- 1Anaesthesiology and Critical Care Department, Dijon Bourgogne University Hospital, Dijon, France
- 2University of Medicine and Pharmacy “Carol Davila”, Bucharest, Romania
- 3Department of Cardiology, Euroecolab, Emergency Institute for Cardiovascular Diseases “Prof. Dr. C. C. Iliescu”, Bucharest, Romania
- 4Department of Translational Medicine, Università del Piemonte Orientale, Novara, Italy
- 5University of Burgundy Franche Comté, LNC UMR1231, Dijon, France
Introduction: Two parallel paradigms of cardiovascular efficiency and haemodynamic optimisation coexist in haemodynamic research. Targeting ventriculo-arterial (VA) coupling [i.e., the ratio between arterial and ventricular elastance (EV)] and electromechanical coupling are two promising approaches in acute circulatory failure. However, validation of the parameters of electromechanical coupling in critically ill patients is ongoing. Furthermore, a unifying link between VA and electromechanical coupling may exist, as EV is correlated with different times of the cardiac cycle.
Materials and Methods: This study was a retrospective analysis of a prospectively collected observational database from one tertiary center ICU. We analyzed the relationship between electromechanical dyssynchrony and acute circulatory failure hemodynamics before and after treatment (i.e., fluid expansion, dobutamine, or norepinephrine infusion). The relationship between electromechanical coupling and VA coupling was also investigated. Adult patients with haemodynamic instability were included. Haemodynamic parameters, including arterial pressure, cardiac index, VA coupling, stroke work index/pressure–volume area (SWI/PVA), t-IVT, and Tei's index, were collected before and after treatment. A t-IVT of >12 s/min was classified as intraventricular dyssynchrony.
Results: We included 54 patients; 39 (72.2%) were classified as having intraventricular dyssynchrony at baseline. These patients with baseline dyssynchrony showed a statistically significant amelioration of t-IVT (from 18 ± 4 s to 14 ± 6 s, p = 0.001), left ventricular EV [from 1.1 (0.72–1.52) to 1.33 (0.84–1.67) mmHg mL−1, p = 0.001], VA coupling [from 2 (1.67–2.59) to 1.80 (1.40–2.21), p = 0.001], and SWI/PVA [from 0.58 (0.49–0.65) to 0.64 (0.51–0.68), p = 0.007]. Patients without baseline dyssynchrony showed no statistically significant results. The improvement in VA coupling was mediated by an amelioration of EV. All patients improved their arterial pressure and cardiac index with treatment. The haemodynamic treatment group exhibited no effect on changing t-IVT.
Conclusion: Acute circulatory failure is associated with electromechanical dyssynchrony. Cardiac electromechanical coupling was improved by haemodynamic treatment only if altered at baseline. The improvement of cardiac electromechanical coupling was associated with the improvement of markers of cardiocirculatory efficacy and efficiency (i.e., SWI/PVA and VA coupling). This study was the first to demonstrate a possible link between cardiac electromechanical coupling and VA coupling in patients with acute circulatory failure.
Introduction
Targeting ventriculo-arterial coupling is a promising approach in patients with cardiocirculatory failure (1–3), because of its intrinsic connection with myocardium energetics and cardiovascular efficiency (4, 5). Ventriculo-arterial coupling is an integrative concept that numerically encompasses the volume/pressure interaction of the left ventricle (LV) with the arterial system, being described as the ratio between the global arterial elastance (EA) and left ventricular elastance (EV) (3, 6). Several intensive care (ICU) studies have shown the potential of ventriculo-arterial coupling determination for haemodynamic optimisation in different types of circulatory failure (7–9).
Naturally, EV is linked with the LV ejection fraction (LVEF). In a study underlying the limits of LVEF in heart failure, EV was found to be strongly correlated with different times of the cardiac cycle (10)—a relation that was also suggested by other studies (11, 12). This finding opens an interesting research niche regarding the relation between ventriculo-arterial coupling and electromechanical coupling—a subject that has never been studied in critically ill patients. Electromechanical coupling is a parallel study paradigm in haemodynamic research. Several echocardiographic time intervals and parameters are associated with cardiac performance, thus with clinical outcomes (13–16). Tei's index, which is defined as the sum of isovolumic contraction time and relaxation time divided by the ejection time, is described as an index of global myocardial performance and as a prognostic factor in patients presenting with acute myocardial infarction (17). The isovolumic time/heterovolumic time ratio (I/H) index, which is defined as the sum of isovolumic relaxation and contraction time divided by the sum of ejection and filling time, has also been considered a potential indicator of myocardial time yield and efficiency (18). Another marker of heart time efficiency is the total isovolumic time (t-IVT), which is correlated with cardiac activation and performance (19, 20). Prolonged t-IVT is a predictor of future cardiac events after cardiac surgery (21). Reducing the echocardiographic measured time of isovolumic contraction—an indirect marker of electromechanical efficiency—has improved cardiac output and, consequently, heart energetics (22, 23). A correlation between cardiac time intervals and the indicators of systolic function is found in patients admitted to cardiac ICU (24).
Nevertheless, validation of echocardiographic parameters in critically ill patients is in progress (24). Studies evaluating the effect of different haemodynamic ICU treatments (i.e., inotrope, vasopressor, fluid therapy) on cardiac time intervals in patients with acute cardiocirculatory failure are lacking. The clinical role of intraventricular dyssynchrony is unknown in general ICU patients, although it may represent a therapeutic target (22). At present, no study has evaluated the link between electromechanical coupling and ventriculo-arterial coupling in acute circulatory failure, even though answering this question is of clinical and physiological interest. First, identifying the right patients with acute circulatory failure benefitting from haemodynamic interventions is of clinical importance, as the therapeutic arsenal is rather parsimonious, with few and not-so-new available drugs with potential deleterious adverse effects (25). Second, the relationship between ventriculo-arterial and electromechanical coupling may unify two parallel paradigms of haemodynamic research, deepening the understanding of circulatory pathophysiological mechanisms.
The main study objective was to investigate the relationship between electromechanical dyssynchrony and acute circulatory failure and to assess its response to haemodynamic treatment. The secondary objective was to evaluate the relationship between electromechanical coupling and ventriculo-arterial coupling.
Methods
Patients and Ethics
This study was a retrospective analysis of a prospectively collected observational database for studying the ventriculo-arterial coupling in the ICU (5). All patients received written information and provided their consent to participate. The study received the approval of the local independent ethics committee, and it was conducted according to the standards of the 1964 Declaration of Helsinki.
This observational study was performed in a cardiovascular ICU at a university hospital. The patients included had haemodynamic instability (MAP < 60 mmHg and/or PAS < 90 mmHg), were aged 18 years or above, and were under controlled positive ventilation. For these patients, the intensivist decided to perform a haemodynamic therapeutic intervention (i.e., fluid expansion, norepinephrine infusion increase, or starting dobutamine infusion) in accordance with the unit's care protocol. The non-inclusion criteria were those who had low echogenicity, a pacemaker, bundle branch block, permanent atrial fibrillation, more than mild aortic regurgitation, or mitral regurgitation, and right heart dysfunction.
Haemodynamic Parameters
Systolic blood pressure (SAP), diastolic blood pressure (DAP), and mean blood pressure (MAP) were measured using an invasive arterial catheter. Transthoracic echocardiography (CX50 Ultrasound System and an S5-1 Sector Array Transducer, Philips Medical System, Suresnes, France) was performed by a physician blinded to the results of the study. The echocardiographic parameters were calculated as the average of five measurements (regardless of the respiratory cycle). The data were acquired and stored for further analysis. LVEF, end-systolic volume (ESV), and end-diastolic volume (EDV) were calculated using Simpson's method on a four-chamber view. The diameter of the left ventricular outflow tract was measured on a long-axis parasternal view at the time of patient inclusion. The aortic velocity time integral (VTIAo), pre-ejection time and systolic time were measured using a pulsed Doppler on a five-chamber apical view. Stroke volume (SV) (mL) was calculated as VTIAo × aortic area and was expressed as indexed SV (SVi) = SV/body surface area (mL m−2). Cardiac index (CI) (L min−1 m−2) was calculated as SVi × heart rate (HR). The indexed systemic vascular resistance (SVRi) (mmHg mL−1 m−2) was obtained using the formula SVRi = MAP–central venous pressure/CI. Total arterial compliance (mL mmHg−1) was calculated as the ratio between the SV and the arterial pulse pressure.
Ventriculo-arterial coupling is defined as the ratio between EA and left EV, with 1 ± 0.36 as the normal value (26, 27). Ventriculo-arterial coupling was calculated using the non-invasive single-beat echocardiographic method, as previously described and recommended by the European Society of Cardiology consensus (3, 12, 28). EA was calculated using the formula EA = 0.9 × SAP/SV, where SAP is systolic arterial pressure, and SV is stroke volume. The indexed stroke work (SW) was calculated as end-systolic pressure (ESP) × SV. The pressure–volume area (PVA), which corresponds to the total energy generated by each cardiac contraction, was calculated using the formula PVA = SW + PE, where PE is the potential energy stored at the end of systole (4). PE was calculated as ESP × ([ESV – V0]/2), assuming that V0 is negligible compared to the ESV. Using these variables, the SW index/pressure–volume area (SWI/PVA) ratio was further calculated, corresponding to the mechanical efficiency of converting the total mechanical energy (i.e., PVA) available to the left ventricular SW (4).
Echocardiographic Time Interval Measurement
T-IVT was calculated using the formula t-IVT = 60 – (t-ET + t-FT), where t-ET is the total ejection time, and t-FT is the total filling time (19). A t-IVT higher than 12 s/min was classified as systolic electromechanical inefficiency (i.e., intraventricular dyssynchrony) (24). A 10% improvement in t-IVT after treatment was considered a positive response. The Tei index was calculated as Tei index = isovolumic contraction time + isovolumic relaxation time/ejection time (29). The I/H index was calculated as I/H index = (isovolumic contraction time + isovolumic relaxation time)/(filling time + ejection time) (18).
Study Procedures
General demographic parameters were collected at the moment of inclusion (i.e., age, gender, type of surgery, and ventilation parameters). Patients were evaluated at two consecutive steps: (1) at the baseline and after therapeutic intervention and (2) after 15 min of haemodynamic stability, which is a change in MAP of <10%. The indication for haemodynamic treatment with fluid expansion, norepinephrine, or dobutamine infusion was left at the discretion of the physician in charge of the patient. All patients were sedated by continuous infusion of propofol and were fully adapted to volume-controlled mechanical ventilation. All patients were mechanically ventilated in a pressure-controlled mode with a positive end-expiratory pressure (PEEP) of 5 cm H2O. Ventilator settings (i.e., inspired oxygen fraction, tidal volume, respiratory rate, and PEEP) and propofol infusion rate were not modified during the study period.
Statistical Analyses
The database was complete; thus, no imputation strategy for missing data was necessary. The normal distribution of variables was assessed using histograms and the Shapiro-Wilk test. Data were expressed as numbers, proportions (in percent), medians [25–75% interquartile range], or as means (± standard deviation), as appropriate. The correlation was assessed using Pearson's or Spearman's tests, as appropriate. Paired data were compared using the Wilcoxon signed rank test.
Logistic regression models were performed to evaluate the relation between the baseline characteristics, the type of hemodynamic intervention, and baseline dyssynchrony status. Logistic regression models were further performed to evaluate the relation between the baseline characteristics, the type of hemodynamic intervention, and the response to the treatment. The responsive patients were defined as those with a decrease of at least 10% of baseline total isovolumic time after treatment. The conditions of validity for the logistic regression were verified, in order to have at least 5–10 events for each independent variable included in the model.
In the absence of previous data, we estimated that a sample size of 40 patients would allow us to demonstrate a correlation between t-IVT and CI or EA/EV higher than 0.4 using Spearman's correlation coefficient with a risk alpha of 5% and a power of 80%. Considering a 10% risk of loss or missing data, we decided to include 54 patients.
Statistical analyses were performed using RStudio (version 1.1.447©2009–2018 RStudio, Inc.) and SPSS® (IBM SPSS Statistics for Windows, version 21.0. Armonk, NY, IBM Corp.). A bilateral p-value of 0.05 was considered statistically significant.
Results
Fifty-four patients were included in the study. Their general characteristics are summarized in Table 1. Their mean age was 66 ± 12 years; they were predominantly males (78%), ASA III (75.9%) and IV (20.4%). The most common surgery was valvular surgery (52%), followed by coronary artery bypass graft surgery (19%). Approximately 43% of the patients underwent fluid expansion therapy, and 37 and 20% had norepinephrine and dobutamine infusions, respectively.
The haemodynamic parameters before and after therapeutic intervention for all cohorts are presented in Table 2. 39 (72%) of the 54 patients had a t-IVT over 12 s, and they were classified as having intraventricular dyssynchrony. Based on the logistic regression analysis, no demographic, baseline hemodynamic parameters and type of acute circulatory failure (hypovolemic, vasoplegic, cardiogenic) were associated to dyssynchrony (i.e., a t-IVT over 12 s) (Figure 1, Supplementary Table S1). In the whole cohort, baseline t-IVT was not correlated to CI, LVEF, HR, blood pressure, EV, cardiac power, or VA-coupling. The MAP, SVi, CI, and LVEF significantly changed with treatment in all cohorts, without modifying peripheral resistances and HR. The patients with intraventricular dyssynchrony had an indexed EDV of 56 (44–81) ml/m2, and the patients without baseline dyssynchrony had an index EDV of 62 (52–96) ml/m2, without a statistically significant difference (p = 0.161).
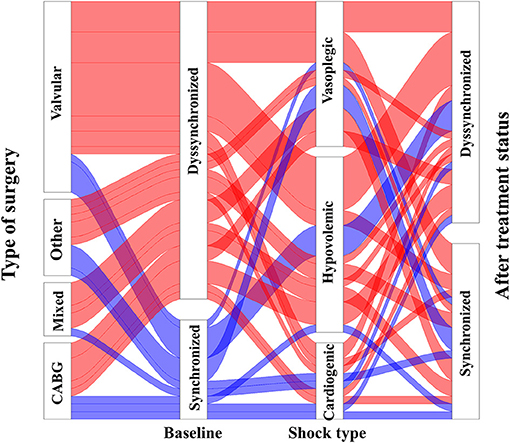
Figure 1. Representation of baseline ventricular dyssynchrony (t-IVT), and its evolution according to baseline and hemodynamic treatment.
Impact of Haemodynamic Interventions on Total Isovolumic Time
The haemodynamic parameters are presented in Table 2, according to the study group (i.e., with or without baseline intraventricular dyssynchrony) and before and after treatment. Changes of t-IVT was independent of type of hemodynamic treatment (p > 0.05, Figure 1, Supplementary Table S2). At baseline, prolonged t-IVT was due to a decreased filling time (33 ± 4 s vs. 26 ± 5 s, p < 0.001) and the ejection time being similar between the two groups (18.6 ± 4.6 s vs. 17.6 ± 2.7 s, p = 0.505). The baseline ratio of ejection time to filling time was high in patients with intraventricular dyssynchrony (0.73 ± 0.2 vs. 0.59 ± 0.2, p = 0.031).
Patients with baseline intraventricular dyssynchrony significantly improved their t-IVT (Figure 2, Table 2). This improvement was mainly due to an increase in total ejection time. By contrast, patients without baseline dyssynchrony significantly prolonged their t-IVT with treatment (Figure 2) because of an increase in filling time without changing the ejection time. The type of haemodynamic treatment showed no effect on t-IVT changes (p = 0.906). Patients with intraventricular dyssynchrony significantly improved EV, ventriculo-arterial coupling (Figure 3A), and SWI/PVA (Figure 3B). The improvement of ventriculo-arterial coupling was due to an increase in EV without changing EA.
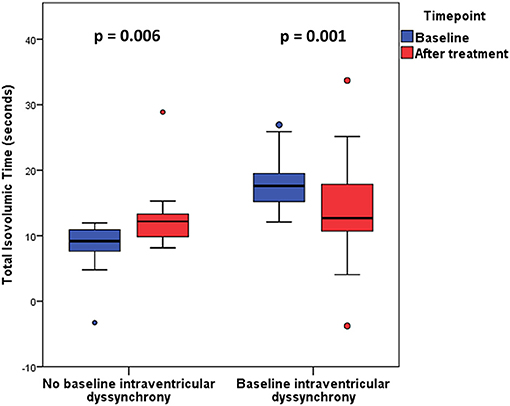
Figure 2. Total Isovolumic time before and after treatment in patients with and without baseline intraventricular dyssynchrony.
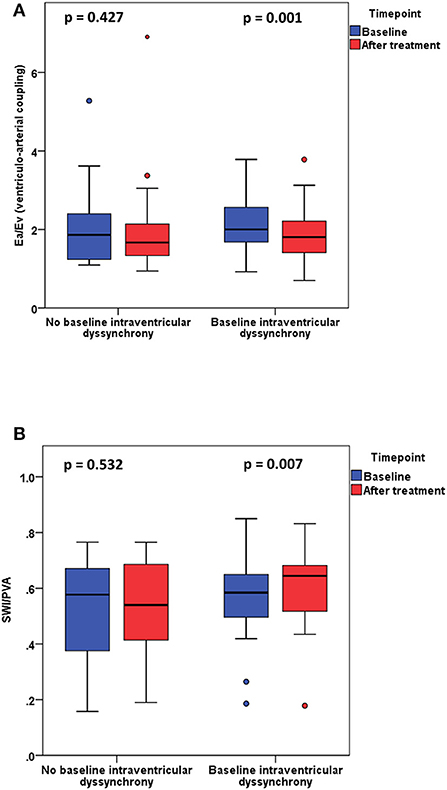
Figure 3. (A) Ventriculo-arterial coupling (EA/EV) before and after treatment in patients with and without baseline intraventricular dyssynchrony. (B) Stroke work index/pressure volume area (SWI/PVA) before and after treatment in patients with and without baseline intraventricular dyssynchrony.
Impact of Haemodynamic Interventions on I/H and Tei Indices
The Tei index decreased with haemodynamic treatment (0.83 ± 0.3 vs. 0.77 ± 0.3, p = 0.001). The decrease was highly statistically significant in the group with baseline intraventricular dyssynchrony. The Tei-index was correlated with t-IVT at baseline (rho = 0.684, p < 0.001) and after treatment (rho = 0.719, p < 0.001). The changes in the Tei index were different between the treatment subgroups (p = 0.03). The Tei index response seemed higher in the norepinephrine subgroup [−32% (−47; −14)] compared with that in the fluid expansion subgroup [−8% (−30; 14)] and dobutamine subgroup [−3% (−27; 53)].
The I/H index did not change with treatment in the cohort as a whole. Nevertheless, it significantly improved in patients with intraventricular dyssynchrony, whereas it worsened in patients without intraventricular dyssynchrony. I/H index and t-IVT were correlated at baseline (rho = 0.997, p < 0.001) and after treatment (rho = 1, p < 0.001). The type of haemodynamic treatment was not associated with I/H index changes (p = 0.875).
Discussion
This study demonstrated a link between cardiac electromechanical coupling and ventriculo-arterial coupling in patients suffering from acute circulatory failure. Our results can be summarized as follows: (1) patients suffering from acute circulatory failure exhibited cardiac electromechanical uncoupling, independently of the macro-hemodynamic status, type of shock, and patient's characteristics, (2) the cardiac electromechanical coupling was improved by haemodynamic treatment only if altered at baseline (i.e., in patients with intraventricular dyssynchrony), and (3) the improvement of cardiac electromechanical coupling was associated with improvement of markers of cardiocirculatory efficacy and efficiency (i.e., SWI/PVA and ventriculo-arterial coupling).
Ventriculo-arterial coupling considers the complex relationship between ventricular and arterial system elastances, and it exhibits a strong link with cardiac energetics and cardiac efficiency (3, 4, 30, 31). The mechanism of ventriculo-arterial coupling improvement in our cohort, and specifically for patients with baseline dyssynchrony, was mainly through the amelioration of EV. The increase in EV with a steady EA induced the improvement of ventriculo-arterial coupling.
An association between t-IVT and macro-haemodynamic parameters (blood pressure, cardiac output) was observed in patients in the general ICU (24). Baseline t-IVT is able to predict further post-operative events (21). We did not find such an association in our cohort. This discrepancy could be explained by the fact that we studied patients during acute hemodynamic resuscitation phase, where the patients are mostly hemodynamically instable with arterial hypotension and myocardial perfusion mismatch. Several studies have highlighted the complex relationship between ventricular dyssynchrony, aortic blood pressure, coronary flow, and myocardial contractility (32–34). Tavazzi et al. (24) already demonstrated that the underlying disease (i.e., cardiovascular or respiratory) was not associated to ventricular dyssynchrony. As we did not find any association between the type of cardiac surgery and prevalence of ventricular dyssynchrony, we confirmed this point. t-IVT should probably be analyzed as a global marker of ventricular dyssynchrony that reflect whole myocardial performance independently of underlying myocardial disease per se.
In our study, the improvement of t-IVT in patients with baseline dyssynchrony was associated with an improvement in ejection time in contrast with the filling time. At baseline, the ratio between ejection time and filling time was high in the case of dyssynchrony. After haemodynamic intervention, this ratio slightly increased in the dyssynchrony group and significantly increased in the other groups. Given that the t-IVT may be considered as “wasted time” during the heart contraction cycle (18), any improvement of the cardiac electromechanical coupling may be associated with an improvement in cardiac function EV. Tavazzi et al. (22) previously demonstrated that patients suffering from cardiogenic shock can be treated with t-IVT-based pacemaker resynchronisation. Although evaluating the effects of each haemodynamic treatment is not an objective of the study, the robustness of t-IVT was suggested by the fact that we observed the same response for the Tei index and I/H index, which are the two known indexes of cardiac efficiency. These indices showed good correlation values.
Clinical Implications
The study results are of interest, as the Chen's non-invasive determination of ventriculo-arterial coupling does not explore the ventricular loading (3). Our findings confirmed the hypotheses of previous studies that acute circulatory failure can be associated with intraventricular dyssynchrony, and that t-IVT is a potential target for titrating-specific haemodynamic therapy (22). t-IVT has additional value to ventriculo-arterial coupling in patients suffering from acute circulatory failure. We observed a high prevalence of intraventricular dyssynchrony in ICU patients (24), and the cardiologic literature on this subject has already demonstrated an association between electromechanical dyssynchrony and clinical outcomes (11, 13). Studies have suggested an effect on the electromechanical coupling of several haemodynamic treatments.
Several ICU studies considered the titration of haemodynamic treatments based on ventriculo-arterial coupling measurements (7, 9, 35), and algorithms have even been proposed (25, 36). During septic shock, fluid expansion can improve left ventricular relaxation (37). HR modulation improves ventriculo-arterial coupling in patients with septic shock; however, no data are available regarding the echocardiographic time intervals in general ICU patients (38). The interference of norepinephrine infusion with diastolic function has also been noted (39). Tavazzi et al. (23) obtained haemodynamic improvement using echocardiography-guided pacemaker optimisation of t-IVT—a method that is not easily available at bedside. In our study, the changes in t-IVT were independent of the haemodynamic treatment used for acute circulatory failure, suggesting a role for the relationship between myocardial perfusion, myocardial contractility, and ventricular dyssynchrony in acute circulatory failure.
A future perspective is the evaluation of targeting t-IVT in patients with septic shock. The optimisation of ventriculo-arterial coupling is feasible and associated with haemodynamic and tissue perfusion improvement (40). Considering septic cardiomyopathy (41), t-IVT determination may provide further understanding of the complex pathophysiology and management aspects of critically ill patients. Furthermore, our results suggested that haemodynamically unstable patients with prolonged t-IVT benefit more from further haemodynamic interventions. In the era of “less is more” ICU approach, this finding is of clinical significance in acute settings. As it suggests, t-IVT may be used to discern patients in whom rapid and further therapeutic interventions must be administered, from patients for whom a temporary conservatory approach must be selected.
Limitations
Our study can be discussed on several points. This study is an observational study that demonstrated association but not causal relation. One limitation is the heterogeneity and the limited number of patients in the study. We performed a sensitivity analysis to determine which factors were associated with ventricular dyssynchrony. None of baseline demographic and hemodynamic parameters were associated to ventricular dyssynchrony. Moreover, we were unable to demonstrate association between the type of hemodynamic treatment and ventricular dyssynchrony. The changes in t-IVT were independent of the type of haemodynamic treatment, and the proportion of each haemodynamic intervention did not differ between the two groups of patients. The aforementioned relationship between blood pressure, coronary blood perfusion, and ventricular dyssynchrony may explain (in part) our results, and the fact that during acute phase of shock the main determinant of ventricular dyssynchrony may be heart blood perfusion and cardiovascular interactions. The sample size of the present study may be limited but we performed a sample size calculation based on the study of Tavazzi et al. (34). Previous studies evaluating the t-IVT and/or the hemodynamic effect had sample size close to this of the present study (7, 21). In addition, the purpose of our work was not to precisely describe the individual effects of each haemodynamic treatment on the components of ventriculo-arterial coupling or t-IVT. Several studies already considered and described these effects (7–9). Another point of discussion is the risk of mathematical coupling between t-IVT and EV because we used Chen's method, which includes systolic ejection time. However, t-IVT also includes isovolumic relaxation time, which is not only fully determined by ejection time. Moreover, the t-IVT formula includes the filling time. We observed the changes of each component (i.e., systolic and filling time) of this index. In addition, we also evaluated other indices, that is, the Tei and I/H indexes, to broaden our perspective. The present study may be considered as the first one to highlight an association between ventricular dyssynchrony and ventriculo-arterial coupling in acute circulatory failure. Further studies may confirm our results, and they will focus on the mechanisms involved in ventricular dyssynchrony during acute circulatory failure.
Conclusion
Acute circulatory failure is associated with electromechanical dyssynchrony (i.e., prolonged t-IVT). Patients with electromechanical dyssynchrony are those who further benefit from haemodynamic treatment in terms of cardiac efficacy and efficiency. T-IVT may represent a potential target for haemodynamic monitoring and optimisation in patients suffering from acute circulatory failure.
Data Availability Statement
The raw data supporting the conclusions of this article will be made available by the authors, without undue reservation.
Ethics Statement
The studies involving human participants were reviewed and approved by the Comité de Protection des Personnes Nord-Ouest II CHU - Place V. Pauchet, 80054 AMIENS Cedex 1. The patients/participants provided their written informed consent to participate in this study.
Author Contributions
SA and P-GG conceived the study, analyzed the data, and drafted the manuscript. VC and P-GG collected the data. BP, MN, and BB provided a critical revision of the manuscript. All authors contributed to the article and approved the submitted version.
Conflict of Interest
The authors declare that the research was conducted in the absence of any commercial or financial relationships that could be construed as a potential conflict of interest.
Publisher's Note
All claims expressed in this article are solely those of the authors and do not necessarily represent those of their affiliated organizations, or those of the publisher, the editors and the reviewers. Any product that may be evaluated in this article, or claim that may be made by its manufacturer, is not guaranteed or endorsed by the publisher.
Supplementary Material
The Supplementary Material for this article can be found online at: https://www.frontiersin.org/articles/10.3389/fcvm.2022.907891/full#supplementary-material
References
1. Trambaiolo P, Figliuzzi I, Salvati M, Bertini P, Brizzi G, Tocci G, et al. Ventriculo-arterial coupling in the intensive cardiac care unit: a non-invasive prognostic parameter. Int J Cardiol. (2022) 348:85–9. doi: 10.1016/j.ijcard.2021.12.026
2. Monge García MI, Santos A. Understanding ventriculo-arterial coupling. Ann Transl Med. (2020) 8:795. doi: 10.21037/atm.2020.04.10
3. Ikonomidis I, Aboyans V, Blacher J, Brodmann M, Brutsaert DL, Chirinos JA, et al. The role of ventricular-arterial coupling in cardiac disease and heart failure: assessment, clinical implications and therapeutic interventions. A consensus document of the European society of cardiology working group on aorta & peripheral vascular diseases, European association of cardiovascular imaging, and heart failure association. Eur J Heart Fail. (2019) 21:402–24. doi: 10.1002/ejhf.1436
4. Takaoka H, Takeuchi M, Odake M, Yokoyama M. Assessment of myocardial oxygen consumption (Vo2) and systolic pressure-volume area (PVA) in human hearts. Eur Heart J. (1992) 13:85–90. doi: 10.1093/eurheartj/13.suppl_E.85
5. Andrei S, Nguyen M, Longrois D, Popescu BA, Bouhemad B, Guinot PG. Ventriculo-arterial coupling is associated with oxygen consumption and tissue perfusion in acute circulatory failure. Front Cardiovasc Med. (2022) 9:842554. doi: 10.3389/fcvm.2022.842554
6. Sunagawa K, Sagawa K, Maughan WL. Ventricular interaction with the loading system. Ann Biomed Eng. (1984) 12:163–89. doi: 10.1007/BF02584229
7. Guinot PG, Longrois D, Kamel S, Lorne E, Dupont H. Ventriculo-arterial coupling analysis predicts the hemodynamic response to norepinephrine in hypotensive postoperative patients: a prospective observational study. Crit Care Med. (2018) 46:e17–25. doi: 10.1097/CCM.0000000000002772
8. Zhou X, Pan J, Wang Y, Wang H, Xu Z, Zhuo W. Left ventricular-arterial coupling as a predictor of stroke volume response to norepinephrine in septic shock—a prospective cohort study. BMC Anesthesiol. (2021) 21:56. doi: 10.1186/s12871-021-01276-y
9. Huette P, Abou-Arab O, Longrois D, Guinot PG. Fluid expansion improve ventriculo-arterial coupling in preload-dependent patients: a prospective observational study. BMC Anesthesiol. (2020) 20:171. doi: 10.1186/s12871-020-01087-7
10. Antohi EL, Chioncel O, Mihaileanu S. Overcoming the limits of ejection fraction and ventricular-arterial coupling in heart failure. Front Cardiovasc Med. (2021) 8:750965. doi: 10.3389/fcvm.2021.750965
11. Shishido T, Hayashi K, Shigemi K, Sato T, Sugimachi M, Sunagawa K. Single-beat estimation of end-systolic elastance using bilinearly approximated time-varying elastance curve. Circulation. (2000) 102:1983–9. doi: 10.1161/01.CIR.102.16.1983
12. Chen CH, Fetics B, Nevo E, Rochitte CE, Chiou KR, Ding PA, et al. Non-invasive single-beat determination of left ventricular end-systolic elastance in humans. J Am Coll Cardiol. (2001) 38:2028–34. doi: 10.1016/S0735-1097(01)01651-5
13. Tei C. New non-invasive index for combined systolic and diastolic ventricular function. J Cardiol. (1995) 26:135–6.
14. Tei C, Ling LH, Hodge DO, Bailey KR, Oh JK, Rodeheffer RJ, et al. New index of combined systolic and diastolic myocardial performance: a simple and reproducible measure of cardiac function—a study in normals and dilated cardiomyopathy. J Cardiol. (1995) 26:357–66. doi: 10.1016/S0894-7317(05)80111-7
15. Bennett S, Cubukcu A, Wong CW, Griffith T, Oxley C, Barker D, et al. The role of the tei index in assessing for cardiotoxicity from anthracycline chemotherapy: a systematic review. Echo Res Pract. (2021) 8(1):R1–11. doi: 10.1530/ERP-20-0013
16. Bajraktari G, Batalli A, Poniku A, Ahmeti A, Olloni R, Hyseni V, et al. Left ventricular markers of global dyssynchrony predict limited exercise capacity in heart failure, but not in patients with preserved ejection fraction. Cardiovasc Ultrasound. (2012) 10:36. doi: 10.1186/1476-7120-10-36
17. Bennett S, Wong CW, Griffiths T, Stout M, Khan JN, Duckett S, et al. The prognostic value of tei index in acute myocardial infarction: a systematic review. Echo Res Pract. (2020) 7:49–58. doi: 10.1530/ERP-20-0017
18. Schwammenthal E, Adler Y, Amichai K, Sagie A, Behar S, Hod H, et al. Prognostic value of global myocardial performance indices in acute myocardial infarction: comparison to measures of systolic and diastolic left ventricular function. Chest. (2003) 124:1645–51. doi: 10.1378/chest.124.5.1645
19. Duncan AM, Francis DP, Henein MY, Gibson DG. Limitation of cardiac output by total isovolumic time during pharmacologic stress in patients with dilated cardiomyopathy: activation-mediated effects of leftbundle branch block and coronary artery disease. J Am Coll Cardiol. (2003) 41:121–8. doi: 10.1016/S0735-1097(02)02665-7
20. Duncan AM, Francis DP, Henein MY, Gibson DG. Importance of left ventricular activation in determining myocardial performance (tei) index: comparison with total isovolumic time. Int J Cardiol. (2004) 95:211–7. doi: 10.1016/j.ijcard.2003.07.007
21. Bajraktari G, Duncan A, Pepper J, Henein M. Prolonged total isovolumic time predicts cardiac events following coronary artery bypass surgery. Eur J Echocardiogr. (2008) 9:779–83. doi: 10.1093/ejechocard/jen146
22. Tavazzi G, Kontogeorgis A, Bergsland NP, Price S. Resolution of cardiogenic shock using echocardiography-guided pacing optimization in intensive care: a case series. Crit Care Med. (2016) 44:e755–761. doi: 10.1097/CCM.0000000000001655
23. Tavazzi G, Kontogeorgis A, Guarracino F, Bergsland N, Martinez-Naharro A, Pepper J, et al. Heart rate modification of cardiac output following cardiac surgery: the importance of cardiac time intervals*. Crit Care Med. (2017) 45:e782–8. doi: 10.1097/CCM.0000000000002410
24. Tavazzi G, Dammassa V, Corradi F, Klersy C, Patel B, Pires AB, et al. Correlation between echocardiographic and hemodynamic variables in cardiothoracic intensive care unit. J Cardiothorac Vasc Anesth. (2020) 34:1263–9. doi: 10.1053/j.jvca.2020.01.052
25. Guinot PG, Martin A, Berthoud V, Voizeux P, Bartamian L, Santangelo E, et al. Vasopressor-sparing strategies in patients with shock: a scoping-review and an evidence-based strategy proposition. J Clin Med. (2021) 10:3164. doi: 10.3390/jcm10143164
26. Starling MR. Left ventricular-arterial coupling relations in the normal human heart. Am Heart J. (1993) 125:1659–66. doi: 10.1016/0002-8703(93)90756-Y
27. Chen CH, Nakayama M, Nevo E, Fetics BJ, Maughan WL, Kass DA. Coupled systolic-ventricular and vascular stiffening with age: implications for pressure regulation and cardiac reserve in the elderly. J Am Coll Cardiol. (1998) 32:1221–7. doi: 10.1016/S0735-1097(98)00374-X
28. Nguyen M, Berhoud V, Bartamian L, Martin A, Ellouze O, Bouhemad B, et al. Agreement between different non-invasive methods of ventricular elastance assessment for the monitoring of ventricular-arterial coupling in intensive care. J Clin Monit Comput. (2020) 34:893–901. doi: 10.1007/s10877-019-00397-7
29. Kim WH, Otsuji Y, Seward JB, Tei C. Estimation of left ventricular function in right ventricular volume and pressure overload. Detection of early left ventricular dysfunction by tei index. Jpn Heart J. (1999) 40:145–54. doi: 10.1536/jhj.40.145
30. Ky B, French B, May Khan A, Plappert T, Wang A, Chirinos JA, et al. Ventricular-arterial coupling, remodeling, and prognosis in chronic heart failure. J Am Coll Cardiol. (2013) 62:1165–72. doi: 10.1016/j.jacc.2013.03.085
31. Chang MC, Mondy JS, Meredith JW, Holcroft JW. Redefining cardiovascular performance during resuscitation: ventricular stroke work, power, and the pressure-volume diagram. J Trauma. (1998) 45:470–8. doi: 10.1097/00005373-199809000-00007
32. Fan L, Namani R, Choy JS, Kassab GS, Lee LC. Effects of mechanical dyssynchrony on coronary flow: insights from a computational model of coupled coronary perfusion with systemic circulation. Front Physiol. (2020) 11:915. doi: 10.3389/fphys.2020.00915
33. Munneke AG, Lumens J, Arts T, Delhaas T. A closed-loop modeling framework for cardiac-to-coronary coupling. Front Physiol. (2022) 13:830925. doi: 10.3389/fphys.2022.830925
34. van Houwelingen MJ, Merkus D, Te Lintel Hekkert M, van Dijk G, Hoeks APG, Duncker DJ. Coronary-aortic interaction during ventricular isovolumic contraction. Med Biol Eng Comput. (2011) 49:917–24. doi: 10.1007/s11517-011-0770-y
35. Chang MC, Martin RS, Scherer LA, Meredith JW. Improving ventricular-arterial coupling during resuscitation from shock: effects on cardiovascular function and systemic perfusion. J Trauma. (2002) 53:679–85. doi: 10.1097/00005373-200210000-00010
36. Guarracino F, Ferro B, Morelli A, Bertini P, Baldassarri R, Pinsky MR. Ventriculoarterial decoupling in human septic shock. Crit Care. (2014) 18:R80. doi: 10.1186/cc13842
37. Mahjoub Y, Benoit-Fallet H, Airapetian N, Lorne E, Levrard M, Seydi AA, et al. Improvement of left ventricular relaxation as assessed by tissue doppler imaging in fluid-responsive critically ill septic patients. Intensive Care Med. (2012) 38:1461–70. doi: 10.1007/s00134-012-2618-9
38. Morelli A, Singer M, Ranieri VM, D'Egidio A, Mascia L, Orecchioni A, et al. Heart rate reduction with esmolol is associated with improved arterial elastance in patients with septic shock: a prospective observational study. Intensive Care Med. (2016) 42:1528–34. doi: 10.1007/s00134-016-4351-2
39. Niwa H, Hirota Y, Sibutani T, Idohji Y, Hori T, Sugiyama K, et al. The effects of epinephrine and norepinephrine administered during local anesthesia on left ventricular diastolic function. Anesth Prog. (1991) 38:221–6.
40. Zhou X, Zhang Y, Pan J, Wang Y, Wang H, Xu Z, et al. Optimizing left ventricular-arterial coupling during the initial resuscitation in septic shock—a pilot prospective randomized study. BMC Anesthesiol. (2022) 22:31. doi: 10.1186/s12871-021-01553-w
Keywords: electromechanical coupling, ventriculo-arterial coupling, shock, fluid therapy, inotrope, vasopressor, total isovolumic time, cardiac resynchronization
Citation: Andrei S, Popescu BA, Caruso V, Nguyen M, Bouhemad B and Guinot P-G (2022) Role of Electromechanical Dyssynchrony Assessment During Acute Circulatory Failure and Its Relation to Ventriculo-Arterial Coupling. Front. Cardiovasc. Med. 9:907891. doi: 10.3389/fcvm.2022.907891
Received: 30 March 2022; Accepted: 24 May 2022;
Published: 21 June 2022.
Edited by:
Alberto Giannoni, Sant'Anna School of Advanced Studies, ItalyReviewed by:
Joris Lemson, Radboud University Nijmegen, NetherlandsIacopo Fabiani, Gabriele Monasterio Tuscany Foundation (CNR), Italy
Copyright © 2022 Andrei, Popescu, Caruso, Nguyen, Bouhemad and Guinot. This is an open-access article distributed under the terms of the Creative Commons Attribution License (CC BY). The use, distribution or reproduction in other forums is permitted, provided the original author(s) and the copyright owner(s) are credited and that the original publication in this journal is cited, in accordance with accepted academic practice. No use, distribution or reproduction is permitted which does not comply with these terms.
*Correspondence: Stefan Andrei, c3RlZmFuLm0uYW5kcmVpQGdtYWlsLmNvbQ==