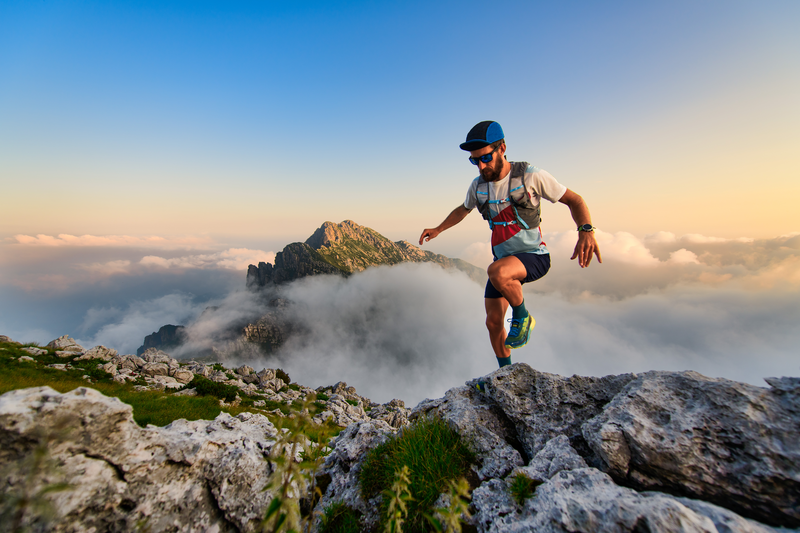
94% of researchers rate our articles as excellent or good
Learn more about the work of our research integrity team to safeguard the quality of each article we publish.
Find out more
ORIGINAL RESEARCH article
Front. Cardiovasc. Med. , 05 July 2022
Sec. Heart Failure and Transplantation
Volume 9 - 2022 | https://doi.org/10.3389/fcvm.2022.915918
Background: We sought to explore the significance of resting cardiac power/mass in predicting adverse outcome in patients with heart failure with preserved ejection fraction (HFpEF).
Methods: This prospective cohort study included patients with HFpEF and without significant valve disease or right ventricular dysfunction. Cardiac power was normalized to left ventricular (LV) mass and expressed in W/100 g of LV myocardium. Multivariate Cox regression analysis was used to evaluate the association between resting cardiac power/mass and composite endpoint, which included all-cause mortality and heart failure (HF) hospitalization.
Results: A total of 2,089 patients were included in this study. After an average follow-up of 4.4 years, 612 (29.30%) patients had composite endpoint, in which 331 (15.84%) died and 391 (18.72%) experienced HF hospitalization. In multivariate Cox regression analysis, resting power/mass < 0.7 W/m2 was independently associated with composite endpoint, all-cause mortality, cardiovascular mortality and HF hospitalization, with hazard ratios (HR) of 1.309 [95% confidence interval (CI): 1.108–1.546, P = 0.002], 1.697 (95%CI: 1.344–2.143, P < 0.001), 2.513 (95%CI: 1.711–3.689, P < 0.001), and 1.294 (95%CI: 1.052–1.592, P = 0.015), respectively. For composite endpoint, cardiovascular mortality and HF hospitalization, the C statistic increased significantly when incorporating resting cardiac power/mass into a model with established risk factors. For composite endpoint, the continuous net reclassification index after adding resting cardiac power/mass in the original model with N-terminal pro-brain natriuretic peptide was 13.1% (95%CI: 2.9–21.6%, P = 0.007), and the integrated discrimination index was 1.9% (95%CI: 0.8–3.2%, P < 0.001).
Conclusion: Resting cardiac power determined by non-invasive echocardiography is independently associated with the risk of adverse outcomes in HFpEF patients and provides incremental prognostic information.
Chronic heart failure (HF), characterized by decreased cardiac systolic and/or diastolic function, is a primary cause of morbidity and mortality worldwide (1). A primary concern in HF management lies in accurately and comprehensively evaluating cardiac function, especially left ventricular (LV) function, as the left ventricle is responsible for most of the physiological functions of the heart. When evaluating LV function, clinicians usually adopt the traditional indexes including LV ejection fraction (EF) and cardiac output. Although well known, these measurements may fail to provide a comprehensive assessment of LV function. In addition, nearly half of all HF patients have preserved EF (namely HFpEF) (2). Thus, EF alone seems inadequate to identify high-risk individuals among HF patients.
Cardiac power refers to the rate at which the heart pumps blood out and delivers it to the periphery (3). Thus, it is an integrative and quantitative indicator of overall cardiac performance that combines cardiac pressure and volume loads. Normally, 3 mmHg of right atrial pressure, 120/80 mmHg of arterial pressure, and 5 L/min of cardiac output produce ∼1 W of resting cardiac power. Some studies have shown that higher resting and peak cardiac power are associated with a lower mortality in patients with chronic and advanced HF (4, 5). Furthermore, a retrospective study conducted by Anand et al. (6) showed that in patients with normal EF and no HF undergoing exercise stress echocardiography, higher cardiac power was independently associated with a lower prevalence of complications, as well as a lower risk of all-cause mortality and the incidence of subsequent HF, suggesting the potential prognostic value of cardiac power.
Whereas cardiac power has been well studied in HF patients with reduced EF (HFrEF) and in those with normal EF but no HF (4–7), its significance and role in predicting adverse outcomes in patients with HFpEF remains unclear. Thus, in this study we aimed to evaluate the role of cardiac power indexed to LV mass in patients with stable HFpEF.
This study was approved by the Ethics Board of the No. 988 Hospital of Joint Logistic Support Force of the Chinese PLA and was conducted in line with the ethical guidelines of the 1975 Declaration of Helsinki. Written informed consent was obtained from each patient. The datasets used and/or analyzed during the current study are available from the corresponding author on reasonable request.
This prospective cohort study recruited patients who were hospitalized in the Cardiology Department of the No. 988 Hospital of Joint Logistic Support Force of the Chinese PLA (Zhengzhou, China) from April 2012 to December 2021. We used the following criteria to select HFpEF patients: those with (i) history of HF hospitalization; (ii) HF syndromes and/or signs, (iii) EF ≥ 50%, and (iv) N-terminal pro-brain natriuretic peptide (NT-proBNP) ≥ 125 pg/mL. Additionally, eligible HFpEF patients were required to be stable and well-compensated without medication changes for at least 6 weeks prior to enrollment. Patients who had one of the following conditions were excluded: EF < 50% at rest, enlarged right ventricle, significant valve disease (≥ moderate stenosis or regurgitation, prosthetic valve replacement, surgical or percutaneous valve repair, rheumatic valve disease), hospitalization for uncompensated HF or unstable coronary heart disease in the prior 6 weeks, heart transplant, metastatic malignant tumor, severe liver disease or receiving palliative care. Via electronic medical records, we collected patient detailed medical history, baseline clinical characteristics, laboratory indexes and echocardiographic parameters.
Cardiac power normalized by LV mass at rest was calculated by the following formulas, in which 0.222 is the conversion constant to W/100 g of LV myocardium: resting cardiac power/mass = 0.222 × cardiac output × mean blood pressure (BP)/LV mass; cardiac output = stroke volume × heart rate; mean BP = diastolic BP + 1/3 × systolic BP (5). In each patient, stroke volume was calculated from the product of EF (biplane modified Simpson’s method) and LV end-diastolic volume by 2D echocardiography, assuming normal LV geometry, which is a reasonable assumption in the situation of normal EF, no right ventricular dysfunction, and no significant valvular heart disease (6). The following formula was used to calculate LV mass: LV mass (g) = 0.8 × 1.04 × [(interventricular septal thickness + LV end diastolic diameter + LV posterior wall thickness)3 - (LV end diastolic diameter)3] + 0.6 (8). Indexing each measure for body surface area is not needed since their ratio takes care of this normalization.
To evaluate the severity of patient comorbidities, we defined comorbidity score as the number of patient comorbidities referring to the Charlson Comorbidity Index (CCI) (9). For the present study, we included the comorbidities that score 1 point in the CCI (myocardial infarction, peripheral vascular disease, cerebrovascular disease, dementia, chronic lung disease, connective tissue disease, ulcer disease, mild liver disease and diabetes), as well as anemia, hypertension and atrial fibrillation, and excluded those comorbidities scoring ≥ 2 points in CCI (such as severe liver disease, metastatic tumors and leukemia). As our analysis was entirely among HFpEF patients, the 1 point added for HF was not included in the final number of comorbidities.
Until December 31, 2021, all patients were followed up via telephone or medical record every 6 months for the composite endpoint which consisted of all-cause mortality or HF hospitalization, and the causes of death was also recorded. Furthermore, we contacted the attending physician of each patient who had an event to reconfirm their outcome. For patients who did not have an event, survival time was defined as the period from the day of physical examination to the last date of follow-up.
Categorical variables are presented as frequencies (%), and continuous variables are presented as the mean ± standard deviation or median (interquartile range). Differences between groups were evaluated by the chi-squared test for categorical variables and Student’s t-test or the Mann–Whitney U-test for continuous variables, as appropriate.
We log-transformed (log10) NT-proBNP, and used the median values of resting cardiac power/mass and log NT-proBNP as cutoffs. The log-rank test was used to compare survival times on Kaplan–Meier curves across different groups. The prognostic value of resting cardiac power/mass was evaluated by using a Cox proportional hazards model adjusted for the following covariates: age, gender, body mass index (BMI, calculated by weight divided by the square of height), New York Heart Association (NYHA) class, LVEF, comorbidity score, estimated glomerular filtration rate (eGFR, calculated by a modified Modification of Diet in Renal Disease equation), angiotensin-converting enzyme inhibitors (ACEI)/angiotensin receptor antagonists (ARB), beta blockers and aldosterone antagonists. The prognostic discrimination of resting cardiac power/mass was assessed by comparing the incremental improvement of the Harrell’s C statistic, as well as the integrated discrimination improvement (IDI) and the continuous net reclassification improvement (NRI) at the event rate. Sensitivity analysis was further performed to explore the association between resting cardiac power/mass (as a continuous variable) and all-cause mortality and HF hospitalization among the following subgroups: age (< 75 or ≥ 75 years), BMI (< 18.5 kg/m2, 18.5–23.9 kg/m2, 24–27.9 kg/m2 or ≥ 28 kg/m2), NYHA class (class I, II, III or IV), comorbidity score (0–3 or ≥ 4), eGFR (< 60 mL/min/1.73 m2 or ≥ 60 mL/min/1.73 m2) and log NT-proBNP (< 2.5 or ≥ 2.5). R software version 4.0.3 (Institute for Statistics and Mathematics, Vienna, Austria1) and SPSS 26.0 software (IBM Corporation, Armonk, NY, United States) were used to perform statistical analyses. A P-value < 0.05 was considered significant.
Exclusion of ineligible patients produced a final cohort of 2089 HFpEF patients. Baseline measurements of resting cardiac power/mass were available for the 2089 patients. Detailed baseline characteristics are shown in Table 1. The median value of resting cardiac power/mass was 0.7 W/m2, which was used as a cutoff value for grouping. Compared with patients with resting cardiac power/mass ≥ 0.7 W/m2, those with resting cardiac power/mass < 0.7 W/m2 were older, had higher NT-proBNP, more comorbidities and larger percentage of NYHA class IV, ischemic heart disease and atrial fibrillation, used more cardiovascular medications, while had lower eGFR. We listed the echocardiography parameters of patients in Supplementary Table 1, which showed that compared with patients with resting cardiac power/mass ≥ 0.7 W/m2, those with resting cardiac power/mass < 0.7 W/m2 had larger interventricular septal thickness, left ventricular posterior wall thickness, tricuspid regurgitation velocity, left ventricular end systolic volume, left ventricular mass index, and relative wall thickness (all P < 0.05), besides, the former also had larger left atrial volume index than the latter, though without statistical significance (P = 0.068).
The 2089 HFpEF patients were followed up for 4.4 years on average, with no patients lost to follow-up. Among them, 612 (29.30%) patients experienced composite endpoint, in which 331 (15.84%) experienced death from any cause, 147 (7.04%) died from cardiovascular causes, and 391 (18.72%) experienced HF hospitalization. Of these, 357 (34.97%) patients who had composite endpoint, 216 (65.26%) patients who died and 225 (57.54%) patients hospitalized with HF had resting cardiac power/mass values below 0.7 W/m2.
Kaplan–Meier curves of the incidences of adverse outcomes are presented in Figures 1, 2 across different category methods. The incidences of adverse outcomes in patients with resting cardiac power/mass < 0.7 W/m2 were significantly higher than those with resting cardiac power/mass ≥ 0.7 W/m2 (each log-rank P < 0.001, Figure 1). To compare the outcomes of patients with different levels of cardiac power/mass and NT-proBNP, we divided the cohort into four groups based upon the median values of resting cardiac power/mass and log NT-proBNP (Figure 2). Patients with either a lower resting cardiac power/mass or higher NT-proBNP had an increased risk of meeting either endpoint compared with the reference group that had higher resting cardiac power/mass and lower NT-proBNP (P < 0.001).
Figure 1. Kaplan–Meier survival curves for prediction of composite endpoint (A), all-cause mortality (B), cardiovascular mortality (C) and heart failure hospitalization (D) in patients with higher resting cardiac power/mass (≥ 0.7 W/m2) and lower resting cardiac power/mass (< 0.7 W/m2).
Figure 2. Kaplan–Meier survival curves for prediction of composite endpoint (A), all-cause mortality (B), cardiovascular mortality (C) and heart failure hospitalization (D) according to resting cardiac power/mass and NT-proBNP levels.
After adjustment for commonly recognized risk factors (age, gender, BMI, NYHA class, LVEF, comorbidity score, eGFR, use of ACEI/ARB, beta blocker and aldosterone antagonist, log NT-proBNP), in multivariate analysis, resting cardiac power/mass < 0.7 W/m2 was independently associated with the incidence of composite endpoint, all-cause mortality, cardiovascular mortality and HF hospitalization, with hazard ratios (HR) of 1.309 [95% confidence interval (CI): 1.108–1.546, P = 0.002], 1.697 (95%CI: 1.344–2.143, P < 0.001), 2.513 (95%CI: 1.711–3.689, P < 0.001), and 1.294 (95%CI: 1.052–1.592, P = 0.015), respectively (Table 2).
We further explored the predictive value of resting cardiac power/mass by C-index (Table 3). The individual addition of log NT-proBNP or resting cardiac power/mass in the model significantly improved the C statistic for predicting composite endpoint, cardiovascular mortality, and HF hospitalization. Also, the two indicators significantly increased the C statistic for predicting adverse outcomes when they were incorporated into the established model.
For composite endpoint, the continuous NRI after adding resting cardiac power/mass in the original model with N-terminal pro-brain natriuretic peptide was 13.1% (95%CI: 2.9–21.6%, P = 0.007), and the IDI was 1.9% (95%CI: 0.8–3.2%, P < 0.001). For all-cause mortality, the continuous NRI after the addition of resting cardiac power/mass in the model with established risk factors and NT-proBNP was 17.0% (95%CI: 11.4–28.3%, P = 0.040), and the IDI was 2.3% (95%CI: 0.7–8.7%, P = 0.020). For cardiovascular mortality, the continuous NRI after the addition of resting cardiac power/mass in the model with established risk factors and NT-proBNP was 33.1% (95%CI: 4.9–55.3%, P = 0.007), and the IDI was 7.5% (95%CI: 2.2–14.4%, P < 0.001). For HF hospitalization, the continuous NRI after the addition of resting cardiac power/mass in the model with established risk factors and NT-proBNP was 6.0% (95%CI: 4.7–15.2%, P = 0.026), and the IDI was 1.7% (95%CI: 1.2–4.3%, P = 0.007). We additionally compared the predictive value between NT-proBNP and resting cardiac power/mass, and listed the results in Supplementary Table 2, which showed that the addition of rest cardiac power/mass improved the predictive value of the model based on NT-proBNP.
Resting cardiac power/mass was independently associated with all-cause mortality across most patient subgroups (Figure 3), including the groups of age ≥ 75 years, BMI ≥ 28 kg/m2, NYHA class II– IV, comorbidity score 0–3 and ≥ 4, eGFR ≥ 60 mL/min/1.73 m2, and log NT-proBNP < 2.5 and ≥ 2.5. Similarly, it presented independent prognostic value for HF hospitalization in multiple subsets (Figure 4), including the groups of age ≥ 75 and < 75 years, BMI < 18.5,18.5–23.9 and ≥ 28 kg/m2, NYHA class II– IV, comorbidity score 0–3 and ≥ 4, eGFR ≥ 60 mL/min/1.73 m2, and log NT-proBNP < 2.5 and ≥ 2.5. Combined with the results shown in Table 2, the sensitivity analyses indicate that resting cardiac power/mass is an independent predictor for these adverse outcomes in patients with stable HFpEF.
Figure 3. Resting cardiac power/mass for the prediction of all-cause mortality: subgroup analysis. The prognostic value of resting cardiac power/mass is considered in several patient subgroups, after adjustment for age, gender, BMI, NYHA class, LVEF, comorbidity score, eGFR, log NT-proBNP, ACEI/ARB, beta blocker, and aldosterone antagonist. Other abbreviations as in Table 1.
Figure 4. Resting cardiac power/mass for the prediction of heart failure hospitalization: subgroup analysis. The prognostic value of resting cardiac power/mass is considered in several patient subgroups, after adjustment for age, gender, BMI, NYHA class, LVEF, comorbidity score, eGFR, log NT-proBNP, ACEI/ARB, beta blocker, and aldosterone antagonist. Other abbreviations as in Table 1.
In the present study, we comprehensively investigated the potential prognostic role of cardiac power estimated by non-invasive echocardiography in patients with stable HFpEF. In this study, we found that (i) cardiac power normalized to LV mass at rest was independently associated with adverse outcomes in patients with HFpEF, and that (ii) incorporating resting cardiac power/mass (reflective of comprehensive cardiac function) and NT-proBNP (indicative of myocardial stretch) into a model with established risk factors enhanced the prognostic value for those endpoints.
As is well known, LV diastolic dysfunction plays a fundamental and predominant role in the pathophysiology of HFpEF (10) and elevates filling pressure, which further promotes dyspnea, impairs exercise capacity, and increases mortality and incidence of HF hospitalization (11). Non-invasive echocardiography is an indirect approach to measuring LV diastolic function. Due to its safety and rapidity, echocardiography is usually preferred for clinicians before performing invasive right heart catheterization. However, individual parameters of echocardiography, such as left atrial volume index and the mitral annular diastolic velocity, have their own limitations and may fail to accurately determine the degree as well as the severity of LV dysfunction. Moreover, a combination of these parameters undoubtedly increases the work burden on clinical staff and is not conducive to clinical application.
Despite having a “preserved” EF, patients with HFpEF nonetheless experience abnormalities in LV systolic performance (12, 13). Multiple studies have demonstrated that patients with HFpEF present with subtle impairments in systolic function at rest, and this alteration tends to increase during exertion, which impairs LV suction, decreases cardiac output, and elevates LV filling pressures (14, 15). LV systolic dysfunction in HFpEF also predicts increased risk of adverse outcomes (16, 17). Some scholars question the ability of EF to truly reflect the LV systolic function of HFpEF (18). HFpEF is often complicated with myocardial concentric hypertrophy, which inevitably generates a normal or supernormal EF, even when stroke volume has declined. Therefore, a non-invasive method that can quantify LV pump function better than EF would be a major step forward.
Cardiac power is a comprehensive quantitative indicator that can be used to evaluate cardiac function via non-invasive echocardiography (4, 19). It is superior to variables such as cardiac output because it covers both pressure load and volume load (20). Because cardiac power depends on the volume of the muscle that produces the power, standardizing cardiac power with LV mass can improve the applicability of this indicator in different populations (4, 5, 19) and facilitate comparison between individuals. In addition, cardiac power may be more promising than EF as predictor of mortality in severe heart failure. It has been reported to be the strongest predictor of in-hospital mortality in patients with cardiac shock resulting from acute myocardial infarction (20), and has recently been used to evaluate the response of patients with advanced HF to mechanical circulatory support systems (21).
In the present study, we found that the HFpEF patients with lower resting cardiac power/mass were more likely to be older, used more cardiovascular medications, have higher NYHA class and NT-proBNP level, as well as more comorbidities, indicating that the patients in this subgroup might have poorer health at baseline and more risk factors for adverse outcomes. After adjustment for multiple covariates, such as age and comorbidities, our study brought new evidence that resting cardiac power/mass is independently associated with composite endpoint, all-cause mortality, cardiovascular mortality, and HF hospitalization in patients with HFpEF. Meanwhile, resting cardiac power/mass significantly promoted the prediction efficiency of both traditional risk factors and NT-proBNP, supporting a pathophysiological link between reduced cardiac performance and the mortality and HF progression later in life as aging and comorbidities advance. Furthermore, the results of our sensitivity analysis showed that although not all subgroups showed a statistically significant association, the risk of adverse outcomes within these subgroups was higher in patients with lower resting cardiac power/mass than in those with higher resting cardiac power/mass, indicating the stable and independent prediction efficacy of resting cardiac power/mass among HFpEF patients. Clinically, resting cardiac power/mass is easily obtained by measuring blood pressure and stroke volume, the latter of which can be measured by Doppler echocardiography. Intriguingly, the technical setup used to determine cardiac power is very similar to that used in a standard diastolic stress test, so determining whether integrated application of the two tests may provide incremental diagnostic or prognostic significance in HFpEF patients deserves further exploration.
Our study has some limitations: first, it should be noted that this was a single-center study and 95.79% of our patients were male because we included patients from the veteran population, thus we failed to observe the association between rest cardiac power and adverse outcomes in gender subgroup, and our conclusion might be more suitable for male patients. We further conducted Cox proportional hazard model and adjusted gender, age and other covariates, and the results were still significant, suggesting that rest cardiac power/mass predicts the adverse outcomes in HFpEF patients independent of gender, therefore the results of this study were still representative to some extent, and studies conducted with more female patients are needed to validate our findings. Secondly, compared with invasive measurements, the non-invasive measurements of stroke volume may be more inclined to error. Thirdly, this study failed to perform speckle tracking echocardiography and thus lacked the information of global longitudinal strain, which helps determine the impaired systolic function in patients with normal ejection fraction and should be fully considered in the further studies. Last, the prognostic value of peak or reserved cardiac power indexed to LV mass has not been evaluated in patients with HFpEF.
This study explored the association between resting cardiac power/mass and the risk of adverse outcomes in patients with HFpEF, finding that lower resting cardiac power/mass is an independent predictor of these adverse outcomes and also has incremental prognostic value over established risk factors and NT-proBNP. Cardiac power as an integrated indicator of cardiac performance may be considered for risk stratification of long-term adverse outcomes in patients with HFpEF. This measurement provides more comprehensive and accurate guidance for treatment and prognostic evaluation of patients with HFpEF, and further promotes the integration and optimization of cardiac function monitoring indicators.
The original contributions presented in this study are included in the article/Supplementary Material, further inquiries can be directed to the corresponding author.
This study was approved by the Ethics Board of the No. 988 Hospital of Joint Logistic Support Force of the Chinese PLA. The patients/participants provided their written informed consent to participate in this study.
SW designed the protocol, provided methodological expertise, drafted the manuscript and performed statistical analyses. AC and XD supervised patient recruitment and study procedures and conducted study procedures. All authors read and approved the final manuscript.
The authors declare that the research was conducted in the absence of any commercial or financial relationships that could be construed as a potential conflict of interest.
All claims expressed in this article are solely those of the authors and do not necessarily represent those of their affiliated organizations, or those of the publisher, the editors and the reviewers. Any product that may be evaluated in this article, or claim that may be made by its manufacturer, is not guaranteed or endorsed by the publisher.
We thank John Daniel from Liwen Bianji (Edanz) (www.liwenbianji.cn/ac) for editing the English text of a draft of this manuscript. This manuscript has been preprinted (DOI: 10.21203/rs.3.rs-430111/v1) (22).
The Supplementary Material for this article can be found online at: https://www.frontiersin.org/articles/10.3389/fcvm.2022.915918/full#supplementary-material
1. Steinberg BA, Zhao X, Heidenreich PA, Peterson ED, Bhatt DL, Cannon CP, et al. Trends in patients hospitalized with heart failure and preserved left ventricular ejection fraction: prevalence, therapies, and outcomes. Circulation. (2012) 126:65–75. doi: 10.1161/circulationaha.111.080770
2. Redfield MM. Heart failure with preserved ejection fraction. N Engl J Med. (2016) 375:1868–77. doi: 10.1056/NEJMcp1511175
3. Nicholls DP, O’Dochartaigh C, Riley MS. Circulatory power–a new perspective on an old friend. Eur Heart J. (2002) 23:1242–5. doi: 10.1053/euhj.2002.3229
4. Cortigiani L, Sorbo S, Miccoli M, Scali MC, Simioniuc A, Morrone D, et al. Prognostic value of cardiac power output to left ventricular mass in patients with left ventricular dysfunction and dobutamine stress echo negative by wall motion criteria. Eur Heart J Cardiovasc Imaging. (2017) 18:153–8. doi: 10.1093/ehjci/jew073
5. Dini FL, Mele D, Conti U, Ballo P, Citro R, Menichetti F, et al. Peak power output to left ventricular mass: an index to predict ventricular pumping performance and morbidity in advanced heart failure. J Am Soc Echocardiogr. (2010) 23:1259–65. doi: 10.1016/j.echo.2010.08.030
6. Anand V, Kane GC, Scott CG, Pislaru SV, Adigun RO, McCully RB, et al. Prognostic value of peak stress cardiac power in patients with normal ejection fraction undergoing exercise stress echocardiography. Eur Heart J. (2021) 42:776–85. doi: 10.1093/eurheartj/ehaa941
7. Lang CC, Karlin P, Haythe J, Lim TK, Mancini DM. Peak cardiac power output, measured noninvasively, is a powerful predictor of outcome in chronic heart failure. Circ Heart Fail. (2009) 2:33–8. doi: 10.1161/circheartfailure.108.798611
8. Nagueh SF, Smiseth OA, Appleton CP, Byrd BF III, Dokainish H, Edvardsen T, et al. Recommendations for the evaluation of left ventricular diastolic function by echocardiography: an update from the American society of echocardiography and the European association of cardiovascular imaging. Eur Heart J Cardiovasc Imaging. (2016) 17:1321–60. doi: 10.1093/ehjci/jew082
9. Charlson ME, Pompei P, Ales KL, MacKenzie CR. A new method of classifying prognostic comorbidity in longitudinal studies: development and validation. J Chronic Dis. (1987) 40:373–83. doi: 10.1016/0021-9681(87)90171-8
10. Borlaug BA. The pathophysiology of heart failure with preserved ejection fraction. Nat Rev Cardiol. (2014) 11:507–15. doi: 10.1038/nrcardio.2014.83
11. Reddy YNV, Olson TP, Obokata M, Melenovsky V, Borlaug BA. Hemodynamic correlates and diagnostic role of cardiopulmonary exercise testing in heart failure with preserved ejection fraction. JACC Heart Fail. (2018) 6:665–75. doi: 10.1016/j.jchf.2018.03.003
12. Obokata M, Reddy YNV, Borlaug BA. Diastolic dysfunction and heart failure with preserved ejection fraction: understanding mechanisms by using noninvasive methods. JACC Cardiovasc Imaging. (2020) 13:245–57.
13. Abudiab MM, Redfield MM, Melenovsky V, Olson TP, Kass DA, Johnson BD, et al. Cardiac Output response to exercise in relation to metabolic demand in heart failure with preserved ejection fraction. Eur J Heart Fail. (2013) 15:776–85. doi: 10.1093/eurjhf/hft026
14. Reddy YNV, Andersen MJ, Obokata M, Koepp KE, Kane GC, Melenovsky V, et al. Arterial stiffening with exercise in patients with heart failure and preserved ejection fraction. J Am Coll Cardiol. (2017) 70:136–48. doi: 10.1016/j.jacc.2017.05.029
15. Borlaug BA, Kane GC, Melenovsky V, Olson TP. Abnormal right ventricular-pulmonary artery coupling with exercise in heart failure with preserved ejection fraction. Eur Heart J. (2016) 37:3293–302. doi: 10.1093/eurheartj/ehw241
16. Borlaug BA, Lam CSP, Roger VRL, Rodeheffer RJ, Redfield MM. Contractility and ventricular systolic stiffening in hypertensive heart disease insights into the pathogenesis of heart failure with preserved ejection fraction. J Am Coll Cardiol. (2009) 54:410–8. doi: 10.1016/j.jacc.2009.05.013
17. Shah AM, Claggett B, Sweitzer NK, Shah SJ, Anand IS, Liu L, et al. Prognostic importance of impaired systolic function in heart failure with preserved ejection fraction and the impact of spironolactone. Circulation. (2015) 132:402–14. doi: 10.1161/circulationaha.115.015884
18. Smiseth OA, Aalen JM, Skulstad H. Heart failure and systolic function: time to leave diagnostics based on ejection fraction? Eur Heart J. (2021) 42:786–8.
19. Pugliese NR, Fabiani I, Mandoli GE, Guarini G, Galeotti GG, Miccoli M, et al. Echo-derived peak cardiac power output-to-left ventricular mass with cardiopulmonary exercise testing predicts outcome in patients with heart failure and depressed systolic function. Eur Heart J Cardiovasc Imaging. (2019) 20:700–8. doi: 10.1093/ehjci/jey172
20. Fincke U, Hochman JS, Lowe AM, Menon V, Slater JN, Webb JG, et al. Cardiac power is the strongest hemodynamic correlate of mortality in cardiogenic shock: a report from the shock trial registry. J Am Coll Cardiol. (2004) 44:340–8. doi: 10.1016/j.jacc.2004.03.060
21. Rab T, Ratanapo S, Kern KB, Basir MB, McDaniel M, Meraj P, et al. Cardiac shock care centers: jacc review topic of the week. J Am Coll Cardiol. (2018) 72:1972–80. doi: 10.1016/j.jacc.2018.07.074
Keywords: cardiac power, heart failure with preserved ejection fraction, echocardiography, prognosis, adverse outcome
Citation: Wang S, Chen A and Duan X (2022) Resting Cardiac Power Predicts Adverse Outcome in Heart Failure Patients With Preserved Ejection Fraction: A Prospective Study. Front. Cardiovasc. Med. 9:915918. doi: 10.3389/fcvm.2022.915918
Received: 08 April 2022; Accepted: 20 June 2022;
Published: 05 July 2022.
Edited by:
Maria-Angela Losi, University of Naples Federico II, ItalyReviewed by:
Giovanna Gallo, Sapienza University of Rome, ItalyCopyright © 2022 Wang, Chen and Duan. This is an open-access article distributed under the terms of the Creative Commons Attribution License (CC BY). The use, distribution or reproduction in other forums is permitted, provided the original author(s) and the copyright owner(s) are credited and that the original publication in this journal is cited, in accordance with accepted academic practice. No use, distribution or reproduction is permitted which does not comply with these terms.
*Correspondence: Shiqi Wang, Y2pxd3NxQDEyNi5jb20=
Disclaimer: All claims expressed in this article are solely those of the authors and do not necessarily represent those of their affiliated organizations, or those of the publisher, the editors and the reviewers. Any product that may be evaluated in this article or claim that may be made by its manufacturer is not guaranteed or endorsed by the publisher.
Research integrity at Frontiers
Learn more about the work of our research integrity team to safeguard the quality of each article we publish.