- 1Department of Cardiovascular Medicine, Graduate School of Medicine, University of Tokyo, Bunkyo-ku, Japan
- 2Department of Therapeutic Strategy for Heart Failure, University of Tokyo, Bunkyo-ku, Japan
- 3Advanced Medical Center for Heart Failure, University of Tokyo, Bunkyo-ku, Japan
- 4Department of Computational Radiology and Preventive Medicine, The University of Tokyo Hospital, Bunkyo-ku, Japan
- 5Department of Cardiac Surgery, University of Tokyo, Bunkyo-ku, Japan
Aims: We compared hemodynamics and clinical events after heart transplantation (HTx) in patients stratified by the severity of residual pulmonary vascular resistance (PVR) after left ventricular assist device (LVAD) implantation for bridge to transplantation.
Methods: We retrospectively analyzed patients who had undergone HTx at the University of Tokyo Hospital. We defined the high PVR group as patients with PVR of >3 Wood Units (WU) as measured by right heart catheterization performed 1 month after LVAD implantation.
Results: We included 85 consecutive HTx recipients, 20 of whom were classified in the high PVR group and 65 in the low PVR group. The difference in PVR between the two groups became apparent at 2 years after HTx (the high PVR group: 1.77 ± 0.41 WU, the low PVR group: 1.24 ± 0.59 WU, p = 0.0009). The differences in mean pulmonary artery pressure (mPAP), mean right arterial pressure (mRAP), and mean pulmonary capillary wedge pressure (mPCWP) tended to increase from the first year after HTx, and were all significantly higher in the high PVR group at 3 years after HTx (mPAP: 22.7 ± 9.0 mm Hg vs. 15.4 ± 4.3 mm Hg, p = 0.0009, mRAP: 7.2 ± 3.6 mm Hg vs. 4.1 ± 2.1 mm Hg, p = 0.0042, and mPCWP: 13.4 ± 4.5 mm Hg, 8.8 ± 3.3 mm Hg, p = 0.0040). In addition, pulmonary artery pulsatility index was significantly lower in the high PVR group than in the low PVR group at 3 years after HTx (2.51 ± 1.00 vs. 5.21 ± 3.23, p = 0.0033). The composite event including hospitalization for heart failure, diuretic use, and elevated intracardiac pressure (mRAP ≥ 12 mm Hg or mPCWP ≥ 18 mm Hg) between the two groups was significantly more common in the high PVR group. Residual high PVR was still an important predictor (hazard ratio 6.5, 95% confidence interval 2.0–21.6, and p = 0.0023) after multivariate Cox regression analysis.
Conclusion: Our study demonstrates that patients with residual high PVR under LVAD implantation showed the increase of right and left atrial pressure in the chronic phase after HTx.
Introduction
Heart transplantation (HTx) is a treatment method for severe heart failure in which a patient’s heart, which cannot be saved by conventional treatment methods, is replaced with a healthy heart from a brain-dead person. However, shortage of donor heart is a common worldwide issue that limits the benefit of HTx (1, 2). To overcome the issue about shortage of donor, mechanical circulatory support is available as bridge to transplant (BTT) therapy (3). Indeed, in Japan, most patients require a ventricular assist device as a BTT device that can be used for a long time before HTx. Generally, patients with severe heart failure often have pulmonary hypertension, which is a poor prognostic factor (4). Sustained retrograde propagation of increased left atrial pressure to the pulmonary artery due to left heart failure leads to contraction and remodeling of the pulmonary artery, resulting in increased pulmonary vascular resistance (PVR) and, ultimately, right ventricular dysfunction (5, 6). In patients with heart failure, a left ventricular assist device (LVAD) reduces the load on the left ventricle and PVR (7–9) but long-term burden derived from left-sided heart failure on the pulmonary vascular system is thought to cause irreversible changes in the pulmonary vascular system (6). LVAD support alleviates the burden of left side heart and decrease the left atrial pressure significantly, resulting in improvement of pulmonary hypertension (10). However, some cases in which PVR increase could not be improved sufficiently under LVAD support. Since the recipient’s pulmonary vascular system remains unaffected by HTx, recipient pulmonary vascular degeneration may affect the post-HTx course. There are many unclear points about the impacts of pre-HTx PVR on long-term clinical course after HTx. Therefore, in this study, we investigated the clinical parameters and hemodynamics after HTx in patients stratified by the severity of PVR before HTx, specifically after LVAD implantation.
Materials and Methods
Patient Selection
All patients were implanted with LVAD at our institution between October 2004 and September 2018 or visited our institution’s outpatient clinic after undergoing LVAD implantation at another institution during the same period. Patients who underwent right heart catheterization for hemodynamic evaluation 1 month after LVAD implantation and were ultimately able to undergo cardiac transplantation were selected. Patients who died within 30 days of HTx were excluded (N = 2). LVAD therapy included HeartMate II (Thoratec Corporation, Pleasanton, United States), EVAHEART (Sun Medical Company, Moriyama, Tokyo, Japan), Jarvik 2000 (Century Medical, Inc., Tokyo, Japan), DuraHeart (TERUMO, Tokyo, Japan), HVAD (Medtronic PLC, Dublin, Ireland), and Nipro-VAD (Nipro Corporation., Osaka, Japan). The study protocol conformed to the tenets of the Declaration of Helsinki, and was reviewed and approved by the University of Tokyo Institutional Review Board (approval number: 2650).
Procedure of Heart Transplantation
All transplanted hearts were obtained from a brain-dead donor with a beating heart, stored in a cooled cardiac preservation solution, and transported cold. All heart transplants were performed using standard procedures with bicaval anastomoses. All patients who underwent transplantation were treated with standard immunosuppressive therapy using three drugs: calcineurin inhibitor, mycophenolate mofetil, and prednisolone. In the middle of the course, there were some cases in which mycophenolate mofetil was changed to everolimus. The doses of calcineurin inhibitor and everolimus were strictly controlled by adjusting the trough concentration according to the number of weeks after transplantation. Prednisolone administration was decreased over time and discontinued within a year. When mild acute cellular rejection (ACR; grade ≤ 1R) was present, only optimization of immunosuppression was performed, but when moderate ACR [grade ≥ 2R, which means that two or more foci of mononuclear cells (lymphocytes/macrophages) with associated myocyte damage are present (11, 12)] was present, optimization of immunosuppression and pulse steroids was performed. When antibody-mediated rejection was diagnosed, optimization of maintenance immunosuppression, intravenous gamma globulin, pulse steroids, or plasmapheresis was considered.
Cardiac Catheterization
One month after LVAD implantation, right heart catheterization was performed for hemodynamic evaluation. Conversely, after HTx, right heart catheterization and endomyocardial biopsy from the right ventricular middle septum were regularly performed 1, 2, 3, 4, 6, 8, 12, 18, and 24 weeks according to the institutional protocol. It was performed yearly, 1 year after HTx. In this study, we used right heart catheterization data 1 month after LVAD implantation and 24 weeks, 1 year, 2 years, and 3 years after HTx. Right heart catheterization was performed by inserting a Swan Ganz catheter through the jugular or femoral vein. Right atrial pressure (RAP), right ventricular pressure, pulmonary artery pressure (PAP), pulmonary artery wedge pressure (PCWP), cardiac output (CO), and cardiac index (CI) were measured by Fick’s principle. In this study, we used mean right atrioventricular pressure (mRAP), mean pulmonary artery pressure (mPAP), mean pulmonary artery wedge pressure (mPCWP), and CI. The PVR, pulmonary artery pulsatility index (PAPi), diastolic pressure gradient (DPG), and transpulmonary gradient (TPG) were calculated as follows; PVR, (mPAP-mPCWP)/CO; PAPi, (sPAP-dPAP)/mRAP; DPG, dPAP-mPCWP; and TPG, mPAP-mPCWP. On the LVAD support, the setting of LVAD speed was generally set with reference of clinical findings and echocardiographic findings before right heart catheterization. In the right heart catheterization, hemodynamic data and echocardiographic measurements were recorded at the patient’s initial baseline LVAD speed. The LVAD speed was then lowered to several speeds with repeat collection of hemodynamic and echocardiographic data. The LVAD speed was then increased to several speeds with repeat data collection at each interval. We got the data of hemodynamics at the first LVAD speed in right heart catheterization. The right heart catheterization was identically performed for different LVADs.
Blood Test
Blood tests were performed 1 month after LVAD implantation and sequentially after HTx. In this study, we collected data at 1 month after LVAD implantation and 4 weeks, 24 weeks, 1 year, and 3 years after HTx.
Echocardiography
Echocardiography was performed sequentially after HTx. Normal echocardiographic parameters, including left ventricular diastolic dimension (LVDs), left ventricular dimension (LVDd), left ventricular ejection fraction (LVEF), and E/e’, were evaluated. The e’ value is the average of the values measured at both the septum and the lateral wall. We collected the data 1 week, 24 weeks, 1 year, and 3 years after HTx.
Endomyocardial Biopsy
Using the diagnostic criteria for ACR by the International Society for Heart and Lung Transplantation (ISHLT), cellular rejection of grade 2R (ISHLT2004)/3A (ISHLT1990), or higher was classified as positive ACR.
Evaluated Variables
Right ventricular failure (RVF) was defined and classified according to the International Mechanically Assisted Circulatory Support (INTERMACS) Registry, in which late RVF was defined as meeting the moderate or severe INTERMACS RVF definition after discharge from the index LVAD implant, presence of inotrope, use of intravenous or inhaled pulmonary vasodilators for a duration of >1 week, or needing RV assist device implantation (13).
Donor-recipient sex mismatch was defined as a female donor to a male recipient (14). Donor-recipient body weight mismatch was defined as the ratio of donor-to-recipient weight to <−20% or >30% (12).
In this study, using the general definition of pulmonary hypertension (15), cases with PVR > 3 Wood units (WU) on right heart catheterization 1 month after LVAD implantation were classified as the high PVR group and others were defined as the low PVR group.
During follow-up after HTx, we defined worsening heart failure as the composite including heart failure hospitalization, diuretic use for the treatment of congestion, and elevated intracardiac pressure (16).
Statistical Analysis
Data were expressed as mean ± standard deviation or median (interquartile range). Statistical analysis was performed using JMP software (version 14.2; SAS Institute, Cary, NC, United States). Student’s t-test or Mann–Whitney U test was used for continuous variables, while Fisher’s exact test was used for categorical variables. Right heart catheter and blood data from a baseline of 1 month after LVAD implantation to each period after HTx and echocardiography data from the harvest to each period after HTx were analyzed using the paired t-test or Wilcoxon signed-rank test. The Bonferroni method was used to assess the significance of the multiple comparisons. To examine changes over time in PVR, PAPi, mRAP, mPAP, mPCWP, and CI, the mean value of each parameter was graphed in patients stratified by baseline PVR. Kaplan–Meier survival analysis was performed to assess (1) survival after HTx; (2) hospitalization for heart failure; and (3) composite events of hospitalization for heart failure, diuretic use for the treatment of congestion, and elevated intracardiac pressure (mRAP ≥ 12 mm Hg or mPCWP ≥ 18 mm Hg) between the two groups, which were compared using a log-rank test. Cox regression analysis was performed to explore significant predictors of the composite events of heart failure hospitalization, diuretic use for the treatment of congestion, and elevated intracardiac pressure. The cutoff value of each variable, except BMI and eGFR, for the hazard analysis was calculated using a receiver operating characteristic curve. We selected values that maximized the sum of sensitivity and specificity as the cutoff values to calculate the area under the curve. Multivariate analysis was performed using variables for which P < 0.1 was obtained by univariate analysis. Statistical significance was set at a P-value < 0.05.
Results
Baseline Patient and Transplanted Heart Characteristics
Flow of patients through the study is presented in Figure 1. A total of 218 patients were implanted with LVAD during the study period. Of these, 19 were excluded due to death before HTx, 9 were excluded due to removal of LVAD due to functional recovery, 42 were excluded because they were still awaiting HTx under LVAD, and 63 were excluded due to absence of right heart catheter data at 1 month after LVAD implantation. A total of 85 patients were eligible for the final analysis of which 20 patients were classified in the high PVR group and 65 in the low PVR group. Table 1 shows the baseline characteristics (which was measured on LVAD support) of two groups. Age at HTx was significantly older in the high PVR group (high PVR group, 45.7 ± 12.0 years; low PVR group, 39.0 ± 13.1 years; p = 0.043). BMI was also significantly higher in the high PVR group (high PVR group, 23.2 ± 3.9; low PVR group, 21.5 ± 3.1; p = 0.046). According to the types of LVAD, 29 patients were implanted with HeartMate II, 20 patients with EVAHERAT, 16 patients with Jarvik 2000, 13 patients with DuraHeart, 6 patients with Nipro-LVAD, and 1 patient with HVAD. No significant differences were found in the LVAD model between the two groups. There was no significant difference in the presence of significant tricuspid regurgitation. During LVAD support, RVF was observed in 12 patients (14.1%), which was not associated with the presence of high PVR. Table 2 shows the clinical characteristics in the donors and relationship between donors and recipients in the two groups. There was no significant difference in basic donor and echocardiographic parameters at the time of harvest between the two groups. Donor-to-recipient body weight mismatch was significantly higher in the low PVR group.
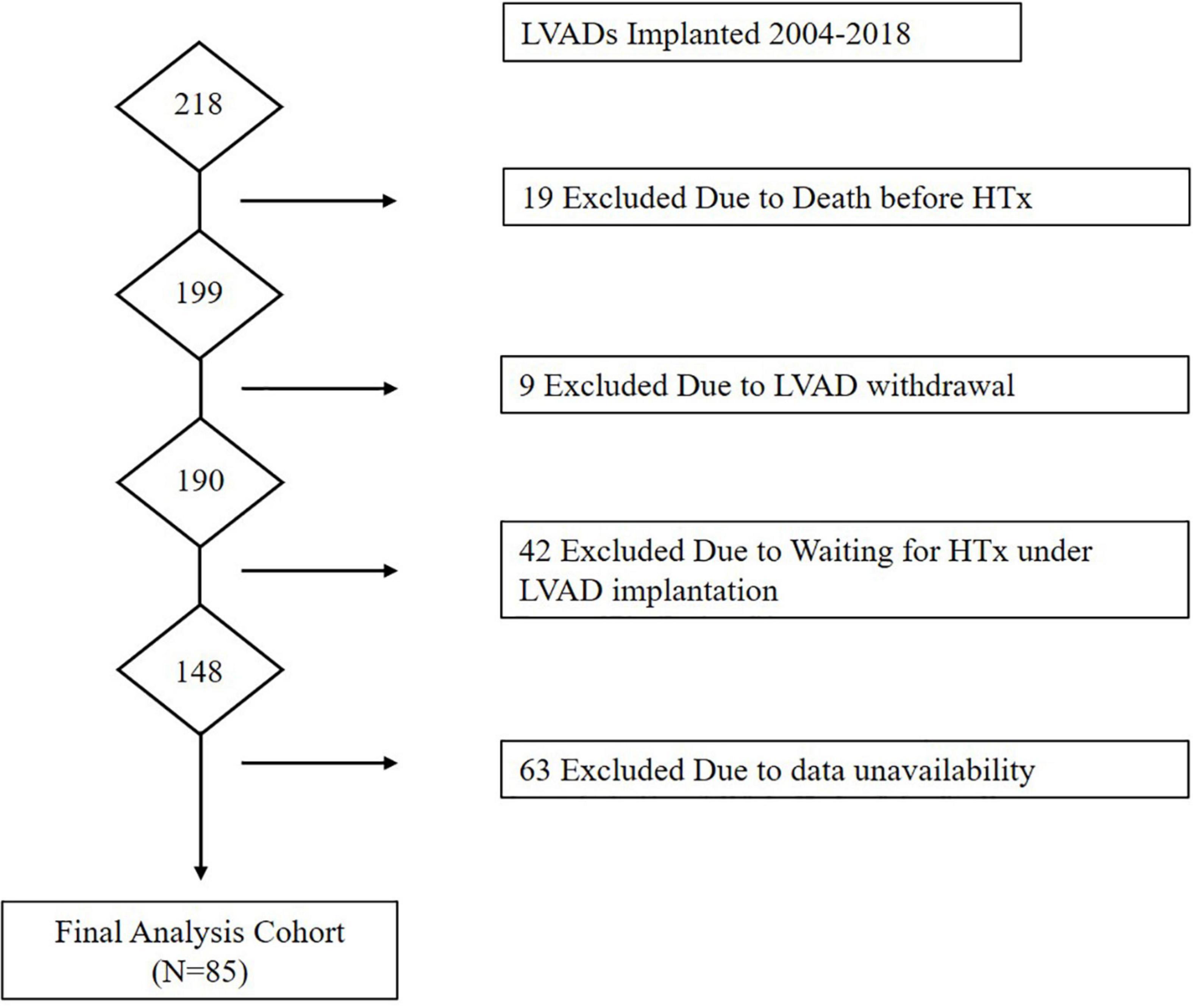
Figure 1. Flow of patients through the Study. HTx, heart transplantation; LVAD, left ventricular assist device.
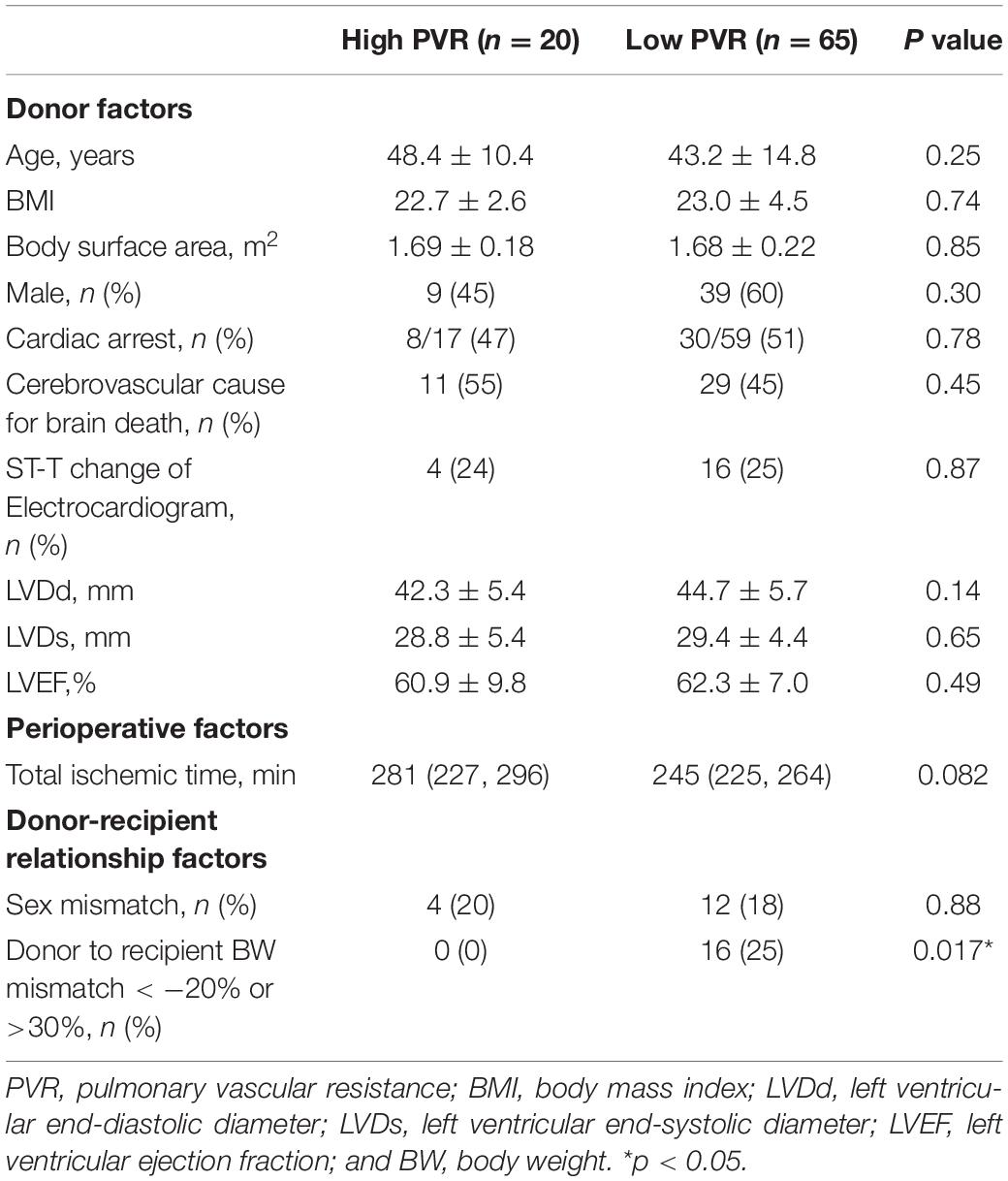
Table 2. Baseline demographic and clinical parameters of donors, clinical course after HTx and donor-recipient relationship factors in two groups.
Acute Cellular Rejection After Heart Transplantation
Table 3 shows the incidence of ACR within and after 1 year post-HTx in both groups. There was no significant difference in ACR between the two groups in either the acute or chronic phase.
Change of Clinical Parameters After Heart Transplantation
Figure 2 shows the changes in clinical parameters after HTx. After HTx, PVR improved in both groups [high PVR group, 3.48 ± 0.64 WU (baseline) vs. 1.54 ± 0.53 WU (24 weeks after HTx), p < 0.0001; low PVR group, 1.65 ± 0.59 WU (baseline) vs. 1.32 ± 0.61 WU (24 weeks after HTx), p = 0.0016]. The PVR levels in both groups became comparable 1 year after HTx; however, the difference between each group became evident 2 years after HTx (Figure 2A). In contrast, the DPG and TPG levels were comparable in all times between two groups (Supplementary Figure 1). The differences in mPAP, mRAP, and mPCWP also tended to increase from 1 year after HTx and were all significantly higher in the high PVR group at 3 years after HTx [mPAP, 22.7 ± 9.0 mm Hg (high PVR group) vs. 15.4 ± 4.3 mm Hg (low PVR group), p = 0.0009; mRAP, 7.2 ± 3.6 mm Hg (high PVR group) vs. 4.1 ± 2.1 mm Hg (low PVR group), p = 0.0042; and mPCWP, 13.4 ± 4.5 mm Hg (high PVR group) vs. 8.8 ± 3.3 mm Hg (low PVR group), p = 0.0040] (Figures 2B–D). In the high PVR group, PAPi tended to decrease gradually in the chronic phase after HTx, which became significantly lower than that in the low PVR group at 3 years after HTx [2.51 ± 1.00 (high PVR group) vs. 5.21 ± 3.23 (low PVR group), p = 0.0033] (Figure 2F). BNP level was significantly higher in the high PVR group at 3 years after HTx, whereas eGFR was comparable in all times between the two groups (Figures 3A,B). Moreover, there was no significant difference in LVEF, LVDd/Ds and E/e’ and right ventricular function parameters between the two groups (Figures 3C–F and Supplementary Figure 2). By contrast, there was no significant difference in the presence of significant tricuspid regurgitation 3 years after HTx.
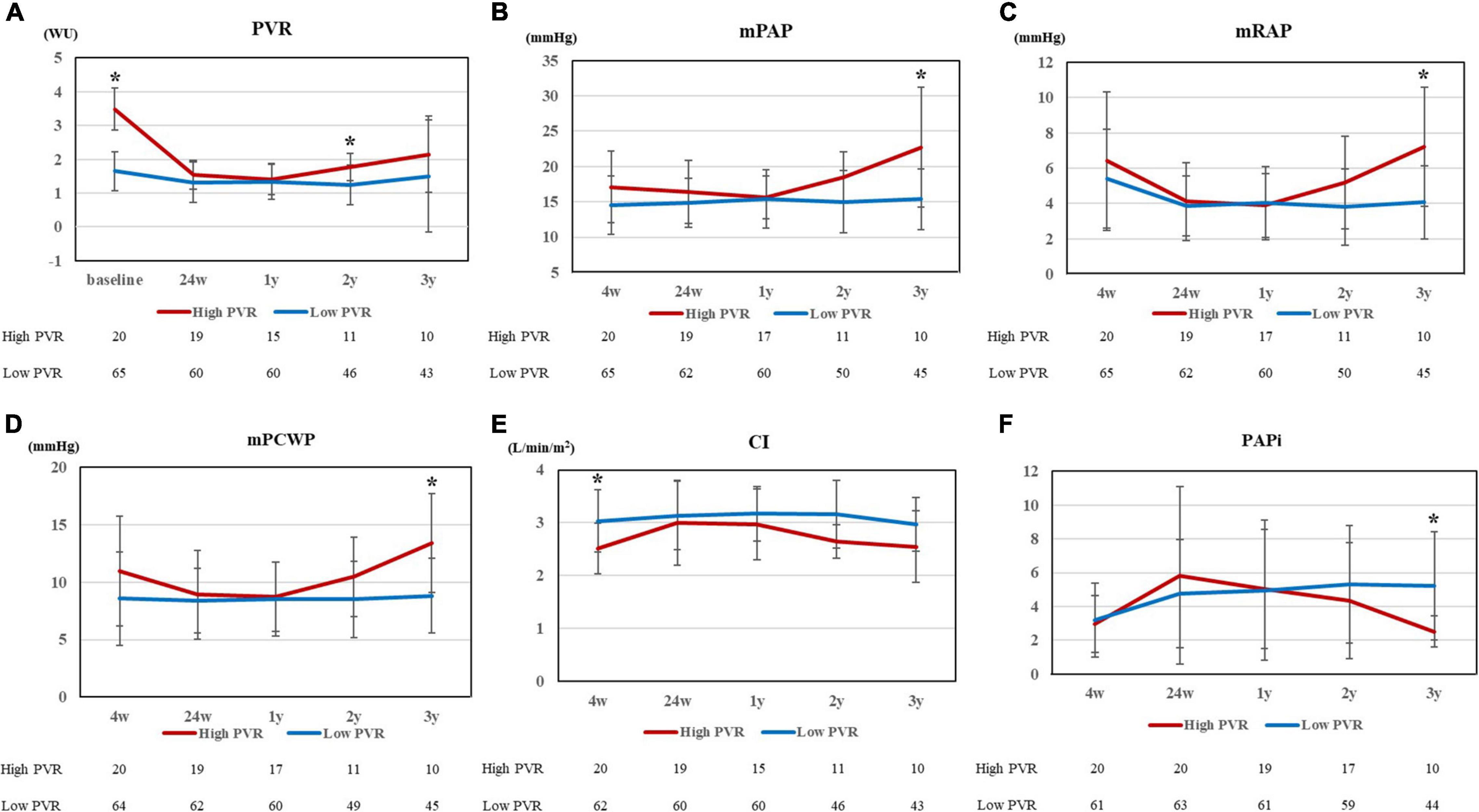
Figure 2. (A) PVR at 5 points (baseline, 24 weeks, 1 year, 2 years, and 3 years after HTx), (B) mPAP, (C) mRAP, (D) mPCWP, (E) CI, and (F) PAPi at 5 points (4 weeks, 24 weeks, 1 year, 2 years, and 3 years after HTx) for high and low PVR group. PVR, pulmonary vascular resistance; HTx, heart transplantation; mPAP, mean pulmonary artery pressure; mRAP, mean right arterial pressure; mPCWP, mean pulmonary capillary wedge pressure; CI, cardiac index; and PAPi, pulmonary artery pulsatility index. *p < 0.01.
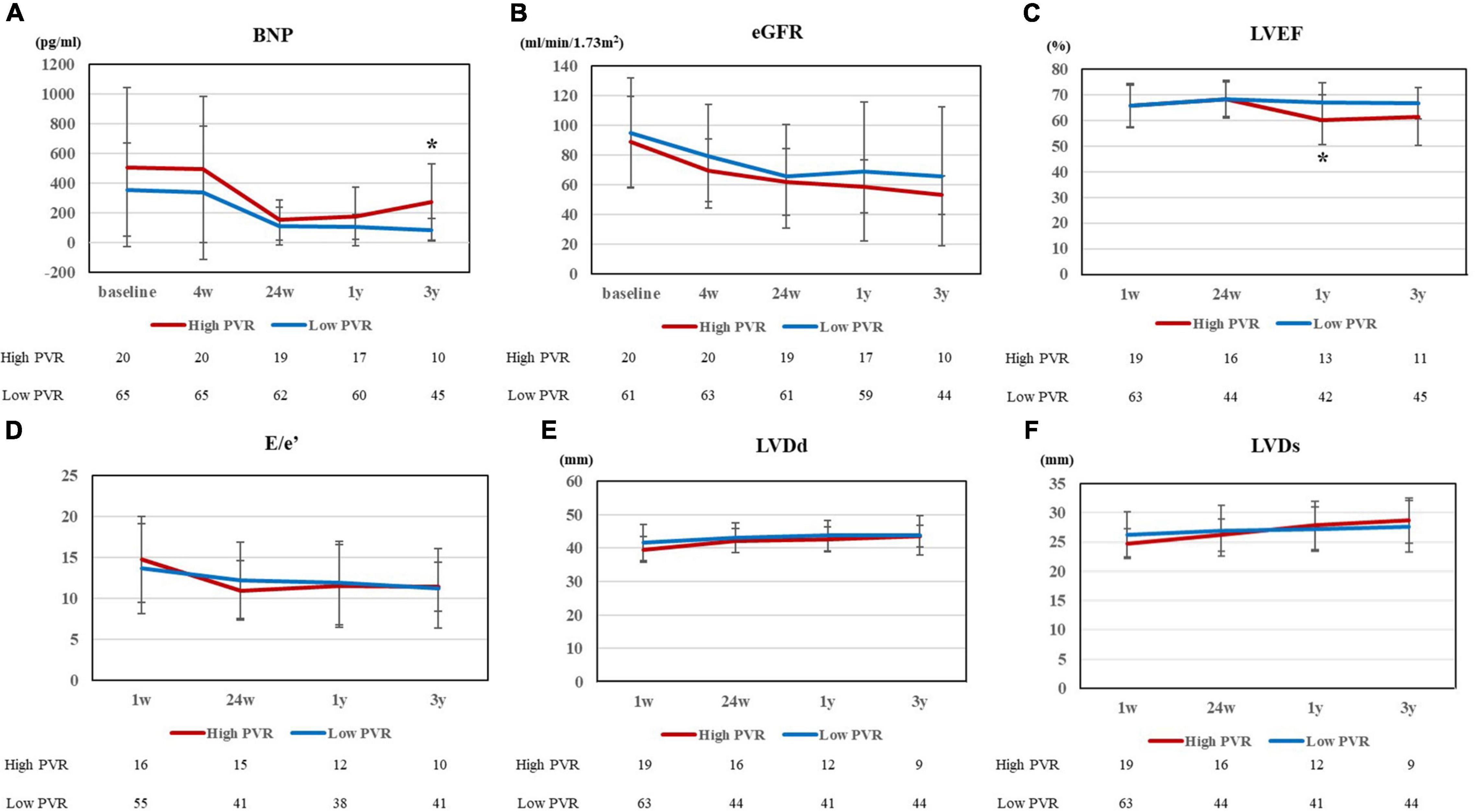
Figure 3. (A) BNP and (B) eGFR at 5 points (baseline, 4 weeks, 24 weeks, 1 year, and 3 years after HTx), (C) LVEF, (D) E/e’, (E) LVDd, and (F) LVDs at 4 points (1 week, 24 weeks, 1 year, and 3 years after HTx) for high and low PVR group. BNP, brain natriuretic peptide; eGFR, estimated glomerular filtration ratio; HTx, heart transplantation; LVEF, left ventricular ejection fraction; LVDd, left ventricular end diastolic dimension; LVDs, left ventricular end systolic dimension; and PVR, pulmonary vascular resistance. *p < 0.01.
Table 4 shows the oral medications that were used 3 years after HTx. The number of patients using loop diuretics was significantly higher in the high PVR group (p = 0.016). These results suggested that increased burden on both left and right ventricles in patients of the high PVR group. In contrast, there were no significant differences in immunosuppressants, beta-blockers, ACE inhibitors, or MRAs between the two groups.
Clinical Events After Heart Transplantation
In the Kaplan–Meier survival curve analysis, the presence of high PVR after LVAD implantation did not affect survival after HTx (p = 0.30, Figure 4A). During the follow-up period after HTx, one patient in the high PVR group was hospitalized for heart failure, while two patients in the low PVR group were hospitalized for heart failure after HTx (p = 0.54 Figure 4B). Subsequently, we examined the risk factors for the composite events of worsening heart failure, which included heart failure hospitalization, diuretic use for the treatment of congestion, and elevated intracardiac pressure. These were significantly more common in the high PVR group (p = 0.0017, Figure 4C). As shown in Table 5, residual PVR and donor age were important predictors of this combined event [(1) residual PVR, hazard ratio (HR) 4.9, 95% CI 1.6–14.7, p = 0.0058; (2) donor age, HR 6.8, 95% CI 2.2–21.0, p = 0.0008]. By performing multivariate analysis using both these factors and pre-HTx eGFR, residual PVR proved to be a significant predictor for this combined event (HR 6.5, 95% CI 2.0–21.6, p = 0.0023).
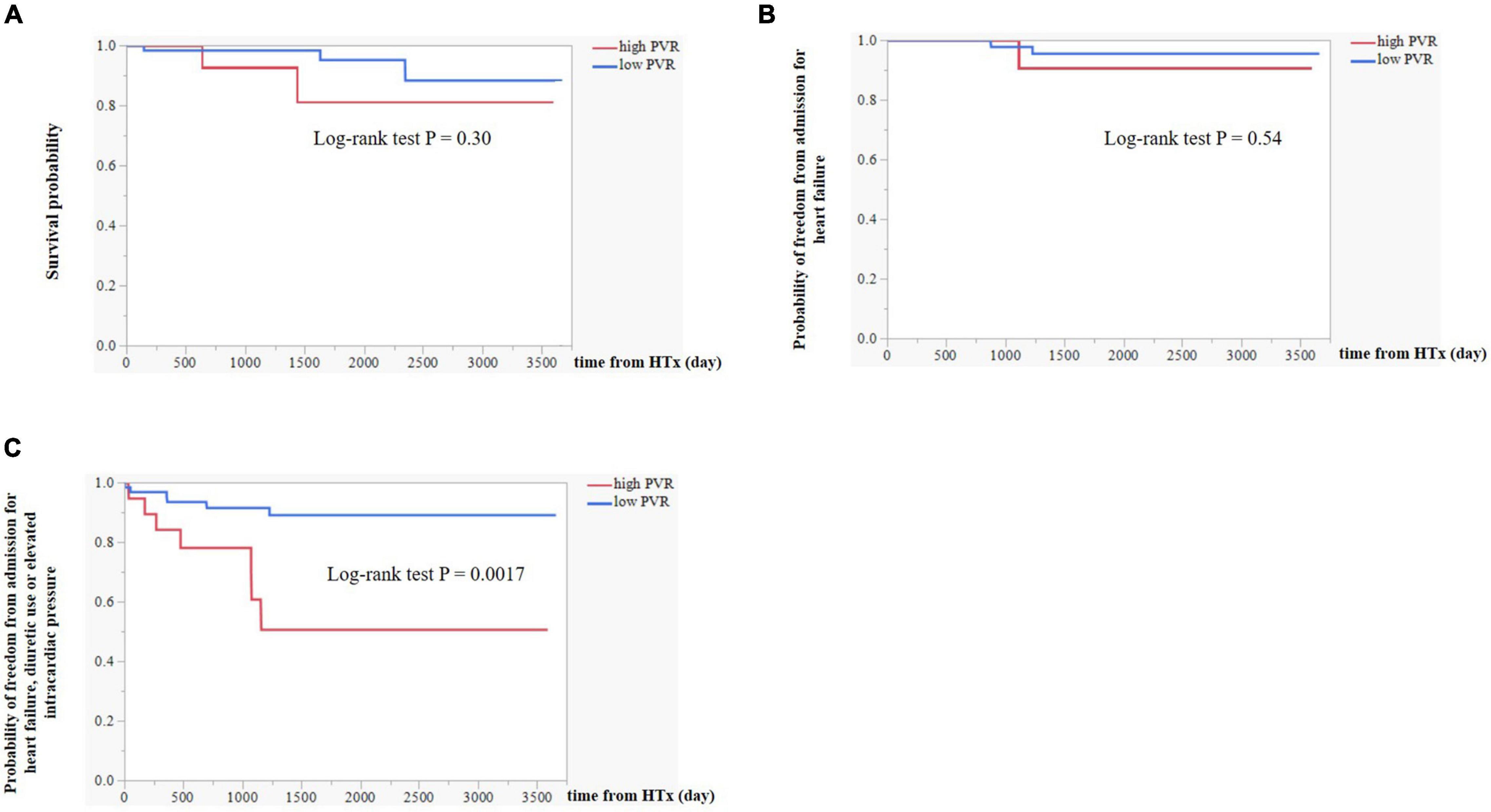
Figure 4. (A) Kaplan–Meier survival curve analysis between high PVR group and low PVR group. (B) Differences in event-free survival curves of admission for heart failure between high PVR group and low PVR group. (C) Differences in event-free survival curves of the composite event including admission for heart failure, diuretic use or elevated intracardiac pressure (mRAP ≥ 12 mm Hg or mPCWP ≥ 18 mm Hg) between high PVR group and low PVR group. PVR, pulmonary vascular resistance; mRAP, mean right atrial pressure; and mPCWP, mean pulmonary capillary wedge pressure.
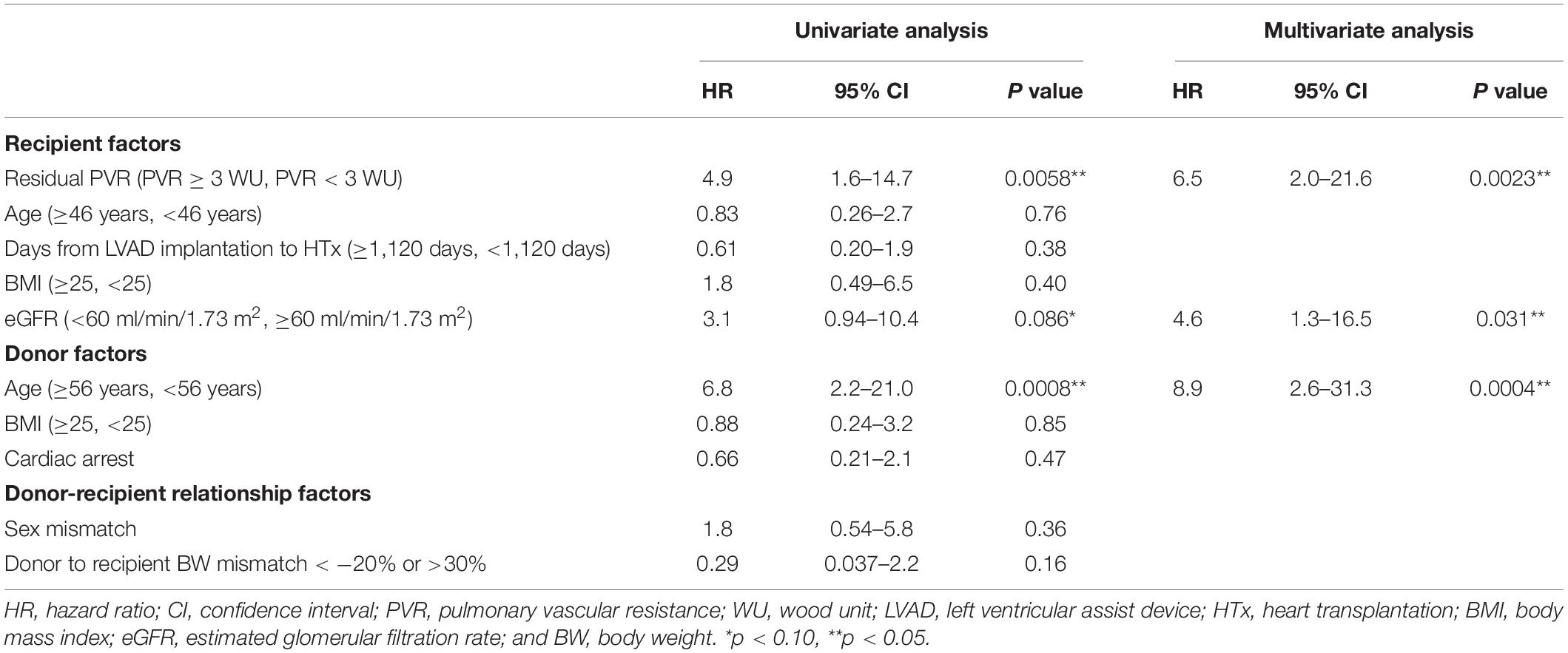
Table 5. Univariate and multivariate Cox proportional analysis of factors that determined the risk of the composite event of admission for heart failure, diuretic use or elevated intracardiac pressure (mRAP ≥ 12 mm Hg or mPCWP ≥ 18 mm Hg).
Discussion
In this study, we examined post-heart transplant hemodynamics and prognosis in patients stratified by the severity of PVR after LVAD implantation as BTT. The result was that residual high PVR under LVAD support corresponded to high right atrial→RAP and PCWP and low PAPi in the long term after HTx. As a result, patients with residual high PVR corresponded to high percentage of diuretic use. The novel point of this manuscript was a concise demonstration of the effect derived from residual high PVR during LVAD support on cardiovascular function after HTx, which had been not reported yet.
Residual Pulmonary Vascular Resistance Increase After Left Ventricular Assist Device Implantation
There were some reports that high PVR before HTx has a detrimental effect after HTx (17–19). Chang et al. demonstrated mild to moderate preoperative pulmonary hypertension is associated with increased risk of developing early post-transplant pulmonary hypertension, which corresponded to early mortality after HTx. Conversely, there are also conflicting reports that high PVR does not affect the prognosis after HTx. Vakil et al. reported pretransplant pulmonary hypertension did not affect survival after HTx using UNOS registry, which included approximately 10% of patients with MCS support before HTx (20). Lindelow et al. reported that high PVR decreased after HTx and it did not have an impact on the survival after HTx (21). There were other similar results that pretransplant high PVR did not affect the survival after HTx (22–25). For explaining these conflicting results, the timing and patient condition of evaluating PVR seems to be critical for each result. Indeed, Shah et al. examined posttransplant survival between patients with high and low PVR, which was measured before mechanical support for bridging to HTx (24), whereas Drakos et al. used the value of PVR just before heart transplant (26). The characteristics of this study was that all patients were supported by LVAD before HTx and PVR was measured during LVAD support. Generally, the burden of pulmonary vasculature from left-sided heart failure is alleviated by LVAD support (8), so that the high PVR measured during LVAD support (“residual high PVR”), which was investigated in the current study, might reflect the increase in pulmonary vascular tone due to pathological remodeling, which indicates irreversible change. Indeed, in this study, the effect derived from the high PVR under LVAD support had a significant impact on the hemodynamics and status in heart failure in the long term even more than 2 years after HTx. Bollano et al. (27) demonstrated that patients with pulmonary hypertension (mPAP ≥ 23 mm Hg) 1 year after HTx had a significantly higher rate of death or retransplantation during the 5-year follow-up after HTx (p = 0.009). These results demonstrated the remaining pulmonary hypertension after HTx had continuously negative impact on the long-term clinical course after HTx. In the discussion about pulmonary vascular tone, the difficulty in accurately measuring pulmonary vascular tone abnormalities is noted. Indeed, the abnormality could not be detected by the value of PVR temporally after HTx in this study. It might be due to low sensitivity of PVR for the detection of pathological pulmonary vascular tone in normal hemodynamics, which is also the case in DPG and TPG. The more useful marker for the detection of pulmonary vascular tone should be developed in further study, which might lead to more concise elucidation between pulmonary vasculature and cardiac function. Lundgren et al. demonstrated the deranged PVR response during exercise despite normal PVR at rest after HTx (28). It might be difficult to represent the abnormalities in pulmonary vasculature by the hemodynamics at rest condition. Moreover, the exercise load test had been reported to evaluate pulmonary vascular dysfunction more concisely in pulmonary hypertension (29). A study on pulmonary vascular dysfunction in patients after HTx using exercise load test is warranted.
Right Heart Dysfunction After Heart Transplantation
The result of this study revealed long-term right heart dysfunction after HTx might be derived from remaining high resistance of pulmonary artery before HTx. Multivariate analysis revealed that this factor affected late requirement of diuretics to the same extent with recipient renal function and donor factors, such as donor age. Generally, non-specific primary graft failure is considered the most important cause of right heart dysfunction early after HTx (12, 30). Primary graft failure is commonly precipitated by various pathways (31). Primary graft dysfunction become obvious in the left ventricle (19%), both ventricles (7%), and right ventricle (5%; 32). The determining factors for the development of primary graft failure were reported to be transplant operation associating factors, such as ischemic time. Conversely, there are some reports about the change in cardiac function of transplanted heart in the chronic phase (33). Goland et al. demonstrated the gradual impairment of both ventricles early after HTx, which was finely exemplified by tissue Doppler imaging on echocardiography (34). D’Andrea et al. demonstrated that the decrease in heart function in the right side was more obvious than that in the left side after HTx (35). This gradual decrease in right ventricle function might clarify the burden of pulmonary vasculature in this study. Compared with the low PVR group, PAPi, which is one parameter of right heart function, was significantly lower and RAP was significantly higher in the high PVR group at 3 years after HTx, suggesting that high PVR led to the burden of right ventricle. The consistent trend of high PVR in the high PVR group suggested sustained burden from pulmonary vasculature on the right ventricle led to the derangement of right heart, leading to graft dysfunction. Whal et al. demonstrated no difference in right ventricular function between patients with and without pretransplant pulmonary hypertension (36), which was different from our results. It might be due to different definitions of high PVR. Indeed, the definition of high PVR in the current study was measured under LVAD support, which corresponded to more severe pulmonary vascular dysfunction. In the current study, PCWP was also significantly higher in the high PVR group 3 years after HTx. There was no case of AMR, which is one of the factors that increase PCWP after HTx (37), in our study. In addition, the percentage of donor with high age (such as more than 60) was comparable in patients with high and low PVR groups, which suggested the difference in hemodynamics might not be derived from the characteristics of donor heart. A mediation analysis demonstrated the increase in PCWP 3 years after HTx was mainly derived from the increase in right sided pressures (Supplementary Figure 3) and it was suggested that the load of left side might be derived from right side burden. According to the impact of transplant vasculopathy, there was no significant difference in hemodynamic parameters in patients with and without obvious transplant vasculopathy (Supplementary Figure 4). However, we couldn’t perform concise evaluations about microvascular graft dysfunction and further studies investigating the association between right and left side burden of transplanted heart should be performed.
By Kaplan–Meier event-free survival curve, there was no significant difference in survival rate or admission due to heart failure after HTx between the high and low PVR groups. However, there were significantly more cases of loop diuretic use, and the BNP level was significantly increased in the high PVR group, both of which suggested the higher risk of heart failure in patients in the high PVR group after HTx. We analyzed the risk of worsening heart failure which was defined as heart failure hospitalization, diuretic use, and elevated intracardiac pressure. Indeed, the event of outpatient diuretic intensification as the sign of worsening heart failure in outpatient setting have been getting more attention (16, 38, 39), which was demonstrated to be comparable in the risk prognostication with heart failure hospitalization in patients with heart failure. However, the addition of diuretics and the increase in intracardiac pressure may be derived from renal dysfunction, and it is much difficult to distinguish the cause. Moreover, the significant decrease in PAPi corresponded to right ventricular dysfunction, which might critically limit exercise capacity (40). The increase in diuretic use or decrease in right ventricular function inevitably decrease the quality of life after HTx, which should be noted. However, there was no significant change in echocardiographic parameters in RV functions. We should investigate about the mechanistic insights including RV function of the findings in the future study.
Study Limitations
First, besides the inherent limitation of a retrospective study, we examined small cases in a single center. We should further increase the study patients including different patient cohorts to verify the finding in this study. Second, patient selection bias can be mentioned. In this study, patients undergoing right heart catheterization after LVAD implantation were divided into two groups. We excluded cases in which different right heart catheterization protocol was adopted because of LVAD implantation in other institutions. Other severe cases, such as early death after cardiac transplantation and death during the perioperative period of the LVAD, were excluded. Moreover, 19 patients died during LVAD placement, of which 3 had high PVR and 14 had low PVR and 2 had missing data.
Third, the types of LVAD were not unified; extracorporeal LVAD, LVAD with implantable axial flow pumps, and LVAD with implantable centrifugal pumps were used. Baseline PVR could be affected by the different hemodynamics of various LVAD. Moreover, PVR after LVAD implantation was somewhat dependent on the characteristics derived from LVAD support, which might be changed in different mechanical supports, such as total artificial heart (23). In addition, the value of PVR might be affected by the addition of pulmonary vasodilatory agents such as phosphodiesterase 5 (PDE5) inhibitors. However, there was no significant difference in PDE5 inhibitors between two groups. Fourth, we checked PVR at one time point “1 month after LVAD implantation” during LVAD support so that PVR at the timing of HTx might be slightly different from that measured. Indeed, Gulati et al. demonstrated the change in PVR after LVAD implantation (41). They showed the highest percentage of high PVR (defined as PVR > 3 WU) in patients under LVAD 1 month after LVAD implantation (35%), which was gradually decreased later, such as 25% at 3 months, 20% at 6 months, and 15% at 24 months after LVAD implantation. The value of PAP and PVR continuously fluctuates during all the time after the LVAD implantation. Therefore, the appropriate timing for measurement of PVR after LVAD implantation is extremely difficult to decide. However, in this study, the exact value of PVR was not comparatively important but the identification of patients with residual high PVR was critical for the analysis. Therefore, the timing of PVR under LVAD support seems to be a somewhat low priority issue. In addition, there was no significant difference in the duration from LVAD implantation to HTx between groups. Fifth, we used composite endpoint of worsening heart failure, which might be subject to bias. However, the percentage of loop medication and the hemodynamic data supports the validity of the result of composite endpoint.
Conclusion
Our study demonstrates that patients with residual high PVR under LVAD showed the increase of right and left atrial pressure in the chronic phase after HTx. The residual high PVR under LVAD implantation corresponded to the increase of diuretic use in the chronic phase after HTx.
Data Availability Statement
The raw data supporting the conclusions of this article will be made available by the authors, without undue reservation.
Ethics Statement
The studies involving human participants were reviewed and approved by the Institutional Review Board of the University of Tokyo Graduate School (assignment number: 2650). Written informed consent for participation was not required for this study in accordance with the national legislation and the institutional requirements.
Author Contributions
NK: conceptualization, methodology, data curation, validation, and writing – original draft, review, and editing. EA: conceptualization, methodology, validation, data curation, and writing – review and editing. MH, MA, SS, OK, MO, and IK: supervision. MT, CB, JI, HY, AS, KN, YI, and KF: data curation. All authors contributed to the article and approved the submitted version.
Funding
This work was supported by the Ministry of Education, Culture, Sports, Science and Technology of Japan through Grant-in-Aid 21K08047 (to EA).
Conflict of Interest
EA belongs to the Department, endowed by NIPRO-Corp, Terumo-Corp., Senko-Medical-Instrument-Mfg., Century-Medical, Inc., ONO-pharmaceutical-Co., Ltd. Medtronic-JAPAN Co., Ltd, Nippon-Shinyaku Co., Ltd, Mochida Pharmaceutical Co.; Boehringer Ingelheim Pharmaceuticals Inc., Abiomed-Inc, AQuA-Inc, Fukuda-Denshi Co., Ltd, and Sun-Medical-Technology-Research Corp.
The remaining authors declare that the research was conducted in the absence of any commercial or financial relationships that could be construed as a potential conflict of interest.
Publisher’s Note
All claims expressed in this article are solely those of the authors and do not necessarily represent those of their affiliated organizations, or those of the publisher, the editors and the reviewers. Any product that may be evaluated in this article, or claim that may be made by its manufacturer, is not guaranteed or endorsed by the publisher.
Supplementary Material
The Supplementary Material for this article can be found online at: https://www.frontiersin.org/articles/10.3389/fcvm.2022.904350/full#supplementary-material
References
1. Goldstein BA, Thomas L, Zaroff JG, Nguyen J, Menza R, Khush KK. Assessment of heart transplant waitlist time and pre- and post-transplant failure: a mixed methods approach. Epidemiology. (2016) 27:469–76. doi: 10.1097/EDE.0000000000000472
2. Gummert JF. Heart transplantation in bad oeynhausen, germany: the heart transplant program at the heart and diabetes center bad Oeynhausen, University Hospital, Ruhr - University Bochum University, Germany. Eur Heart J. (2017) 38:3411–3. doi: 10.1093/eurheartj/ehx698
3. John R, Pagani FD, Naka Y, Boyle A, Conte JV, Russell SD, et al. Post-cardiac transplant survival after support with a continuous-flow left ventricular assist device: impact of duration of left ventricular assist device support and other variables. J Thorac Cardiovasc Surg. (2010) 140:174–81. doi: 10.1016/j.jtcvs.2010.03.037
4. Kjaergaard J, Akkan D, Iversen KK, Kjoller E, Køber L, Torp-Pedersen C, et al. Prognostic importance of pulmonary hypertension in patients with heart failure. Am J Cardiol. (2007) 99:1146–50. doi: 10.1016/j.amjcard.2006.11.052
5. Rosenkranz JS, Gibbs SR, Wachter R, De Marco T, Vonk-Noordegraaf A, Vachiery J-L. Left ventricular heart failure and pulmonary hypertension. Eur Heart J. (2016) 37:942–54. doi: 10.1093/eurheartj/ehv512
6. Fernández AI, Yotti R, González-Mansilla A, Mombiela T, Gutiérrez-Ibanes E, Perez del Villar C, et al. The biological bases of Group2 pulmonary hypertension. Int J Mol Sci. (2019) 23:5884.
7. John R, Liao K, Kamdar F, Eckman P, Boyle A, Colvin-Adams M. Effects on pre-and posttransplant pulmonary hemodynamics in patients with continuous-flow left ventricular assist devices. J Thorac Cardiovasc Surg. (2010) 140:447–52. doi: 10.1016/j.jtcvs.2010.03.006
8. Mikus E, Stepanenko A, Krabatsch T, Loforte A, Dandel M, Lehmkuhl HB, et al. Reversibility of fixed pulmonary hypertension in left ventricular assist device support recipients. Eur J Cardiothorac Surg. (2011) 40:971–7. doi: 10.1016/j.ejcts.2011.01.019
9. Torre-Amione G, Southard RE, Loebe MM, Youker KA, Bruckner B, Estep JD, et al. Reversal of secondary pulmonary hypertension by axial and pulsatile mechanical circulatory support. J Heart Lung Transplant. (2010) 29:195–200. doi: 10.1016/j.healun.2009.05.030
10. Selim AM, Wadhwani L, Burdorf A, Raichlin E, Lowes B, Zolty R. Left ventricular assist devices in pulmonary hypertension group 2 with significantly elevated pulmonary vascular resistance: a bridge to cure. Heart Lung Circ. (2019) 28:946–52. doi: 10.1016/j.hlc.2018.04.299
11. Stewart S, Winters GL, Fishbein MC, Tazelaar HD, Kobashigawa J, Abrams J, et al. Revision of the 1990 working formulation for the standardization of nomenclature in the diagnosis of heart rejection. J Heart Lung Transplant. (2005) 24:1710–20.
12. Costanzo MR, Dipchand A, Starling R, Anderson A, Chan M, Desai S, et al. International society of heart and lung transplantation guidelines. The international society of heart and lung transplantation guidelines for the care of heart transplant recipients. J Heart Lung Transplant. (2010) 29:914–56.
13. Lampert BC, Teuteberg JJ. Right ventricular failure after left ventricular assist devices. J Heart Lung Transplant. (2015) 3:1123–30.
14. Ayesta A, Urrútia G, Madrid E, Vernooij RWM, Vicent L, Martínez-Sellés M. Sex-mismatch influence on survival after heart transplantation: a systematic review and meta-analysis of observational studies. Clin Transplant. (2019) 33:e13737. doi: 10.1111/ctr.13737
15. Hoeper MM, Bogaard HJ, Condliffe R, Frantz R, Khanna D, Kurzyna M, et al. Definitions and diagnosis of pulmonary hypertension. J Am Coll Cardiol. (2013) 62:42–50.
16. Madelaire C, Gustafsson F, Stevenson LW, Kristensen SL, Køber L, Andersen J, et al. One-year mortality after intensification of outpatient diuretic therapy. J Am Heart Assoc. (2020) 9:e016010. doi: 10.1161/JAHA.119.016010
17. Chang PP, Longenecker JC, Wang NY, Baughman KL, Conte JV, Hare JM, et al. Mild vs severe pulmonary hypertension before heart transplantation: different effects on post transplantation pulmonary hypertension and mortality. J Heart Lung Transplant. (2005) 24:998–1007. doi: 10.1016/j.healun.2004.07.013
18. Chen JM, Levin HR, Michler RE, Prusmack CJ, Rose EA, Aaronson KD. Reevaluating the significance of pulmonary hypertension before cardiac transplantation: determination of optimal thresholds and quantification of the effect of reversibility on perioperative mortality. J Thorac Cardiovasc Surg. (1997) 114:627–34. doi: 10.1016/S0022-5223(97)70053-9
19. Crawford TC, Leary PJ, Fraser CD III, Suarez-Pierre A, Magruder JT, Baumgartner WA, et al. Impact of the new pulmonary hypertension definition on heart transplant outcomes: expanding the hemodynamic risk profile. Chest. (2020) 157:151–61. doi: 10.1016/j.chest.2019.07.028
20. Vakil K, Duval S, Sharma A, Adabag S, Abidi KS, Taimeh Z, et al. Impact of pre-transplant pulmonary hypertension on survival after heart transplantation: a UNOS registry analysis. Int J Cardiol. (2014) 176:595–9. doi: 10.1016/j.ijcard.2014.08.072
21. Lindelöw B, Andersson B, Waagstein F, Bergh CH. High and low pulmonary vascular resistance in heart transplant candidates. A 5-year follow-up after heart transplantation shows continuous reduction in resistance and no difference in complication rate. Eur Heart J. (1999) 20:148–56. doi: 10.1053/euhj.1998.1155
22. Klotz S, Wenzelburger F, Stypmann J, Welp H, Drees G, Schmid C, et al. Reversible pulmonary hypertension in heart transplant candidates: to transplant or not to transplant. Ann Thorac Surg. (2006) 82:1770–3.
23. Goland S, Czer LS, Kass RM, De Robertis MA, Mirocha J, Coleman B, et al. Pre-existing pulmonary hypertension in patients with end-stage heart failure: impact on clinical outcome and hemodynamic follow-up after orthotopic heart transplantation. J Heart Lung Transplant. (2007) 26:312–8. doi: 10.1016/j.healun.2006.12.012
24. Shah R, Patel DB, Mankad AK, Rennyson SL, Tang DG, Quader MA, et al. Pulmonary hypertension after heart transplantation in patients bridged with the total artificial heart. ASAIO J. (2016) 62:69–73. doi: 10.1097/MAT.0000000000000298
25. Ando M, Takayama H, Kurlansky PA, Han J, Garan AR, Topkara VK, et al. Effect of pulmonary hypertension on transplant outcomes in patients with ventricular assist devices. Ann Thorac Surg. (2020) 110:158–64. doi: 10.1016/j.athoracsur.2019.09.095
26. Drakos SG, Kfoury AG, Gilbert EM, Horne BD, Long JW, Stringham JC, et al. Effect of reversible pulmonary hypertension on outcomes after heart transplantation. J Heart Lung Transplant. (2007) 26:319–23. doi: 10.1016/j.healun.2007.01.012
27. Bollano E, Andersson B, Hjalmarsson C, Dellgren G, Daka B, Karason K. Early post-transplant elevated pulmonary artery pressure predicts adverse outcome in cardiac recipients. Int J Cardiol Heart Vasc. (2020) 26:100438. doi: 10.1016/j.ijcha.2019.100438
28. Lundgren J, Rådegran G. Hemodynamic characteristics including pulmonary hypertension at rest and during exercise before and after heart transplantation. J Am Heart Assoc. (2015) 4:e001787. doi: 10.1161/JAHA.115.001787
29. Guth S, Wiedenroth CB, Rieth A, Richter MJ, Gruenig E, Ghofrani HA, et al. Exercise right heart catheterisation before and after pulmonary endarterectomy in patients with chronic thromboembolic disease. Eur Respir J. (2018) 52:30139773. doi: 10.1183/13993003.00458-2018
30. Cosío Carmena MD, Gómez Bueno M, Almenar L, Delgado JF, Arizón JM, González Vilchez F, et al. Primary graft failure after heart transplantation: characteristics in a contemporary cohort and performance of the RADIAL risk score. J Heart Lung Transplant. (2013) 32:1187–95. doi: 10.1016/j.healun.2013.08.004
31. Chen JM, Levin HR, Rose EA, Addonizio LJ, Landry DW, Sistino JJ, et al. Experience with right ventricular assist devices for perioperative right-sided circulatory failure. Ann Thorac Surg. (1996) 61:305–10. doi: 10.1016/0003-4975(95)01010-6
32. Nicoara A, Ruffin D, Cooter M, Patel CB, Thompson A, Schroder JN, et al. Primary graft dysfunction after heart transplantation: incidence, trends, and associated risk factors. Am J Transplant. (2018) 18:1461–70. doi: 10.1111/ajt.14588
33. Marfella R, Amarelli C, Cacciatore F, Balestrieri ML, Mansueto G, D’Onofrio N, et al. Lipid accumulation in hearts transplanted from nondiabetic donors to diabetic recipients. J Am Coll Cardiol. (2020) 75:1249–62. doi: 10.1016/j.jacc.2020.01.018
34. Goland S, Siegel RJ, Burton K, De Robertis MA, Rafique A, Schwarz E, et al. Changes in left and right ventricular function of donor hearts during the first year after heart transplantation. Heart. (2011) 97:1681–6. doi: 10.1136/hrt.2010.220871
35. D’Andrea A, Riegler L, Nunziata L, Scarafile R, Gravino R, Salerno G, et al. Right heart morphology and function in heart transplantation recipients. J Cardiovasc Med. (2013) 14:648–58. doi: 10.2459/JCM.0b013e32835ec634
36. Wahl A, Feller M, Wigger E, Tanner H, Stoupis C, Carrel T, et al. Pretransplant pulmonary hypertension and long-term allograft right ventricular function. Eur J Cardiothorac Surg. (2010) 37:61–7. doi: 10.1016/j.ejcts.2009.05.021
37. Michaels PJ, Espejo ML, Kobashigawa J, Alejos JC, Burch C, Takemoto S, et al. Humoral rejection in cardiac transplantation: risk factors, hemodynamic consequences and relationship to transplant coronary artery disease. J Heart Lung Transplant. (2003) 22:58–69. doi: 10.1016/s1053-2498(02)00472-2
38. Khan MS, Butler J, Greene SJ. Recognizing the significance of outpatient worsening heart failure. J Am Heart Assoc. (2020) 9:e017485. doi: 10.1161/JAHA.120.017485
39. Ferreira JP, Liu J, Claggett BL, Vardeny O, Pitt B, Pfeffer MA, et al. Outpatient diuretic intensification as endpoint in heart failure with preserved ejection fraction trials: an analysis from TOPCAT. Eur J Heart Fail. (2021):2376. doi: 10.1002/ejhf.2376
40. Verset L, Lavie-Badie Y, Guitard J, Delmas C, Biendel-Piquet C, Cazalbou S, et al. Impact of right ventricular systolic function after heart transplantation on exercise capacity. Echocardiography. (2020) 37:706–14. doi: 10.1111/echo.14650
Keywords: heart transplantation, pulmonary vascular resistance, left ventricular assist device, heart failure, rejection
Citation: Kakuda N, Amiya E, Hatano M, Tsuji M, Bujo C, Ishida J, Yagi H, Saito A, Narita K, Isotani Y, Fujita K, Ando M, Shimada S, Kinoshita O, Ono M and Komuro I (2022) Residual Pulmonary Vascular Resistance Increase Under Left Ventricular Assist Device Support Predicts Long-Term Cardiac Function After Heart Transplantation. Front. Cardiovasc. Med. 9:904350. doi: 10.3389/fcvm.2022.904350
Received: 25 March 2022; Accepted: 16 May 2022;
Published: 01 June 2022.
Edited by:
Kiyotake Ishikawa, Icahn School of Medicine at Mount Sinai, United StatesReviewed by:
Tomasz Zieliński, National Institute of Cardiology, PolandJoel David Schilling, Washington University in St. Louis, United States
Copyright © 2022 Kakuda, Amiya, Hatano, Tsuji, Bujo, Ishida, Yagi, Saito, Narita, Isotani, Fujita, Ando, Shimada, Kinoshita, Ono and Komuro. This is an open-access article distributed under the terms of the Creative Commons Attribution License (CC BY). The use, distribution or reproduction in other forums is permitted, provided the original author(s) and the copyright owner(s) are credited and that the original publication in this journal is cited, in accordance with accepted academic practice. No use, distribution or reproduction is permitted which does not comply with these terms.
*Correspondence: Eisuke Amiya, YW1peWFlLXRreUB1bWluLmFjLmpw