- 1Section of Geriatric Medicine, Department of Medicine, University of Verona, Verona, Italy
- 2Section of Geriatric Medicine, Department of Surgery, Dentistry, Pediatric, and Gynecology, University of Verona, Verona, Italy
Arterial stiffness and subendocardial perfusion impairment may play a significant role in heart failure (HF) outcomes. The aim of the study was to examine the main predictors of 30-day readmission in geriatric patients, hospitalized with HF, explore hemodynamical parameters, arterial stiffness indexes, and subendocardial viability ratio (SEVR). In total, 41 hospitalized patients, affected by HF, were included; they underwent clinical evaluation, routine laboratory testing, and echocardiography. At the time of admission, after the achievement of clinical stability (defined as switching from intravenous to oral diuretic therapy), and at discharge, arterial tonometry was performed to evaluate carotid-femoral pulse wave velocity (PWVcf) and SEVR (then corrected for hemoglobin concentration and oxygen saturation). Through the evaluations, a significant progressive decrease in PWVcf was described (17.79 ± 4.49, 13.54 ± 4.54, and 9.94 ± 3.73 m/s), even after adjustment for age, gender, mean arterial pressure (MAP) variation, and left ventricular ejection fraction (LVEF). A significant improvement was registered for both SEVR (83.48 ± 24.43, 97.94 ± 26.84, and 113.29 ± 38.02) and corrected SEVR (12.74 ± 4.69, 15.71 ± 5.30, and 18.55 ± 6.66) values, and it was still significant when adjusted for age, gender, MAP variation, and LVEF. After discharge, 26.8% of patients were readmitted within 30 days. In a multivariate binary logistic regression analysis, PWVcf at discharge was the only predictor of 30-day readmission (odds ratio [OR] 1.957, 95% CI 1.112–3.443). In conclusion, medical therapy seems to improve arterial stiffness and subendocardial perfusion in geriatric patients hospitalized with heart failure. Furthermore, PWVcf is a valid predictor of 30-day readmission. Its feasibility in clinical practice may provide an instrument to detect patients with HF at high risk of rehospitalization.
Introduction
Heart Failure (HF) is a frequent cardiovascular disorder, which shows a sharp increase in prevalence among the elderly (1, 2), with a remarkable clinical and comprehensive burden. Different patterns of HF have been described, and they account for different outcomes (3) and consequently different management of the acute and chronic phases of the disease. Therefore, detecting different subsets of HF is of paramount importance to tailor the treatment and the management of every patient and to provide effective results in terms of outcomes.
Arterial stiffness can be considered the morpho-physiologic expression of vascular aging, and it is strongly entailed in HF development, primarily affecting the diastolic function (4). Cardiovascular aging is characterized by a complex network of inflammatory and atherogenic pathways (5), which leads to reduced arterial wall elasticity. A progressive decrease of arterial compliance (6) results in increased blood pressure (BP), and mostly, in systolic blood pressure (SBP). Pressure overload drives cardiac remodeling, involving left ventricular (LV) wall thickening and left atrial dilation (4). Tonometric evaluation of pulse wave velocity (PWV), and in particular, carotid-femoral PWV (PWVcf) is a reliable predictor of mortality risk in different subsets of patients (7, 8), and it is a part of the latest European guidelines on arterial hypertension (9). Furthermore, interesting evidence points out a relationship between PWV and HF (10) showing worse outcomes in patients with HF with increased PWV (11, 12) and in HF with reduced ejection fraction (HFrEF) patients with reduced pulse pressure (PP) (10, 13).
Lately, several studies shed light on subendocardial perfusion impairment, which can be easily estimated by using subendocardial viability ratio (SEVR) provided by pulse wave analysis (PWA) (14), as the ratio between diastolic pressure time index (DPTI) and systolic pressure time index (SPTI) (15). Considering DTPI, which is the area under the diastolic phase in the aortic pulse wave profile, as an estimation of myocardial oxygen supply, STPI (the area under the systolic phase) as an index of cardiac tissue oxygen consumption (16), and SEVR represents myocardial workload, oxygen supply, and perfusion (17). Therefore, low values of SEVR mirror an impaired subendocardial perfusion (15). SEVR is related to several pathological conditions, such as chronic kidney disease (18), metabolic syndrome (19) hypertension (20), and orthostatic hypotension (21), peripheral arterial disease (22), and aortic stenosis (23), but still, less is known about the relationship between SEVR and HF in geriatric patients.
Although their roles have not been completely explored, hemodynamical parameters may represent a remarkable tool to stratify patients with HF during the in-hospital stay and early after discharge.
Furthermore, geriatric patients affected by HF often show an increased hospital admission or emergency department visits, due to worsening HF symptoms (24–26). Developing and intriguing research is looking for possible predictors of rehospitalization (24) and great importance is given to the “vulnerable time,” which immediately follows the discharge for acute HF (27).
Therefore, the aims of the present study were to evaluate arterial stiffness parameters in a cohort of consecutive in-patients affected by HF, to describe their trend over the time of in-hospital staying, and to identify whether any of these parameters may predict early readmission.
Materials and Methods
Study Population
The study cohort consisted of 41 consecutive patients (14 men and 27 women), aged over 70 years, hospitalized at the Geriatric Section of Verona University Hospital, affected by HF, and prospectively enrolled. The diagnosis of HF was made upon clinical presentation and suggestive chest X-ray imaging. All patients were prescribed intravenous diuretic medications. Patients with tachyarrhythmias with heart rate (HR) > 120 bpm (which would prevent the proper evaluation of arterial stiffness) and severe behavioral disorders (which would prevent a proper compliance during the evaluation) were excluded.
Each patient underwent a comprehensive clinical evaluation; demographical and clinical data included age, sex, BP, HR, and body weight measurement (Salus scale, Milan, Italy). A detailed clinical history was recorded, with particular interest in the presence of atrial fibrillation (AF), coronary artery disease (CAD), arterial hypertension, chronic kidney disease (defined as the presence of an estimated glomerular filtration rate (eGFR), by the Cockroft-Gault equation, lower than 60 ml/min/1.73 m2), and type 2 Diabetes Mellitus (DM2). Functional status was evaluated by Activity Daily Living (ADL) scale and Instrumental Activity Daily Living (IADL) scale. Cognitive profile was described by Mini-Mental State Examination (MMSE) and Montreal Cognitive Assessment (MOCA) test; whenever the MMSE score was > 24, the MOCA test was performed. A geriatric depression scale (GDS) was performed to investigate the psychological condition; Mini Nutritional Assessment (MNA) was used to evaluate the nutritional status. Charlson Comorbidity Index (CCI) was calculated for each patient, considering the Charlson age-adjusted index higher than 5 points as a predictor of poor clinical outcomes (28).
Brachial BP was measured twice using an aneroid sphygmomanometer (Heine Optothecnik, Gilching, Germany) in the subject’s non-dominant arm. The average of the readings was considered as the subject’s BP. Mean arterial pressure (MAP) was then derived, following the formula: MAP (mmHg) = DBP + 1/3 (SBP − DBP). PP, strongly related to cardiovascular risk and coronary heart disease (29, 30), was derived as the difference between SBP and DBP.
During the in-hospital stay, each patient underwent an echocardiographic evaluation, performed by experienced echocardiographers, and the left ventricular ejection fraction (LVEF) was therefore listed.
At the time of admission, venous blood samples for all metabolic assessments were obtained. Creatinine was measured by a modular analyzer (Roche Cobas 8000; Monza, Italy). eGFR was calculated by a Cockroft-Gault formula. Hemoglobin, albumin, and N-terminal pro-brain natriuretic peptide (nt-proBNP) were also measured.
Arterial Stiffness and Subendocardial Viability Ratio Evaluation
The PWA was performed non-invasively using a small portable device called PulsePen (Diatecne, Milan, Italy) (31). Its software, WPulsePen 2.3.2, provides central aortic pressure values, an assessment of arterial pulse wave contours, estimation of reflection waves, and measurements of PWV. A detailed description of PWA calculation was provided in our previous studies (19, 21). PWA provides information about different arterial segments, as it can analyze both elastic arteries by the PWVcf and peripheral muscular arteries by carotid-radial PWV (PWVcr) (32).
PulsePen software also defines the augmentation pressure (AP), which is the systolic pressure increment caused by the reflection wave (from the periphery to the center), and the augmentation index (AI), which is the ratio between augmentation pressure and PP.
As previously described (19, 21), PulsePen software, by PWV traces analysis, provides SEVR measurement, which represents an indirect estimation of myocardial perfusion, relative to left ventricle workload. SEVR is derived as the ratio between DTPI (which is the area under the diastolic phase in the aortic profile, representing an estimation of myocardial oxygen supply) and STPI (the area under the systolic phase, representing cardiac tissue oxygen consumption) (16). Thus, SEVR indirectly reflects the adequacy of subendocardial perfusion.
A critical threshold for SEVR of 0.5 (50%) has been suggested (33), above which an insufficient subendocardial perfusion may be detected. As suggested by previous studies (16), since subendocardial oxygen supply depends both on coronary flow and arterial oxygen saturation, and it can be compromised in case of severe anemia or hypoxia, SEVR was adjusted for both hemoglobin and arterial oxygen concentration (34), following the formulas: SEVR CaO2 1/4 CaO2 DTPI/STPI and CaO2 1/41.34 × blood hemoglobin concentration (g/dl) × arterial oxygen saturation (%) + 0.003 × arterial pressure of oxygen (mmHg). According to previous evidence, the critical value for SEVR CaO2 was considered 10 (34).
Within 24 h from admission, after clinical stability achievement (which was considered as the switching time from intravenous to oral diuretics administration) and at discharge time, both PWV and SEVR were measured, by applanation tonometry. BP was noted each time (Heine Optothecnik, Gilching, Germany). MAP was then derived.
Evaluation of 30-Day Readmission
One month after discharge, a telephonic follow-up checked the outcome as alive/dead and whether the patient had been readmitted to the hospital or not.
The study was approved by the Ethical Committee of the University of Verona.
Statistical Analyses
Results are shown as mean value ± standard deviation (SD). Variables not normally distributed were log-transformed before analysis. Independent samples t-tests were used to compare baseline characteristics of patients with EF ≥ or < 50%. Analysis of variance (ANOVA) was performed when comparing continuous data throughout the follow-time, at admission, clinical stability, and discharge time. The analysis was then adjusted for age, gender, MAP variation, and LVEF.
Binary regression analysis was performed to evaluate 30-day readmission predictors, considering as independent variables age, gender, MAP, AF, PWVcf at discharge time, EF, eGFR, ADL, MMSE; DM2, previous HF hospitalization, CCI, albumin concentration, and length of in-hospital stay.
In order to evaluate the correlation between subclinical organ damage (PWV > 10 m/s) and re-hospitalization, a chi-square test was performed.
A significance threshold level of 0.05 was used throughout the study. All statistical analyses were performed using SPSS 23.0 version for Windows (IBM, Armonk, New York, NY, United States).
Results
Our study population included 41 individuals, mean age 85.7 ± 5.5 years, of whom 27 were women. The main baseline characteristics of the study population are listed in Table 1. When considering the 30-day outcome, 26.8% (n = 11) of the overall population was re-hospitalized and 7.3% (n = 3) of patients died.
None of the baseline hemodynamic variables was different at baseline between patients readmitted after 30 days and patients not readmitted.
The cohort was then sorted in two different subgroups, relying on the echocardiographic EF: 26 (63%) subjects showed preserved EF (EF ≥ 50%), while 15 (37%) patients constituted a reduced EF subgroup, presenting EF < 50%.
Compared to preserved EF subjects, patients with reduced EF showed significantly higher concentrations of nt-proBNP (15,044.93 ± 1, 2281.71 vs. 3,228.08 ± 2,049.42 pg/ml, p < 0.001; Table 1).
Among the hemodynamic variables considered, PP resulted in the only one significantly difference between the two HF subgroups: at the time of admission, it was higher in individuals with preserved EF than in a reduced EF subgroup (64.16 ± 12.02 vs. 55.53 ± 9.18 mmHg, p = 0.015).
The comorbidity burden, evaluated by CCI and the duration of in-hospital stay, did not show any statistical difference between subpopulations.
Through the three evaluations, a progressive decrease in PWVcf was described (17.79 ± 4.49, 13.54 ± 4.54, and 9.94 ± 3.73 m/s, respectively, p < 0.001); the trend remained significant even after adjustment for age, gender, MAP variation, and LVEF (p < 0.001; Figure 1A).
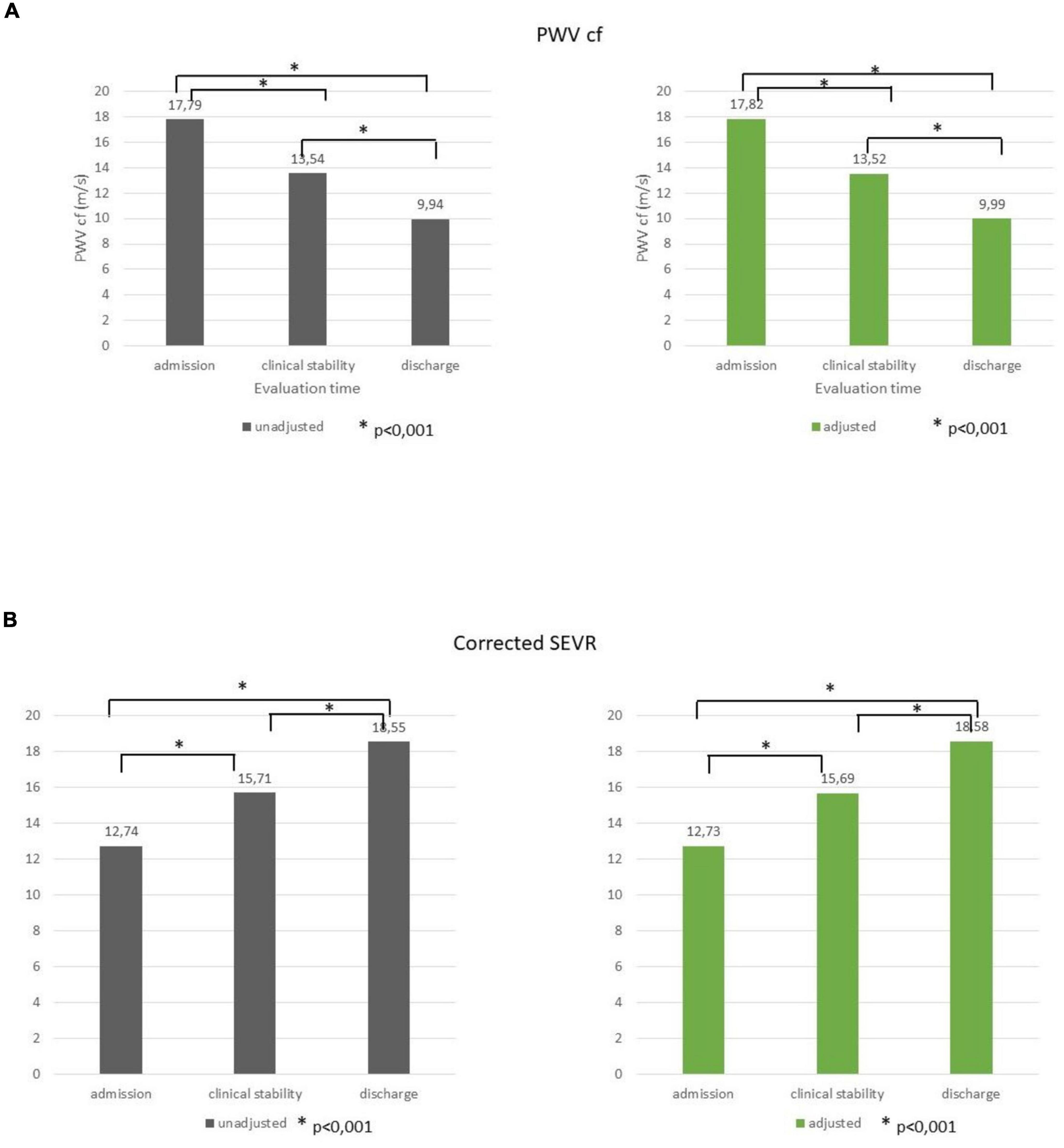
Figure 1. PWVcf (A) and corrected SEVR (B) values at admission, clinical stability, and at discharge before and after adjustment for age, gender, MAP variation, and LVEF. *p < 0.001.
Systolic BP, DBP, and MAP also displayed a decremental trend during the evaluations (Table 2, p < 0.001).
A significant improvement was registered for both SEVR (83.48 ± 24.43, 97.94 ± 26.84, and 113.29 ± 38.02, respectively) and corrected SEVR (12.74 ± 4.69, 15.71 ± 5.30, and 18.55 ± 6.66, respectively) values (p < 0.001 for both), and it was still significant when adjusted for age, gender, MAP variation, and LVEF (p < 0.001; Figure 1B).
In a multivariate binary logistic regression analysis (Table 3), considering 30-day readmission as a dependent variable and age, gender, EF, eGFR, ADL, MMSE, CCI, DM2, AF, previous hospitalizations due to HF, MAP at discharge, and PWVcf at discharge as independent variables. PWVcf at discharge was the only predictor of readmission (odds ratio [OR] 1.957, 95% CI1.112–3.443, p = 0.020).
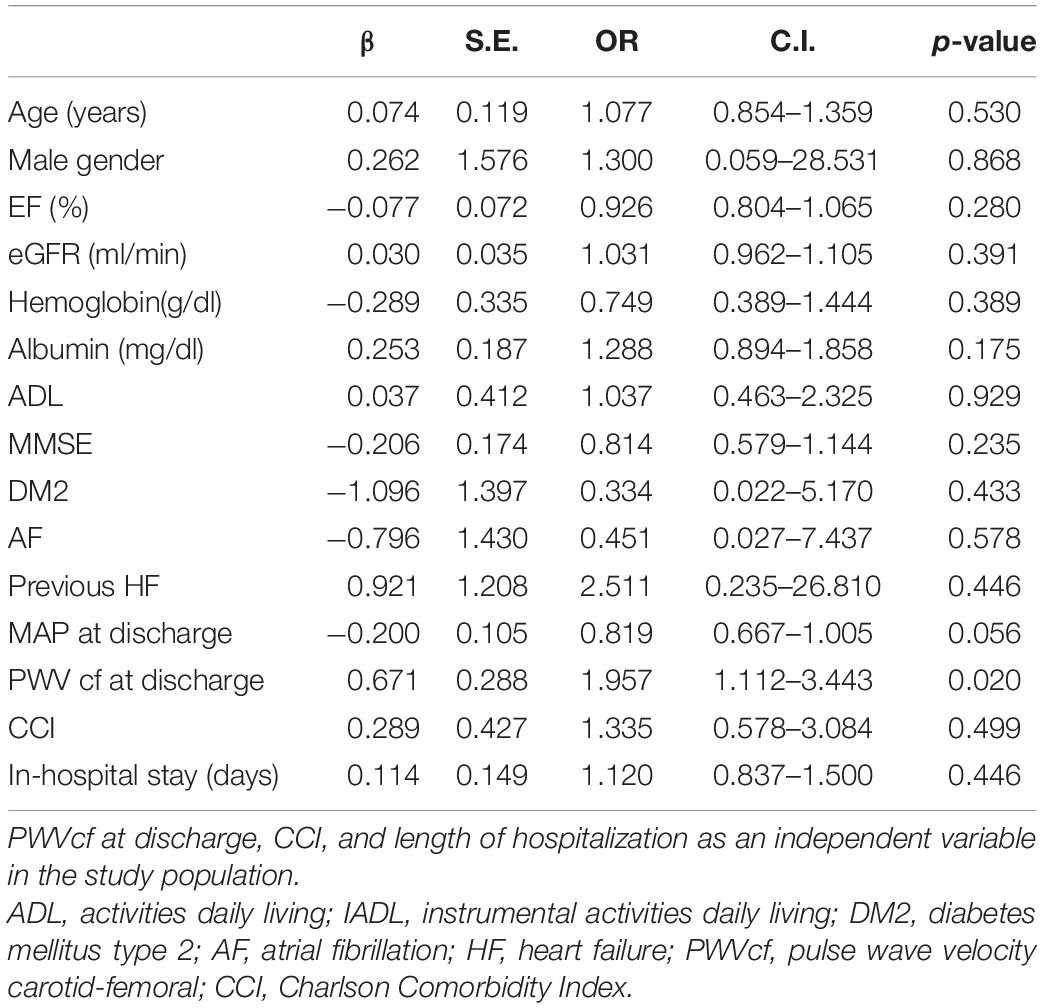
Table 3. Binary logistic regression considering 30-day hospital readmission as a dependent variable and age, gender, EF, eGFR, ADL, DM2, AF, previous HF, and MAP at discharge as independent variables.
Therefore, for each m/s of PWVcf increase at discharge time, we might assume a 95% increase in readmission risk.
Considering PWVcf = 10 m/s as threshold value for subclinical vascular damage (6) and excluding the deceased patients (n = 3), 8 over a total of 13 patients with PWVcf ≥ 10 m/s underwent rehospitalization within 30 days from discharge, whereas only 3 over a total of 25 individuals with PWVcf < 10 m/s were actually readmitted (p = 0.001).
Discussion
Our study, led on HF patients, shows that PWVcf decreases with the optimization of the medical therapy and, irrespective of EF, SEVR increases in line with the clinical improvement. Moreover, the greater the PWV at discharge, the higher the risk of hospital readmission within the following 30 days. All the arterial stiffness parameters (PWVcf, PP, and AP e AI) and SEVR were improved along with the three evaluations during in-hospital stay, and this result was independent of the EF.
Carotid-femoral pulse wave velocity significantly decreases throughout the three evaluations, reflecting clinical improvement, even after adjustment for several variables (age, gender, MAP variation, and EF). These findings confirm and complement, since observed during hospitalization, previous evidence by Kim et al., who showed that PWV decreases in patients with HF after the achievement of clinical stability, which was evaluated after 3 months from discharge (35). These results are also in line with Zheng et al. (36) who found improvement in PWV in patients with hypertension after hypotensive treatment. We may speculate that in patients with HF, a proper medical therapy may improve cardiac pre-load and after-load resulting thus in reduced arterial wall stress and, probably, leading to a decrease in PWV.
To the best of our knowledge, this is the first study, which evaluated tonometric SEVR at admission, after the achievement of clinical stability, and at discharge time. Interestingly, SEVR and corrected-SEVR progressively increased throughout the three evaluations, supporting a relatively quick improvement in subendocardial perfusion in response to medical therapy.
Interestingly, as subendocardial oxygen delivery relies not only on coronary blood flow but also on arterial oxygen concentration, the observed improvement of corrected SEVR in our patients can more precisely represent the actual balance between arterial perfusion and oxygen delivery (16).
After the achievement of clinical stability, the improvement in ventricular workload as a consequence of the medical therapy, and, the reduced wall stress, may play a compelling role in increasing subendocardial perfusion. Corrected SEVR has been considered a feasible and reproducible technique, aimed at detecting preclinical organ damage in patients with HF (14); furthermore, as far as we could demonstrate with our data, corrected SEVR may be also useful to test eventual improvement after proper medical treatment.
Rehospitalization is a relevant problem in patients with HF. More than 1 patient over 4 were readmitted to the hospital in our study population after 30 days. Our readmission rate was more frequent than those observed in some previous studies (37–39) over the same follow-up time, yet the discrepancies could be explained by the age of the patients (much older in our population than in the others (37–39), high comorbidity burden (which was evaluated in our study, whether not entirely specified in other ones), and in line with Molvin et al. who observed a 33.5% readmission rate, in a Swedish cohort of 268 patients (mean age 75 years) over a follow-up time of 5 months (40).
Moreover, we could demonstrate that PWVcf, at discharge time, even after the adjustment for several independent variables (age, gender, EF, eGFR, ADL, MMSE, CCI, DM2, AF, previous HF hospitalization, and MAP at discharge), was the only significant predictor of rehospitalization. Subjects with PWVcf greater than 10 m/s, which is considered a marker of subclinical organ damage by the European Society of Hypertension Guidelines, showed more than twofold higher risk of readmission within the first 30 days after discharge when compared with those with values lower than 10 m/s (61% rehospitalization, as compared to the 26% recorded). Thus, our data show that PWVcf, besides being a valid marker of long-term subclinical organ damage both in the general population and in patients with increased cardiovascular risk (41–44), may be a predictor of early hospital readmission in patients with HF. These findings are in line and complement previous observations that PWV in patients with HFrEF, as evaluated by ultrasound, could predict death or hospitalization, over a follow-up time of 36 ± 19 months (45).
Limitations of our study should be acknowledged to interpret the results. First, the small sample size of our cohort may affect the statistical significance of some associations; moreover, we were neither allowed to sort the study population into the three HF subcategories suggested by ESC guidelines [HFrEF, HFmrEF, and HFpEF, (3)] nor to subdivide women and men populations. Furthermore, we did not know the precise cause of mortality or hospital readmission, which may have been of interest, in order to consider the burden of HF as compared to other comorbidities.
Nonetheless, the strength of our study was that we were able to evaluate PWVcf and SEVR for three consecutive times, providing a detailed trend of arterial stiffness and subendocardial perfusion indexes for each patient. In addition, our study relies on a feasible and reproducible technique, applanation tonometry, which might be easily performed on patients with HF in several geriatric settings, suggesting that pulse wave analysis may be included in the comprehensive assessment of HF elderly patients, as a short-term outcome and early rehospitalization predictor.
Conclusion
In conclusion, medical therapy seems to improve arterial stiffness and subendocardial perfusion in geriatric patients hospitalized with heart failure. Noteworthy, PWVcf is a valid predictor of 30-day readmission, and owing to its feasibility and reproducibility in clinical practice, it may provide an instrument to detect patients with HF at high risk of rehospitalization, in order to plan a tailored management and follow-up, in the early phase after hospital discharge.
Data Availability Statement
The raw data supporting the conclusions of this article will be made available by the authors, without undue reservation.
Ethics Statement
The studies involving human participants were reviewed and approved by the Ethical Committee University of Verona. The patients/participants provided their written informed consent to participate in this study.
Author Contributions
FF contributed to conceptualization, methodology, statistical analyses, and writing—original draft. AG contributed to validation, data curation, and writing—original draft. AF and SU contributed to data collection. EZ, GM, and AR contributed to methodology and editing. MZ contributed to conceptualization, methodology, writing—review and editing, and supervision. All authors contributed to the article and approved the submitted version.
Conflict of Interest
The authors declare that the research was conducted in the absence of any commercial or financial relationships that could be construed as a potential conflict of interest.
Publisher’s Note
All claims expressed in this article are solely those of the authors and do not necessarily represent those of their affiliated organizations, or those of the publisher, the editors and the reviewers. Any product that may be evaluated in this article, or claim that may be made by its manufacturer, is not guaranteed or endorsed by the publisher.
References
1. Conrad N, Judge A, Tran J, Mohseni H, Hedgecott D, Crespillo AP, et al. Temporal trends and patterns in heart failure incidence: a population-based study of 4 million individuals. Lancet. (2018) 391:572–80. doi: 10.1016/S0140-6736(17)32520-5
2. van Riet EES, Hoes AW, Wagenaar KP, Limburg A, Landman MAJ, Rutten FH. Epidemiology of heart failure: the prevalence of heart failure and ventricular dysfunction in older adults over time. A systematic review. Eur J Heart Fail. (2016) 18:242–52. doi: 10.1002/ejhf.483
3. McDonagh TA, Metra M, Adamo M, Gardner RS, Baumbach A, Böhm M, et al. 2021 ESC Guidelines for the diagnosis and treatment of acute and chronic heart failure. Eur J Heart Fail. (2022) 24:4–131. doi: 10.1002/ejhf.2333
4. Strait JB, Lakatta EG. Aging-associated cardiovascular changes and their relationship to heart failure. Heart Fail Clin. (2012) 8:143–64. doi: 10.1016/j.hfc.2011.08.011
5. Bank AJ. Physiologic aspects of drug therapy and large artery elastic properties. Vasc Med. (1997) 2:44–50. doi: 10.1177/1358863X9700200107
6. Vlachopoulos C, Xaplanteris P, Aboyans V, Brodmann M, Cífková R, Cosentino F, et al. The role of vascular biomarkers for primary and secondary prevention. A position paper from the European Society of Cardiology Working Group on peripheral circulation. Endorsed by the Association for Research into Arterial Structure and Physiology (ARTERY) Society. Atherosclerosis. (2015) 241:507–32. doi: 10.1016/j.atherosclerosis.2015.05.007
7. Patoulias D, Papadopoulos C, Stavropoulos K, Imprialos K, Doumas M. Updated Meta-Analysis of Trials Assessing the Cardiovascular Efficacy of Sodium-Glucose Co-Transporter-2 Inhibitors and Glucagon-Like Peptide-1 Receptor Agonists in Black Patients. Am J Cardiol. (2020) 137:133–5. doi: 10.1016/j.amjcard.2020.10.004
8. Laurent S, Cockcroft J, Van Bortel L, Boutouyrie P, Giannattasio C, Hayoz D, et al. Expert consensus document on arterial stiffness: methodological issues and clinical applications. Eur Heart J. (2006) 27:2588–605. doi: 10.1093/eurheartj/ehl254
9. Williams B, Mancia G, Spiering W, Rosei EA, Azizi M, Burnier M, et al. 2018 ESC/ESH Guidelines for themanagement of arterial hypertension. Eur Heart J. (2018) 39:3021–104. doi: 10.1093/eurheartj/ehy339
10. Weber T, O’Rourke MF, Ammer M, Kvas E, Punzengruber C, Eber B. Arterial Stiffness and Arterial Wave Reflections Are Associated With Systolic and Diastolic Function in Patients With Normal Ejection Fraction. Am J Hypertens. (2008) 21:1194–202. doi: 10.1038/ajh.2008.277
11. Regnault V, Lagrange J, Pizard A, Safar ME, Fay R, Pitt B, et al. Opposite Predictive Value of Pulse Pressure and Aortic Pulse Wave Velocity on Heart Failure With Reduced Left Ventricular Ejection Fraction. Hypertension. (2014) 63:105–11. doi: 10.1161/HYPERTENSIONAHA.113.02046
12. Sung S-H, Yu W-C, Cheng H-M, Lee C-W, Lin M-M, Chuang S-Y, et al. Excessive wave reflections on admission predict post-discharge events in patients hospitalized due to acute heart failure. Eur J Heart Fail. (2012) 14:1348–55. doi: 10.1093/eurjhf/hfs124
13. Marti CN, Gheorghiade M, Kalogeropoulos AP, Georgiopoulou VV, Quyyumi AA, Butler J. Endothelial Dysfunction, Arterial Stiffness, and Heart Failure. J Am Coll Cardiol. (2012) 60:1455–69. doi: 10.1016/j.jacc.2011.11.082
14. Salvi P, Baldi C, Scalise F, Grillo A, Salvi L, Tan I, et al. Comparison Between Invasive and Noninvasive Methods to Estimate Subendocardial Oxygen Supply and Demand Imbalance. J Am Heart Assoc. (2021) 10:e021207. doi: 10.1161/JAHA.121.021207
15. Buckberg GD, Fixler DE, Archie JP, Hoffman JI. Experimental subendocardial ischemia in dogs with normal coronary arteries. Circ Res. (1972) 30:67–81. doi: 10.1161/01.RES.30.1.67
16. Salvi P, Revera M, Faini A, Giuliano A, Gregorini F, Agostoni P, et al. Changes in subendocardial viability ratio with acute high-altitude exposure and protective role of acetazolamide. Hypertension. (2013) 61:793–9. doi: 10.1161/HYPERTENSIONAHA.111.00707
17. Ekart R, Bevc S, Hojs N, Knehtl M, Dvoršak B, Hojs R. Albuminuria is Associated With Subendocardial Viability Ratio in Chronic Kidney Disease Patients. Kidney Blood Press Res. (2015) 40:565–74. doi: 10.1159/000368532
18. Ekart R, Bevc S, Hojs N, Hojs R. Derived Subendocardial Viability Ratio and Cardiovascular Events in Patients with Chronic Kidney Disease. CardioRenal Med. (2019) 9:41–50. doi: 10.1159/000493512
19. Fantin F, Giani A, Gasparini L, Rossi AP, Zoico E, Mazzali G, et al. Impaired subendocardial perfusion in patients with metabolic syndrome. Diabetes Vasc Dis Res. (2021) 18:147916412110471. doi: 10.1177/14791641211047135
20. Tsiachris D, Tsioufis C, Syrseloudis D, Roussos D, Tatsis I, Dimitriadis K, et al. Subendocardial viability ratio as an index of impaired coronary flow reserve in hypertensives without significant coronary artery stenoses. J Hum Hypertens. (2012) 26:64–70. doi: 10.1038/jhh.2010.127
21. Fantin F, Giani A, Macchi F, Amadio G, Rossi AP, Zoico E, et al. Relationships between subendocardial perfusion impairment, arterial stiffness and orthostatic hypotension in hospitalized elderly individuals. J Hypertens. (2021) 39:2379–87. doi: 10.1097/HJH.0000000000002944
22. Scandale G, Dimitrov G, Recchia M, Carzaniga G, Minola M, Perilli E, et al. Arterial stiffness and subendocardial viability ratio in patients with peripheral arterial disease. J Clin Hypertens. (2018) 20:478–84. doi: 10.1111/jch.13213
23. Müller C, Goliasch G, Schachinger S, Kastl S, Neunteufl T, Delle-Karth G, et al. Transcatheter aortic valve replacement (TAVR) leads to an increase in the subendocardial viability ratio assessed by pulse wave analysis. PLoS One. (2018) 13:e0207537. doi: 10.1371/journal.pone.0207537
24. Shah A, Mentz RJ, Sun J-L, Rao VN, Alhanti B, Blumer V, et al. Emergency department visits versus hospital readmissions among patients hospitalized for heart failure. J Card Fail. (2022). S1071-9164(21)00491-7. doi: 10.1016/j.cardfail.2021.11.025
25. Thaker R, Pink K, Garapati S, Zarandi D, Shah P, Ramasubbu K, et al. Identify early and involve everyone: interdisciplinary comprehensive care pathway developed for inpatient management and transitions of care for heart failure patients reported using SQUIRE 2.0 guidelines. Cureus. (2022) 14:e21123. doi: 10.7759/cureus.21123
26. Logeart D, Berthelot E, Bihry N, Eschalier R, Salvat M, Garcon P, et al. Early and short-term intensive management after discharge for patients hospitalized with acute heart failure: a randomized study (ECAD-HF). Eur J Heart Fail. (2022) 24:219–26. doi: 10.1002/ejhf.2357
27. Abdin A, Anker SD, Butler J, Coats AJS, Kindermann I, Lainscak M, et al. ‘Time is prognosis’ in heart failure: time-to-treatment initiation as a modifiable risk factor. ESC Hear Fail. (2021) 8:4444–53. doi: 10.1002/ehf2.13646
28. Charlson M, Szatrowski TP, Peterson J, Gold J. Validation of a combined comorbidity index. J Clin Epidemiol (1994) 47:1245–51. doi: 10.1016/0895-4356(94)90129-5
29. Benetos A, Safar M, Rudnichi A, Smulyan H, Richard JL, Ducimetière P, et al. Pulse pressure: a predictor of long-term cardiovascular mortality in a french male population. Hypertension (1997) 30:1410–5. doi: 10.1161/01.HYP.30.6.1410
30. Franklin SS, Khan SA, Wong ND, Larson MG, Levy D. Is pulse pressure useful in predicting risk for coronary heart disease? The Framingham Heart Study. Circulation (1999) 100:354–60. doi: 10.1161/01.CIR.100.4.354
31. Salvi P, Lio G, Labat C, Ricci E, Pannier B, Benetos A. Validation of a new non-invasive portable tonometer for determining arterial pressure wave and pulse wave velocity: the PulsePen device. J Hypertens (2004) 22:2285–93. doi: 10.1097/00004872-200412000-00010
32. McVeigh GE, Bratteli CW, Morgan DJ, Alinder CM, Glasser SP, Finkelstein SM, et al. Age-related abnormalities in arterial compliance identified by pressure pulse contour analysis: aging and arterial compliance. Hypertension (1999) 33:1392–8. doi: 10.1161/01.HYP.33.6.1392
33. Barnard RJ, MacAlpin R, Kattus AA, Buckberg GD. Ischemic response to sudden strenuous exercise in healthy men. Circulation (1973) 48:936–42. doi: 10.1161/01.CIR.48.5.936
34. Brazier J, Cooper N, Buckberg G. The adequacy of subendocardial oxygen delivery: the interaction of determinants of flow, arterial oxygen content and myocardial oxygen need. Circulation (1974) 49:968–77. doi: 10.1161/01.CIR.49.5.968
35. Kim D-B, Baek SH, Jang SW, Her S-H, Shin D-I, Park CS, et al. Improvement of Arterial Stiffness in the Transition From Acute Decompensated Heart Failure to Chronic Compensated Heart Failure. Clin Cardiol (2013) 36:358–62. doi: 10.1002/clc.22127
36. Zheng M, Huo Y, Wang X, Xu X, Qin X, Tang G, et al. A prospective study on pulse wave velocity (PWV) and response to anti-hypertensive treatments. Int J Cardiol (2015) 178:226–31. doi: 10.1016/j.ijcard.2014.10.049
37. Johnson AE, Routh S, Taylor CN, Leopold M, Beatty K, McNamara DM, et al. Developing and implementing an mHealth heart failure self-care program to reduce readmissions: randomized controlled trial. JMIR Cardio (2022) 6:e33286. doi: 10.2196/33286
38. Ayatollahi Y, Liu X, Namazi A, Jaradat M, Yamashita T, Shen JJ, et al. Early Readmission risk identification for hospitalized older adults with decompensated heart failure. Res Gerontol Nurs (2018) 11:190–7. doi: 10.3928/19404921-20180322-01
39. Chan RK, Dinh DT, Hare DL, Lockwood S, Neil C, Prior D, et al. Management of acute decompensated heart failure in rural versus metropolitan settings: an australian experience. Hear Lung Circ (2022) 31:491–8. doi: 10.1016/j.hlc.2021.08.020
40. Molvin J, Jujic A, Bachus E, Gallo W, Tasevska-Dinevska G, Holm H, et al. Cardiovascular biomarkers predict post-discharge re-hospitalization risk and mortality among Swedish heart failure patients. ESC Hear Fail (2019) 6:992–9. doi: 10.1002/ehf2.12486
41. Prenner SB, Chirinos JA. Arterial stiffness in diabetes mellitus. Atherosclerosis (2015) 238:370–9. doi: 10.1016/j.atherosclerosis.2014.12.023
42. Fantin F, Comellato G, Rossi AP, Grison E, Zoico E, Mazzali G, et al. Relationship between neck circumference, insulin resistance and arterial stiffness in overweight and obese subjects. Eur J Prev Cardiol (2017) 24:1532–40. doi: 10.1177/2047487317721655
43. Fantin F, Di Francesco V, Rossi A, Giuliano K, Marino F, Cazzadori M, et al. Abdominal obesity and subclinical vascular damage in the elderly. J Hypertens (2010) 28:333–9. doi: 10.1097/HJH.0b013e328333d23c
44. Smulyan H, Lieber A, Safar ME. Hypertension, diabetes type II, and their association: role of arterial stiffness. Am J Hypertens (2016) 29:5–13.
Keywords: heart failure, SEVR, PWV, hospital readmission, older patients
Citation: Fantin F, Giani A, Franconi A, Zoico E, Urbani S, Rossi AP, Mazzali G and Zamboni M (2022) Arterial Stiffness, Subendocardial Impairment, and 30-Day Readmission in Heart Failure Older Patients. Front. Cardiovasc. Med. 9:918601. doi: 10.3389/fcvm.2022.918601
Received: 12 April 2022; Accepted: 06 May 2022;
Published: 17 June 2022.
Edited by:
Paolo Salvi, Istituto Auxologico Italiano (IRCCS), ItalyReviewed by:
Maheswara Reddy Koppula, ECMC, United StatesAndrea Grillo, Azienda Sanitaria Universitaria Giuliano Isontina (ASUGI), Italy
Copyright © 2022 Fantin, Giani, Franconi, Zoico, Urbani, Rossi, Mazzali and Zamboni. This is an open-access article distributed under the terms of the Creative Commons Attribution License (CC BY). The use, distribution or reproduction in other forums is permitted, provided the original author(s) and the copyright owner(s) are credited and that the original publication in this journal is cited, in accordance with accepted academic practice. No use, distribution or reproduction is permitted which does not comply with these terms.
*Correspondence: Francesco Fantin, ZnJhbmNlc2NvLmZhbnRpbkB1bml2ci5pdA==