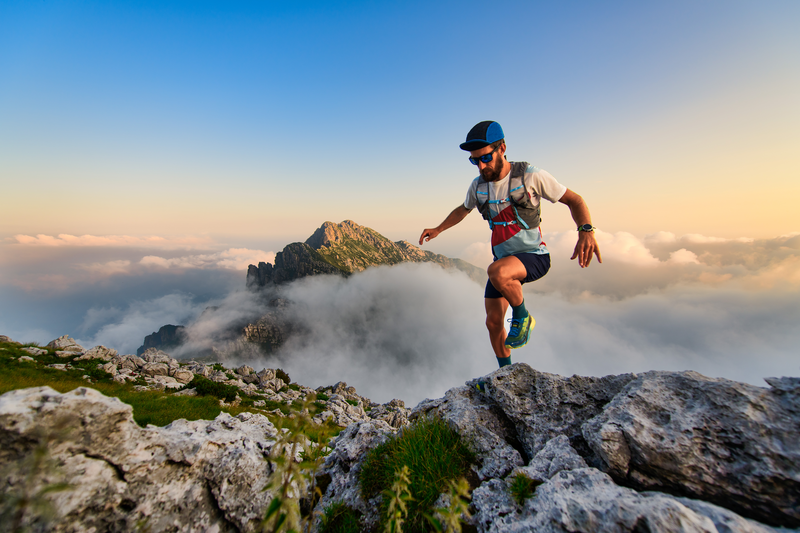
94% of researchers rate our articles as excellent or good
Learn more about the work of our research integrity team to safeguard the quality of each article we publish.
Find out more
SYSTEMATIC REVIEW article
Front. Cardiovasc. Med. , 25 April 2022
Sec. Heart Valve Disease
Volume 9 - 2022 | https://doi.org/10.3389/fcvm.2022.877140
This article is part of the Research Topic Advances in the Imaging and Treatment of Valvular Heart Disease View all 22 articles
Background: Preliminary studies indicated that enhanced plasma levels of lipoprotein(a) [lp(a)] might link with the risk of calcific aortic valve disease (CAVD), but the clinical association between them remained inconclusive. This systematic review and meta-analysis were aimed to determine this association.
Methods: We comprehensively searched PubMed, Embase, Web of Science, and Scopus databases for studies reporting the incidence of CAVD and their plasma lp(a) concentrations. Pooled risk ratio (RR) and 95% confidence interval (95% CI) were calculated to evaluate the effect of lp(a) on CAVD using the random-effects model. Subgroup analyses by study types, countries, and the level of adjustment were also conducted. Funnel plots, Egger's test and Begg's test were conducted to evaluate the publication bias.
Results: Eight eligible studies with 52,931 participants were included in this systematic review and meta-analysis. Of these, four were cohort studies and four were case-control studies. Five studies were rated as high quality, three as moderate quality. The pooled results showed that plasma lp(a) levels ≥50 mg/dL were associated with a 1.76-fold increased risk of CAVD (RR, 1.76; 95% CI, 1.47–2.11), but lp(a) levels ≥30 mg/dL were not observed to be significantly related with CAVD (RR, 1.28; 95% CI, 0.98–1.68). We performed subgroup analyses by study type, the RRs of cohort studies revealed lp(a) levels ≥50 mg/dL and lp(a) levels ≥30 mg/dL have positive association with CAVD (RR, 1.70; 95% CI, 1.39–2.07; RR 1.38; 95% CI, 1.19–1.61).
Conclusion: High plasma lp(a) levels (≥50 mg/dL) are significantly associated with increased risk of CAVD.
Calcific aortic valve disease (CAVD) is one of the most common valve disorders (1). CAVD is characterized by calcification and remodeling of the valve leaflets, which often progresses to aortic sclerosis and stenosis, eventually leading to heart failure, angina, death, and other serious adverse cardiovascular events (2, 3). 2020 VHD guideline recommended the intervention of symptomatic AVS mainly apply to SAVR and TAVI. Surgical treatment is not performed routinely in asymptomatic patients (4). With more and more in-depth studies of pathogenesis, researchers are exploring targeted drugs to delay disease progression (5). A meta-analysis included three RCT and five observational studies to analyze the efficacy of ACEI/ARB to CAVD (6), the results showed that there was no statistically significant difference in all-cause mortality between the two groups, but the AVR rate of the treatment group was lower than that of the control group, which needed large-scale RCT to prove. Multiple rigorous RCTs have shown negative efficacy of statins (7–9). Besides, researchers also explored the targets on phosphate/calcium-metabolism and nitric oxide and IGF-1 signaling pathway, which need to be further proved (5).
Globally, about 10–30% of the population has high lp(a) levels ≥50 mg/dl (10). Epidemiological and genetic evidence suggested that high lp(a) concentration had a direct relationship with cardiovascular disease (11). Previous cytological studies have shown that lipoprotein(a) [lp(a)] played an important role in the pathogenesis of CAVD through increasing inflammation and oxidative stress, promoting calcium deposition of valvular interstitial cells (VICs) (12, 13). Mendelian randomization studies suggested that LPA genotype, which could mediate the levels of lp(a), had a strong relationship with CAVD (14). Multiple genome-wide association studies had shown that the rs10455872 genetic variant in LPA, which was associated with higher lp(a) levels, was independently related to an augmented risk of CAVD (15, 16). However, evidence from clinical studies was inconsistent. Some studies indicated comparing to those without CAVD, patients with CAVD had significantly higher levels of lp(a) (17, 18), while some studies suggested there were no statistical differences in lp(a) levels between CAVD and controls (19). In response to the lack of systematic and comprehensive evidence on the clinical association between lp(a) and CAVD. We performed an extensive systematic review and meta-analysis to assess whether elevated lp(a) significantly affected the incidence of CAVD.
The systematic review and meta-analysis were performed following the recommendations of the Preferred Reporting Items for Systematic Reviews and Meta-analyses (PRISMA) (20) and Meta-analyses of Observational Studies in Epidemiology (MOOSE) checklist (21). The protocol has been registered in PROSPERO database (registration number: CRD42021273149).
Two authors (Liu QY and Yu YQ) systematically searched the electronic databases, including PubMed (MEDLINE), Embase, Web of Science, and Scopus, up to 31 August 2021. Searching terms included [“lipoprotein(a)” or “lp (a)” or “lipoprotein”] and (“calcific aortic valve disease” or “calcific aortic valve stenosis” or “aortic valve stenosis” or “aortic stenosis” or “aortic valve sclerosis” or “aortic sclerosis” or “aortic valve calcification”) without language or sample size restrictions. The reference lists of relevant reviews, original reports were also searched for potential eligible records. The initial screening of eligible studies was based on the titles and abstracts.
All cohort studies and case-control studies that had investigated the association between lp(a) and the risk of CAVD were eligible for inclusion. 2016 ESC/EAS Guidelines suggested that lp(a) ≥50 mg/dL had a significant risk of CVD (22). European Atherosclerosis Society Consensus Panel recommended that 50 mg/dL as the cut-off value of lp(a) elevation to screen the risk of CVD (23). In the U.S, the general cut-off value for lp(a) elevation is 30 mg/dL higher (24). Canadian Guidelines for the Management of Dyslipidemia pointed out that lp(a) ≥30 mg/dL continuously increased the risk of CVD (25). Analyzing Chinese studies on the risk of lp(a) cut-off value, the Expert Statement suggested 30 mg/dl might be the cut point for the increased risk of CVD (26). And lots of studies revealed both two cut-off values might be applicable to assess CAVD risk (27, 28). Therefore, we considered 30 and 50 mg/dL as the cut-off points for grouping and merging as most studies reported lp(a) as a categorical variable. The exclusion criteria were as follows: (1) patients with rheumatic diseases; (2) duplications or conference abstracts; (3) missing data and data that were impossible to extract or calculate from the published results. The eligibility of the included studies was assessed by two reviewers (Liu QY and Yu YQ) independently. Any disputes were resolved by consensus with all the authors.
Data extraction was completed by two reviewers (Liu QY and Lai RM) independently. If there were disagreements, a third reviewer would be consulted (Ju JQ). Information extracted from each included study comprised first author's name, publication year, country or region where it was performed, study design, and follow-up duration. Demographic data included the number of participants and primary characteristics were obtained.
Both cohort studies and case-control studies were estimated the risk of bias according to the Newcastle–Ottawa quality assessment scale (NOS), with a maximum score of 9 (29). The summary scores in 0–3, 4–6, 7–9 scale were classified into high, medium, and low quality. Qualitative assessment was assessed independently by two reviewers (Wang TX and Fan YX). Any disputes were evaluated by the third author (Xi RX).
The pooled CAVD prevalence was compared between individuals with lp(a) levels <50 vs. ≥50 mg/dL and with lp(a) levels <30 vs. ≥30 mg/dL, respectively. Adjusted risk estimates of CAVD reported directly in the included studies were extracted, including odds ratios (ORs), hazard ratio (HRs), and risk ratios (RRs). Adjusted factors were currently identified risk factors related to the occurrence and development of CAVD, including age, sex, hypertension, smoking, dyslipidemia, BNP, diabetes, and obesity, and at least one of them was required to be adjusted in advance (30). And plasma Lp(a) concentration is primarily genetically determined by variation in the LPA gene (17). Studies without adjusted values were replaced with unadjusted values. Given the incidence of CAVD is <20%, the OR was approximately equal to RR (31, 32). Therefore, RRs and 95% CIs were used to estimate the combined effects.
The overall effect was calculated by a Z-test, and a two-tailed P < 0.05 was deemed statistically significant. Chi-square Cochran's Q-test and I square statistics were used to assess potential heterogeneity among studies. In detail, I square >50% was defined as high statistical heterogeneity, I square of 0–50% indicates low heterogeneity, I square = 0% indicates no heterogeneity, respectively (33). Considering the potential heterogeneity of the included studies, we employed a random-effects model to calculate the pooled RR estimates, and heterogeneity assessment with P ≤ 0.10 was considered as a significance set (34).
Subgroup analysis was performed to investigate the source of heterogeneity, based on study types, countries, and the level of adjustment (the number of covariates). Potential publication and small sample bias were evaluated by Egger's and Begg's test (35). The funnel plot was provided for visual inspection of any bias. Statistical analyses were accomplished with Stata (Version 12.0, College Station, Texas).
A total of 2,032 articles were identified in four databases. After the final full-text screening, eight eligible studies were included for systematic review and meta-analysis (27, 28, 36–41). There were seven studies assessing the association of lp(a) concentration >50 mg/dL with CAVD, five studies assessing the association of lp(a) concentration >30 mg/dL with CAVD. Figure 1 shows a detailed flow diagram.
Of the eight included studies, four were cohort studies, and four were case-control studies. Three included studies originated from America, four from Europe, and the remaining one study was unknown. The age of participants ranged from 51 to 75 years. The proportion of females was 35–57%. Five studies evaluated the association between AVS and lp(a), three estimated associations between aortic valve calcification (AVC) and lp(a). In the four included cohort studies, outcome events occurred in 1,383 of 52,134 participants during follow-up. The case-control studies comprised 425 cases and 372 controls. Characteristics of the included studies are displayed in Table 1. As shown in Table 2, seven studies reported the association using the cut-off value of 50 mg/dL of plasma lp(a), and five studies reported the association using the cut-off value of 30 mg/dL. The adjusted covariates which affected the relationship between lp(a) and CAVD in analyses are provided in Supplementary Table 1. The cardiovascular biomarkers of included studies are shown in Supplementary Table 2.
The NOS quality assessment scores ranged from 5 to 8 in cohort studies and 6 to 7 in case-control studies. Two out of four cohort studies were identified as high quality, two as moderate quality. Three out of four case-control studies were identified as high quality, one as moderate quality. Scoring details are provided in Supplementary Table 3.
Lp(a) 50 mg/dL group analysis We extracted all the effect estimates of CAVD from seven studies grouped with 50 mg/dL of lp(a) level. One study used the Cox regression to calculate the HR. The rest of the studies used logistic regression and reported ORs and RRs.
Transforming the effect estimates into RRs as described previously, we performed two analyses by study types. The summarized result of the risk ratio was 1.76 (95% CI, 1.47–2.11), which suggested elevated lp(a) level might increase the risk of CAVD by 76% (P < 0.001). And lp(a) ≥50 mg/dL might be a risk indicator for CAVD. However, the included studies had substantial statistical heterogeneity (I square = 59.2%), which needed further analysis. The association between lp(a) ≥50 mg/dL and CAVD were significant in both cohort studies (RR, 1.70; 95% CI, 1.39–2.07) and case-control studies (RR, 2.73; 95% CI, 1.41–5.28) (Figure 2).
Lp(a) 30 mg/dL group analysis Five studies, which consisted of two cohort studies and three case-control studies, examined the association between lp(a) ≥30 mg/dL and CAVD. The pooled RR was 1.28 (95% CI, 0.98–1.68), which indicated lp(a) concentration of 30 mg/dL or higher was not significantly associated with CAVD (P = 0.072; Figure 3). There was significant heterogeneity among studies (I square = 64.7%), which needed further analysis. The result of cohort studies showed that lp(a) ≥30 mg/dL might have an association with the increased risk of CAVD (RR, 1.38; 95% CI, 1.19–1.61). Whereas, the case-control studies showed the association between lp(a) and CAVD was not statistically significant (RR, 1.27; 95% CI 0.78–2.06). In summary, the lp(a) cut-off value of 50 mg/dL seemed more convincing than 30 mg/dL as a risk factor for CAVD.
When we screened the relevant studies, many focused on the relationship between lp(a) and AVC or AVS. AVC and AVS are the preclinical and post-clinical phases of CAVD, regarded as the representative of CAVD. So we analyzed the associations of lp(a) with these two outcomes, respectively. In the lp(a) 50 mg/dL group, four studies reported the association with AVS, three reported the association with AVC. The separate meta-analysis indicated that lp(a) ≥50 mg/dL was significantly correlated with AVC (RR, 1.46; 95% CI, 1.23–1.73) and AVS (RR, 1.95; 95% CI, 1.93–1.96). In the lp(a) 30 mg/dL group, two studies reported the association with AVS, three reported the association with AVC. And the relationship of lp(a) ≥30 mg/dL with AVC (RR, 1.40; 95% CI, 1.20–1.63) is stronger than with AVS (RR, 1.11; 95% CI, 0.66–1.87). As shown in Table 3.
Subgroup analyses stratified according to study types, countries, and level of adjustment, the statistically significant association between lp(a) ≥50 mg/dL and CAVD risk was observed in all subgroups. When the subgroup analysis was conducted considering the study types in lp(a) 30 mg/dL group, a significant positive effect of lp(a) ≥30 mg/dL on CAVD was noted in the cohort studies (RR, 1.38; 95% CI, 1.19–1.61), while the result of case-control studies had a positive trend without statistical significance (RR, 1.27; 95% CI, 0.78–2.06). A subgroup analysis stratified by different countries, lp(a) ≥30 mg/dL was associated with a higher risk of CAVD in Europe (RR, 1.75; 95% CI, 1.03–2.99), but not in America (RR, 1.19; 95% CI, 0.87–1.63). In a subgroup analysis by the level of adjustment (i.e., the median of the adjusted covariates), the pooled RR in studies adjusted for six or more covariates (RR, 1.39; 95% CI, 1.20–1.62) was more marked than in the less than six covariates group (RR, 1.18; 95% CI, 0.64–2.17). The details are shown in Table 4.
Publication bias assessment of the above two groups was performed using funnel plots at first. There might be asymmetry in the two diagrams figure (Supplementary Figure 1). Therefore, the Egger's test and the Begg's test were carried out for further verification, which suggested these findings might not have publication bias or small sample effect [lp(a)50 mg/dL group: Begg's test, P = 0.230; Egger's test, P = 0.499; lp(a) 30 mg/dL group: Begg's test, P > 0.99; Egger's test, P > 0.99].
We evaluated the relationship between elevated plasma lp(a) level and CAVD. The major findings were as follows: (1) The incidence of CAVD was higher in the high lp(a) level group than in the low-level group, as reported by most of the original studies. (2) The pooled results of lp(a) 50 mg/dL group supported that plasma lp(a) ≥50 mg/dL might be a risk factor for CAVD. Whereas, there was insufficient evidence for the association between plasma lp(a) ≥30 mg/dL and CAVD. Also, substantial heterogeneity between studies was not well-explained by subgroup analysis with a 30 mg/dL lp(a) cut-off value, which called for caution when interpreting the result. (3) Patients with plasma lp(a) ≥50 mg/dL might have a higher risk of CAVD than those with lp(a) ≥30 mg/dL. Therefore, we speculated that the magnitude of lp(a) concentration might have a dose-response relationship with CAVD. (4) The progression of CAVD has multiple stages, including calcification and stenosis, etc. AVC, an independent predictor of cardiovascular events (42), not only may progress to AVS (43), but increases the risk of CAD and all-cause mortality (44, 45). Severe AVS directly correlates with heart failure and death (2). Therefore, the lp(a) effect on AVC and AVS were analyzed separately. The results showed that high plasma lp(a) (≥30 mg/dL and ≥50 mg/dL) was associated with AVC, the relationship of AVS with lp(a) ≥50 mg/dL was significant but not with lp(a) ≥30 mg/dL. (5) The subgroup analysis by study types in lp(a) 30 mg/dL group concluded a positive finding in the cohort studies instead of case-control studies. Included case-control studies have the disadvantages of small sample size and the inherent nature of recall and select bias, which might cause inaccurate reporting of the results (46). And due to the larger sample size, the cohort studies had narrower confidence intervals and higher weight with more accurate estimates. Therefore, large sample cohort studies are needed to further explore the relationship between lp(a) ≥30 mg/dL and CAVD. The analysis result in Europe was more significant than in America, and no meaningful association of lp(a) ≥30 mg/dL was found in America. The participants of two American studies mainly involved Asians, Hispanics, Caucasians and Blacks, and the initial results were inconsistent among different races. However, the race of European studies was relatively homogeneous. Therefore, there are possible ethnic effect factors in the pathogenesis of lp(a)-mediated CAVD (27, 40). And a research article from the MESA study pointed out a possible race/ethnicity-related modification of lp(a) and coronary heart disease events (24). Their findings suggested that the 30 mg/dL cutoff for lp(a) is inappropriate in Caucasian and Hispanic individuals, and the higher 50 mg/dL cutoff should be considered. In contrast, the 30 mg/dL cutoff remains suitable in Black individuals. From the present evidence, the cut-off values of lp(a) differed according to different race/ethnicity groups. But most studies agreed on the cut-off values of 30 and 50 mg/dL. That's why we analyzed using both cut-off values in our meta-analysis. Our subgroup analysis results agree with the findings from Guan et al. (24). (6) The Egger's test and the Begg's test had certified that there was no publication bias in these studies. The number of included studies was <10 and the estimates we used were inherently related to their standard errors, leading to relatively imprecise test power of the funnel plot. Whereas, it was undeniable that selection bias and true heterogeneity might also be related to the asymmetry, such as unavailable unpublished studies, which inevitably lead to publication bias. And we conducted the subgroup analysis to investigate the source of heterogeneity, and this deficiency was made up to some extent.
Besides other conventional risk factors like age, sex, hypertension, and smoking, dyslipidemia played a vital role in calcific aortic valve disease (30). Lipids oxidation may promote chronic low-grade inflammation in the aortic valve, which frequently induces the osteogenic process of VICs (47–49). However, whether LDL-C or HDL-C has casual associations with CAVD remains controversial (50, 51). And two meta-analyses about the effect of statins on aortic valve stenosis demonstrated statins might not retard the progression of valve stenosis even though LDL-C concentration was reduced (52, 53). Instead, attention has turned to lp(a). A rapidly-growing body of evidence demonstrated that lp(a) has a bright prospect in predicting and treating CAVD. A previous systematic review speculated plasma lp(a) might be related to the occurrence and progression of AVS. However, this study did not perform a quantitative meta-analysis (54). Our work, which has been thoroughly searched, rigorously screened and quantitatively analyzed, found that lp(a) ≥50 mg/dL could potentially be a proper risk factor for CAVD. Therefore, lp(a) concentration could be more suitable for assessing the incidence of CAVD, compared with that of LDL-C and HDL-C.
Several plausible mechanisms may account for the underlying pathophysiology of lp(a)-mediated CAVD. Lp(a) is a low-density lipoprotein-like structure, which mainly contains apolipoprotein B (apo B) covalently bound to apolipoprotein(a) [apo(a)] (55, 56), transporting some pro-inflammatory and pro-osteogenic mediators. When aortic valve leaflets are damaged to mechanical or shear stress, excessive lp(a) could infiltrate and accumulate the valve to stimulate inflammation, calcification, and fibrosis (57–59). Oxidized phospholipids (OxPL), primarily carried by lp(a) complexes, has been demonstrated the association with CAVD (60, 61). Zheng et al. have revealed that the high content of lp(a) and OxPL-apoB was independently associated with increased active tissue calcification and clinical events such as AVR or all-cause mortality (12). OxPL might be hydrolyzed to lysophosphatidylcholine (LPC) in the presence of phospholipases, promoting valvular inflammation, thickening and mineralization (62). Lipoprotein-associated phospholipase A2 (Lp-PLA2) and autotaxin (ATX) might be the key phospholipases in the metabolism of lp(a)-OxPL. Lp-PLA2, with a high affinity to lp(a), could convert OxPL into LPC, increasing the expression of phosphate-related genes (63, 64). ATX, mainly combined with apo(a) of lp(a) particles, could transform LPC into LysoPA, which drives the inflammatory and osteogenic program through the NF-κB/IL-6/BMP pathway (41, 65). In addition, lp(a) could directly mediate remodeling and calcification of VICs through inducing MAPKs signaling pathway and the expression of pro-osteogenic factors like bone-specific transcription factor SP7 (osterix), BMP-2, and BMP-4 (66).
Welsh et al. revealed reducing the baseline lp(a) levels by 80%, patients with lp(a)≥175 nmol/l and baseline CVD could decrease the risk of CVD by 20% (67). And the conclusions of this study suggested that lowering lp(a) levels might be an important way to intervene CAVD. Pharmacotherapies of lp(a) include PCSK9 inhibitor and nucleic acid antisense, etc. In the FOURIER study, it was found that PCSK9 inhibitors could reduce the plasma Lp(a) level on average 26.9%, and patients with higher Lp(a) levels had a 23% lower risk of major cardiovascular events (68). In the ODYSSEY OUTCOMES trial, the reduction of Lp(a) levels by PCSK9 inhibitors was associated with a decrease in the risk of cardiovascular disease (69). Mipomersen is a 2′-O-methoxyethyl modified second-generation antisense oligonucleotide, which binds to homologous Apo B messenger RNA to inhibit the synthesis of ApoB-100. It can significantly reduce Apo B and Lp(a) levels (70). IONIS-APO(a)Rx, an oligonucleotide targeting lp(a), could reduce lp(a) levels by 66–92%, OxPL-ApoB and OxPL-apo(a) decreased moderately, which play a vital role in pathogenesis of CAVD (71).
There are several strengths of our meta-analysis. Firstly, this is the first meta-analysis assessing the association between lp(a) and CAVD. Secondly, our analysis included a comprehensive search strategy, a considerable number of participants, a subgroup analysis for heterogeneity. And we integrated all relevant cross-sectional and case-control studies, allowing us to determine whether there was a significant relationship between lp(a) and CAVD. Thirdly, most of the included studies have controlled the confounders, which ensured the reliability of the outcomes. Finally, we investigated the relationship between high lp(a) levels (≥50 and ≥30 mg/dL) and the incidence of CAVD, respectively, which provided some guidance for patients with elevated plasma lp(a) levels to assess their risk of CAVD. However, heterogeneity was observed in our meta-analysis, even though most of studies had adjusted multiple potential confounders. Therefore, the results of the present analyses should be interpreted with caution.
Based on the current cohort studies and case-control studies, we could conclude that patients with lp(a) ≥50 mg/dL were at a significantly high risk of CAVD. However, further large-scale prospective cohort studies with high quality and adequate control for confounders are necessary to draw a firm conclusion on the causality between plasma lp(a) and CAVD.
The original contributions presented in the study are included in the article/Supplementary Material, further inquiries can be directed to the corresponding author/s.
QL and JJ conceived the study. QL and YY searched the studies, screened the studies, analyzed the data, and wrote the manuscript. QL and RL extracted the data. TW, YF, and RX assessed the risk of bias. JL, JJ, and HX reviewed and revised the manuscript. All authors contributed to the article and approved the submitted version.
This research was supported by grant CI2021A00917 from the China Academy of Chinese Medical Sciences Innovation Fund, grant ZYYCXTD-C-202007 from the Innovation Team and Talents Cultivation Program of National Administration of Traditional Chinese Medicine, and grant ZZ13-YQ-017 from the Central Public Welfare Research Institutes of China Academy of Chinese Medical Sciences.
The authors declare that the research was conducted in the absence of any commercial or financial relationships that could be construed as a potential conflict of interest.
All claims expressed in this article are solely those of the authors and do not necessarily represent those of their affiliated organizations, or those of the publisher, the editors and the reviewers. Any product that may be evaluated in this article, or claim that may be made by its manufacturer, is not guaranteed or endorsed by the publisher.
The authors thank Xiyuan Hospital, China Academy of Chinese Medical Sciences, for their support of this work and the reviewers for allowing us to make improvements to the manuscript.
The Supplementary Material for this article can be found online at: https://www.frontiersin.org/articles/10.3389/fcvm.2022.877140/full#supplementary-material
1. Yadgir S, Johnson CO, Aboyans V, Adebayo OM, Adedoyin RA, Afarideh M, et al. Global, regional, and national burden of calcific aortic valve and degenerative mitral valve diseases, 1990-2017. Circulation. (2020) 141:1670–80. doi: 10.1161/circulationaha.119.043391
2. Lindman BR, Clavel MA, Mathieu P, Iung B, Lancellotti P, Otto CM, et al. Calcific aortic stenosis. Nat Rev Dis Prim. (2016) 2:16006. doi: 10.1038/nrdp.2016.6
3. Dutta P, Lincoln J. Calcific aortic valve disease: a developmental biology perspective. Curr Cardiol Rep. (2018) 20:21. doi: 10.1007/s11886-018-0968-9
4. Otto CM, Nishimura RA, Bonow RO, Carabello BA, Erwin JP III, Gentile F, et al. 2020 ACC/AHA guideline for the management of patients with valvular heart disease: executive summary: a report of the American College of Cardiology/American Heart Association Joint Committee on Clinical Practice Guidelines. Circulation. (2021). 143:e35–71. doi: 10.1161/cir.0000000000000932
5. Kraler S, Blaser MC, Aikawa E, Camici GG, Lüscher TF. Calcific aortic valve disease: from molecular and cellular mechanisms to medical therapy. Eur Heart J. (2022) 43:683–97. doi: 10.1093/eurheartj/ehab757
6. Andersson C, Abdulla J. Is the use of renin-angiotensin system inhibitors in patients with aortic valve stenosis safe and of prognostic benefit? A systematic review and meta-analysis. Eur Heart J Cardiovasc Pharmacother. (2017) 3:21–7. doi: 10.1093/ehjcvp/pvw027
7. Chan KL, Teo K, Dumesnil JG, Ni A, Tam J. Effect of Lipid lowering with rosuvastatin on progression of aortic stenosis: results of the aortic stenosis progression observation: measuring effects of rosuvastatin (ASTRONOMER) trial. Circulation. (2010) 121:306–14. doi: 10.1161/circulationaha.109.900027
8. Rossebø AB, Pedersen TR, Boman K, Brudi P, Chambers JB, Egstrup K, et al. Intensive lipid lowering with simvastatin and ezetimibe in aortic stenosis. N Engl J Med. (2008) 359:1343–56. doi: 10.1056/NEJMoa0804602
9. Dichtl W, Alber HF, Feuchtner GM, Hintringer F, Reinthaler M, Bartel T, et al. Prognosis and risk factors in patients with asymptomatic aortic stenosis and their modulation by atorvastatin (20 mg). Am J Cardiol. (2008) 102:743–8. doi: 10.1016/j.amjcard.2008.04.060
10. Enas EA, Varkey B, Dharmarajan TS, Pare G, Bahl VK. Lipoprotein(a): an independent, genetic, and causal factor for cardiovascular disease and acute myocardial infarction. Indian Heart J. (2019) 71:99–112. doi: 10.1016/j.ihj.2019.03.004
11. Nordestgaard BG, Langsted A. Lipoprotein (a) as a cause of cardiovascular disease: insights from epidemiology, genetics, and biology. J Lipid Res. (2016) 57:1953–75. doi: 10.1194/jlr.R071233
12. Zheng KH, Tsimikas S, Pawade T, Kroon J, Jenkins WSA, Doris MK, et al. Lipoprotein(a) and oxidized phospholipids promote valve calcification in patients with aortic stenosis. J Am Coll Cardiol. (2019) 73:2150–62. doi: 10.1016/j.jacc.2019.01.070
13. Yu B, Khan K, Hamid Q, Mardini A, Siddique A, Aguilar-Gonzalez LP, et al. Pathological significance of lipoprotein(a) in aortic valve stenosis. Atherosclerosis. (2018) 272:168–74. doi: 10.1016/j.atherosclerosis.2018.03.025
14. Thanassoulis G, Campbell CY, Owens DS, Smith JG, Smith AV, Peloso GM, et al. Genetic associations with valvular calcification and aortic stenosis. N Engl J Med. (2013) 368:503–12. doi: 10.1056/NEJMoa1109034
15. Cairns BJ, Coffey S, Travis RC, Prendergast B, Green J, Engert JC, et al. A replicated, genome-wide significant association of aortic stenosis with a genetic variant for lipoprotein(a): meta-analysis of published and novel data. Circulation. (2017) 135:1181–3. doi: 10.1161/circulationaha.116.026103
16. Arsenault BJ, Boekholdt SM, Dubé MP, Rhéaume E, Wareham NJ, Khaw KT, et al. Lipoprotein(a) levels, genotype, and incident aortic valve stenosis: a prospective Mendelian randomization study and replication in a case-control cohort. Circ Cardiovasc Genet. (2014) 7:304–10. doi: 10.1161/circgenetics.113.000400
17. Kamstrup PR, Tybjærg-Hansen A, Nordestgaard BG. Elevated lipoprotein(a) and risk of aortic valve stenosis in the general population. J Am Coll Cardiol. (2014) 63:470–7. doi: 10.1016/j.jacc.2013.09.038
18. Ljungberg J, Holmgren A, Bergdahl IA, Hultdin J, Norberg M, Näslund U, et al. Lipoprotein(a) and the apolipoprotein B/A1 ratio independently associate with surgery for aortic stenosis only in patients with concomitant coronary artery disease. J Am Heart Assoc. (2017) 6:e007160. doi: 10.1161/jaha.117.007160
19. Mahabadi AA, Kahlert P, Kahlert HA, Dykun I, Balcer B, Forsting M, et al. Comparison of lipoprotein(a)-levels in patients ≥70 years of age with versus without aortic valve stenosis. Am J Cardiol. (2018) 122:645–9. doi: 10.1016/j.amjcard.2018.04.046
20. Moher D, Liberati A, Tetzlaff J, Altman DG. Preferred reporting items for systematic reviews and meta-analyses: the PRISMA statement. BMJ. (2009) 339:b2535. doi: 10.1136/bmj.b2535
21. Stroup DF, Berlin JA, Morton SC, Olkin I, Williamson GD, Rennie D, et al. Meta-analysis of observational studies in epidemiology: a proposal for reporting. Meta-analysis Of Observational Studies in Epidemiology (MOOSE) group. JAMA. (2000) 283:2008–12. doi: 10.1001/jama.283.15.2008
22. Catapano AL, Graham I, De Backer G, Wiklund O, Chapman MJ, Drexel H, et al. 2016 ESC/EAS guidelines for the management of dyslipidaemias: the task force for the management of dyslipidaemias of the European Society of Cardiology (ESC) and European Atherosclerosis Society (EAS) Developed with the special contribution of the European Association for Cardiovascular Prevention & Rehabilitation (EACPR). Atherosclerosis. (2016). 253:281–344. doi: 10.1016/j.atherosclerosis.2016.08.018
23. Nordestgaard BG, Chapman MJ, Ray K, Borén J, Andreotti F, Watts GF, et al. Lipoprotein(a) as a cardiovascular risk factor: current status. Eur Heart J. (2010) 31:2844–53. doi: 10.1093/eurheartj/ehq386
24. Guan W, Cao J, Steffen BT, Post WS, Stein JH, Tattersall MC, et al. Race is a key variable in assigning lipoprotein(a) cutoff values for coronary heart disease risk assessment: the Multi-Ethnic Study of Atherosclerosis. Arterioscler Thromb Vasc Biol. (2015) 35:996–1001. doi: 10.1161/atvbaha.114.304785
25. Anderson TJ, Grégoire J, Pearson GJ, Barry AR, Couture P, Dawes M, et al. 2016 Canadian Cardiovascular Society Guidelines for the management of dyslipidemia for the prevention of cardiovascular disease in the adult. Can J Cardiol. (2016). 32:1263–82. doi: 10.1016/j.cjca.2016.07.510
26. Li JJ, Ma CS, Zhao D, Yan XW, Ye P, Chen H, et al. Expert statement on the relationship between lipoprotein(a) and cardiovascular disease risk and clinical management. Chin Circu J. (2021) 36:1158–67. doi: 10.3969/j.issn.1000-3614.2021.12.003
27. Cao J, Steffen BT, Budoff M, Post WS, Thanassoulis G, Kestenbaum B, et al. Lipoprotein(a) levels are associated with subclinical calcific aortic valve disease in white and black individuals: the multi-ethnic study of atherosclerosis. Arterioscler Thromb Vasc Biol. (2016) 36:1003–9. doi: 10.1161/atvbaha.115.306683
28. Zheng KH, Arsenault BJ, Kaiser Y, Khaw KT, Wareham NJ, Stroes ESG, et al. apoB/apoA-I ratio and Lp(a) associations with aortic valve stenosis incidence: insights from the EPIC-Norfolk prospective population study. J Am Heart Assoc. (2019) 8:e013020. doi: 10.1161/jaha.119.013020
29. Stang A. Critical evaluation of the Newcastle-Ottawa scale for the assessment of the quality of nonrandomized studies in meta-analyses. Eur J Epidemiol. (2010) 25:603–5. doi: 10.1007/s10654-010-9491-z
30. Small A, Kiss D, Giri J, Anwaruddin S, Siddiqi H, Guerraty M, et al. Biomarkers of Calcific Aortic Valve Disease. Arterioscler Thromb Vasc Biol. (2017) 37:623–32. doi: 10.1161/atvbaha.116.308615
31. Yi B, Zeng W, Lv L, Hua P. Changing epidemiology of calcific aortic valve disease: 30-year trends of incidence, prevalence, and deaths across 204 countries and territories. Aging. (2021) 13:12710–32. doi: 10.18632/aging.202942
32. Grant RL. Converting an odds ratio to a range of plausible relative risks for better communication of research findings. BMJ. (2014) 348:f7450. doi: 10.1136/bmj.f7450
33. Higgins JP, Thompson SG. Quantifying heterogeneity in a meta-analysis. Stat Med. (2002) 21:1539–58. doi: 10.1002/sim.1186
34. Borenstein M, Hedges LV, Higgins JP, Rothstein HR. A basic introduction to fixed-effect and random-effects models for meta-analysis. Res Synth Methods. (2010) 1:97–111. doi: 10.1002/jrsm.12
35. Lin L, Chu H. Quantifying publication bias in meta-analysis. Biometrics. (2018) 74:785–94. doi: 10.1111/biom.12817
36. Afshar M, Kamstrup PR, Williams K, Sniderman AD, Nordestgaard BG, Thanassoulis G. Estimating the population impact of lp(a) lowering on the incidence of myocardial infarction and aortic stenosis-brief report. Arterioscler Thromb Vasc Biol. (2016) 36:2421–3. doi: 10.1161/atvbaha.116.308271
37. Glader CA, Birgander LS, Söderberg S, Ildgruben HP, Saikku P, Waldenström A, et al. Lipoprotein(a), Chlamydia pneumoniae, leptin and tissue plasminogen activator as risk markers for valvular aortic stenosis. Eur Heart J. (2003) 24:198–208. doi: 10.1016/s0195-668x(02)00385-8
38. Vongpromek R, Bos S, Ten Kate GJ, Yahya R, Verhoeven AJ, de Feyter PJ, et al. Lipoprotein(a) levels are associated with aortic valve calcification in asymptomatic patients with familial hypercholesterolaemia. J Intern Med. (2015) 278:166–73. doi: 10.1111/joim.12335
39. Wilkinson MJ, Ma GS, Yeang C, Ang L, Strachan M, DeMaria AN, et al. The prevalence of lipoprotein(a) measurement and degree of elevation among 2710 patients with calcific aortic valve stenosis in an academic echocardiography laboratory setting. Angiology. (2017) 68:795–8. doi: 10.1177/0003319716688415
40. Makshood M, Joshi PH, Kanaya AM, Ayers C, Budoff M, Tsai MY, et al. Lipoprotein (a) and aortic valve calcium in South Asians compared to other race/ethnic groups. Atherosclerosis. (2020) 313:14–19. doi: 10.1016/j.atherosclerosis.2020.09.010
41. Nsaibia MJ, Mahmut A, Boulanger MC, Arsenault BJ, Bouchareb R, Simard S, et al. Autotaxin interacts with lipoprotein(a) and oxidized phospholipids in predicting the risk of calcific aortic valve stenosis in patients with coronary artery disease. J Intern Med. (2016) 280:509–17. doi: 10.1111/joim.12519
42. Owens DS, Budoff MJ, Katz R, Takasu J, Shavelle DM, Carr JJ, et al. Aortic valve calcium independently predicts coronary and cardiovascular events in a primary prevention population. JACC Cardiovasc Imaging. (2012) 5:619–25. doi: 10.1016/j.jcmg.2011.12.023
43. Cueff C, Serfaty JM, Cimadevilla C, Laissy JP, Himbert D, Tubach F, et al. Measurement of aortic valve calcification using multislice computed tomography: correlation with haemodynamic severity of aortic stenosis and clinical implication for patients with low ejection fraction. Heart. (2011) 97:721–6. doi: 10.1136/hrt.2010.198853
44. Utsunomiya H, Yamamoto H, Kunita E, Kitagawa T, Ohashi N, Oka T, et al. Combined presence of aortic valve calcification and mitral annular calcification as a marker of the extent and vulnerable characteristics of coronary artery plaque assessed by 64-multidetector computed tomography. Atherosclerosis. (2010) 213:166–72. doi: 10.1016/j.atherosclerosis.2010.08.070
45. Blaha MJ, Budoff MJ, Rivera JJ, Khan AN, Santos RD, Shaw LJ, et al. Relation of aortic valve calcium detected by cardiac computed tomography to all-cause mortality. Am J Cardiol. (2010) 106:1787–91. doi: 10.1016/j.amjcard.2010.08.019
46. Setia MS. Methodology Series Module 2: Case-control Studies. Indian J Dermatol. (2016) 61:146–51. doi: 10.4103/0019-5154.177773
47. Nadlonek NA, Lee JH, Weyant MJ, Meng X, Fullerton DA. Ox-LDL induces PiT-1 expression in human aortic valve interstitial cells. J Surg Res. (2013) 184:6–9. doi: 10.1016/j.jss.2013.05.001
48. Audet A, Côté N, Couture C, Bossé Y, Després JP, Pibarot P, et al. Amyloid substance within stenotic aortic valves promotes mineralization. Histopathology. (2012) 61:610–9. doi: 10.1111/j.1365-2559.2012.04265.x
49. Abdelbaky A, Corsini E, Figueroa AL, Subramanian S, Fontanez S, Emami H, et al. Early aortic valve inflammation precedes calcification: a longitudinal FDG-PET/CT study. Atherosclerosis. (2015) 238:165–72. doi: 10.1016/j.atherosclerosis.2014.11.026
50. Hofmanis J, Hofmane D, Svirskis S, Mackevics V, Tretjakovs P, Lejnieks A, et al. HDL-C role in acquired aortic valve stenosis patients and its relationship with oxidative stress. Medicina. (2019) 55:416. doi: 10.3390/medicina55080416
51. Nazarzadeh M, Pinho-Gomes AC, Bidel Z, Dehghan A, Canoy D, Hassaine A, et al. Plasma lipids and risk of aortic valve stenosis: a Mendelian randomization study. Eur Heart J. (2020) 41:3913–20. doi: 10.1093/eurheartj/ehaa070
52. Zhao Y, Nicoll R, He YH, Henein MY. The effect of statins on valve function and calcification in aortic stenosis: a meta-analysis. Atherosclerosis. (2016) 246:318–24. doi: 10.1016/j.atherosclerosis.2016.01.023
53. De Vecchis R, Di Biase G, Esposito C, Ciccarelli A, Cioppa C, Giasi A, et al. Statin use for nonrheumatic calcific aortic valve stenosis: a review with meta-analysis. J Cardiovasc Med. (2013) 14:559–67. doi: 10.2459/JCM.0b013e3283587267
54. Guddeti RR, Patil S, Ahmed A, Sharma A, Aboeata A, Lavie CJ, et al. Lipoprotein(a) and calcific aortic valve stenosis: a systematic review. Prog Cardiovasc Dis. (2020) 63:496–502. doi: 10.1016/j.pcad.2020.06.002
55. Schmidt K, Noureen A, Kronenberg F, Utermann G. Structure, function, and genetics of lipoprotein(a). J Lipid Res. (2016) 57:1339–59. doi: 10.1194/jlr.R067314
56. Ehnholm C, Garoff H, Renkonen O, Simons K. Protein and carbohydrate composition of Lp(a)lipoprotein from human plasma. Biochemistry. (1972) 11:3229–32. doi: 10.1021/bi00767a015
57. Thanassoulis G. Lipoprotein (a) in calcific aortic valve disease: from genomics to novel drug target for aortic stenosis. J Lipid Res. (2016) 57:917–24. doi: 10.1194/jlr.R051870
58. Nielsen LB, Stender S, Kjeldsen K, Nordestgaard BG. Specific accumulation of lipoprotein(a) in balloon-injured rabbit aorta in vivo. Circ Res. (1996) 78:615–26. doi: 10.1161/01.res.78.4.615
59. Hsieh G, Rizk T, Berman AN, Biery DW, Blankstein R. The current landscape of lipoprotein(a) in calcific aortic valvular disease. Curr Opin Cardiol. (2021) 36:542–8. doi: 10.1097/hco.0000000000000901
60. Kamstrup PR, Hung MY, Witztum JL, Tsimikas S, Nordestgaard BG. Oxidized phospholipids and risk of calcific aortic valve disease: the Copenhagen general population study. Arterioscler Thromb Vasc Biol. (2017) 37:1570–8. doi: 10.1161/atvbaha.116.308761
61. Capoulade R, Chan KL, Yeang C, Mathieu P, Bossé Y, Dumesnil JG, et al. Oxidized phospholipids, lipoprotein(a), and progression of calcific aortic valve stenosis. J Am Coll Cardiol. (2015) 66:1236–46. doi: 10.1016/j.jacc.2015.07.020
62. Youssef A, Clark JR, Koschinsky ML, Boffa MB. Lipoprotein(a): expanding our knowledge of aortic valve narrowing. Trends Cardiovasc Med. (2021) 31:305–311. doi: 10.1016/j.tcm.2020.06.001
63. Stafforini DM. Biology of platelet-activating factor acetylhydrolase (PAF-AH, lipoprotein associated phospholipase A2). Cardiovasc Drugs Ther. (2009) 23:73–83. doi: 10.1007/s10557-008-6133-8
64. Vickers KC, Castro-Chavez F, Morrisett JD. Lyso-phosphatidylcholine induces osteogenic gene expression and phenotype in vascular smooth muscle cells. Atherosclerosis. (2010) 211:122–9. doi: 10.1016/j.atherosclerosis.2010.04.005
65. Bouchareb R, Mahmut A, Nsaibia MJ, Boulanger MC, Dahou A, Lépine JL, et al. Autotaxin derived from lipoprotein(a) and valve interstitial cells promotes inflammation and mineralization of the aortic valve. Circulation. (2015) 132:677–90. doi: 10.1161/circulationaha.115.016757
66. Yu B, Hafiane A, Thanassoulis G, Ott L, Filwood N, Cerruti M, et al. Lipoprotein(a) induces human aortic valve interstitial cell calcification. JACC Basic Transl Sci. (2017) 2:358–71. doi: 10.1016/j.jacbts.2017.03.015
67. Welsh P, Welsh C, Celis-Morales CA, Brown R, Ho FK, Ferguson LD, et al. Lipoprotein(a) and cardiovascular disease: prediction, attributable1 risk fraction, and estimating benefits from novel interventions. Eur J Prev Cardiol. (2022) 28:1991–2000. doi: 10.1093/eurjpc/zwaa063
68. Bittner VA, Szarek M, Aylward PE, Bhatt DL, Diaz R, Edelberg JM, et al. Effect of alirocumab on lipoprotein(a) and cardiovascular risk after acute coronary syndrome. J Am Coll Cardiol. (2020) 75:133–44. doi: 10.1016/j.jacc.2019.10.057
69. Szarek M, Bittner VA, Aylward P, Baccara-Dinet M, Bhatt DL, Diaz R, et al. Lipoprotein(a) lowering by alirocumab reduces the total burden of cardiovascular events independent of low-density lipoprotein cholesterol lowering: ODYSSEY OUTCOMES trial. Eur Heart J. (2020) 41:4245–55. doi: 10.1093/eurheartj/ehaa649
70. Santos RD, Raal FJ, Catapano AL, Witztum JL, Steinhagen-Thiessen E, Tsimikas S. Mipomersen, an antisense oligonucleotide to apolipoprotein B-100, reduces lipoprotein(a) in various populations with hypercholesterolemia: results of 4 phase III trials. Arterioscler Thromb Vasc Biol. (2015) 35:689–99. doi: 10.1161/atvbaha.114.304549
71. Viney NJ, van Capelleveen JC, Geary RS, Xia S, Tami JA, Yu RZ, et al. Antisense oligonucleotides targeting apolipoprotein(a) in people with raised lipoprotein(a): two randomised, double-blind, placebo-controlled, dose-ranging trials. Lancet. (2016) 388:2239–53. doi: 10.1016/s0140-6736(16)31009-1
Keywords: lipoprotein(a), calcific aortic valve disease, aortic valve stenosis, aortic valve calcification, systematic review and meta-analysis
Citation: Liu Q, Yu Y, Xi R, Li J, Lai R, Wang T, Fan Y, Zhang Z, Xu H and Ju J (2022) Association Between Lipoprotein(a) and Calcific Aortic Valve Disease: A Systematic Review and Meta-Analysis. Front. Cardiovasc. Med. 9:877140. doi: 10.3389/fcvm.2022.877140
Received: 16 February 2022; Accepted: 28 March 2022;
Published: 25 April 2022.
Edited by:
Ronak Rajani, Guy's and St Thomas' NHS Foundation Trust, United KingdomReviewed by:
Simon Kraler, University of Zurich, SwitzerlandCopyright © 2022 Liu, Yu, Xi, Li, Lai, Wang, Fan, Zhang, Xu and Ju. This is an open-access article distributed under the terms of the Creative Commons Attribution License (CC BY). The use, distribution or reproduction in other forums is permitted, provided the original author(s) and the copyright owner(s) are credited and that the original publication in this journal is cited, in accordance with accepted academic practice. No use, distribution or reproduction is permitted which does not comply with these terms.
*Correspondence: Hao Xu, eHVoYW90Y21AaG90bWFpbC5jb20=; Jianqing Ju, anVqaWFucWluZ0AxNjMuY29t
†These authors have contributed equally to this work
Disclaimer: All claims expressed in this article are solely those of the authors and do not necessarily represent those of their affiliated organizations, or those of the publisher, the editors and the reviewers. Any product that may be evaluated in this article or claim that may be made by its manufacturer is not guaranteed or endorsed by the publisher.
Research integrity at Frontiers
Learn more about the work of our research integrity team to safeguard the quality of each article we publish.