- 1Division of Endocrinology, Metabolism and Molecular Medicine, Department of Internal Medicine, Charles R. Drew University of Medicine and Science, Los Angeles, CA, United States
- 2David Geffen School of Medicine, University of California, Los Angeles, Los Angeles, CA, United States
- 3Research and Development Service, Bay Pines VA Healthcare System, Bay Pines, FL, United States
- 4Laboratorio de Neurobiología, Facultad de Ciencias de la Salud, Universidad San Sebastián, Concepción, Chile
- 5Friends Research Institute, Cerritos, CA, United States
Electronic cigarettes or e-cigarettes are the most frequently used tobacco product among adolescents. Despite the widespread use of e-cigarettes and the known detrimental cardiac consequences of nicotine, the effects of e-cigarettes on the cardiovascular system are not well-known. Several in vitro and in vivo studies delineating the mechanisms of the impact of e-cigarettes on the cardiovascular system have been published. These include mechanisms associated with nicotine or other components of the aerosol or thermal degradation products of e-cigarettes. The increased hyperlipidemia, sympathetic dominance, endothelial dysfunction, DNA damage, and macrophage activation are prominent effects of e-cigarettes. Additionally, oxidative stress and inflammation are unifying mechanisms at many levels of the cardiovascular impairment induced by e-cigarette exposure. This review outlines the contribution of e-cigarettes in the development of cardiovascular diseases and their molecular underpinnings.
Introduction
Tobacco consumption has been present in the Americas since prehistoric times (1). After tobacco was introduced from indigenous inhabitants of the Western hemisphere to Europeans through members of Columbus' crew (1), the effects of tobacco use have been a subject of scientific controversy. In the 16th century, the Spaniard physician and botanist Nicolás Monardes published a book explaining the therapeutic effects of tobacco use for dozens of health problems (2). Although in the 17th century, King James I of England spoke about the dangerous effects of tobacco (3), the systematic observations of the negative health effects of tobacco took longer than expected. In the first part of the 20th century, pathologists observed a strong association between lung cancer and cigarette smoking (4). The rate of smoking peaked in the 60s, with about 42% of the adult population in the United States being tobacco smokers in 1965 (5). By 2019, however, the smoking rate among adults aged 18 years or older went down to 14.0% (6). Currently, cigarette smoking is still the leading cause of preventable death, contributing to chronic obstructive pulmonary disease, several types of cancer, diabetes, and cardiovascular disease (CVD) (7), and is an additive risk factor in COVID-19 (8).
Although the use of cigarettes has decreased in the last few years, addiction to nicotine has continued due to the introduction of electronic cigarettes or e-cigarettes to the market. In 2004, a Beijing-based company, Ruyan Group (Holdings) Ltd., China, patented and launched e-cigarettes (9) that delivered nicotine to users without burning tobacco (10). E-cigarettes are battery-powered devices that produce an aerosol generated by heating a solution (e-liquid) consisting of nicotine, glycerol, propylene glycol, and flavors. The first generation of e-cigarettes tried to mimic the experience of smoking conventional cigarettes. The later generation devices contain high-powered atomizers and use higher nicotine concentrations in the e-liquids, increasing the speed of delivery and yield of nicotine like conventional cigarettes (11). JUUL and other pod-mods use nicotine formulations derived from the nicotine salts in loose-leaf tobacco (12, 13). Nicotine salts in pod-mods such as JUUL reduce harshness and result in a satisfying experience even at high nicotine concentrations (14). Although e-cigarettes were initially marketed as a smoking cessation tool, they will likely lead to future conventional cigarette smoking in people that have never smoke (15, 16). Especially concerning is the effect of e-cigarettes on the youth, and it is noteworthy that 19.6% of high school students used e-cigarettes in 2020 (17). Smokers who use e-cigarettes in an attempt to stop smoking often end up using both products. These dual users have been found to have higher cardiovascular risk factors than single users (18).
Smoking is associated with 11% of cardiovascular deaths worldwide (19). Currently, heart disease is the leading cause of death in the United States. Atherosclerosis is a chronic inflammatory condition associated with the accumulation of lipids and fibrous elements in the arteries where inflammatory cells are recruited to the arterial walls. The effects of conventional cigarettes on the cardiovascular system have been extensively studied (20, 21). Although e-cigarettes have the potential to be less harmful than conventional cigarettes due to their reduced number of harmful chemicals, the precise toxicological and mechanistic data of the effects e-cigarettes have on the cardiovascular system remain to be elucidated. A cross-sectional analysis of cardiovascular symptoms showed that e-cigarette users have a higher risk of coronary heart disease, arrhythmia, chest pain, or palpitations (22).
One of the complexities of studying the cardiovascular effects of e-cigarettes is the large variety of devices and chemical compositions of e-liquids. Different e-cigarettes' devices may produce different chemical products by thermal degradation of e-cigarette liquids and differences in the size of fine particulate matter (PM2.5) or ultrafine particles (UFPs). The negative effects of PM2.5 and UFPs on the cardiovascular system are well-established (23, 24). In indoor studies, e-cigarettes produce PM2.5 and UFPs concentrations ~45 and 20 times higher, respectively, than recommended by the World Health Organization (25). Additionally, the heating temperature in e-cigarettes creates metal particles (copper, nickel, and silver) from the atomizer unit (26) that are delivered into the bloodstream through the lungs.
The literature on the consequences of second-hand e-cigarette vaping is limited. However, chemical components are partially exhaled by users of electronic cigarettes. Consequently, it is important to study the effect of second-hand e-cigarette aerosol on CVD (27). Furthermore, exhaled nicotine and other e-liquid components can deposit onto surfaces and subsequently negatively affect the health of those exposed (third-hand exposure). In addition, toxicological studies of the components of e-cigarettes such as glycerol, propylene glycol, and artificial flavors have been mainly tested via oral administration, but very few studies have investigated the effect of aerosolizing the content of e-cigarettes at a high temperature. It is also widely known that E-liquid thermal decomposition produces the breakdown of glycerol and propylene glycol into toxic aldehydes, acetaldehyde, acrolein, and formaldehyde (28, 29). Acrolein produces lipid peroxidation and modifies the component of high-density lipoprotein (HDL) Apolipoprorein-I (ApoA-I). This fact leads to speculation on the role of chronic e-cigarette consumption in the development of atherosclerosis (30). Additionally, acrolein produces vascular oxidative stress (31) and platelet activation, a risk factor for thrombotic vascular events (32). Fortunately, the concentrations of acrolein produced by e-cigarettes are likely to be too low to have effects of clinical relevance (33). Given that the concentration of the carbonyl compounds positively correlates with the voltage and temperature of e-cigarettes (34), tight monitoring of the acrolein production from e-cigarettes may be necessary.
Increasing the complexity of the toxicological analysis of e-cigarettes is the existence of more than 7,000 flavors for e-liquids in the market (35). Most of these thousands of flavors have been tested for oral ingestion safety; however, there is no complete data on their safety of the exposure to these components once heated and inhaled. For instance, experimental evidence shows that vanillin, cinnamaldehyde, eugenol, and acetylpyridine flavors induce nitric oxide and pro-inflammatory interleukins in endothelial cells (36), and vanilla custard e-vapor extract increased necrotic and apoptotic HL-1 cardiomyocyte cells (37). Adding to this complexity, e-cigarette users modify devices and solutions, which may further impact the toxicological characteristic of e-cigarettes (38). Figure 1 summarizes the proposed mechanisms of e-cigarettes' effects on the cardiovascular system.
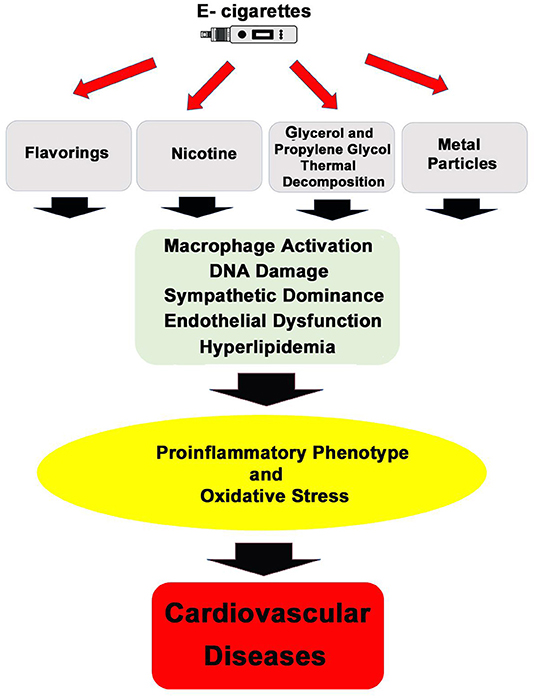
Figure 1. General mechanisms of action by which inhalation of e-cigarettes' aerosol can promote cardiovascular diseases.
Nicotine
Nicotine is the most studied biologically active chemical present in e-cigarettes, and several of the cardiovascular effects of e-cigarettes have been attributed to this alkaloid from the tobacco plant (39). Nicotine is a highly addictive drug, having well-established effects on the metabolism (40–42) and cardiovascular system (7, 39, 43). Higher levels of nicotine in e-cigarettes have been associated with an increase in the frequency and intensity of combustible cigarette smoking (44).
The acute and chronic effects of nicotine differ as chronic exposure induces fast desensitization of the nicotine receptors. For instance, although nicotine in e-cigarettes can acutely increase blood pressure, chronic smoking has not been linked to higher blood pressure in most epidemiological studies (45). Nicotine has systemic hemodynamic effects that are mediated by the activation of the sympathetic nervous system. Thus, acute nicotine treatment can stimulate cardiac output by producing systemic vasoconstriction and increasing heart rate (45). Nicotine activates macrophages which infiltrate atherosclerotic lesions and release cytokines such as the TNF-1β and IL-1β that increase inflammation (46). The most common complication of atherosclerosis is the formation of a thrombus that leads to a stroke or a myocardial infarction. Nicotine has thrombogenic activity by activating platelets and coagulation cascades (20).
Nicotinic Acetylcholine Receptors
Nicotine binds to the nicotinic acetylcholine receptors (nAChRs), which are integral membrane proteins that belong to the ligand-gated ion channel superfamily (47). Several nAChRs subunit combinations can form great diversity of functional receptors with a variety of specialized functions and properties depending on the cell type (48). Although the roles of nAChRs in synaptic transmission in the central (CNS) and peripheral nervous systems (PNS) are the most studied, nAChRs are present in several non-neuronal cells (49). In the cardiovascular system, vascular endothelial cells and smooth muscle cells (VSMC) express several subunits of nAChRs (50, 51). The activation of endothelial cells can lead to the release of vasoconstrictor substances. For instance, nicotine increases the release of endothelin-1 from human umbilical vein endothelial cells (52). Carotid arteries treated with nicotine show an impairment of endothelial-dependent relaxation associated with a decreased eNOS expression (53). In aortic smooth muscle cells, nicotine enhances insulin-induced mitogenesis through up-regulation of α7nAChR, a phenomenon associated with atherosclerosis (54). Additionally, nicotine has pro-angiogenic effects through the activation of α7nAChR (55). Nicotine produces arterial stiffness through extracellular matrix remodeling by upregulating matrix metalloproteinases (56). In the right ventricle, nicotine treatment lead to α7nAChR activation and fibroblast proliferation, collagen production, and extracellular matrix remodeling (57). Therefore, genetic or pharmacological inhibition of the α7nAChR rescues the effects of nicotine on right ventricular fibrosis (57).
pH and Nicotine
E-cigarettes are formulated to have different pH levels, perhaps designed to increase their sensory impact (58). Traditional e-cigarette products use e-liquid with free-base nicotine while a new generation of e-cigarettes, the pod-mods, such as JUUL, use nicotine salts (12). The pH of the E-liquid is a function of the concentration of the free-base nicotine and the concentration of the nicotine salt. If the E-liquid contains free-base nicotine, the pH is high. If the E-liquid contains nicotine salt, the pH can be lower as a range from 5 to 7 (12). Previous studies have reported that the pH of e-liquids ranges from 8 to 10 for conventional e-cigarettes (59) and around 6 for JUUL (12, 60). Regarding the health effects, two aspects of e-liquid pH are often discussed: (1) Different sensory experiences. High pH e-cigarette aerosol appeared to be harsher, while lower pH close to physiological levels provides a more satisfying experience (13, 14). (2) Nicotine in an aqueous solution can exist in two main forms: monoprotonated [NicH+] and unprotonated [Nic] forms. The ratio of the concentrations of the unprotonated vs. protonated nicotine [Nic]/[NicH+] is a function of pH (the Henderson–Hasselbalch equation):
Where pKa is the logarithmic acid dissociation constant. Protonated nicotine is the ligand of nAChR (61). Theoretically, high protonated nicotine [NicH+] in the e-cigarette aerosol can have a greater impact on cells expressing the nAChRs in the respiratory tract (12). On the other hand, unprotonated nicotine [Nic] is lipophilic; thus, following inhalation, it would more readily diffuse across pulmonary cell membrane (12). High [Nic] can induce a rapid rising phase and higher peak concentrations in the arterial blood, and as a consequence, greater cardiovascular effects, increasing the risk of cardiovascular events such as cardiac arrhythmia, fluctuations of blood pressure and disruption of hemodynamic processes (62).
Nicotine and Metabolic Syndrome
Smoking is a risk factor for insulin resistance in a dose-dependent manner (63). Smokers have lower glucose uptake and are more insulin resistant compared to nonsmokers (64). They also have higher plasma triglyceride (TG) and lower HDL-cholesterol levels (64). These findings on insulin resistance in smokers have been reproduced (65–71) and support other studies showing that insulin resistance can lead to both dyslipidemia (72) and endothelial dysfunction (73) seen in smokers. The degree of insulin resistance was also positively correlated to tobacco consumption (66), and, in long-term users of nicotine gum, to serum cotinine levels. Cotinine is a metabolite from nicotine; serum or urine levels of cotinine are considered to reflect the degree of nicotine use (63, 74). This implies nicotine as the causative agent of insulin resistance. In contrast to its effects on insulin sensitivity, cigarette smoking does not affect insulin secretion (64, 75, 76). Even acute smoking (one cigarette) impaired insulin sensitivity in healthy young men (66) and impaired glucose tolerance and insulin sensitivity in both smokers and non-smokers (77). Data from the Copenhagen male study indicated that only those smokers who have characteristic dyslipidemia associated with insulin resistance were at greatly increased CVD risk (78). Accordingly, with the effect of nicotine, e-cigarette users have higher fasting glucose levels than never users (79). In large-population-based studies, cigarette smoking was associated with an increased incidence of Type 2 diabetes mellitus (DM) (80) and metabolic syndrome [as defined by the National Cholesterol Education Program (81)]. A systematic meta-analysis confirmed the association between smoking and DM (82), and an editorial suggested that 12% of DM in the US is attributable to smoking (83). Cigarette smokers who were insulin sensitive did not display any abnormalities of lipoprotein metabolism (84). In contrast, cigarette smokers who were also insulin resistant had significantly higher plasma concentrations of TG and VLDL-cholesterol. Insulin resistance predicts the development of age-related diseases, including hypertension, stroke, coronary artery disease, cancer, and type 2 DM (85). Thus, it can be argued that a defect leading to increased CVD risk in smokers is insulin resistance and that the multiple adverse consequences associated with insulin resistance, including dyslipidemia and endothelial dysfunction, are responsible for the accelerated atherogenesis in these individuals (86).
Impact of e-Cigarette Exposure on the Generation of Oxidative Stress and Inflammation
Atherosclerosis is a chronic inflammatory condition associated with the accumulation of lipids and fibrous elements in the arteries where inflammatory cells are recruited to the arterial walls. Increased production of reactive oxygen species (ROS) is a unifying mechanism for several risk factors that induce arteriosclerosis, endothelial cell dysfunction, and cardiac dysfunction (87–91). Increased ROS can produce activation of pro-apoptotic signaling resulting in cardiac remodeling and dysfunction (92). Mitochondria are both a major source of ROS and the primary target of ROS damage (93). Given that mitochondrial DNA (mtDNA) lacks protection from histones and has proximity to the source of ROS, it is very susceptible to oxidative alterations of nucleotides in the sequence of its coding regions (94). Thus, mtDNA mutations can lead to mitochondrial dysfunction and inefficient energy production of cardiac cells (95). Chronic ROS production results in the accumulation of mtDNA mutations, oxidized proteins, and lipids, leading to mitochondrial dysfunction and energy deficits in the heart (96).
E-cigarettes induce increased ROS in vitro and in vivo in endothelial cells (97), which leads to DNA damage (97), mt DNA mutations (39), and lipid peroxidation, all of which indicate oxidative stress and ROS- mediated damage of cells. ROS can directly impair the nitric oxide (NO)-mediated vasorelaxation by quenching NO (98). Exposure to e-cigarettes aerosols for 12 weeks induced an inflammatory phenotype consisting in high levels of lipid peroxidation and mitochondrial DNA mutations in a nicotine-dependent manner (39). Recently, it has been reported an increase in both lipid peroxidation and inflammation induced by e-cigarettes were associated with heart fibrosis in rats (99).
The role of inflammation in the development of atherosclerosis (100) and heart failure (101) is well-established. Transcriptomic analysis of hearts exposed to e-cigarettes showed that mice exposed to e-cigarettes had dysregulation of signaling factors involved in inflammation, circadian rhythm regulation, and leukocyte extravasation (39). In primary microvascular endothelial cells, e-cigarettes, and conventional cigarettes decreased the expression of the tight junctional protein Zonula Occludens-1 (ZO-1), suggesting that they alter the blood-brain barrier integrity (102). This effect was also accompanied by the activation of Nuclear factor-erythroid factor 2-related factor 2 (Nrf2), a main cellular transcription factor of the oxidative stress response, and Platelet endothelial cell adhesion molecule 1 (PECAM-1), a pro-inflammatory adhesion molecule (102). Also, this study revealed an upregulation of the inflammatory proteins, Intercellular adhesion molecule-1 (ICAM-1), and Vascular cell adhesion protein 1 (VCAM-1) in the brain homogenates of mice exposed to e-cigarettes (102).
Platelets from healthy volunteers exposed to e-cigarettes aerosol show an increase in the expression of globular complement protein C1q receptor (gC1qR) and calreticulin cC1q receptor (cC1qR), two proteins that are associated with the atherosclerotic events, platelet activation and aggregation. In a randomized crossover trial, 25 tobacco smokers were exposed to sham vaping, e-cigarettes without nicotine, and e-cigarettes with nicotine. Plasma myeloperoxidase, an enzyme highly expressed in neutrophils and macrophages used as a marker of an inflammatory process (103), was increased after exposure to e-cigarettes with nicotine, but not in patients exposed to sham vaping or e-cigarettes without nicotine (43). Other studies have shown increased inflammatory and oxidative stress in non-smokers even when exposed to e-cigarettes without nicotine (104). These data suggest a clear effect of e-cigarettes with nicotine on the production of oxidative stress and inflammation; however, further work is needed to uncover the effects of the non-nicotine e-cigarette contributions to atherosclerosis.
Blood Lipids
High levels of triglycerides and low levels of high-density lipoproteins (HDL) are risk factors for cardiovascular disease (105, 106). Nicotine promotes loss of body weight and the disturbance of lipoprotein metabolism through the secretion of catecholamines, such as norepinephrine. Catecholamine secretion favors the elevation of LDL and very low density lipoproteins (VLDL) and is also associated with decreased HDL levels (107–109). Higher levels of LDL and VLDL are also known risk factors for cardiovascular diseases (110–112). A health survey in Korean men showed significantly elevated triglyceride levels in dual users of conventional cigarettes and e-cigarettes compared to non-smokers (18). In addition, there was no significant difference in triglyceride levels between dual users and conventional cigarette-only smokers. However, another work has shown that e-cigarette users have higher triglycerides and lower HDL than never users (79). HDL cholesterol was significantly lower in both dual users and conventional cigarette only smokers compared to those who never have smoked (18). E-cigarette vapers had increased levels of LDL and VLDL compared to nonsmokers (113). Habitual e-cigarette users had increased oxidized LDL levels compared with non-user control individuals (114). Oxidized LDL can lead to atherosclerosis as it can contribute to the buildup of atherosclerotic plaques (20). In a gender-specific effect, a plasma lipidome analysis showed that female but not male e-cigarette users had decreased plasmalogens levels (115). Plasmalogens have a protective role against lipid peroxidation (116). Clinical trials, animal experiments, and surveys have shown associations between e-cigarette use and negative health outcomes concerning blood lipids and potential cardiovascular diseases; however, more clinical, animal, and epidemiological studies will be needed to establish causation with these negative health outcomes.
Free Fatty Acids
Adipocyte dysfunction produces systemic inflammation, a pathogenic mechanism underlying the well-known associations between obesity, cardiovascular pathology, hypertension, and metabolic syndrome (117). Thus, adipose tissue, an important regulator of the cardiovascular system (118, 119), produces bioactive factors that regulate lipid levels and is involved in inflammation, oxidative stress, and insulin resistance (120). Epidemiological studies have shown that the combination of smoking and obesity results in a higher mortality risk (121). Additionally, smokers have a subclinical systemic inflammation with decreased adiponectin levels in plasma (122). The role of the adipose tissue on the effects of smoking in vascular pathology is highlighted by evidence showing that the epicardial adipose tissue and subcutaneous adipose tissue in smokers has higher levels of inflammatory adipokines, namely TNF-α and IL-6 than found in this tissue in non-smokers (123).
In cultured 3T3L1 adipocytes, nicotine-induced AMP-activated protein kinase (AMPK) phosphorylation, lipolysis, and oxidative stress in a concentration-dependent manner (124). Furthermore, the activation of nAChRs by nicotine stimulated AMPK activation led to the release of free fatty acids (FFAs) from rodent adipocytes (125–128). Additionally, systemic administration of nicotine produced lipolysis by inducing the release of catecholamines that bind to β-adrenergic receptors located in adipocytes (129).
In humans, cigarette smoking and obesity have been associated with increased levels of FFAs (130), which, in turn, are correlated with an increased risk for CVD (131, 132). FFAs have been broadly studied in their contribution to the induction of metabolic changes that lead to metabolic syndrome (133) and adverse cardiovascular outcomes (134). Increased FFAs produce a low inflammatory state that is characterized by infiltration and expansion of lymphocytes and macrophages, which produce pro-inflammatory cytokines that interfere with insulin signaling (135). FFAs are likely one of the key elements in ectopic lipid accumulation, lipotoxicity, mitochondrial dysfunction (136–138), and cardiomyopathy (132, 139). Improvements in whole-body insulin sensitivity can be obtained by pharmacological reduction of chronically elevated plasma FFA levels (140, 141). Our laboratory has shown that e-cigarettes produce increased plasma levels of FFA and intramyocardial lipid accumulation (39). Additionally, we have shown that inhibition of lipolysis using acipimox inhibited the hepatic metabolic changes induced by consuming a high-fat diet plus nicotine (142). Increased FFA promotes inflammation in adipose tissue through the activation of the toll-like receptor 4 (TLR4) signaling (118, 143). Our team found that e-cigarettes induce a cardiac inflammatory phenotype associated with cardiac dysfunction and atherosclerosis (39). Associated with this phenotype, we also found increased levels of serum FFA and oxidative stress (39). Therefore, we postulate that the nicotine present in e-cigarettes increases the levels of FFA and ROS, leading to atherosclerosis and cardiac dysfunction (39).
In a hyperlipidemic, low-density lipoprotein receptor null mouse model, nicotine stimulated macrophages to secrete inflammatory cytokines, creating a pro-inflammatory microenvironment in the sub-endothelium that increased the aortic lesion size by 2.5 times (46). Human monocytes are sensitive to cigarette smoking, NF-κB activation, and the production of pro-inflammatory cytokines such as IL-8 (144). After infiltration in the arterial wall, monocytes differentiate into macrophages and engulf oxidized low density lipoproteins (LDL) with the help of scavenger receptors creating foam cells, which secrete cytokines that, in turn, recruit more immune cells (145). In Apolipoprotein E (ApoE) null mice, e-cigarettes induced a cardiac inflammatory phenotype associated with increased serum levels of FFA and atherosclerosis (39). Together, this evidence suggests that the effects of nicotine and e-cigarettes on FFA, adipokines, and inflammatory cells are potent modulators of cardiovascular physiology. We depict a possible mechanism of these effects in Figure 2. Aside from the increase of FFA by e-cigarettes, the reported changes in adipose tissue by nicotine warrant further examination of the role of adipocyte tissue on the recruitment and activation of immune cells to the heart and vasculature.
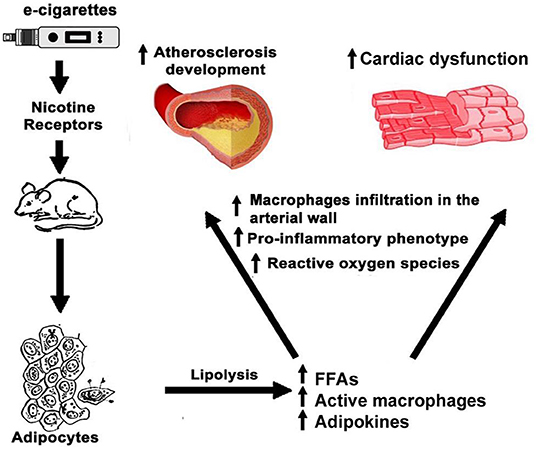
Figure 2. Effects of e-cigarettes on adipocytes and cardiovascular system. In adipocyte tissue, nicotine in e-cigarettes produces the release of FFA and adipokines leading to the activation of macrophages and an inflammatory phenotype that detrimentally affects the cardiovascular system function.
Sympathetic Dominance
Cardiac sympathetic activation is a risk factor for cardiovascular disease (146), and habitual e-cigarette users have an increased activation sympathetic system (114). Accordingly, e-cigarettes have been reported to produce sympathetic dominance in humans (114) and mouse models (147). Sympathetic and parasympathetic terminals innervate sinoatrial (SA) and atrioventricular (AV) nodes in the heart. In contrast, arteries and veins only receive sympathetic innervation (148). nAChRs are the key mediators of synaptic transmission in autonomic ganglia (148). Nicotine binding to nAChRs led to a release of adrenaline from the adrenal medulla and noradrenaline from postganglionic sympatric nerves, activating the sympathetic nervous system (7, 149). Blood vessels have α1 and β2 adrenoreceptors, which produce vasoconstriction and vasodilatation, respectively. Since the higher presence of α1 receptor in vessels, high adrenaline concentrations induce vasoconstriction through activation of α1 receptors (148). β1 adrenergic receptors are expressed in the heart, and their activation leads to increased cardiac contractility and heart rate (148). The chronic activation of the sympathetic system produces an increased cardiac overload and inflammation, leading to cardiac remodeling (150). Consistently with the relevance of this mechanism, β-blockers reduce heart failure mortality (151). Inhaling e-cigarettes (JUUL with nicotine) acutely increased sympathetic neural outflow in young, healthy non-smokers. In contrast, inhalation of a placebo e-cigarette without nicotine elicited no sympathetic dominance (152). Therefore, the sympathetic dominance produced by e-cigarettes is mainly produced by nicotine.
Sympathetic dominance activates the Splenocardiac axis. In this pro-inflammatory axis, the sympathetic stimulation of hematopoietic tissues increases circulating pro-inflammatory monocytes, increasing atherosclerosis and ischemic heart disease (153). The finding that e-cigarettes activated metabolic activity on spleen and blood vessel walls suggests the activation of the Splenocardiac axis (154).
Sympathetic activity plays a central role in the control of blood pressure. The acute effect of nicotine producing increased blood pressure has been well-researched through independent studies on nicotine and other cigarette studies (155–158). Inhalation of nicotine aerosol equivalent to cigarette smoking induces acute high magnitude irregular fluctuations of blood pressure in a pregnant rat model, with the arterial blood pressure being continuously measured (62). The irregular fluctuations of blood pressure primarily result from nicotine-induced cardiac arrhythmia (62) and probably can not be detected by the intermittent measurement with the conventional Korotkoff method. However, several epidemiological studies have found inconsistent results in comparing blood pressure levels among traditional cigarette smokers and non-smokers (159). E-cigarettes release nicotine and, after thermal degradation of propylene glycol and glycerin, release aldehydes (160), all of which can potentially cause an acute increase in blood pressure (161). As mentioned above, nicotine elevates blood pressure through the release of norepinephrine and epinephrine (162). The effects of aldehydes derived from the use of e-cigarettes are less studied, and the current potential effects and mechanisms are inferred from animal studies directly exposed to aldehydes. In rats, inhaled aerosol of acetaldehydes and propionaldehydes increased blood pressure by activating the sympathetic nervous system through the stimulation of the release of catecholamines (163). Previous studies have shown that aliphatic aldehydes, besides formaldehyde, had sympathomimetic activity. Aliphatic aldehydes most likely regulate blood pressure by the activation of the sympathetic system found in a study with anesthetized rats described above (163). More studies are needed to further understand the discrepancy between the effects of different types of aldehydes and the potential acute and chronic effects on blood pressure.
A comparison study found similar rates of acute increase in blood pressure when comparing e-cigarette and conventional cigarette use in smokers (164, 165). E-cigarette devices as the vape pod, JUUL, increased the blood pressure by 6 mm Hg acutely in comparison to nicotine-free e-cigarettes (152). Nevertheless, the authors have mentioned that the 6 mmHg of increase could be underestimated because the participants were not experienced e-cigarette users, which could be less nicotine absorption (152). Compared with nonsmokers, conventional cigarettes and e-cigarettes users have a similar pattern of increase in systolic blood pressure, with lesser effects in e-cigarette users (166). A small double-blinded clinical trial revealed that e-cigarettes with nicotine, but not without nicotine increased the peripheral systolic blood pressure and heart rate in humans (167). Conventional smokers with arterial hypertension that switched to e-cigarettes showed improvements in systolic and diastolic blood pressure (168). Three other acute comparison studies found that e-cigarette users had decreased blood pressure when compared to conventional cigarette users (169–171). Overall, a meta-analysis of the immediate effects of e-cigarettes found that acute use of nicotine e-cigarettes was associated with increased heart rate, systolic blood pressure, diastolic blood pressure (172). In an acute study, following 45 min of exposure to e-cigarette aerosol, blood pressure levels rose to similar levels of those who smoked tobacco cigarettes for 15 min, suggesting that a longer time is needed for e-cigarette exposure than conventional cigarette exposure on blood pressure (167). One of the acute studies also used carotid- pulse wave velocity to show that an increase of e-cigarette usage from 5 to 30 min increased arterial stiffness to a similar level to that of tobacco smokers (164).
A mouse study indicated little changes in blood pressure among mice that were exposed to filtered air, e-cigarettes, and conventional cigarettes for 8 months (147). A human study that lasted 3.5 years, found that there was no significant difference in long-term changes in blood pressure when comparing daily e-cigarette users and non-users (173).
Most of the acute comparison studies investigated the temporary increase of blood pressure levels after a short period of e-cigarettes use, most likely due to sympathetic nervous system activation. There are still discrepancies in these acute studies on whether e-cigarette use induced a lower elevation in blood pressure when compared to the use of conventional cigarettes. More data is necessary to fully understand the chronic implications of e-cigarettes use and the potential effects of the ingredients on blood pressure.
Platelet Activation and Thrombogenesis
Platelet adhesion is a common trait of CVD (174). A whole-body e-cigarette mouse exposure protocol showed that e-cigarettes induce platelet activation with enhanced aggregation (169). Fine particulate matter also increased platelet adhesion and activation (175). Exposing mice to e-cigarettes for either 5 or 14 consecutive days increased the activation of platelets as well as shortened thrombosis after exposure to e-cigarettes (176).
Mice with short-term whole-body exposure to e-cigarettes developed a prothrombotic phenotype with hyperactive platelets and higher integrin and phosphatidylserine expression (176). E-cigarettes increased phosphorylated protein kinase B (Akt) and extracellular signal-regulated kinases (ERK), which are involved in platelet function (176, 177).
Conventional cigarettes and e-cigarettes produced a significant increase in platelet activation in non-smokers (178). However, there was also a lower increase of platelet aggregation following e-cigarette use than with conventional cigarette use (178). In a similar study comparing acute effects of conventional cigarette use and e-cigarette use, there was a similar increase in both groups of Nox2, a protein that regulates platelet-activation-associated thrombosis (165). In vitro studies of platelet exposure to e-cigarette aerosol also found increases in CD40 and P-selectin (175), which are markers for active platelets and thrombo-inflammation, respectively (161, 179). In in vivo studies, exposure to e-cigarettes for 5–7 days led to enhanced P-selectin levels after e-cigarette usage (176).
Clinical studies involving tobacco users with controlled conventional cigarette compared to e-cigarette with nicotine use showed acute increases in CD40 and P-selectin markers in conventional cigarette and e-cigarette users (165, 178, 180). Another study, looking at e-cigarette use with and without nicotine, found a similar increase of CD40 and P-selectin in users of e-cigarettes containing nicotine. In the groups that inhaled aerosol without nicotine, only CD40 increased (181). In summary, clinical and preclinical studies indicate that e-cigarettes consumption increases platelet aggregation, which can have a negative impact by potentiating cardiovascular events.
Vascular Trauma and Coronary Vascular Disease
The effects of conventional cigarettes in vascular injury and CVD are well-established (7). Daily users of e-cigarettes have an increased risk factor for myocardial infarction (182). This risk appeared similar between e-cigarette and conventional cigarette smokers and was increased in users of both cigarettes and e-cigarettes (dual users) (183). The Framingham Heart Study showed a strong association between aortic stiffness and a higher incidence of cardiovascular events (184). Arterial stiffening leads to increased cardiovascular risk, including heart failure, myocardial infarction, and increased mortality. Hemodamicaly, arterial stiffening leads to increased blood pressure, cardiac workload, and decreased myocardial perfusion. Structural components of the arterial wall mainly determine arterial stiffening. Estimation of arterial stiffness is commonly measured by aortic-femoral pulse wave velocity (PWV), that is, the time that it takes for the arterial pulse to propagate from the carotid to the femoral artery. Several studies have shown that e-cigarettes increase PWV in humans (43, 164, 185). Mice exposed to e-cigarettes for 5 days a week for 8 months showed increased aortic arterial stiffness measured by PWV (147).
To differentiate the vascular effects of nicotine and carriers, a single-blind crossover design study was performed with patients exposed to vaping without nicotine, vaping with nicotine, and sham-vaping. Results from these clinical studies showed that nicotine from e-cigarettes reduced microvessel endothelial function, increased arterial stiffness, and triggered an increase in plasma myeloperoxidase (43). Nicotine-free e-cigarettes did not change microcirculatory function as well as arterial stiffness and oxidative stress markers (43). In healthy volunteers, two biomarkers for heightened vascular risk, microvesicles, and endothelial progenitor cells, were increased following exposure to e-cigarettes (180), and these effects were shown to be dependent on nicotine (181). Ex vivo experiments of wire tension myography and force transduction showed an increased thoracic aortic tension in response to vasoactive-inducing compounds in mice chronically exposed to e-cigarettes (147). In animal models, mice were exposed for 60 weeks to the e-cigarette with a concentration of nicotine from 0 to 24 mg/mL showed endothelial dysfunction and an increase in endothelial ROS, in addition to a thickening of the vessel wall were dependent on nicotine concentration (186). Experiments in mice showed that e-cigarettes produced uncoupling of eNOS, and peroxynitrite formation may lead to vascular endothelial dysfunction (187). Additionally, ROS causes endothelial dysfunction by directly quenching NO (98). On the other hand, flow-mediated dilation, a marker for the presence of subclinical atherosclerosis, was studied in conventional cigarettes smokers, e-cigarettes smokers, and non-smokers. Conventional cigarette smokers develop impairment of flow-mediated dilation compared to non-smokers, and electronic cigarette smokers have similar flow-mediated dilation as non-smokers. Therefore, this work suggests that the impairment of flow-mediated vasodilation may be nicotine-independent (188). An article on the beneficial effects of the switch from traditional cigarettes to e-cigarettes showed an early beneficial impact on endothelial function after this switch (189). The improvement in flow-mediated dilatation was mainly observed in females and non-dual users with little effect in dual users (189). However, one potential limitation of this study is the lack of a non-smoker control group.
Pulse wave analysis (PWA) is a technique commonly used to determine systemic arterial stiffness. The primary outcome derived from PWA is the augmentation index (AIx), which is normalized to the heart rate. Several groups have shown an increase in the AIx after e-cigarette and conventional cigarette use (43, 167, 185). Chaumont et al. (43) associated these hemodynamic parameters and ROS changes to nicotine without the influence of the non-nicotine components in the e-cigarettes.
The two most studied mechanisms for the effect of conventional cigarettes on vasculature are oxidative stress and the promotion of a pro-inflammatory state (7). In endothelial cells, e-cigarette and conventional cigarette extracts produce DNA damage, ROS generation, and apoptosis; however, cytotoxic effects were blunted by antioxidants (97), suggesting a pivotal role of ROS. These effects were greater in conventional cigarettes than e-cigarettes (97). E-cigarette exposure caused endothelial dysfunction through ROS in human endothelial cells derived from induced pluripotent stem cells (iPSC), and their conditioned media induced a pro-inflammatory state in macrophages (190). These effects were potentiated in cinnamon-flavored products (190).
In human umbilical vein endothelial (HUVEC) cells, e-cigarettes produced complement deposition, a phenomenon present in atherosclerotic lesions (191). This inflammatory phenomenon was related to a reduction of metabolic activity of endothelial cells (191). Consistently, sections of aortic root stained with Oil Red O from ApoE KO mice exposed to e-cigarettes aerosol, producing blood cotinine levels equivalent to that found in heavy smokers, showed increased development of atherosclerotic lesions (192). Additionally, e-cigarettes induced an innate immune response associated with aberrant neutrophilic activation in human airway samples (193). A similar model of e-cigarette induced atherosclerosis showed that damaged mitochondrial DNA in circulating blood may produce the increase of Toll-like receptor 9 (TLR9), leading to increased pro-inflammatory cytokines and macrophages activation (194). Additionally, pharmacological inhibition of TLR9 can attenuate the e-cigarettes exacerbated atherosclerosis in ApoE KO mice (194). Therefore, growing literature shows evidence for e-cigarette-induced vascular injury.
Cardiac Function
Smoking is a significant predictor of mortality in people with heart failure (118, 195). The components of e-cigarettes or their heat-produced derivatives have been shown to have an effect on cardiac physiology. For instance, formaldehyde decreased in left ventricle end-systolic pressure and cardiac output (196), and acetaldehyde produced myocardial mitochondrial damage (197). Nicotine aerosol inhalation induces acute cardiac arrhythmia where sinoatrial (SA) block, sinus arrest, atrioventricular (A-V) block and supraventricular escape rhythm were demonstrated by ECG analysis in rats suggesting a disturbance of parasympathetic and sympathetic cardiac control mediated by the nAChRs (62). Mouse models for e-cigarette exposure with pharmacokinetics resembling human e-cigarettes have been developed (192). In the ApoE KO mice model exposed to 12 weeks of e-cigarettes with cotinine levels similar to the range of heavy smokers, RNA-seq analysis revealed dysregulation of inflammatory pathways (39). Additionally, M-Mode echocardiographic analysis showed a decreased left ventricular fractional shortening (LV%FS), ejection fraction (LVEF), and velocity of circumferential fiber shortening (VCF) in mice exposed to e-cigarettes with nicotine (39). However, exposure to e-cigarettes without nicotine did not affect cardiac function (39). Such changes in cardiac function were associated with increased ultrastructural abnormalities indicative of cardiac dysfunction and MDA generation, a marker of oxidative stress but without hypertrophy (39). These changes were not associated with changes in the gross morphology of the heart. Recently, C57BL/6J mice on an HFD were exposed to e-cigarettes in the presence (2.4% nicotine) or absence (0% nicotine) of nicotine and saline aerosol for 12 weeks (198). Indeed, we found a decrease in LV%FS, LVEF, and VCF coupled with ultrastructural abnormalities indicative of cardiomyopathy in mice treated with e-cigarette (2.4% nicotine) compared to e-cigarette (0% nicotine) or saline exposed mice (198). Therefore, nicotine seems to be necessary for the induction of systolic dysfunction induced by e-cigarettes in this mouse model (39). More extended exposure to e-cigarettes of 60 weeks, led to the development of cardiac left ventricular hypertrophy with an increased systemic vascular resistance (186). Accordingly, another study showed that mice exposed to e-cigarettes for 3 months had increased systolic blood pressure and diastolic blood pressure, associated with cardiac and renal fibrosis and a systemic inflammatory state (199). Recently, a similar phenotype produced by e-cigarettes in rats showed fibrosis, inflammation, and oxidative stress, but with cardiac hypertrophy (99).
Cardiovascular Studies of Smokers Using e-Cigarettes as a Tool for Smoking Cessation
A meta-analysis of cardiovascular outcomes of smokers switching from traditional cigarettes to e-cigarettes did not show any improvement in stroke, myocardial infarction, or coronary heart disease outcomes (200). However, this work showed a reduction in adverse respiratory effects in smokers who switched to e-cigarettes (200). Additionally, larger studies have shown that the smokers who do not halt smoking often continue using both conventional cigarettes and e-cigarettes (dual users) (18). Compared with those who only smoke conventional cigarettes, dual users have a higher cardiovascular risk (201).
Heat-Not-Burn Tobacco Cigarettes and CVD Safety
The tobacco industry's most recent development is a product deemed as a heat-not-burn (HnB) tobacco cigarettes (202, 203). These products claim to “heat” tobacco (rolled cast leaf sheets of tobacco soaked in propylene glycol) to temperatures around 350 Celsius rather than burn it at roughly 600°C. This creates an aerosol that contains nicotine which can be inhaled by users and is promoted to be less toxic and harmful than e-cigarette aerosol or smoke from combusted cigarettes (204). Phillip Morris International (PMI) is spearheading this new “technology.” Their new product (I-Quit-Ordinary-Smoking (IQOS) is being marketed to 1 day replace conventional cigarettes (205). Before this product gains traction, studies on its acute and long-term CVD safety are urgently needed.
Discussion: Conclusions and Future
Although the public endorses the perception that e-cigarettes are safe (206), their long-term effects on human health will take a long time to be fully elucidated. In the last several years, e-cigarettes have been progressively regulated. The tobacco prevention act gave authority to the Food and Drug Administration (FDA) to regulate the production, distribution, and marketing of e-cigarettes. The evolving nature of the presented data in this work calls for more regulation, which would include additional safety testing for new flavors and devices that continue to emerge. Studies comparing the CVD effect of e-cigarettes vs. conventional cigarettes and especially those who use e-cigarettes to attempt to quit conventional cigarettes are urgently needed.
The past several years have been important in establishing the effects of e-cigarettes on CVD. Although the reduction of conventional cigarettes harm by substituting them with e-cigarettes remains under discussion, the molecular mechanism of e-cigarette effects in the cardiovascular system clearly is emerging. The proposed mechanisms for the effects of e-cigarettes on CVD are shared with common diseases that affect the general population. Mechanisms such as oxidative stress, inflammation, lipid accumulation, and sympathetic dominance are commonly present in non-smoking patients with atherosclerosis and diabetic cardiomyopathy (207). This leads to increased concern for people with metabolic or cardiovascular comorbidities that use e-cigarettes. Therefore, these shared mechanisms call for future studies investigating the impact of e-cigarettes on the cardiovascular disease on susceptible populations or representative animal models of these conditions. Additionally, the variety of flavors and delivery systems that change in different vendors will need to be studied. Future discussions in the field may be informed by the different effects of nicotine on the variety of nAChRs and target organs.
Nicotine has a dichotomic role as the main substance in harm-reduction products and a harmful substance for the CVS. Therefore, the solution for stopping the deleterious effects of smoking is nicotine cessation and not shifting the source of nicotine delivery. Alternatively, e-cigarettes may be an imperfect, comparatively safer alternative to conventional cigarettes that could be used as a cessation tool; however, the efficiency of this intervention is still uncertain. As a requirement for a specific e-cigarette to be designed as safer than conventional cigarettes, they need to be compared to conventional cigarettes. E-cigarette devices and e-liquids keep changing, and the safety information obtained today may not be valid in a few years. Likely, the scientific discussion that started centuries ago about smoking and health for Dr. Monardes (2) is certain to continue with e-cigarettes. We hope that consensus will come in a shorter time than the one established for conventional cigarettes.
Author Contributions
JE-D and TF conceived and planned the work. JE-D, XMS, and TF wrote the manuscript. JE-D, XMS, CL, KH, JR, MJ, VE, KR, AS-H, and TF contributed to interpreting the literature and editing the manuscript. All authors contributed to the article and approved the submitted version.
Funding
This work was supported by the NIH grants: NIGMS (SC2GM135127), NIMHD (S21MD000103), NHLBI (R01HL135623), NIDA (R42DA044788), vouchers from the NIH Accelerating Excellence in Translational Science (AXIS) (U54MD007598), and NIDA (R25DA050723). California TRDRP grant (28CP-0040), and DODCDMRP grant (PR190942) to TF. VE was funded by an ANID-FONDECYT (1190264).
Conflict of Interest
The authors declare that the research was conducted in the absence of any commercial or financial relationships that could be construed as a potential conflict of interest.
Publisher's Note
All claims expressed in this article are solely those of the authors and do not necessarily represent those of their affiliated organizations, or those of the publisher, the editors and the reviewers. Any product that may be evaluated in this article, or claim that may be made by its manufacturer, is not guaranteed or endorsed by the publisher.
References
1. Musk AW, de Klerk NH. History of tobacco and health. Respirology. (2003) 8:286–90. doi: 10.1046/j.1440-1843.2003.00483.x
2. Monardes N, Clusius C. De Simplicibus Medicamentis ex Occidentali India Delatis, Quorum in Medicina Usus Est. Belgium: Antverpiae: Ex officina Christophori Plantini (1574). 88 p.
3. Gately I. Tobacco: The Story of How Tobacco Seduced the World. New York, NY: Grove Press (2001). 403 p.
4. White C. Research on smoking and lung cancer: a landmark in the history of chronic disease epidemiology. Yale J Biol Med. (1990) 63:29–46.
5. Giovino GA, Schooley MW, Zhu BP, Chrismon JH, Tomar SL, Peddicord JP, et al. Surveillance for selected tobacco-use behaviors–United States, 1900-1994. MMWR CDC Surveill Summ. (1994) 43:1–43.
6. Centers for Disease Control Prevention. Current Cigarette Smoking Among Adults in the United States. (2019). Available online at: https://www.cdc.gov/tobacco/data_statistics/fact_sheets/adult_data/cig_smoking/index.htm (accessed March 19, 2022).
7. How Tobacco Smoke Causes Disease: The Biology and Behavioral Basis for Smoking-Attributable Disease: A Report of the Surgeon General. Atlanta, GA: Publications and Reports of the Surgeon General (2010).
8. Gallo M, Street ME, Guerra F, Fanos V, Marcialis MA. A review of current knowledge on Pollution, Cigarette Smoking and COVID-19 diffusion and their relationship with inflammation. Acta Biomed. (2020) 91:e2020148. doi: 10.23750/abm.v91i4.10263
9. Hon L. A Non-Smokable Electronic Spray Cigarette (CA 2518174) (Patent Notice). Canada: Canadian Patent Office Record (2005).
10. Polosa R, Caponnetto P, Morjaria JB, Papale G, Campagna D, Russo C. Effect of an electronic nicotine delivery device (e-Cigarette) on smoking reduction and cessation: a prospective 6-month pilot study. BMC Public Health. (2011) 11:786. doi: 10.1186/1471-2458-11-786
11. Wagener TL, Floyd EL, Stepanov I, Driskill LM, Frank SG, Meier E, et al. Have combustible cigarettes met their match? The nicotine delivery profiles and harmful constituent exposures of second-generation and third-generation electronic cigarette users. Tob Control. (2017) 26:e23–e8. doi: 10.1136/tobaccocontrol-2016-053041
12. Shao XM, Friedman TC. Pod-mod vs. conventional e-cigarettes: nicotine chemistry, pH, and health effects. J Appl Physiol 1985. (2020) 128:1056–8. doi: 10.1152/japplphysiol.00717.2019
13. Shao XM, Friedman TC. Last word on viewpoint: pH Buffer capacity and pharmacokinetics: two remaining questions. J Appl Physiol 1985. (2020) 128:1063–4. doi: 10.1152/japplphysiol.00165.2020
14. Barrington-Trimis JL, Leventhal AM. Adolescents' Use of “Pod Mod” E-cigarettes - urgent concerns. N Engl J Med. (2018) 379:1099–102. doi: 10.1056/NEJMp1805758
15. Bold KW, Kong G, Camenga DR, Simon P, Cavallo DA, Morean ME, et al. Trajectories of E-cigarette and conventional cigarette use among youth. Pediatrics. (2018) 141:e20171832. doi: 10.1542/peds.2017-1832
16. Vogel EA, Cho J, McConnell RS, Barrington-Trimis JL, Leventhal AM. Prevalence of electronic cigarette dependence among youth and its association with future use. JAMA Netw Open. (2020) 3:e1921513. doi: 10.1001/jamanetworkopen.2019.21513
17. Gentzke AS, Wang TW, Jamal A, Park-Lee E, Ren C, Cullen KA, et al. Tobacco product use among middle and high school students - United States, 2020. MMWR Morb Mortal Wkly Rep. (2020) 69:1881–8. doi: 10.15585/mmwr.mm6950a1
18. Kim CY, Paek YJ, Seo HG, Cheong YS, Lee CM, Park SM, et al. Dual use of electronic and conventional cigarettes is associated with higher cardiovascular risk factors in Korean men. Sci Rep. (2020) 10:5612. doi: 10.1038/s41598-020-62545-3
19. Ezzati M, Henley SJ, Thun MJ, Lopez AD. Role of smoking in global and regional cardiovascular mortality. Circulation. (2005) 112:489–97. doi: 10.1161/CIRCULATIONAHA.104.521708
20. Csordas A, Bernhard D. The biology behind the atherothrombotic effects of cigarette smoke. Nat Rev Cardiol. (2013) 10:219–30. doi: 10.1038/nrcardio.2013.8
21. Jha P, Ramasundarahettige C, Landsman V, Rostron B, Thun M, Anderson RN, et al. 21st-century hazards of smoking and benefits of cessation in the United States. N Engl J Med. (2013) 368:341–50. doi: 10.1056/NEJMsa1211128
22. Wang JB, Olgin JE, Nah G, Vittinghoff E, Cataldo JK, Pletcher MJ, et al. Cigarette and e-cigarette dual use and risk of cardiopulmonary symptoms in the Health eHeart Study. PLoS One. (2018) 13:e0198681. doi: 10.1371/journal.pone.0198681
23. Burnett RT, Pope CA 3rd, Ezzati M, Olives C, Lim SS, Mehta S, et al. An integrated risk function for estimating the global burden of disease attributable to ambient fine particulate matter exposure. Environ Health Perspect. (2014) 122:397–403. doi: 10.1289/ehp.1307049
24. Terzano C, Di Stefano F, Conti V, Graziani E, Petroianni A. Air pollution ultrafine particles: toxicity beyond the lung. Eur Rev Med Pharmacol Sci. (2010) 14:809–21.
25. Li L, Lin Y, Xia T, Zhu Y. Effects of electronic cigarettes on indoor air quality and health. Annu Rev Public Health. (2020) 41:363–80. doi: 10.1146/annurev-publhealth-040119-094043
26. Williams M, Villarreal A, Bozhilov K, Lin S, Talbot P. Metal and silicate particles including nanoparticles are present in electronic cigarette cartomizer fluid and aerosol. PLoS One. (2013) 8:e57987. doi: 10.1371/journal.pone.0057987
27. Zhang Y, Sumner W, Chen DR. In vitro particle size distributions in electronic and conventional cigarette aerosols suggest comparable deposition patterns. Nicotine Tob Res. (2013) 15:501–8. doi: 10.1093/ntr/nts165
28. Jensen RP, Luo W, Pankow JF, Strongin RM, Peyton DH. Hidden formaldehyde in e-cigarette aerosols. N Engl J Med. (2015) 372:392–4. doi: 10.1056/NEJMc1413069
29. Ogunwale MA, Li M, Ramakrishnam Raju MV, Chen Y, Nantz MH, Conklin DJ, et al. Aldehyde detection in electronic cigarette aerosols. ACS Omega. (2017) 2:1207–14. doi: 10.1021/acsomega.6b00489
30. Shao B, Fu X, McDonald TO, Green PS, Uchida K, O'Brien KD, et al. Acrolein impairs ATP binding cassette transporter A1-dependent cholesterol export from cells through site-specific modification of apolipoprotein A-I. J Biol Chem. (2005) 280:36386–96. doi: 10.1074/jbc.M508169200
31. Kuntic M, Oelze M, Steven S, Kroller-Schon S, Stamm P, Kalinovic S, et al. Short-term e-cigarette vapour exposure causes vascular oxidative stress and dysfunction: evidence for a close connection to brain damage and a key role of the phagocytic NADPH oxidase (NOX-2). Eur Heart J. (2019) 41:2472–483. doi: 10.1093/eurheartj/ehz772
32. Sithu SD, Srivastava S, Siddiqui MA, Vladykovskaya E, Riggs DW, Conklin DJ, et al. Exposure to acrolein by inhalation causes platelet activation. Toxicol Appl Pharmacol. (2010) 248:100–10. doi: 10.1016/j.taap.2010.07.013
33. Mayer B. Acrolein exposure from electronic cigarettes. Eur Heart J. (2020) 41:1523. doi: 10.1093/eurheartj/ehaa002
34. Kosmider L, Sobczak A, Fik M, Knysak J, Zaciera M, Kurek J, et al. Carbonyl compounds in electronic cigarette vapors: effects of nicotine solvent and battery output voltage. Nicotine Tob Res. (2014) 16:1319–26. doi: 10.1093/ntr/ntu078
35. Zhu SH, Sun JY, Bonnevie E, Cummins SE, Gamst A, Yin L, et al. Four hundred and sixty brands of e-cigarettes and counting: implications for product regulation. Tob Control. (2014) 23(Suppl. 3):iii3–iii9. doi: 10.1136/tobaccocontrol-2014-051670
36. Fetterman JL, Weisbrod RM, Feng B, Bastin R, Tuttle ST, Holbrook M, et al. Flavorings in tobacco products induce endothelial cell dysfunction. Arterioscler Thromb Vasc Biol. (2018) 38:1607–15. doi: 10.1161/ATVBAHA.118.311156
37. Abouassali O, Chang M, Chidipi B, Martinez JL, Reiser M, Kanithi M, et al. In vitro and in vivo cardiac toxicity of flavored electronic nicotine delivery systems. Am J Physiol Heart Circ Physiol. (2021) 320:H133–H43. doi: 10.1152/ajpheart.00283.2020
38. Brown CJ, Cheng JM. Electronic cigarettes: product characterisation and design considerations. Tob Control. (2014) 23(Suppl. 2):ii4–ii10. doi: 10.1136/tobaccocontrol-2013-051476
39. Espinoza-Derout J, Hasan KM, Shao XM, Jordan MC, Sims C, Lee DL, et al. Chronic intermittent electronic cigarette exposure induces cardiac dysfunction and atherosclerosis in apolipoprotein-E knockout mice. Am J Physiol Heart Circ Physiol. (2019) 317:H445–H59. doi: 10.1152/ajpheart.00738.2018
40. Espinoza-Derout J, Shao XM, Bankole E, Hasan KM, Mtume N, Liu Y, et al. Hepatic DNA damage induced by electronic cigarette exposure is associated with the modulation of NAD+/PARP1/SIRT1 axis. Front Endocrinol (Lausanne). (2019) 10:320. doi: 10.3389/fendo.2019.00320
41. Hasan KM, Friedman TC, Shao X, Parveen M, Sims C, Lee DL, et al. E-cigarettes and Western diet: important metabolic risk factors for hepatic diseases. Hepatology. (2019) 69:2442–54. doi: 10.1002/hep.30512
42. Sinha-Hikim AP, Sinha-Hikim I, Friedman TC. Connection of nicotine to diet-induced obesity and non-alcoholic fatty liver disease: cellular and mechanistic insights. Front Endocrinol (Lausanne). (2017) 8:23. doi: 10.3389/fendo.2017.00023
43. Chaumont M, de Becker B, Zaher W, Culie A, Deprez G, Melot C, et al. Differential effects of E-cigarette on microvascular endothelial function, arterial stiffness and oxidative stress: a randomized crossover trial. Sci Rep. (2018) 8:10378. doi: 10.1038/s41598-018-28723-0
44. Goldenson NI, Leventhal AM, Stone MD, McConnell RS, Barrington-Trimis JL. Associations of electronic cigarette nicotine concentration with subsequent cigarette smoking and vaping levels in adolescents. JAMA Pediatr. (2017) 171:1192–9. doi: 10.1001/jamapediatrics.2017.3209
45. Benowitz NL, Burbank AD. Cardiovascular toxicity of nicotine: implications for electronic cigarette use. Trends Cardiovasc Med. (2016) 26:515–23. doi: 10.1016/j.tcm.2016.03.001
46. Lau PP, Li L, Merched AJ, Zhang AL, Ko KW, Chan L. Nicotine induces proinflammatory responses in macrophages and the aorta leading to acceleration of atherosclerosis in low-density lipoprotein receptor (-/-) mice. Arterioscler Thromb Vasc Biol. (2006) 26:143–9. doi: 10.1161/01.ATV.0000193510.19000.10
47. Picciotto MR, Caldarone BJ, King SL, Zachariou V. Nicotinic receptors in the brain. Links between molecular biology and behavior. Neuropsychopharmacology. (2000) 22:451–65. doi: 10.1016/S0893-133X(99)00146-3
48. Taly A, Corringer PJ, Guedin D, Lestage P, Changeux JP. Nicotinic receptors: allosteric transitions and therapeutic targets in the nervous system. Nat Rev Drug Discov. (2009) 8:733–50. doi: 10.1038/nrd2927
49. Albuquerque EX, Pereira EF, Alkondon M, Rogers SW. Mammalian nicotinic acetylcholine receptors: from structure to function. Physiol Rev. (2009) 89:73–120. doi: 10.1152/physrev.00015.2008
50. Moccia F, Frost C, Berra-Romani R, Tanzi F, Adams DJ. Expression and function of neuronal nicotinic ACh receptors in rat microvascular endothelial cells. Am J Physiol Heart Circ Physiol. (2004) 286:H486–H91. doi: 10.1152/ajpheart.00620.2003
51. Bruggmann D, Lips KS, Pfeil U, Haberberger RV, Kummer W. Rat arteries contain multiple nicotinic acetylcholine receptor alpha-subunits. Life Sci. (2003) 72:2095–9. doi: 10.1016/S0024-3205(03)00067-5
52. Lee WO, Wright SM. Production of endothelin by cultured human endothelial cells following exposure to nicotine or caffeine. Metabolism. (1999) 48:845–8. doi: 10.1016/S0026-0495(99)90216-0
53. Conklin BS, Surowiec SM, Ren Z, Li JS, Zhong DS, Lumsden AB, et al. Effects of nicotine and cotinine on porcine arterial endothelial cell function. J Surg Res. (2001) 95:23–31. doi: 10.1006/jsre.2000.6004
54. Wada T, Naito M, Kenmochi H, Tsuneki H, Sasaoka T. Chronic nicotine exposure enhances insulin-induced mitogenic signaling via up-regulation of α7 nicotinic receptors in isolated rat aortic smooth muscle cells. Endocrinology. (2007) 148:790–9. doi: 10.1210/en.2006-0907
55. Heeschen C, Weis M, Aicher A, Dimmeler S, Cooke JP. A novel angiogenic pathway mediated by non-neuronal nicotinic acetylcholine receptors. J Clin Invest. (2002) 110:527–36. doi: 10.1172/JCI0214676
56. Jacob-Ferreira AL, Palei AC, Cau SB, Moreno H Jr, Martinez ML, et al. Evidence for the involvement of matrix metalloproteinases in the cardiovascular effects produced by nicotine. Eur J Pharmacol. (2010) 627:216–22. doi: 10.1016/j.ejphar.2009.10.057
57. Vang A, da Silva Goncalves Bos D, Fernandez-Nicolas A, Zhang P, Morrison AR, Mancini TJ, et al. α7 Nicotinic acetylcholine receptor mediates right ventricular fibrosis and diastolic dysfunction in pulmonary hypertension. JCI Insight. (2021) 6:e142945. doi: 10.1172/jci.insight.142945
58. Henningfield J, Pankow J, Garrett B. Ammonia and other chemical base tobacco additives and cigarette nicotine delivery: issues and research needs. Nicotine Tob Res. (2004) 6:199–205. doi: 10.1080/1462220042000202472
59. Stepanov I, Fujioka N. Bringing attention to e-cigarette pH as an important element for research and regulation. Tob Control. (2015) 24:413–4. doi: 10.1136/tobaccocontrol-2014-051540
60. Talih S, Salman R, El-Hage R, Karam E, Karaoghlanian N, El-Hellani A, et al. Characteristics and toxicant emissions of JUUL electronic cigarettes. Tob Control. (2019) 28:678–80. doi: 10.1136/tobaccocontrol-2018-054616
61. Xiu X, Puskar NL, Shanata JA, Lester HA, Dougherty DA. Nicotine binding to brain receptors requires a strong cation-pi interaction. Nature. (2009) 458:534–7. doi: 10.1038/nature07768
62. Shao XM, Lopez-Valdes HE, Liang J, Feldman JL. Inhaled nicotine equivalent to cigarette smoking disrupts systemic and uterine hemodynamics and induces cardiac arrhythmia in pregnant rats. Sci Rep. (2017) 7:16974. doi: 10.1038/s41598-017-17301-5
63. Eliasson B, Attvall S, Taskinen MR, Smith U. The insulin resistance syndrome in smokers is related to smoking habits. Arterioscler Thromb. (1994) 14:1946–50. doi: 10.1161/01.ATV.14.12.1946
64. Facchini FS, Hollenbeck CB, Jeppesen J, Chen YD, Reaven GM. Insulin resistance and cigarette smoking. Lancet. (1992) 339:1128–30. doi: 10.1016/0140-6736(92)90730-Q
65. Assali AR, Beigel Y, Schreibman R, Shafer Z, Fainaru M. Weight gain and insulin resistance during nicotine replacement therapy. Clin Cardiol. (1999) 22:357–60. doi: 10.1002/clc.4960220512
66. Attvall S, Fowelin J, Lager I, Von Schenck H, Smith U. Smoking induces insulin resistance–a potential link with the insulin resistance syndrome. J Intern Med. (1993) 233:327–32. doi: 10.1111/j.1365-2796.1993.tb00680.x
67. Hautanen A, Adlercreutz H. Hyperinsulinaemia, dyslipidaemia and exaggerated adrenal androgen response to adrenocorticotropin in male smokers. Diabetologia. (1993) 36:1275–81. doi: 10.1007/BF00400805
68. Janzon L, Berntorp K, Hanson M, Lindell SE, Trell E. Glucose tolerance and smoking: a population study of oral and intravenous glucose tolerance tests in middle-aged men. Diabetologia. (1983) 25:86–8. doi: 10.1007/BF00250893
69. Kong C, Nimmo L, Elatrozy T, Anyaoku V, Hughes C, Robinson S, et al. Smoking is associated with increased hepatic lipase activity, insulin resistance, dyslipidaemia and early atherosclerosis in Type 2 diabetes. Atherosclerosis. (2001) 156:373–8. doi: 10.1016/S0021-9150(00)00664-X
70. Ronnemaa T, Ronnemaa EM, Puukka P, Pyorala K, Laakso M. Smoking is independently associated with high plasma insulin levels in nondiabetic men. Diabetes Care. (1996) 19:1229–32. doi: 10.2337/diacare.19.11.1229
71. Targher G, Alberiche M, Zenere MB, Bonadonna RC, Muggeo M, Bonora E. Cigarette smoking and insulin resistance in patients with noninsulin-dependent diabetes mellitus. J Clin Endocrinol Metab. (1997) 82:3619–24. doi: 10.1210/jc.82.11.3619
72. Laws A, Reaven GM. Evidence for an independent relationship between insulin resistance and fasting plasma HDL-cholesterol, triglyceride and insulin concentrations. J Intern Med. (1992) 231:25–30. doi: 10.1111/j.1365-2796.1992.tb00494.x
73. Steinberg HO, Chaker H, Leaming R, Johnson A, Brechtel G, Baron AD. Obesity/insulin resistance is associated with endothelial dysfunction. Implications for the syndrome of insulin resistance. J Clin Invest. (1996) 97:2601–10. doi: 10.1172/JCI118709
74. Eliasson B, Taskinen MR, Smith U. Long-term use of nicotine gum is associated with hyperinsulinemia and insulin resistance. Circulation. (1996) 94:878–81. doi: 10.1161/01.CIR.94.5.878
75. Epifano L, Di Vincenzo A, Fanelli C, Porcellati F, Perriello G, De Feo P, et al. Effect of cigarette smoking and of a transdermal nicotine delivery system on glucoregulation in type 2 diabetes mellitus. Eur J Clin Pharmacol. (1992) 43:257–63. doi: 10.1007/BF02333019
76. Persson PG, Carlsson S, Svanstrom L, Ostenson CG, Efendic S, Grill V. Cigarette smoking, oral moist snuff use and glucose intolerance. J Intern Med. (2000) 248:103–10. doi: 10.1046/j.1365-2796.2000.00708.x
77. Frati AC, Iniestra F, Ariza CR. Acute effect of cigarette smoking on glucose tolerance and other cardiovascular risk factors. Diabetes Care. (1996) 19:112–8. doi: 10.2337/diacare.19.2.112
78. Jeppesen J, Hein HO, Suadicani P, Gyntelberg F. Low triglycerides-high high-density lipoprotein cholesterol and risk of ischemic heart disease. Arch Intern Med. (2001) 161:361–6. doi: 10.1001/archinte.161.3.361
79. Majid S, Keith RJ, Fetterman JL, Weisbrod RM, Nystoriak J, Wilson T, et al. Lipid profiles in users of combustible and electronic cigarettes. Vasc Med. (2021) 26:483–8. doi: 10.1177/1358863X211009313
80. Foy CG, Bell RA, Farmer DF, Goff DC Jr, Wagenknecht LE. Smoking and incidence of diabetes among U.S. adults: findings from the Insulin Resistance Atherosclerosis Study. Diabetes Care. (2005) 28:2501–7. doi: 10.2337/diacare.28.10.2501
81. Expert Expert Panel on Detection Evaluation and and Treatment of High Blood Cholesterol in Adults. Executive Summary of The Third Report of The National Cholesterol Education Program (NCEP) Expert Panel on Detection, Evaluation, And Treatment of High Blood Cholesterol In Adults (Adult Treatment Panel III). JAMA. (2001) 285:2486–97. doi: 10.1001/jama.285.19.2486
82. Willi C, Bodenmann P, Ghali WA, Faris PD, Cornuz J. Active smoking and the risk of type 2 diabetes: a systematic review and meta-analysis. JAMA. (2007) 298:2654–64. doi: 10.1001/jama.298.22.2654
83. Ding EL, Hu FB. Smoking and type 2 diabetes: underrecognized risks and disease burden. JAMA. (2007) 298:2675–6. doi: 10.1001/jama.298.22.2675
84. Farin HM, Abbasi F, Kim SH, Lamendola C, McLaughlin T, Reaven GM. The relationship between insulin resistance and dyslipidaemia in cigarette smokers. Diabetes Obes Metab. (2007) 9:65–9. doi: 10.1111/j.1463-1326.2006.00574.x
85. Facchini FS, Hua N, Abbasi F, Reaven GM. Insulin resistance as a predictor of age-related diseases. J Clin Endocrinol Metab. (2001) 86:3574–8. doi: 10.1210/jcem.86.8.7763
86. Reaven G, Tsao PS. Insulin resistance and compensatory hyperinsulinemia: the key player between cigarette smoking and cardiovascular disease? J Am Coll Cardiol. (2003) 41:1044–7. doi: 10.1016/S0735-1097(02)02982-0
87. Landmesser U, Drexler H. The clinical significance of endothelial dysfunction. Curr Opin Cardiol. (2005) 20:547–51. doi: 10.1097/01.hco.0000179821.11071.79
88. Forstermann U, Xia N, Li H. Roles of vascular oxidative stress and nitric oxide in the pathogenesis of atherosclerosis. Circ Res. (2017) 120:713–35. doi: 10.1161/CIRCRESAHA.116.309326
89. Tsutsui H, Kinugawa S, Matsushima S. Oxidative stress and heart failure. Am J Physiol Heart Circ Physiol. (2011) 301:H2181–H90. doi: 10.1152/ajpheart.00554.2011
90. Faria A, Persaud SJ. Cardiac oxidative stress in diabetes: mechanisms and therapeutic potential. Pharmacol Ther. (2017) 172:50–62. doi: 10.1016/j.pharmthera.2016.11.013
91. Kattoor AJ, Pothineni NVK, Palagiri D, Mehta JL. Oxidative stress in atherosclerosis. Curr Atheroscler Rep. (2017) 19:42. doi: 10.1007/s11883-017-0678-6
92. Cesselli D, Jakoniuk I, Barlucchi L, Beltrami AP, Hintze TH, Nadal-Ginard B, et al. Oxidative stress-mediated cardiac cell death is a major determinant of ventricular dysfunction and failure in dog dilated cardiomyopathy. Circ Res. (2001) 89:279–86. doi: 10.1161/hh1501.094115
93. Dai DF, Chiao YA, Marcinek DJ, Szeto HH, Rabinovitch PS. Mitochondrial oxidative stress in aging and healthspan. Longev Healthspan. (2014) 3:6. doi: 10.1186/2046-2395-3-6
94. Nita M, Grzybowski A. The role of the reactive oxygen species and oxidative stress in the pathomechanism of the age-related ocular diseases and other pathologies of the anterior and posterior eye segments in adults. Oxid Med Cell Longev. (2016) 2016:3164734. doi: 10.1155/2016/3164734
95. Lee SR, Kim N, Noh YH, Xu Z, Ko KS, Rhee BD, et al. Mitochondrial DNA, mitochondrial dysfunction, and cardiac manifestations. Front Biosci (Landmark Ed). (2017) 22:1177–94. doi: 10.2741/4541
96. Tocchi A, Quarles EK, Basisty N, Gitari L, Rabinovitch PS. Mitochondrial dysfunction in cardiac aging. Biochim Biophys Acta. (2015) 1847:1424–33. doi: 10.1016/j.bbabio.2015.07.009
97. Anderson C, Majeste A, Hanus J, Wang S. E-cigarette aerosol exposure induces reactive oxygen species, DNA damage, and cell death in vascular endothelial cells. Toxicol Sci. (2016) 154:332–40. doi: 10.1093/toxsci/kfw166
98. Paolocci N, Biondi R, Bettini M, Lee CI, Berlowitz CO, Rossi R, et al. Oxygen radical-mediated reduction in basal and agonist-evoked NO release in isolated rat heart. J Mol Cell Cardiol. (2001) 33:671–9. doi: 10.1006/jmcc.2000.1334
99. Mayyas F, Aldawod H, Alzoubi KH, Khabour O, Shihadeh A, Eissenberg T. Comparison of the cardiac effects of electronic cigarette aerosol exposure with waterpipe and combustible cigarette smoke exposure in rats. Life Sci. (2020) 251:117644. doi: 10.1016/j.lfs.2020.117644
101. de Almeida A, de Almeida Rezende MS, Dantas SH, de Lima Silva S, de Oliveira J, de Lourdes Assuncao Araujo de Azevedo F, et al. Unveiling the role of inflammation and oxidative stress on age-related cardiovascular diseases. Oxid Med Cell Longev. (2020) 2020:1954398. doi: 10.1155/2020/1954398
102. Kaisar MA, Villalba H, Prasad S, Liles T, Sifat AE, Sajja RK, et al. Offsetting the impact of smoking and e-cigarette vaping on the cerebrovascular system and stroke injury: is Metformin a viable countermeasure? Redox Biol. (2017) 13:353–62. doi: 10.1016/j.redox.2017.06.006
103. Khan AA, Alsahli MA, Rahmani AH. Myeloperoxidase as an active disease biomarker: recent biochemical and pathological perspectives. Med Sci (Basel). (2018) 6:33. doi: 10.3390/medsci6020033
104. Chatterjee S, Tao JQ, Johncola A, Guo W, Caporale A, Langham MC, et al. Acute exposure to e-cigarettes causes inflammation and pulmonary endothelial oxidative stress in nonsmoking, healthy young subjects. Am J Physiol Lung Cell Mol Physiol. (2019) 317:L155–L66. doi: 10.1152/ajplung.00110.2019
105. Austin MA, Hokanson JE, Edwards KL. Hypertriglyceridemia as a cardiovascular risk factor. Am J Cardiol. (1998) 81:7B−12B. doi: 10.1016/S0002-9149(98)00031-9
106. Cheung MC, Wolfbauer G, Brown BG, Albers JJ. Relationship between plasma phospholipid transfer protein activity and HDL subclasses among patients with low HDL and cardiovascular disease. Atherosclerosis. (1999) 142:201–5. doi: 10.1016/S0021-9150(98)00190-7
107. Brischetto CS, Connor WE, Connor SL, Matarazzo JD. Plasma lipid and lipoprotein profiles of cigarette smokers from randomly selected families: enhancement of hyperlipidemia and depression of high-density lipoprotein. Am J Cardiol. (1983) 52:675–80. doi: 10.1016/0002-9149(83)90396-X
108. Campbell SC, Moffatt RJ, Stamford BA. Smoking and smoking cessation-The relationship between cardiovascular disease and lipoprotein metabolism: a review. Atherosclerosis. (2008) 201:225–35. doi: 10.1016/j.atherosclerosis.2008.04.046
109. Penesova A, Radikova Z, Cizmarova E, Kvetnanský R, Blazicek P, Vlcek M, et al. The role of norepinephrine and insulin resistance in an early stage of hypertension. Ann N Y Acad Sci. (2008) 1148:490–4. doi: 10.1196/annals.1410.036
110. Kim S, Cho W, Kim I, Lee SH, Oh GT, Park YM. Oxidized LDL induces vimentin secretion by macrophages and contributes to atherosclerotic inflammation. J Mol Med (Berl). (2020) 98:973–83. doi: 10.1007/s00109-020-01923-w
111. Whayne TF, Alaupovic P, Curry MD, Lee ET, Anderson PS, Schechter E. Plasma apolipoprotein B and VLDL-, LDL-, and HDL-cholesterol as risk factors in the development of coronary artery disease in male patients examined by angiography. Atherosclerosis. (1981) 39:411–24. doi: 10.1016/0021-9150(81)90026-5
112. Cohen JC, Boerwinkle E, Mosley TH Jr, Hobbs HH. Sequence variations in PCSK9, low LDL, and protection against coronary heart disease. N Engl J Med. (2006) 354:1264–72. doi: 10.1056/NEJMoa054013
113. Badea M, Gaman L, Delia C, Ilea A, Leasu F, Henriquez-Hernandez LA, et al. Trends of lipophilic, antioxidant and hematological parameters associated with conventional and electronic smoking habits in middle-age romanians. J Clin Med. (2019) 8:665. doi: 10.3390/jcm8050665
114. Moheimani RS, Bhetraratana M, Yin F, Peters KM, Gornbein J, Araujo JA, et al. Increased cardiac sympathetic activity and oxidative stress in habitual electronic cigarette users: implications for cardiovascular risk. JAMA Cardiol. (2017) 2:278–84. doi: 10.1001/jamacardio.2016.5303
115. Middlekauff HR, William KJ, Su B, Haptonstall K, Araujo JA, Wu X, et al. Changes in lipid composition associated with electronic cigarette use. J Transl Med. (2020) 18:379. doi: 10.1186/s12967-020-02557-9
116. Sindelar PJ, Guan Z, Dallner G, Ernster L. The protective role of plasmalogens in iron-induced lipid peroxidation. Free Radic Biol Med. (1999) 26:318–24. doi: 10.1016/S0891-5849(98)00221-4
117. Berg AH, Scherer PE. Adipose tissue, inflammation, and cardiovascular disease. Circ Res. (2005) 96:939–49. doi: 10.1161/01.RES.0000163635.62927.34
118. Wang Z, Wang D, Wang Y. Cigarette smoking and adipose tissue: the emerging role in progression of atherosclerosis. Mediators Inflamm. (2017) 2017:3102737. doi: 10.1155/2017/3102737
119. Oikonomou EK, Antoniades C. The role of adipose tissue in cardiovascular health and disease. Nat Rev Cardiol. (2019) 16:83–99. doi: 10.1038/s41569-018-0097-6
120. Lau DC, Dhillon B, Yan H, Szmitko PE, Verma S. Adipokines: molecular links between obesity and atheroslcerosis. Am J Physiol Heart Circ Physiol. (2005) 288:H2031–H41. doi: 10.1152/ajpheart.01058.2004
121. Koster A, Leitzmann MF, Schatzkin A, Adams KF, van Eijk JT, Hollenbeck AR, et al. The combined relations of adiposity and smoking on mortality. Am J Clin Nutr. (2008) 88:1206–12. doi: 10.3945/ajcn.2008.26298
122. Bergmann S, Siekmeier R. Influence of smoking and body weight on adipokines in middle aged women. Eur J Med Res. (2009) 14(Suppl. 4):21–6. doi: 10.1186/2047-783X-14-S4-21
123. Mach L, Bedanova H, Soucek M, Karpisek M, Nemec P, Orban M. Tobacco smoking and cytokine levels in human epicardial adipose tissue: impact of smoking cessation. Atherosclerosis. (2016) 255:37–42. doi: 10.1016/j.atherosclerosis.2016.10.022
124. An Z, Wang H, Song P, Zhang M, Geng X, Zou MH. Nicotine-induced activation of AMP-activated protein kinase inhibits fatty acid synthase in 3T3L1 adipocytes: a role for oxidant stress. J Biol Chem. (2007) 282:26793–801. doi: 10.1074/jbc.M703701200
125. Wu Y, Song P, Zhang W, Liu J, Dai X, Liu Z, et al. Activation of AMPKalpha2 in adipocytes is essential for nicotine-induced insulin resistance in vivo. Nat Med. (2015) 21:373–82. doi: 10.1038/nm.3826
126. Sztalryd C, Hamilton J, Horwitz BA, Johnson P, Kraemer FB. Alterations of lipolysis and lipoprotein lipase in chronically nicotine-treated rats. Am J Physiol. (1996) 270(2 Pt 1):E215–E23. doi: 10.1152/ajpendo.1996.270.2.E215
127. Ratzmann KP, Meyer LW, Riemer D. [Studies on the mechanism of nicotine-induced lipolysis in man]. Z Gesamte Inn Med. (1974) 29:410–3.
128. Bizzi A, Tacconi MT, Medea A, Garattini S. Some aspects of the effect of nicotine on plasma FFA and tissue triglycerides. Pharmacology. (1972) 7:216–24. doi: 10.1159/000136292
129. Andersson K, Arner P. Systemic nicotine stimulates human adipose tissue lipolysis through local cholinergic and catecholaminergic receptors. Int J Obes Relat Metab Disord. (2001) 25:1225–32. doi: 10.1038/sj.ijo.0801654
130. Mjos OD. Lipid effects of smoking. Am Heart J. (1988) 115(1 Pt 2):272–5. doi: 10.1016/0002-8703(88)90649-7
131. Pararasa C, Bailey CJ, Griffiths HR. Ageing, adipose tissue, fatty acids and inflammation. Biogerontology. (2015) 16:235–48. doi: 10.1007/s10522-014-9536-x
132. Chong CR, Clarke K, Levelt E. Metabolic remodeling in diabetic cardiomyopathy. Cardiovasc Res. (2017) 113:422–30. doi: 10.1093/cvr/cvx018
133. Kashyap S, Belfort R, Gastaldelli A, Pratipanawatr T, Berria R, Pratipanawatr W, et al. A sustained increase in plasma free fatty acids impairs insulin secretion in nondiabetic subjects genetically predisposed to develop type 2 diabetes. Diabetes. (2003) 52:2461–74. doi: 10.2337/diabetes.52.10.2461
134. Miedema MD, Maziarz M, Biggs ML, Zieman SJ, Kizer JR, Ix JH, et al. Plasma-free fatty acids, fatty acid-binding protein 4, and mortality in older adults (from the Cardiovascular Health Study). Am J Cardiol. (2014) 114:843–8. doi: 10.1016/j.amjcard.2014.06.012
135. Ferrante AW Jr. The immune cells in adipose tissue. Diabetes Obes Metab. (2013) 15(Suppl. 3):34–8. doi: 10.1111/dom.12154
136. Chow J, Rahman J, Achermann JC, Dattani MT, Rahman S. Mitochondrial disease and endocrine dysfunction. Nat Rev Endocrinol. (2016) 13:92–104. doi: 10.1038/nrendo.2016.151
137. Hirabara SM, Curi R, Maechler P. Saturated fatty acid-induced insulin resistance is associated with mitochondrial dysfunction in skeletal muscle cells. J Cell Physiol. (2010) 222:187–94. doi: 10.1002/jcp.21936
138. Hoeks J, van Herpen NA, Mensink M, Moonen-Kornips E, van Beurden D, Hesselink MK, et al. Prolonged fasting identifies skeletal muscle mitochondrial dysfunction as consequence rather than cause of human insulin resistance. Diabetes. (2010) 59:2117–25. doi: 10.2337/db10-0519
139. Boden G. Obesity, insulin resistance and free fatty acids. Curr Opin Endocrinol Diabetes Obes. (2011) 18:139–43. doi: 10.1097/MED.0b013e3283444b09
140. Cusi K. Role of insulin resistance and lipotoxicity in non-alcoholic steatohepatitis. Clin Liver Dis. (2009) 13:545–63. doi: 10.1016/j.cld.2009.07.009
141. Cusi K, Kashyap S, Gastaldelli A, Bajaj M, Cersosimo E. Effects on insulin secretion and insulin action of a 48-h reduction of plasma free fatty acids with acipimox in nondiabetic subjects genetically predisposed to type 2 diabetes. Am J Physiol Endocrinol Metab. (2007) 292:E1775–E81. doi: 10.1152/ajpendo.00624.2006
142. Friedman TC, Sinha-Hikim I, Parveen M, Najjar SM, Liu Y, Mangubat M, et al. Additive effects of nicotine and high-fat diet on hepatic steatosis in male mice. Endocrinology. (2012) 153:5809–20. doi: 10.1210/en.2012-1750
143. Shi H, Kokoeva MV, Inouye K, Tzameli I, Yin H, Flier JS. TLR4 links innate immunity and fatty acid-induced insulin resistance. J Clin Invest. (2006) 116:3015–25. doi: 10.1172/JCI28898
144. Karimi K, Sarir H, Mortaz E, Smit JJ, Hosseini H, De Kimpe SJ, et al. Toll-like receptor-4 mediates cigarette smoke-induced cytokine production by human macrophages. Respir Res. (2006) 7:66. doi: 10.1186/1465-9921-7-66
145. Bobryshev YV. Monocyte recruitment and foam cell formation in atherosclerosis. Micron. (2006) 37:208–22. doi: 10.1016/j.micron.2005.10.007
146. Grassi G, Seravalle G, Mancia G. Sympathetic activation in cardiovascular disease: evidence, clinical impact and therapeutic implications. Eur J Clin Invest. (2015) 45:1367–75. doi: 10.1111/eci.12553
147. Olfert IM, DeVallance E, Hoskinson H, Branyan KW, Clayton S, Pitzer CR, et al. Chronic exposure to electronic cigarettes results in impaired cardiovascular function in mice. J Appl Physiol 1985. (2018) 124:573–82. doi: 10.1152/japplphysiol.00713.2017
148. Gordan R, Gwathmey JK, Xie LH. Autonomic and endocrine control of cardiovascular function. World J Cardiol. (2015) 7:204–14. doi: 10.4330/wjc.v7.i4.204
149. Haass M, Kubler W. Nicotine and sympathetic neurotransmission. Cardiovasc Drugs Ther. (1997) 10:657–65. doi: 10.1007/BF00053022
150. Jankowska EA, Ponikowski P, Piepoli MF, Banasiak W, Anker SD, Poole-Wilson PA. Autonomic imbalance and immune activation in chronic heart failure - pathophysiological links. Cardiovasc Res. (2006) 70:434–45. doi: 10.1016/j.cardiores.2006.01.013
151. Aronow WS, Ahn C, Kronzon I. Effect of propranolol versus no propranolol on total mortality plus nonfatal myocardial infarction in older patients with prior myocardial infarction, congestive heart failure, and left ventricular ejection fraction > or = 40% treated with diuretics plus angiotensin-converting enzyme inhibitors. Am J Cardiol. (1997) 80:207–9. doi: 10.1016/S0002-9149(97)00320-2
152. Gonzalez JE, Cooke WH. Acute effects of electronic cigarettes on arterial pressure and peripheral sympathetic activity in young nonsmokers. Am J Physiol Heart Circ Physiol. (2021) 320:H248–H55. doi: 10.1152/ajpheart.00448.2020
153. Libby P, Nahrendorf M, Swirski FK. Leukocytes link local and systemic inflammation in ischemic cardiovascular disease: an expanded “cardiovascular continuum”. J Am Coll Cardiol. (2016) 67:1091–103. doi: 10.1016/j.jacc.2015.12.048
154. Boas Z, Gupta P, Moheimani RS, Bhetraratana M, Yin F, Peters KM, et al. Activation of the “Splenocardiac Axis” by electronic and tobacco cigarettes in otherwise healthy young adults. Physiol Rep. (2017) 5:e13393. doi: 10.14814/phy2.13393
155. Robertson D, Tseng CJ, Appalsamy M. Smoking and mechanisms of cardiovascular control. Am Heart J. (1988) 115(1 Pt 2):258–63. doi: 10.1016/0002-8703(88)90646-1
156. Jaquet FS, Shapiro D, Uijtdehaage SHJ. The acute effects of smoking on heart-rate and blood-pressure - an ambulatory study. Hum Psychopharm Clin. (1994) 9:273–83. doi: 10.1002/hup.470090406
157. Gao YJ, Holloway AC, Su LY, Takemori K, Lu C, Lee RM. Effects of fetal and neonatal exposure to nicotine on blood pressure and perivascular adipose tissue function in adult life. Eur J Pharmacol. (2008) 590:264–8. doi: 10.1016/j.ejphar.2008.05.044
158. Yarlioglues M, Kaya MG, Ardic I, Calapkorur B, Dogdu O, Akpek M, et al. Acute effects of passive smoking on blood pressure and heart rate in healthy females. Blood Press Monit. (2010) 15:251–6. doi: 10.1097/MBP.0b013e32833e439f
159. Freitas SRS, Alvim RO. Smoking and blood pressure phenotypes: new perspective for an old problem. Am J Hypertens. (2017) 30:554–5. doi: 10.1093/ajh/hpx039
160. Gillman IG, Pennington ASC, Humphries KE, Oldham MJ. Determining the impact of flavored e-liquids on aldehyde production during Vaping. Regul Toxicol Pharmacol. (2020) 112:104588. doi: 10.1016/j.yrtph.2020.104588
161. Aloui C, Prigent A, Sut C, Tariket S, Hamzeh-Cognasse H, Pozzetto B, et al. The signaling role of CD40 ligand in platelet biology and in platelet component transfusion. Int J Mol Sci. (2014) 15:22342–64. doi: 10.3390/ijms151222342
162. Benowitz NL, Hansson A, Jacob P 3rd. Cardiovascular effects of nasal and transdermal nicotine and cigarette smoking. Hypertension. (2002) 39:1107–12. doi: 10.1161/01.HYP.0000018825.76673.EA
163. Egle JL Jr. Effects of inhaled acetaldehyde and propionaldehyde on blood pressure and heart rate. Toxicol Appl Pharmacol. (1972) 23:131–5. doi: 10.1016/0041-008X(72)90212-8
164. Vlachopoulos C, Ioakeimidis N, Abdelrasoul M, Terentes-Printzios D, Georgakopoulos C, Pietri P, et al. Electronic cigarette smoking increases aortic stiffness and blood pressure in young smokers. J Am Coll Cardiol. (2016) 67:2802–3. doi: 10.1016/j.jacc.2016.03.569
165. Biondi-Zoccai G, Sciarretta S, Bullen C, Nocella C, Violi F, Loffredo L, et al. Acute effects of heat-not-burn, electronic vaping, and traditional tobacco combustion cigarettes: the Sapienza University of Rome-vascular assessment of proatherosclerotic effects of smoking (SUR - VAPES) 2 randomized trial. J Am Heart Assoc. (2019) 8:e010455. doi: 10.1161/JAHA.118.010455
166. Benowitz NL, St Helen G, Nardone N, Addo N, Zhang JJ, Harvanko AM, et al. Twenty-four-hour cardiovascular effects of electronic cigarettes compared with cigarette smoking in dual users. J Am Heart Assoc. (2020) 9:e017317. doi: 10.1161/JAHA.120.017317
167. Franzen KF, Willig J, Cayo Talavera S, Meusel M, Sayk F, Reppel M, et al. E-cigarettes and cigarettes worsen peripheral and central hemodynamics as well as arterial stiffness: a randomized, double-blinded pilot study. Vasc Med. (2018) 23:419–25. doi: 10.1177/1358863X18779694
168. Polosa R, Morjaria JB, Caponnetto P, Battaglia E, Russo C, Ciampi C, et al. Blood pressure control in smokers with arterial hypertension who switched to electronic cigarettes. Int J Environ Res Public Health. (2016) 13:1123. doi: 10.3390/ijerph13111123
169. Farsalinos KE, Tsiapras D, Kyrzopoulos S, Savvopoulou M, Voudris V. Acute effects of using an electronic nicotine-delivery device (electronic cigarette) on myocardial function: comparison with the effects of regular cigarettes. BMC Cardiovasc Disord. (2014) 14:78. doi: 10.1186/1471-2261-14-78
170. Yan XS, D'Ruiz C. Effects of using electronic cigarettes on nicotine delivery and cardiovascular function in comparison with regular cigarettes. Regul Toxicol Pharmacol. (2015) 71:24–34. doi: 10.1016/j.yrtph.2014.11.004
171. D'Ruiz CD, O'Connell G, Graff DW, Yan XS. Measurement of cardiovascular and pulmonary function endpoints and other physiological effects following partial or complete substitution of cigarettes with electronic cigarettes in adult smokers. Regul Toxicol Pharmacol. (2017) 87:36–53. doi: 10.1016/j.yrtph.2017.05.002
172. Larue F, Tasbih T, Ribeiro PAB, Lavoie KL, Dolan E, Bacon SL. Immediate physiological effects of acute electronic cigarette use in humans: a systematic review and meta-analysis. Respir Med. (2021) 190:106684. doi: 10.1016/j.rmed.2021.106684
173. Polosa R, Cibella F, Caponnetto P, Maglia M, Prosperini U, Russo C, et al. Health impact of E-cigarettes: a prospective 3.5-year study of regular daily users who have never smoked. Sci Rep. (2017) 7:13825. doi: 10.1038/s41598-017-14043-2
174. Shim CY, Liu YN, Atkinson T, Xie A, Foster T, Davidson BP, et al. Molecular imaging of platelet-endothelial interactions and endothelial von willebrand factor in early and mid-stage atherosclerosis. Circ Cardiovasc Imaging. (2015) 8:e002765. doi: 10.1161/CIRCIMAGING.114.002765
175. Hom S, Chen L, Wang T, Ghebrehiwet B, Yin W, Rubenstein DA. Platelet activation, adhesion, inflammation, and aggregation potential are altered in the presence of electronic cigarette extracts of variable nicotine concentrations. Platelets. (2016) 27:694–702. doi: 10.3109/09537104.2016.1158403
176. Qasim H, Karim ZA, Silva-Espinoza JC, Khasawneh FT, Rivera JO, Ellis CC, et al. Short-term E-cigarette exposure increases the risk of thrombogenesis and enhances platelet function in mice. J Am Heart Assoc. (2018) 7:e009264. doi: 10.1161/JAHA.118.009264
177. Woulfe D, Jiang H, Morgans A, Monks R, Birnbaum M, Brass LF. Defects in secretion, aggregation, and thrombus formation in platelets from mice lacking Akt2. J Clin Invest. (2004) 113:441–50. doi: 10.1172/JCI200420267
178. Nocella C, Biondi-Zoccai G, Sciarretta S, Peruzzi M, Pagano F, Loffredo L, et al. Impact of tobacco versus electronic cigarette smoking on platelet function. Am J Cardiol. (2018) 122:1477–81. doi: 10.1016/j.amjcard.2018.07.029
179. Theoret JF, Yacoub D, Hachem A, Gillis MA, Merhi Y. P-selectin ligation induces platelet activation and enhances microaggregate and thrombus formation. Thromb Res. (2011) 128:243–50. doi: 10.1016/j.thromres.2011.04.018
180. Antoniewicz L, Bosson JA, Kuhl J, Abdel-Halim SM, Kiessling A, Mobarrez F, et al. Electronic cigarettes increase endothelial progenitor cells in the blood of healthy volunteers. Atherosclerosis. (2016) 255:179–85. doi: 10.1016/j.atherosclerosis.2016.09.064
181. Mobarrez F, Antoniewicz L, Hedman L, Bosson JA, Lundback M. Electronic cigarettes containing nicotine increase endothelial and platelet derived extracellular vesicles in healthy volunteers. Atherosclerosis. (2020) 301:93–100. doi: 10.1016/j.atherosclerosis.2020.02.010
182. Alzahrani T, Pena I, Temesgen N, Glantz SA. Association between electronic cigarette use and myocardial infarction. Am J Prev Med. (2018) 55:455–61. doi: 10.1016/j.amepre.2018.05.004
183. Bhatta DN, Glantz SA. electronic cigarette use and myocardial infarction among adults in the US population assessment of tobacco and health. J Am Heart Assoc. (2019) 8:e012317. doi: 10.1161/JAHA.119.012317
184. Cooper LL, Palmisano JN, Benjamin EJ, Larson MG, Vasan RS, Mitchell GF, et al. Microvascular function contributes to the relation between aortic stiffness and cardiovascular events: the Framingham Heart Study. Circ Cardiovasc Imaging. (2016) 9:e004979. doi: 10.1161/CIRCIMAGING.116.004979
185. Ikonomidis I, Vlastos D, Kourea K, Kostelli G, Varoudi M, Pavlidis G, et al. Electronic cigarette smoking increases arterial stiffness and oxidative stress to a lesser extent than a single conventional cigarette: an acute and chronic study. Circulation. (2018) 137:303–6. doi: 10.1161/CIRCULATIONAHA.117.029153
186. El-Mahdy MA, Mahgoup EM, Ewees MG, Eid MS, Abdelghany TM, Zweier JL. Long-term electronic cigarette exposure induces cardiovascular dysfunction similar to tobacco cigarettes: role of nicotine and exposure duration. Am J Physiol Heart Circ Physiol. (2021) 320:H2112–H29. doi: 10.1152/ajpheart.00997.2020
187. El-Mahdy MA, Ewees MG, Eid MS, Mahgoup EM, Khaleel SA, Zweier JL. Electronic cigarette exposure causes vascular endothelial dysfunction due to NADPH oxidase activation and eNOS uncoupling. Am J Physiol Heart Circ Physiol. (2022) 322:H549–H67. doi: 10.1152/ajpheart.00460.2021
188. Haptonstall KP, Choroomi Y, Moheimani R, Nguyen K, Tran E, Lakhani K, et al. Differential effects of tobacco cigarettes and electronic cigarettes on endothelial function in healthy young people. Am J Physiol Heart Circ Physiol. (2020) 319:H547–H56. doi: 10.1152/ajpheart.00307.2020
189. George J, Hussain M, Vadiveloo T, Ireland S, Hopkinson P, Struthers AD, et al. Cardiovascular effects of switching from tobacco cigarettes to electronic cigarettes. J Am Coll Cardiol. (2019) 74:3112–20. doi: 10.1016/j.jacc.2019.09.067
190. Lee WH, Ong SG, Zhou Y, Tian L, Bae HR, Baker N, et al. Modeling cardiovascular risks of E-cigarettes with human-induced pluripotent stem cell-derived endothelial cells. J Am Coll Cardiol. (2019) 73:2722–37. doi: 10.1016/j.jacc.2019.03.476
191. Barber KE, Ghebrehiwet B, Yin W, Rubenstein DA. Endothelial cell inflammatory reactions are altered in the presence of e-cigarette extracts of variable nicotine. Cell Mol Bioeng. (2017) 10:124–33. doi: 10.1007/s12195-016-0465-4
192. Shao XM, Lopez B, Nathan D, Wilson J, Bankole E, Tumoyan H, et al. A mouse model for chronic intermittent electronic cigarette exposure exhibits nicotine pharmacokinetics resembling human vapers. J Neurosci Methods. (2019) 326:108376. doi: 10.1016/j.jneumeth.2019.108376
193. Reidel B, Radicioni G, Clapp PW, Ford AA, Abdelwahab S, Rebuli ME, et al. E-cigarette use causes a unique innate immune response in the lung, involving increased neutrophilic activation and altered mucin secretion. Am J Respir Crit Care Med. (2018) 197:492–501. doi: 10.1164/rccm.201708-1590OC
194. Li J, Huynh L, Cornwell WD, Tang MS, Simborio H, Huang J, et al. Electronic cigarettes induce mitochondrial DNA damage and trigger TLR9 (toll-like receptor 9)-mediated atherosclerosis. Arterioscler Thromb Vasc Biol. (2021) 41:839–53. doi: 10.1161/ATVBAHA.120.315556
195. Yap J, Sim D, Lim CP, Chia SY, Go YY, Jaufeerally FR, et al. Predictors of two-year mortality in Asian patients with heart failure and preserved ejection fraction. Int J Cardiol. (2015) 183:33–8. doi: 10.1016/j.ijcard.2015.01.063
196. Takeshita D, Nakajima-Takenaka C, Shimizu J, Hattori H, Nakashima T, Kikuta A, et al. Effects of formaldehyde on cardiovascular system in in situ rat hearts. Basic Clin Pharmacol Toxicol. (2009) 105:271–80. doi: 10.1111/j.1742-7843.2009.00442.x
197. Jin YZ, Wang GF, Wang Q, Zhang XY, Yan B, Hu WN. Effects of acetaldehyde and L-carnitine on morphology and enzyme activity of myocardial mitochondria in rats. Mol Biol Rep. (2014) 41:7923–8. doi: 10.1007/s11033-014-3686-4
198. Hasan KM, Friedman TC, Parveen M, Espinoza-Derout J, Bautista F, Razipour MM, et al. Electronic cigarettes cause alteration in cardiac structure and function in diet-induced obese mice. PLoS One. (2020) 15:e0239671. doi: 10.1371/journal.pone.0239671
199. Crotty Alexander LE, Drummond CA, Hepokoski M, Mathew D, Moshensky A, Willeford A, et al. Chronic inhalation of e-cigarette vapor containing nicotine disrupts airway barrier function and induces systemic inflammation and multiorgan fibrosis in mice. Am J Physiol Regul Integr Comp Physiol. (2018) 314:R834–R47. doi: 10.1152/ajpregu.00270.2017
200. Goniewicz ML, Miller CR, Sutanto E, Li D. How effective are electronic cigarettes for reducing respiratory and cardiovascular risk in smokers? A systematic review. Harm Reduct J. (2020) 17:91. doi: 10.1186/s12954-020-00440-w
201. Osei AD, Mirbolouk M, Orimoloye OA, Dzaye O, Uddin SMI, Benjamin EJ, et al. Association between E-cigarette use and cardiovascular disease among never and current combustible-cigarette smokers. Am J Med. (2019) 132:949–54.e2. doi: 10.1016/j.amjmed.2019.02.016
202. Schaller JP, Keller D, Poget L, Pratte P, Kaelin E, McHugh D, et al. Evaluation of the tobacco heating system 2.2. Part 2: chemical composition, genotoxicity, cytotoxicity, and physical properties of the aerosol. Regul Toxicol Pharmacol. (2016) 81(Suppl. 2):S27–S47. doi: 10.1016/j.yrtph.2016.10.001
203. Kim SJC, Friedman TC. A New ingenious enemy: heat-not-burn products. tobacco use insights. Tob Use Insights. (2022) 15:1179173X221076419. doi: 10.1177/1179173X221076419
204. St Helen G, Jacob Iii P, Nardone N, Benowitz NL. IQOS: examination of Philip Morris International's claim of reduced exposure. Tob Control. (2018) 27(Suppl. 1):s30–s6. doi: 10.1136/tobaccocontrol-2018-054321
205. Elias J, Ling PM. Invisible smoke: third-party endorsement and the resurrection of heat-not-burn tobacco products. Tob Control. (2018) 27(Suppl. 1):s96–s101. doi: 10.1136/tobaccocontrol-2018-054433
206. Sharma A, McCausland K, Jancey J. Adolescent's health perceptions of e-cigarettes: a systematic review. Am J Prev Med. (2021) 60:716–25. doi: 10.1016/j.amepre.2020.12.013
Keywords: electronic cigarettes, nicotine, heart failure, cardiovascular disease, atherosclerosis
Citation: Espinoza-Derout J, Shao XM, Lao CJ, Hasan KM, Rivera JC, Jordan MC, Echeverria V, Roos KP, Sinha-Hikim AP and Friedman TC (2022) Electronic Cigarette Use and the Risk of Cardiovascular Diseases. Front. Cardiovasc. Med. 9:879726. doi: 10.3389/fcvm.2022.879726
Received: 20 February 2022; Accepted: 11 March 2022;
Published: 07 April 2022.
Edited by:
Jinwei Tian, The Second Affiliated Hospital of Harbin Medical University, ChinaReviewed by:
Nazareno Paolocci, Johns Hopkins University, United StatesYi-Fei Dong, The Second Affiliated Hospital of Nanchang University, China
Copyright © 2022 Espinoza-Derout, Shao, Lao, Hasan, Rivera, Jordan, Echeverria, Roos, Sinha-Hikim and Friedman. This is an open-access article distributed under the terms of the Creative Commons Attribution License (CC BY). The use, distribution or reproduction in other forums is permitted, provided the original author(s) and the copyright owner(s) are credited and that the original publication in this journal is cited, in accordance with accepted academic practice. No use, distribution or reproduction is permitted which does not comply with these terms.
*Correspondence: Jorge Espinoza-Derout, am9yZ2Vlc3Bpbm96YWRlcm91dEBjZHJld3UuZWR1