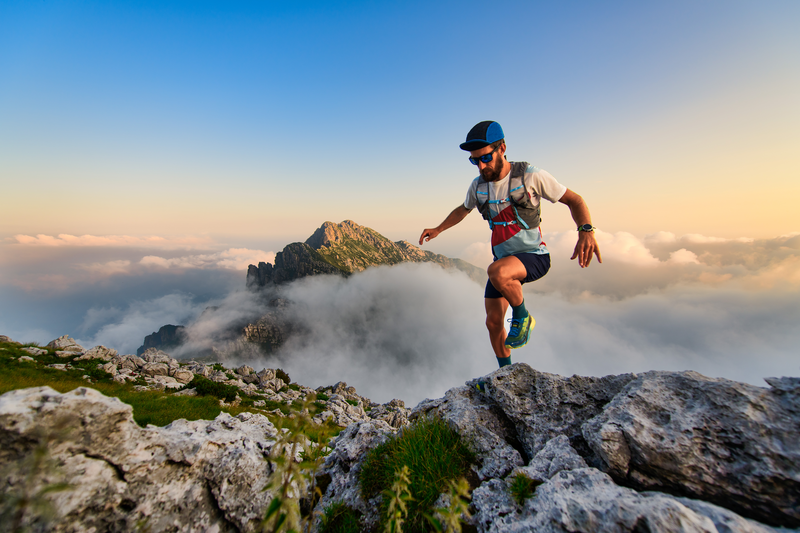
94% of researchers rate our articles as excellent or good
Learn more about the work of our research integrity team to safeguard the quality of each article we publish.
Find out more
ORIGINAL RESEARCH article
Front. Cardiovasc. Med. , 14 June 2022
Sec. Hypertension
Volume 9 - 2022 | https://doi.org/10.3389/fcvm.2022.896564
Background: Liddle syndrome is a form of monogenic hypertension caused by mutations in the three homologous subunits of the epithelial sodium channels (ENaCs), α, β, and γ. It is characterized by early-onset refractory hypertension, hypokalemia, low renin activity, and hypoaldosteronism. In this study, we report a novel frame-shift mutation in SCNN1B responsible for Liddle syndrome in a Chinese family.
Methods: DNA samples were collected from all participants. Whole-exome sequencing was performed in the proband to detect possible causative variants. Sanger sequencing was then conducted in the other family members to verify the candidate variant, and in 100 patients with hypertension and 100 normotensive controls to exclude population genetic polymorphism.
Results: We identified a novel frame-shift mutation (c.1691_1693delinsG) in SCNN1B that was responsible for Liddle syndrome in this family. This mutation leads to the substitution of Arg in place of Gln at codon site 564 and generates a new stop codon at 592, influencing the crucial PY motif and resulting in reduced inactivation of the ENaCs. Aside from the proband, eight family members carried the mutation. Intra-familial phenotypic heterogeneity was observed in the blood pressure and serum potassium levels. Amiloride therapy combined with a low sodium diet is effective to alleviate the symptoms of patients with Liddle syndrome.
Conclusion: c.1691_1693delinsG, a novel frame-shift mutation in the β subunit of ENaC, was identified in a Chinese family with Liddle syndrome by whole-exome sequencing. Phenotypic heterogeneity can make diagnosis of Liddle syndrome difficult on the basis of clinical or biochemical characteristics alone. Genetic analysis is a useful tool allowing timely and accurate diagnosis of Liddle syndrome and playing a guiding role in precise treatment of the disease.
Systemic hypertension is a commonly recognized health problem affecting over 1.2 billion adults worldwide (1). Chronic elevated blood pressure or hypertensive emergencies can lead to morbid complications such as stroke, heart failure, and renal failure (2). Significantly, 15–20% of cases of hypertension occur secondary to some definable causes, and for those, precise determination of the etiology could help to avoid severe cardiovascular outcomes (3). Monogenic hypertension plays an important role in the development of secondary hypertension, such as Liddle syndrome, apparent mineralocorticoid excess, and congenital adrenal hyperplasia (4).
Liddle syndrome is an autosomal dominant form of hereditary hypertension with early penetrance caused by mutations in the epithelial sodium channels (ENaCs). ENaCs, which are responsible for the rate-limiting step of water and sodium reabsorption in the aldosterone-sensitive-distal nephron, consist of α, β, and γ homologous subunits, encoded by SCNN1A, SCNN1B, and SCNN1G, respectively (5). Any mutation in the subunits can induce the constitutive activation of ENaCs by either accumulative channel density or an increased probability of the channels being open, thereby resulting in excessive sodium reabsorption and volume expansion (6, 7). The disease is classically characterized by severe hypertension, hypokalemia, and metabolic alkalosis in the setting of low/suppressed aldosterone and renin levels (8). However, a variable phenotype of Liddle syndrome is observed in clinical work. Some patients are misdiagnosed and may suffer catastrophic complications because of a lack of awareness of the genotype-phenotype heterogeneity (9). Genetic sequencing, considered a gold standard, is useful and effective for the diagnosis of Liddle syndrome. The blood pressure and potassium levels can be improved greatly by adopting a low potassium diet and tailored therapy including amiloride or triamterene, which are specific antagonists of ENaCs, but patients show no response to spironolactone treatment.
In the present study, we report a Chinese family with Liddle syndrome and identify a novel frame-shift mutation (c.1691_1693delinsG) in SCNN1B by whole-exome sequencing. Sanger sequencing indicated that eight family members also carried the variant in addition to the proband. Intra-familial phenotypic heterogeneity was observed in terms of the blood pressure and serum potassium levels. Tailored therapy is beneficial and cost-effective for Liddle syndrome patients to avoid catastrophic complications.
The proband was an 18-year-old male patient referred to the Department of Cardiology at Fuwai Hospital (Beijing, China) to identify the etiology of his refractory hypertension and hypokalemia. A positive family history of early-onset hypertension was found in his family. In addition to the proband, 11 family relatives participated in this study (Figure 1). Furthermore, to exclude population genetic polymorphism, 100 patients with hypertension and 100 normotensive controls were enrolled into this study, of which the samples’ statistical power was fully assessed by online tool SSizer (10).
Figure 1. Pedigree of the Chinese family with Liddle syndrome. The arrow indicates the proband; black symbols represent Liddle syndrome patients identified by genetic testing; and gray symbols indicate member without genetic testing.
Imaging examinations, including echocardiography, and abdominal CT of the kidneys, adrenal glands, and renal arteries, were performed on the proband. Laboratory examinations, comprising electrolyte levels, urinary catecholamines, plasma renin activity (PRA), and plasma aldosterone concentration (PAC), were also assessed during the proband’s hospitalization.
We collected venous blood samples from all participants and genomic DNA was extracted using the QIAamp DNA Blood Mini kit (QIAGEN, Hilden, Germany) for genetic testing. Whole-exome sequencing was performed in the proband. After assessing the DNA quality, exomes were captured by Agilent SureSelect Human All Exon V6 kits based on standard protocols and then sequenced using the Illumina HiSeq2000 platform. The raw data were filtered, removing the sequence adaptors and low-quality reads (defined as those with more than 10% undetermined base information in single-end sequencing reads, or low-quality bases in single-end sequencing reads exceeding 50% of the length). Then, the clean reads were mapped to the reference genome (GRCh37/hg19) by Burrows-Wheeler Aligner (beta version1), and the Sambamba tool (11) (version: 0.8.22) was used to mark duplicate reads. We used the SAM tool (12, 13) (version: 0.1.193) to identify single nucleotide variants (SNVs)/indels, and the CoNIFER tool (version: 0.34) to detect copy number variation. ANNOVAR software (14) (subversion: 3225) was used to annotate filtered variants. In silico analysis software, including Polyphen2 (15) (version: 2.2.3), MutationTaster2021 (16), and SIFT,6 were used to predict the pathogenicity of variants.
Furthermore, we used Sanger sequencing in other family members to verify the candidate variant. To exclude population genetic polymorphism, we also performed Sanger sequencing on 100 hypertensives and 100 normotensive controls. Polymerase chain reaction (PCR) was used to amplify exon 13 of SCNN1B for further familial co-segregation analysis (forward primer, 5′-CCCACCCAAGAATCACCTCC-3′; reverse primer, 5′-TCAGGACAGGTAGGGACGAG-3′) and the results were sequenced by ABI Prism 377 DNA sequencer (Applied Biosystems, Foster City, CA, United States).
This study was approved by the Ethics Committee of Fuwai Hospital and was performed in accordance with the Declaration of Helsinki. Informed consent was obtained from every participant.
The proband (IV-3) was diagnosed with hypertension (180/110 mm Hg) at the age of 17 when he went to the hospital complaining of abdominal pain. Since then, he has been treated with anti-hypertensive therapy. However, despite taking amlodipine, spironolactone, and irbesartan hydrochlorothiazide, his blood pressure status was still poor (170/100 mm Hg) and his potassium level was low, fluctuating from 2.38 to 3.45 mmol/L. To identify the etiology of the refractory hypertension with hypokalemia, he was admitted to Fuwai Hospital. Physical examination of the proband, including height, weight, and sexual development, was unremarkable. Biochemical examination indicated hypokalemia (2.67 mmol/L; reference value, 3.5–5.3 mmol/L), low PRA (1.0 μIU/mL; reference value, 2.8–39.9 μIU/mL), low PAC (1.0 ng/dL; reference value, 3.0–23.6 ng/dL), and metabolic alkalosis (HCO3–, 28.6 mmol/L; reference value, 21.0–27.0 mmol/L; Actual Base Excess, 4.3 mmol/L; reference value, −3.0 to 3.0 mmol/L). Urinary catecholamines and gonadal hormones were within the normal range (Table 1). Microalbuminuria was discovered, and echocardiography showed thickening of the ventricular septum. Results of abdominal CT of the kidneys, adrenal glands, and renal arteries were all normal.
The proband’s mother (III-4) was diagnosed with hypertension (160/110 mm Hg) at the age of 24 years when she was pregnant. After delivery, her blood pressure slightly decreased, reaching 150/98 mm Hg. His grandfather (II-1) suffered from hypertension when he was 21 years old and died of esophageal cancer at the age of 52 years. In addition, his great-uncle (II-3), maternal uncle (III-1), and elder aunt (III-5) presented with severe early-onset hypertension, and the former (II-3) suffered from a stroke at the age of 60 years old. The proband’s father (III-3), grandmother (II-2), and younger aunt (III-6) were normotensive. Detailed clinical data of family members are listed in Table 2. On the basis of the combination of laboratory results, lack of response to spironolactone and the positive family history, monogenic hypertension was highly suspected for the proband.
We found a novel heterozygous frame-shift mutation (c.1691_1693delinsG) in the SCNN1B gene, which was not detected in the gnomAD database, the 1000 Genomes Project database, or the Human Gene Mutation Database. The mutation leads to a substitution of Arg in place of Gln at codon 564 and generates a new stop codon at 592, which influences the PY motif. In silico analysis, including MutationTaster2021 suggested that the variant was pathogenic. Sanger sequencing results indicated that there were eight additional family members affected with the causative mutation (Figure 2), but it was not found in any of the 100 hypertension patients or the 100 normotensive controls.
Figure 2. Sequence analysis result of exon 13 of SCNN1B. (A) The frame-shift mutation (c.1691_1693delinsG) identified in affected patients with Liddle syndrome; (B) corresponding normal sequence in unaffected subjects; (C) predicted alteration of the protein sequence due to the mutant SCNN1B.
All individuals carrying the causative mutation were advised to switch to a healthier lifestyle, including a low sodium diet and more exercise. Additionally, every patient was prescribed a Amiloride/Hydrochlorothiazide combination, each tablet of which contained amiloride (2.5 mg) and hydrochlorothiazide (25 mg), except for IV-1 and IV-2 whose mother refused to accept medicine for them. The patients took one tablet per day, except for the proband and his maternal uncle, who took a double dose of the amiloride because they were overweight. After a 3-month follow-up, the blood pressure and potassium levels improved significantly (Table 2).
In this report, we identified a novel frame-shift mutation (c.1691_1693delinsG) in the SCNN1B gene in a Chinese family. Family genetic screening indicated that a total of nine family members were carrying the pathogenic mutation. Intra-familial phenotypic heterogeneity was prominent in this case. Namely, the proband presented with classical Liddle syndrome symptoms, while some members with the same mutation had only mild hypertension and hypokalemia, or even normotension and normokalemia. Tailored therapy, including amiloride and a low sodium diet, play an important role in controlling the disease. Timely genetic testing aids in accurate diagnosis of Liddle syndrome.
In 1963, Liddle syndrome was first described by Liddle et al. in a Caucasian female who presented with hypertension, hypokalemia, and metabolic alkalosis in the absence of hyperaldosteronism (17). In 1994, Shimkets et al. first uncovered the molecular defect of Liddle syndrome by analyzing the β subunit of ENaC in several Liddle syndrome kindreds (18). The disorder results from gain-of-function mutations of SCNN1A, SCNN1B, and SCNN1G, encoding the α, β, and γ subunits of ENaCs, respectively. In the kidney, ENaCs are mainly distributed in the apical membrane of the principal cells located in the aldosterone-sensitive-distal nephron, which play a vital role in maintaining sodium and water reabsorption. Each subunit of ENaC contains a proline-rich segment (PY motif), which is located in the C-terminus and functions by binding the WW domains of the ubiquitin ligase protein Nedd4–2, thereby inducing internalization and proteasomal degradation of the ENaCs. Any deletion or alteration of the PY motif leads to the reduced inactivation of the ENaCs, resulting in an increased density and constitutive activation of ENaCs and consequently followed by excessive sodium reabsorption and plasma volume expansion (19). Additionally, increased sodium reabsorption generates a luminal negative voltage that promotes an elevated secretion level of potassium, leading to abnormal hypokalemia (20).
The worldwide prevalence of Liddle syndrome, irrespective of race or sex, remains unknown. So far, there have been relatively few studies on the prevalence of Liddle syndrome. In 2010, Tapolyai et al. conducted a cross-sectional cohort of 149 patients characterized by hypertension and hypokalemia or metabolic alkalosis and detected a high prevalence (6%, 9/149) of Liddle syndrome biochemical phenotypes (21). In a Chinese early-onset hypertensive population, the prevalence of Liddle syndrome confirmed by genetic testing has been estimated as 1.52% (5/330) and 0.91% (7/766) (22, 23). However, because of the variable penetrance of Liddle syndrome and the incomplete application of genetic testing, the prevalence may be underestimated.
Until now, more than 24 mutations in SCNN1B have been reported worldwide, with the most common variants altering the conserved PY motif in the carboxy-terminal cytoplasmic tail of ENaC. The mutation in this report (c.1691_1693delinsG), which is also near the C-terminus, results in the substitution of an Arg amino acid in place of a Gln at position 564 and a truncated open reading frame that ends at position 592. To date, several mutations have been described that are close to the locus reported herein, including missense and nonsense mutations. R563Q has been identified as related to a hypertensive phenotype, and might alter the three-dimensional structure of the β subunit, resulting in weakened intracellular interactions and upregulated ENaC activity (24, 25). Liu et al. identified a nonsense mutation in SCNN1B gene (c.C1690T) which introduces a new stop codon instead of a Gln at codon 564 (23). In addition, R566X is considered a hot-spot locus which leads to the removal of the cytoplasmic carboxyl tail (18, 22, 23, 26–31). Previously, Cui et al. screened SCNN1B and SCNN1G of 12 patients with Liddle syndrome and detected transition from C to T at various sites of SCNN1B (c.C1690T, c.C1702T), which leads to premature termination of the protein (28). In renal epithelial cells derived from the distal nephron, Snyder et al. found that βR566X coexpressed with wild-type α and γ subunits generated more than twice as much amiloride-sensitive current as did expression of wild-type αβγ hENaC, indicating that truncation of βhENaC led to increased Na+ current (32). A quantitative assay was developed by Firsov et al. (7). This method was based on the binding of a monoclonal antibody directed against a FLAG reporter epitope introduced in the α, β, and γ subunits of ENaC, and demonstrated a significant correlation between the macroscopic amiloride-sensitive sodium current (INa) with the number of ENaC on the plasma membrane (7). Schild et al. investigated sequential deletions in the C-terminus of the βENaC, and reported that a functional domain, – PY motif regulated channel activity (33). Staub et al. found that mutations in the PY motif of βENaC abolished Nedd4-WW binding in the two-hybrid binding assay, and the protein-protein interaction of ENaC with Nedd4 mediated internalization of ENaC (34).
The effect of a frame-shift mutation of SCNN1B has been verified in a previous expression study in Xenopus laevis oocytes, which is caused by insertion of a cytosine at the codon 594 and truncates the C-terminus at codon 607, disrupting 46 amino acids of βhENaC (32). Snyder et al. introduced serial stop codons into βhENaC and illustrated that deletion of 19 residues was sufficient to reproduce the effect of disease-associated mutations (32). Their data identified that truncation in the C-terminus of the β subunit deleted the conserved PY motif, and induced an increase of transmembranic sodium transport, compared with expression of the wild-type αβγENaC (32). The deletion of the PY motif leads to constitutive activation of the ENaC, and results in Liddle syndrome.
According to previous studies and in silico prediction softwares, the frame-shift mutation identified in this report also leads to the absence of the PY motif, by generating a new stop codon at 592 (original stop codon at 641). As a result, the inactivation of the ENaCs is reduced, consequently followed by increased number of ENaC on the plasma membrane and excessive sodium reabsorption. Increased sodium reabsorption leads to plasma volume expansion and promotes secretion of potassium, causing refractory hypertension with hypokalemia of the proband.
Phenotypic variability is common in the clinical and biochemical characteristics of Liddle syndrome, and this was also observed in this study (35, 36). The blood pressure level, degree of hypokalemia, therapeutic effect of amiloride, and targeted organ damage were the key features in the heterogeneity analysis (22, 27). Previously, genotype–phenotype correlation analysis has been conducted in two Liddle syndrome families with various mutations by Gong et al., which revealed the existence of inter- and intra-familial phenotypic variability (27). Even patients in the same family carrying the same pathogenic mutation may present with mild hypertension or even normotension (22). In terms of the serum potassium condition, hypokalemia was observed in 71.8% of Liddle syndrome patients, and 78% of pediatric Liddle syndrome patients (37, 38). In this study, subjects IV-3 and III-1 presented typically with early-onset severe hypertension, whereas subjects III-7 and III-5 showed mild hypertension, and subjects IV-1 and IV-2 had normal blood pressure. Moreover, the incidence rate of hypokalemia in this family was slightly lower than that previously reported, around 66.7%, which may be due to the limited patient number in the pedigree.
Because some Liddle syndrome patients harbor atypical clinical symptoms and biochemical characteristics, they may be easily misdiagnosed with primary aldosteronism or primary hypertension (23, 39). Long-term uncontrolled blood pressure often results in severe disease complications and targeted organ damage, such as heart failure, renal failure, or even sudden death. To avoid these, clinicians should consider Liddle syndrome when they encounter patients presenting with early-onset hypertension, suppressed PRA, low renin level, and hypokalemia. Genetic testing is considered an essential and rapid tool to ensure that patients with Liddle syndrome can be diagnosed in an accurate and timely fashion. In addition, genetic screening of high-risk family members based on the proband is necessary and efficient for detecting potential Liddle syndrome patients. Once diagnosed, ENaC inhibitors (such as amiloride and triamterene) in combination with a low sodium diet should be individualized. Liu et al. reported that an average follow-up study for 4 years of 17 Liddle syndrome patients showed that blood pressure and potassium levels can be controlled well by the adoption of amiloride therapy (23).
In conclusion, we have identified a novel frame-shift mutation in the β subunit of ENaC in a Chinese family with Liddle syndrome by whole-exome sequencing, further refining the known genetic mutations of SCNN1B. Patients with Liddle syndrome may be misdiagnosed when diagnosis is based on clinical or biochemical characteristics alone because of the existence of phenotypic heterogeneity. Genetic analysis is necessary to ensure timely and accurate diagnosis of Liddle syndrome and to apply tailored treatment to prevent the occurrence of disease complications.
The data presented in the study are deposited in the Sequence Read Archive (SRA) repository, accession number SAMN2886425.
The studies involving human participants were reviewed and approved by the Ethics Committee of Fuwai Hospital. Written informed consent to participate in this study was provided by the participants’ legal guardian/next of kin. Written informed consent was obtained from the individual(s), and minor(s)’ legal guardian/next of kin, for the publication of any potentially identifiable images or data included in this article.
Y-TL, X-CL, FL, and X-LZ designed the study and modified the manuscript. Y-TL, X-CL, Z-MZ, DZ, and LS collected the clinical information and performed the data analysis. YZ, PF, LZ, and Y-XL performed the experiments. Y-TL and X-CL wrote the manuscript. All authors reviewed this work.
This work was supported by the National Key Research and Development Program of China (2016YFC1300100) and the Non-profit Central Research Institute Fund of Chinese Academy of Medical Sciences (2019XK320057 and 2019XK320058).
The authors declare that the research was conducted in the absence of any commercial or financial relationships that could be construed as a potential conflict of interest.
All claims expressed in this article are solely those of the authors and do not necessarily represent those of their affiliated organizations, or those of the publisher, the editors and the reviewers. Any product that may be evaluated in this article, or claim that may be made by its manufacturer, is not guaranteed or endorsed by the publisher.
We thank all participants for taking part in this study and agencies that aided the efforts of the authors.
1. Rossier BC, Bochud M, Devuyst O. The hypertension pandemic: an evolutionary perspective. Physiology (Bethesda). (2017) 32:112–25. doi: 10.1152/physiol.00026.2016
2. Marik PE, Rivera R. Hypertensive emergencies: an update. Curr Opin Crit Care. (2011) 17:569–80. doi: 10.1097/MCC.0b013e32834cd31d
3. Tziomalos K. Secondary hypertension: novel insights. Curr Hypertens Rev. (2020) 16:11. doi: 10.2174/1573402115666190416161116
4. Raina R, Krishnappa V, Das A, Amin H, Radhakrishnan Y, Nair NR, et al. Overview of monogenic or mendelian forms of hypertension. Front Pediatr. (2019) 7:263. doi: 10.3389/fped.2019.00263
5. Alvarez de la Rosa D, Navarro-Gonzalez JF, Giraldez T. ENaC modulators and renal disease. Curr Mol Pharmacol. (2013) 6:35–43. doi: 10.2174/1874467211306010005
6. Salih M, Gautschi I, van Bemmelen MX, Di Benedetto M, Brooks AS, Lugtenberg D, et al. A missense mutation in the extracellular domain of alphaENaC causes liddle syndrome. J Am Soc Nephrol. (2017) 28:3291–9. doi: 10.1681/ASN.2016111163
7. Firsov D, Schild L, Gautschi I, Mérillat AM, Schneeberger E, Rossier BC. Cell surface expression of the epithelial Na channel and a mutant causing Liddle syndrome: a quantitative approach. Proc Natl Acad Sci U S A. (1996) 93:15370–5. doi: 10.1073/pnas.93.26.15370
8. Warnock DG. Liddle syndrome: an autosomal dominant form of human hypertension. Kidney Int. (1998) 53:18–24. doi: 10.1046/j.1523-1755.1998.00728.x
9. Jin Y, Qiu W, Yao J. Liddle syndrome: a case report. Med Clin (Barc). (2021): [Online ahead of print], doi: 10.1016/j.medcli.2021.03.035
10. Li F, Zhou Y, Zhang X, Tang J, Yang Q, Zhang Y, et al. SSizer: determining the sample sufficiency for comparative biological study. J Mol Biol. (2020) 432:3411–21. doi: 10.1016/j.jmb.2020.01.027
11. Tarasov A, Vilella AJ, Cuppen E, Nijman IJ, Prins P. Sambamba: fast processing of NGS alignment formats. Bioinformatics (Oxford, England). (2015) 31:2032–4. doi: 10.1093/bioinformatics/btv098
12. Li H, Handsaker B, Wysoker A, Fennell T, Ruan J, Homer N, et al. The Sequence Alignment/Map format and SAMtools. Bioinformatics (Oxford, England). (2009) 25:2078–9. doi: 10.1093/bioinformatics/btp352
13. Li H. A statistical framework for SNP calling, mutation discovery, association mapping and population genetical parameter estimation from sequencing data. Bioinformatics (Oxford, England). (2011) 27:2987–93. doi: 10.1093/bioinformatics/btr509
14. Wang K, Li M, Hakonarson H. ANNOVAR: functional annotation of genetic variants from high-throughput sequencing data. Nucleic Acids Res. (2010) 38:e164. doi: 10.1093/nar/gkq603
15. Adzhubei I, Jordan DM, Sunyaev SR. Predicting functional effect of human missense mutations using PolyPhen-2. Curr Protoc Hum Genet. (2013) Chapter 7:Unit7.20. doi: 10.1002/0471142905.hg0720s76
16. Steinhaus R, Proft S, Schuelke M, Cooper DN, Schwarz JM, Seelow D. MutationTaster2021. Nucleic Acids Res. (2021) 49:W446–51. doi: 10.1093/nar/gkab266
17. Liddle GW. A familial renal disorder simulating primary aldosteronism but with negligible aldosterone secretion. Trans Assoc Am Phys. (1963) 76: 199–213.
18. Shimkets RA, Warnock DG, Bositis CM, Nelson-Williams C, Hansson JH, Schambelan M, et al. Liddle’s syndrome: heritable human hypertension caused by mutations in the beta subunit of the epithelial sodium channel. Cell. (1994) 79:407–14. doi: 10.1016/0092-8674(94)90250-x
19. Zennaro MC, Boulkroun S, Fernandes-Rosa F. Inherited forms of mineralocorticoid hypertension. Best Pract Res Clin Endocrinol Metab. (2015) 29:633–45. doi: 10.1016/j.beem.2015.04.010
20. Rossier BC, Staub O, Hummler E. Genetic dissection of sodium and potassium transport along the aldosterone-sensitive distal nephron: importance in the control of blood pressure and hypertension. FEBS Lett. (2013) 587:1929–41. doi: 10.1016/j.febslet.2013.05.013
21. Tapolyai M, Uysal A, Dossabhoy NR, Zsom L, Szarvas T, Lengvarszky Z, et al. High prevalence of liddle syndrome phenotype among hypertensive US Veterans in Northwest Louisiana. J Clin Hypertens (Greenwich). (2010) 12:856–60. doi: 10.1111/j.1751-7176.2010.00359.x
22. Wang LP, Yang KQ, Jiang XJ, Wu HY, Zhang HM, Zou YB, et al. Prevalence of liddle syndrome among young hypertension patients of undetermined cause in a Chinese Population. J Clin Hypertens (Greenwich). (2015) 17:902–7. doi: 10.1111/jch.12598
23. Liu K, Qin F, Sun X, Zhang Y, Wang J, Wu Y, et al. Analysis of the genes involved in Mendelian forms of low-renin hypertension in Chinese early-onset hypertensive patients. J Hypertens. (2018) 36:502–9. doi: 10.1097/HJH.0000000000001556
24. Rayner BL, Owen EP, King JA, Soule SG, Vreede H, Opie LH, et al. A new mutation, R563Q, of the beta subunit of the epithelial sodium channel associated with low-renin, low-aldosterone hypertension. J Hypertens. (2003) 21:921–6. doi: 10.1097/00004872-200305000-00016
25. Jones ES, Owen EP, Davidson JS, Van Der Merwe L, Rayner BL. The R563Q mutation of the epithelial sodium channel beta-subunit is associated with hypertension. Cardiovasc J Afr. (2011) 22:241–4. doi: 10.5830/CVJA-2010-084
26. Shi JY, Chen X, Ren Y, Long Y, Tian HM. [Liddle’s syndrome caused by a novel mutation of the gamma-subunit of epithelial sodium channel gene SCNN1G in Chinese]. Zhonghua Yi Xue Yi Chuan Xue Za Zhi. (2010) 27:132–5. doi: 10.3760/cma.j.issn.1003-9406.2010.02.003
27. Gong L, Chen J, Shao L, Song W, Hui R, Wang Y. Phenotype-genotype analysis in two Chinese families with Liddle syndrome. Mol Biol Rep. (2014) 41:1569–75. doi: 10.1007/s11033-013-3003-7
28. Cui Y, Tong A, Jiang J, Wang F, Li C. Liddle syndrome: clinical and genetic profiles. J Clin Hypertens (Greenwich). (2017) 19:524–9. doi: 10.1111/jch.12949
29. Polfus LM, Boerwinkle E, Gibbs RA, Metcalf G, Muzny D, Veeraraghavan N, et al. Whole-exome sequencing reveals an inherited R566X mutation of the epithelial sodium channel β-subunit in a case of early-onset phenotype of Liddle syndrome. Cold Spring Harb Mol Case Stud. (2016) 2:a001255. doi: 10.1101/mcs.a001255
30. Melander O, Orho M, Fagerudd J, Bengtsson K, Groop PH, Mattiasson I, et al. Mutations and variants of the epithelial sodium channel gene in Liddle’s syndrome and primary hypertension. Hypertension. (1998) 31:1118–24. doi: 10.1161/01.hyp.31.5.1118
31. Kyuma M, Ura N, Torii T, Takeuchi H, Takizawa H, Kitamura K, et al. A family with liddle’s syndrome caused by a mutation in the beta subunit of the epithelial sodium channel. Clin Exp Hypertens. (2001) 23:471–8. doi: 10.1081/ceh-100104238
32. Snyder PM, Price MP, McDonald FJ, Adams CM, Volk KA, Zeiher BG, et al. Mechanism by which Liddle’s syndrome mutations increase activity of a human epithelial Na+ channel. Cell. (1995) 83:969–78. doi: 10.1016/0092-8674(95)90212-0
33. Schild L, Lu Y, Gautschi I, Schneeberger E, Lifton RP, Rossier BC. Identification of a PY motif in the epithelial Na channel subunits as a target sequence for mutations causing channel activation found in Liddle syndrome. EMBO J. (1996) 15:2381–7.
34. Staub O, Dho S, Henry P, Correa J, Ishikawa T, McGlade J, et al. WW domains of Nedd4 bind to the proline-rich PY motifs in the epithelial Na+ channel deleted in Liddle’s syndrome. EMBO J. (1996) 15:2371–80.
35. Fan P, Lu CX, Zhang D, Yang KQ, Lu PP, Zhang Y, et al. Liddle syndrome misdiagnosed as primary aldosteronism resulting from a novel frameshift mutation of SCNN1B. Endocr Connect. (2018) 7:1528–34. doi: 10.1530/EC-18-0484
36. Ding X, Jia N, Zhao C, Zhong Y, Dai D, Zhao Y, et al. A family with Liddle’s syndrome caused by a new c.1721 deletion mutation in the epithelial sodium channel β-subunit. Exp Ther Med. (2019) 17:2777–84. doi: 10.3892/etm.2019.7270
37. Tetti M, Monticone S, Burrello J, Matarazzo P, Veglio F, Pasini B, et al. Liddle syndrome: review of the literature and description of a new case. Int J Mol Sci. (2018) 19:812. doi: 10.3390/ijms19030812
38. Fan P, Pan XC, Zhang D, Yang KQ, Zhang Y, Tian T, et al. Pediatric liddle syndrome caused by a novel SCNN1G variant in a Chinese family and characterized by early-onset hypertension. Am J Hypertens. (2020) 33:670–5. doi: 10.1093/ajh/hpaa037
Keywords: Liddle syndrome, frame-shift mutation, SCNN1B, monogenic hypertension, genetic testing
Citation: Lu Y-T, Liu X-C, Zhou Z-M, Zhang D, Sun L, Zhang Y, Fan P, Zhang L, Liu Y-X, Luo F and Zhou X-L (2022) A Novel Frame-Shift Mutation in SCNN1B Identified in a Chinese Family Characterized by Early-Onset Hypertension. Front. Cardiovasc. Med. 9:896564. doi: 10.3389/fcvm.2022.896564
Received: 15 March 2022; Accepted: 17 May 2022;
Published: 14 June 2022.
Edited by:
Chao-Ling Yang, Oregon Health & Science University, United StatesReviewed by:
Kerim Mutig, Charité – Universitätsmedizin Berlin, GermanyCopyright © 2022 Lu, Liu, Zhou, Zhang, Sun, Zhang, Fan, Zhang, Liu, Luo and Zhou. This is an open-access article distributed under the terms of the Creative Commons Attribution License (CC BY). The use, distribution or reproduction in other forums is permitted, provided the original author(s) and the copyright owner(s) are credited and that the original publication in this journal is cited, in accordance with accepted academic practice. No use, distribution or reproduction is permitted which does not comply with these terms.
*Correspondence: Fang Luo, bHVvZmFuZ0BmdXdhaWhvc3BpdGFsLm9yZw==; Xian-Liang Zhou, emhvdXhpYW5saWFuZzAzMjZAaG90bWFpbC5jb20=
†These authors have contributed equally to this work and share first authorship
Disclaimer: All claims expressed in this article are solely those of the authors and do not necessarily represent those of their affiliated organizations, or those of the publisher, the editors and the reviewers. Any product that may be evaluated in this article or claim that may be made by its manufacturer is not guaranteed or endorsed by the publisher.
Research integrity at Frontiers
Learn more about the work of our research integrity team to safeguard the quality of each article we publish.