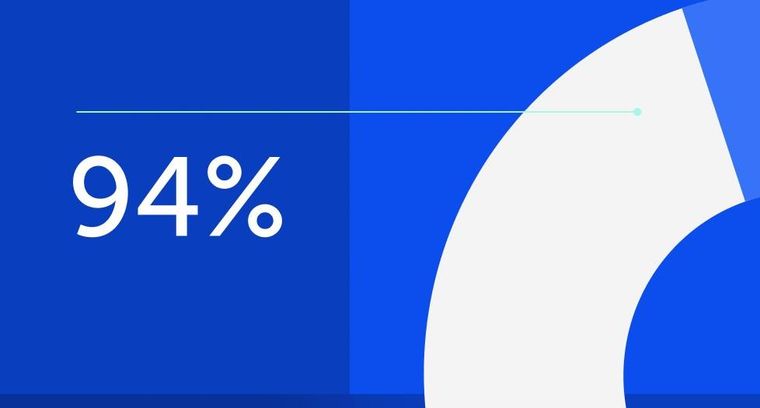
94% of researchers rate our articles as excellent or good
Learn more about the work of our research integrity team to safeguard the quality of each article we publish.
Find out more
ORIGINAL RESEARCH article
Front. Cardiovasc. Med., 18 August 2022
Sec. Cardiovascular Imaging
Volume 9 - 2022 | https://doi.org/10.3389/fcvm.2022.917026
This article is part of the Research TopicAdvances in Predicting Future Adverse Coronary Events: The role of cardiovascular imaging and coronary physiology indicesView all 10 articles
Purpose: Several adults with repaired tetralogy of Fallot (rToF) undergo pulmonary valve replacement (PVR) to reduce the right ventricular volume and retain right ventricular function. However, there is currently no consensus on the ideal time for PVR surgery in asymptomatic patients with rTOF with pulmonary regurgitation (PR). Clinical outcomes after PVR are also indeterminate. Recently, myocardial strain and strain rate derived from cardiac magnetic resonance (CMR) feature tracking were found to be more sensitive to right ventricular dysfunction than conventional parameters and therefore may add prognostic value in patients with rToF. We aimed to analyze whether pre-PVR left ventricular (LV) strain and strain rate detected by CMR feature tracking are associated with midterm outcomes after PVR in patients with rToF.
Methods: Seventy-eight asymptomatic patients with rToF who required PVR due to moderate or severe PR were prospectively enrolled between January 2014 and June 2020. CMR cine sequences were obtained, and feature tracking parameters were measured preoperatively. Adverse events were documented during the follow-up. Receiver operating characteristic analysis was performed to determine the cutoff value. Kaplan–Meier curves were drawn with log-rank statistics; moreover, univariate and multivariate Cox proportional hazards regression analyses and Harrel C-indices were analyzed.
Results: During 3.6 ± 1.8 years of follow-up, 25 adverse events were recorded. Kaplan–Meier survival curves and univariate Cox analysis verified that patients with significantly reduced radial strain (RS), circumferential strain (CS), longitudinal strain (LS), RS rate at systole and diastole (RSRs and RSRe), and circumferential and LS rates at diastole (CSRe and LSRe) had worse event-free survival. After multivariate correction, only LS and LSRe remained significantly associated with adverse outcomes (hazard ratio = 1.243 [1.083–1.428] and 0.067 [0.017–0.258], respectively, all p < 0.05). The cutoff values of LS and LSRe were −12.30 (%) and 1.07 (s–1), respectively.
Conclusion: The LV strain and strain rate prior to PVR are important prognostic factors for adverse events after PVR in rToF.
Tetralogy of Fallot (ToF) is one of the most common types of congenital heart disease, affecting 356 per million live births (1). When surgical repair of ToF (rToF) is performed in early childhood, the natural history of the disease dramatically changes and survival in adulthood improves. However, pulmonary regurgitation (PR) usually occurs after rToF, leading to poor prognosis. There is 40–85% of patients who develop moderate to severe PR in 5–10 years after repair (2, 3). Pulmonary valve replacement (PVR) was introduced to reduce late complications, prior symptoms, and worsening function (4). Although an increasing number of studies have reported PVR as the treatment of choice for severe PR after rToF, the effects of PVR on ventricular remodeling remain controversial.
The guidelines issued by the AHA/ACC and ESC recommend the right and left ventricular end-diastolic volume index (RVEDVI and LVEDVI), tricuspid regurgitation (TR), arrhythmia, and other cardiac function and clinical parameters to determine the timing of PVR surgery (4, 5). The AHA/ACC recommends that adults with previous ToF and severe PR undergo PVR surgery when they have moderate to severe RV dysfunction or enlargement. ESC experts believed that normalization of RV size after reintervention is unlikely as soon as the end-systolic index exceeds 80 ml/m2 and the end-diastolic volume index exceeds 160 ml/m2; however, this cutoff for reintervention may not correlate with clinical benefit. A more sensitive parameter that can predict adverse outcomes at an earlier stage may help identify the optimal time for PVR surgery (6).
In comparison with the traditional measurements, including ejection function (EF) and ventricular volume, the myocardial strain detected by cardiac magnetic resonance (CMR) feature tracking, which reflects myocardial deformation, has added value to predict the outcome of various cardiovascular diseases (7, 8). Although without impaired EF, myocardial strain could still be a sensitive parameter for evaluating ventricular dysfunction and may add clinical value during follow-up (9).
Owing to ventricular coupling, LV strain is also implicated in RV volume overload (10). Thus, LV strain has the potential to provide valuable information prior to PVR. However, there is little data and no consensus on the use of CMR feature tracking to help determine the timing of surgical intervention for PR in patients with rToF (6).
To date, there have been studies comparing the changes in strain and strain rate before and after PVR (11–13) or the relationship between strain and ventricular function parameters (5, 14, 15); however, few of these studies analyzed the relationship between strain and post-PVR surgical outcomes. We aimed to comprehensively evaluate the predictive value of LV strain and strain rate using a custom feature-tracking algorithm applied to a conventional CMR cine in asymptomatic patients with rToF and PVR. We assumed that LV strain and strain rate could have a potential predictive value.
This prospective study was conducted at our hospital. The study protocol was approved by the ethics committee of our hospital. All participants provided written informed consent prior to enrolment.
Asymptomatic patients were defined as those who maintained a functional status in New York Heart Association class I or II without arrhythmia or heart failure symptoms/signs that cause syncope (16).
The severity of PR was divided into absent, trivial, mild, moderate, and severe by transthoracic echocardiography (16). Patients underwent follow-up through either a clinic or via telephone inquiring with patients or their contacts every 3 months after enrolment. Adverse events that occurred after PVR surgery were recorded. Adverse events included sudden cardiac death (unexpected death either within 1 h of the onset of cardiac symptoms without progressive cardiac deterioration, when asleep, or within 24 h of last being seen alive) (17), cardiac transplantation, application of implantable cardioverter defibrillator (ICD) (18), heart failure (19), and arrhythmia-induced syncope (“syncope caused by arrhythmias” was adjudicated based on clinical symptom and ECG monitor). The syncope cases were defined position-independent, with few prodromal symptoms, and may occur with cyanosis, dyspnea, arrhythmias, weak heart sounds, and associated ECG-detected arrhythmias) (20, 21), reoperation for PVR, sustained atrial or ventricular arrhythmias analyzed by 24-h Holter monitoring (last ≥ 30s but not causing syncope), and cardiac catheterization ablation (22).
Patients with rToF who were candidates for PVR were initially screened for this study from January 2014 to June 2020. According to the indications for PVR in asymptomatic patients with moderate or severe PR after rToF recommended by Boston Children’s Hospital in 2013 (22), asymptomatic patients with moderate to severe pulmonary valve regurgitation after rToF (regurgitation fraction by CMR ≥ 25%) meeting at least two of the following conditions were included: (1) RVEDVI > 150 ml/m2, (2) RV end-systolic volume index (RVESVI) > 80 ml/m2, (3) RVEF < 47%, (4) LVEF < 55%, (5) QRS duration > 160 ms, (6) sustained tachyarrhythmia related to right heart volume load, and (7) the presence of other hemodynamically significant lesions.
The following exclusion criteria were established: (1) obvious symptoms, (2) the presence of residual severe right ventricle outflow tract obstruction (RVOTO; ECG calculated gradient ≥ 60 mmHg), (3) RV pressure surpassing or equal to LV pressure, (4) ICD insertion or history of PVR, (5) contraindications for CMR or surgery, and (6) incomplete or poor-quality CMR (9).
CMR examinations were performed on a 3.0 T system within 6 months prior to PVR surgery using a 3.0 T scanner (Ingenia, Philips Healthcare, Best, Netherlands) with front and back surface coils and retrospective ECG triggering for capture of the entire cardiac cycle by the same senior operator. Cine images were obtained using a balanced steady-state free precession (SSFP) sequence, including 2-chamber, 4-chamber, and short-axis acquisitions with 25 image frames per cardiac cycle. The short-axis scans covered the entire LV (nine slices; the following standard acquisition parameters used for routine clinical imaging were applied: field of view, 321 × 321 mm2; matrix, 184 × 256; slice thickness, 6 mm; TR/TE/flip-angle: 3 ms/1.6 ms/45°; temporal resolution, 43 ms; and parallel acquisition technique factor, 2). The two-dimensional phase-contrast images perpendicular to the main pulmonary artery (PA) were used for quantification of the PR fraction (field of view, 350 × 321 mm2; matrix, 140 × 123; slice thickness, 6 mm; TR/TE/flip-angle: 4.9 ms/2.9 ms/10°; parallel acquisition technique factor, 1).
All PVR surgeries were completed under cardiopulmonary bypass with mild hypothermia or a beating heart (without right-to-left shunt) by the same surgeon. A longitudinal incision was made in the pulmonary artery trunk or the position where the patch was previously placed. The replacement valve was inserted in the orthotropic position.
The LV and RV cardiac function analysis and strain analysis were measured using CVI 42 software (Circle Cardiovascular Imaging Inc., Calgary, Canada) with the help of artificial intelligence (with more than 3 years of work experience and more than 600 cases of cardiac MRI analysis) (23). The endocardial and epicardial borders of the LV were semi-automatically defined using the AI function of the CVI following manual adjustments. Measurements included RA and LA volumes, diastolic and systolic RV and LV volumes, mass, ventricular stroke volumes, and EFs. The ventricular volumes and mass were indexed by body surface area (BSA) into RA volume index (RAVI), LA volume index (LAVI), LVEDVI, LV end-systolic volume index (LVESVI), indexed RVEDVI, and RVESVI. Heart rate and QRS duration were obtained using electrocardiography. The PR fraction was calculated from the CMR flow velocity mapping. The severity of RVOTO was reflected by the pulmonary artery systolic gradient from the ECG. RV mass/volume ratio was calculated to reflect the degree of right ventricular hypertrophy (RVH). The RV pressure was measured using TR.
The global cardiac strain and strain rate representing the average of the entire heart were calculated from CMR cine images using the mature feature tracking software CVI 42 version 5.12. The method has been described in detail in a previous study (15). In brief, the LV 4-chamber, LV 3-chamber, and LV 2-chamber were selected for post-processing to calculate the peak longitudinal strain (LS), peak systolic strain rate, and peak early diastolic strain rate (LSRe). The entire LV short-axis SSFP cine images were analyzed for radial strain (RS), circumferential strain (CS), peak systolic strain rate (RSRs and CSRs), and early diastolic strain rate (RSRe and CSRe). The endocardial and epicardial borders of the LV were semi-automatically defined using the AI function of the CVI following manual adjustments. The peak radial, circumferential, and LSs of the LV were identified as the highest or lowest peak of the global strain curve. Similarly, the peak systolic and early diastolic strain rates of the LV were calculated as the highest or lowest peak of the global strain rate curve (Figure 1) (24).
Figure 1. Representative short-axis and long-axis images (A), strain curves (B), and strain rate curves (C) in a participant who underwent pulmonary valve replacement (PVR). The epicardial and endocardial borders (excluding papillary muscles and trabeculae) at end-diastole are semi-manually defined in the LV 4-chamber, LV 2-chamber, and LV 3-chamber to calculate longitudinal strain and strain rate. The entire LV short-axis SSFP cine images are used to calculate radial and circumferential strain and strain rate.
Intra- and inter-observer reliabilities were assessed in 20 randomly selected patients using Bland–Altman plots. Intra-observer reliability was derived from repeated measurements by one radiologist (**) after at least 1 week of blinding to previous results. Inter-observer reliability was independently assessed by two radiologists with more than 3 years of work experience and more than 650 cases of cardiac MRI analysis (** and AA), where one radiologist measured once and then a second radiologist measured again (blinded to the first radiologist’s measurements).
Statistical analyses were performed using SPSS Statistics (version 22.0; IBM Corp., Armonk, NY, United States) and MedCalc (version 18.2.1, Ostend, Belgium). Continuous data were descriptively reviewed and statistically analyzed using the Kolmogorov–Smirnov test to check for normality of the continuous variables. Continuous variables were described as means ± standard deviations or as median values with interquartile range, with respect to the normality of distribution. Comparisons between demographic and strain variables determined in the adverse event and non-adverse event groups were performed using either Student’s t-test or Wilcoxon rank-sum test on the basis of whether the data were normally distributed (24). The Bonferroni method was used to correct multiple comparisons. Categorical variables were summarized by frequencies and percentages and compared between patients with and without the outcomes using the chi-squared test or Fisher’s exact test.
To visualize the difference in event-free survival between different patient categories, Kaplan–Meier curves were drawn, followed by the log-rank test. The optimal preoperative strain and strain rate cutoffs were identified using receiver operating characteristic (ROC) curve analysis using the Youden index. The ROC curve was then drawn with the corresponding calculated area under the curve (AUC). The parameters satisfying the pH assumption were included in the univariate Cox regression analysis. Univariate Cox regression analysis was used to evaluate the predictive value of the strain and strain rate. Multivariate analysis Cox regression (forward LR) was performed for those parameters that were confirmed to be statistically significant in univariate analysis (P < 0.05) to identify the independent risk factors of prospective adverse events. Harrel C-indices were used to compare the relative predictive abilities of strain and strain rate. Statistical significance was set at P < 0.05.
From January 2014 to June 2020, 78 asymptomatic patients with rToF with moderate or severe PR (21.5 years old, 44% male) were included for analysis with an average follow-up duration of 3.6 ± 1.8 years. The reasons for failure to be included are detailed in the study flowchart (Figure 2). The patients involved in our study did not have coronary artery anatomy abnormalities; only two patients had aortic arch abnormalities (patent ductus arteriosus). Demographic, surgical, ECG, and CMR parameters are summarized in Table 1. If appropriate, the parameters were indexed to BSA. On average, the age of the patients who underwent primary rToF was 3 (interquartile range [IQR]: 0–9) years. The most common approach was the transannular patch (n = 56). The mean QRS duration (150.78 ± 30.89 ms) was extended. On average, patients had slightly reduced LVEF (50.47 ± 10.94%) and RVEF (38.37 ± 12.53%). Meanwhile, the RVEDVI and RVESVI were significantly enlarged (168.8 ± 54.36 and 104.42 ± 43.06 ml/m2, respectively). The mean PR fraction was 49.98 ± 18.58%.
Figure 2. This flow chart shows the number of patients at each stage of the study. PVR, pulmonary valve replacement; ICD, implantable cardioverter defibrillator; ToF, Tetralogy of Fallot; CMR, cardiac magnetic resonance.
Table 1. Baseline demographic, electrocardiographic and CMR parameters of the study subjects (n = 78).
A total of 25 (32%) patients had noted adverse events (cardiovascular death, 3; ICD for ventricular tachycardia combined with atrioventricular block, 1; heart failure, 9; syncope caused by arrhythmias, 6; sustained atrial or ventricular arrhythmias, 4; and cardiac catheterization ablation for atrial fibrillation, 2), and 53 (68%) patients did not have any adverse events. Significant differences were noted between the two groups in LV RS, CS, and LS (all p < 0.001; Table 2 and Figure 3). The values in patients with adverse events were lower. Similarly, patients with adverse events noted tended to have lower absolute preoperative LV RSRs (1.37 ± 0.31 vs. 1.88 ± 0.59 s–1, p < 0.001) and RSRe (−1.2 ± 0.57 vs. −2.28 ± 0.93 s–1, p < 0.001). Furthermore, patients with adverse events noted had lower absolute preoperative CSRe (0.90 ± 0.26 vs. 1.23 ± 0.33 s–1, p < 0.001) and LSRe (0.79 ± 0.31 vs. 1.34 ± 0.35 s–1, p < 0.001) than those without adverse events. Significant differences were also observed in heart rate, age at surgical repair, age at PVR, LVEF, LVESVI, RVESVI, RV mass, and RV mass index between the two groups (Table 2). However, the QRS duration and PR fraction between the two groups did not exhibit a significant difference.
Table 2. Comparison of baseline parameters and LV strain parameters (mean ± SD/median and interquartile range) between patients with or without adverse events.
Figure 3. LV peak radial strain (RS), peak circumferential strain (CS), peak longitudinal strain (LS), peak radial strain rate at end-systole (RSRs), peak circumferential strain rate at end-systole (CSRs), peak longitudinal strain rate at end-systole (LSRs), peak radial strain rate at end-diastole (RSRe), peak circumferential strain rate at end-diastole (CSRe), and peak longitudinal strain rate at end-diastole (LSRe) in patients with adverse events (n = 25) and patients without adverse events (n = 53).
After ROC analysis, the corresponding cutoff values, sensitivity, specificity, and AUC of clinical parameters, different preoperative function parameters, strains, and strain rates for predicting adverse outcomes were obtained (Table 3). We found that the AUCs of the strain parameters were the best among the abovementioned parameters. The cutoff values of RS, CS, and LS were 22.2% (68.00% sensitivity, 92.45% specificity), −12.35% (88.00% sensitivity, 96.23% specificity), and −12.3% (96.00% sensitivity, 73.58% specificity), respectively. With regard to strain rates, the cutoff values of RSRs, RSRe, CSRe, and LSRe were 1.69 s–1 (92.00% sensitivity, 60.38% specificity), −1.65 s–1 (88.00% sensitivity, 75.47% specificity), 0.84 s–1 (56.00% sensitivity, 90.57% specificity), and 1.07 s–1 (92.00% sensitivity, 67.92% specificity), respectively. The AUCs of RS, CS, LS, RSRs, RSRe, CSRe, and LSRe were 0.863, 0.959, 0.916, 0.792, 0.889, 0.788, and 0.871, respectively (Figure 4 and Table 3).
Figure 4. Receiver operating characteristic (ROC) curve analysis of the prognostic performance of peak radial strain (RS), peak circumferential strain (CS), peak longitudinal strain (LS), peak systole radial strain rate (RSRs), peak early diastole radial strain rate (RSRe), peak circumferential strain rate at end-diastole (CSRe), and peak early diastole longitudinal strain rate (LSRe) in asymptomatic patients with repaired tetralogy of Fallot (rToF) who required pulmonary valve replacement (PVR) for moderate or severe pulmonary regurgitation.
The Kaplan–Meier survival curves (Figure 5) verified that patients with significantly reduced RS, CS, LS, RSRs, RSRe, CSRe, and LSRe had worse event-free survival than those who did not have a significant reduction. The 5-year event-free cumulative survival rates were 68.6, 91, and 94% for patients with a preoperative RS of > 22.2% (p < 0.001), preoperative CS of < −12.35% (p < 0.001), and preoperative LS of < −12.3% (p < 0.001), respectively. As for the strain rate, the 5-year event-free cumulative survival rates were 74, 82.4, and 65.2% for patients with a preoperative RSRs of > 1.69 s–1 (p < 0.001), preoperative RSRe of < −1.65 s–1 (p < 0.001), and preoperative CSRe of ≥ 0.84 s–1 (p = 0.004), respectively. The median survival time was 62% for patients with a preoperative LSRe of > 1.07 s–1 (p < 0.001).
Figure 5. Kaplan–Meier survival curves for patient subgroups stratified by peak radial strain (RS), peak circumferential strain (CS), peak longitudinal strain (LS), peak systole radial strain rate (RSRs), peak early diastole radial strain rate (RSRe), peak circumferential strain rate at end-diastole (CSRe), and peak early diastole longitudinal strain rate (LSRe).
In univariate Cox analysis, both strain (RS, CS, and LS) and strain rate (RSRs, RSRe, CSRe, and LSRe) were significantly associated with adverse outcomes in patients with rToF after PVR (Table 4). The HRs of RS, CS, LS, RSRs, RSRe, CSRe, and LSRe were 0.845, 1.336, 1.342, 0.198, 2.450, 0.199, and 0.053, respectively. The C-indices of RS, CS, LS, RSRs, RSRe, CSRe, and LSRe were 0.811, 0.826, 0.801, 0.73, 0.624, 0.805, 0.75, 0.683, and 0.834, respectively. After multivariate correction, the predictive values of preoperative LS and LSRe remained statistically significant. Specifically, multivariate Cox regression analysis identified LS (HR = 1.243 [1.083–1.428]) and LSRe (HR = 0.067 [0.017–0.258]) as strong predictors of adverse events (Table 5).
Table 4. Univariate associations of clinical and cardiac magnetic resonance (CMR) characteristics with adverse events.
Table 5. Multiple multivariable Cox to evaluate the associations of clinical and cardiac magnetic resonance (CMR) characteristics with adverse events.
Regarding cardiac function parameters, in the univariate Cox analysis, RVESVI, LVEDVI, and LVESVI were associated with worse outcomes after PVR, whereas RVEDVI, RVEF, and LVEF were not. In addition to NYHA class III or IV prior to PVR, severe tricuspid regurgitation, ≥ 3 previous cardiac surgeries, sustained atrial arrhythmias prior to PVR, and the time interval between PVR and endpoint were related to adverse events after PVR (Table 4). However, after multiple multivariate corrections, only the predictive values of preoperative LS and LSRe remained significant (Table 5).
In a subgroup of 20 patients, reliability of strain was generally high with an intra-observer difference of approximately 0.01 ± 0.28%–0.12 ± 0.26%, and an inter-observer difference of approximately 0.01 ± 0.24%–0.15 ± 0.31%. Similarly, intra- and inter-observer differences were also small for strain rates (approximately 0.01 ± 0.35–0.13 ± 0.24 s–1 and 0.01 ± 0.29–0.10 ± 0.30 s–1, respectively; Bland–Altman plots are presented in Supplementary Figures 1, 2).
In the present study, we found the following findings: (1) factors, including conventional volume parameters of both ventricles, ≥ 3 previous cardiac surgeries, severe TR, sustained atrial arrhythmias prior to PVR, and NYHA III/IV, were all associated with outcomes of PVR in patients with rToF; (2) preoperative LV RS, CS, LS, RSRs, CSRe, RSRe, and LSRe were significantly associated with adverse outcomes after PVR surgery in patients with rToF, suggesting that they serve as a novel index for risk stratification assessment in patients with rToF after PVR; and (3) multiple variable analysis demonstrated that patients with the highest incidence of adverse events were those with a severe reduction in LV LS and/or LSRe prior to PVR surgery.
Progressive PR is one of the most common complications occurring after rToF, despite the current treatment strategies used to treat ToF, demonstrating long-term survival (30-year survival rate between 68.5 and 90.5%) (25). In response to longstanding regurgitation, the RV progressively dilates to adapt to the increasing load (26), and the patients’ exercise tolerance progressively reduces. In such situations, PVR is an effective method to help restore pulmonary valve function and improve cardiac function while alleviating symptoms (27). Our study confirmed for the first time that a severe decrease in RS, CS, LS, RSRs, RSRe, and LSRe was associated with an increase in the incidence of adverse outcomes during a midterm follow-up. Among these, LS and LSRe before PVR were the strongest risk factors for postoperative outcomes. After adjusting for other clinical and cardiac function parameters, these strain parameters maintained their prognostic values. This was supported by several CMR feature tracking-derived myocardial function indices, in which LSRe was utilized in the assessment of LV diastolic function and proved to be the most stable parameter with high reproducibility at both intra- and inter-observer levels (28).
Several factors influence the prognosis of PVR, most of which are clinical indicators or surgery-related factors. Jang et al. (29) conducted a retrospective study of 131 PVRs to explore the midterm clinical results of PVR after rToF. In univariate analysis, they found that the type of valve implanted, large valve implantation, and young age for rToF (<15 years) were risk factors for repeated PVR. Dorobantu et al. found that PVR after 35 years of age was associated with worse outcomes (30). In our study, we ascertained that the age for surgical repair of the ToF and PVR was significantly higher in patients with no events than in those with events; however, this difference did not affect the outcomes after PVR. Moreover, Jang et al. (29) found that the type of implanted valve and valve size were risk factors, whereas our study did not. The use of only biological valves may be the culprit, whereas, in our study, the patients used mechanical valves as well as homogenous valves, in addition to biological valves. We did not record this data in terms of valve size.
Furthermore, in our study, the pre-PVR LV and RVEF were not associated with adverse events, whereas previous studies, including the INDICTOR study (31), found that RVEF has a predictive value for mortality (P = 0.03). The reason may be that the RVEF values of our study, in both the event and no event groups, were relatively low (<40%), and our study had a shorter average follow-up time (3.6 vs. 9.5 years). Similar to the INDICTOR study, we found that LVESVI and atrial arrhythmias prior to PVR are related to prognosis; however, we did not find the predicted value of the RV mass-to-volume ratio and RV systolic blood pressure, as mentioned in their study. In addition, we found that LVEDVI and RVESVI were correlated with prognosis, which is consistent with the findings of other studies. However, after incorporating the strain parameter into the multivariate analysis, the predictive value of these parameters ceased to exist.
Moreover, Roderick et al. found that prolonged QRS duration prior to PVR is the main determinant of the poor long-term follow-up outcome of patients with ToF (32); however, our study did not find such a result. Some studies found that VO2 peak (%), which assesses the degree of exercise tolerance, may be a useful predictor of adverse events after PVR (33, 34). Although we were not able to obtain the results of the cardiopulmonary exercise experiment, we also concluded that patients with NYHA III/IV have a poor prognosis based on the NYHA classification, which correlates with the study conducted by Anna et al. (35). Anna et al. also found that ≥ 3 previous cardiac surgeries were associated with worse outcomes, which is consistent with the findings of our study. Their study also found that older age at rToF and greater BSA at PVR were factors influencing poor prognosis; however, this phenomenon was not observed in our study.
One study demonstrated that in multivariate analysis, severe preoperative TR (HR = 2.49; 95% confidence interval [CI], 1.11–5.52), right ventricular end-systolic volume (HR = 1.02; 95% CI, 1.01–1.03), and age at PVR can predict adverse events (36). However, in our study, the value of severe preoperative TR and right ventricular end-systolic volume to predict adverse events was only found in the univariate analysis. In the multivariate analysis, these parameters were not useful. Age at PVR was not significantly different between univariate and multivariate analyses.
This study had some limitations that must be highlighted. For instance, our study was conducted in one medical center with a relatively small sample size; thus, in addition to GLS, the feasibility of other parameters, such as GCS, should be further explored. Large-sample multicenter studies with possible confounders are required to validate and improve the power of assessing events. Another limitation of the study is the cardiac endpoint utilized, which includes a wide range of heterogeneous events from hard to softer ones. Moreover, it was determined that the number of outcomes was modest, although this is the largest study of patients with rToF after PVR (31). The number of hard events was small because the sample size was small, and contrastingly, partly because the cardiac function of such patients significantly improved after PVR (13). In addition, due to limited research conditions, we did not report inter-study reproducibility. However, inter-study reproducibility of strain measured by CMR feature tracking has been reported in a previous study, showing a satisfactory coefficient of variation and intraclass correlation coefficient (37). Lastly, it was shown that significant differences were noted in all strain and strain rate values between different vendors. Because we only provided the strain and strain rate data of the Circle CVI software (38), the data of other vendors, such as TomTec Arena, QStrain Medis, and Segment Medviso, still need to be further supplemented by other studies.
In conclusion, PVR in patients with rToF has relatively low mortality and fewer adverse events. Preoperative LV RS, CS, LS, RSRs, RSRe, CSRe, and LSRe were significantly associated with adverse outcomes after PVR. In particular, LS and LSRe assessed with CMR are independent predictors of survival in patients with rToF after PVR and offer incremental information for risk stratification beyond clinical parameters, biomarkers, and standard CMR parameters.
The raw data supporting the conclusions of this article will be made available by the authors, without undue reservation.
The studies involving human participants were reviewed and approved by Fuwai Hospital Ethics Department. Written informed consent to participate in this study was provided by the participants or their legal guardian/next of kin.
SZ, SL, and ML: guarantors of integrity of entire study. BZ, SY, ZF, and ML: literature research. BZ, SY, FH, and YJ: clinical studies. BZ and ML: statistical analysis. BZ, SZ, SL, and ML: manuscript editing. All authors have study concepts/study design or data acquisition or data analysis/interpretation, manuscript drafting or manuscript revision for important intellectual content, approval of final version of submitted manuscript, and agreed to ensure any questions related to the work are appropriately resolved.
This work was supported by the Construction Research Project of the Key Laboratory (Cultivation) of Chinese Academy of Medical Sciences (2019PT310025), the National Natural Science Foundation of China (81971588), the Youth Key Program of High-level Hospital Clinical Research (2022-GSP-QZ-5), the Capital Health Research and Development of Special (2020-2-4034), and the Clinical and Translational Fund of Chinese Academy of Medical Sciences (2019XK320063). The Capital Health Research and Development of Special Fund (2022-1-4032, SL).
The authors declare that the research was conducted in the absence of any commercial or financial relationships that could be construed as a potential conflict of interest.
All claims expressed in this article are solely those of the authors and do not necessarily represent those of their affiliated organizations, or those of the publisher, the editors and the reviewers. Any product that may be evaluated in this article, or claim that may be made by its manufacturer, is not guaranteed or endorsed by the publisher.
The Supplementary Material for this article can be found online at: https://www.frontiersin.org/articles/10.3389/fcvm.2022.917026/full#supplementary-material
Supplementary Figure 1 | Bland–Altman analysis for intra-observer reproducibility of strain and strain rate (the blue line indicates the mean value; the dashed red line indicates 95% CI).
Supplementary Figure 2 | Bland–Altman analysis for inter-observer reproducibility of strain and strain rate (the blue line indicates the mean value; the dashed red line indicates 95% CI).
1. Hoffman JI, Kaplan S. The incidence of congenital heart disease. J AmColl Cardiol. (2002) 39:1890–900. doi: 10.1016/S0735-1097(02)01886-7
2. Mercer-Rosa L, Yang W, Kutty S, Rychik J, Fogel M, Goldmuntz E. Quantifying pulmonary regurgitation and right ventricular function in surgically repaired tetralogy of Fallot: a comparative analysis of echocardiography and magnetic resonance imaging. Circ Cardiovasc Imaging. (2012) 5:637–43. doi: 10.1161/CIRCIMAGING.112.972588
3. Mouws EMJP, de Groot NMS, van de Woestijne PC, de Jong PL, Helbing WA, van Beynum IM, et al. Tetralogy of Fallot in the current era. Semin Thorac Cardiovasc Surg. (2019) 31:496–504. doi: 10.1053/j.semtcvs.2018.10.015
4. Stout KK, Daniels CJ, Aboulhosn JA, Bozkurt B, Broberg CS, Colman JM, et al. 2018 AHA/ACC Guideline for the management of adults with congenital heart disease: a report of the American college of cardiology/American heart association task force on clinical practice guidelines. Circulation. (2018) 139:e698–800. doi: 10.1161/CIR.0000000000000602
5. Baumgartner H, De Backer J, Babu-Narayan SV, Budts W, Chessa M, Diller GP, et al. 2020 ESC Guidelines for the management of adult congenital heart disease. Eur Heart J. (2021) 42:563–645. doi: 10.15829/1560-4071-2021-4702
6. Sabate Rotes A, Bonnichsen CR, Reece CL, Connolly HM, Burkhart HM, Dearani JA, et al. Long-term follow-up in repaired tetralogy of fallot: can deformation imaging help identify optimal timing of pulmonary valve replacement? J Am Soc Echocardiogr. (2014) 27:1305–10. doi: 10.1016/j.echo.2014.09.012
7. Buss SJ, Breuninger K, Lehrke S, Voss A, Galuschky C, Lossnitzer D, et al. Assessment ofmyocardial deformation with cardiac magnetic resonance strain imaging improves risk stratification in patients with dilated cardiomyopathy. Eur Heart J Cardiovasc Imaging. (2015) 16:307–15. doi: 10.1093/ehjci/jeu181
8. De Siqueira MEM, Pozo E, Fernandes VR, Sengupta PP, Modesto K, Gupta SS, et al. Characterization and clinical significance of right ventricular mechanics in pulmonary hypertension evaluated with cardiovascular magnetic resonance feature tracking. J Cardiovasc Magn Reson. (2016) 18:1–12. doi: 10.1186/s12968-016-0258-x
9. Kraigher-Krainer E, Shah AM, Gupta DK, Santos A, Claggett B, Pieske B, et al. Impaired systolic function by strain imaging in heart failure with preserved ejection fraction. J Am Coll Cardiol. (2014) 63:447–56. doi: 10.1016/j.jacc.2013.09.052
10. Menting ME, van den Bosch AE, McGhie JS, Eindhoven JA, Cuypers JA, Witsenburg M, et al. Assessment of ventricular function in adults with repaired Tetralogy of Fallot using myocardial deformation imaging. Eur Heart J Cardiovasc Imaging. (2015) 16:1347–57. doi: 10.1093/ehjci/jev090
11. Jing L, Wehner GJ, Suever JD, Charnigo RJ, Alhadad S, Stearns E, et al. Left and right ventricular dyssynchrony and strains from cardiovascular magnetic resonance feature tracking do not predict deterioration of ventricular function in patients with repaired tetralogy of Fallot. J Cardiovasc Magn Reson. (2016) 18:49. doi: 10.1186/s12968-016-0268-8
12. Schmidt B, Dick A, Treutlein M, Schiller P, Bunck AC, Maintz D, et al. Intra- and inter-observer reproducibility of global and regional magnetic resonance feature tracking derived strain parameters of the left and right ventricle. Eur J Radiol. (2017) 89:97–105. doi: 10.1016/j.ejrad.2017.01.025
13. Heng EL, Gatzoulis MA, Uebing A, Sethia B, Uemura H, Smith GC, et al. Immediate and midterm cardiac remodeling after surgical pulmonary valve replacement in adults with repaired tetralogy of Fallot: a prospective cardiovascular magnetic resonance and clinical study. Circulation. (2017) 136:1703–13. doi: 10.1161/CIRCULATIONAHA.117.027402
14. Monti CB, Secchi F, Capra D, Guarnieri G, Lastella G, Barbaro U, et al. Right ventricular strain in repaired tetralogy of Fallot with regards to pulmonary valve replacement. Eur J Radiol. (2020) 131:109235. doi: 10.1016/j.ejrad.2020.109235
15. Kutty S, Deatsman SL, Russell D, Nugent ML, Simpson PM, Frommelt PC, et al. Pulmonary valve replacement improves but does not normalize right ventricular mechanics in repaired congenital heart disease: a comparative assessment using velocity vector imaging. J Am Soc Echocardiogr. (2008) 21:1216–21. doi: 10.1016/j.echo.2008.08.009
16. He F, Feng Z, Chen Q, Jiao Y, Hua Z, Zhang H, et al. Whether pulmonary valve replacement in asymptomatic patients with moderate or severe regurgitation after tetralogy of fallot repair is appropriate: a case-control study. J Am Heart Assoc. (2019) 8:e010689. doi: 10.1161/JAHA.118.010689
17. Halliday BP, Baksi AJ, Gulati A, Ali A, Newsome S, Izgi C, et al. Outcome in dilated cardiomyopathy related to the extent, location, and pattern of late gadolinium enhancement. JACC Cardiovasc Imaging. (2019) 12(8 Pt. 2):1645–55. doi: 10.1016/j.jcmg.2018.07.015
18. Li ZL, He S, Xia CC, Peng WL, Li L, Liu KL, et al. Global longitudinal diastolic strain rate as a novel marker for predicting adverse outcomes in hypertrophic cardiomyopathy by cardiac magnetic resonance tissue tracking. Clin Radiol. (2021) 76:e19–78. doi: 10.1016/j.crad.2020.08.019
19. Stacey RB, Vera T, Morgan TM, Jordan JH, Whitlock MC, Hall ME, et al. Asymptomatic myocardial ischemia forecasts adverse events in cardiovascular magnetic resonance dobutamine stress testing of high-risk middle-aged and elderly individuals. J Cardiovasc Magn Reson. (2018) 20:75. doi: 10.1186/s12968-018-0492-5
20. Writing Committee Members, Shen WK, Sheldon RS, Benditt DG, Cohen MI, Forman DE, et al. 2017 ACC/AHA/HRS guideline for the evaluation and management of patients with syncope: a report of the American college of cardiology/American heart association task force on clinical practice guidelines and the heart rhythm society. Heart Rhythm. (2017) 14:e155–217. doi: 10.1016/j.hrthm.2017.03.004
21. Brignole M, Moya A, de Lange FJ, Deharo JC, Elliott PM, Fanciulli A, et al. 2018 ESC Guidelines for the diagnosis and management of syncope. Eur Heart J. (2018) 39:1883–948. doi: 10.5603/KP.2018.0161
22. Geva T. Indications for pulmonary valve replacement in repaired tetralogy of Fallot: the quest continues. Circulation. (2013) 128:1855–7. doi: 10.1161/CIRCULATIONAHA.113.005878
23. Zhuang B, Li S, Xu J, Zhou D, Yin G, Zhao S, et al. Age- and sex-specific reference values for atrial and ventricular structures in the validated normal chinese population: a comprehensive measurement by cardiac MRI. J Magn Reson Imaging. (2020) 52:1031–43. doi: 10.1002/jmri.27160
24. Zhuang B, Cui C, Sirajuddin A, He J, Wang X, Yue G, et al. Detection of myocardial fibrosis and left ventricular dysfunction with cardiac MRI in a hypertensive swine model. Radiol Cardiothorac Imaging. (2020) 2:e190214. doi: 10.1148/ryct.2020190214
25. van der Ven JPG, van den Bosch E, Bogers A, Helbing WA. Current outcomes and treatment of tetralogy of Fallot. F1000Res. (2019) 8:F1000 Faculty Rev-1530.
26. Scherptong RW, Mollema SA, Blom NA, Kroft LJ, de Roos A, Vliegen HW, et al. Right ventricular peak systolic longitudinal strain is a sensitive marker for right ventricular deterioration in adult patients with tetralogy ofFallot. Int J Cardiovasc Imaging. (2009) 25:669–76. doi: 10.1007/s10554-009-9477-7
27. Giardini A, Specchia S, Coutsoumbas G, Donti A, Formigari R, Fattori R, et al. Impact of pulmonary regurgitation and right ventricular dysfunction on oxygen uptake recovery kinetics in repaired tetralogy of Fallot. Eur J Heart Fail. (2006) 8:736–43. doi: 10.1016/j.ejheart.2006.01.012
28. Yim D, Mertens L, Morgan CT, Friedberg MK, Grosse-Wortmann L, Dragulescu A. Impact of surgical pulmonary valve replacement on ventricular mechanics in children with repaired tetralogy of Fallot. Int J Cardiovasc Imaging. (2017) 33:711–20. doi: 10.1007/s10554-016-1046-2
29. Jang W, Kim YJ, Choi K, Lim HG, Kim WH, Lee JR. Mid-term results of bioprosthetic pulmonary valve replacement in pulmonary regurgitation after tetralogy of Fallot repair. Eur J Cardio Thorac Surg. (2012) 42:e1–8. doi: 10.1093/ejcts/ezs219
30. Dorobantu DM, Sharabiani MTA, Taliotis D, Parry AJ, Tulloh RMR, Bentham JR, et al. Age over 35 years is associated with increased mortality after pulmonary valve replacement in repaired tetralogy of Fallot: results from the UK national congenital heart disease audit database. Eur J Cardiothorac Surg. (2020) 58:825–31. doi: 10.1093/ejcts/ezaa069
31. Geva T, Mulder B, Gauvreau K, Babu-Narayan SV, Wald RM, Hickey K, et al. Preoperative predictors of death and sustained ventricular tachycardia after pulmonary valve replacement in patients with repaired tetralogy of Fallot enrolled in the INDICATOR Cohort. Circulation. (2018) 138:2106–15. doi: 10.1161/CIRCULATIONAHA.118.034740
32. Scherptong RW, Hazekamp MG, Mulder BJ, Wijers O, Swenne CA, van der Wall EE, et al. Follow-up after pulmonary valve replacement in adults with tetralogy of Fallot: association between QRS duration and outcome. J Am Coll Cardiol. (2010) 56:1486–92. doi: 10.1016/j.jacc.2010.04.058
33. Hwang TW, Kim SO, Kim MS, Jang SI, Kim SH, Lee SY, et al. Short-Term change of exercise capacity in patients with pulmonary valve replacement after tetralogy of Fallot repair. Korean Circ J. (2017) 47:254–62. doi: 10.4070/kcj.2016.0226
34. Babu-Narayan SV, Diller GP, Gheta RR, Bastin AJ, Karonis T, Li W, et al. Clinical outcomes of surgical pulmonary valve replacement after repair of tetralogy of Fallot and potential prognostic value of preoperative cardiopulmonary exercise testing. Circulation. (2014) 129:18–27. doi: 10.1161/CIRCULATIONAHA.113.001485
35. Sabate Rotes A, Eidem BW, Connolly HM, Bonnichsen CR, Rosedahl JK, Schaff HV, et al. Long-term follow-up after pulmonary valve replacement in repaired tetralogy of Fallot. Am J Cardiol. (2014) 114:901–8. doi: 10.1016/j.amjcard.2014.06.023
36. Bokma JP, Winter MM, Oosterhof T, Vliegen HW, van Dijk AP, Hazekamp MG, et al. Severe tricuspid regurgitation is predictive for adverse events in tetralogy of Fallot. Heart. (2015) 101:794–9. doi: 10.1136/heartjnl-2014-306919
37. Morton G, Schuster A, Jogiya R, Kutty S, Beerbaum P, Nagel E. Inter-study reproducibility of cardiovascular magnetic resonance myocardial feature tracking. J Cardiovasc Magn Reson. (2012) 14:43. doi: 10.1186/1532-429X-14-43
Keywords: strain rate, strain, cardiac magnetic resonance imaging, pulmonary valve replacement, repaired tetralogy of Fallot
Citation: Zhuang B, Yu SQ, Feng Z, He F, Jiang Y, Zhao S, Lu M and Li S (2022) Left ventricular strain derived from cardiac magnetic resonance can predict outcomes of pulmonary valve replacement in patients with repaired tetralogy of Fallot. Front. Cardiovasc. Med. 9:917026. doi: 10.3389/fcvm.2022.917026
Received: 10 April 2022; Accepted: 26 July 2022;
Published: 18 August 2022.
Edited by:
Michail Papafaklis, University Hospital of Ioannina, GreeceReviewed by:
Alexandros Kasiakogias, Royal Brompton Hospital, United KingdomCopyright © 2022 Zhuang, Yu, Feng, He, Jiang, Zhao, Lu and Li. This is an open-access article distributed under the terms of the Creative Commons Attribution License (CC BY). The use, distribution or reproduction in other forums is permitted, provided the original author(s) and the copyright owner(s) are credited and that the original publication in this journal is cited, in accordance with accepted academic practice. No use, distribution or reproduction is permitted which does not comply with these terms.
*Correspondence: Minjie Lu, Y29vbGthbkAxNjMuY29t; Shoujun Li, ZHJsaXNob3VqdW5md0AxNjMuY29t
Disclaimer: All claims expressed in this article are solely those of the authors and do not necessarily represent those of their affiliated organizations, or those of the publisher, the editors and the reviewers. Any product that may be evaluated in this article or claim that may be made by its manufacturer is not guaranteed or endorsed by the publisher.
Research integrity at Frontiers
Learn more about the work of our research integrity team to safeguard the quality of each article we publish.