- 1Université de Strasbourg (UNISTRA), Faculté de Médecine, Hôpitaux Universitaires de Strasbourg, Nouvel Hôpital Civil, Service de Médecine Intensive-Réanimation, Strasbourg, France
- 2Intensive Cardiac Care Unit, Cardiology Department, University Hospital of Rangueil, Toulouse, France
- 3Department of Medical Oncology, Strasbourg-Europe Cancer Institute (ICANS), Strasbourg, France
- 4Medical Intensive Care Unit, Saint-Louis Hospital, Assistance Publique des Hôpitaux de Paris, University of Paris, Paris, France
- 5Intensive Care Unit, Department of Acute Medicine, University Hospital, Basel, Switzerland
- 6Department of Clinical Research, University of Basel, Basel, Switzerland
- 7INSERM (French National Institute of Health and Medical Research), UMR 1260, Regenerative Nanomedicine (RNM), FMTS, Strasbourg, France
Sophisticated cancer treatments, cardiovascular risk factors, and aging trigger acute cardiovascular diseases in an increasing number of cancer patients. Among acute cardiovascular diseases, cancer treatment, as well as the cancer disease itself, may induce a cardiogenic shock. Although increasing, these cardiogenic shocks are still relatively limited, and their management is a matter of debate in cancer patients. Etiologies that cause cardiogenic shock are slightly different from those of non-cancer patients, and management has some specific features always requiring a multidisciplinary approach. Recent guidelines and extensive data from the scientific literature can provide useful guidance for the management of these critical patients. Even if no etiologic therapy is available, maximal intensive supportive measures can often be justified, as most of these cardiogenic shocks are potentially reversible. In this review, we address the major etiologies that can lead to cardiogenic shock in cancer patients and discuss issues related to its management.
Introduction
Cancer and cardiovascular diseases are the two most prevalent diseases worldwide and the leading cause of death in modern countries (1). However, due to advances in medicine, the mortality rate among cancer patients (CPs) has decreased dramatically over the last three decades (2, 3). Therefore, more of these CPs who also share many cardiovascular risk factors (4) develop fatal heart diseases (5), such as cardiogenic shock (CS) which can be caused by many distinct etiologies (Figure 1).
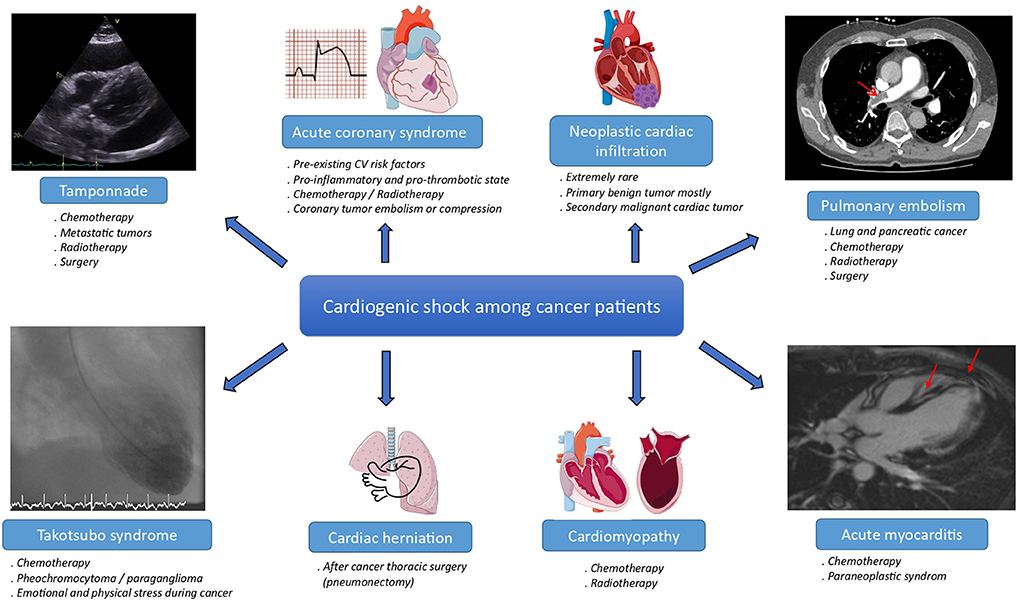
Figure 1. Main etiologies of cardiogenic shock among cancer patients. CV, cardiovascular. The meaning of the red arrow is “Magnetic resonance imaging of acute myocarditis”.
Cardiogenic shock may result from cancer itself, directly or indirectly through increased coronary risk and thromboembolic events, tamponade, or paraneoplastic syndrome. In these situations, CS belongs to cardio-oncology syndrome type one in the new classification of cardio-oncology syndromes published in the Journal of the International Cardio-Oncology Society (ICOS) (6). Cardiogenic shock may also result from collateral side effects of cancer treatments, which belong to cardio-oncology syndrome type two (6). The inflammation in cardio-oncology type two syndrome has been described as a hallmark of cancer therapy-induced cardiovascular complications, whether through an increase in proinflammatory cytokines such as interleukin-1 or inflammasome (7).
Cancer treatments are improving, but many therapies such as surgery, radiotherapy, chemotherapy, targeted therapies (e.g., hormone therapies, angiogenesis inhibitors), and immunotherapy might have significant cardiotoxicity. Consequently, cardio-oncology has emerged as a new and fast-growing subspecialty in recent years (8) with cardio-oncology teams and cardio-oncology services being progressively implemented in hospitals (9).
Cardiogenic shock is defined as a primary cardiac dysfunction with low cardiac output and without hypovolemia leading to critical organ hypoperfusion and tissue hypoxia. Diagnostic criteria include persistent hypotension and signs of compromised end-organ perfusion (10, 11). In recent times, CS remains one of the greatest challenges in cardiology and intensive care medicine. Compared to acute heart failure, CS has a 10-fold higher in-hospital mortality rate, remaining >40% despite medical and surgical advances (12). Given the specificities of different cancers or their treatments, management of CS in this particular setting requires a comprehensive knowledge of the various determinants in addition to a multidisciplinary collaboration between intensivists, cardiologists, cardiac surgeons, and oncologists. To help physicians evaluate and manage CP with acute cardiovascular disease, recent guidelines were published by a Task Force including the Association of Acute Cardiovascular Care (ACVC) and the Council of Cardio-Oncology (CO council) of the European Society of Cardiology (ESC) in 2021 (13).
In this review, we aim to summarize the latest evidence on CS among oncological patients to provide an overview of research areas and knowledge that are often at the crossroads of several medical specialties.
Epidemiology
Epidemiology data underlying the relationship between CS and cancer are scarce, as most of the published data are case reports. In the largest European prospective multicenter study, which included CS patients from a broad spectrum of etiologies, 7% were CPs. In this registry, 30-day mortality was 29.4% in CPs. Interestingly, in this study, cancer was not an independent variable associated with 30-day mortality (14).
In contrast, in the administrative Nationwide Inpatient Sample Database which includes around 500,000 patients hospitalized for CS between 2004 and 2011, cancer was independently associated with poor outcomes. Having a solid tumor, with or without metastases, accounted for the most disadvantageous prognostic factors with an odds ratio (OR) of 2.05 and 1.50 respectively (15). In another study, based on the same database but for the period 2010 and 2014, CS was higher in patients with colon cancer and lower in patients with multiple myeloma, while patients with lung cancer had the highest risk of dying from CS (16).
Cardiogenic shock related to acute coronary syndromes
In the largest published registry of acute coronary syndrome (ACS) in CP, the proportion of ACS among patients with cancer on treatment was ~3%. This number has been increasing since the early 2000s and the most common types of associated malignancies are lung, prostate, and breast cancers (17). The risk of ACS in patients with a current or historical diagnosis of cancer is more than two times higher as compared to the general population (18). Indeed, CPs are often older and may share traditional cardiovascular risk factors (4). In addition, cardiovascular toxicity induced by cancer therapies may cause ACS through different pathophysiological mechanisms (Table 1). Coronary vasospasm is one of the most described mechanisms and is typically caused by 5-fluoro-uracil (5-FU) or its prodrug capecitabine (19). Other mechanisms may include plaque rupture resulting from cisplatin and vinca alkaloids (20), or coronary thrombosis due to pro-inflammatory and prothrombotic conditions associated with increased platelet aggregability induced by specific cancer therapies (e.g., cisplatin, vascular endothelial growth factor (VEGF) signaling pathway inhibitors and cyclophosphamide) (21). Direct endothelial injury associated with accelerated coronary artery disease induced by radiotherapy (22) can cause ACS typically within 10 to 30 years following treatment but rarely during treatment (23). Moreover, mortality caused by ACS is higher in oncological patients (24, 25) with malignancy being considered an independent predictor of increased risk of repeated revascularization and stent thrombosis (17). Patients with ACS and malignancies might also be more likely to experience CS (26), even if this additional risk is not found in all studies (27).
Clinical presentation and diagnostic algorithms of ACS in CPs are relatively close to those in patients without cancer. However, ACS symptoms can frequently be atypical in CPs, mistaken with cancer symptoms such as cancer-related anemia (28), with less than one-third of them experiencing chest pain, and less than half of them having dyspnea (29).
Acute coronary syndrome management in CS remains almost identical to non-CPs, although sometimes complicated by increased comorbidities in CPs (30) or cytopenia. Chemotherapy-induced thrombocytopenia may be caused by DNA synthesis inhibition in megakaryocyte development, leading to megakaryocyte progenitor cell death due to alkylating agents or by oxaliplatin-dependent antibodies cross-reaction with platelet antigens (31). Consensus statements for ACS management in CPs advise early echocardiography to evaluate left ventricular function and exclude cancer or cancer therapy-related complications (13). Cardiotoxic cancer therapy should be at least temporarily interrupted after multidisciplinary discussion, especially if a causal relation is suspected (13). Experts advise that if a stent is needed, a drug-eluting stent (preferred over balloon angioplasty or bare metal stent) together with shortened dual antiplatelet therapy duration (mandatory for only 1 month for instance) is probably the safest choice in CPs undergoing percutaneous coronary intervention (PCI) who do not need short-term surgery. Aspirin and clopidogrel should be preferred to ticagrelor and prasugrel because of the high bleeding risk and limited data regarding both efficacy and safety in patients with active cancer (13). Thrombocytopenia due to cancer or cancer therapy is observed in about 10% of patients, which makes ACS management challenging because of an increased risk of bleeding complications. In CPs, experts advise that aspirin and clopidogrel can be administered if platelets are >10,000 and >30,000/μL, respectively. Experts also suggest that a minimum platelet count of 30,000 and 50,000/μL is required for PCI and coronary artery bypass grafting respectively (13).
Cardiogenic/obstructive shock related to acute pulmonary embolism
Incidence of venous thromboembolic events (VTE) in CPs is around 15% (32). Cancer patients have an estimated seven-fold increased risk for VTE compared to the general population, especially in the first few months after cancer diagnosis (33). Thus, VTE is the second leading cause of death after cancer progression (34). Lung and pancreatic cancer are the most common malignancies associated with VTE (35, 36). In addition, lung and colorectal cancers are associated with the highest thromboembolic risk leading to pulmonary embolism (PE) (37).
The association between cancer and hypercoagulable state, sometimes eponymously referred to as Trousseau syndrome, has been known for more than a century. In addition to the usual risk factors, the hypercoagulable state of cancer is driven by activation of the coagulation cascade and platelet aggregation caused by tumor expression of procoagulant proteins released into the circulation such as tissue factor, plasminogen activator inhibitor-1, and podoplanin (38). Furthermore, cancer treatments such as surgery, hospitalization, central venous catheters, anti-tumor drugs [e.g., cisplatin, 5-fluorouracil (5-FU), tamoxifen], as well as supportive therapies (e.g., erythropoietin), may increase thrombosis via still incompletely understood mechanisms (39).
The risk of acute PE is therefore relatively high in CPs and its incidence is increased by surgery, chemotherapy, radiotherapy, and disease progression (40). In a retrospective study assessing the routine histologic examination of more than 1,300 surgical embolectomies in a general population, of whom 30% had a history of malignant disease, direct neoplastic emboli accounted for <1%. Among these <1% of patients, neoplastic embolism was the first manifestation of an underlying neoplasm in most cases, mainly caused by unknown lung cancer (41). This implies that CPs are much more likely to develop non-neoplastic thromboembolism than neoplastic emboli, although few rare cases of neoplastic arterial embolism leading to fulminant pulmonary hypertension complicated by right ventricular failure and cardiogenic shock have been identified (42, 43).
In a recent study, acute PE in CPs was associated with a 90% increase in all-cause inpatient mortality (44). These patients are less likely to present with chest pain (45) but most of them report dyspnea (40).
Guidelines recommend the use of thrombolysis for high-risk PE unless contraindicated (46). The only absolute contraindication for thrombolysis in cancer is central nervous system neoplasm, for which pulmonary embolectomy is recommended (46). Even though thrombolysis is not formally contraindicated in CPs, they are less likely to receive thrombolysis (47, 48) due to bleeding risk concerns and probably the intensity of cancer care.
Recent results on a retrospective database showed that surgical thrombectomy in patients with cancer is complicated with worse in-hospital outcomes, including mortality and more post-procedural bleeding than thrombolysis (49). Hence, another interesting option that should be considered, according to recent French and European guidelines, is embolectomy via percutaneous catheter-directed treatment for patients with high-risk PE in whom intravenous thrombolysis is contraindicated (46, 50).
Mortality due to PE is more important during the first 3 months but even CPs who survive beyond this period have an increased risk of death compared to the general population (51). However, with a median survival time of more than 2 years, maximal treatment intensity during the acute phase should be considered in CPs with PE (52), especially in young patients with few comorbidities and early-stage cancer.
Finally, cancer-associated arterial thromboembolism is a less frequent but key part of Trousseau syndrome. In the CATS cohort, a cohort of 1,880 patients with active newly diagnosed or relapsed cancer, the frequency of arterial thromboembolism was 2.6% during a median prospective observation time of 2 years (53). This was mainly caused by lung and kidney cancer. Thus, in CPs, the development of arterial thromboembolism has been reported to be associated with a three to five-fold increased risk of death (54).
Cardiogenic shock related to acute cardiomyopathy
Cardiomyopathy-associated ventricular dysfunction is another typical clinical scenario that may lead to CS in CPs, mostly due to cardiotoxic anticancer agents. Numerous guidelines emphasize the need to identify patients with an increased risk of developing cardiovascular toxicity (55–57). Although slightly different, all definitions include patients with previous heart disease and abnormal left ventricular function, elevated cardiac biomarkers before initiation of anticancer therapy, prior mediastinal radiotherapy, and patients with prior or ongoing anthracycline treatment or HER2 (Human Epidermal Growth Factor Receptor-2) targeted agents such as trastuzumab and trastuzumab-derived antibody-drug conjugates (58).
Among chemotherapy drugs that are most likely to induce cardiotoxicity, anthracyclines (e.g., doxorubicin) have been used since the late 1950s to treat solid and hematologic cancers such as lymphoma, leukemia, sarcoma, and breast cancer. Anthracyclines are associated with several cardiovascular toxicities, including dilated cardiomyopathy with left ventricular systolic dysfunction leading to heart failure (59). In an Italian prospective study assessing more than 2,600 patients, the overall incidence of anthracycline-induced cardiotoxicity was 9% (60). This cardiotoxicity was dose-dependent, with the highest incidence observed during the first year after the completion of chemotherapy in 98% of the cases. However, early detection and heart failure therapy allow full or partial recovery in 82% of patients (60). Notably, in this study, the more severe the cardiotoxicity was, the more tendency there was for life-threatening arrhythmias or conduction disturbances requiring pacemaker implantation (60).
Anthracycline-associated cardiotoxicity is now thought to occur at the time of first exposure, a hypothesis supported by the finding of troponin release after administration, mainly as a consequence of increased intracellular Ca2+concentration, oxidative stress, DNA damage, and impairment of DNA repair through inhibition of the topoisomerase II, activation of cell senescence, and cell death (61). Recent evidence points to the involvement of many mechanisms mainly converging toward mitochondrial dysfunction (62).
In the case of anthracycline-induced CS, treatment is based on the empiric management of CS. Through antioxidant effects (via decreased NO production) and reduction of intracellular Ca2+ in cardiomyocytes, sodium-glucose cotransporter 2 (SGLT-2) inhibitors, also called gliflozins, may be a promising cardioprotective strategy in anthracycline-associated cardiotoxicity (63).
However, although described in case reports, rapid recovery of cardiac function appears to be rare (64); some case reports even reported bridges to recovery months after long-term cardiac support device implantation (65, 66).
Besides anthracyclines, anticancer agents including chemotherapies and certain targeted therapies have been associated with acute heart failure (Table 2). Indeed, the transmembrane receptor HER4 partners with HER2 in cardiomyocytes, the latter being a target in cancer therapy. Of note, a number of anti-HER2 agents are approved in HER2-positive breast [e.g., monoclonal antibodies, antibody-drug conjugates, and tyrosine kinase inhibitors (TKIs)] and gastric cancer (e.g., monoclonal antibodies, antibody-drug conjugates) (67). Among them, trastuzumab, a targeted therapy to the HER2 receptor in breast cancer and all its derivatives such as trastuzumab emtansine or trastuzumab deruxtecan, can be responsible for acute cardiac toxicity, which is usually reversible as opposed to the aforementioned anthracyclines (59). For patients receiving cardiotoxic chemotherapy, such as anthracycline or other anti-HER2 therapy, guidelines recommend a three-monthly left ventricular ejection fraction monitoring (68).
Cardiogenic shock related to myocarditis
Myocarditis is an inflammatory condition leading to inflammatory cell infiltration into the myocardium. It can be a consequence of anticancer treatment (Table 3) or paraneoplastic syndrome, which can lead to CS in cancer patients (69).
Identified many years ago, myocarditis secondary to the antimetabolite 5-FU is a very rare complication related to an inflammatory response, driven by apoptosis of myocardial and endothelial cells (70). Cyclophosphamide, a nitrogen mustard alkylating agent increasingly used to treat various types of cancers and autoimmune conditions, can rarely lead to myocarditis, occurring within 1–3 weeks, usually after high doses (>1.5 g/m2/day) (71).
Most recently, novel therapies harnessing the immune system, such as immune checkpoint inhibitors (ICI), have been proven to be associated with myocarditis. Although rare (less than 1%), it often occurs about 1 month after the first dose (72). ICI-related myocarditis usually appears in a fulminant presentation with a high fatality rate of almost 50% (73). The histopathological features of ICI-associated myocarditis imply myocardial infiltration of T-lymphocytes, both CD4+ and CD8+, and macrophages leading to myocyte death, without B-lymphocytes being present (74). A potential pathophysiological hypothesis for ICI-myocarditis is that cardiomyocytes may share targeted antigens with the malignancy, thus becoming targets of the same activated T-cells clones, leading to lymphocytic infiltration of the myocardium (75).
Besides cancer therapy, paraneoplastic syndromes are other possible triggers of myocarditis in CP. Catecholaminergic myocarditis has been associated with pheochromocytoma (76, 77), giant-cell myocarditis with lymphoma, sarcoma, lung cancer, and thymomas (78), and eosinophilic myocarditis has been associated in eosinophilic leukemia and lung cancer (79).
As in other causes of myocarditis, empirical treatments of myocarditis are often based on immunosuppressive therapies such as high-dose corticosteroids (61).
In specific ICI-associated myocarditis, high-dose intravenous corticosteroids and withdrawal of ICI are considered the first-line therapy (62), while abatacept (CTLA-4 agonist), alemtuzumab (anti-CD52 antibody), and anti-thymocyte globulin (anti-CD3 antibody) have been suggested in corticosteroid-resistant forms (63).
Cardiogenic shock related to Takotsubo syndrome
Takotsubo syndrome (TTS) among CP has been mainly reported either as a cardiotoxic effect of antineoplastic treatment (Table 4), as a complication of specific tumors [such as pheochromocytoma and paraganglioma (80)], or as a complication of the significant emotional and physical stress that frequently accompanies cancer. TTS is a clinical syndrome that generally presents as chest pain mimicking ACS or as an acute heart failure marked by severe left ventricular systolic dysfunction mostly characterized by apical akinesis or ballooning with hyperdynamic basal segments, usually following an emotion or physical stressor, predominantly affecting post-menopausal women. TTS leading to CS occurs in ~10% of all cases according to the International Takotsubo Registry (InterTAK), which is the largest registry to date (81).
Although the pathophysiology of the syndrome is still not well understood, commonly hypothesized mechanisms include circulating plasma catecholamines surge, inflammation, estrogen deficiency, microvascular dysfunction, and spasm of the epicardial coronary vessels (82). In the latest International Expert Consensus Document on Takotsubo Syndrome, malignancy, chemotherapy, and radiotherapy are listed among its triggers (83). 5-FU or its prodrug (capecitabine) have been involved in up to 50% of reported cases of TTS leading to CS (84). Its hypothetical mechanism may be coronary vasospasm and direct cardiotoxicity through the production of free radicals and microthrombi related to 5-FU–mediated kallikrein stimuli (84).
Interactions between TTS and malignancy are probably more complex than initially thought, as many studies now report that cancer will frequently be diagnosed within a few years following TTS. In a 4-year follow-up study, 9.6% of TTS patients developed malignancies (85) while Burgdorf observed cancer diagnosis in up to 14% of TTS patients in a 3-year follow-up study (86). These data suggest shared environmental or genetic triggers. Thus, reports have suggested variations in common signaling pathways related to survival cascades and cardioprotective roles which are thought to be up-regulated in adrenergic stress, such as the phosphatidyl inositol-3 kinase/protein kinase-B activation (87) and BCL2-associated athanogene3 protein polymorphism (88) as a potential link between cancer and TTS.
Prevalence of cancer in patients presenting with TTS has been reported to account for up to 28.5% (89). Therefore, cancer screening may be useful for patients who do not have a clear TTS-triggering stressor. In comparison to patients without cancer, the co-existence of cancer and TTS results in increased hospital length-of-stay with increased risk of mechanical ventilation (90, 91), cardiac arrest (92), and all-cause in-hospital and long-term mortality (93, 94). However, in the latest analysis of the InterTAK Registry, CPs with TTS did not experience more CS than non-CP (90).
Solid tumors appear more likely to develop TTS compared to hematologic malignancies (95). According to the InterTAK Registry, breast cancer was the most prevalent type of malignancy-related TTS in 26.2% of the cohort followed by tumors affecting the gastrointestinal system and the respiratory tract with a prevalence of 16.1% and 15.4%, respectively. Hematological malignancies were less prevalent than solid tumors, affecting up to 10% of patients (90). These differences are probably also partly due to the specificities of cancer treatment used for each cancer type.
In addition to the tumor type, the stage of cancer is also an important factor to be considered, as TTS appears to be more prevalent in patients with advanced or recurrent disease (95). Although, this could be explained by treatment selection bias.
Even if TTS in CPs remains poorly understood and multifactorial, several hypotheses are currently considered, such as the emotional trauma of the cancer diagnosis, the inflammatory state of cancer, and the physical stress of various cancer treatments (96, 97).
Guidelines regarding TTS management are lacking as no prospective randomized clinical trials have been performed in this patient population. Nevertheless, a recent international expert consensus endorsed by the ESC advises avoiding inotropes such as adrenaline, noradrenaline, dobutamine, and milrinone (83) because TTS patients treated with catecholamine drugs suffer a 20% increased mortality (98), although this may represent a selection bias due to the initial presentation of the patients. Instead, in TTS leading to CS, experts suggest considering the Ca2+-sensitizer levosimendan or short-term mechanical circulatory support (MCS) such as an axial flow pump (AFP) (e.g., impella) or venoarterial-extracorporeal membrane oxygenation (VA-ECMO) (83).
Experts also suggest looking for the presence of left ventricular outflow tract obstruction, which occurs in about 20% of TTS patients with CS (99). In this situation, experts suggest intravenous fluid, short-acting beta-blocker, and AFP to avoid diuretics, nitroglycerin, or intra-aortic balloon pump (IABP) (83).
Finally, pheochromocytomas and paragangliomas are rare neuroendocrine tumors that can cause catecholamine-induced myocardial dysfunction that may be complicated with CS in 2% of patients (76). To be differentiated from the classic phenotype of TTS, these conditions are labeled as TTS phenocopies (82) and may share genetic predispositions (100). Even though there are no guidelines, VA-ECMO support could be a life-saving therapy, allowing myocardial recovery within a few days (77). After hemodynamic stabilization, treatment should include α-blockade to negate the effects of the excess hormones secreted by the pheochromocytoma and minimize intraoperative hemodynamic instability, with elective tumor removal scheduled under stable conditions (77).
Cardiogenic/obstructive shock related to cardiac tamponade
In 1935, thoracic surgeon Claude Beck first described the classic Beck triad in patients with acute cardiac tamponade including “hypotension, increased jugular venous pressure, and a small and quiet heart.”
In recent years, cancer represents ~25% of the cardiac tamponade etiology. Prognosis of cardiac tamponade is essentially related to the etiology, thereby patients with cancer and metastatic involvement of the pericardium usually have a bad short-term prognosis as it is the sign of advanced cancer. In a recent study, factors associated with poor prognosis at 2-years after pericardiocentesis for malignant effusions were age >65 years, platelet counts <20,000/μL, lung cancer, presence of malignant cells in the effusion, and drainage duration (101).
Pericardial effusion develops in up to 21% of patients with underlying malignancy (102). Cancers most frequently presenting with involvement of the pericardium are mainly solid malignancies, such as advanced lung cancer (~30% of patients with lung cancer present pericardial effusion), malignant melanoma (40–70% of patients), breast cancer (~25% of patients), and less frequently hematological malignancies such as leukemia and lymphomas (about 15% of patients) (103).
Most malignancy-related pericardial effusions are caused by direct or metastatic invasion of a non-cardiac tumor. Primary malignant pericardial mesotheliomas or cardiac synovial sarcomas are very rare (104). It has been suggested that the pathophysiology of non-neoplastic effusions in CPs is related to obstruction of the mediastinal lymphatic system by tumor infiltration, which can also result from radiotherapy-induced fibrosis, especially after chemoradiotherapy for lung and esophageal cancers (105).
Effusions can also be paraneoplastic as a result of pericarditis (106). Cancer treatments themselves may affect the pericardium indirectly by increasing the risk of opportunistic viral infections-causing pericarditis or directly by causing pericarditis as with radiation therapy (23), chemotherapy (e.g., cyclophosphamide, anthracyclines), targeted therapy (e.g., TKIs), or immunotherapy (107).
Consensus statements for cardiac tamponade management in CP recommend that immediate echo-guided pericardiocentesis should be preferentially performed (13). Indeed, in a recent clinical report, percutaneous pericardiocentesis with extended catheter drainage was safe and effective in CPs, including those with thrombocytopenia managed by platelet transfusion support (101). A prolonged drainage (2–5 days) together with intrapericardial instillation of sclerosing agents (e.g., bleomycin) is suggested by experts to reduce the risk of recurrences (13). Since surgical pericardiotomy is less effective in CPs and associated with more complications, experts suggest that it should be conducted only when a safe percutaneous approach is not possible (13). In case of recurrent effusions, experts advise the creation of a pericardial window, surgically or via percutaneous balloon pericardiotomy, to reduce the risk of repeated interventions by allowing drainage into an adjacent space, usually the pleura (13), even if the outcome is poor in these situations.
Experts advise treating acute malignant pericarditis in the same way as in non-CPs with non-steroidal anti-inflammatory drugs and colchicine in the absence of contraindications (13) to relieve symptoms and reduce the risk of relapse as well as to avoid the development of constrictive pericarditis (108). Even if pericardial effusion in ICI-related pericarditis is not often associated with hemodynamic compromise and tamponade (109), experts suggest additional treatment with methylprednisolone (1 mg/kg/day) while temporarily discontinuing the ICI (13).
Physicians should be aware that neoplastic tamponades appear to be at the greatest risk for effusive-constrictive pericarditis (110) and pericardial decompression syndrome compared to non-CPs (111). Pericardial decompression syndrome is a very rare but potentially fatal complication following pericardial drainage manifesting with paradoxical hemodynamic deterioration and/or pulmonary edema, commonly associated with ventricular dysfunction. The onset of this syndrome after the procedure varied widely, ranging from “immediate” to 48 h (111).
Cardiogenic/obstructive shock related to cardiac herniation
Cardiac herniation is a very rare complication mainly encountered after cancer thoracic surgery with a high mortality rate (50–100%) (112). Though it was first reported in medical literature in 1948 after a pneumonectomy of the left lung for a carcinoma (113), this disease remains largely unknown (114).
Although it looks similar on both sides of the heart, the pathophysiology mechanism leading to hemodynamic failure is different (115). On the right side, the protrusion of the heart through an ignored or inadequately closed pericardial sac defect usually following a pericardiotomy may enable the heart to rotate its tip to the right around the superior vena cava/inferior vena cava axis, resulting in torsion of these large vessels and leading to a dramatic reduction of cardiac preload and thus cardiac output (116). On the left side, it involves protrusion and/or rotation of the left ventricular through the pericardial defect causing its strangulation (116).
Usually occurring within the first 24 h after surgery, a case report has described a sudden cardiac herniation up to 6 months after a right pneumonectomy (117). The only effective resuscitative treatment seems to be an emergency re-thoracotomy with the closure of the pericardial defect and restoration and fixation of the herniated heart to its normal position (118).
Cardiogenic shock related to neoplastic cardiac infiltration
Primary cardiac tumors are extremely rare, with an autopsy frequency ranging from 0.001 to 0.03% (104). Most of these primary lesions are usually benign (119) but can also be malignant, such as cardiac sarcoma, which accounts for ~2% of primary cardiac tumors (120). In contrast, cardiac metastases are slightly more common (121), with up to 12% of CPs having metastases to the heart or pericardium at autopsy, although most of them remain clinically silent (122, 123). Thus, only 1% of total extracardiac malignancies have clinically symptomatic cardiac involvement, mainly caused by melanoma, lymphoma, leukemia, and carcinoma of the lung, breast, and esophagus (121, 124). The pathophysiology includes a direct extension (e.g., lung carcinoma), hematogenous seeding (e.g., melanoma, lymphoma), venous extension (e.g., renal carcinoma), and retrograde lymphatic seeding (e.g., breast carcinoma) (119).
Although the literature is full of case reports and autopsy studies, the prevalence of cardiac infiltration leading to CS is unknown. The reference treatment for primary cardiac tumors remains cardiac resection surgery, if possible, with few exceptions (125). Resection is usually not indicated for secondary malignant cardiac tumors and treatment mainly relies on other anticancer therapies (126).
General issues for consideration regarding cardiogenic shock among cancer patients
Cancer and cardiovascular diseases are the leading causes of mortality worldwide. Evidence shows that these diseases have common risk factors, in an aging population, and are interconnected by adverse effects of cancer treatments on cardiovascular status (Table 5) (127). However, patients with cancer were excluded from most of the large cardiology studies and registries (128). Therefore, there is very little information on the impact of cancer in CS, even though data are emerging (5).
The occurrence of acute cardiovascular diseases in the cancer trajectory often causes interruption of potentially effective treatment, precluding completion of the therapy and influencing oncologic prognosis.
Core CS therapeutic principles do not differ substantially from non-CPs, even if there are some specificities such as those aforementioned. Complexity rather lies in the treatment intensity of CPs that can reasonably be implemented in the best interest of the patient.
According to a recent experts' review on critically ill oncology and hematology patients, no predefined criteria or prognostic scores of intensive care unit (ICU) or cardiac care unit triage for admission should be used (129). Each situation being different and challenging, the benefit-risk assessment must be discussed in an urgent multidisciplinary manner based on multiple criteria such as performance status (130) and frailty (131, 132) (Figure 2).
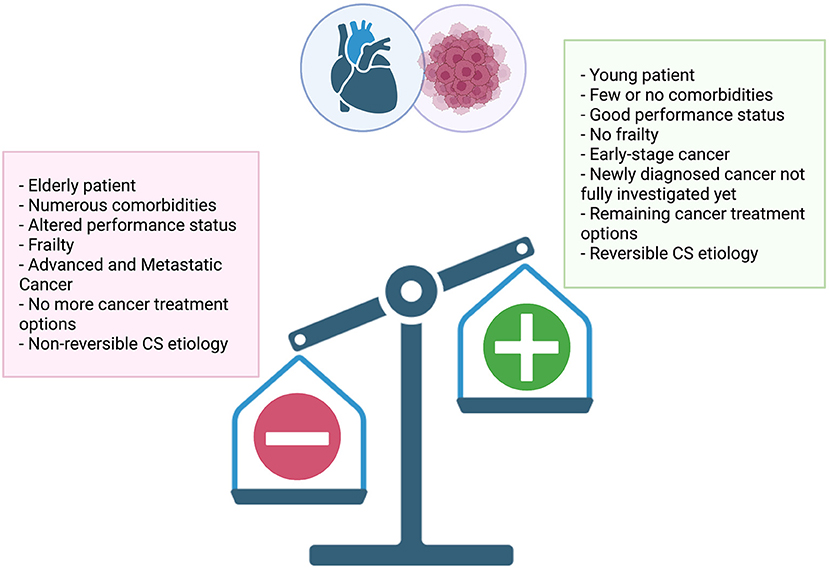
Figure 2. Multiple criteria to consider when assessing treatment intensity of cardiogenic shock in cancer patients.
Experts also suggest that time-limited trials should be used for CPs, meaning unlimited ICU management with a full-code status for a limited period before a re-evaluation of the clinical situation (129).
The appropriate length of time for full-code status (doing everything that can be done, including cancer chemotherapy and short-term MCS) seems to be 1 week in CPs with solid tumors. To be noted, in the case of multiple organ failure, full-code management for 4 to 5 days leads to similar outcomes as unlimited aggressive care (133). Full-code status seems to be at least 2 weeks in hematology patients unless they are in multiple organ failure, in which case 1 week would be enough to provide the same survival as with unlimited aggressive care (133).
For instance, a full code status should be warranted in CS cases of newly diagnosed malignancies, PE in CPs with good performance status and no frailty, acute cardiac toxicity after complete cancer remission, and clinical response undetermined or still unpredictable (59). If needed, short-term MCS for refractory CS should be implemented before the onset of multi-organ failure in selected patients as a strategy to buy time for cardiac recovery (bridge-to-recovery strategy) or bridge-to-other therapies (bridge-to-decision strategy) (134).
Although evidence for the potential effect of cardioprotective drug therapy in preventing or mitigating the cardiotoxic effects of cancer therapy is incomplete to date, these therapies may play an important role in the future (135, 136).
Conclusion
Cancer and cardiovascular diseases are the most prevalent diseases worldwide. Cardiogenic shock among cancer patients is an issue that can occur as a result of various causes and is likely to increase in the coming years. With improvements in cancer therapy and intensive care medicine, cardiogenic shock in cancer patients no longer means poor survival prognosis and initiation of palliative care. Rather, full code status with unlimited intensive care management (no restriction) is indeed worthwhile in some very speific situations. Multidisciplinary collaboration between intensivists, cardiologists, cardiac surgeons, and oncologists is essential in these critical situations.
Author contributions
AC, FM, and HM wrote the manuscript, reviewed, and edited the article before submission. CD, JG, LZ, and MS revised the manuscript. AC and HM created the figure with BioRenders.com subscribed to HM. All authors approved the final manuscript.
Conflict of interest
The authors declare that the research was conducted in the absence of any commercial or financial relationships that could be construed as a potential conflict of interest.
Publisher's note
All claims expressed in this article are solely those of the authors and do not necessarily represent those of their affiliated organizations, or those of the publisher, the editors and the reviewers. Any product that may be evaluated in this article, or claim that may be made by its manufacturer, is not guaranteed or endorsed by the publisher.
Abbreviations
5-FU, 5-fluoro-uracil; ACS, acute coronary syndrome; AFP, axial flow pump; CP, cancer patient; CS, cardiogenic shock; ESC, European Society of Cardiology; HER2, Human Epidermal Growth Factor Receptor-2; IABP, intra-aortic balloon pump; ICI, immune checkpoint inhibitors; ICOS, International Cardio-Oncology Society; InterTAK, International Takotsubo Registry; MCS, mechanical circulatory support; PE, pulmonary embolism; TKIs, tyrosine kinase inhibitors; TTS, Takotsubo syndrome; VA-ECMO, venoarterial-extracorporeal membrane oxygenation; VTE, venous thromboembolic events.
References
1. Dagenais GR, Leong DP, Rangarajan S, Lanas F, Lopez-Jaramillo P, Gupta R, et al. Variations in common diseases, hospital admissions, and deaths in middle-aged adults in 21 countries from five continents (pure): a prospective cohort study. Lancet. (2020) 395:785–94. doi: 10.1016/S0140-6736(19)32007-0
2. Santucci C, Carioli G, Bertuccio P, Malvezzi M, Pastorino U, Boffetta P, et al. Progress in cancer mortality, incidence, and survival: a global overview. Eur J Cancer Prev. (2020) 29:367–81. doi: 10.1097/CEJ.0000000000000594
3. Siegel RL, Miller KD, Fuchs HE, Jemal A. Cancer statistics, 2022. CA Cancer J Clin. (2022) 72:7–33. doi: 10.3322/caac.21708
4. Koene RJ, Prizment AE, Blaes A, Konety SH. Shared risk factors in cardiovascular disease and cancer. Circulation. (2016) 133:1104–14. doi: 10.1161/CIRCULATIONAHA.115.020406
5. Stoltzfus KC, Zhang Y, Sturgeon K, Sinoway LI, Trifiletti DM, Chinchilli VM, et al. Fatal heart disease among cancer patients. Nat Commun. (2020) 11:2011. doi: 10.1038/s41467-020-15639-5
6. de Boer RA, Aboumsallem JP, Bracun V, Leedy D, Cheng R, Patel S, et al. A new classification of cardio-oncology syndromes. Cardiooncology. (2021) 7:24. doi: 10.1186/s40959-021-00110-1
7. Pretre V, Papadopoulos D, Regard J, Pelletier M, Woo J. Interleukin-1 (Il-1) and the Inflammasome in Cancer. Cytokine. (2022) 153:155850. doi: 10.1016/j.cyto.2022.155850
8. Cardinale D, Colombo A, Lamantia G, Colombo N, Civelli M, De Giacomi G, et al. Cardio-oncology: a new medical issue. Ecancermedicalscience. (2008) 2:126. doi: 10.3332/ecancer.2008.126
9. Lancellotti P, Suter TM, Lopez-Fernandez T, Galderisi M, Lyon AR, Van der Meer P, et al. Cardio-oncology services: rationale, organization, and implementation. Eur Heart J. (2019) 40:1756–63. doi: 10.1093/eurheartj/ehy453
10. Reyentovich A, Barghash MH, Hochman JS. Management of refractory cardiogenic shock. Nat Rev Cardiol. (2016) 13:481–92. doi: 10.1038/nrcardio.2016.96
11. Tehrani BN, Truesdell AG, Psotka MA, Rosner C, Singh R, Sinha SS, et al. A standardized and comprehensive approach to the management of cardiogenic shock. JACC Heart Fail. (2020) 8:879–91. doi: 10.1016/j.jchf.2020.09.005
12. Arrigo M, Jessup M, Mullens W, Reza N, Shah AM, Sliwa K, et al. Acute heart failure. Nat Rev Dis Primers. (2020) 6:16. doi: 10.1038/s41572-020-0151-7
13. Gevaert SA, Halvorsen S, Sinnaeve PR, Sambola A, Gulati G, Lancellotti P, et al. Evaluation and management of cancer patients presenting with acute cardiovascular disease: a consensus document of the acute cardiovascular care (ACVC) association and the esc council of cardio-oncology-part 1: acute coronary syndromes and acute pericardial diseases. Eur Heart J Acute Cardiovasc Care. (2021) 10:947–59. doi: 10.1093/ehjacc/zuab056
14. Delmas C, Roubille F, Lamblin N, Bonello L, Leurent G, Levy B, et al. Baseline characteristics, management, and predictors of early mortality in cardiogenic shock: insights from the frenshock registry. ESC Heart Fail. (2022) 9:408–19. doi: 10.1002/ehf2.13734
15. Shaefi S, O'Gara B, Kociol RD, Joynt K, Mueller A, Nizamuddin J, et al. Effect of cardiogenic shock hospital volume on mortality in patients with cardiogenic shock. J Am Heart Assoc. (2015) 4:e001462. doi: 10.1161/JAHA.114.001462
16. Rodriguez M, Sabharwal B, Wei X, Krittanawong C, Verheyen E, Herzog E. The effect of cancer on outcomes of acute heart failure exacerbations: a 5-year nationwide analysis. J Am Coll Cardiol. (2018) 71:A693–A. doi: 10.1016/S0735-1097(18)31234-8
17. Bharadwaj A, Potts J, Mohamed MO, Parwani P, Swamy P, Lopez-Mattei JC, et al. Acute myocardial infarction treatments and outcomes in 6=.5 million patients with a current or historical diagnosis of cancer in the USA. Eur Heart J. (2020) 41:2183–93. doi: 10.1093/eurheartj/ehz851
18. Navi BB, Reiner AS, Kamel H, Iadecola C, Okin PM, Elkind MSV, et al. Risk of arterial thromboembolism in patients with Cancer. J Am Coll Cardiol. (2017) 70:926–38. doi: 10.1016/j.jacc.2017.06.047
19. Anand AJ. Fluorouracil cardiotoxicity. Ann Pharmacother. (1994) 28:374–8. doi: 10.1177/106002809402800314
20. Herrmann J, Yang EH, Iliescu CA, Cilingiroglu M, Charitakis K, Hakeem A, et al. Vascular toxicities of cancer therapies: the old and the new–an evolving avenue. Circulation. (2016) 133:1272–89. doi: 10.1161/CIRCULATIONAHA.115.018347
21. Costa I, Andrade FTA, Carter D, Seleme VB, Costa MS, Campos CM, et al. Challenges and management of acute coronary syndrome in cancer patients. Front Cardiovasc Med. (2021) 8:590016. doi: 10.3389/fcvm.2021.590016
22. Desai MY, Jellis CL, Kotecha R, Johnston DR, Griffin BP. Radiation-associated cardiac disease: a practical approach to diagnosis and management. JACC Cardiovasc Imaging. (2018) 11:1132–49. doi: 10.1016/j.jcmg.2018.04.028
23. Belzile-Dugas E, Eisenberg MJ. Radiation-induced cardiovascular disease: review of an underrecognized pathology. J Am Heart Assoc. (2021) 10:e021686. doi: 10.1161/JAHA.121.021686
24. Landes U, Kornowski R, Bental T, Assali A, Vaknin-Assa H, Lev E, et al. Long-term outcomes after percutaneous coronary interventions in cancer survivors. Coron Artery Dis. (2017) 28:5–10. doi: 10.1097/MCA.0000000000000429
25. Nakatsuma K, Shiomi H, Morimoto T, Watanabe H, Nakagawa Y, Furukawa Y, et al. Influence of a history of cancer on long-term cardiovascular outcomes after coronary stent implantation (an observation from coronary revascularization demonstrating outcome study-kyoto registry cohort-2). Eur Heart J Qual Care Clin Outcomes. (2018) 4:200–7. doi: 10.1093/ehjqcco/qcy014
26. Lange SA, Feld J, Kuhnemund L, Koppe J, Makowski L, Engelbertz CM, et al. Acute and long-term outcomes of st-elevation myocardial infarction in cancer patients, a 'real world' analysis with 175,000 patients. Cancers (Basel). (2021) 13(24). doi: 10.3390/cancers13246203
27. Peng X, Wang Z, Cao M, Zheng Y, Tian Y, Yu L, et al. A concomitant cancer diagnosis is associated with poor cardiovascular outcomes among acute myocardial infarction patients. Front Cardiovasc Med. (2022) 9:758324. doi: 10.3389/fcvm.2022.758324
28. Madeddu C, Gramignano G, Astara G, Demontis R, Sanna E, Atzeni V, et al. Pathogenesis and treatment options of cancer related anemia: perspective for a targeted mechanism-based approach. Front Physiol. (2018) 9:1294. doi: 10.3389/fphys.2018.01294
29. Al-Hawwas M, Tsitlakidou D, Gupta N, Iliescu C, Cilingiroglu M, Marmagkiolis K. Acute coronary syndrome management in cancer patients. Curr Oncol Rep. (2018) 20:78. doi: 10.1007/s11912-018-0724-8
30. Sarfati D, Koczwara B, Jackson C. the impact of comorbidity on cancer and its treatment. CA Cancer J Clin. (2016) 66:337–50. doi: 10.3322/caac.21342
31. Liebman HA. Thrombocytopenia in cancer patients. Thromb Res. (2014) 133 Suppl 2:S63–9. doi: 10.1016/S0049-3848(14)50011-4
32. Biedka M, Ziolkowska E, Windorbska W. Acute pulmonary embolus in the course of cancer. Contemp Oncol (Pozn). (2012) 16:388–93. doi: 10.5114/wo.2012.31766
33. Blom JW, Doggen CJ, Osanto S, Rosendaal FR. Malignancies, prothrombotic mutations, and the risk of venous thrombosis. JAMA. (2005) 293:715–22. doi: 10.1001/jama.293.6.715
34. Khorana AA, Francis CW, Culakova E, Kuderer NM, Lyman GH. Thromboembolism is a leading cause of death in cancer patients receiving outpatient chemotherapy. J Thromb Haemost. (2007) 5:632–4. doi: 10.1111/j.1538-7836.2007.02374.x
35. Sorensen HT, Mellemkjaer L, Olsen JH, Baron JA. Prognosis of cancers associated with venous thromboembolism. N Engl J Med. (2000) 343:1846–50. doi: 10.1056/NEJM200012213432504
36. Paneesha S, McManus A, Arya R, Scriven N, Farren T, Nokes T, et al. Frequency, demographics and risk (according to tumour type or site) of cancer-associated thrombosis among patients seen at outpatient dvt clinics. Thromb Haemost. (2010) 103:338–43. doi: 10.1160/TH09-06-0397
37. Mahe I, Chidiac J, Bertoletti L, Font C, Trujillo-Santos J, Peris M, et al. The clinical course of venous thromboembolism may differ according to cancer site. Am J Med. (2017) 130:337–47. doi: 10.1016/j.amjmed.2016.10.017
38. Khorana AA, Mackman N, Falanga A, Pabinger I, Noble S, Ageno W, et al. Cancer-associated venous thromboembolism. Nat Rev Dis Primers. (2022) 8:11. doi: 10.1038/s41572-022-00336-y
39. Falanga A, Marchetti M. Anticancer treatment and thrombosis. Thromb Res. (2012) 129:353–9. doi: 10.1016/j.thromres.2011.10.025
40. Junjun L, Pei W, Ying Y, Kui S. Prognosis and risk factors in older patients with lung cancer and pulmonary embolism: a propensity score matching analysis. Sci Rep. (2020) 10:1272. doi: 10.1038/s41598-020-58345-4
41. Bois MC, Eckhardt MD, Cracolici VM, Loe MJ, Ocel JJ, Edwards WD, et al. Neoplastic embolization to systemic and pulmonary arteries. J Vasc Surg. (2018) 68:204–12 e7. doi: 10.1016/j.jvs.2017.09.045
42. Mury C, Schneider AG, Nobile A, Rotman S, Liaudet L. Acute pulmonary hypertension caused by tumor embolism: a report of two cases. Pulm Circ. (2015) 5:577–9. doi: 10.1086/682225
43. Steiner S, Plehn G, Reinecke P, Cohnen M, Schwartzkopff B, Hennersdorf MG, et al. Disseminated microvascular pulmonary tumor cell embolism: a rare cause of fulminant pulmonary hypertension. Onkologie. (2004) 27:566–8. doi: 10.1159/000081340
44. Shalaby K, Kahn A, Silver ES, Kim MJ, Balakumaran K, Kim AS. Outcomes of acute pulmonary embolism in hospitalized patients with cancer. BMC Pulm Med. (2022) 22:11. doi: 10.1186/s12890-021-01808-9
45. Au C, Gupta E, Khaing P, Dibello J, Chengsupanimit T, Mitchell EP, et al. Clinical presentations and outcomes in pulmonary embolism patients with cancer. J Thromb Thrombolysis. (2021) 51:430–6. doi: 10.1007/s11239-020-02298-y
46. Konstantinides SV, Meyer G, Becattini C, Bueno H, Geersing GJ, Harjola VP, et al. 2019 Esc guidelines for the diagnosis and management of acute pulmonary embolism developed in collaboration with the european respiratory society (Ers). Eur Heart J. (2020) 41:543–603. doi: 10.1093/eurheartj/ehz405
47. Casazza F, Becattini C, Rulli E, Pacchetti I, Floriani I, Biancardi M, et al. Clinical presentation and in-hospital death in acute pulmonary embolism: does cancer matter? Intern Emerg Med. (2016) 11:817–24. doi: 10.1007/s11739-016-1431-9
48. Weeda ER, Hakamiun KM, Leschorn HX, Tran E. Comorbid cancer and use of thrombolysis in acute pulmonary embolism. J Thromb Thrombolysis. (2019) 47:324–7. doi: 10.1007/s11239-018-1772-5
49. Waqas Ullah AC, Mohamed Z, Chaitu D, Heba O, Rashid A, Parveen R, et al. Outcomes of cancer patients with pulmonary embolism treated with thrombolysis therapy or thrombectomy. J Am Coll Cardiol. (2022) 22:3. doi: 10.1016/S0735-1097(22)01592-3
50. Delmas C, Aissaoui N, Meneveau N, Bouvaist H, Rousseau H, Puymirat E, et al. Reperfusion therapies in pulmonary embolism-state of the art and expert opinion: a position paper from the “unite de soins intensifs de cardiologie” group of the french society of cardiology. Arch Cardiovasc Dis. (2020) 113:749–59. doi: 10.1016/j.acvd.2020.06.002
51. Kearon C. Natural history of venous thromboembolism. Circulation. (2003) 107:I22–30. doi: 10.1161/01.CIR.0000078464.82671.78
52. Alotaibi G, Wu C, Senthilselvan A, McMurtry MS. Short- and long-term mortality after pulmonary embolism in patients with and without cancer. Vasc Med. (2018) 23:261–6. doi: 10.1177/1358863X18754692
53. Grilz E, Konigsbrugge O, Posch F, Schmidinger M, Pirker R, Lang IM, et al. Frequency, risk factors, and impact on mortality of arterial thromboembolism in patients with cancer. Haematologica. (2018) 103:1549–56. doi: 10.3324/haematol.2018.192419
54. De Stefano V. Arterial thrombosis and cancer: the neglected side of the coin of trousseau syndrome. Haematologica. (2018) 103:1419–21. doi: 10.3324/haematol.2018.197814
55. Curigliano G, Lenihan D, Fradley M, Ganatra S, Barac A, Blaes A, et al. Management of cardiac disease in cancer patients throughout oncological treatment: esmo consensus recommendations. Ann Oncol. (2020) 31:171–90. doi: 10.1016/j.annonc.2019.10.023
56. Zamorano JL, Lancellotti P, Rodriguez Munoz D, Aboyans V, Asteggiano R, Galderisi M, et al. 2016 Esc position paper on cancer treatments and cardiovascular toxicity developed under the auspices of the esc committee for practice guidelines: the task force for cancer treatments and cardiovascular toxicity of the european society of cardiology (Esc). Eur Heart J. (2016) 37:2768–801. doi: 10.1093/eurheartj/ehw211
57. Armenian SH, Lacchetti C, Barac A, Carver J, Constine LS, Denduluri N, et al. Prevention and monitoring of cardiac dysfunction in survivors of adult cancers: american society of clinical oncology clinical practice guideline. J Clin Oncol. (2017) 35:893–911. doi: 10.1200/JCO.2016.70.5400
58. Alexandre J, Cautela J, Ederhy S, Damaj GL, Salem JE, Barlesi F, et al. Cardiovascular toxicity related to cancer treatment: a pragmatic approach to the american and european cardio-oncology guidelines. J Am Heart Assoc. (2020) 9:e018403. doi: 10.1161/JAHA.120.018403
59. Montisci A, Palmieri V, Liu JE, Vietri MT, Cirri S, Donatelli F, et al. Severe cardiac toxicity induced by cancer therapies requiring intensive care unit admission. Front Cardiovasc Med. (2021) 8:713694. doi: 10.3389/fcvm.2021.713694
60. Cardinale D, Colombo A, Bacchiani G, Tedeschi I, Meroni CA, Veglia F, et al. Early detection of anthracycline cardiotoxicity and improvement with heart failure therapy. Circulation. (2015) 131:1981–8. doi: 10.1161/CIRCULATIONAHA.114.013777
61. Cardinale D, Iacopo F, Cipolla CM. Cardiotoxicity of anthracyclines. Front Cardiovasc Med. (2020) 7:26. doi: 10.3389/fcvm.2020.00026
62. Murabito A, Hirsch E, Ghigo A. Mechanisms of anthracycline-induced cardiotoxicity: is mitochondrial dysfunction the answer? Front Cardiovasc Med. (2020) 7:35. doi: 10.3389/fcvm.2020.00035
63. Quagliariello V, De Laurentiis M, Rea D, Barbieri A, Monti MG, Carbone A, et al. The sglt-2 inhibitor empagliflozin improves myocardial strain, reduces cardiac fibrosis and pro-inflammatory cytokines in non-diabetic mice treated with doxorubicin. Cardiovasc Diabetol. (2021) 20:150. doi: 10.1186/s12933-021-01346-y
64. Binaghi G, Congia D, Cossa S, Massidda S, Pasqualucci D, Pilo F, et al. Cardiogenic shock during first infusion of anthracycline chemotherapy in a patient with hodgkin lymphoma: an unusual event. Cardiology. (2018) 139:7–10. doi: 10.1159/000480291
65. Freilich M, Stub D, Esmore D, Negri J, Salamonsen R, Bergin P, et al. Recovery from anthracycline cardiomyopathy after long-term support with a continuous flow left ventricular assist device. J Heart Lung Transplant. (2009) 28:101–3. doi: 10.1016/j.healun.2008.10.002
66. Takami Y, Hoshino N, Kato Y, Sakurai Y, Amano K, Higuchi Y, et al. Recovery from anthracycline-induced cardiomyopathy with biventricular assist and valve repairs: a case report and literature review. Int J Artif Organs. (2018) 41:413–7. doi: 10.1177/0391398818772497
67. Dent SF, Morse A, Burnette S, Guha A, Moore H. Cardiovascular toxicity of novel her2-targeted therapies in the treatment of breast cancer. Curr Oncol Rep. (2021) 23:128. doi: 10.1007/s11912-021-01114-x
68. Stone JR, Kanneganti R, Abbasi M, Akhtari M. Monitoring for chemotherapy-related cardiotoxicity in the form of left ventricular systolic dysfunction: a review of current recommendations. JCO Oncol Pract. (2021) 17:228–36. doi: 10.1200/OP.20.00924
69. Tschope C, Ammirati E, Bozkurt B, Caforio ALP, Cooper LT, Felix SB, et al. Myocarditis and inflammatory cardiomyopathy: current evidence and future directions. Nat Rev Cardiol. (2021) 18:169–93. doi: 10.1038/s41569-020-00435-x
70. Sasson Z, Morgan CD, Wang B, Thomas G, MacKenzie B, Platts ME. 5-fluorouracil related toxic myocarditis: case reports and pathological confirmation. Can J Cardiol. (1994) 10:861–4.
71. Gottdiener JS, Appelbaum FR, Ferrans VJ, Deisseroth A, Ziegler J. Cardiotoxicity associated with high-dose cyclophosphamide therapy. Arch Intern Med. (1981) 141:758–63. doi: 10.1001/archinte.1981.00340060066015
72. Mahmood SS, Fradley MG, Cohen JV, Nohria A, Reynolds KL, Heinzerling LM, et al. Myocarditis in patients treated with immune checkpoint inhibitors. J Am Coll Cardiol. (2018) 71:1755–64. doi: 10.1016/j.jacc.2018.02.037
73. Makunts T, Saunders IM, Cohen IV, Li M, Moumedjian T, Issa MA, et al. Myocarditis occurrence with cancer immunotherapy across indications in clinical trial and post-marketing data. Sci Rep. (2021) 11:17324. doi: 10.1038/s41598-021-96467-5
74. Moslehi J, Lichtman AH, Sharpe AH, Galluzzi L, Kitsis RN. Immune checkpoint inhibitor-associated myocarditis: manifestations and mechanisms. J Clin Invest. (2021) 131:5. doi: 10.1172/JCI145186
75. Johnson DB, Balko JM, Compton ML, Chalkias S, Gorham J, Xu Y, et al. Fulminant myocarditis with combination immune checkpoint blockade. N Engl J Med. (2016) 375:1749–55. doi: 10.1056/NEJMoa1609214
76. Santos JRU, Brofferio A, Viana B, Pacak K. Catecholamine-induced cardiomyopathy in pheochromocytoma: how to manage a rare complication in a rare disease? Horm Metab Res. (2019) 51:458–69. doi: 10.1055/a-0669-9556
77. Hekimian G, Kharcha F, Brechot N, Schmidt M, Ghander C, Lebreton G, et al. Extracorporeal membrane oxygenation for pheochromocytoma-induced cardiogenic shock. Ann Intensive Care. (2016) 6:117. doi: 10.1186/s13613-016-0219-4
78. Laufs H, Nigrovic PA, Schneider LC, Oettgen H, Del NP, Moskowitz IP, et al. Giant cell myocarditis in a 12-year-old girl with common variable immunodeficiency. Mayo Clin Proc. (2002) 77:92–6. doi: 10.4065/77.1.92
79. Cheung CC, Constantine M, Ahmadi A, Shiau C, Chen LYC. Eosinophilic myocarditis. Am J Med Sci. (2017) 354:486–92. doi: 10.1016/j.amjms.2017.04.002
80. S YH, Falhammar H. Pheochromocytoma- and paraganglioma-triggered takotsubo syndrome. Endocrine. (2019) 65:483–93. doi: 10.1007/s12020-019-02035-3
81. Templin C, Ghadri JR, Diekmann J, Napp LC, Bataiosu DR, Jaguszewski M, et al. Clinical features and outcomes of takotsubo (stress) cardiomyopathy. N Engl J Med. (2015) 373:929–38. doi: 10.1056/NEJMoa1406761
82. Pelliccia F, Kaski JC, Crea F, Camici PG. Pathophysiology of Takotsubo Syndrome. Circulation. (2017) 135:2426–41. doi: 10.1161/CIRCULATIONAHA.116.027121
83. Ghadri JR, Wittstein IS, Prasad A, Sharkey S, Dote K, Akashi YJ, et al. International expert consensus document on Takotsubo Syndrome (Part Ii): diagnostic workup, outcome, and management. Eur Heart J. (2018) 39:2047–62. doi: 10.1093/eurheartj/ehy077
84. Carbone A, Bottino R, Russo V, D'Andrea A, Liccardo B, Maurea N, et al. Takotsubo cardiomyopathy as epiphenomenon of cardiotoxicity in patients with cancer: a meta-summary of case reports. J Cardiovasc Pharmacol. (2021) 78:e20–e9. doi: 10.1097/FJC.0000000000001026
85. Sattler K, El-Battrawy I, Lang S, Zhou X, Schramm K, Tulumen E, et al. Prevalence of cancer in takotsubo cardiomyopathy: short and long-term outcome. Int J Cardiol. (2017) 238:159–65. doi: 10.1016/j.ijcard.2017.02.093
86. Burgdorf C, Kurowski V, Bonnemeier H, Schunkert H, Radke PW. Long-term prognosis of the transient left ventricular dysfunction syndrome (tako-tsubo cardiomyopathy): focus on malignancies. Eur J Heart Fail. (2008) 10:1015–9. doi: 10.1016/j.ejheart.2008.07.008
87. Nef HM, Mollmann H, Hilpert P, Troidl C, Voss S, Rolf A, et al. Activated cell survival cascade protects cardiomyocytes from cell death in tako-tsubo cardiomyopathy. Eur J Heart Fail. (2009) 11:758–64. doi: 10.1093/eurjhf/hfp076
88. Citro R, d'Avenia M, De Marco M, Giudice R, Mirra M, Ravera A, et al. Polymorphisms of the antiapoptotic protein bag3 may play a role in the pathogenesis of tako-tsubo cardiomyopathy. Int J Cardiol. (2013) 168:1663–5. doi: 10.1016/j.ijcard.2013.03.050
89. Brunetti ND, Tarantino N, Guastafierro F, De Gennaro L, Correale M, Stiermaier T, et al. Malignancies and outcome in takotsubo syndrome: a meta-analysis study on cancer and stress cardiomyopathy. Heart Fail Rev. (2019) 24:481–8. doi: 10.1007/s10741-019-09773-6
90. Cammann VL, Sarcon A, Ding KJ, Seifert B, Kato K, Di Vece D, et al. Clinical features and outcomes of patients with malignancy and takotsubo syndrome: observations from the international takotsubo registry. J Am Heart Assoc. (2019) 8:e010881. doi: 10.1161/JAHA.118.010881
91. Joy PS, Guddati AK, Shapira I. Outcomes of Takotsubo Cardiomyopathy in hospitalized cancer patients. J Cancer Res Clin Oncol. (2018) 144:1539–45. doi: 10.1007/s00432-018-2661-1
92. Zaghlol R, Kashyap K, Al-Shbool G, Basyal B, Desale S, Campia U, et al. Usefulness of malignancy as a predictor of worsein-hospital outcomes in patients with takotsubo cardiomyopathy. Am J Cardiol. (2019) 123:995–1001. doi: 10.1016/j.amjcard.2018.11.054
93. Desai R, Desai A, Abbas SA, Patel U, Bansod S, Damarlapally N, et al. National prevalence, trends and outcomes of takotsubo syndrome in hospitalizations with prior history of mediastinal/intrathoracic cancer and radiation therapy. Int J Cardiol. (2020) 309:14–8. doi: 10.1016/j.ijcard.2020.02.036
94. Nguyen TH, Stansborough J, Ong GJ, Surikow S, Price TJ, Horowitz JD. Antecedent cancer in takotsubo syndrome predicts both cardiovascular and long-term mortality. Cardiooncology. (2019) 5:20. doi: 10.1186/s40959-019-0053-6
95. Giza DE, Lopez-Mattei J, Vejpongsa P, Munoz E, Iliescu G, Kitkungvan D, et al. Stress-induced cardiomyopathy in cancer patients. Am J Cardiol. (2017) 120:2284–8. doi: 10.1016/j.amjcard.2017.09.009
96. Madias JE. What is/are the trigger(s) of takotsubo syndrome in cancer patients receiving chemotherapy? Int J Cardiol. (2016) 222:253. doi: 10.1016/j.ijcard.2016.07.246
97. Desai A, Noor A, Joshi S, Kim AS. Takotsubo cardiomyopathy in cancer patients. Cardiooncology. (2019) 5:7. doi: 10.1186/s40959-019-0042-9
98. Templin C, Ghadri JR, Napp LC. Takotsubo (Stress) cardiomyopathy. N Engl J Med. (2015) 373:2689–91. doi: 10.1056/NEJMc1512595
99. De Backer O, Debonnaire P, Gevaert S, Missault L, Gheeraert P, Muyldermans L. Prevalence, associated factors and management implications of left ventricular outflow tract obstruction in takotsubo cardiomyopathy: a two-year, two-center experience. BMC Cardiovasc Disord. (2014) 14:147. doi: 10.1186/1471-2261-14-147
100. Amar J, Brunel J, Cardot Bauters C, Jacques V, Delmas C, Odou MF, et al. Genetic biomarkers of life-threatening pheochromocytoma-induced cardiomyopathy. Endocr Relat Cancer. (2022) doi: 10.1530/ERC-21-0373
101. El Haddad D, Iliescu C, Yusuf SW, William WN Jr, Khair TH, et al. Outcomes of cancer patients undergoing percutaneous pericardiocentesis for pericardial effusion. J Am Coll Cardiol. (2015) 66:1119–28. doi: 10.1016/j.jacc.2015.06.1332
102. Jama GM, Scarci M, Bowden J, Marciniak SJ. Palliative treatment for symptomatic malignant pericardial effusiondagger. Interact Cardiovasc Thorac Surg. (2014) 19:1019–26. doi: 10.1093/icvts/ivu267
103. Jeon HW, Cho DG, Park JK, Hyun KY, Choi SY, Suh JH, et al. Prognostic factors affecting survival of patients with cancer-related pericardial effusion managed by surgery. World J Surg Oncol. (2014) 12:249. doi: 10.1186/1477-7819-12-249
104. Burazor I, Aviel-Ronen S, Imazio M, Markel G, Grossman Y, Yosepovich A, et al. Primary malignancies of the heart and pericardium. Clin Cardiol. (2014) 37:582–8. doi: 10.1002/clc.22295
105. Takata N, Kataoka M, Hamamoto Y, Tsuruoka S, Kanzaki H, Uwatsu K, et al. Risk factors for pericardial effusion after chemoradiotherapy for thoracic esophageal cancer-comparison of four-field technique and traditional two opposed fields technique. J Radiat Res. (2018) 59:291–7. doi: 10.1093/jrr/rry029
106. Ghosh AK, Crake T, Manisty C, Westwood M. Pericardial disease in cancer patients. Curr Treat Options Cardiovasc Med. (2018) 20:60. doi: 10.1007/s11936-018-0654-7
107. Ala CK, Klein AL, Moslehi JJ. Cancer treatment-associated pericardial disease: epidemiology, clinical presentation, diagnosis, and management. Curr Cardiol Rep. (2019) 21:156. doi: 10.1007/s11886-019-1225-6
108. Adler Y, Charron P, Imazio M, Badano L, Baron-Esquivias G, Bogaert J, et al. 2015 Esc guidelines for the diagnosis and management of pericardial diseases: the task force for the diagnosis and management of pericardial diseases of the european society of cardiology (Esc)endorsed by: the european association for cardio-thoracic surgery (Eacts). Eur Heart J. (2015) 36:2921–64. doi: 10.1093/eurheartj/ehv318
109. Salem JE, Manouchehri A, Moey M, Lebrun-Vignes B, Bastarache L, Pariente A, et al. Cardiovascular toxicities associated with immune checkpoint inhibitors: an observational, retrospective, pharmacovigilance study. Lancet Oncol. (2018) 19:1579–89. doi: 10.1016/S1470-2045(18)30608-9
110. Kim KH, Miranda WR, Sinak LJ, Syed FF, Melduni RM, Espinosa RE, et al. Effusive-constrictive pericarditis after pericardiocentesis: incidence, associated findings, and natural history. JACC Cardiovasc Imaging. (2018) 11:534–41. doi: 10.1016/j.jcmg.2017.06.017
111. Pradhan R, Okabe T, Yoshida K, Angouras DC, DeCaro MV, Marhefka GD. Patient characteristics and predictors of mortality associated with pericardial decompression syndrome: a comprehensive analysis of published cases. Eur Heart J Acute Cardiovasc Care. (2015) 4:113–20. doi: 10.1177/2048872614547975
112. Veronesi G, Spaggiari L, Solli PG, Pastorino U. Cardiac dislocation after extended pneumonectomy with pericardioplasty. Eur J Cardiothorac Surg. (2001) 19:89–91. doi: 10.1016/S1010-7940(00)00612-6
113. Bettman RB, Tannenbaum WJ. Herniation of the heart: through a pericardial incision. Ann Surg. (1948) 128:1012–4. doi: 10.1097/00000658-194811000-00021
114. Scagliola R, Seitun S, Rosa GM. Cardiac herniation: a practical review in the emergency setting. Am J Emerg Med. (2022) 53:222–7. doi: 10.1016/j.ajem.2022.01.017
115. Alimi F, Marzouk M, Mgarrech I, Chemchik H, Limayem F. Cardiac herniation after left intrapericardial pneumonectomy. Asian Cardiovasc Thorac Ann. (2016) 24:590–2. doi: 10.1177/0218492315589199
116. Schummer W. Cardiac herniation with torsion after right pneumonectomy. Indian J Crit Care Med. (2017) 21:473–4. doi: 10.4103/ijccm.IJCCM_60_17
117. Zandberg FT, Verbeke SJ, Snijder RJ, Dalinghaus WH, Roeffel SM, Van Swieten HA. Sudden cardiac herniation 6 months after right pneumonectomy. Ann Thorac Surg. (2004) 78:1095–7. doi: 10.1016/S0003-4975(03)01404-8
118. Cassorla L, Katz JA. Management of cardiac herniation after intrapericardial pneumonectomy. Anesthesiology. (1984) 60:362–4. doi: 10.1097/00000542-198404000-00016
119. Maleszewski JJ, Bois MC, Bois JP, Young PM, Stulak JM, Klarich KW. Neoplasia and the heart: pathological review of effects with clinical and radiological correlation. J Am Coll Cardiol. (2018) 72:202–27. doi: 10.1016/j.jacc.2018.05.026
120. Dergel M, Gofus J, Smolak P, Stejskal V, Hanke I, Matejka J, et al. Surgical treatment of primary cardiac tumors: 20-year single center experience. Kardiochir Torakochirurgia Pol. (2022) 19:36–40. doi: 10.5114/kitp.2022.114553
121. Burazor I, Aviel-Ronen S, Imazio M, Goitein O, Perelman M, Shelestovich N, et al. Metastatic cardiac tumors: from clinical presentation through diagnosis to treatment. BMC Cancer. (2018) 18:202. doi: 10.1186/s12885-018-4070-x
122. Klatt EC, Heitz DR. Cardiac metastases. Cancer. (1990) 65(6):1456-9. doi: 10.1002/1097-0142(19900315)65:6<1456::aid-cncr2820650634>3.0.co;2-5
123. Gupta R, Meghrajani V, Desai R, Gupta N. Primary malignant cardiac tumors: a rare disease with an adventurous journey. J Am Heart Assoc. (2020) 9:e016032. doi: 10.1161/JAHA.120.016032
124. Roberts WC. Primary and secondary neoplasms of the heart. Am J Cardiol. (1997) 80:671–82. doi: 10.1016/S0002-9149(97)00587-0
125. Bussani R, Castrichini M, Restivo L, Fabris E, Porcari A, Ferro F, et al. Cardiac tumors: diagnosis, prognosis, and treatment. Curr Cardiol Rep. (2020) 22:169. doi: 10.1007/s11886-020-01420-z
126. Tyebally S, Chen D, Bhattacharyya S, Mughrabi A, Hussain Z, Manisty C, et al. Cardiac tumors: jacc cardiooncology state-of-the-art review. JACC CardioOncol. (2020) 2:293–311. doi: 10.1016/j.jaccao.2020.05.009
127. Paterson DI, Wiebe N, Cheung WY, Mackey JR, Pituskin E, Reiman A, et al. Incident cardiovascular disease among adults with cancer. JACC: CardioOncology. (2022) 4:85–94. doi: 10.1016/j.jaccao.2022.01.100
128. Bonsu J, Charles L, Guha A, Awan F, Woyach J, Yildiz V, et al. Representation of patients with cardiovascular disease in pivotal cancer clinical trials. Circulation. (2019) 139:2594–6. doi: 10.1161/CIRCULATIONAHA.118.039180
129. Azoulay E, Schellongowski P, Darmon M, Bauer PR, Benoit D, Depuydt P, et al. The intensive care medicine research agenda on critically ill oncology and hematology patients. Intensive Care Med. (2017) 43:1366–82. doi: 10.1007/s00134-017-4884-z
130. Zampieri FG, Bozza FA, Moralez GM, Mazza DD, Scotti AV, Santino MS, et al. The effects of performance status one week before hospital admission on the outcomes of critically ill patients. Intensive Care Med. (2017) 43:39–47. doi: 10.1007/s00134-016-4563-5
131. Darvall JN, Bellomo R, Bailey M, Young PJ, Rockwood K, Pilcher D. Impact of frailty on persistent critical illness: a population-based cohort study. Intensive Care Med. (2022) 48:343–51. doi: 10.1007/s00134-022-06617-0
132. Volle K, Delmas C, Ferrieres J, Toulza O, Blanco S, Lairez O, et al. Prevalence and prognosis impact of frailty among older adults in cardiac intensive care units. CJC Open. (2021) 3:1010–8. doi: 10.1016/j.cjco.2021.03.009
133. Shrime MG, Ferket BS, Scott DJ, Lee J, Barragan-Bradford D, Pollard T, et al. Time-Limited trials of intensive care for critically ill patients with cancer: how long is long enough? JAMA Oncol. (2016) 2:76–83. doi: 10.1001/jamaoncol.2015.3336
134. Chieffo A, Dudek D, Hassager C, Combes A, Gramegna M, Halvorsen S, et al. Joint eapci/acvc expert consensus document on percutaneous ventricular assist devices. Eur Heart J Acute Cardiovasc Care. (2021) 10:570–83. doi: 10.1093/ehjacc/zuab015
135. Omland T, Heck SL, Gulati G. The role of cardioprotection in cancer therapy cardiotoxicity: jacc: cardiooncology state-of-the-art review. JACC CardioOncol. (2022) 4:19–37. doi: 10.1016/j.jaccao.2022.01.101
Keywords: heart failure, cardiogenic shock, cancer patient, cardio-oncology, cancer therapy
Citation: Curtiaud A, Delmas C, Gantzer J, Zafrani L, Siegemund M, Meziani F and Merdji H (2022) Cardiogenic shock among cancer patients. Front. Cardiovasc. Med. 9:932400. doi: 10.3389/fcvm.2022.932400
Received: 29 April 2022; Accepted: 04 July 2022;
Published: 22 August 2022.
Edited by:
Canan G. Nebigil, INSERM U1260 Nanomedicine Régénératrice (RNM), FranceReviewed by:
Kalliopi Keramida, National and Kapodistrian University of Athens, GreeceVincenzo Quagliariello, G. Pascale National Cancer Institute Foundation (IRCCS), Italy
Copyright © 2022 Curtiaud, Delmas, Gantzer, Zafrani, Siegemund, Meziani and Merdji. This is an open-access article distributed under the terms of the Creative Commons Attribution License (CC BY). The use, distribution or reproduction in other forums is permitted, provided the original author(s) and the copyright owner(s) are credited and that the original publication in this journal is cited, in accordance with accepted academic practice. No use, distribution or reproduction is permitted which does not comply with these terms.
*Correspondence: Hamid Merdji, aGFtaWQubWVyZGppQGNocnUtc3RyYXNib3VyZy5mcg==