- 1Department of Cardiology, Beijing Anzhen Hospital, Capital Medical University, Beijing, China
- 2Jiangxi Provincial People’s Hospital, The First Affiliated Hospital of Nanchang Medical College, Nanchang, China
- 3Department of Cardiology, Beijing Daxing Hospital, Capital Medical University, Beijing, China
This review aimed to summarize the evidence of elevated remnant cholesterol and the risks of atherosclerotic cardiovascular disease (ASCVD) and to search for further guidance in clinical therapy. The lipids-lowering treatments such as statins and ezetimibe targeted on low-density lipoprotein cholesterol (LDL-C) have always been the first-line therapy for ASCVD. However, even after statins or new lipid-lowering drugs lowered LDL-C to recommended concentrations, and with other risk factors well-controlled, such as high blood pressure, the risks of developing ASCVD remained. Remnant cholesterol (RC) referred to the cholesterol contained in all remnant lipoprotein particles, which was the cholesterol in the hydrolyzed very-low-density lipoprotein and intermediate-density lipoprotein in the fasting state, and the cholesterol in the chylomicron remnants in the postprandial state. Evidence from in vitro and animal pathogenic mechanisms studies, epidemiology, and genetic studies all indicated that RC played an important role in predicting the incidence of ASCVD. As a new indicator to reflect atherosclerosis, especially when LDL-C has been controlled to a recommended level, RC was considered as a priority treatment target for people at high risk of ASCVD. The use of statins, fibrates, APOC3 inhibitors, PCSK9 inhibitors, and omega-3 fatty acids to reduce RC levels in the plasma may provide long-term benefits. However, the standardized detection of RC was still controversial, and more studies on appropriate treatments of elevated RC are urgently needed. These positive trials may benefit more patients at high ASCVD risks worldwide in the future.
Introduction
Over the past years, atherosclerotic cardiovascular disease (ASCVD) has become a chronicle epidemic disease. Despite significant progress in the prevention and treatment of ASCVD, it remains the leading cause of death worldwide. The report on the global burden of ASCVD from 1990 to 2019 showed that in 2019, nearly 197 million people suffered from ASCVD, and 9.14 million people died from ischemic heart disease (IHD) (1). Dyslipidemia has always been a major causative factor in the incidence and progress of ASCVD. Moreover, high systolic blood pressure (54.6%), high low-density lipoprotein cholesterol (LDL-C) level (46.6%), smoking (23.9%), high blood glucose (23.8%), and obesity/overweight (22.7%) were the global top five leading influencing factors of the ASCVD disability-adjusted life year (DALY) (2). Thus, the lipids-lowering treatments such as statins and ezetimibe targeted on LDL-C have always been the first-line therapy for ASCVD. In recent years, compared with the 2016 European Society of Cardiology (ESC) guideline, the new guideline based on more research evidence has been greatly updated. For the blood lipids management in patients with ASCVD, more strict target recommendations should be given, such as LDL-C should be reduced to <1.4 mmol/L (55 mg/dl), and more than 50% compared with baseline (3, 4).
However, even after statins or new lipid-lowering drugs lowered LDL-C to recommended concentrations, and with other risk factors well-controlled, such as high blood pressure, the risks of developing ASCVD remained (5, 6). When LDL-C was less than 2.6 mmol/L, atherogenic dyslipidemia was characterized by: high levels of triglyceride (TG) (TG ≥1.70 mmol/L) and low levels of high-density lipoprotein cholesterol (HDL-C) (women <1.29 mmol/L, men <1.03 mmol/L), which were common in diabetes, obesity, metabolic syndrome, and ASCVD patients with renal failure disease (7). Elevated TG levels in plasma indicated an increase in chylomicron (CM) or very-low-density lipoprotein (VLDL) particles, also known as triglyceride-rich lipoproteins (TRLs) (8). The metabolized TRLs were also called triglyceride-rich remnant lipoprotein particles (RLPs), which were listed as an emerging atherogenic risk factor by the American Heart Association (AHA) in 2001 (9). There was evidence showing that it was the cholesterol components of RLPs, the remnant cholesterol (RC), rather than TG, that increased ASCVD risks (10). In this review, we defined RC as the cholesterol contents of a subset of the TRLs called remnants, i.e., CM remnants in the non-fasting state, and VLDL and intermediate-density lipoprotein (IDL) in the fasting state (11). The review aimed to summarize the evidence of elevated RC and the risks of ASCVD, and to search for further guidance in clinical therapy.
Metabolism and measurements of remnant cholesterol
The hydrophobic properties of triglycerides as well as its lipolysis products underlie the association between TG and atherosclerosis. Inefficient triglyceride transport has led to the accumulation of plasma TRLs and their partial lipolysis products, also known as remnants (12). RC referred to the cholesterol contained in RLPs particles, which was the cholesterol in the VLDL and IDL in the fasting state, and the cholesterol in the CM remnants in the postprandial state (13). Chylomicrons of different sizes needed to be converted into metabolic remnants, while VLDL needed to be metabolized to LDL to be cleaned (14). After being digested, CM and VLDL entered into the plasma and began to be lipolysis by lipoprotein lipase (LPL), which needed to be activated under the activation of apolipoprotein C II. As the research showed before, apoC-II and apoA-5 could promote the activity of LPL (15, 16), whereas apoC-I, apoC-III and apoE could inhibit LPL activity (17). The relative content of apoE and apoC-III could be the key determinant of granules undergoing receptor-mediated catabolism. Residual clearance was delayed in patients with apoE dysfunction [such as type III hyperlipidemia (18)], while apoC-III has been confirmed as an inhibitor of TRL clearance (19). After gradually losing TG, phospholipid, apolipoprotein A (APOA), and apolipoprotein C (APOC), CM remnants and VLDL remnant-like particles with higher density and smaller molecular weight were formed, respectively (20). As a product of VLDL hydrolysis, IDL was also a kind of RC (21) (Figure 1).
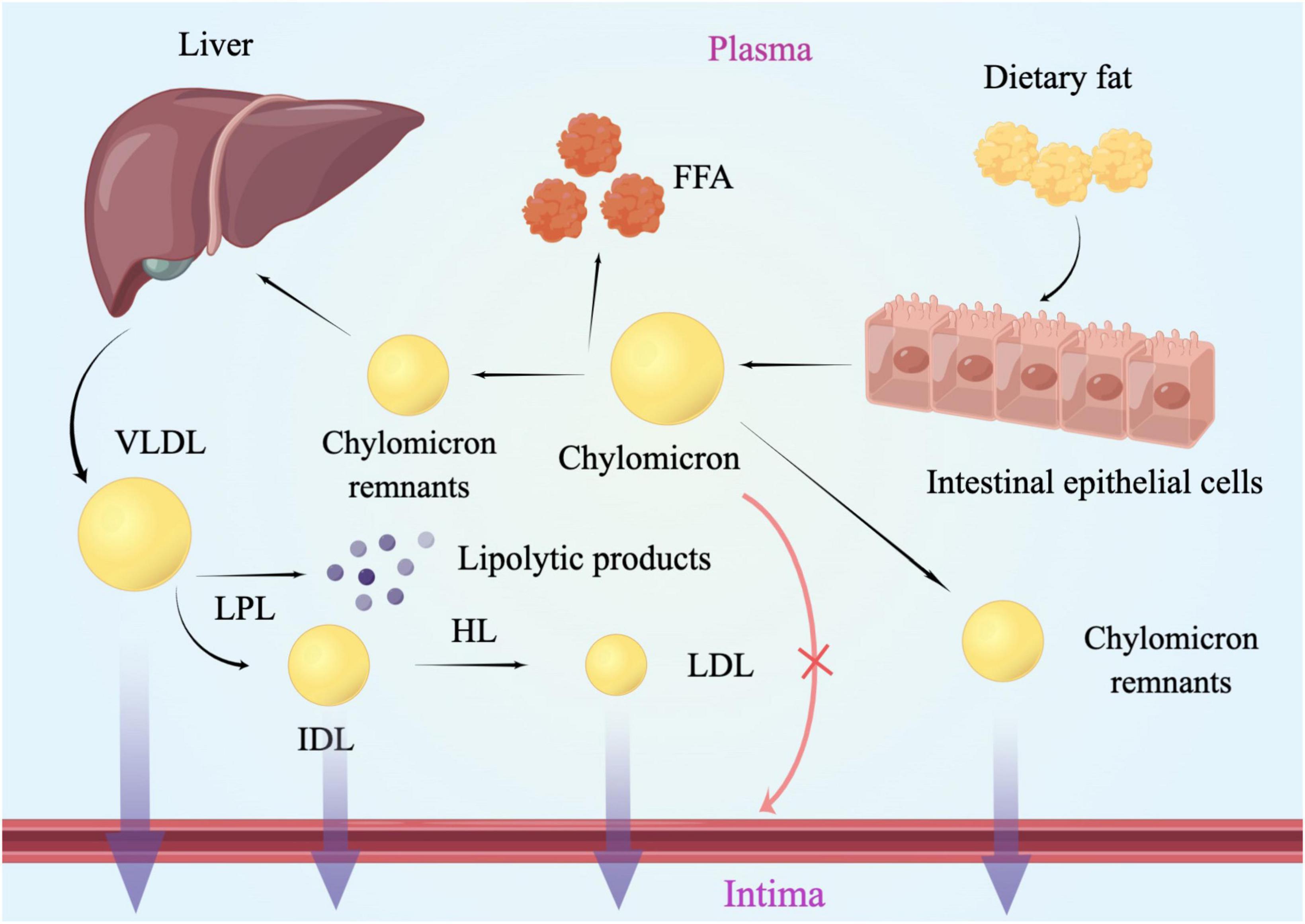
Figure 1. Lipoproteins metabolism and the arterial wall. Chylomicrons of different sizes needed to be converted into metabolic remnants, while VLDL needed to be metabolized to LDL to be cleaned. After being digested, CM and VLDL entered into the plasma and began to be lipolysis by lipoprotein lipase. Then CM remnants and VLDL remnant-like particles with higher density and smaller molecular weight were formed, respectively. Lipoproteins larger than 75 nm were not easily available to get across the arterial intima, such as chylomicrons. LPL, lipoprotein lipase; HL, hepatic lipase; VLDL, very-low-density lipoprotein; IDL, intermediate-density lipoprotein; LDL, low-density lipoprotein; FFA, free fatty acid; RC, remnant cholesterol.
The measurements of RC in the plasma were generally divided into two major types: the calculated methods and the directly measured methods (22, 23). The calculated RC (CRC) was defined as total cholesterol (TC) minus LDL-C minus HDL-C (24), which did not require special detection equipment, and was more convenient and easier to implement in clinical diagnosis and therapy. However, it was worth noting that for the detection of LDL-C in the formula, when TG < 5.6 mmol/L, the Friedewald equation was used, while when TG ≥ 5.6 mmol/L, the method of direct detection was used (25, 26). In addition to CRC, the directly measured RC (MRC) had a variety of detection methods, including direct automatic assay, immune separation, and ultracentrifugation. The direct automated assay used enzymes and surfactants to detect cholesterol content in CM and VLDL (27, 28). The immune separation method was detecting the cholesterol content of a small subunit on TRLs, and the cholesterol content of CM remnants and VLDL remnants were measured after the removal of ApoA1 and ApoB100 by antibodies. Chylomicron remnants can be quantitatively evaluated by serum apolipoprotein B-48 concentration (8). The directly measured methods were complicated and the price was relatively expensive, which limits the large-scale clinical use (29). Jepsen et al. and Cao et al. have compared the CRC and MRC in cardiovascular outcomes of patients with IHD. The results showed that both RC were considered to be associated with an increased risk of major adverse cardiovascular events (MACEs) (22, 30), and the calculated RC showed a stronger correlation (30).
Epidemiologic studies about remnant cholesterol levels and atherosclerotic cardiovascular disease risks
Non-fasting remnant cholesterol and atherosclerotic cardiovascular disease
Since the fasting state means no diets at least for 8 h, which is usually implied in the early morning, abundant research investigated the relationship between non-fasting lipids levels and ASCVD. So far, many prospective studies and clinical trials have demonstrated that elevated non-fasting RC levels can predict the increased risk of the incidence and development of arteriosclerotic cardiovascular disease, which is more capable than LDL-C levels (10, 13, 22, 31, 32). A prospective study of 73,513 patients from the Copenhagen General Population Study (the CGPS) and the Copenhagen Ischemic Heart Disease Study (the CIHDS) with 34 years of follow-up found that every 1 mmol/L increase in non-fasting RC was associated with a 40% increase in the risk of ASCVD. However, for the levels of LDL-C, every 1 mmol/L increase in RC was only associated with a 10% increase in the risk of ASCVD (31). It has confirmed a higher risk of non-fasting RC than LDL-C. Another survey of 82,890 people from the Copenhagen Heart Center showed that with an increased RC level, patients had an increased risk of all-cause mortality, which was not associated with an increased LDL-C level. And compared with the patients with RC levels less than 0.5 mmol/L (19 mg/dl), the risk of all-cause mortality in patients with RC more than 5 mmol/L (58 mg/dl) was 1.4 times higher (27). Furthermore, a recent prospective study involving 2,973 patients with acute myocardial infarction or ischemic stroke found that patients with RC < 0.8 mmol/L could reduce the incidence of recurrent cardiovascular events by at least 20% (10).
Fasting remnant cholesterol and atherosclerotic cardiovascular disease
Castañer et al. investigated the relationship between lipid levels in plasma and MACEs (e.g. myocardial infarction, stroke, or cardiovascular death) in the PREDIMED study, a cohort having the high-risk cardiovascular disease, which included 6,901 individuals. They found that every 0.26 mmol/L increase in RC leads to a 21% increase in the risk of cardiovascular events. According to the multivariate adjustment analysis in this research, RC but not LDL-C level, was associated with cardiovascular events in overweight or obese subjects, independent of lifestyle and other risk factors. And RC at 0.78 mmol/L was defined as the cut-off of higher risks of ASCVD (11). Elshazly et al. analyzed the data from 5,754 patients with ASCVD who underwent serial intravascular ultrasonography and compared the development of atheroma volume and 2 years follow-up MACEs at different RC levels. It has been demonstrated that in statin-treated ASCVD patients, the elevated RC was positively correlated with coronary atherosclerosis volume progression (+0.53 ± 0.26 vs. −0.15 ± 0.25%, p < 0.001) and the MACEs (23 vs. 14%, log-rank p < 0.001), which was regardless of conventional lipid parameters, C-reactive protein, or other clinical risk factors (33). Furthermore, we previously conducted a retrospective study including 12,563 patients, in which RC was calculated as TC minus LDL-C minus HDL-C (34). The study reported that the elevated fasting RC level in the coronary artery disease (CAD) group was statistically significantly different from the non-CAD group (0.51 vs. 0.49, p < 0.001), and further analysis found that RC was an independent risk factor for ASCVD, with a causal odds ratio of 1.952 (CI = 1.276–2.988, p = 0.002). In addition, according to the concordance/discordance groups analysis, the level of RC seemed more capable to predict the ASCVD risks than the LDL-C level (Figure 2), which reached the same conclusion as Castañer et al. (11).
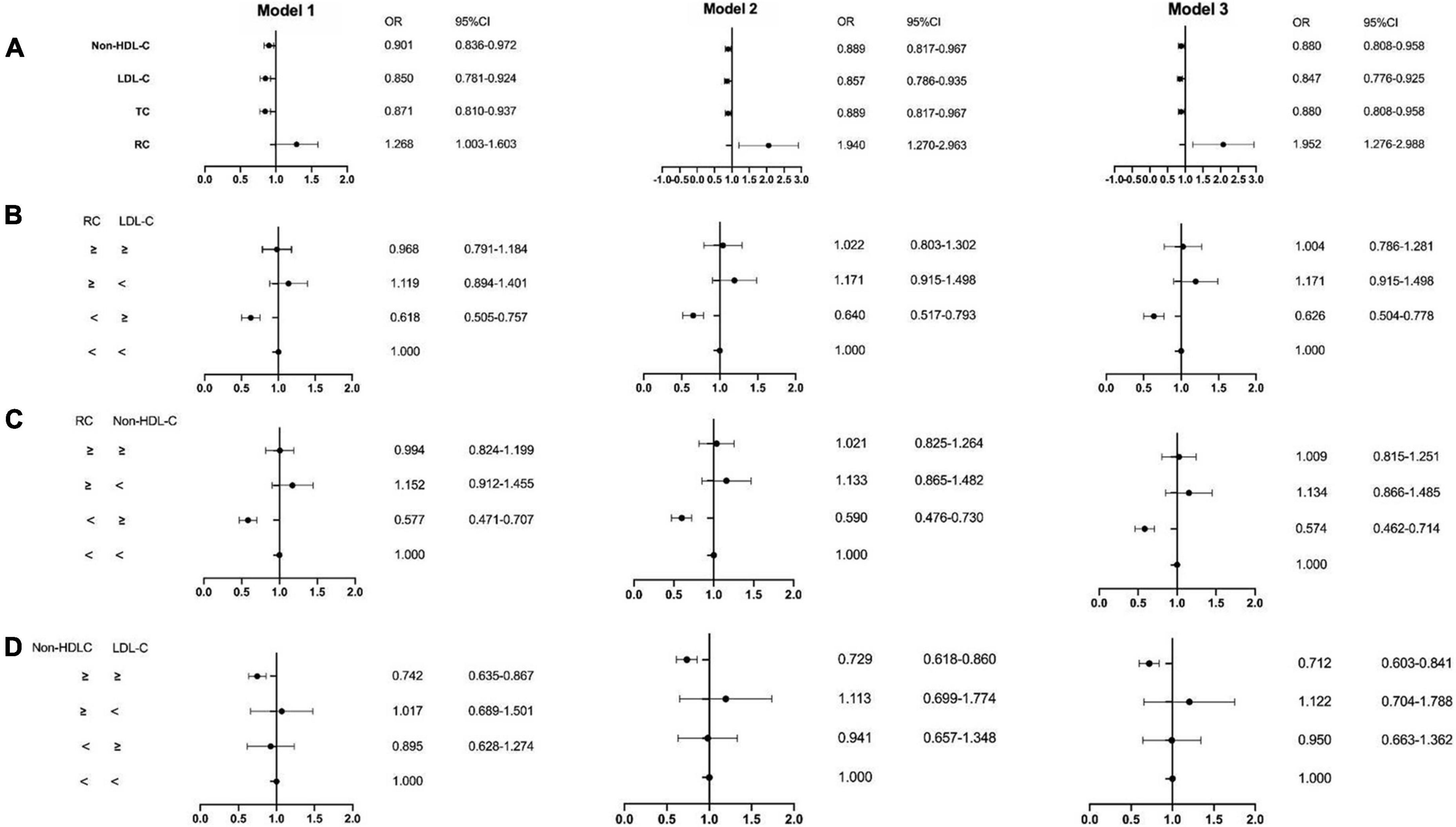
Figure 2. The result of multivariable logistic regression analyze. Model 1: age, gender, hypertension, hypercholesterolemia, smoking, diabetes, BMI; Model 2: Model 1, SBP, DBP, FBG, HbA1C, HDL-C, TG; Model 3: Model 2, creatinine, uric acid, hs-CRP, BNP, WBC, homocysteine. The medians of the RC, LDL-C and non-HDL-C index were calculated to divide all patients into two different groups: low (less than the medians) and high (equal to or greater than the medians). Then patients were categorized into four groups according to having a low or high RC index and LDL-C, RC and non-HDL-C, non-HDL-C and LDL-C as follows: low/low, low/high, high/low, and high/high. (A) Non-HDL-C, LDL-C, TC and RC; (B) RC and LDL-C group; (C) RC and non-HDL-C group; (D) non-HDL-C and LDL-C group. LDL-C, low-density lipoprotein cholesterol; HDL-C, high-density lipoprotein cholesterol; RC, remnant cholesterol; OR, odds ratios; CI, confidence intervals. Modified from Wang et al. (34).
So far, epidemiological research has confirmed that triglycerides is an independent risk factor for atherosclerotic coronary heart disease (35). The relationship between triglycerides and coronary heart disease was statistically significant when the adjusted multivariate logistic regression models were performed on triglycerides and other factors (14, 36). And the level of RC seemed more clinically predictive than TG (37). As the research mentioned above showed, both non-fasting and fasting RC could lead to a higher risk in the incidence and progress of ASCVD. Different from the fasting RC, the non-fasting RC also contains the cholesterol in chylomicron remnants. More mechanisms will be discussed later.
Mechanisms
The plasma levels of RC were shown to be inversely correlated with HDL-C (38), which could prevent inflammation and oxidative stress and promote cholesterol efflux to reduce lesion formation (39). Although the association was attenuated after correction for the level of HDL-C, a consensus was emerging based on abundant clinical and population data and genetic studies that fasting and non-fasting plasma triglycerides were predictive of ASCVD (10, 38). As studies have reported before, damage to the vascular endothelial cells could promote the development of atherosclerosis. Nordestgaard et al. demonstrated that the most necessary condition for TRLs promoting atherosclerosis was the ability to invade the arterial intima, which was directly determined by the size of lipoproteins. Lipoproteins larger than 75 nm were not easily available to get across the arterial intima, such as chylomicrons (40) (Figure 1). While lipoproteins of 20–60 nm could easily get across the arterial intima (41). A human and animal study has also shown that medium-sized TRLs could enter into the intima of the arterial wall, however, much slower than the LDL particles (42). TRLs could be phagocytosed by peripheral blood macrophages through the VLDL receptor, APOB 48 receptor, or LDL receptor-related protein (43) without modification (44). Moreover, saturated fatty acids (FAs) and phospholipids containing oxidized FAs that were generated by lipolysis of TRLs could be taken up into parenchymal cells and could induce maladaptive inflammatory responses (12). As the remnants were digested, the triglycerides, proteins, and phospholipids were degraded, while a large number of undigested cholesterol droplets remained in macrophages (41). Indeed, it was the undigested cholesterol droplets, not triglycerides that could accumulate in intimal foam cells (42, 45). Elevated RC in the plasma could promote the lipids invasion of the arterial wall. After passing through the vascular endothelial layer, the RC could accumulate and be absorbed by macrophages and smooth muscle cells (SMC), further became the foam cells, and finally transformed to the part of atherosclerotic plaques (8, 46–48) (Figure 3). In addition, it has a stronger atherosclerotic ability due to the needless oxidative modification. Once RC entered the intima, it could attach to the extracellular proteoglycans, preferentially to LDL-C (45). Meanwhile, RC could increase the production of reactive oxygen species and trigger endothelial cell dysfunction (49). Lipoprotein lipase released free fatty acids (FFAs) and monoacylglycerols during the hydrolysis of RC, which may lead to local inflammation and affected the formation and progression of atherosclerosis (50). Furthermore, RC could also promote platelet aggregation and microthrombus formation by accelerating the formation of prothrombinase complex and upregulating the expression of plasminogen activator inhibitor-1 and its antigens (51, 52).
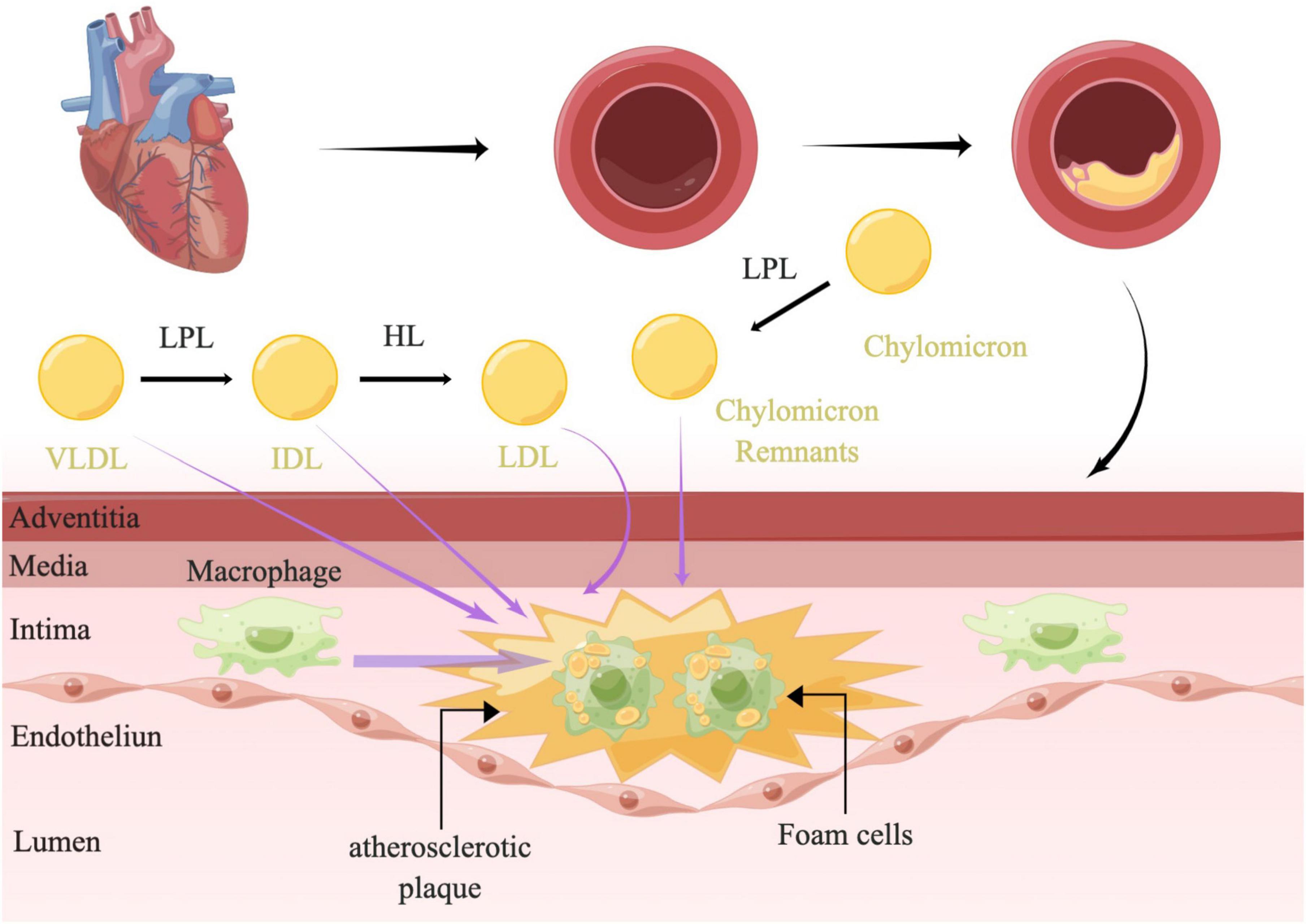
Figure 3. The mechanism of atherosclerosis. LPL along the capillary lumen surface hydrolyzed VLDL, resulting in VLDL remnants/IDL particles and lipolysis products. The IDL particles were further catabolized into LDL by HL. LPL could also hydrolyzed chylomicrons to produce chylomicron remnants. TRLs and their remnants readily penetrate the arterial wall and can be taken up by scavenger receptors on macrophages directly without oxidative modification, leading to formation of foam cells and atherosclerotic plaque development. TRLs, triglyceride-rich lipoproteins; LPL, lipoprotein lipase; HL, hepatic lipase; VLDL, very-low-density lipoprotein; IDL, intermediate-density lipoprotein; LDL, low-density lipoprotein; RC, remnant cholesterol.
A study showed that the level of RC in patients in the non-fasting state was 0.2 mmol/L higher than that in the fasting state (53). The reason could be that TG was hydrolyzed into FFAs and diglycerides in the stomach and proximal small intestine. Free fatty acids could be taken up by muscle cells and adipocytes, while cholesterol-rich chylomicrons remnants needed to be cleared by binding to LDL receptor or LDL receptor-associated protein-1 through liver APOE (30). Most studies detect RC in the non-fasting state, but the fasting state is also extremely important for accurately judging inherited abnormal lipoprotein metabolism.
Genetic studies
Plasma levels of lipids, such as triglycerides or RC, were determined by common or rare genetic variants (54, 55), and lifestyle factors such as diet, obesity, alcohol consumption, and physical activity (56). It suggested that lifetime exposure to elevated RC levels caused by genetic abnormalities was associated with a greater risk of cardiovascular disease. In the epidemiological etiology inference, the method of Mendelian randomization has always been applied to exclude confounding factors between lipids and ASCVD. Therefore, genetic evidence could provide insight into whether lifelong raised TRLs and RC are causally connected with low-grade inflammation, ASCVD, and more MACEs. Tada et al. illustrated that RC was associated with CAD in the general population, and serum RC levels were associated with the incident and progress of ASCVD in patients with familial hypercholesterolemia (FH) (57). Varbo et al. screened 15 kinds of genes that may affect blood lipid levels and genotyped 73,413 cases in the Copenhagen research to observe the incidence of ASCVD of each genotype. The results showed that each 1 mmol/L increase in the non-fasting RC was associated with a 2.8-fold increase in the risk of ASCVD, which suggested that the non-fasting RC was a risk factor of ASCVD, independently of LDL-C (10) (Figure 4, top section). In addition, the elevated RC was associated with a 2.2-fold increased risk of myocardial infarction when there was a variant in the APOA5 (apolipoprotein A5) gene, and the corresponding observational estimate was 1.7 times (58)(Figure 4, middle section). Meanwhile, Nordestgaard et al. analyzed four gene mutations that specifically affected the RC levels, including APOA5, GCKR, LPL, and TRIB1, and compared the causal odds ratio and observed hazard ratio of patients exposed to each different gene mutation. The results found that every 1 mmol/L increase in RC, the causal odds ratio for myocardial infarction increased 1.7 times and the observed hazard ratio increased 1.4 times (59) (Figure 4, bottom section). Finally, high concentrations of RC were genetically associated with increased low-grade inflammation, which was not the same as the high concentrations of LDL-C. Thus, it was suggested that an inflammatory component of atherosclerosis was driven by TRLs (24).
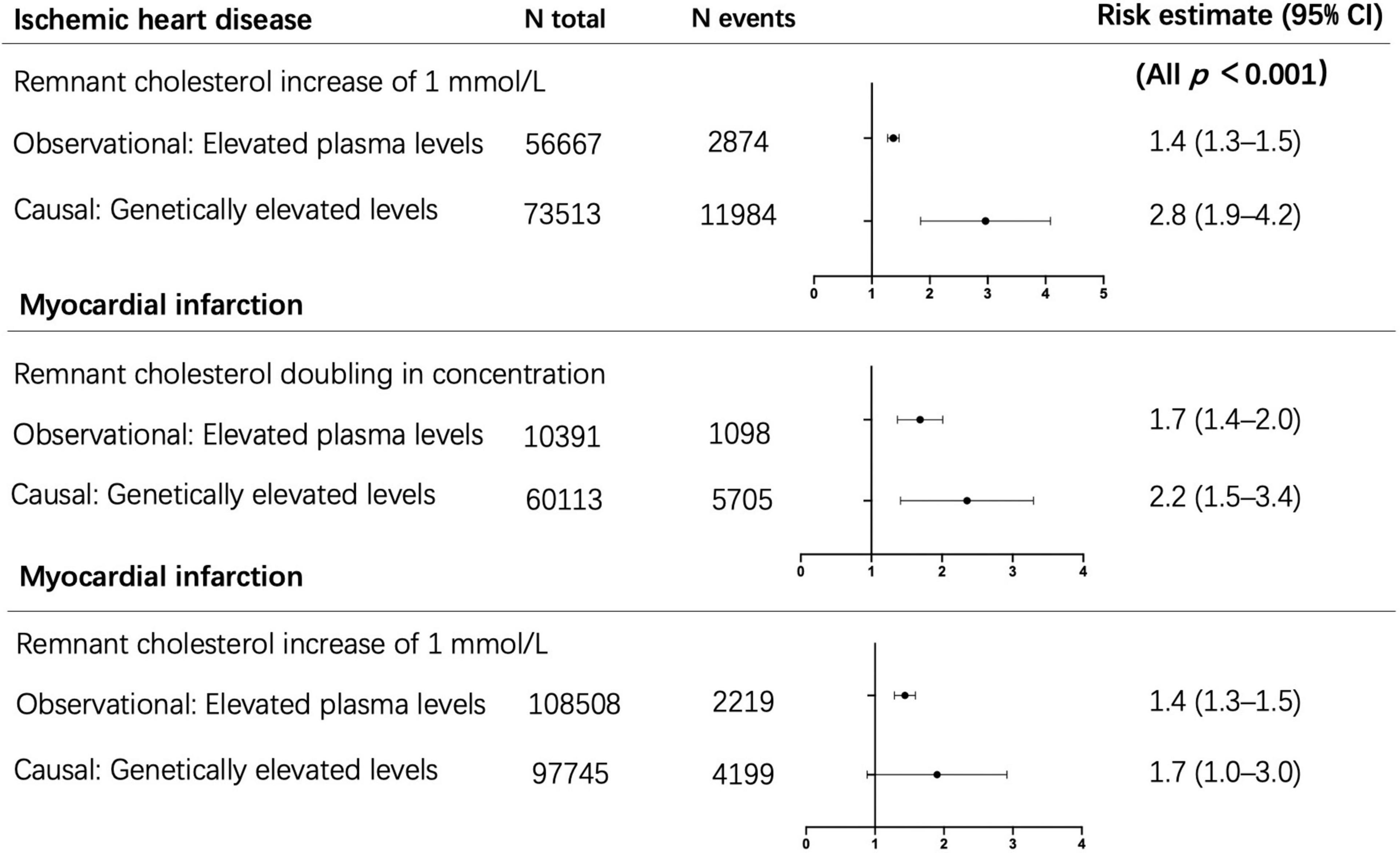
Figure 4. Observational and causal (by use of genetics) associations of elevated remnant cholesterol with risk of ischemic heart disease and myocardial infarction. Top section adapted from Varbo et al. (10). Middle section adapted from Jørgensen et al. (58). Bottom section adapted from Nordestgaard et al. (59). N, number.
New treatments of elevated remnant cholesterol
As the 2021 ESC guideline recommends (3), lifestyle interventions are fundamental to control elevated TRLs levels, including weight loss, increased physical activity, limiting alcohol intake, and avoiding fructose or high-carbohydrate foods. And for the drug interventions that lowered RC level were typically designed to lower plasma triglycerides. For the patients with hypertriglyceridemia (TG > 5.6 mmol/L or >500 mg/dl), if the TG level is more than 2.3 mmol/L (or >200 mg/dl), high-intensity statins are recommended. In patients with type 2 diabetes with or without ASCVD, if the TG level is more than 2.3 mmol/L (or >200 mg/dl) after statin treatments, statins or ezetimibe with fenofibrate could be considered, which may receive more macrovascular and microvascular benefits. Although the guideline has not directly recommended decreasing the RC level in the plasma, the awareness of controlling other blood lipid components except for LDL-C in ASCVD patients is arousing, which will undoubtedly benefit people who meet LDL-C targets but still have residual cardiovascular risks. For the new treatments of elevated RC, there were abundant new studies being conducted, which mainly concentrated on statins, fibrates, and omega-3 fatty acids (OM3FAs). The PREVAIL US Trial showed that all statins could effectively reduce the level of RC in ASCVD patients, and pitavastatin was stronger than other statins (60). For the fibrates, Tsunoda et al. demonstrated that fibrates could also reduce the RC levels. Compared with placebo, it could not only reduce TG, but also RC levels in patients with type 2 diabetes (61). And the ACCORD-Lipid study showed that the fenofibrate was effective for ASCVD risk reduction in patients with type 2 diabetes, which have already treated with statins (62). Ishibashi et al. also illustrated that as a novel selective peroxisome proliferator-activated receptor α modulator (SPPARMα) that possessed unique PPARα activity and selectivity, pemafibrate could lowering RC as well as TG (63). However, in the Helsinki Heart Study, gemfibrozil has reduced the risk of ASCVD by 32% without any significant effects on preventing stroke or all-cause mortality (64). Again, in the FIELD study (65), fenofibrate did not demonstrate a reduction in the rate of the coronary events (coronary heart disease death or non-fatal myocardial infarction). Furthermore, a meta-analysis of 10 trials enrolling 77,917 participants showed that low-dose OM3FAs supplementation was not associated with significant cardiovascular benefits (66). But the REDUCE-IT trial changed this view. In patients with a history of cardiovascular disease, diabetes, or other risk factors, after statin treatments, the TG level was 1.52–5.63 mmol/L, and the LDL-C level was 1.06–2.59 mmol/L. High-dose (4 g/day) icosapent ethyl was given after stains, and the risk of ASCVD was significantly reduced by 25% after 4.9 years of follow-up (67).
Insights from genetic studies have also given us new prospects in therapeutic drug targeting, and the results are worth looking forward to, such as angiopoietin-like protein 4 antibodies, APOC3 inhibitor, and APOB antisense therapy (59, 68–70). For example, antisense inhibition of APOC3, which encoded apolipoprotein C3, has indicated promising results regarding the reduction of triglyceride levels in preclinical models and a phase I clinical trial (68, 71). However, before any of these potential new therapies for lowering RC levels reach clinical application, they should be demonstrated to be effective without any essentially side effects, either as a supplement to statin therapy or used alone in statin-intolerant patients.
Conclusion and future directions
In conclusion, dyslipidemia was considered to be the main risk factor of ASCVD. Evidence from in vitro and animal pathogenic mechanisms studies, epidemiology, and genetic studies all indicated that RC plays an important role in predicting the incidence of ASCVD. As a new indicator to reflect atherosclerosis, especially when LDL-C has been controlled to a recommended level, RC was considered as a priority treatment target for people at high risk of ASCVD. Furthermore, stains were demonstrated to be effective in reducing cardiovascular disease and MACEs with few essential side effects. Meanwhile, the use of APOC3 inhibitors, PCSK9 inhibitors, and omega-3 FAs to reduce RC levels in the plasma may provide long-term benefits. However, the standardized detection of RC was still controversial, and more studies on appropriate treatments of elevated RC are urgently needed. Most desirable was a large randomized placebo-controlled trial in patients with mild-moderately elevated TG with recommended controlled LDL-C, treated with a potent statin and another RC lowering drug. These positive trials may benefit more patients at high ASCVD risks worldwide in the future.
Author contributions
KW carried out the experiments, acquired the data, and wrote the first draft of the manuscript. RW carried out the experiments and wrote sections of the manuscript. JY, XL, HS, and YZ recruited the subjects, performed the patients’ assessments, and critically reviewed the manuscript for intellectual content. KW, ZF, and YS performed the statistical analyses. HG conceived and designed the study and handled funding and supervision. All authors contributed to the article and approved the submitted version.
Funding
This study was supported by the China National Natural Scientific Foundation (81973841 and 81573744), National Key Research and Development Program of China (2017YFC0908800), Beijing Municipal Administration of Hospitals’ Mission Plan (SML20180601), Capital’s Funds for Health Improvement and Research (CFH 2020-2-2063), and Science and Technology Program of Beijing Municipal Education Commission (KM200910025012).
Acknowledgments
Figures 1, 3 were drawn by Figdraw (ID: WTYPT12282 and ID: TYAOS8999d).
Conflict of interest
The authors declare that the research was conducted in the absence of any commercial or financial relationships that could be construed as a potential conflict of interest.
Publisher’s note
All claims expressed in this article are solely those of the authors and do not necessarily represent those of their affiliated organizations, or those of the publisher, the editors and the reviewers. Any product that may be evaluated in this article, or claim that may be made by its manufacturer, is not guaranteed or endorsed by the publisher.
Abbreviations
ASCVD, atherosclerotic cardiovascular disease; CAD, coronary artery disease; RC, remnant cholesterol; TC, total cholesterol; TG, triglyceride; LDL-C, low-density lipoprotein cholesterol; HDL-C, high-density lipoprotein cholesterol; FFA, free fatty acid; ACS, acute coronary syndrome; STEMI, ST-segment elevation myocardial infarction; NSTEMI, non-ST-segment elevation myocardial infarction; LPL, lipoprotein lipase; HL, hepatic lipase; Apo, apolipoprotein; APOA5, apolipoprotein A5; APOC3, apolipoprotein C3.
References
1. Prasad DS, Kabir Z. Editorial for manuscript number EJPC-D-21-01502R1. Burden of ischemic heart disease and its attributable risk factors in 204 countries and territories, 1990-2019. Eur J Prev Cardiol. (2022) 29:420–31.
2. Safiri, S, Karamzad N, Singh K, Carson-Chahhoud K, Adams C, Nejadghaderi SA, et al. Burden of ischemic heart disease and its attributable risk factors in 204 countries and territories, 1990-2019. Eur J Prev Cardiol. (2022) 11:420–31. doi: 10.1093/eurjpc/zwab213
3. Visseren FLJ, Mach F, Smulders YM, Carballo D, Koskinas KC, Bäck M, et al. 2021 ESC guidelines on cardiovascular disease prevention in clinical practice. Eur J Prev Cardiol. (2022) 29:5–115.
4. Averna M, Banach M, Bruckert E, Drexel H, Farnier M, Gaita D, et al. Practical guidance for combination lipid-modifying therapy in high- and very-high-risk patients: a statement from a European atherosclerosis society task force. Atherosclerosis. (2021) 325:99–109. doi: 10.1016/j.atherosclerosis.2021.03.039
5. Silverman MG, Ference BA, Im K, Wiviott SD, Giugliano RP, Grundy SM, et al. Association between lowering LDL-C and cardiovascular risk reduction among different therapeutic interventions: a systematic review and meta-analysis. JAMA. (2016) 316:1289–97. doi: 10.1001/jama.2016.13985
6. Pirillo A, Norata GD, Catapano AL. Beyond LDL-C levels, does remnant cholesterol estimation matter? Eur J Prev Cardiol. (2020) 27:1088–90. doi: 10.1177/2047487319899622
7. Tenenbaum A, Fisman EZ, Motro M, Adler Y. Atherogenic dyslipidemia in metabolic syndrome and type 2 diabetes: therapeutic options beyond statins. Cardiovasc Diabetol. (2006) 5:20. doi: 10.1186/1475-2840-5-20
8. Masuda D, Yamashita S. Postprandial hyperlipidemia and remnant lipoproteins. J Atheroscler Thromb. (2017) 24:95–109. doi: 10.5551/jat.RV16003
9. Chandra R, Mellis B, Garza K, Hameed SA, Jurica JM, Hernandez AV, et al. Remnant lipoprotein size distribution profiling via dynamic light scattering analysis. Clin Chim Acta. (2016) 462:6–14. doi: 10.1016/j.cca.2016.08.012
10. Varbo A, Benn M, Tybjærg-Hansen A, Jørgensen AB, Frikke-Schmidt R, Nordestgaard BG. Remnant cholesterol as a causal risk factor for ischemic heart disease. J Am Coll Cardiol. (2013) 61:427–36. doi: 10.1016/j.jacc.2012.08.1026
11. Castañer O, Pintó X, Subirana I, Amor AJ, Ros E, Hernáez Á, et al. Remnant cholesterol, not LDL cholesterol, is associated with incident cardiovascular disease. J Am Coll Cardiol. (2020) 76:2712–24. doi: 10.1016/j.jacc.2020.10.008
12. Borén J, Taskinen MR, Björnson E, Packard CJ. Metabolism of triglyceride-rich lipoproteins in health and dyslipidaemia. Nat Rev Cardiol. (2022) 19:577–92. doi: 10.1038/s41569-022-00676-y
13. Nordestgaard BG, Benn M, Schnohr P, Tybjaerg-Hansen A. Nonfasting triglycerides and risk of myocardial infarction, ischemic heart disease, and death in men and women. JAMA. (2007) 298:299–308. doi: 10.1001/jama.298.3.299
14. Esan O, Wierzbicki AS. Triglycerides and cardiovascular disease. Curr Opin Cardiol. (2021) 36:469–77. doi: 10.1097/HCO.0000000000000862
15. Dai W, Zhang Z, Yao C, Zhao S. Emerging evidences for the opposite role of apolipoprotein C3 and apolipoprotein A5 in lipid metabolism and coronary artery disease. Lipids Health Dis. (2019) 18:220. doi: 10.1186/s12944-019-1166-5
16. Wolska A, Reimund M, Remaley AT. Apolipoprotein C-II: the re-emergence of a forgotten factor. Curr Opin Lipidol. (2020) 31:147–53. doi: 10.1097/MOL.0000000000000680
17. Kersten S. Long-lost friend is back in the game. J Lipid Res. (2021) 62:100072. doi: 10.1016/j.jlr.2021.100072
18. Packard CJ, Boren J, Taskinen MR. Causes and consequences of hypertriglyceridemia. Front Endocrinol. (2020) 11:252. doi: 10.3389/fendo.2020.00252
19. Borén J, Packard CJ, Taskinen MR. The roles of ApoC-III on the metabolism of triglyceride-rich lipoproteins in humans. Front Endocrinol. (2020) 11:474. doi: 10.3389/fendo.2020.00474
20. Sandesara PB, Virani SS, Fazio S, Shapiro MD. The forgotten lipids: triglycerides, remnant cholesterol, and atherosclerotic cardiovascular disease risk. Endocr Rev. (2019) 40:537–57. doi: 10.1210/er.2018-00184
21. Oda H, Yorioka N, Okushin S, Nishida Y, Kushihata S, Ito T, et al. Remnant-like particle cholesterol may indicate atherogenic risk in patients on chronic hemodialysis. Nephron. (1997) 76:7–14. doi: 10.1159/000190133
22. Cao YX, Zhang HW, Jin JL, Liu HH, Zhang Y, Gao Y, et al. The longitudinal association of remnant cholesterol with cardiovascular outcomes in patients with diabetes and pre-diabetes. Cardiovasc Diabetol. (2020) 19:104. doi: 10.1186/s12933-020-01076-7
23. Varbo A, Nordestgaard BG. Directly measured vs. calculated remnant cholesterol identifies additional overlooked individuals in the general population at higher risk of myocardial infarction. Eur Heart J. (2021) 42:4833–43. doi: 10.1093/eurheartj/ehab293
24. Varbo A, Benn M, Tybjærg-Hansen A, Nordestgaard BG. Elevated remnant cholesterol causes both low-grade inflammation and ischemic heart disease, whereas elevated low-density lipoprotein cholesterol causes ischemic heart disease without inflammation. Circulation. (2013) 128:1298–309. doi: 10.1161/CIRCULATIONAHA.113.003008
25. Martin SS, Blaha MJ, Elshazly MB, Brinton EA, Toth PP, McEvoy JW, et al. Friedewald-estimated versus directly measured low-density lipoprotein cholesterol and treatment implications. J Am Coll Cardiol. (2013) 62:732–9. doi: 10.1016/j.jacc.2013.01.079
26. Szili-Torok T, Tietge UJF. Remnant cholesterol is just another word for triglycerides when calculating LDL-C using the friedewald formula. J Am Coll Cardiol. (2021) 77:1841. doi: 10.1016/j.jacc.2021.01.053
27. Varbo A, Freiberg JJ, Nordestgaard BG. Extreme nonfasting remnant cholesterol vs extreme LDL cholesterol as contributors to cardiovascular disease and all-cause mortality in 90000 individuals from the general population. Clin Chem. (2015) 61:533–43. doi: 10.1373/clinchem.2014.234146
28. Nakada Y, Kurosawa H, Tohyama J, Inoue Y, Ikewaki K. Increased remnant lipoprotein in patients with coronary artery disease–evaluation utilizing a newly developed remnant assay, remnant lipoproteins cholesterol homogenous assay (RemL-C). J Atheroscler Thromb. (2007) 14:56–64. doi: 10.5551/jat.14.56
29. Lupton JR, Faridi KF, Martin SS, Sharma S, Kulkarni K, Jones SR, et al. Deficient serum 25-hydroxyvitamin D is associated with an atherogenic lipid profile: the Very Large Database of Lipids (VLDL-3) study. J Clin Lipidol. (2016) 10:72–81.e1. doi: 10.1016/j.jacl.2015.09.006
30. Jepsen AM, Langsted A, Varbo A, Bang LE, Kamstrup PR, Nordestgaard BG. Increased remnant cholesterol explains part of residual risk of all-cause mortality in 5414 patients with ischemic heart disease. Clin Chem. (2016) 62:593–604. doi: 10.1373/clinchem.2015.253757
31. Kaltoft M, Langsted A, Nordestgaard BG. Triglycerides and remnant cholesterol associated with risk of aortic valve stenosis: mendelian randomization in the Copenhagen General Population Study. Eur Heart J. (2020) 41:2288–99. doi: 10.1093/eurheartj/ehaa172
32. Langsted A, Madsen CM, Nordestgaard BG. Contribution of remnant cholesterol to cardiovascular risk. J Intern Med. (2020) 288:116–27. doi: 10.1111/joim.13059
33. Elshazly MB, Mani P, Nissen S, Brennan DM, Clark D, Martin S, et al. Remnant cholesterol, coronary atheroma progression and clinical events in statin-treated patients with coronary artery disease. Eur J Prev Cardiol. (2020) 27:1091–100. doi: 10.1177/2047487319887578
34. Kexin W, Yaodong D, Wen G, Rui W, Jiaxin Y, Xiaoli L, et al. Association of increased remnant cholesterol and the risk of coronary artery disease: a Retrospective Study. Front Cardiovasc Med. (2021) 8:740596. doi: 10.3389/fcvm.2021.740596
35. Wong ND, Budoff MJ, Ferdinand K, Graham IM, Michos ED, Reddy T, et al. Atherosclerotic cardiovascular disease risk assessment: an American society for preventive cardiology clinical practice statement. Am J Prev Cardiol. (2022) 10:100335. doi: 10.1016/j.ajpc.2022.100335
36. Grundy SM, Stone NJ, Bailey AL, Beam C, Birtcher KK, Blumenthal RS, et al. 2018 AHA/ACC/AACVPR/AAPA/ABC/ACPM/ADA/AGS/APhA/ASPC/NLA/PCNA guideline on the management of blood cholesterol: a report of the American college of cardiology/American heart association task force on clinical practice guidelines. Circulation. (2019) 139:e1082–143. doi: 10.1161/CIR.0000000000000624
37. Ginsberg HN, Packard CJ, Chapman MJ, Borén J, Aguilar-Salinas CA, Averna M, et al. Triglyceride-rich lipoproteins and their remnants: metabolic insights, role in atherosclerotic cardiovascular disease, and emerging therapeutic strategies-a consensus statement from the European Atherosclerosis Society. Eur Heart J. (2021) 42:4791–806. doi: 10.1093/eurheartj/ehab551
38. McPherson R. Remnant cholesterol: “Non-(HDL-C + LDL-C)” as a coronary artery disease risk factor. J Am Coll Cardiol. (2013) 61:437–9.
39. Linton MF, Yancey PG, Davies SS, Jerome WG, Linton EF, Song WL, et al. editors. The role of lipids and lipoproteins in atherosclerosis. In: Feingold KR, Anawalt B, Boyce A, Chrousos G, de Herder WW, Dhatariya K, editors. Endotext. South Dartmouth, MA: MDText.com, Inc (2000).
40. Nordestgaard BG, Stender S, Kjeldsen K. Reduced atherogenesis in cholesterol-fed diabetic rabbits. Giant lipoproteins do not enter the arterial wall. Arteriosclerosis. (1988) 8:421–8. doi: 10.1161/01.ATV.8.4.421
41. Nordestgaard BG, Varbo A. Triglycerides and cardiovascular disease. Lancet. (2014) 384:626–35. doi: 10.1016/S0140-6736(14)61177-6
42. Nordestgaard BG, Tybjaerg-Hansen A, Lewis B. Influx in vivo of low density, intermediate density, and very low density lipoproteins into aortic intimas of genetically hyperlipidemic rabbits. Roles of plasma concentrations, extent of aortic lesion, and lipoprotein particle size as determinants. Arterioscler Thromb. (1992) 12:6–18. doi: 10.1161/01.ATV.12.1.6
43. Brown ML, Ramprasad MP, Umeda PK, Tanaka A, Kobayashi Y, Watanabe T, et al. A macrophage receptor for apolipoprotein B48: cloning, expression, and atherosclerosis. Proc Natl Acad Sci USA. (2000) 97:7488–93. doi: 10.1073/pnas.120184097
44. Takahashi S, Sakai J, Fujino T, Hattori H, Zenimaru Y, Suzuki J, et al. The very low-density lipoprotein (VLDL) receptor: characterization and functions as a peripheral lipoprotein receptor. J Atheroscler Thromb. (2004) 11:200–8. doi: 10.5551/jat.11.200
45. Shaikh M, Wootton R, Nordestgaard BG, Baskerville P, Lumley JS, La Ville AE, et al. Quantitative studies of transfer in vivo of low density, Sf 12-60, and Sf 60-400 lipoproteins between plasma and arterial intima in humans. Arterioscler Thromb. (1991) 11:569–77. doi: 10.1161/01.ATV.11.3.569
46. Quispe R, Martin SS, Michos ED, Lamba I, Blumenthal RS, Saeed A, et al. Remnant cholesterol predicts cardiovascular disease beyond LDL and ApoB: a primary prevention study. Eur Heart J. (2021) 42:4324–32. doi: 10.1093/eurheartj/ehab432
47. Burnett JR, Hooper AJ, Hegele RA. Remnant cholesterol and atherosclerotic cardiovascular disease risk. J Am Coll Cardiol. (2020) 76:2736–9. doi: 10.1016/j.jacc.2020.10.029
48. Varbo A, Nordestgaard BG. Remnant lipoproteins. Curr Opin Lipidol. (2017) 28:300–7. doi: 10.1097/MOL.0000000000000429
49. Wang L, Gill R, Pedersen TL, Higgins LJ, Newman JW, Rutledge JC. Triglyceride-rich lipoprotein lipolysis releases neutral and oxidized FFAs that induce endothelial cell inflammation. J Lipid Res. (2009) 50:204–13. doi: 10.1194/jlr.M700505-JLR200
50. Kawakami A, Aikawa M, Alcaide P, Luscinskas FW, Libby P, Sacks FM. Apolipoprotein CIII induces expression of vascular cell adhesion molecule-1 in vascular endothelial cells and increases adhesion of monocytic cells. Circulation. (2006) 114:681–7. doi: 10.1161/CIRCULATIONAHA.106.622514
51. Olufadi R, Byrne CD. Effects of VLDL and remnant particles on platelets. Pathophysiol Haemost Thromb. (2006) 35:281–91. doi: 10.1159/000093221
52. Lin A, Nerlekar N, Rajagopalan A, Yuvaraj J, Modi R, Mirzaee S, et al. Remnant cholesterol and coronary atherosclerotic plaque burden assessed by computed tomography coronary angiography. Atherosclerosis. (2019) 284:24–30. doi: 10.1016/j.atherosclerosis.2019.02.019
53. Nordestgaard BG, Langsted A, Mora S, Kolovou G, Baum H, Bruckert E, et al. Fasting is not routinely required for determination of a lipid profile: clinical and laboratory implications including flagging at desirable concentration cut-points-a joint consensus statement from the European atherosclerosis society and European federation of clinical chemistry and laboratory medicine. Eur Heart J. (2016) 37:1944–58. doi: 10.1093/eurheartj/ehw152
54. Johansen CT, Kathiresan S, Hegele RA. Genetic determinants of plasma triglycerides. J Lipid Res. (2011) 52:189–206. doi: 10.1194/jlr.R009720
55. Hegele RA, Ban MR, Hsueh N, Kennedy BA, Cao H, Zou GY, et al. A polygenic basis for four classical Fredrickson hyperlipoproteinemia phenotypes that are characterized by hypertriglyceridemia. Hum Mol Genet. (2009) 18:4189–94. doi: 10.1093/hmg/ddp361
56. Chapman MJ, Ginsberg HN, Amarenco P, Andreotti F, Borén J, Catapano AL, et al. Triglyceride-rich lipoproteins and high-density lipoprotein cholesterol in patients at high risk of cardiovascular disease: evidence and guidance for management. Eur Heart J. (2011) 32:1345–61. doi: 10.1016/S1567-5688(11)70033-2
57. Tada H, Kawashiri MA, Nohara A, Sakata K, Inazu A, Mabuchi H, et al. Remnant-like particles and coronary artery disease in familial hypercholesterolemia. Clin Chim Acta. (2018) 482:120–3. doi: 10.1016/j.cca.2018.04.007
58. Jørgensen AB, Frikke-Schmidt R, West AS, Grande P, Nordestgaard BG, Tybjærg-Hansen A. Genetically elevated non-fasting triglycerides and calculated remnant cholesterol as causal risk factors for myocardial infarction. Eur Heart J. (2013) 34:1826–33. doi: 10.1093/eurheartj/ehs431
59. Nordestgaard BG, Nicholls SJ, Langsted A, Ray KK, Tybjærg-Hansen A. Advances in lipid-lowering therapy through gene-silencing technologies. Nat Rev Cardiol. (2018) 15:261–72. doi: 10.1038/nrcardio.2018.3
60. Miller PE, Martin SS, Joshi PH, Jones SR, Massaro JM, D’Agostino RB, et al. Pitavastatin 4 mg provides significantly greater reduction in remnant lipoprotein cholesterol compared with pravastatin 40 mg: results from the short-term phase IV PREVAIL US trial in patients with primary hyperlipidemia or mixed dyslipidemia. Clin Ther. (2016) 38:603–9. doi: 10.1016/j.clinthera.2016.02.001
61. Tsunoda F, Asztalos IB, Horvath KV, Steiner G, Schaefer EJ, Asztalos BF. Fenofibrate, HDL, and cardiovascular disease in Type-2 diabetes: the DAIS trial. Atherosclerosis. (2016) 247:35–9. doi: 10.1016/j.atherosclerosis.2016.01.028
62. Ginsberg HN, Elam MB, Lovato LC, Crouse JR III, Leiter LA, Linz P, et al. Effects of combination lipid therapy in type 2 diabetes mellitus. N Engl J Med. (2010) 362:1563–74. doi: 10.1056/NEJMoa1001282
63. Ishibashi S, Yamashita S, Arai H, Araki E, Yokote K, Suganami H, et al. Effects of K-877, a novel selective PPARα modulator (SPPARMα), in dyslipidaemic patients: a randomized, double blind, active- and placebo-controlled, phase 2 trial. Atherosclerosis. (2016) 249:36–43. doi: 10.1016/j.atherosclerosis.2016.02.029
64. Frick MH, Elo O, Haapa K, Heinonen OP, Heinsalmi P, Helo P, et al. Helsinki Heart Study: primary-prevention trial with gemfibrozil in middle-aged men with dyslipidemia. Safety of treatment, changes in risk factors, and incidence of coronary heart disease. N Engl J Med. (1987) 317:1237–45. doi: 10.1056/NEJM198711123172001
65. Keech A, Simes RJ, Barter P, Best J, Scott R, Taskinen MR, et al. Effects of long-term fenofibrate therapy on cardiovascular events in 9795 people with type 2 diabetes mellitus (the FIELD study): randomised controlled trial. Lancet. (2005) 366:1849–61. doi: 10.1016/S0140-6736(05)67667-2
66. Aung T, Halsey J, Kromhout D, Gerstein HC, Marchioli R, Tavazzi L, et al. Associations of Omega-3 fatty acid supplement use with cardiovascular disease risks: meta-analysis of 10 trials involving 77 917 individuals. JAMA Cardiol. (2018) 3:225–34. doi: 10.1001/jamacardio.2017.5205
67. Bhatt DL, Steg PG, Miller M, Brinton EA, Jacobson TA, Ketchum SB, et al. Cardiovascular risk reduction with icosapent ethyl for hypertriglyceridemia. N Engl J Med. (2019) 380:11–22. doi: 10.1056/NEJMoa1812792
68. Graham MJ, Lee RG, Bell TA III, Fu W, Mullick AE, Alexander VJ, et al. Antisense oligonucleotide inhibition of apolipoprotein C-III reduces plasma triglycerides in rodents, nonhuman primates, and humans. Circ Res. (2013) 112:1479–90. doi: 10.1161/CIRCRESAHA.111.300367
69. Guyton JR, Slee AE, Anderson T, Fleg JL, Goldberg RB, Kashyap ML, et al. Relationship of lipoproteins to cardiovascular events: the AIM-HIGH Trial (Atherothrombosis Intervention in Metabolic Syndrome With Low HDL/High Triglycerides and Impact on Global Health Outcomes). J Am Coll Cardiol. (2013) 62:1580–4.
70. D’Erasmo L, Gallo A, Di Costanzo A, Bruckert E, Arca M. Evaluation of efficacy and safety of antisense inhibition of apolipoprotein C-III with volanesorsen in patients with severe hypertriglyceridemia. Expert Opin Pharmacother. (2020) 21:1675–84. doi: 10.1080/14656566.2020.1787380
Keywords: remnant cholesterol, lipoprotein, atherosclerosis, cardiovascular disease, general population
Citation: Wang K, Wang R, Yang J, Liu X, Shen H, Sun Y, Zhou Y, Fang Z and Ge H (2022) Remnant cholesterol and atherosclerotic cardiovascular disease: Metabolism, mechanism, evidence, and treatment. Front. Cardiovasc. Med. 9:913869. doi: 10.3389/fcvm.2022.913869
Received: 06 April 2022; Accepted: 26 September 2022;
Published: 17 October 2022.
Edited by:
Wilfried Le Goff, Institut National de la Santé et de la Recherche Médicale (INSERM), FranceReviewed by:
Anatol Kontush, INSERM U1166 Unité de Recherche sur les Maladies Cardiovasculaires, du Métabolisme et de la Nutrition, FranceDaisaku Masuda, Rinku General Medical Center, Japan
Copyright © 2022 Wang, Wang, Yang, Liu, Shen, Sun, Zhou, Fang and Ge. This is an open-access article distributed under the terms of the Creative Commons Attribution License (CC BY). The use, distribution or reproduction in other forums is permitted, provided the original author(s) and the copyright owner(s) are credited and that the original publication in this journal is cited, in accordance with accepted academic practice. No use, distribution or reproduction is permitted which does not comply with these terms.
*Correspondence: Zhe Fang, NAL7150@163.com; Hailong Ge, azccmu@163.com