- 1Department of Endocrinology, Beijing Chao-Yang Hospital, Capital Medical University, Beijing, China
- 2Medical Examination Center, Beijing Chao-Yang Hospital, Capital Medical University, Beijing, China
Background: Homocysteine (Hcy) is an independent risk factor for cardiovascular disease, while mechanisms are unclear. Despite inconsistent and limited, epidemiological and experimental studies indicated that hyperhomocysteinemia (HHcy) affected lipid metabolism. This study aims to investigate the association of plasma Hcy with traditional lipid profiles and remnant cholesterol (RC) in Chinese adults.
Methods: In total, 7,898 subjects aged 20–79 years who underwent a physical examination at Beijing Chao-Yang Hospital in Beijing were included in this study. Fasting plasma total cholesterol (TC), triglyceride (TG), high-density lipoprotein cholesterol (HDL-C), low-density lipoprotein cholesterol (LDL-C), apolipoprotein A1 (ApoA1), apolipoprotein B (ApoB), lipoprotein (a) [Lp(a)], Hcy, and other metabolic risk factors were measured by routine automated laboratory methods. RC was calculated as TC minus HDL-C and LDL-C. The linear regression model and logistic regression model were used to assess the relationship between Hcy and lipids after adjusting potential confounders.
Results: Of the subjects, the median level of plasma Hcy was 13.0 μmol/L and 32.3% had HHcy. Plasma Hcy was negatively associated with HDL-C, ApoA1, and Lp(a) and positively associated with TG levels after adjusting age, sex, body mass index, blood pressure, alanine transaminase, aspartate transaminase, creatinine, uric acid, and glucose. HHcy significantly increased the risk of low HDL-C [odds ratio (OR) 1.26; 95%CI (1.11–1.44); p < 0.001]. The net mediation effects of ApoA1 on the relationship between Hcy and HDL-C before and after adjusting confounders were 46.9 and 30.6%, respectively. More interestingly, the RC level was significantly elevated in subjects with HHcy after adjusting other influencing factors (p = 0.025). Hcy presented a positive correlation with RC levels after adjusting the above confounding factors (β = 0.073, p = 0.004), and the correlation was still significant even after controlling other lipids, including TG, LDL-C, HDL-C, ApoA1, ApoB, and Lp(a).
Conclusion: Our study showed that plasma Hcy was not only significantly associated with conventional atherogenic lipids but also independently correlated with RC levels beyond other lipids after controlling potential confounders. This finding proposes that identifying Hcy-related dyslipidemia risk, both traditional lipids and RC residual risk, is clinically relevant as we usher in a new era of targeting Hcy-lowering therapies to fight against dyslipidemia or even cardiovascular disease.
Introduction
Atherosclerotic cardiovascular disease (ASCVD) is a leading cause of death worldwide, thus early prevention and treatment are extremely important. In addition to obesity, hyperglycemia, hypertension, and dyslipidemia, it has been confirmed that homocysteine (Hcy) is an emerging and independent risk factor for cardiovascular diseases, including ischemic heart disease, stroke, and peripheral vascular disease (1, 2). The meta-analysis suggested that patients with heart failure and ischemic stroke had significantly elevated plasma Hcy levels compared with normal controls (3, 4). It was estimated by Boushey et al. that approximately 10% of coronary artery disease might be attributed to hyperhomocysteinemia (HHcy) (5). Inversely, B vitamins supplementation, a therapy to lower plasma Hcy levels, appeared to decrease cardiovascular events in subjects with normal renal function (6).
The mechanism linking HHcy to the risk of ASCVD is still unclear. Hcy is a type of thiol-containing amino acid and is one of the critical intermediates of the methionine cycle and cysteine metabolism (7). Previous epidemiological studies and animal experiments implicated that inflammation reaction, oxidative stress, endothelial dysfunction, endoplasmic reticulum (ER) stress, and epigenetic control of gene expression were all potential mediators of HHcy-induced ASCVD (8–10). Additionally, it was also shown that HHcy increased the biosynthesis and secretion of cholesterol and triglyceride (TG) and suppressed the protein synthesis of apolipoprotein A1 (ApoA1), which is the main apolipoprotein of high-density lipoprotein cholesterol (HDL-C), and thus decreased HDL-C levels in animal and cellular experiments (11, 12). Moreover, Julve et al. demonstrated that the antioxidant function of HDL-C was impaired in HHcy mice induced by methionine (13). Nevertheless, the link between HHcy and lipid profiles in clinical and epidemiological studies was controversial. Some studies showed that Hcy had a positive correlation with low-density lipoprotein cholesterol (LDL-C), TG, and cholesterol and a negative correlation with HDL-C (7, 14), while others suggested that HHcy was not associated with atherogenic dyslipidemia after adjusting potential confounders (15), which might be attributable to the differences in demographic characteristics, including age, sex, race, and geographic regions, food intake, physical activity, and confounding factors included in data analyses among the studies.
With the widely used LDL-C-lowing therapy via statin, residual cardiovascular risk got more attention even among subjects with low LDL-C levels (16). Other than the conventional plasma lipid profile, triglyceride-rich lipoproteins (TGRL) or remnant cholesterol (RC) was also shown to be associated with the development of ASCVD (17, 18). Castañer et al. reported that RC, not LDL-C, was related with cardiovascular outcomes in overweight or obese individuals (19). Mendelian randomized studies suggested a causal relationship between the cholesterol content rather than TG of remnant particles and ischemic heart disease (20–22). Another study showed that RC-induced ASCVD was proportional to apolipoprotein B (ApoB) concentrations (23). A recently published primary prevention study demonstrated that elevated RC levels can predict ASCVD independent of traditional risk factors, including LDL-C and ApoB levels (24). Therefore, the specific content of RC increasing the ASCVD risk was unclear. Moreover, evidence linking Hcy, RC, and ASCVD was limited. Only one epidemiological study explored the relationship between HHcy and RC in US adults and did not support the association after controlling potential confounders (15).
In this study, in addition to investigating whether plasma Hcy is independently associated with conventional lipid profiles after adjusting confounders, we also focused on the relationship between Hcy and RC levels and determined whether the relationship exists beyond other lipids. We sought to add Chinese evidence for a better understanding of the link between Hcy and lipids and provide insight into the prevention and treatment of dyslipidemia and associated ASCVD in individuals with HHcy.
Materials and Methods
Study Population
This is a single-center cross-sectional study in Beijing, North China. We collected data from the routine health check-up of subjects aged 20–79 years at the Medical Examination Center of Beijing Chao-Yang Hospital from April 2016 to August 2021. All participants underwent anthropometric, plasma Hcy, and biochemistry parameter measurements, and medical histories were also recorded. Individuals with the following conditions were excluded: pregnant or breastfeeding women; severe hepatic dysfunction with alanine transaminase (ALT) or aspartate transaminase (AST) exceeding three times the upper limit of the normal range; severe renal dysfunction, which was defined as creatinine (Cr) exceeding two times the upper limit of the normal range; using antihypertensive, glucose-lowering, and lipid-lowering medications; and with missing covariates. Finally, 7,898 participants were included in the data analyses. This study was approved by the Ethics Committee of the Beijing Chao-Yang Hospital affiliated with Capital Medical University. Written informed consent was obtained from each subject.
Clinical Parameter Measurements
All subjects underwent anthropometric measurements, including age, sex, height, body weight, and blood pressure, by certified doctors. Standing height and body weight were assessed by a wall-mounted stadiometer to the nearest 0.1 cm and 0.1 kg. Systolic blood pressure (SBP) and diastolic blood pressure (DBP) were measured three times consecutively after a 5 min rest using a standard sphygmomanometer. The average values of the three recorded blood pressure were used for data analyses. Body mass index (BMI) was calculated as body weight divided by height squared (kg/m2).
After at least 10 h of overnight fasting, peripheral venous samples were collected for laboratory tests. Fasting blood glucose (FBG), total cholesterol (TC), TG, HDL-C, ApoA1, ApoB, lipoprotein (a) [Lp(a)], ALT, AST, Cr, urea, uric acid (UA), and Hcy were measured by routine automated laboratory methods [(Hitachi 7060C automatic biochemistry analysis system) in Beijing Chao-Yang Hospital. Hemoglobin A1c (HbA1c) was assessed by high-performance liquid chromatography. LDL-C was calculated by the Friedewald formula. Non-HDL-C was calculated as TC minus HDL-C, and RC was defined as non-HDL-C minus LDL-C (22). The estimated glomerular filtration rate (eGFR) was calculated by the Cockcroft-Gaulu equation: eGFR = [140-age (years)] × body weight (kg) × 1.23/Cr (μmol/L) (× 0.85 if female). The powerful surrogate marker for fatty liver disease, Zhejiang University (ZJU) index, was calculated by BMI (kg/m2) + FBG (mmol/L)+ TG (mmol/L)+ 3 × ALT (U/L)/AST (U/L) (+2 if female) (25). The fibrosis-4 (FIB4) index was defined as: age (years) × AST (U/L)/(platelet count (109/L) × √ ALT (U/L)] (26).
Definitions
For this study, the definition was in accordance with the international standard: lean (BMI < 25 kg/m2), overweight (BMI ≥ 25 kg/m2), obesity (BMI ≥ 30 kg/m2) (27), hypertension (SBP ≥ 140 mmHg or DBP ≥ 90 mmHg) (28), hyperglycemia (FBG ≥ 5.6 mmol/L) (29). The atherogenic lipid profiles were in accordance with the criteria of China Adult Dyslipidemia Prevention Guide (2007): hypercholesterolemia (TC ≥ 5.18 mmol/L), hypertriglyceridemia (TG ≥ 1.7 mmol/L), low HDL-C (HDL-C < 1.04 mmol/L), and high LDL-C (LDL-C ≥ 3.37 mmol/L). Consistent with published studies, Hcy ≥ 15 μmol/L was defined as HHcy (7, 30).
Statistical Analysis
The normality of the data was assessed using the Kolmogorov–Smirnov test. Normally distributed continuous parameters were expressed as mean ± standard deviation (SD) and were compared using Student’s t-tests. Non-normally distributed continuous indices, such as Hcy, ALT, AST, TG, RC, Lp(a), glucose, and HbA1c, were expressed as median and interquartile range [median (IQR25–75)] or median ± 95%CI and were compared using Student’s t-tests after natural log-transformed. A general linear model was used to compare data between groups after adjusting for potential confounding factors. Categorical variables were compared using χ2 tests. When performing correlation and regression analysis, non-normally distributed continuous variables were naturally log-transformed. Partial correlation coefficients were used to explore the relationship between RC and other plasma lipids after adjusting confounding factors. A linear regression model was used to assess the relationship between Hcy and plasma lipids. To further evaluate the potential associations of HHcy with atherogenic dyslipidemia, a logistic regression model was performed. A mediation analysis was performed using the PROCESS Procedure for SPSS Release 3.3 for HDL-C levels before and after adjustment. Animal and cellular studies showed that Hcy suppressed the protein synthesis of ApoA1, which is the main apolipoprotein of HDL-C and decreased HDL-C levels (11, 12). Therefore, we speculated that Hcy similarly influenced HDL-C levels through regulating ApoA1 in humans and detected whether ApoA1 plays a role in mediating the relationship between Hcy and HDL-C levels by using template Model 4 (Figure 1A). A two-tailed p<0.05 was considered statistically significant. SPSS 22.0 (Chicago, IL, United States) and Graphpad Prism 9.0 (San Diego, CA, United States) were used for the statistical analysis.
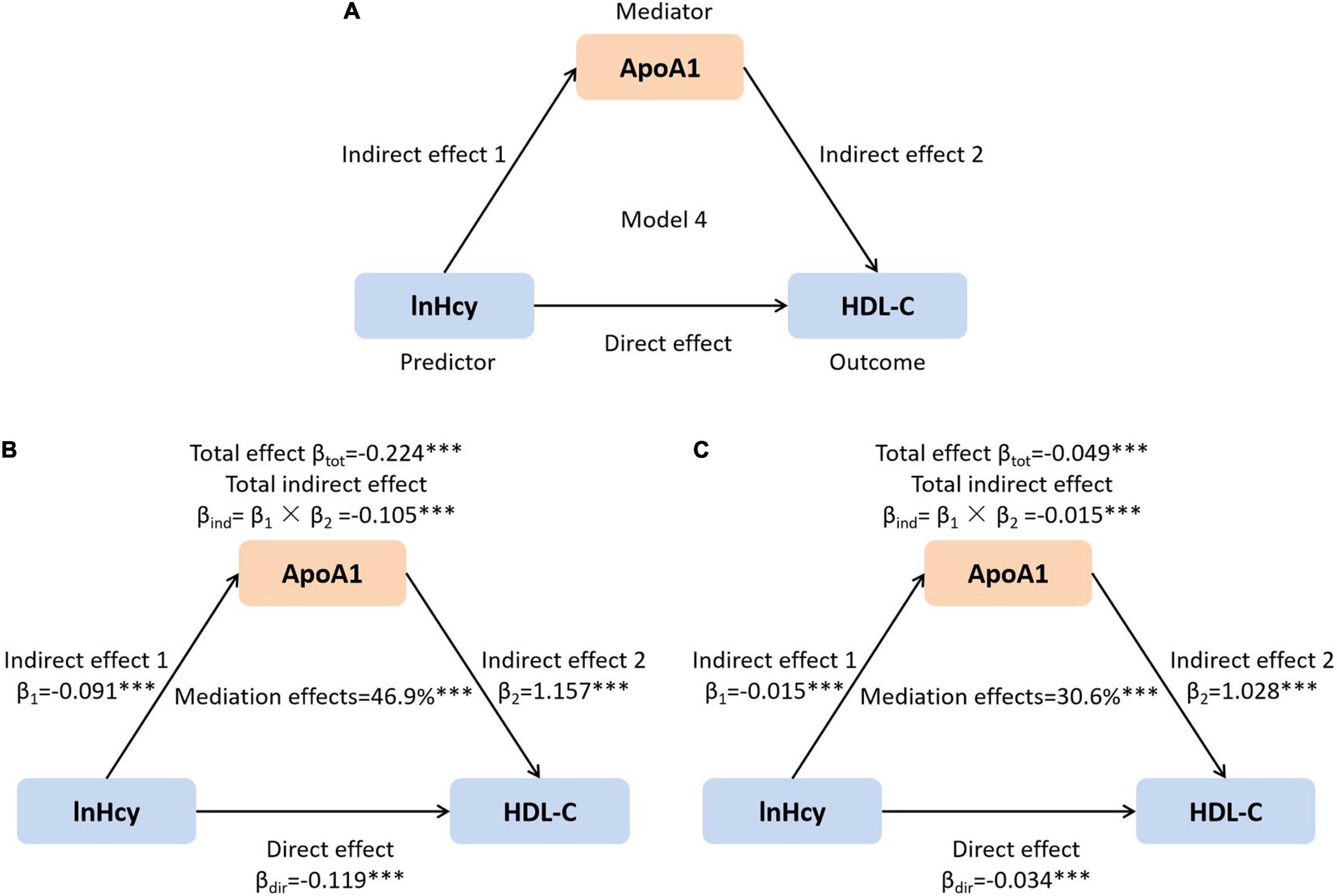
Figure 1. The mediation role of ApoA1 in the association of lnHcy with HDL-C. (A) Mediation model; (B) unadjusted; and (C) adjusted for age, sex, BMI, SBP, DBP, ALT, AST, Cr, UA, and glucose. ***p < 0.001. ApoA1, apolipoprotein A1; Hcy, homocysteine; HDL-C, high-density lipoprotein cholesterol; BMI, body mass index; SBP, systolic blood pressure; DBP, diastolic blood pressure; ALT, alanine aminotransferase; AST, aspartate aminotransferase; Cr, creatinine; UA, uric acid.
Results
Demographic and Laboratory Characteristics of Study Subjects
A total number of 7,898 subjects with an average age of 41.9 years were finally included in this study. Of the subjects, 4,154 (52.6%) were males. Compared with females, males had significantly higher age, BMI, SBP, DBP, ALT, AST, ZJU index, Cr, urea, UA, LDL-C, non-HDL-C, TG, ApoB, glucose, HbA1c, and prevalence of hyperglycemia and hypertension, whereas lower eGFR, HDL-C, and ApoA1 levels (all p < 0.001). The median level of plasma Hcy was 13.0 μmol/L in the whole population, which was also higher in males than females (p < 0.001) (Table 1).
Comparisons of Metabolic Parameters Between Subjects With Hyperhomocysteinemia and Those With Normal Range of Homocysteine
As the elevated level of plasma Hcy is an independent predictor of cardiovascular disease (31), we divided the subjects into HHcy group (Hcy ≥ 15 μmol/L) and normal group (Hcy < 15 μmol/L) and compared the metabolic parameters. As shown in Table 2, of the 7,898 subjects, 32.3% (2,550) had HHcy. They presented higher levels of SBP, DBP, ALT, AST, ZJU index, Cr, urea, UA, LDL-C, non-HDL-C, TG, and ApoB and lower levels of eGFR, HDL-C, and ApoA1 than those with normal range of Hcy (all p < 0.001). HHcy was more common in males than in females. Higher percentage of subjects with HHcy developed hyperglycemia and hypertension than those with normal range of Hcy (all p < 0.001). As sex, age, and BMI, which are all important influencing factors for metabolic health, were different between the two groups, thus we further adjusted these factors. SBP, DBP, ALT, AST, ZJU index, Cr, UA, glucose, and HbA1c levels were still significantly higher in HHcy group after adjustment (all p#< 0.001). However, for the plasma lipid profiles, only HDL-C was significantly decreased in subjects with HHcy compared with those with normal range of Hcy after adjusting potential confounding factors (p#< 0.001).
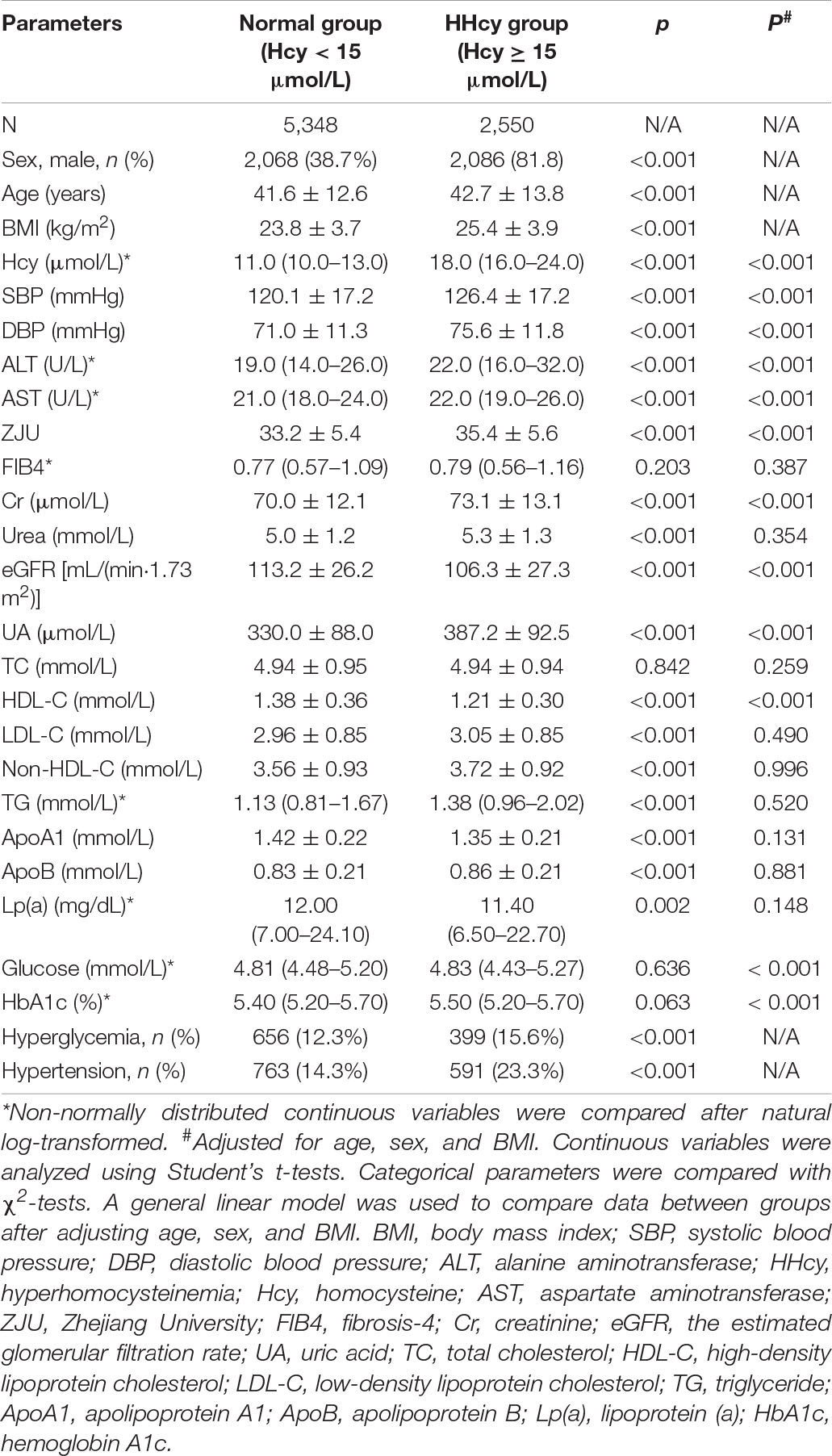
Table 2. The comparison of clinical parameters between subjects with hyperhomocysteinemia (HHcy) and those with normal range of homocysteine (Hcy).
Plasma Homocysteine Was Associated With Lipid Profiles in This Adult Population
In light of the essential role of dyslipidemia in the development of cardiovascular disease, we further did a linear correlation analysis between the lnHcy level and plasma lipids. As shown in Table 3, before adjusting confounding factors, plasma Hcy levels were significantly positively correlated with LDL-C, non-HDL-C, TG, and ApoB and inversely related with HDL-C, ApoA1, and Lp(a) levels (all p < 0.001). Then, we adjusted the potential confounders after excluding multicollinearity variables. After adjusting age, sex, and BMI, there was still an inverse relationship between plasma Hcy and HDL-C (p < 0.001). Even after further adjusting SBP, DBP, ALT, AST, Cr, UA, and glucose, the negative association of Hcy with HDL-C (p < 0.001), ApoA1 (p = 0.035), and Lp(a) (p = 0.049) and the positive association with TG levels (p = 0.043) still existed.
Hyperhomocysteinemia Increased the Risk of Low High-Density Lipoprotein Cholesterol, in Which Apolipoprotein A1 Might Play a Role
Furthermore, we evaluated the risk of atherogenic dyslipidemia in subjects with HHcy. Compared with those with a normal range of plasma Hcy, the prevalence of low HDL-C, high LDL-C, and hypertriglyceridemia increased by 1.26 (p < 0.001), 0.18 (p = 0.002), and 0.76 times (p < 0.001), respectively, in individuals with HHcy before adjusting confounding factors. Nevertheless, only the association of HHcy with low HDL-C was still significant after adjusting age, sex, BMI, SBP, DBP, ALT, AST, Cr, UA, and glucose in the whole population [odds ratio (OR) 1.26; 95%CI (1.11–1.44); p < 0.001] (Table 4).
The relationship between HHcy and low HDL-C was inconsistent in different subgroups (Table 5). Significant associations were observed in subjects relatively old [OR 1.40; 95% CI (1.16–1.69); p < 0.001], both males [OR 1.23; 95% CI (1.07–1.41); p = 0.003] and females [OR 1.42; 95% CI (1.01–2.01); p = 0.046], both overweight or obese subjects and lean individuals [OR 1.27; 95%CI (1.08–1.49); p = 0.004 and OR 1.35; 95% CI (1.09–1.66); p = 0.006, respectively], and those without hyperglycemia [OR 1.27; 95% CI (1.10–1.47); p = 0.001] and hypertension [OR 1.34; 95% CI (1.16–1.55); p < 0.001] after adjusting other confounding factors. However, sex, age, BMI, and blood glucose level did not significantly affect the risk of low HDL-C in subjects with HHcy (p for interaction > 0.05). The low HDL-C risk was only significantly higher in those without hypertension than subjects with hypertension (p for interaction = 0.029).
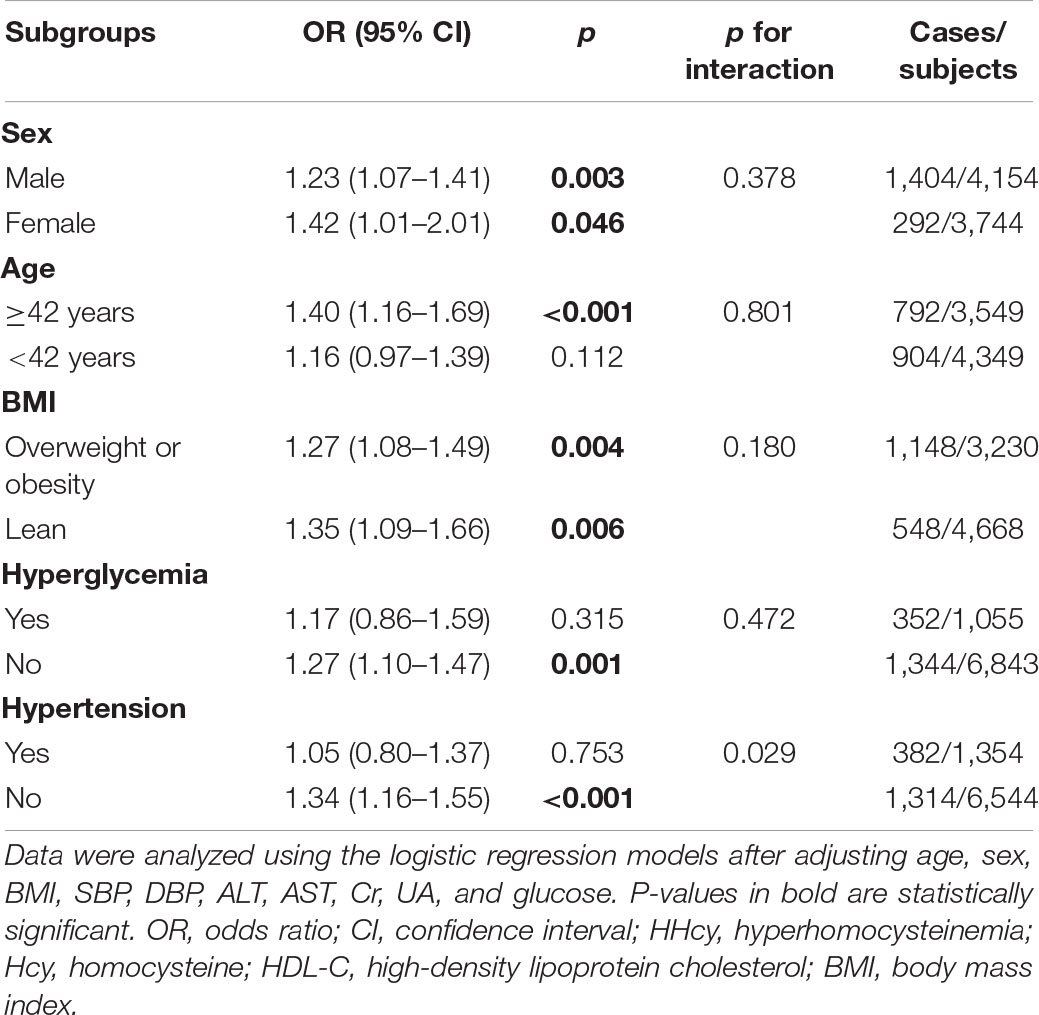
Table 5. Risk of prevalent low HDL-C in different subgroups among subjects with HHcy compared with those with normal plasma Hcy.
It has been reported that Hcy could reduce the HDL-C level by suppressing the synthesis of ApoA1 in mice (12, 32). In this study, the Hcy level was inversely related with plasma ApoA1. Therefore, we further assessed the mediation role of ApoA1 in the effects of plasma Hcy on HDL-C in this population. As shown in Figures 1B,C, the total and direct effects of Hcy on HDL-C, as well as the total indirect effect via ApoA1, were all significant (all p < 0.001). The net mediation effects of ApoA1 before and after adjusting confounders were 46.9 and 30.6%, respectively.
Remnant Cholesterol Was Dramatically Elevated in Subjects With Hyperhomocysteinemia and Had a Close Relationship With Other Plasma Lipids
Low-density lipoprotein cholesterol has been well-recognized as the atherogenic lipoprotein. However, no obvious correlation between HHcy and high LDL-C was detected in our study. Recently, emerging evidence implicated the critical role of TGRLs and their remnants (also named as RC) in ASCVD (19, 24). We assessed the level of RC in our study and showed that plasma RC was significantly increased in subjects with overweight and obesity, hyperglycemia, hypertension, and HHcy compared with their control groups, respectively (Figures 2A–D). Interestingly, the RC level was also dramatically increased in subjects with HHcy compared with those with normal range of Hcy after adjusting age, sex, BMI, SBP, DBP, ALT, AST, Cr, UA, and glucose (p = 0.025).
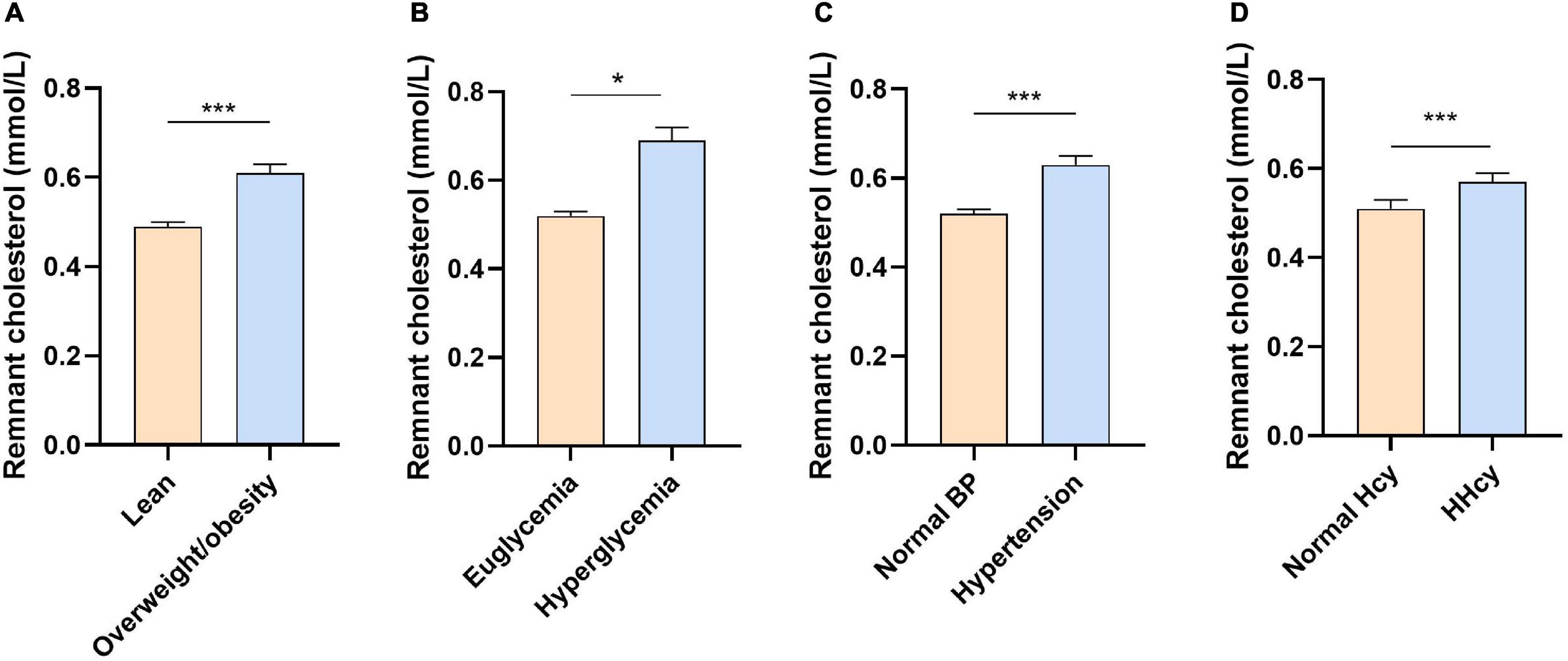
Figure 2. The concentration of remnant cholesterol in different groups. (A) Overweight or obesity vs. lean; (B) hyperglycemia vs. euglycemia; (C) hypertension vs. normal blood pressure; and (D) HHcy vs. normal Hcy. Data were presented as median ± 95% CI and were analyzed using Student’s t-test after natural log-transformed. *p < 0.05 and ***p < 0.001. BP, blood pressure; HHcy, hyperhomocysteinemia; Hcy, homocysteine.
We further assessed the relationships between RC and other plasma lipids using the partial correlation coefficient analysis. As shown in Table 6, a significant positive association of RC with TG, TC, non-HDL-C, ApoA1, and ApoB and an inverse association with HDL-C, LDL-C, and Lp(a) were detected after adjusting confounding influencing factors (all p < 0.001, except ApoB p = 0.011).
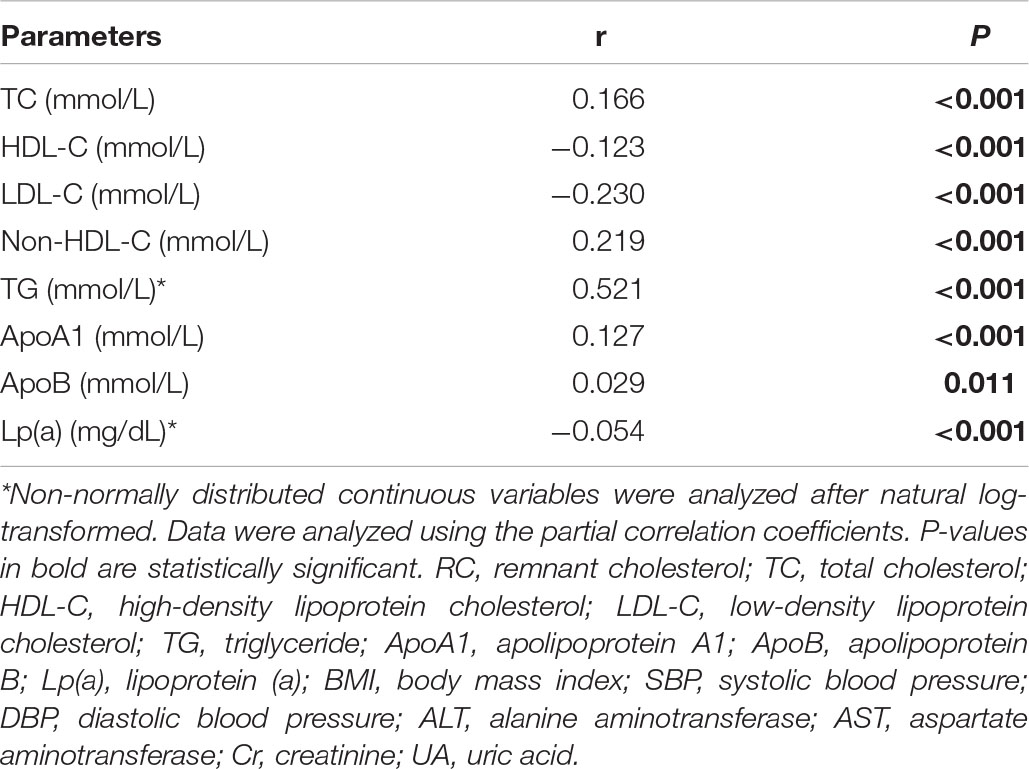
Table 6. The relationship between lnRC and other plasma lipids after adjusting for age, sex, BMI, SBP, DBP, ALT, AST, Cr, UA, and glucose.
Homocysteine Was Independently Associated With Plasma Remnant Cholesterol Level Beyond Other Lipids
Finally, we analyzed the linear association of plasma Hcy with RC (Table 7) and found a significant positive correlation between Hcy and RC after adjusting age, sex, BMI, SBP, DBP, ALT, AST, Cr, UA, and glucose (β = 0.073, p = 0.004). This association was still significant even after adjusting the closely correlated plasma lipid profiles, including TG, LDL-C, HDL-C, ApoA1, ApoB, and Lp(a) (model 4–9), which indicated that plasma Hcy was independently related with RC beyond other lipids.
Discussion
In the large cross-sectional study among Chinese adults, we found that elevated Hcy levels were positively associated with plasma TG and inversely correlated with HDL-C, ApoA1, and Lp(a) after controlling age, sex, BMI, SBP, DBP, ALT, AST, Cr, UA, and glucose. HHcy significantly increased the risk of low HDL-C. More interestingly, Hcy was also independently correlated with plasma RC levels beyond other conventional lipids, including TG, LDL-C, HDL-C, ApoA1, ApoB, and Lp(a). Our findings suggest that identifying Hcy-related dyslipidemia risk, both traditional atherogenic lipids and RC residual risk, is clinically significant as we usher in a new era of targeted Hcy-lowering therapies to fight against dyslipidemia and ASCVD. Future studies are required to decipher the mechanism linking plasma Hcy and lipids, especially RC, independent of other traditional cardiovascular risk factors and to determine whether reducing Hcy improves lipids and clinical outcomes.
Plasma Hcy is an independent risk factor for ASCVD (33–36). Nevertheless, the mechanism linking Hcy to cardiovascular disease is not fully understood. Experimental studies indicated that HHcy-induced lipid alterations might play a role (11, 12). Barter et al. also proposed that HDL-C might be a link between Hcy and ASCVD (31). Although evidence is inconsistent and limited, previous epidemiological and clinical studies investigated the association of Hcy with plasma lipids. An Iran study carried out in patients with myocardial infarction first showed that Hcy was negatively correlated with HDL-C and positively related with LDL-C (37). Another study from India also demonstrated that plasma Hcy had an inverse relationship with HDL-C and a positive relationship with TG and very-low-density lipoprotein (VLDL) in subjects with coronary artery disease (38). Real et al. uncovered the inverse correlation between plasma Hcy and HDL-C in individuals with heterozygous familial hypercholesterolemia (39), which was confirmed by Guéant-Rodriguez et al. in the elderly population (40). Other studies also showed that HHcy could enhance LDL oxidation, which was modulated by the genetic and dietary factors (41, 42). In China, Huang et al. first explored the association of plasma Hcy with lipid profile in 192 hyperlipidemia patients and 208 normal individuals and found that HHcy was the risk factor for both hypercholesterolemia and hypertriglyceridemia (43). Then, Xiao et al. showed that plasma Hcy correlated inversely with ApoA1 and HDL-C among 2,058 patients undergoing coronary artery angiography (44). Subsequently, the positive correlation with TC, TG, and LDL-C and the inverse relationship with HDL-C of plasma Hcy were verified in Chinese patients who underwent physical examinations in Guangxi (45) and in a community-based population from Hunan (7). However, some studies did not support the correlation between Hcy and plasma lipids. Yadav et al. observed no significant correlation between plasma Hcy and TC, TG, and HDL-C in patients with ischemic heart disease (46). Another study found no significant association of Hcy with plasma lipids among 155 patients with diabetes (47). Lupton et al. suggested lower levels of LDL-C, non-HDL-C, and HDL-C, whereas higher levels of TG and VLDL-C in the fourth Hcy quartile among US adults before adjustment. Nevertheless, these associations disappeared after controlling for age, sex, HbA1c, insulin, Cr, and urea (15). In this study among Chinese adults aged 20–29 years in Beijing, we found that plasma Hcy levels were positively correlated with TG and inversely correlated with HDL-C, ApoA1, and Lp(a) after adjusting potential confounders including age, sex, BMI, SBP, DBP, ALT, AST, Cr, UA, and glucose. The prevalence of HHcy was 32.3%, which was lower than that of the study carried out in Guangxi (50.8%) (45). HHcy only significantly increased the risk of low HDL-C beyond the confounders. The different findings between this study and other studies might be attributable to the age, race, sex, diets, folic acid and vitamin B intake, geographic regions, physical activity, and sample number of the study population. Additionally, the indicators and confounding factors included in data analyses among the studies were also different. Our study included more plasma lipids, such as ApoA1, ApoB, and Lp(a), and controlled more influencing factors, such as glucose, blood pressure, and liver and kidney function, which were significantly elevated in subjects with HHcy in this study and were shown to be associated with Hcy levels in prior studies when performing the correlation analysis (15, 48, 49). In the future, more standard, large-scale, and prospective studies are needed to clarify the association of Hcy with plasma lipids.
Pharmacological LDL-C-lowering therapy via statin had obtained a significant impact on decreasing the incidence of ASCVD (50). However, distinct residual ASCVD risk has been reported among statin-treated patients, even with low levels of LDL-C (51). Low HDL-C was one of the most important factors for the residual risk, but there is no effective HDL-C-raising therapy till present (52). High Lp(a) was recently considered as a causal risk factor for ASCVD, whereas Lp(a) concentrations were reported to be primarily determined by genetics, and Lp(a)-lowering therapy is limited (53, 54). Although the residual risk may be attributable to many other factors, such as sedentary lifestyles, smoking, hyperglycemia, hypertension, and inflammation, the scientists have focused their interest in the TGRL or RC (16, 55, 56). The National Lipid Association writing group also recommended performing advanced lipid measurements, including RC, in these patients (57). TGRLs are highly heterogeneous lipoprotein particles derived from the intestine and liver and contain large amounts of TG and cholesterol, as well as transport approximately one-third of cholesterol in ApoB-containing lipoprotein particles (58). The cholesterol content of remnant lipoproteins was defined as RC and was highly atherogenic (59). When it comes to the association of Hcy with plasma RC levels, evidence is scarce. Only one study from the Very Large Database of Lipids in US adults showed that RC was 2–6% higher in the highest Hcy quartile before adjustment. When they controlled age, sex, HbA1c, insulin, Cr, and urea, the association disappeared (15). In this study, RC was defined as intermediate-density lipoprotein cholesterol (IDL-C) plus VLDL-C. In this study, RC was calculated as non-HDL-C minus LDL-C. Results showed that RC levels were significantly increased with Hcy elevation. After adjusting age, sex, BMI, SBP, DBP, ALT, AST, Cr, UA, and glucose, the positive association of Hcy with RC was still significant. The differences in populations, adjusted confounders, and the calculation method of RC might partially explain the inconsistency. Our study preliminarily indicated that HHcy-induced high level of RC might be an important mediating factor for the ASCVD risk.
A previous study demonstrated that the risk of myocardial infarction increased consecutively with an increase in RC even after adjusting LDL-C (60). Despite RC-related ASCVD risk proportional to the change of ApoB being reported (23), a recently published prevention study carried out in ASCVD-free US individuals showed that elevated plasma RC levels were associated with ASCVD independent of LDL-C and ApoB (24). In this study, the LDL-C was estimated by the Martin/Hopkins equation according to non-HDL-C and TG levels using an adjustable factor for the TG:VLDL ratio (61). These studies suggested that RC might modify ASCVD risk beyond the total particles. They also illustrated that there might be some indirect atherogenic mechanisms of RC, such as reflecting the increased activity of key lipid regulatory proteins, apolipoprotein C3 (ApoC3), or angiopoietin-like protein 3 (ANGPTL3). Consistently, our study showed that the association of plasma Hcy with RC levels was independent of other lipids, including TG, HDL-C, LDL-C, ApoA1, ApoB, and Lp(a), despite the estimation method of LDL-C is different from the study carried out by Quispe et al. (24). Mechanisms linking HHcy to high RC levels beyond the cholesterol, ApoA1, ApoB, and Lp(a) might exist and requires more investigation and verification.
The mechanisms underlying the association of plasma Hcy with lipid profile have been investigated in basic experimental studies. Liao et al. (12) and Mikael et al. (32) both showed that Hcy could inhibit the hepatic synthesis and expression of ApoA1, the main apolipoprotein of HDL-C, which explained not only the inverse association of Hcy with ApoA1 and HDL-C but also the Hcy-induced ASCVD risk. Our study similarly uncovered an inverse relationship between Hcy and plasma ApoA1 and HDL-C and showed that ApoA1 was an important mediator for the effects of plasma Hcy on HDL-C levels even after controlling potential confounders. In addition, it has been reported that Hcy could induce ER stress to activate sterol regulatory element binding proteins (SREBP), an important transcriptional factor involved in cholesterol and TG biosynthesis and thus increased cholesterol and TG levels (11). Moreover, the oxidative stress induced by protein misfolding in ER (62, 63) and global DNA hypomethylation modification (64) were both the potential mechanisms by which Hcy affected lipid metabolism. In the future, more studies need to be carried out to decipher the mechanism linking Hcy to atherogenic dyslipidemia, especially RC levels.
As published studies and our study both demonstrated that HHcy level increased the risks of dyslipidemia, how about decreasing the plasma Hcy? Recent small-scale clinical studies and animal experiments have preliminarily investigated the effects of folic acid supplementation, a well-acknowledged method to decrease Hcy, on lipid metabolism. Baszczuk et al. showed that 15 mg of folic acid per day for 45 days caused a significant growth of HDL-C and ApoA1 and a reduction of ApoB in 42 patients with primary hypertension (65). Vijayakumar et al. found that 400 μg of folic acid two times a day for 8 weeks significantly decreased LDL-C levels and the LDL-C/HDL-C ratio among 25 postmenopausal diabetic Korean women (66). The newest meta-analysis of 38 randomized controlled trials in adults summarized that folic acid supplementation decreased plasma TG and TC levels but did not affect LDL-C or HDL-C (67). Atherosclerotic and high-fat-diet-fed animal models also showed that folic acid increased HDL-C and enhanced antioxidative and anti-inflammation capacity (68, 69). Inconsistently, Satapathy et al. added folic acid and vitamin B12 for 8 weeks to 80 subjects with type 2 diabetes mellitus and did not find an improvement of lipid profiles (70). In the future, large-scale randomized controlled trials in different populations are required to clarify the beneficial effects of Hcy-lowering therapy on plasma lipids, particularly RC levels.
There are some limitations in our study. First, we did not collect covariates, such as dietary intake, vitamin B supplements intake, physical activity, and smoking and alcohol consumption information, which might affect the associations of Hcy with lipids. Second, the plasma levels of folic acid and vitamin B12 were not detected in our population, which was shown to regulate plasma Hcy levels. Third, RC was estimated by TC minus LDL-C and HDL-C but was not directly measured. Fourth, this is a single center study carried out in the Chinese population, thus cannot represent other regions and populations. In addition, this is a cross-sectional study and cannot uncover the causality between Hcy and lipids. Finally, the mechanism underlying the effects of plasma Hcy on lipid profiles is still largely unclear and needs more investigation.
Conclusion
In conclusion, to the best of our knowledge, this is one of the largest studies to explore the association of plasma Hcy levels with conventional lipid profiles in China, and this is the first study to focus on the relationship between Hcy and RC levels in Chinese adults. Our study demonstrated that plasma Hcy levels were independently positively associated with TG and negatively associated with HDL-C, ApoA1, and Lp(a) after adjusting all potential confounders. HHcy significantly increased the risk of low HDL-C. The reduction of ApoA1 partially mediated this effect. More importantly, plasma Hcy was significantly associated with RC levels after adjusting confounding factors and even beyond other lipids, including TG, HDL-C, LDL-C, ApoA1, ApoB, and Lp(a). This study added Chinese evidence to the Hcy-induced dyslipidemia and provide novel insight for the prevention and treatment of atherogenic dyslipidemia or even ASCVD risk in patients with HHcy.
Data Availability Statement
The original contributions presented in this study are included in the article/supplementary material, further inquiries can be directed to the corresponding author/s.
Ethics Statement
The studies involving human participants were reviewed and approved by the Ethics Committee of the Beijing Chao-Yang Hospital affiliated with Capital Medical University. The patients/participants provided their written informed consent to participate in this study.
Author Contributions
LZ and JL analyzed the data and drafted the manuscript. YA integrated the data. YW was responsible for recruiting subjects, collecting data, and revised the manuscript. GW contributed to the study design, data interpretation, and reviewed the manuscript. All authors read and approved the final version of this manuscript.
Funding
This study was supported by the Glod Seed Scientific Foundation of Beijing Chao-Yang Hospital (No. CYJZ202152) and the National Natural Science Foundation of China (Nos. 81770792 and 81972137).
Conflict of Interest
The authors declare that the research was conducted in the absence of any commercial or financial relationships that could be construed as a potential conflict of interest.
Publisher’s Note
All claims expressed in this article are solely those of the authors and do not necessarily represent those of their affiliated organizations, or those of the publisher, the editors and the reviewers. Any product that may be evaluated in this article, or claim that may be made by its manufacturer, is not guaranteed or endorsed by the publisher.
Acknowledgments
We thank all the subjects for their contributions to this study.
References
1. de Oliveira Leite L, Costa Dias Pitangueira J, Ferreira Damascena N, Ribas de Farias Costa P. Homocysteine levels and cardiovascular risk factors in children and adolescents: systematic review and meta-analysis. Nutr Rev. (2021) 79:1067–78. doi: 10.1093/nutrit/nuaa116
2. Yuan S, Mason AM, Carter P, Burgess S, Larsson SC. Homocysteine, B vitamins, and cardiovascular disease: a mendelian randomization study. BMC Med. (2021) 19:97. doi: 10.1186/s12916-021-01977-8
3. Zhang T, Jiang Y, Zhang S, Tie T, Cheng Y, Su X, et al. The association between homocysteine and ischemic stroke subtypes in Chinese: a meta-analysis. Medicine (Baltimore). (2020) 99:e19467. doi: 10.1097/md.0000000000019467
4. Jin N, Huang L, Hong J, Zhao X, Chen Y, Hu J, et al. Elevated homocysteine levels in patients with heart failure: a systematic review and meta-analysis. Medicine (Baltimore). (2021) 100:e26875. doi: 10.1097/md.0000000000026875
5. Boushey CJ, Beresford SA, Omenn GS, Motulsky AG. A quantitative assessment of plasma homocysteine as a risk factor for vascular disease. Probable benefits of increasing folic acid intakes. JAMA. (1995) 274:1049–57. doi: 10.1001/jama.1995.03530130055028
6. Spence JD, Yi Q, Hankey GJ. B vitamins in stroke prevention: time to reconsider. Lancet Neurol. (2017) 16:750–60. doi: 10.1016/s1474-4422(17)30180-1
7. Niu X, Chen J, Wang J, Li J, Zeng D, Wang S, et al. A Cross-sectional study on the relationship between homocysteine and lipid profiles among Chinese population from hunan. Lipids. (2021) 56:93–100. doi: 10.1002/lipd.12279
8. Esse R, Barroso M, Tavares de Almeida I, Castro R. The contribution of homocysteine metabolism disruption to endothelial dysfunction: state-of-the-art. Int J Mol Sci. (2019) 20:867. doi: 10.3390/ijms20040867
9. Perła-Kaján J, Jakubowski H. Dysregulation of epigenetic mechanisms of gene expression in the pathologies of hyperhomocysteinemia. Int J Mol Sci. (2019) 20:3140. doi: 10.3390/ijms20133140
10. Kaplan P, Tatarkova Z, Sivonova MK, Racay P, Lehotsky J. Homocysteine and mitochondria in cardiovascular and cerebrovascular systems. Int J Mol Sci. (2020) 21:7698. doi: 10.3390/ijms21207698
11. Werstuck GH, Lentz SR, Dayal S, Hossain GS, Sood SK, Shi YY, et al. Homocysteine-induced endoplasmic reticulum stress causes dysregulation of the cholesterol and triglyceride biosynthetic pathways. J Clin Invest. (2001) 107:1263–73. doi: 10.1172/jci11596
12. Liao D, Tan H, Hui R, Li Z, Jiang X, Gaubatz J, et al. Hyperhomocysteinemia decreases circulating high-density lipoprotein by inhibiting apolipoprotein A-I Protein synthesis and enhancing HDL cholesterol clearance. Circ Res. (2006) 99:598–606. doi: 10.1161/01.Res.0000242559.42077.22
13. Julve J, Escolà-Gil JC, Rodríguez-Millán E, Martín-Campos JM, Jauhiainen M, Quesada H, et al. Methionine-induced hyperhomocysteinemia impairs the antioxidant ability of high-density lipoproteins without reducing in vivo macrophage-specific reverse cholesterol transport. Mol Nutr Food Res. (2013) 57:1814–24. doi: 10.1002/mnfr.201300133
14. Momin M, Jia J, Fan F, Li J, Dou J, Chen D, et al. Relationship between plasma homocysteine level and lipid profiles in a community-based Chinese population. Lipids Health Dis. (2017) 16:54. doi: 10.1186/s12944-017-0441-6
15. Lupton JR, Quispe R, Kulkarni K, Martin SS, Jones SR. Serum homocysteine is not independently associated with an atherogenic lipid profile: the very large database of lipids (VLDL-21) study. Atherosclerosis. (2016) 249:59–64. doi: 10.1016/j.atherosclerosis.2016.03.031
16. Lawler PR, Akinkuolie AO, Chu AY, Shah SH, Kraus WE, Craig D, et al. Atherogenic lipoprotein determinants of cardiovascular disease and residual risk among individuals with low low-density lipoprotein cholesterol. J Am Heart Assoc. (2017) 6:e005549. doi: 10.1161/jaha.117.005549
17. Aday AW, Lawler PR, Cook NR, Ridker PM, Mora S, Pradhan AD. Lipoprotein particle profiles, standard lipids, and peripheral artery disease incidence. Circulation. (2018) 138:2330–41. doi: 10.1161/circulationaha.118.035432
18. Ginsberg HN, Packard CJ, Chapman MJ, Borén J, Aguilar-Salinas CA, Averna M, et al. Triglyceride-rich lipoproteins and their remnants: metabolic insights, role in atherosclerotic cardiovascular disease, and emerging therapeutic strategies-a consensus statement from the European Atherosclerosis Society. Eur Heart J. (2021) 42:4791–806. doi: 10.1093/eurheartj/ehab551
19. Castañer O, Pintó X, Subirana I, Amor AJ, Ros E, Hernáez Á, et al. Remnant cholesterol, not LDL cholesterol, is associated with incident cardiovascular disease. J Am Coll Cardiol. (2020) 76:2712–24. doi: 10.1016/j.jacc.2020.10.008
20. Jørgensen AB, Frikke-Schmidt R, West AS, Grande P, Nordestgaard BG, Tybjærg-Hansen A. Genetically elevated non-fasting triglycerides and calculated remnant cholesterol as causal risk factors for myocardial infarction. Eur Heart J. (2013) 34:1826–33. doi: 10.1093/eurheartj/ehs431
21. Varbo A, Benn M, Tybjærg-Hansen A, Jørgensen AB, Frikke-Schmidt R, Nordestgaard BG. Remnant cholesterol as a causal risk factor for ischemic heart disease. J Am Coll Cardiol. (2013) 61:427–36. doi: 10.1016/j.jacc.2012.08.1026
22. Sandesara PB, Virani SS, Fazio S, Shapiro MD. The forgotten lipids: triglycerides, remnant cholesterol, and atherosclerotic cardiovascular disease risk. Endocr Rev. (2019) 40:537–57. doi: 10.1210/er.2018-00184
23. Ference BA, Kastelein JJP, Ray KK, Ginsberg HN, Chapman MJ, Packard CJ, et al. Association of triglyceride-lowering LPL variants and LDL-C-lowering LDLR variants with risk of coronary heart disease. JAMA. (2019) 321:364–73. doi: 10.1001/jama.2018.20045
24. Quispe R, Martin SS, Michos ED, Lamba I, Blumenthal RS, Saeed A, et al. Remnant cholesterol predicts cardiovascular disease beyond LDL and ApoB: a primary prevention study. Eur Heart J. (2021) 42:4324–32. doi: 10.1093/eurheartj/ehab432
25. Li X, Qin P, Cao L, Lou Y, Shi J, Zhao P, et al. Dose-response association of the ZJU index and fatty liver disease risk: a large cohort in China. J Gastroenterol Hepatol. (2021) 36:1326–33. doi: 10.1111/jgh.15286
26. Ishiba H, Sumida Y, Tanaka S, Yoneda M, Hyogo H, Ono M, et al. The novel cutoff points for the FIB4 index categorized by age increase the diagnostic accuracy in NAFLD: a multi-center study. J Gastroenterol. (2018) 53:1216–24. doi: 10.1007/s00535-018-1474-y
29. American Diabetes Association. 2. Classification and diagnosis of diabetes: standards of medical care in diabetes–2021. Diabetes Care. (2021) 44(Suppl. 1):S15–33. doi: 10.2337/dc21-S002
30. Wang Q, Zhang J, Zhao K, Xu B. Hyperhomocysteinemia is an independent risk factor for intracranial aneurysms: a case-control study in a Chinese Han population. Neurosurg Rev. (2020) 43:1127–34. doi: 10.1007/s10143-019-01138-9
31. Barter PJ, Rye KA. Homocysteine and cardiovascular disease: is HDL the link? Circ Res. (2006) 99:565–6. doi: 10.1161/01.RES.0000243583.39694.1f
32. Mikael LG, Genest J Jr., Rozen R. Elevated homocysteine reduces apolipoprotein A-I expression in hyperhomocysteinemic mice and in males with coronary artery disease. Circ Res. (2006) 98:564–71. doi: 10.1161/01.RES.0000204825.66410.0b
33. Clarke R, Daly L, Robinson K, Naughten E, Cahalane S, Fowler B, et al. Hyperhomocysteinemia: an independent risk factor for vascular disease. N Engl J Med. (1991) 324:1149–55. doi: 10.1056/nejm199104253241701
34. Moustapha A, Naso A, Nahlawi M, Gupta A, Arheart KL, Jacobsen DW, et al. Prospective study of hyperhomocysteinemia as an adverse cardiovascular risk factor in end-stage renal disease. Circulation. (1998) 97:138–41. doi: 10.1161/01.cir.97.2.138
35. Okada E, Oida K, Tada H, Asazuma K, Eguchi K, Tohda G, et al. Hyperhomocysteinemia is a risk factor for coronary arteriosclerosis in Japanese patients with type 2 diabetes. Diabetes Care. (1999) 22:484–90. doi: 10.2337/diacare.22.3.484
36. Tian D, Qin Q, Li M, Li X, Xu Q, Lv Q. Homocysteine impairs endothelial cell barrier function and angiogenic potential via the progranulin/EphA2 pathway. Front Pharmacol. (2020) 11:614760. doi: 10.3389/fphar.2020.614760
37. Qujeq D, Omran TS, Hosini L. Correlation between total homocysteine, low-density lipoprotein cholesterol and high-density lipoprotein cholesterol in the serum of patients with myocardial infarction. Clin Biochem. (2001) 34:97–101. doi: 10.1016/s0009-9120(01)00187-4
38. Mahalle N, Kulkarni MV, Garg MK, Naik SS. Vitamin B12 deficiency and hyperhomocysteinemia as correlates of cardiovascular risk factors in Indian subjects with coronary artery disease. J Cardiol. (2013) 61:289–94. doi: 10.1016/j.jjcc.2012.11.009
39. Real JT, Martinez-Hervas S, Garcia-Garcia AB, Chaves FJ, Civera M, Ascaso JF, et al. Association of C677T polymorphism in MTHFR gene, high homocysteine and low HDL cholesterol plasma values in heterozygous familial hypercholesterolemia. J Atheroscler Thromb. (2009) 16:815–20. doi: 10.5551/jat.2196
40. Guéant-Rodriguez RM, Spada R, Moreno-Garcia M, Anello G, Bosco P, Lagrost L, et al. Homocysteine is a determinant of ApoA-I and both are associated with ankle brachial index, in an ambulatory elderly population. Atherosclerosis. (2011) 214:480–5. doi: 10.1016/j.atherosclerosis.2010.11.031
41. Pfanzagl B, Tribl F, Koller E, Möslinger T. Homocysteine strongly enhances metal-catalyzed LDL oxidation in the presence of cystine and cysteine. Atherosclerosis. (2003) 168:39–48. doi: 10.1016/s0021-9150(03)00057-1
42. Koubaa N, Nakbi A, Smaoui M, Abid N, Chaaba R, Abid M, et al. Hyperhomocysteinemia and elevated ox-LDL in tunisian type 2 diabetic patients: role of genetic and dietary factors. Clin Biochem. (2007) 40:1007–14. doi: 10.1016/j.clinbiochem.2007.05.017
43. Huang L, Song XM, Zhu WL, Li Y. Plasma homocysteine and gene polymorphisms associated with the risk of hyperlipidemia in northern Chinese subjects. Biomed Environ Sci. (2008) 21:514–20. doi: 10.1016/s0895-3988(09)60011-8
44. Xiao Y, Zhang Y, Lv X, Su D, Li D, Xia M, et al. Relationship between lipid profiles and plasma total homocysteine, cysteine and the risk of coronary artery disease in coronary angiographic subjects. Lipids Health Dis. (2011) 10:137. doi: 10.1186/1476-511x-10-137
45. Qin YY, Wang P, Qin JQ, Wei AQ, Huang P, Lai ZF, et al. Prevalence of hyperhomocysteinemia during routine physical examination in Guangxi province, China and related risk factors. J Clin Lab Anal. (2018) 32:e22178. doi: 10.1002/jcla.22178
46. Yadav AS, Bhagwat VR, Rathod IM. Relationship of plasma homocysteine with lipid profile parameters in ischemic heart disease. Indian J Clin Biochem. (2006) 21:106–10. doi: 10.1007/bf02913076
47. de Luis DA, Fernandez N, Arranz ML, Aller R, Izaola O, Romero E. Total homocysteine levels relation with chronic complications of diabetes, body composition, and other cardiovascular risk factors in a population of patients with diabetes mellitus type 2. J Diabetes Complications. (2005) 19:42–6. doi: 10.1016/j.jdiacomp.2003.12.003
48. Passaro A, Calzoni F, Volpato S, Nora ED, Pareschi PL, Zamboni PF, et al. Effect of metabolic control on homocysteine levels in type 2 diabetic patients: a 3-year follow-up. J Intern Med. (2003) 254:264–71. doi: 10.1046/j.1365-2796.2003.01184.x
49. Duncan GE, Li SM, Zhou XH. Age and kidney function are the primary correlates of fasting plasma total homocysteine levels in non-diabetic and diabetic adults. Results from the 1999-2002 national health and nutrition examination survey. Nutr Metab (Lond). (2005) 2:13. doi: 10.1186/1743-7075-2-13
50. Baigent C, Blackwell L, Emberson J, Holland LE, Reith C, Bhala N, et al. Efficacy and safety of more intensive lowering of LDL cholesterol: a meta-analysis of data from 170,000 participants in 26 randomised trials. Lancet. (2010) 376:1670–81. doi: 10.1016/s0140-6736(10)61350-5
51. Sirimarco G, Labreuche J, Bruckert E, Goldstein LB, Fox KM, Rothwell PM, et al. Atherogenic dyslipidemia and residual cardiovascular risk in statin-treated patients. Stroke. (2014) 45:1429–36. doi: 10.1161/strokeaha.113.004229
52. Landray MJ, Haynes R, Hopewell JC, Parish S, Aung T, Tomson J, et al. Effects of extended-release niacin with laropiprant in high-risk patients. N Engl J Med. (2014) 371:203–12. doi: 10.1056/NEJMoa1300955
53. Kamstrup PR. Lipoprotein(a) and cardiovascular disease. Clin Chem. (2021) 67:154–66. doi: 10.1093/clinchem/hvaa247
54. Ruscica M, Sirtori CR, Corsini A, Watts GF, Sahebkar A. Lipoprotein(a): knowns, unknowns and uncertainties. Pharmacol Res. (2021) 173:105812. doi: 10.1016/j.phrs.2021.105812
55. Langsted A, Freiberg JJ, Tybjaerg-Hansen A, Schnohr P, Jensen GB, Nordestgaard BG. Nonfasting cholesterol and triglycerides and association with risk of myocardial infarction and total mortality: the copenhagen city heart study with 31 years of follow-up. J Intern Med. (2011) 270:65–75. doi: 10.1111/j.1365-2796.2010.02333.x
56. Ruscica M, Corsini A, Ferri N, Banach M, Sirtori CR. Clinical approach to the inflammatory etiology of cardiovascular diseases. Pharmacol Res. (2020) 159:104916. doi: 10.1016/j.phrs.2020.104916
57. Wilson PWF, Jacobson TA, Martin SS, Jackson EJ, Le NA, Davidson MH, et al. Lipid measurements in the management of cardiovascular diseases: practical recommendations a scientific statement from the national lipid association writing group. J Clin Lipidol. (2021) 15:629–48. doi: 10.1016/j.jacl.2021.09.046
58. Packard CJ, Boren J, Taskinen MR. Causes and consequences of hypertriglyceridemia. Front Endocrinol (Lausanne). (2020) 11:252. doi: 10.3389/fendo.2020.00252
59. Hoogeveen RC, Ballantyne CM. Residual cardiovascular risk at low LDL: remnants, lipoprotein(a), and inflammation. Clin Chem. (2021) 67:143–53. doi: 10.1093/clinchem/hvaa252
60. Varbo A, Freiberg JJ, Nordestgaard BG. Extreme nonfasting remnant cholesterol vs extreme LDL cholesterol as contributors to cardiovascular disease and all-cause mortality in 90000 individuals from the general population. Clin Chem. (2015) 61:533–43. doi: 10.1373/clinchem.2014.234146
61. Martin SS, Blaha MJ, Elshazly MB, Toth PP, Kwiterovich PO, Blumenthal RS, et al. Comparison of a novel method vs the Friedewald equation for estimating low-density lipoprotein cholesterol levels from the standard lipid profile. JAMA. (2013) 310:2061–8. doi: 10.1001/jama.2013.280532
62. Outinen PA, Sood SK, Pfeifer SI, Pamidi S, Podor TJ, Li J, et al. Homocysteine-induced endoplasmic reticulum stress and growth arrest leads to specific changes in gene expression in human vascular endothelial cells. Blood. (1999) 94:959–67.
63. Thampi P, Stewart BW, Joseph L, Melnyk SB, Hennings LJ, Nagarajan S. Dietary homocysteine promotes atherosclerosis in apoE-deficient mice by inducing scavenger receptors expression. Atherosclerosis. (2008) 197:620–9. doi: 10.1016/j.atherosclerosis.2007.09.014
64. Yideng J, Jianzhong Z, Ying H, Juan S, Jinge Z, Shenglan W, et al. Homocysteine-mediated expression of SAHH, DNMTs, MBD2, and DNA hypomethylation potential pathogenic mechanism in VSMCs. DNA Cell Biol. (2007) 26:603–11. doi: 10.1089/dna.2007.0584
65. Baszczuk A, Thielemann A, Musialik K, Kopczynski J, Bielawska L, Dzumak A, et al. The impact of supplementation with folic acid on homocysteine concentration and selected lipoprotein parameters in patients with primary hypertension. J Nutr Sci Vitaminol (Tokyo). (2017) 63:96–103. doi: 10.3177/jnsv.63.96
66. Vijayakumar A, Kim EK, Kim H, Choi YJ, Huh KB, Chang N. Effects of folic acid supplementation on serum homocysteine levels, lipid profiles, and vascular parameters in post-menopausal Korean women with type 2 diabetes mellitus. Nutr Res Pract. (2017) 11:327–33. doi: 10.4162/nrp.2017.11.4.327
67. Asbaghi O, Ashtary-Larky D, Bagheri R, Nazarian B, Pourmirzaei Olyaei H, Rezaei Kelishadi M, et al. Beneficial effects of folic acid supplementation on lipid markers in adults: a GRADE-assessed systematic review and dose-response meta-analysis of data from 21,787 participants in 34 randomized controlled trials. Crit Rev Food Sci Nutr. (2021):1–19. doi: 10.1080/10408398.2021.1928598
68. Cui S, Li W, Lv X, Wang P, Huang G, Gao Y. Folic acid attenuates homocysteine and enhances antioxidative capacity in atherosclerotic rats. Appl Physiol Nutr Metab. (2017) 42:1015–22. doi: 10.1139/apnm-2017-0158
69. Sid V, Shang Y, Siow YL, Hewage SM, House JD, Karmin O. Folic acid supplementation attenuates chronic hepatic inflammation in high-fat diet fed mice. Lipids. (2018) 53:709–16. doi: 10.1002/lipd.12084
Keywords: homocysteine, high-density lipoprotein cholesterol, apolipoprotein A1, triglyceride, remnant cholesterol, Chinese adults
Citation: Zhou L, Liu J, An Y, Wang Y and Wang G (2022) Plasma Homocysteine Level Is Independently Associated With Conventional Atherogenic Lipid Profile and Remnant Cholesterol in Adults. Front. Cardiovasc. Med. 9:898305. doi: 10.3389/fcvm.2022.898305
Received: 17 March 2022; Accepted: 10 May 2022;
Published: 13 June 2022.
Edited by:
Wei Li, Marshall University, United StatesReviewed by:
Hilda E. Ghadieh, American University of Beirut, LebanonJosep Julve, Institut de Recerca de l’Hospital de la Santa Creu i Sant Pau, Spain
Copyright © 2022 Zhou, Liu, An, Wang and Wang. This is an open-access article distributed under the terms of the Creative Commons Attribution License (CC BY). The use, distribution or reproduction in other forums is permitted, provided the original author(s) and the copyright owner(s) are credited and that the original publication in this journal is cited, in accordance with accepted academic practice. No use, distribution or reproduction is permitted which does not comply with these terms.
*Correspondence: Ying Wang, hdwangying0517@163.com; Guang Wang, wangguang@bjcyh.com
†These authors have contributed equally to this work