) also indicated the importance of preventive efforts across the adult life course. Obviously, these results need further confirmation in more stratified cases.
In addition, genetic evidence has also been found that RC was the risk factor for atherosclerosis. Varbo et al. (9) performed the Mendelian randomization method by detecting the genes of 73,513 people from the Copenhagen study and selected 15 genotypes to observe the incidence of ischemic heart disease (IHD) for each type of gene. The results indicated that for every 1 mmol/l increase of non-fasting RC concentration, the risk of IHD increased by 2.8 times. In another Mendelian randomized trial, Varbo et al. (60) found that elevated RC concentrations in non-fasting status were causally related to inflammation and IHD, whereas increased LDL-C was only related causally to IHD without inflammation. Jørgensen et al. (61) also indicated that genetic variation in ApoA5 related to stepwise increases of the RC concentration and with comparable increases in the risk of MI. Thus, these results illustrated that exposure to elevated RC concentrations caused by genetic abnormalities could bring a greater risk of cardiovascular diseases.
In our study, sensitivity analysis found that the source of heterogeneity might be the research conducted by Martin et al. (12). In this study, the RC evaluation method was significantly different from others, in which the sum of VLDL3-C and IDL-C was used to calculate RC and fasting state was unknown. At present, no uniform method to measure RC concentration has been provided, and accurate measurement is still challenging, which might be the main reason for conflict in the findings (14–16). This was mainly because RC was composed of different lipids and lipoproteins. Then, its rapid and continuous catabolism, the size, quantity, density, and composition of lipoprotein residues were highly dynamic, which was difficult to distinguish from its precursors (non-remnant lipoproteins) (47). Currently, the simplest way to estimate RC concentration is through calculation method (62); that is, RC was calculated as total cholesterol (TC) minus LDL-C minus HDL-C [i.e., RC = (TC)–(LDL-C)–(HDL-C)]. Although it was not as accurate as the method to direct the detection of RC, it has been widely applied at present due to its convenience and simplicity (10, 52–54). Apart from the calculation, there were also several direct methods to identify and quantify RC depending on their specific ingredients, such as immunoseparation (4, 13, 50, 51), direct homogenous assays (50, 52), preparative ultrafiltration (63), and nuclear magnetic resonance (64). In our study, subgroup analysis indicated that elevated RC concentration measured by the immunoseparation method had higher cardiovascular risk. Furthermore, whether in a fasting state during the detection also had an impact on the RC measurement. The subgroup analysis of our study indicated that the CHD patients with elevated RC concentrations in the fasting state had a higher risk of poor prognosis. Apparently, no optimal way of accurately quantifying RC measurement currently exists, so there was a lack of uniform RC cut-off levels to define high RC concentration. However, as increasing importance has been attached to RC, a consensus definition of RC with accurate and reproducible quantitative measurement approaches is eagerly required.
Limitations
This study also had potential limitations. First, the studies included in this study were all published in English, which might have a language bias. Second, the differences in the types of CHD and RC measurement approaches in each study may lead to clinical heterogeneity. Third, the included studies adjusted some confounders, but other unadjusted risk factors may exist. Some traditional CHD factors cannot be extracted adequately from the included studies, which might also lead to bias. Thus, further studies of stratified analysis for the risk factors of CHD outcome are necessary. Fourth, there were insufficient relevant data to compare the prognostic effect between LDL-C and RC from the included studies. Then, it is worth answering this valuable question in future studies. Finally, there was a significant publication bias in our study, suggesting the possible presence of negative results that were not published. Therefore, future studies are needed before a firm conclusion can be drawn concerning the association between RC concentration and CHD outcome.
Conclusion
This meta-analysis of 10 cohort studies showed that CHD patients with elevated RC concentrations had a higher risk of adverse cardiovascular outcomes. Measurement of RC concentration has the potential to improve risk classification in patients with CHD. However, future larger sample sizes and higher quality studies are still required to confirm the findings.
Data availability statement
The original contributions presented in this study are included in this article/Supplementary material, further inquiries can be directed to the corresponding authors.
Author contributions
YT, WW, and LQ performed the literature search, data extraction, and quality assessment. YT performed data analysis and drafted the manuscript. XY and LC critically revised the study. HW and ZZ designed the study, interpreted the data, and revised the manuscript. All authors contributed intellectually to this manuscript and have approved this final version.
Funding
This study was supported by the Chengdu High-level Key Clinical Specialty Construction Project, Sichuan Province, China, No. 202112001.
Conflict of interest
The authors declare that the research was conducted in the absence of any commercial or financial relationships that could be construed as a potential conflict of interest.
Publisher’s note
All claims expressed in this article are solely those of the authors and do not necessarily represent those of their affiliated organizations, or those of the publisher, the editors and the reviewers. Any product that may be evaluated in this article, or claim that may be made by its manufacturer, is not guaranteed or endorsed by the publisher.
Supplementary material
The Supplementary Material for this article can be found online at: https://www.frontiersin.org/articles/10.3389/fcvm.2022.951523/full#supplementary-material
References
1. Roth G, Mensah G, Johnson C, Addolorato G, Ammirati E, Baddour L, et al. Global burden of cardiovascular diseases and risk factors, 1990-2019: update from the GBD 2019 Study. J Am Coll Cardiol. (2020) 76:2982–3021. doi: 10.1016/j.jacc.2020.11.010
2. Fuster V, Kelly B, Vedanthan R. Promoting global cardiovascular health: moving forward. Circulation. (2011) 123:1671–8. doi: 10.1161/CIRCULATIONAHA.110.009522
3. Salinas C, Chapman M. Remnant lipoproteins: are they equal to or more atherogenic than LDL? Curr Opin Lipidol. (2020) 31:132–9. doi: 10.1097/mol.0000000000000682
4. Fujihara Y, Nakamura T, Horikoshi T, Obata J, Fujioka D, Watanabe Y, et al. Remnant lipoproteins are residual risk factor for future cardiovascular events in patients with stable coronary artery disease and on-statin low-density lipoprotein cholesterol levels < 70 mg/dL. Circ J. (2019) 83:1302–8. doi: 10.1253/circj.CJ-19-0047
5. Miller Y, Choi S, Fang L, Tsimikas S. Lipoprotein modification and macrophage uptake: role of pathologic cholesterol transport in atherogenesis. Subcell Biochem. (2010) 51:229–51. doi: 10.1007/978-90-481-8622-8_8
6. Rosenson R, Davidson M, Hirsh B, Kathiresan S, Gaudet D. Genetics and causality of triglyceride-rich lipoproteins in atherosclerotic cardiovascular disease. J Am Coll Cardiol. (2014) 64:2525–40. doi: 10.1016/j.jacc.2014.09.042
7. Holmes M, Millwood I, Kartsonaki C, Hill M, Bennett D, Boxall R, et al. Lipids, lipoproteins, and metabolites and risk of myocardial infarction and stroke. J Am Coll Cardiol. (2018) 71:620–32. doi: 10.1016/j.jacc.2017.12.006
8. Wadström B, Wulff A, Pedersen K, Jensen G, Nordestgaard B. Elevated remnant cholesterol increases the risk of peripheral artery disease, myocardial infarction, and ischaemic stroke: a cohort-based study. Eur Heart J. (2021) 43:3258–69. doi: 10.1093/eurheartj/ehab705
9. Varbo A, Benn M, Tybjærg-Hansen A, Jørgensen A, Frikke-Schmidt R, Nordestgaard B. Remnant cholesterol as a causal risk factor for ischemic heart disease. J Am Coll Cardiol. (2013) 61:427–36. doi: 10.1016/j.jacc.2012.08.1026
10. Elshazly M, Mani P, Nissen S, Brennan D, Clark D, Martin S, et al. Remnant cholesterol, coronary atheroma progression and clinical events in statin-treated patients with coronary artery disease. Eur J Prev Cardiol. (2020) 27:1091–100. doi: 10.1177/2047487319887578
11. Liu H, Li S, Cao Y, Guo Y, Zhu C, Wu N, et al. Association of triglyceride-rich lipoprotein-cholesterol with recurrent cardiovascular events in statin-treated patients according to different inflammatory status. Atherosclerosis. (2021) 330:29–35. doi: 10.1016/j.atherosclerosis.2021.06.907
12. Martin S, Faridi K, Joshi P, Blaha M, Kulkarni K, Khokhar A, et al. Remnant lipoprotein cholesterol and mortality after acute myocardial infarction: further evidence for a hypercholesterolemia paradox from the TRIUMPH Registry. Clin Eardiol. (2015) 38:660–7. doi: 10.1002/clc.22470
13. Kugiyama K, Doi H, Takazoe K, Kawano H, Soejima H, Mizuno Y, et al. Remnant lipoprotein levels in fasting serum predict coronary events in patients with coronary artery disease. Circulation. (1999) 99:2858–60. doi: 10.1161/01.cir.99.22.2858
14. Wilson P, Jacobson T, Martin S, Jackson E, Le N, Davidson M, et al. Lipid measurements in the management of cardiovascular diseases: practical recommendations a scientific statement from the national lipid association writing group. J Clin Lipidol. (2021) 15:629–48. doi: 10.1016/j.jacl.2021.09.046
15. Toth P, Bays H, Brown W, Catapano A, Davidson M, Farnier M, et al. Comparing remnant lipoprotein cholesterol measurement methods to evaluate efficacy of ezetimibe/statin vs statin therapy. J Clin Lipidol. (2019) 13:997–1007.e8. doi: 10.1016/j.jacl.2019.09.001
16. Joshi P, Khokhar A, Massaro J, Lirette S, Griswold M, Martin S, et al. Remnant lipoprotein cholesterol and incident coronary heart disease: the jackson heart and framingham offspring cohort studies. J Am Heart Assoc. (2016) 5:e002765. doi: 10.1161/jaha.115.002765
17. Stroup D, Berlin J, Morton S, Olkin I, Williamson G, Rennie D, et al. Meta-analysis of observational studies in epidemiology: a proposal for reporting. meta-analysis of observational studies in epidemiology (MOOSE) group. JAMA. (2000) 283:2008–12. doi: 10.1001/jama.283.15.2008
18. Moher D, Liberati A, Tetzlaff J, Altman D, PRISMA Group. Preferred reporting items for systematic reviews and meta-analyses: the PRISMA statement. PLoS Med. (2009) 6:e1000097.
19. Tierney J, Stewart L, Ghersi D, Burdett S, Sydes M. Practical methods for incorporating summary time-to-event data into meta-analysis. Trials. (2007) 8:16. doi: 10.1186/1745-6215-8-16
20. Stang A. Critical evaluation of the Newcastle-Ottawa scale for the assessment of the quality of nonrandomized studies in meta-analyses. Eur J Epidemiol. (2010) 25:603–5. doi: 10.1007/s10654-010-9491-z
21. Higgins J, Thompson S, Deeks J, Altman D. Measuring inconsistency in meta-analyses. BMJ. (2003) 327:557–60. doi: 10.1136/bmj.327.7414.557
22. Zhang J, Yu K. What’s the relative risk? A method of correcting the odds ratio in cohort studies of common outcomes. JAMA. (1998) 280:1690–1. doi: 10.1001/jama.280.19.1690
23. McNutt L, Wu C, Xue X, Hafner J. Estimating the relative risk in cohort studies and clinical trials of common outcomes. Am J Epidemiol. (2003) 157:940–3. doi: 10.1093/aje/kwg074
24. Ronksley P, Brien S, Turner B, Mukamal K, Ghali W. Association of alcohol consumption with selected cardiovascular disease outcomes: a systematic review and meta-analysis. BMJ. (2011) 342:d671. doi: 10.1136/bmj.d671
25. Quispe R, Martin S, Michos E, Lamba I, Blumenthal R, Saeed A, et al. Remnant cholesterol predicts cardiovascular disease beyond LDL and ApoB: a primary prevention study. Eur Heart J. (2021) 19:4324–32. doi: 10.1093/eurheartj/ehab432
26. Chen Y, Li G, Guo X, Ouyang N, Li Z, Ye N, et al. The effects of calculated remnant-like particle cholesterol on incident cardiovascular disease: insights from a general Chinese population. J Clin Med. (2021) 10:3388. doi: 10.3390/jcm10153388
27. Castañer O, Pintó X, Subirana I, Amor A, Ros E, Hernáez Á, et al. Remnant cholesterol, not LDL cholesterol, is associated with incident cardiovascular disease. J Am Coll Cardiol. (2020) 76:2712–24. doi: 10.1016/j.jacc.2020.10.008
28. Bonfiglio C, Leone C, Silveira L, Guerra R, Misciagna G, Caruso M, et al. Remnant cholesterol as a risk factor for cardiovascular, cancer or other causes mortality: a competing risks analysis. Nutr Metab Cardiov Dis. (2020) 30:2093–102. doi: 10.1016/j.numecd.2020.07.002
29. Saeed A, Feofanova E, Yu B, Sun W, Virani S, Nambi V, et al. Remnant-like particle cholesterol, low-density lipoprotein triglycerides, and incident cardiovascular disease. J Am Coll Cardiol. (2018) 72:156–69. doi: 10.1016/j.jacc.2018.04.050
30. Varbo A, Nordestgaard B. Remnant cholesterol and risk of ischemic stroke in 112,512 individuals from the general population. Ann Neurol. (2019) 85:550–9. doi: 10.1002/ana.25432
31. Wang A, Tian X, Zuo Y, Chen S, Meng X, Chen P, et al. Age dependent association between remnant cholesterol and cardiovascular disease. Atheroscler Plus. (2021) 45:18–24. doi: 10.1016/j.athplu.2021.09.004
32. Ikezaki H, Lim E, Cupples L, Liu C, Asztalos B, Schaefer E. Small dense low-density lipoprotein cholesterol is the most atherogenic lipoprotein parameter in the prospective framingham offspring study. J Am Heart Assoc. (2021) 10:e019140. doi: 10.1161/jaha.120.019140
33. Hussain A, Sun C, Selvin E, Nambi V, Coresh J, Jia X, et al. Triglyceride-rich lipoproteins, apolipoprotein C-III, angiopoietin-like protein 3, and cardiovascular events in older adults: atherosclerosis risk in communities (ARIC) study. Eur J Prev Cardiol. (2021) 14:e53–64. doi: 10.1093/eurjpc/zwaa152
34. Varbo A, Nordestgaard B. Directly measured vs. calculated remnant cholesterol identifies additional overlooked individuals in the general population at higher risk of myocardial infarction. Eur Heart J. (2021) 42:4833–43. doi: 10.1093/eurheartj/ehab293
35. Imke C, Rodriguez B, Grove J, McNamara J, Waslien C, Katz A, et al. Are remnant-like particles independent predictors of coronary heart disease incidence? The Honolulu Heart Study. Arterioscler Thromb Vasc Biol. (2005) 25:1718–22. doi: 10.1161/01.ATV.0000173310.85845.7b
36. Cao Y, Zhang H, Jin J, Liu H, Zhang Y, Gao Y, et al. The longitudinal association of remnant cholesterol with cardiovascular outcomes in patients with diabetes and pre-diabetes. Cardiovasc Diabetol. (2020) 19:104. doi: 10.1186/s12933-020-01076-7
37. Hong L, Yan X, Lu Z, Fan Y, Ye F, Wu Q, et al. Predictive value of non-fasting remnant cholesterol for short-term outcome of diabetics with new-onset stable coronary artery disease. Lipids Health Dis. (2017) 16:7. doi: 10.1186/s12944-017-0410-0
38. Winter M, Wiesbauer F, Blessberger H, Pavo N, Sulzgruber P, Huber K, et al. Lipid profile and long-term outcome in premature myocardial infarction. Eur J Clin Invest. (2018) 48:e13008. doi: 10.1111/eci.13008
39. Sakai K, Koba S, Nakamura Y, Yokota Y, Tsunoda F, Shoji M, et al. Small dense low-density lipoprotein cholesterol is a promising biomarker for secondary prevention in older men with stable coronary artery disease. Geriatr Gerontol Int. (2018) 18:965–72. doi: 10.1111/ggi.13287
40. Varbo A, Freiberg J, Nordestgaard B. Extreme nonfasting remnant cholesterol vs extreme LDL cholesterol as contributors to cardiovascular disease and all-cause mortality in 90000 individuals from the general population. Clin Chem. (2015) 61:533–43. doi: 10.1373/clinchem.2014.234146
41. Varbo A, Freiberg J, Nordestgaard B. Remnant cholesterol and myocardial infarction in normal weight, overweight, and obese individuals from the copenhagen general population study. Clin Chem. (2018) 64:219–30. doi: 10.1373/clinchem.2017.279463
42. Jorgensen A, Frikke-Schmidt R, West A, Grande P, Nordestgaard B, Tybjaerg-Hansen A. Genetically elevated non-fasting triglycerides and calculated remnant cholesterol as causal risk factors for myocardial infarction. Eur Heart J. (2013) 34:1826–33.
43. Wang K, Ding Y, Gao W, Wang R, Yang J, Liu X, et al. Association of increased remnant cholesterol and the risk of coronary artery disease: a retrospective study. Front Cardiovasc Med. (2021) 8:740596. doi: 10.3389/fcvm.2021.740596
44. Kim J, Park J, Jeong S, Schellingerhout D, Park J, Lee D, et al. High levels of remnant lipoprotein cholesterol is a risk factor for large artery atherosclerotic stroke. J Clin Neurol. (2011) 7:203–9. doi: 10.3988/jcn.2011.7.4.203
45. McNamara J, Shah P, Nakajima K, Cupples L, Wilson P, Ordovas J, et al. Remnant-like particle (RLP) cholesterol is an independent cardiovascular disease risk factor in women: results from the Framingham Heart Study. Atherosclerosis. (2001) 154:229–36. doi: 10.1016/s0021-9150(00)00484-6
46. Goliasch G, Wiesbauer F, Blessberger H, Demyanets S, Wojta J, Huber K, et al. Premature myocardial infarction is strongly associated with increased levels of remnant cholesterol. J Clin Lipidol. (2015) 9:801–6. doi: 10.1016/j.jacl.2015.08.009
47. Duran E, Pradhan A. Triglyceride-rich lipoprotein remnants and cardiovascular disease. Clin Chem. (2021) 67:183–96. doi: 10.1093/clinchem/hvaa296
48. Wulff A, Nordestgaard B, Tybjaerg-Hansen A. APOC3 loss-of-function mutations, remnant cholesterol, low-density lipoprotein cholesterol, and cardiovascular risk: mediation- and meta-analyses of 137895 individuals. Arterioscler Thromb Vasc Biol. (2018) 38:660–8. doi: 10.1161/atvbaha.117.310473
49. Feng X, Guo Q, Zhou S, Sun T, Liu Y, Zhou Z, et al. Could remnant-like particle cholesterol become a risk factor in diabetic menopausal women with coronary artery disease? A cross-sectional study of single academic center in China. Lipids Health Dis. (2020) 19:44. doi: 10.1186/s12944-020-01224-8
50. Cao Y, Zhang H, Jin J, Liu H, Zhang Y, Xu R, et al. Prognostic utility of triglyceride-rich lipoprotein-related markers in patients with coronary artery disease. J Lipid Res. (2020) 61:1254–62. doi: 10.1194/jlr.RA120000746
51. Fukushima H, Sugiyama S, Honda O, Koide S, Nakamura S, Sakamoto T, et al. Prognostic value of remnant-like lipoprotein particle levels in patients with coronary artery disease and type II diabetes mellitus. J Am Coll Cardiol. (2004) 43:2219–24. doi: 10.1016/j.jacc.2003.09.074
52. Jepsen A, Langsted A, Varbo A, Bang L, Kamstrup P, Nordestgaard B. Increased remnant cholesterol explains part of residual risk of all-cause mortality in 5414 patients with ischemic heart disease. Clin Chem. (2016) 62:593–604. doi: 10.1373/clinchem.2015.253757
53. Langsted A, Madsen C, Nordestgaard B. Contribution of remnant cholesterol to cardiovascular risk. J Intern Med. (2020) 288:116–27. doi: 10.1111/joim.13059
54. Zhao Q, Zhang T, Cheng Y, Ma Y, Xu Y, Yang J, et al. Prognostic impact of estimated remnant-like particle cholesterol in patients with differing glycometabolic status: an observational cohort study from China. Lipids Health Dis. (2020) 19:179. doi: 10.1186/s12944-020-01355-y
55. Sandesara P, Virani S, Fazio S, Shapiro M. The forgotten lipids: triglycerides, remnant cholesterol, and atherosclerotic cardiovascular disease risk. Endocr Rev. (2019) 40:537–57. doi: 10.1210/er.2018-00184
56. Boren J, Olin K, Lee I, Chait A, Wight T, Innerarity T. Identification of the principal proteoglycan-binding site in LDL. A single-point mutation in apo-B100 severely affects proteoglycan interaction without affecting LDL receptor binding. J Clin Invest. (1998) 101:2658–64. doi: 10.1172/JCI2265
57. Wang L, Gill R, Pedersen T, Higgins L, Newman J, Rutledge J. Triglyceride-rich lipoprotein lipolysis releases neutral and oxidized FFAs that induce endothelial cell inflammation. J Lipid Res. (2009) 50:204–13. doi: 10.1194/jlr.M700505-JLR200
58. Shin H, Kim Y, Kim K, Lee J, Hong K. Remnant lipoprotein particles induce apoptosis in endothelial cells by NAD(P)H oxidase-mediated production of superoxide and cytokines via lectin-like oxidized low-density lipoprotein receptor-1 activation: prevention by cilostazol. Circulation. (2004) 109:1022–8. doi: 10.1161/01.CIR.0000117403.64398.53
59. Duran E, Aday A, Cook N, Buring J, Ridker P, Pradhan A. Triglyceride-rich lipoprotein cholesterol, small dense LDL cholesterol, and incident cardiovascular disease. J Am Coll Cardiol. (2020) 75:2122–35. doi: 10.1016/j.jacc.2020.02.059
60. Varbo A, Benn M, Tybjaerg-Hansen A, Nordestgaard B. Elevated remnant cholesterol causes both low-grade inflammation and ischemic heart disease, whereas elevated low-density lipoprotein cholesterol causes ischemic heart disease without inflammation. Circulation. (2013) 128:1298–309. doi: 10.1161/CIRCULATIONAHA.113.003008
61. Jørgensen A, Frikke-Schmidt R, West A, Grande P, Nordestgaard B, Tybjærg-Hansen A. Genetically elevated non-fasting triglycerides and calculated remnant cholesterol as causal risk factors for myocardial infarction. Eur Heart J. (2013) 34:1826–33.
62. Jacobson T, Ito M, Maki K, Orringer C, Bays H, Jones P, et al. National lipid association recommendations for patient-centered management of dyslipidemia: part 1–full report. J Clin Lipidol. (2015) 9:129–69. doi: 10.1016/j.jacl.2015.02.003
63. Kulkarni K. Cholesterol profile measurement by vertical auto profile method. Clin Lab Med. (2006) 26:787–802. doi: 10.1016/j.cll.2006.07.004
64. Otvos J. Measurement of triglyceride-rich lipoproteins by nuclear magnetic resonance spectroscopy. Clin Cardiol. (1999) 22:II21–7. doi: 10.1002/clc.4960221405
Keywords: remnant cholesterol, coronary heart disease, prognosis, dyslipidemia, meta-analysis
Citation: Tian Y, Wu W, Qin L, Yu X, Cai L, Wang H and Zhang Z (2023) Prognostic value of remnant cholesterol in patients with coronary heart disease: A systematic review and meta-analysis of cohort studies. Front. Cardiovasc. Med. 9:951523. doi: 10.3389/fcvm.2022.951523
Received: 24 May 2022; Accepted: 30 December 2022;
Published: 19 January 2023.
Edited by:
Chu-Huang Chen, The Texas Heart Institute, United StatesReviewed by:
Rizanda Machmud, Andalas University, IndonesiaChueh-Lung Hwang, The University of Texas at Arlington, United States
Copyright © 2023 Tian, Wu, Qin, Yu, Cai, Wang and Zhang. This is an open-access article distributed under the terms of the Creative Commons Attribution License (CC BY). The use, distribution or reproduction in other forums is permitted, provided the original author(s) and the copyright owner(s) are credited and that the original publication in this journal is cited, in accordance with accepted academic practice. No use, distribution or reproduction is permitted which does not comply with these terms.
*Correspondence: Han Wang, wanghan@swjtu.edu.cn; Zhen Zhang,
zhangzhen@swjtu.edu.cn