- 1Department of Medicine, University of Connecticut School of Medicine, Farmington, CT, United States
- 2Department of Medicine, Calhoun Cardiology Center, University of Connecticut School of Medicine, Farmington, CT, United States
Patients with cancer are now living longer than ever before due to the growth and expansion of highly effective antineoplastic therapies. Many of these patients face additional health challenges, of which cardiovascular disease (CVD) is the leading contributor to morbidity and mortality. CVD and cancer share common biological mechanisms and risk factors, including lipid abnormalities. A better understanding of the relationship between lipid metabolism and cancer can reveal strategies for cancer prevention and CVD risk reduction. Several anticancer treatments adversely affect lipid levels, increasing triglycerides and/or LDL-cholesterol. The traditional CVD risk assessment tools do not include cancer-specific parameters and may underestimate the true long-term CVD risk in this patient population. Statins are the mainstay of therapy in both primary and secondary CVD prevention. The role of non-statin therapies, including ezetimibe, PCSK9 inhibitors, bempedoic acid and icosapent ethyl in the management of lipid disorders in patients with cancer remains largely unknown. A contemporary cancer patient needs a personalized comprehensive cardiovascular assessment, management of lipid abnormalities, and prevention of late CVD to achieve optimal overall outcomes.
Introduction
The development of highly effective anticancer therapies over the past few decades has favorably changed the landscape of patients with cancer, who can now achieve high cure rates in early stages of disease and long-term remission in others (1). This oncologic progress, however, has generated a unique patient population who are at a high risk of experiencing a myriad of chronic comorbidities, among which CVD is one of the most important (2). Cancer and CVD share several common risk factors, including advanced age, chronic inflammation, obesity, hyperlipidemia, poor diet, smoking history, and physical inactivity (3, 4). A multi-disciplinary team comprising of primary care, oncology, pharmacy, and cardio-oncology is best poised to serve this special cohort of patients who often pose challenging diagnostic and management dilemmas (5).
Dyslipidemia has been associated with poor outcomes in patients with cancer by promoting tumor invasion and metastasis (6), producing resistance to cancer drugs (7), and enhancing the cardiac and vascular toxicity of anticancer therapies (8). In this review, we discuss the emerging literature on the relationship between lipid abnormalities and carcinogenesis, review anticancer treatment-associated hyperlipidemia, discuss CVD risk assessment and risk reduction in patients with cancer, and highlight the current evidence to support the use of antilipidemic agents in this special patient population.
Hyperlipidemia, Metabolic Syndrome, and Cancer
It is well known that dyslipidemia is a strong predictor of CVD (9, 10). Emerging data suggest that hyperlipidemia may also play a role in carcinogenesis (11). Tumor cells have been shown to require large amounts of sterol metabolites to sustain rapid growth and proliferation (12, 13). A key regulatory transcriptional factor in lipid synthesis and uptake, sterol regulatory element-binding protein (SREBP), has been identified to be dysregulated in various cancer types to accelerate endogenous cholesterol and fatty acid production (14, 15). Another mechanism reported in prostate cancer is reduced cholesterol efflux through ABCA1 (ATP-binding cassette class A) transporters (16). Additional pathways connecting cholesterol and cancer are phosphatidylinositol 3-kinase (PI3-K)/Akt pathways that are part of hedgehog signaling, which when dysregulated can lead to abnormal cell proliferation and tumor growth (11).
Higher levels of saturated and monounsaturated phospholipids in cell membranes have been shown to protect cancer cells from oxidative damage (17). Lipids also serve an important role in cell signaling and migration, as well as post-translational modification of proteins (18, 19). Angiogenesis, a hallmark of cancer, occurs through the secretion of prostaglandin E2, a sterol compound in breast cancer cells (18, 20). All of these functions highlight the importance of lipids in oncogenesis and tumor spread.
Hyperlipidemia is a common comorbidity among cancer patients and survivors. Ray and Husain demonstrated that patients with breast cancer had significant elevations in plasma total cholesterol (TC), low density lipoprotein (LDL)-cholesterol, and triglyceride (TG) levels (21). Shah et al. reported similar findings in patients with breast cancer when compared to patients with benign breast disease (22). In a large cross-sectional study, there was a significant difference in the lipid profiles among different types of cancers (23). Patients with ovarian cancer were observed to have the highest serum TG levels, while those with colorectal cancer had the lowest TG (23). Breast cancer patients had the highest TC and LDL levels, while gastric cancer patients had the lowest values (23). Interestingly, serum LDL levels greater than 110 mg/dL correlated with lymphatic metastasis (23).
Not only hyperlipidemia but also metabolic syndrome (MetS) has been associated with the development of cancer (24). Within the United States, nearly 33% of all adults and about 50% of adults older than 60 have MetS (25). In a systematic review and meta-analysis of 43 studies including 38,940 cancer cases, metabolic syndrome was found to be associated with an increased risk of several cancers including colorectal, liver, pancreas, endometrial, and postmenopausal breast cancers (26). Survivors of childhood cancer (e.g., acute lymphoblastic leukemia) were observed to have roughly two-fold higher prevalence of MetS compared with general adult population (27). Obesity, a key component of MetS, has also been identified as a risk factor for developing cancer (28, 29).
A significant association between MetS and all-cause cancer mortality has been documented in a prospective study, where MetS was associated with a 56% greater age-adjusted risk for cancer mortality (30). Women with breast cancer and MetS had a higher incidence of partial response to therapy, and high blood sugar levels were predictive of a poor response to therapy (31). The American Society of Clinical Oncology has identified obesity as one of the most important determinants of cancer mortality (28, 29).
Statins may play a role in reducing the risk of cancer development and/or progression. Lochhead et al. described the benefits of statin therapy for colorectal cancer patients (32). Patients who used statins for more than 3 years prior to their colorectal cancer diagnosis had a lower tumor stage, lower prevalence of metastasis, and higher five-year cancer-specific survival compared with statin non-users (32). There is also preclinical evidence that statins may directly block the adhesion and migration processes of cancer cells, supporting the anti-carcinogenic potential of statins (33). Anti-angiogenic effect of statins has also been reported in patients with chronic liver disease (34). Statins have been shown to induce apoptosis of hepatoma cells, inhibit intrahepatic angiogenesis, and interfere with tumor cell adhesion in hepatocellular carcinoma (34). A meta-analysis of 26 studies found that long-term statin use may reduce the risk of pancreatic cancer incidence (35). Ahern et al. reviewed the basic science and epidemiologic evidence that statins, particularly simvastatin, may reduce the risk of breast cancer recurrence. They described the broad range of existing literature that supports the anticancer effects of statins and the protective effect of statins on breast cancer prognosis (36).
Anticancer Therapies That Have the Potential to Cause Dyslipidemia
Various drugs used in cancer therapy have been associated with lipid abnormalities, either due to chemotherapy-related gonadal failure or as a direct adverse effect of the medication (Table 1). The National Cancer Institute (NCI) classifies the severity of hypertriglyceridemia and hypercholesterolemia resulting from cancer drugs as categorized in Table 2 (37).
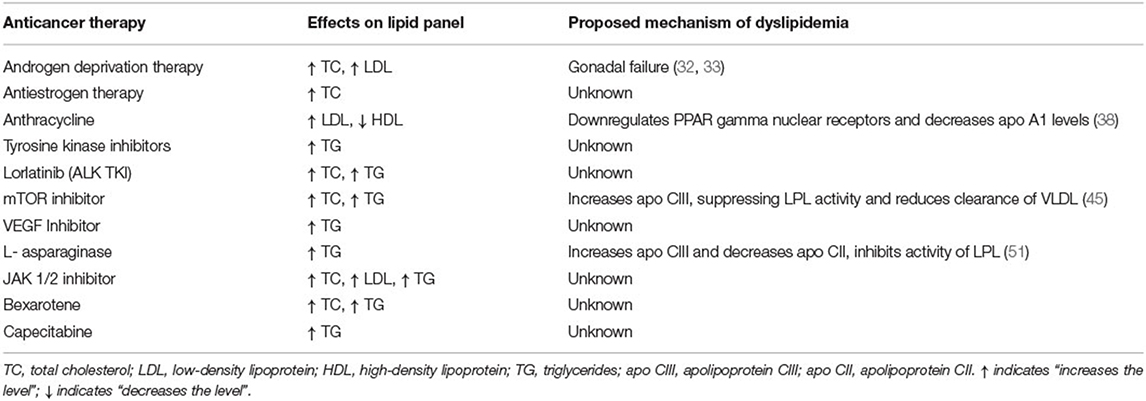
Table 1. List of anticancer therapies associated with dyslipidemia, their adverse effects on lipid profile, and the proposed mechanisms of dyslipidemia.
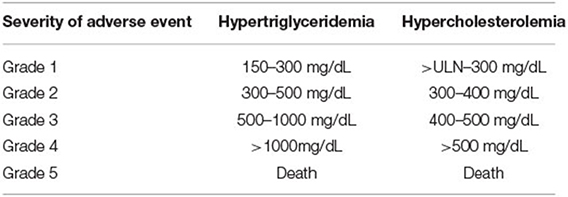
Table 2. National Cancer Institute (NCI) grading of hypertriglyceridemia and hypercholesterolemia secondary to anti-neoplastic agents.
Dyslipidemia From Gonadal Failure
Various combinations of anticancer agents can lead to gonadal failure. Tian et al. examined lipid levels of over 800 patients with early-stage breast cancer in a retrospective study, during and after neoadjuvant or adjuvant chemotherapy and compared them to those of patients who underwent surgery-only therapy without any chemotherapy (38). They found that in individuals receiving chemotherapy, the serum TC, LDL and TG levels increased significantly during chemotherapy treatment, but returned to pre-chemotherapy range about 6 months after completion of therapy (38). There were no differences between the groups receiving different combination of chemotherapy regimens. In a subgroup analysis, it was noted that younger premenopausal women were more prone to dyslipidemia while receiving chemotherapy (38). A similar study also demonstrated that premenopausal women had greater alterations in their lipid panel compared to post-menopausal women (39). This difference could be attributed to changes in lipid levels from a sudden drop in estrogen secondary to chemotherapy-induced ovarian failure (39).
Similarly, in a retrospective analysis, patients with metastatic testicular cancer receiving cisplatin-based chemotherapy were noted to have an increase in TC and LDL levels, along with increased subcutaneous fat deposition and insulin resistance (40). It was also noted that the serum estradiol level was increased in these patients which could contribute to partial hypogonadism, which in turn would affect fat and glucose metabolism (40).
Androgen Deprivation Therapy (ADT)
ADT, including gonadotropin releasing hormones (GnRH) agonists and antagonists, is the mainstay of treatment for prostate cancer (41). They inhibit the production of endogenous testosterone, causing various metabolic effects (41).
GnRH agonists (leuprolide, gosarelin) stimulate the GnRH receptor continuously, resulting in downregulation of the receptor with reduction in luteinizing hormone (LH) and subsequently testosterone levels. In contrast, GnRH antagonists (degarelix) block the same receptors and reduce the release of LH, which in turn reduces the production of testosterone (42). Anti-androgen medications like bicalutaminde and flutamide block the androgen receptors and inhibit dihydrotestosterone (DHT) from binding to it. Abiraterone acetate is an oral agent that blocks testosterone production by inhibiting the cytochrome P enzyme, CYP17 (42). ADT can cause significant changes in lipid profiles.
In a prospective study by Torimoto et al., 39 patients with prostate cancer on ADT were followed for 12 months while on therapy, with serial monitoring of their body composition and lipid levels (43). There was consistent elevation of TC and LDL levels documented throughout the year on ADT (43). Similar findings were reported by Salvador and colleagues during a 6-month follow up in patients on ADT for prostate cancer (44). They also observed no difference in the lipid profile abnormality among patients receiving GnRH agonists or bicalutamide (44). Grossman and Zajac suggested that patients receiving ADT should have a fasting lipid profile checked prior to initiation of therapy and have serial monitoring of lipid levels every 6 months (45). The American Heart Association, American Cancer Society, and American Urological Association released a scientific advisory recommending that patients have interval follow-up within 3-6 months of ADT initiation to monitor blood pressure, lipid profile, and glucose levels (46).
Antiestrogen Therapy
Antiestrogen therapies are primarily used in patients with estrogen receptor positive breast cancer. Tamoxifen is a selective estrogen receptor modulator that binds to estrogen receptors on tumors and suppresses effects of estrogen in the tumor (47). Aromatase inhibitors (AIs), such as anastrazole, letrozole, and exemestane, are selective nonsteroidal aromatase inhibitor that prevent the conversion of androstenedione to estrone and testosterone to estradiol. They are used to treat postmenopausal women with hormone-receptor positive breast cancer. These medications can reduce the tumor mass and delay cancer progression (47).
AIs, but not tamoxifen, have been associated with an increased risk of lipid abnormalities and cardiovascular (CV) events (48). In a meta-analysis by Amir et al., patients on AIs were found to have significantly higher odds of being diagnosed with hypercholesterolemia and CVD when compared with those on tamoxifen (48). Additionally, studies on mice have demonstrated that AIs can directly affect the endothelium and predispose to the development of atherosclerosis, findings which were also illustrated as attenuation of endothelial function in human studies (49). To date, there are no official recommendations for the management of hyperlipidemia secondary to antiestrogen therapies.
Anthracyclines
Doxorubicin has been associated with hyperlipidemia secondary to ovarian failure. However, anecdotal evidence suggests that anthracyclines could also directly affect lipid metabolism (50). Sharma et al. longitudinally followed patients with newly diagnosed breast cancer undergoing treatment with four cycles of doxorubicin and cyclophosphamide (+/- 5-fluorouracil), followed by treatments of paclitaxel and analyzed their serial serum lipid profiles. A continual increase in LDL and decrease in HDL were documented throughout the duration of therapy. In vitro analysis showed that doxorubicin downregulated PPAR gamma nuclear receptors and decreased apoA1 levels, which possibly reduced the production of HDL in the liver. Long-term follow up of cholesterol levels was not performed to assess for any permanent effects on lipid metabolism (50). There are no official recommendations for the management of dyslipidemia in patients receiving anthracycline treatment.
Tyrosine Kinase Inhibitors (TKI)
Dyslipidemia has been mentioned as a possible side effect of TKI (51). Anlotinib is a TKI targeting vascular endothelial growth factor receptor (VEGFR), fibroblast growth factor receptor (FGFR), platelet derived growth factor receptor (PDGFR), stem cell factor receptor (c-Kit), and Ret (52), and is used in the treatment of advanced non-small cell lung cancer (NSCLC) (53).
Early phase clinical trials showed a higher incidence of HTG (41 vs. 23.8%) and hypercholesterolemia (41.8 vs. 14%) in the anlotinib arm than the control arm (52, 53). The time of onset of HTG in the anlotinib group was around 20 days. Most patients were treated with fibrates to lower their triglycerides, and very few needed dose reductions of anlotinib. None required drug discontinuation because of HTG (52, 53). The mechanism for this dyslipidemia is not known.
Lorlatinib
This third-generation TKI targets anaplastic lymphoma kinase (ALK) gene with activity against NSCLC demonstrating resistant ALK mutations (54). In the phase two trial of lorlatinib in patients with NSCLC, the most common adverse effect was hypercholesterolemia (81%) and HTG (60%) with grades 3 and 4 severity of both observed in about 15% of patients (55). The median time to onset of hyperlipidemia from treatment initiation was 15 days (55). All of the 81% of patients were started on a lipid-lowering agent. In patients with grade four hypercholesterolemia, the dose of lorlatinib was held until the cholesterol level decreased to grade two severity, and then successfully reinitiated (55).
The Canadian Cardiovascular Society (CCS) recommends checking a lipid profile at baseline, 1, 2 and 3 months after starting lorlatinib and every 3 months thereafter. They also recommend starting lipid-lowering therapy when LDL is >3.5 mmol/L (~135 mg/dL) with a goal to reduce LDL level by 50% or <2.0mmol/L (77 mg/dL). They recommend withholding lorlatinib if the total cholesterol level is above 12.92 mmol/L (~500 mg/dL), until the levels decrease. The lipid-lowering therapies recommended were pravastatin or rosuvastatin as first-line therapy and ezetimibe for second-line therapy (54). Similar first-line therapy was recommended for isolated HTG (~500 mg/dL). They also recommend holding the medication if the TG level exceeds 11.4 mmol/L (~1,000 mg/dL). Fenofibrate or omega-3 fatty acids could be utilized as second-line therapy (54).
Mechanistic Target of Rapamycin (mTOR) Inhibitors
mTOR inhibitors (e.g., sirolimus) inhibit signaling in the phosphoinositide 3 kinase (PI3K) – Akt-mTOR pathway, which plays a key role in tumor growth and lipid metabolism. While it is a useful anti-cancer therapy and anti-rejection treatment for transplant recipients, inhibition of this pathway leads to reduced clearance of LDL in the blood causing hyperlipidemia (56).
Dyslipidemia with sirolimus use usually begins 2–4 weeks after starting therapy (57, 58). In a retrospective study of renal transplant patients on immunosuppressive regimen including sirolimus, a significant increase in TG levels and a moderate increase in the total cholesterol levels was documented (57). Morrisett et al. demonstrated return of cholesterol levels to normal within 8 weeks after discontinuation of sirolimus (58). It is hypothesized that sirolimus inhibits heparin-induced lipoprotein lipase (LPL) activity resulting in increase of apo-CIII levels, which suppresses LPL activity, hence reducing the clearance of VLDL particles (57).
Given the high incidence of this adverse effect, it is recommended to check lipid panels at baseline and then serially at every cycle for patients on mTOR inhibitors. Some experts recommend checking a fasting lipid panel weekly in early phase trials (59). It is also recommended to start statins in the first month of therapy if the patient has elevated total cholesterol or triglyceride levels (57). Lipid-lowering therapy is typically started with a goal to keep fasting TG <300 mg/dL and LDL <190 mg/dL. For patients started on lipid-lowering medication, a lipid panel should be rechecked with each cycle of therapy (59).
VEGF/VEGFR Inhibitor
VEGF/VEGFR inhibitors lead to dyslipidemia by interfering with the mTOR pathway (60). A meta-analysis revealed that patients on VEGF/VEGFR inhibitors had a higher incidence of hyperlipidemia (41%) compared to placebo (60).
Tivozanib is a VEGFR inhibitor used in patients with renal cell carcinoma (61). In the phase Ib trial of Tivozanib among patients with renal cell cancer, 30% of the recipients of Tivozanib had elevated TG levels with a grade 3/4 degree of HTG documented in up to 11% of the patients. These patients were on a relatively higher dose of tivozanib compared to other patients suggesting a possible dose-related association with HTG (61). The management strategy recommended for Tivozanib related hyperlipidemia is similar to that for mTOR inhibitors (61).
L-Asparaginase
L-asparaginase is used in the treatment of acute lymphoblastic leukemia in children with a well-known adverse effect of lipid abnormalities (62). Parsons et al. serially examined fasting lipid and lipoprotein levels in 38 patients diagnosed with ALL before, during and after asparaginase therapy. Nineteen percent of (7/38) patients had an elevation of TG level to higher than 1000 mg/dL that reverted back to normal at the end of 2 years following therapy (62). Further lipoprotein subclass analysis revealed a significant increase in VLDL levels from 30.5 mg/dL to 396 mg/dL during asparaginase therapy (62). The proposed mechanism is inhibition of LPL, increase in apo-CIII and decrease in apo-CII levels which all lead to an increase in serum TG-rich lipoproteins in the plasma. The onset of HTG is usually 8–14 days after asparaginase therapy (63).
It is recommended that TG levels should be checked in all patients prior to asparaginase therapy. Initiation of early conservative treatment with fibrates can prevent further increase in TG levels and reduce the risk of future complications, such as pancreatitis and sagittal sinus thrombosis (64).
JAK1/2 Inhibitor
Ruxolitinib is an oral JAK1 and JAK2 inhibitor approved for treatment of myelofibrosis (MF) and polycythemia (PV) (65). The COMFORT -I study demonstrating the efficacy of ruxolitinib in MF also showed an increase in TC and LDL levels (66). Anecdotal reports have also described HTG manifesting as steatohepatitis and pancreatitis in patients treated with ruxolitinib (65, 67). It is recommended to monitor lipid levels after starting ruxolitinib, particularly if given in combination with sirolimus for graft-vs. host disease (65).
Bexarotene
This retinoid compound is used in the treatment of patients with refractory cutaneous T-cell lymphoma (68). HTG within 2–4 weeks of starting therapy is a known adverse effect of bexarotene seen in up to 70% of patients secondary to a rise in the production of VLDL (69). The HTG and elevated TC levels are often reversible with discontinuation of therapy. Patients are recommended to have a baseline fasting lipid panel checked prior to starting bexarotene and thereafter be checked weekly for 2–4 weeks. If stable, it can then be checked every 8 weeks. The goal is to maintain fasting triglycerides around ~400 mg/dL. If triglyceride levels rise above 400 mg/dL, it is recommended to consider starting lipid lowering therapy like statins, and if necessary, reduce the dose or interrupt bexarotene (68).
Capecitabine
Capecitabine is a prodrug of 5-fluorouracil (5-FU) commonly used in patients with breast and colon cancer. Multiple case reports of capecitabine-induced HTG exist in the literature (70). Dumana et al. described the case of a patient with breast cancer on capecitabine who developed HTG with levels > 9,000 mg/dL requiring lipid apheresis (71). Following discontinuation of capecitabine, the lipid levels normalized with eventual discontinuation of lipid lowering therapy (71). It has been hypothesized that this HTG may be more prominent in patients with hereditary LPL deficiency (70).
Management of Dyslipidemia in Patients With Cancer
The initial steps for the treatment of dyslipidemia, metabolic syndrome and obesity which are highly prevalent in patients with cancer are the promotion of lifestyle changes, including modification of diet and addition of an exercise routine. A diet that emphasizes consumption of fruits, legumes, nuts, whole grains, and fish is recommended. A heart healthy diet should avoid saturated and trans fats, high sodium intake, processed meats, refined carbohydrates, and sweetened beverages (72). The ACC/AHA 2019 guidelines also recommend that adults exercise at least 150 min of moderate-intensity physical activity or 75 min of vigorous-intensity aerobic physical activity per week. All adults should decrease sedentary behavior to reduce ASCVD risk (72).
Current guidelines recommend the use of statin therapy for the primary prevention of CVD in patients with LDL>190 mg/dL, diabetes mellitus, or elevated 10-year atherosclerotic cardiovascular disease (ASCVD) risk score in patients without diabetes mellitus (73). In addition, statins are recommended for all patients with established ASCVD for secondary prevention. Patients with an intermediate (7.5% to <20%) and high (>20%) 10-year ASCVD risk scores should be considered for moderate- and high- intensity statin therapy, respectively, in addition to lifestyle changes (73). The Canadian Cardiovascular Society (CCS) updated their guidelines in 2021 to propose similar recommendations to the ACC/AHA with the key difference being that they recommend risk stratification using the Framingham Risk Score (74). The Childhood Cancer Survivor Study (CCSS) developed a risk assessment tool that predicts the risk of heart failure, ischemic heart disease, and stroke by age 50 among survivors of childhood cancer (75).
The traditional CVD risk assessment tools, such as the ACC/AHA Risk Estimator/ Pooled Cohort Equation or the Framingham Risk Score, do not include cancer-specific parameters or history of cancer treatment and thus may underestimate the true long-term CVD risk in cancer survivors. A population-based cohort study showed an increase in the medium and long-term risks of CV diseases (including heart failure, coronary artery disease, arrhythmia, stroke, and venous thromboembolism) in the survivors of various adult cancers compared with the general population (76). The increased risks were most pronounced in individuals who had exposure to chemotherapy. Multiple myeloma, lung cancer, non-Hodgkin lymphoma, and breast cancer were associated with significantly higher CVD risk compared with noncancer controls. The increased risk was most pronounced in cancer survivors with two or more CV risk factors (77).
Coronary artery calcium (CAC) scoring may provide additional risk stratification in patients with cancer (78). In a population-based cohort study that evaluated 484 patients undergoing low-dose CT for lung cancer screening, higher CAC scores were associated with an increased risk of CAD (78). The CAC Consortium developed an equation to calculate the risk of death from CVD vs. from cancer (79). They found that the mortality risk from CVD exceeded that from cancer at age 50 if the CAC score is >115 and at age 70 if the CAC sore is > 570 (79). These studies suggest the utility of CAC scoring in identifying individuals with cancer who can benefit from early preventative measures.
To further refine the prediction of CVD risk in the cancer patient, additional measures such as advanced lipid markers (lipoprotein(a), apolipoprotein B) and inflammatory markers (hs-CRP) may be of benefit. Table 3 summarizes the current tools available for cardiovascular risk stratification in cancer patients. Further research is needed to elucidate which tools are the most predictive of CVD risk in this population.
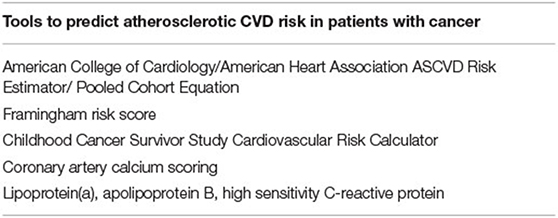
Table 3. List of the risk stratification tools currently available to identify patients with cancer who are at increased risk of developing late atherosclerotic CVD.
Statins
Recommendations guiding the management of hyperlipidemia in patients who are actively undergoing, or have recently completed, cancer treatment are largely lacking. The treatment of hyperlipidemia, and the primary and secondary prevention of CVD, in patients with cancer largely follow the framework proposed by the 2019 ACC/AHA guidelines (72). The management of hypercholesterolemia focuses on lifestyle modification initially, then on the addition of statin therapy, followed by consideration of other medications, such as ezetimibe and proprotein convertase subtilisin/kexin type 9 serine protease (PCSK9) inhibitors. Given the unique characteristics of patients with cancer, including their exposure to potentially cardiotoxic cancer treatment, future research is imperative to determine the ideal strategies to reduce their long-term CV risk.
Statins have been found to have pleiotropic effects, including antioxidant, anti-inflammatory, and immunomodulatory effects, as well as atherosclerotic plaque stabilization (80). They may also have anticancer effects, as discussed in the “Hyperlipidemia, metabolic Syndrome, and cancer” section above. These pleiotropic effects support the importance of statin therapy in this patient population, in which further studies investigating its potential benefits are warranted.
Ezetimibe
Ezetimibe is the current second line therapy for hyperlipidemia. The Simvastatin and Ezetimibe in Aortic Stenosis (SEAS) study investigated the effects of combination ezetimibe/simvastatin compared with placebo on the effects of CV events (81). Initial analyses raised concerns about ezetimibe having potential carcinogenic properties. Further sub-analyses dispelled this hypothesis and found that ezetimibe does not significantly increase the risk of cancer or overall mortality (81). Meta-analyses have demonstrated that ezetimibe has beneficial effects on CVD endpoints, including myocardial infarction and stroke, without increasing all-cause or CV mortality, nor cancer development (82, 83). The addition of ezetimibe to statin therapy has been shown to cause a greater LDL reduction than doubling the statin dose (84). Given its overall benefits and safety, ezetimibe should be the ideal second antilipidemic agent of choice for individuals with cancer who have increased CV risk.
Other Antilipidemic Agents
PCSK9 inhibitors are novel cholesterol-lowering agents that act by attaching to the LDL receptor, reducing its degradation and thus increasing LDL clearance (85). Although the data supporting the use of PCSK9 inhibitors primarily as antilipidemic agent in patients with cancer is limited, preliminary data suggests that it may potentially assist anti-cancer therapy by boosting the effect of immunotherapy by upregulating the MHC-I expression and promoting intratumoral T-cell infiltration making the tumor more responsive to immunotherapy (86). More studies are needed to analyze its lipid-lowering activity in this specific subset of patients.
There is scant data on the use of new lipid-lowering therapies like bempedoic acid in patients with cancer. It (8-hydroxy- 2,2,14,14- tetramethylpentadecanedioic acid) is a small molecule that inhibits ATP citrate lyase, a crucial step in the synthesis of cytosolic acetyl – CoA, which is the building block in cholesterol biosynthesis (87). Currently, it serves as an alternative lipid-lowering treatment in patients intolerable of frontline agents (88).
Icosapent ethyl is another newer agent that acts by reducing hepatic production of TG. There is limited data on the use of this medication in patients with cancer. The REDUCE-IT trial, which demonstrated the CV benefits of icosapent ethyl in patients with elevated TG levels, excluded patients on tamoxifen, cyclophosphamide, and patients with life expectancy of <2 years (89). Further research is needed to better define the role of non-statin therapies, including ezetimibe, PCSK9 inhibitors, bempedoic acid and icosapent ethyl, in the management of dyslipidemia in cancer patients.
Special Considerations for the Use of Lipid-Lowering Therapy in Patients With Cancer
There are special considerations to make when initiating patients on lipid-lowering therapy that are receiving active chemotherapy (Table 4). These include potential drug-drug interactions between dyslipidemia medications and chemotherapy, patients with liver disease, and patients that suffer adverse reactions from dyslipidemia medications.
Drug-Drug Interactions
Some statins are metabolized and cleared by the liver, predisposing potential drug-drug interactions. Simvastatin, lovastatin, and atorvastatin are metabolized by cytochrome p450 3A4 (CYP3A4). Thus, they can interact with other medications that are metabolized by CYP3A4, e.g., antibiotics, antivirals, antiepileptics, calcium channel blockers, and antineoplastic agents (47). Most cases of drug interactions are reported with simvastatin likely arising from competitive effect of anti-cancer drugs on CYP3A4, resulting in hepatotoxity and rhabdomyolysis from augmentation of simvastatin through decreased clearance (90). Nilotinib and ribociclib are considered moderate inhibitors of CYP3A4; lorlatinib and pexidartinib are moderate inducers of CYP3A4 (47). In patients taking TKIs such as imatinib and dasatinib, or mitotane (used in adrenal carcinoma), hepatically metabolized statins should either be tapered to the safest tolerable dose or discontinued and replaced by safer alternatives (e.g., pravastatin or rosuvastatin) (91). Awareness of potential drug-drug interactions is critical in managing patients with cancer. Collaboration with a pharmacist and/or oncologist is important. Pharmacy references or websites that check for drug-drug interactions should be utilized prior to initiating antilipidemic medications in patients who are receiving anticancer drugs, particularly the novel targeted agents.
Cancer Patients With Liver Disease
As mentioned previously, statins are the cornerstone therapy for ASCVD risk reduction. However, myopathy and hepatotoxicity are its known adverse effects (92). This is especially concerning among patients with cancer and liver disease. Pravastatin, rosuvastatin, and pitavastatin are not metabolized by the liver and can be used for this special subset of patients (93). Statin-induced liver injury has primarily been observed with atorvastatin and simvastatin. Studies have found lovastatin to have no increased risk of hepatotoxicity in patients with known liver disease (93). Statins have pleiotropic effects, including potential inhibitory effect on the progression of liver fibrosis to cirrhosis and hepatocellular carcinoma (93). Thus, statins that are not metabolized by the liver can be safely used in patients with concomitant cancer and liver disease.
Potential Adverse Reactions of Lipid-Lowering Therapy
Statins are generally well-tolerated medications. In addition to hepatotoxicity, rhabdomyoloysis, immune-mediated necrotizing myopathy, and myalgias are other known adverse reactions. It is important to monitor liver function tests and test for rhabdomyolysis if patients complain of myalgias. Less serious adverse reactions to statins include nasopharyngitis and diarrhea (47). Ezetimibe is also associated with hepatocellular injury, rhabdomyolysis, myopathy, and myalgias. Postmarketing studies have also found erythema multiforme, anaphylaxis, and angioedema associated with its use (47). PCSK9 inhibitors are well-tolerated with local injection site reactions, e.g., erythema, pain, or bruising, reported as the most common adverse reaction (47). Bempedoic acid is known to have a dose-related hyperuricemic effect and rarely associated with tendon rupture (47). Icosapent ethyl has been associated with an increased risk of bleeding and increased risk of developing atrial fibrillation and atrial flutter (47).
Conclusion
The burden of CVD is exceedingly high in patients with cancer because of a high prevalence of underlying risk factors, such as hyperlipidemia, hypertension, diabetes mellitus, and metabolic syndrome. In addition, anticancer therapies may exert cardio- and vasculo- toxic effects as well as adverse effects on lipid levels. It is essential for medical providers to be aware of these side effects and promptly institute appropriate therapy as well as other CV preventive strategies. Developing CV risk assessment tools that accurately identify cancer patients who are at an increased risk of CVD is needed. Coronary artery calcium scoring and serum markers can potentially aid with risk stratification and deserve further investigation to understand their utility in patients with cancer (Table 5). Statins are the mainstay of therapy in both primary and secondary CVD prevention as well as in the management of hyperlipidemia. The role for non-statin therapies for dyslipidemia management also need further investigation as they may contribute to overall CVD risk reduction (Table 5).
In summary, optimal cardiovascular care of the contemporary cancer patient requires a multidisciplinary approach to accurately define CVD risk, institute appropriate preventive measures, and address the potential adverse cardiometabolic effects of anticancer therapies. An integrative team comprised of primary care, oncology, cardio-oncology, nursing, and pharmacology devoted to the comprehensive and longitudinal care of patients from cancer diagnosis to treatment to survivorship is needed (Figure 1). This team of providers plays an integral role in cancer screening and diagnosis, monitoring for potential adverse events during cancer treatment, and management of chronic health comorbidities in survivorship (94). Understanding the myriad possible early and late side effects of cancer treatment is critical to improve overall morbidity and mortality of cancer survivors.
Author Contributions
AK, MDJ, and TM made substantial contribution to the article design and conception of the work. MDJ, TM, MS, and JT contributed to the acquisition of data and drafting of the manuscript. AK and MDJ made major contributions to analysis, interpretation and editing of the manuscript, made critical revisions, and all authors read and approved the final manuscript.
Funding
The funding for the article processing charge was provided by the Letts O'Brien Fund (UConn Foundation endowment fund #0035192).
Conflict of Interest
The authors declare that the research was conducted in the absence of any commercial or financial relationships that could be construed as a potential conflict of interest.
Publisher's Note
All claims expressed in this article are solely those of the authors and do not necessarily represent those of their affiliated organizations, or those of the publisher, the editors and the reviewers. Any product that may be evaluated in this article, or claim that may be made by its manufacturer, is not guaranteed or endorsed by the publisher.
References
1. Mattiuzzi C. Cancer statistics: a comparison between World Health Organization (WHO) and Global Burden of Disease (GBD). Eur J Public Health. (2020) 30:1026–7. doi: 10.1093/eurpub/ckz216
2. Sturgeon KM, Deng L, Bluethmann SM, Zhou S, Trifiletti D, Jiang C, et al. A population-based study of cardiovascular disease mortality risk in US cancer patients. Eur Heart J. (2019) 40:3889–97. doi: 10.1093/eurheartj/ehz766
3. Aparecida Silveira E, Vaseghi G, de Carvalho Santos AS, Kliemann N, Masoudkabir F, Noll M, et al. Visceral Obesity and Its Shared Role in Cancer and Cardiovascular Disease: a Scoping Review of the Pathophysiology and Pharmacological Treatments. Int J Mol Sci. (2020) 21:E9042. doi: 10.3390/ijms21239042
4. Mohammed T, Singh M, Tiu JG, Kim AS. Etiology and management of hypertension in patients with cancer. Cardio-Oncol Lond Engl. (2021) 7:14. doi: 10.1186/s40959-021-00101-2
5. Zamorano JL, Gottfridsson C, Asteggiano R, Atar D, Badimon L, Bax JJ, et al. The cancer patient and cardiology. Eur J Heart Fail. (2020) 22:2290–309. doi: 10.1002/ejhf.1985
6. Luo X, Cheng C, Tan Z, Li N, Tang M, Yang L, et al. Emerging roles of lipid metabolism in cancer metastasis. Mol Cancer. (2017) 16:76. doi: 10.1186/s12943-017-0646-3
7. Cao Y. Adipocyte and lipid metabolism in cancer drug resistance. J Clin Invest. (2019) 129:3006–17. doi: 10.1172/JCI127201
8. Cortes JE, Jean Khoury H, Kantarjian H, Brümmendorf TH, Mauro MJ, Matczak E, et al. Long-term evaluation of cardiac and vascular toxicity in patients with Philadelphia chromosome-positive leukemias treated with bosutinib. Am J Hematol. (2016) 91:606–16. doi: 10.1002/ajh.24360
9. Duran E, Aday A, Cook N, Buring J, Ridker P, Pradhan A. Triglyceride-Rich Lipoprotein Cholesterol, Small Dense LDL Cholesterol, and Incident Cardiovascular Disease. J Am Coll Cardiol. (2020) 75:2122–35. doi: 10.1016/j.jacc.2020.02.059
10. Collins R, Reith C, Emberson J, Armitage J, Baigent C, Blackwell L. Interpretation of the evidence for the efficacy and safety of statin therapy. Lancet. (2016) 388:2532–61. doi: 10.1016/S0140-6736(16)31357-5
11. Brown AJ. Cholesterol, Statins and Cancer. Clin Exp Pharmacol Physiol. (2007) 34:135–41. doi: 10.1111/j.1440-1681.2007.04565.x
12. Schulze A, Harris AL. How cancer metabolism is tuned for proliferation and vulnerable to disruption. Nature. (2012) 491:364–73. doi: 10.1038/nature11706
13. Röhrig F, Schulze A. The multifaceted roles of fatty acid synthesis in cancer. Nat Rev Cancer. (2016) 16:732–49. doi: 10.1038/nrc.2016.89
14. Sun Y, He W, Luo M, Zhou Y, Chang G, Ren W, et al. SREBP1 regulates tumorigenesis and prognosis of pancreatic cancer through targeting lipid metabolism. Tumor Biol. (2015) 36:4133–41. doi: 10.1007/s13277-015-3047-5
15. Yin F, Sharen G, Yuan F, Peng Y, Chen R, Zhou X, et al. TIP30 regulates lipid metabolism in hepatocellular carcinoma by regulating SREBP1 through the Akt/mTOR signaling pathway. Oncogenesis. (2017) 6:e347–e347. doi: 10.1038/oncsis.2017.49
16. Lee BH, Taylor MG, Robinet P, Smith JD, Schweitzer J, Sehayek E, et al. Dysregulation of Cholesterol Homeostasis in Human Prostate Cancer through Loss of ABCA1. Cancer Res. (2013) 73:1211–8. doi: 10.1158/0008-5472.CAN-12-3128
17. Rysman E, Brusselmans K, Scheys K, Timmermans L, Derua R, Munck S, et al. De novo lipogenesis protects cancer cells fromfree radicals and chemotherapeutics by promoting membrane lipid saturation. Cancer Res. (2010) 70:8117–26. doi: 10.1158/0008-5472.CAN-09-3871
18. Baenke F, Peck B, Miess H, Schulze A. Hooked on fat: the role of lipid synthesis in cancer metabolism and tumour development. Dis Model Mech. (2013) 6:1353–63. doi: 10.1242/dmm.011338
19. Park J, Lee C, Jang J, Ghim J, Kim Y, You S, et al. Phospholipase signalling networks in cancer. Nat Rev Cancer. (2012) 12:782–92. doi: 10.1038/nrc3379
20. Chang S, Liu C, Conway R, Han D, Nithipatikom K, Trifan O, et al. Role of prostaglandin E2-dependent angiogenic switch in cyclooxygenase 2-induced breast cancerprogression. Proc Natl Acad Sci. (2004) 101:591–6. doi: 10.1073/pnas.2535911100
21. Ray G, Husain S. Role of lipids, lipoproteins and vitamins in women with breast cancer. Clin Biochem. (2001) 34:71–6. doi: 10.1016/S0009-9120(00)00200-9
22. Shah F, Shukla S, Shah P, Patel H, Patel P. Significance of Alterations in Plasma Lipid Profile Levels in Breast Cancer. Integr Cancer Ther. (2008) 7:33–41. doi: 10.1177/1534735407313883
23. Ghahremanfard F, Mirmohammadkhani M, Shahnazari B, Gholami G, Mehdizadeh J. The Valuable Role of Measuring Serum Lipid Profile in Cancer Progression. Oman Med J. (2015) 30:353–7. doi: 10.5001/omj.2015.71
24. Uzunlulu M, Telci Caklili O, Oguz A. Association between Metabolic Syndrome and Cancer. Ann Nutr Metab. (2016) 68:173–9. doi: 10.1159/000443743
25. Moore J, Chaudhary N, Akinyemiju T. Metabolic Syndrome Prevalence by Race/Ethnicity and Sex in the United States, National Health and Nutrition Examination Survey, 1988-2012. Prev Chronic Dis. (2017) 14:E24. doi: 10.5888/pcd14.160287
26. Esposito K, Chiodini P, Colao A, Lenzi A, Giugliano D. Metabolic syndrome and risk of cancer: a systematic review and meta-analysis. Diabetes Care. (2012) 35:2402–11. doi: 10.2337/dc12-0336
27. Saultier P, Auquier P, Bertrand Y, Vercasson C, Oudin C, Contet A, et al. Metabolic syndrome in long-term survivors of childhood acute leukemia treated without hematopoietic stem cell transplantation: an L.E.A. study. Haematologica. (2016) 101:1603–10. doi: 10.3324/haematol.2016.148908
28. Ligibel J, Alfano C, Courneya K, Denmark-Wahnefried W, Burger R. American Society of Clinical Oncology Position Statement on Obesity and Cancer. J Clin Oncol. (2014) 32:3568–74. doi: 10.1200/JCO.2014.58.4680
29. Avgerinos K, Spyrou N, Mantzoros C, Dalamaga M. Obesity and cancer risk: Emerging biological mechanisms and perspectives. Metabolism. (2019) 92:121–35. doi: 10.1016/j.metabol.2018.11.001
30. Jaggers J, Sui X, Hooker S. Metabolic syndrome and risk of cancer mortality in men. Eur J Cancer. (2009) 45:1831–8. doi: 10.1016/j.ejca.2009.01.031
31. Stebbing J, Sharma A, North B. A metabolic phenotyping approach to understanding relationships between metabolic syndrome and breast tumour responses to chemotherapy. Ann Oncol. (2012) 23:860–6. doi: 10.1093/annonc/mdr347
32. Lochhead P, Chan A. Statins and colorectal cancer. Clin Gastroenterol Hepatol. (2013) 11:109–18. doi: 10.1016/j.cgh.2012.08.037
33. Murai T. Cholesterol lowering: role in cancer prevention and treatment. Biol Chem. (2015) 396:1–11. doi: 10.1515/hsz-2014-0194
34. Pose E, Trebicka J, Mookerjee RP, Angeli P, Gines P. Statins: old drugs as new therapy for liver diseases? J Hepatol. (2019) 70:194–202. doi: 10.1016/j.jhep.2018.07.019
35. Zhang Y, Liang M, Sun C, Qu G, Shi T, Min M, et al. Statin Use and Risk of Pancreatic Cancer: An Updated Meta-analysis of 26 Studies. Pancreas. (2019) 48:142–50. doi: 10.1097/MPA.0000000000001226
36. Ahern T, Lash T, Christiansen P, Cronin-Fenton D. Statins and breast cancer prognosis: evidence and opportunities. Lancet Oncol. (2014) 15:e461–8. doi: 10.1016/S1470-2045(14)70119-6
37. Trotti A, Colevas A, Setser A. CTCAE. v3. 0: development of a comprehensive grading system for the adverse effects of cancer treatment. Semin Radiat Oncol. (2003) 13:176–81. doi: 10.1016/S1053-4296(03)00031-6
38. Tian W, Yao Y, Fan G. Changes in lipid profiles during and after (neo)adjuvant chemotherapy in women with early-stage breast cancer: a retrospective study. PLoS ONE. (2019) 14:e0221866. doi: 10.1371/journal.pone.0221866
39. He T, Wang C, Tan Q. Adjuvant chemotherapy-associated lipid changes in breast cancer patients: a real-word retrospective analysis. Medicine (Baltimore). (2020) 99:e21498. doi: 10.1097/MD.0000000000021498
40. Willemse PPM, van der Meer R, Burggraaf J, van Elderen SGC, de Kam ML, de Roos A, et al. Abdominal visceral and subcutaneous fat increase, insulin resistance and hyperlipidemia in testicular cancer patients treated with cisplatin-based chemotherapy. Acta Oncol. (2014) 53:351–60. doi: 10.3109/0284186X.2013.819116
41. Choi S, Kam S. Metabolic effects of androgen deprivation therapy. Korean J Urol. (2015) 56:12. doi: 10.4111/kju.2015.56.1.12
42. Crawford E, Heidenreich A, Lawrentschuk N, Tombal B, Pompeo AC, Mendoza-Valdes A, et al. Androgen-targeted therapy in men with prostate cancer: evolving practice and future considerations. Prostate Cancer Prostatic Dis. (2019) 22:24. doi: 10.1038/s41391-018-0079-0
43. Torimoto K, Samma S, Kagebayashi Y, Chihara Y, Tanaka N, Hirayama A, et al. The Effects of Androgen Deprivation Therapy on Lipid Metabolism and Body Composition in Japanese Patients with Prostate Cancer. Jpn J Clin Oncol. (2011) 41:577–81. doi: 10.1093/jjco/hyr005
44. Salvador C, Planas J, Agreda F, Placer J, Trilla E, Lopez M, et al. Analysis of the Lipid Profile and Atherogenic Risk during Androgen Deprivation Therapy in Prostate Cancer Patients. Urol Int. (2013) 90:41–4. doi: 10.1159/000342814
45. Grossman M, Zajac J. Management of side effects of androgen deprivation therapy. Endocrinol Metab Clin North Am. (2011) 40:655–71. doi: 10.1016/j.ecl.2011.05.004
46. Levine G, D'Amico A, Berger P, Clark P, Eckel R, Keating N. Androgen-deprivation therapy in prostate cancer and cardiovascular risk: a science advisory from the American Heart Association, American Cancer Society, and American Urological Association: endorsed by the American Society for Radiation Oncology. Circulation. (2010) 121:833–40. doi: 10.1161/CIRCULATIONAHA.109.192695
47. Estes NAM III, Gersh BJ, Hunt SA, Otto CM UpToDate (2022). Available online at: https://www-uptodate-com.online.uchc.edu/contents/search (accessed January 6, 2022)
48. Amir E, Seruga B, Niraula S, Carlsson L, Ocaña A. Toxicity of adjuvant endocrine therapy in postmenopausal breast cancer patients: a systematic review and meta-analysis. J Natl Cancer Inst. (2011) 103:1299–309. doi: 10.1093/jnci/djr242
49. Maor R, Sara JDS, Wanous AA, Maor E, Pruthi S, Lerman A, et al. Attenuated peripheral endothelial function among women treated with aromatase inhibitors for breast cancer. Coron Artery Dis. (2018) 29:687–93. doi: 10.1097/MCA.0000000000000666
50. Sharma M, Tuaine J, McLaren B. Chemotherapy Agents Alter Plasma Lipids in Breast Cancer Patients and Show Differential Effects on Lipid Metabolism Genes in Liver Cells. PLoS ONE. (2016) 11:148049. doi: 10.1371/journal.pone.0148049
51. Zhou A, Bai Y, Song Y. Anlotinib Versus Sunitinib as First-Line Treatment for Metastatic Renal Cell Carcinoma: A Randomized Phase II Clinical Trial. Oncologist. (2019) 24:e702. doi: 10.1634/theoncologist.2018-0839
52. Si X, Zhang L, Wang H. Management of anlotinib-related adverse events in patients with advanced non-small cell lung cancer: Experiences in ALTER-0303. Thorac Cancer. (2019) 10:551. doi: 10.1111/1759-7714.12977
53. Han B, Li K, Wang Q. Effect of Anlotinib as a Third-Line or Further Treatment on Overall Survival of Patients With Advanced Non–Small Cell Lung Cancer: The ALTER 0303 Phase 3 Randomized Clinical Trial. JAMA Oncol. (2018) 4:1569. doi: 10.1001/jamaoncol.2018.3039
54. Blais N, Adam J, Nguyen J, Gregoire J. Evaluation and Management of Dyslipidemia in Patients Treated with Lorlatinib. Curr Oncol. (2021) 28:265–72. doi: 10.3390/curroncol28010029
55. Bauer T, Felip E, Solomon B. Clinical Management of Adverse Events Associated with Lorlatinib. Oncologist. (2019) 24:1103. doi: 10.1634/theoncologist.2018-0380
56. Verges B, Walter T, Cariou B. Endocrine Side Effects of Anti-Cancer Drugs: Effects of anti-cancer targeted therapies on lipid and glucose metabolism. Eur J Endocrinol. (2014) 170:R43–55. doi: 10.1530/EJE-13-0586
57. Hakeam HA, Al-Jedai AH, Raza SM, Hamawi K. Sirolimus induced dyslipidemia in tacrolimus based vs. tacrolimus free immunosuppressive regimens in renal transplant recipients. Ann Transplant. (2008) 13:46–53.
58. Morrisett J, Abdel-Fattah G, Hoogeveen R, Morrisett JD, Abdel-Fattah G, Hoogeveen R, et al. Effects of sirolimus on plasma lipids, lipoprotein levels, and fatty acid metabolism in renal transplant patients. J Lipid Res. (2002) 43:1170–80. doi: 10.1194/jlr.M100392-JLR200
59. Busaidy N, Farooki A, Dowlati A. Management of Metabolic Effects Associated With Anticancer Agents Targeting the PI3K-Akt-mTOR Pathway. J Clin Oncol. (2012) 30:2919. doi: 10.1200/JCO.2011.39.7356
60. Dai H, Liu C, Li P. Risk of Dyslipidemia Associated with VEGF/VEGFR Inhibitors: a meta-analysis. Transl Oncol. (2020) 13:100779. doi: 10.1016/j.tranon.2020.100779
61. Fishman M, Srinivas S, Hauke R. Phase Ib study of tivozanib (AV-951) in combination with temsirolimus in patients with renal cell carcinoma. Eur J Cancer. (2013) 49:2841. doi: 10.1016/j.ejca.2013.04.019
62. Parsons S, Skapek S, Neufeld E. Asparaginase-Associated Lipid Abnormalities in Children With Acute Lymphoblastic Leukemia. Blood. (1997) 89:1886–95. doi: 10.1182/blood.V89.6.1886
63. Tozuka M, Yamauchi K, Hidaka H, Nakabayashi T, Okumura N, Katsuyama T. Characterization of hypertriglyceridemia induced by L-asparaginase therapy for acute lymphoblastic leukemia and malignant lymphoma. Ann Clin Lab Sci. (1997) 27:351–7.
64. Cohen H, Bielorai B, Harats D, Toren A, Pinhas-Hamiel O. Conservative treatment of L-asparaginase-associated lipid abnormalities in children with acute lymphoblastic leukemia. Pediatr Blood Cancer. (2010) 54:703–6. doi: 10.1002/pbc.22305
65. Bauters T, Bordon V, Laureys G, Dhooge C. Combined use of ruxolitinib and sirolimus: increased monitoring of triglycerides required. Bone Marrow Transplant. (2019) 54:1372–3. doi: 10.1038/s41409-019-0488-2
66. Mesa R, Verstovsek S, Gupta V, Mascarenhas JO, Atallah E, Burn T, et al. Effects of Ruxolitinib Treatment on Metabolic and Nutritional Parameters in Patients With Myelofibrosis From COMFORT-I. Clin Lymphoma Myeloma Leuk. (2015) 15:214. doi: 10.1016/j.clml.2014.12.008
67. Watson A, Brunstein C, Holtan S. Life-Threatening Hypertriglyceridemia in a Patient on Ruxolitinib and Sirolimus for Chronic Graft-versus-Host Disease. Case Rep Transplant. (2018) 2018:1–3. doi: 10.1155/2018/4539757
68. Ligand, Pharmaceuticals Incorporated. Targretin® (bexarotene) [package insert]. U.S. Food and Drug Administration Website. Available online at: https://www.accessdata.fda.gov/drugsatfda_docs/label/1999/21055lbl.pdf
69. de Vries-van der Weij J, de Haan W, Hu L, Kuif M, Oei HL, van der Hoorn JWA, et al. Bexarotene Induces Dyslipidemia by Increased Very Low-Density Lipoprotein Production and Cholesteryl Ester Transfer Protein-Mediated Reduction of High-Density Lipoprotein. Endocrinology. (2009) 150:2368–75. doi: 10.1210/en.2008-1540
70. Kurt M, Babaoglu MO, Yasar U, Shorbagi A, Guler N. Capecitabine-Induced Severe Hypertriglyceridemia: Report of Two Cases. Ann Pharmacother. (2006) 40:328–31. doi: 10.1345/aph.1G348
71. Duman B, Paydas S, Tetiker T, Gunaldi M, Afsar C, Ercolak V, et al. Capecitabine-Induced Hypertriglyceridemia and Hyperglycemia: Two Cases. Pharmacology. (2012) 90:212–5. doi: 10.1159/000342382
72. Arnett DK, Blumenthal RS, Albert MA, Buroker AB, Goldberger ZD, Hahn EJ, et al. 2019 ACC/AHA Guideline on the Primary Prevention of Cardiovascular Disease: A Report of the American College of Cardiology/American Heart Association Task Force on Clinical Practice Guidelines. J Am Coll Cardiol. (2019) 74:e177–232. doi: 10.1016/j.jacc.2019.03.010
73. Lloyd-Jonse D, Braun LT, Ndumele CE, Smith SC Jr, Sperling LS, Virani SS, et al. Use of Risk Assessment Tools to Guide Decision-Making in the Primary Prevention of Atherosclerotic Cardiovascular Disease: a Special Report From the American Heart Association and American College of Cardiology. J Am Coll Cardiol. (2019) 73:3153–67. doi: 10.1161/CIR.0000000000000638
74. Pearson GJ, Thanassoulis G, Anderson TJ, Stone J, Ward R, Wray W. 2021 Canadian Cardiovascular Society Guidelines for the Management of Dyslipidemia for the Prevention of Cardiovascular Disease in Adults. Soc Guidel. (2021) 37:1129–50. doi: 10.1016/j.cjca.2021.03.016
75. Chow EJ, Chen Y, Kremer L, Breslow NE, Hudson MM, Armstrong GT, et al. Individual prediction of heart failure among childhood cancer survivors. J Clin Oncol. (2015) 33:394–402. doi: 10.1200/JCO.2014.56.1373
76. Strongman H, Gadd S, Matthews A, Mansfield KE, Stanway S, Lyon AR, et al. Medium and long-term risks of specific cardiovascular diseases in survivors of 20 adult cancers: a population-based cohort study using multiple linked UK electronic health records databases. Lancet. (2019) 394:1041–54. doi: 10.1016/S0140-6736(19)31674-5
77. Armenian S, Xu L, Ky B, Sun C, Farol L, Pal S. Cardiovascular Disease Among Survivors of Adult-Onset Cancer: A Community-Based Retrospective Cohort Study. J Clin Oncol. (2016) 34:1122–30. doi: 10.1200/JCO.2015.64.0409
78. Garg P, Jorgensen N, McClelland R, Leigh JA, Greenland P, Blaha M, et al. Use of coronary artery calcium testing to improve coronary heart disease risk assessment in a lung cancer screening population: The Multi-Ethnic Study of Atherosclerosis (MESA). J Cardiovasc Comput Tomogr. (2018) 12:493–9. doi: 10.1016/j.jcct.2018.10.001
79. Whelton S, Al Rifai M, Marshall CH, Dardari Z, Shaw LJ, Al-Mallah M, et al. Coronary Artery Calcium and the Age-Specific Competing Risk of Cardiovascular Versus Cancer Mortality: the Coronary Artery Calcium Consortium. Am J Med. (2020) 133:e575–83. doi: 10.1016/j.amjmed.2020.02.034
80. Bahrami A, Parsamanesh N, Atkin SL, Banach M, Sahebkar A. Effect of statins on toll-like receptors: a new insight to pleiotropic effects. Pharmacol Res. (2018) 135:230–8. doi: 10.1016/j.phrs.2018.08.014
81. Green A, Ramey DR, Emneus M, Iachina M, Stavem K, Bolin K. Incidence of cancer and mortality in patients from the Simvastatin and Ezetimibe in Aortic Stenosis (SEAS) trial. Am J Cardiol. (2014) 114:1518–22. doi: 10.1016/j.amjcard.2014.08.016
82. Savarese G, De Ferrari G, Rosano GM, Perrone-Filardi P. Safety and efficacy of ezetimibe: a meta-analysis. Int J Cardiol. (2015) 201:247–52. doi: 10.1016/j.ijcard.2015.08.103
83. Zhan S, Tang M, Liu F, Xia P, Shu M, Wu X. Ezetimibe for the prevention of cardiovascular disease and all-cause mortality events. Cochrane Database Syst Rev. (2018) 11:CD012502. doi: 10.1002/14651858.CD012502.pub2
84. Ferreira AM, da Silva PM. Defining the Place of Ezetimibe/Atorvastatin in the Management of Hyperlipidemia. Am J Cardiovasc Drugs. (2017) 17:169–81. doi: 10.1007/s40256-016-0205-0
85. Page M, Watts G. PCSK9 inhibitors – mechanisms of action. Aust Prescr. (2016) 39:164. doi: 10.18773/austprescr.2016.060
86. Liu X, Bao X, Hu M, Chang H, Jiao M, Cheng J, et al. Inhibition of PCSK9 potentiates immune checkpoint therapy for cancer. Nature. (2020) 588:693–8. doi: 10.1038/s41586-020-2911-7
87. Wei S, Espenshade P. Lipids: Cholesterol Synthesis and Regulation. In: Encyclopedia of Biological Chemistry III. Wei S, Espenshade P. Lipids: Cholesterol Synthesis and Regulation. In: Encyclopedia of Biological Chemistry III. Third. (2021) p. 732–8. Available at: https://www.sciencedirect.com/science/article/pii/B9780128194607000219
88. Di Minno A, Lupoli R, Calcaterra I, Poggio P, Forte F, Spadarella G, et al. Efficacy and Safety of Bempedoic Acid in Patients With Hypercholesterolemia: Systematic Review and Meta-Analysis of Randomized Controlled Trials. J Am Heart Assoc. (2020) 9:e016262. doi: 10.1161/JAHA.119.016262
89. Bhatt DL, Steg PG, Miller M, Brinton EA, Jacobson TA, Ketchum SB, et al. Cardiovascular Risk Reduction with Icosapent Ethyl for Hypertriglyceridemia. N Engl J Med. (2019) 380:11–22. doi: 10.1056/NEJMoa1812792
90. McAlister R, Aston J, Pollack M, Du L, Koyama T, Chism D. Effect of Concomitant pH-Elevating Medications with Pazopanib on Progression-Free Survival and Overall Survival in Patients with Metastatic Renal Cell Carcinoma. Oncologist. (2018) 23:686–92. doi: 10.1634/theoncologist.2017-0578
91. Haouala A, Widmer N, Duchosal MA, Montemurro M, Buclin T, Decosterd LA. Drug interactions with the tyrosine kinase inhibitors imatinib, dasatinib, and nilotinib. Blood. (2011) 117:e75–387. doi: 10.1182/blood-2010-07-294330
92. Thompson PD, Panza G, Zaleski A, Taylor B. Statin-Associated Side Effects. J Am Coll Cardiol. (2016) 67:2395–410. doi: 10.1016/j.jacc.2016.02.071
93. Schierwagen R, Uschner FE, Magdaleno F, Klein S, Trebicka J. Rationale for the use of statins in liver disease. Am J Physiol Gastrointest Liver Physiol. (2017) 312:G407–12. doi: 10.1152/ajpgi.00441.2016
94. Cavallo J,. Building Onco-Primary Care to Close the ‘Black Hole' in Cancer Survivorship Care A Conversation With Kevin Oeffinger, MD. The ASCO Post (2020). Available online at: https://ascopost.com/issues/march-10-2020/building-onco-primary-care-to-close-the-black-hole-in-cancer-survivorship-care/ (cited March 26, 2022).
Keywords: dyslipidemia, cholesterol, cancer, cardiovascular risk reduction, cancer survivor
Citation: de Jesus M, Mohammed T, Singh M, Tiu JG and Kim AS (2022) Etiology and Management of Dyslipidemia in Patients With Cancer. Front. Cardiovasc. Med. 9:892335. doi: 10.3389/fcvm.2022.892335
Received: 08 March 2022; Accepted: 31 March 2022;
Published: 25 April 2022.
Edited by:
Avirup Guha, Augusta University, United StatesReviewed by:
Maria Sol Andres, Royal Brompton Hospital, United KingdomDaniel Chen, University College London, United Kingdom
Nickolas Stabellini, Case Western Reserve University, United States
Copyright © 2022 de Jesus, Mohammed, Singh, Tiu and Kim. This is an open-access article distributed under the terms of the Creative Commons Attribution License (CC BY). The use, distribution or reproduction in other forums is permitted, provided the original author(s) and the copyright owner(s) are credited and that the original publication in this journal is cited, in accordance with accepted academic practice. No use, distribution or reproduction is permitted which does not comply with these terms.
*Correspondence: Agnes S. Kim, YWtpbSYjeDAwMDQwO3VjaGMuZWR1