- 1Department of Cardiology, Cardiovascular Imaging Center, The Second Affiliated Hospital of Harbin Medical University, Harbin, China
- 2Department of Vascular Surgery, Beijing Hospital, National Center of Gerontology, Beijing, China
- 3Institute of Geriatric Medicine, Chinese Academy of Medical Sciences, Beijing, China
- 4Department of Cardiology, The Second Affiliated Hospital of Harbin Medical University, Harbin, China
- 5The Key Laboratory of Myocardial Ischemia, Chinese Ministry of Education, Harbin, China
Background: The differentiation between hypertrophic cardiomyopathy (HCM) and hypertensive heart disease (HHD) is challenging due to similar myocardial hypertrophic phenotype. The purpose of this study is to evaluate the feasibility of cardiovascular magnetic resonance feature tracking (CMR-FT) and late gadolinium enhancement (LGE) to distinguish between HCM and HHD and the potential relationship between myocardial strain and cardiac functional parameters.
Methods: One hundred and seventy subjects (57 HCM, 45 HHD, and 68 controls) underwent 3.0 T CMR, including steady-state free precession cines and LGE images. Global and segmental (basal, mid, and apical) analyses of myocardial radial, circumferential, longitudinal strain, and left ventricular (LV) torsion, as well as global and 16 segments of LGE were assessed. The multivariate analysis was used to predict the diagnostic ability by combining comprehensive myocardial strain parameters and LGE.
Results: Global radial strain (GRS), global circumferential strain (GCS), and LV torsion were significantly higher in the HCM group than in the HHD group (GRS, 21.18 ± 7.52 vs. 14.56 ± 7.46%; GCS, −13.34 ± 3.52 vs. −10.11 ± 4.13%; torsion, 1.79 ± 0.69 vs. 1.23 ± 0.65 deg/cm, all P < 0.001). A similar trend was also seen in the corresponding strain rate. As for segmental strain analysis, basal radial strain (BRS), basal circumferential strain (BCS), basal longitudinal strain (BLS), mid-radial strain (MRS), and mid-circumferential strain (MCS) were higher in the HCM group than in the HHD group (all P < 0.001). The receiver operating characteristic (ROC) results showed that the area under the curve (AUC) of LGE in the mid-interventricular septum (mIVS) was the highest among global and segmental LGE analyses. On the multivariate regression analysis, a combined model of LGE (mIVS) with GRS obtained the highest AUC value, which was 0.835 with 88.89% sensitivity and 70.18% specificity, respectively. In addition, for patients with HCM, GRS, GCS, and global longitudinal strain had correlations with LV ejection fraction (LVEF), maximum interventricular septum thickness (IVST max), and left ventricular mass index (LVMi). Torsion was mildly associated with LVEF.
Conclusion: CMR-FT-derived myocardial strain and torsion provided valuable methods for evaluation of HCM and HHD. In addition, the combination of GRS and LGE (mIVS) achieved the highest diagnostic value.
Introduction
Hypertrophic cardiomyopathy (HCM) is the most common genetically transmitted cardiomyopathy (1). HCM is diagnosed according to the European Society of Cardiology (ESC) guidelines with unexplained left ventricular (LV) hypertrophy (LVH) and a maximal wall thickness ≥ 15 mm (2). Hypertensive heart disease (HHD) is characterized by extensive structural remodeling with a dilated LV cavity and increased LV wall thickness (LVWT) as a result of enhanced arterial pressure (3). Both the HCM and HHD are associated with LVH (2, 4), which can make their differentiation challenging. The increase in LVWT can be formed by increased afterload, changes of the myocardial structure, and alterations caused by genetic defects or infiltrative disease (5). Treatment and risk stratification of the two diseases are totally different; therefore, it is particularly vital and necessary to diagnose and distinguish between these two diseases.
Cardiovascular magnetic resonance (CMR) is the gold standard for evaluation of cardiac morphology, function, and tissue characterization. The presence of late gadolinium enhancement (LGE) represents changes in fibrosis or myocardial scarring, which can significantly predict the prognosis of severe cardiac complications (6). A previous study detected more prevalent mid-wall LGE in patients with HCM relative to patients with HHD (7). Another study demonstrated that LGE served as a significant diagnostic index to discriminate between HCM and HHD by using a semiquantitative score system (5). The interventricular septum (IVS) is the most predominantly hypertrophied segment in HCM disease. While the IVS is also involved in HHD, LV wall thickness tends to be concentric rather than asymmetric for this cohort. Evaluation of LGE in the mid-wall of the IVS might offer novel insights to better characterize these two hypertrophied cohorts.
Cardiovascular magnetic resonance feature tracking (CMR-FT) technology allows for high-resolution evaluation of myocardial deformation and the determination of myocardial strain parameters with excellent consistency and reproducibility (8–10). It tracks tissue motion between epicardial and endocardial borders and measures changes of cardiac dimensions throughout the whole cardiac cycle, thus potentially distinguishing the normal and abnormal cardiac functions (11). Global longitudinal strain (GLS) was significantly higher in the HCM group than in the HHD group and the circumferential strain difference between the endocardium and epicardium has been shown to identify preclinical and overt HCM (8, 12). However, most studies depicted cardiac systolic dysfunction at a global level, rather than at a regional myocardial function level.
Left ventricular torsion obtained from CMR-FT can be used to evaluate the ability of rotation during left ventricular motion, which plays a crucial role in pumping blood out of the heart. As for patients with dilated cardiomyopathy, amplitude of torsion was lower than that in healthy subjects and it was associated with LVEF and clinical endpoints (13, 14). However, LV torsion has not been widely used to characterize HCM and HHD. Moreover, limited studies focused on comprehensive strain analysis for differentiating HCM from HHD, especially the lack of combination with LGE images.
The purpose of this study is to evaluate the feasibility of CMR-FT and LGE to distinguish between HCM and HHD and to investigate the potential relationship between CMR-FT strain and cardiac functional parameters.
Methods
A systematic retrospective analysis was conducted in one hundred and seventy consecutive subjects who underwent CMR from September 2020 to November 2021. All the images exhibited diagnostic quality, enabling the assessment of myocardial strain and LGE. Three cohorts were recruited: (i) 68 healthy volunteers who were free of any history of medical conditions; (ii) 57 patients with HCM, which was defined as nondilated left ventricular hypertrophy with end-diastolic wall thickness ≥ 15 mm (or ≥ 13 mm whose first-degree relatives had diagnosis of HCM) according to current established CMR diagnostic criteria (2); and (iii) 45 patients with HHD, which was defined as increased LVWT (≥12 mm) in the context of concomitant systemic hypertension (3). The exclusion criteria included: subjects with other diseases that accounted for increased LVWT, such as aortic valve disease, infiltrative disease (cardiac amyloidosis, Anderson–Fabry disease, and Danon disease), and systemic disease. Patients with MRI conventional contraindications were also excluded, such as implanted pacemakers, metallic intracranial implants, claustrophobia, and renal function impairment. Apical HCM was excluded, as it was readily differentiated from HHD. This study was approved by local ethics committee and all the subjects gave a written informed consent.
Cardiovascular MRI was performed with a 3.0-Tesla system (Ingenia CX, Philips Healthcare, The Netherlands) using a 32-channel phased-array abdomen coil. Cine images were performed by using a steady-state free precession (SSFP) sequence with a breath-hold and ECG trigger for cardiac morphologic and functional analyses. The scanning parameters were as follows: repetition time (TR)/echo time (TE) = 2.8/1.42 ms, field of view (FOV) = 300 × 300 mm2, voxel = 1.8 × 1.6 × 8.0 mm, flip angle = 45°, and 8-mm slice thickness. LGE images were acquired 10 min after intravenous injection of 0.1 mmol/kg of gadolinium-based contrast agent (Bayer Healthcare, Germany) by using a three-dimensional phase-sensitive inversion recovery (PSIR) sequence; the scanning parameters were as follows: TR/TE = 6.1/3.0 ms, FOV = 300 × 300 mm2, voxel = 1.8 × 1.68 × 8.0 mm, flip angle = 25°, and 8-mm slice thickness. The acquisitions of SSFP cines and PSIR were conducted in 2-chamber, 3-chamber, and 4-chamber long-axis planes, as well as a stack of contiguous short-axis slices, which encompassed the left ventricular from the atrioventricular ring to the apex.
All the CMR studies were postprocessed using a commercially available workstation (cvi42, Circle Cardiovascular Imaging Incorporation, Calgary, Alberta, Canada). LV endocardial and epicardial borders were delineated automatically with manual calibrations throughout the cardiac cycle by a radiologist (with 3 years of experience in MRI) who was blinded to clinical information. LVEF, cardiac output (CO), end-diastolic volume (EDV), end-systolic volume (ESV), interventricular septal thickness (IVST), and cardiac chamber dimensions were measured. LV mass was calculated as the total myocardium volume multiplied by myocardial gravity (1.05 g/ml) without including papillary muscles.
Cardiovascular magnetic resonance feature tracking-derived strain and strain rate were used to evaluate myocardial deformation. Quantitative parameters included radial, circumferential, and longitudinal orientation (Figure 1). Radial strain and circumferential strain were calculated from two-dimensional short-axis planes and longitudinal strain was derived from two-dimensional long-axis plane. Regional strain analysis was also performed by dividing left ventricular into basal, mid, and apical segments by 2-dimension. Strain rate was measured as a derivative of the strain tensor and it represented the rate of deformation (15). The LV torsion was calculated as the ratio of the peak difference of ventricular apical and basal rotation at the same time point in the cardiac cycle to the distance between their short-axis slices (13).
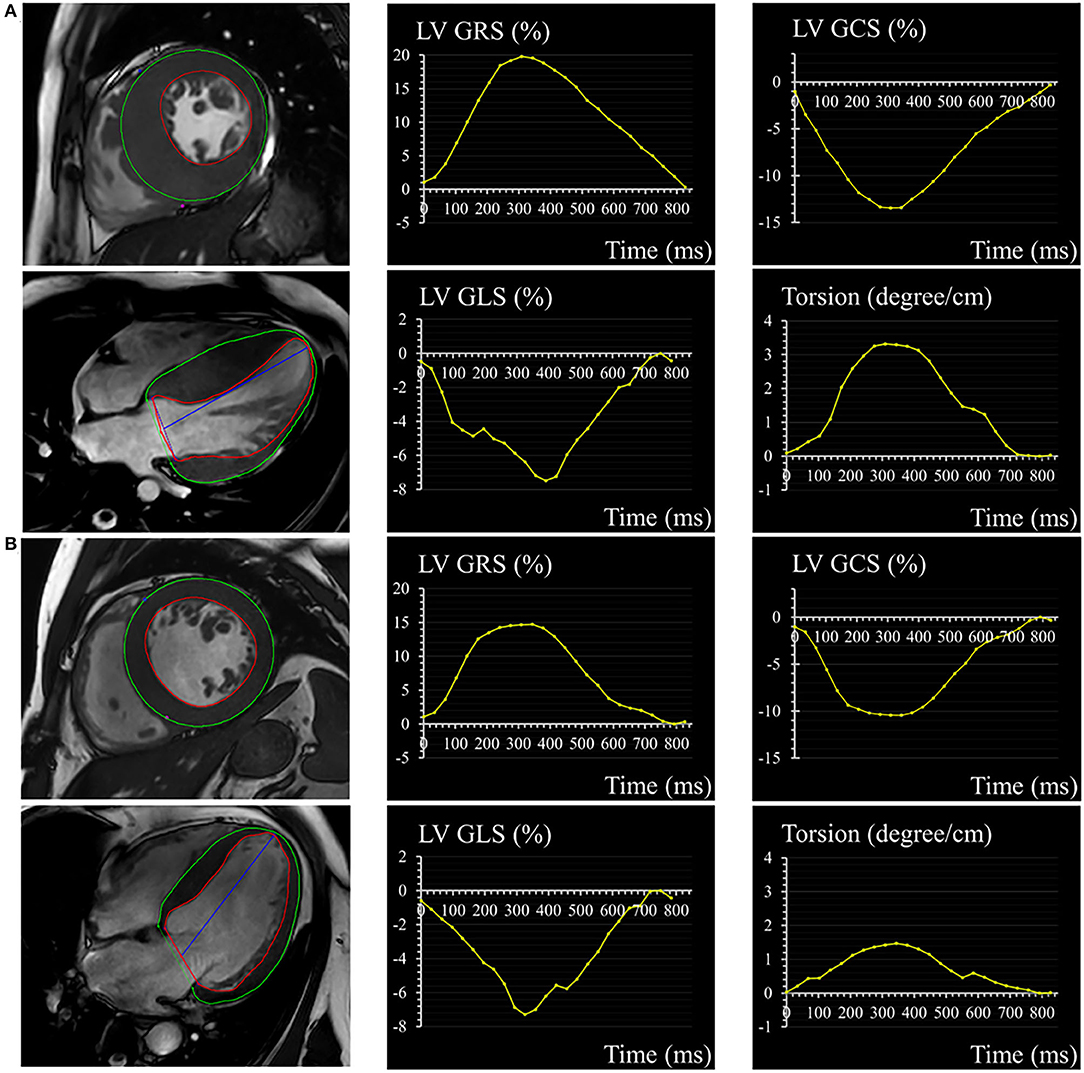
Figure 1. A 32-year-old male with HCM (A) and a 56-year-old male with HHD (B). Epicardial (green) and endocardial (red) contours, as well as the corresponding curves of LV GRS, GCS, GLS, and torsion in HCM and HHD. HCM, hypertrophic cardiomyopathy; HHD, hypertensive heart disease; LV, left ventricular; GRS, global radial strain; GCS, global circumferential strain; GLS, global longitudinal strain.
Late gadolinium enhancement quantification was obtained with mean ± 5 SDs algorithm and expressed as a volumetric proportion of the total LV myocardium. Segmental analysis on LGE was assessed according to the American Heart Association 17-segment model by setting the anterior and inferior right ventricular insertion points (16). To investigate the difference of LGE in different segments of myocardium in HCM and HHD, the segments 8 and 9 were defined as mid-interventricular septal (mIVS) in this study.
Categorical variables were assessed by the Fisher's exact test or the chi-squared test and expressed as numbers with percentage. Normally, distributed continuous variables were presented as mean ± SD and compared by the Student's t-test between the two groups or by ANOVA test with post-hoc Bonferroni analysis among the three groups. Nonnormally distributed continuous variables were shown as medians with interquartile range and verified by the Mann–Whitney U test or the Kruskal–Wallis H test. The Pearson's or Spearman's correlation coefficients were calculated to investigate the potential correlations between myocardial strain and functional CMR parameters. The multivariate logistic regression analysis was calculated to test the diagnostic ability of CMR parameters for distinguishing the two diseases. The area under the curves (AUCs), specificity, sensitivity, and Youden's index were analyzed by the receiver operating characteristic (ROC) curve. DeLong's test was used to compare the AUCs for the pairwise groups. All the data were calculated by SPSS version 26.0.0 (SPSS Incorporation, Chicago, Illinois, USA) or MedCalc (version 20, MedCalc Software, Ostend, Belgium). P < 0.05 was considered as statistically significant.
Results
Participant Characteristics
The study cohort consisted of 170 participants, including 57 patients with HCM (33.5%), 45 patients with HHD (26.5%), and 68 healthy volunteers (40%). Clinical characteristics and basic CMR parameters of the HCM, HHD, and healthy group are given in Table 1. In comparison to patients with HHD, patients with HCM were older (P = 0.014), less male (P = 0.009), had smaller body surface area (P < 0.001), and lower incidence of hypertension (P < 0.001). There was no statistical difference between the HCM and HHD group in terms of prevalence of diabetes mellitus, dyslipidemia, and smoker (all P > 0.05).
Cardiac Function by Cardiovascular Magnetic Resonance
Compared with patients with HHD, patients with HCM had a higher ejection fraction (64.43 ± 13.82 vs. 39.76 ± 18.59%, P < 0.001). Those with HCM also had a lower LV end-diastolic volume index (EDVi) (84.83 ± 35.16 vs. 121.08 ± 46.36 ml/m2, P < 0.001) and lower LV end-systolic volume index (ESVi) [26.3 (19.3, 32.8) vs. 81.6 (30.6, 107.7) ml/m2, P < 0.001]. As for maximum interventricular septum thickness (IVST max), there were higher values in patients with HCM when compared with patients with HHD (23.40 ± 4.48 vs. 15.86 ± 3.73 mm, P < 0.001). The LV end-diastolic diameter (EDD) of patients with HCM was 45.75 ± 7.39 mm, which was lower than that in patients with HHD (60.73 ± 11.59 mm, P < 0.001). There were no significant differences regarding CO, LVMi, and right ventricular EDD between the two groups of LVH.
Myocardial Strain and Late Gadolinium Enhancement in the Hypertrophic Cardiomyopathy and Hypertensive Heart Disease Groups
Global radial strain (GRS) and global circumferential strain (GCS) were significantly higher in the HCM group relative to the HHD group, as shown in Table 2 (GRS, 21.18 ± 7.52 vs. 14.56 ± 7.46%; GCS, −13.34 ± 3.52 vs. −10.11 ± 4.13%; both P < 0.001), whereas comparisons of GLS showed no obvious difference (−9.49 ± 3.06 vs. −9.34 ± 3.76%, P = 0.973). Similar trend could also be detected in corresponding strain rate.
As for segmental strain analysis, the three basal parameters [which included basal radial strain (BRS), basal circumferential strain (BCS), and basal longitudinal strain (BLS)] were higher in the HCM group than in the HHD group (BRS, 24.12 ± 8.21 vs. 15.25 ± 7.88%; BCS, −14.73 ± 3.61 vs. −10.41 ± 4.31%; BLS, −15.93 ± 4.51 vs. −13.55 ± 5.02%; all P < 0.05). Mid-radial strain (MRS) and mid-circumferential strain (MCS) were also higher in patients with HCM relative to patients with HHD (MRS, 19.9 ± 7.83 vs. 13.77 ± 8.27%; MCS, −12.89 ± 3.76 vs. −9.7 ± 4.68%; both P < 0.05), but mid-longitudinal strain (MLS) had no significant difference between the two groups. None of the three apical strain results showed obvious differences between the HCM and HHD cohort. Torsion in the HCM group was higher than that in the HHD group (1.79 ± 0.69 vs. 1.23 ± 0.65 deg/cm, P < 0.001).
Late gadolinium enhancement was assessed in 170 participants and shown in Table 2. Healthy subjects presented no LGE. Patients with HCM in comparison to patients with HHD had higher values of LGE (total enhanced volume percentage) (P < 0.05). On segmental analysis, the differences in LGE values were predominantly localized around the interventricular septum (segments 7, 8, and 9; all P = 0.001), which corresponds to the mid-segment of the anterior, anteroseptal, and inferoseptal of IVS. It was also pronounced for mIVS (P < 0.001) (Figure 2), but there were no significant differences in other segments.
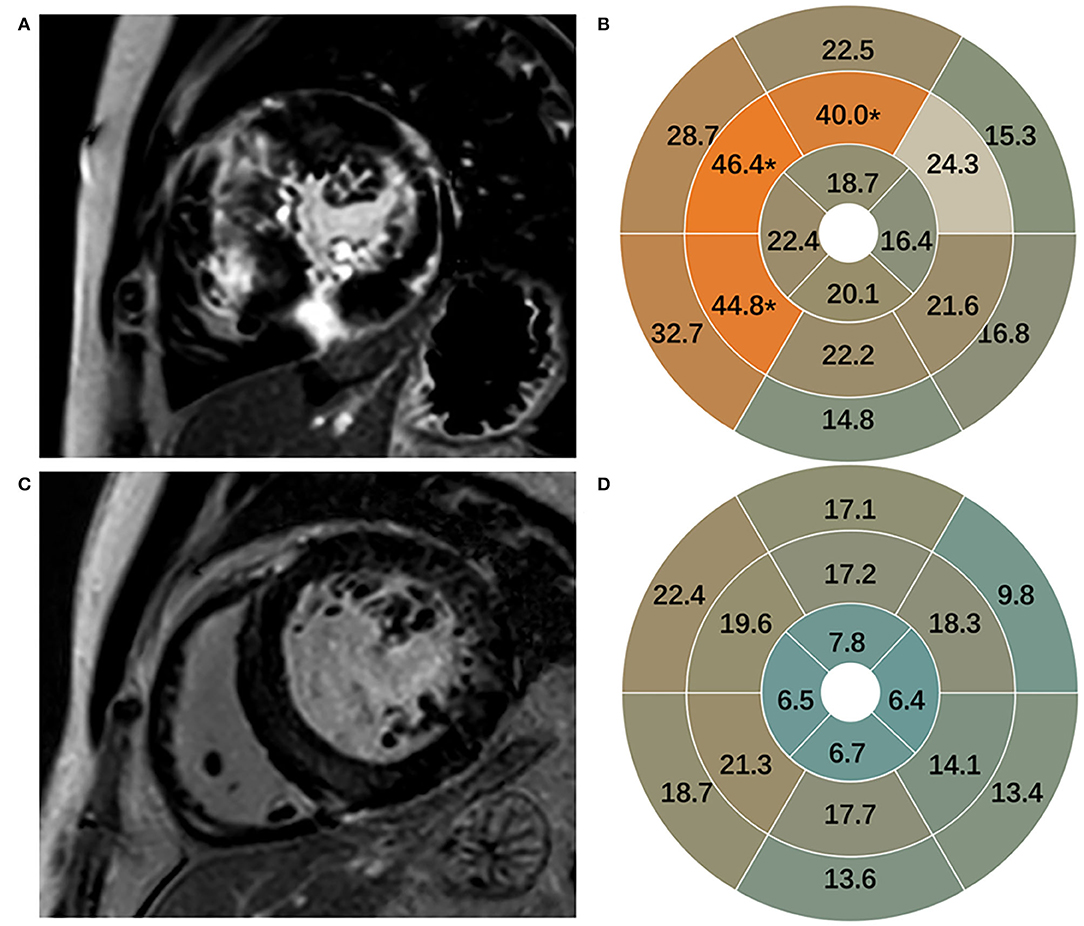
Figure 2. Representative images of LGE in HCM (A) and HHD (C). LGE in 16 segments in HCM (B) and HHD (D). LGE, late gadolinium enhancement; HCM, hypertrophic cardiomyopathy; HHD, hypertensive heart disease.
Correlations of Left Ventricular Strain With Functional and Structural Parameters
In patients with HCM, GRS, GCS, and GLS showed correlations with LVEF, IVST max, and LVMi (Table 3). Especially, GRS and GCS presented strong correlations with LVEF (GRS, R = 0.597, P < 0.001; GCS, R = −0.618, P < 0.001). In addition, torsion was mildly associated with LVEF (R = 0.402, P = 0.002) (Figure 3). In patients with HHD, GRS, GCS, GLS, and torsion were correlated with LVEF and LVMi (all P < 0.001). In the healthy group, GRS, GCS, and torsion were only associated with LVEF (all P < 0.001).
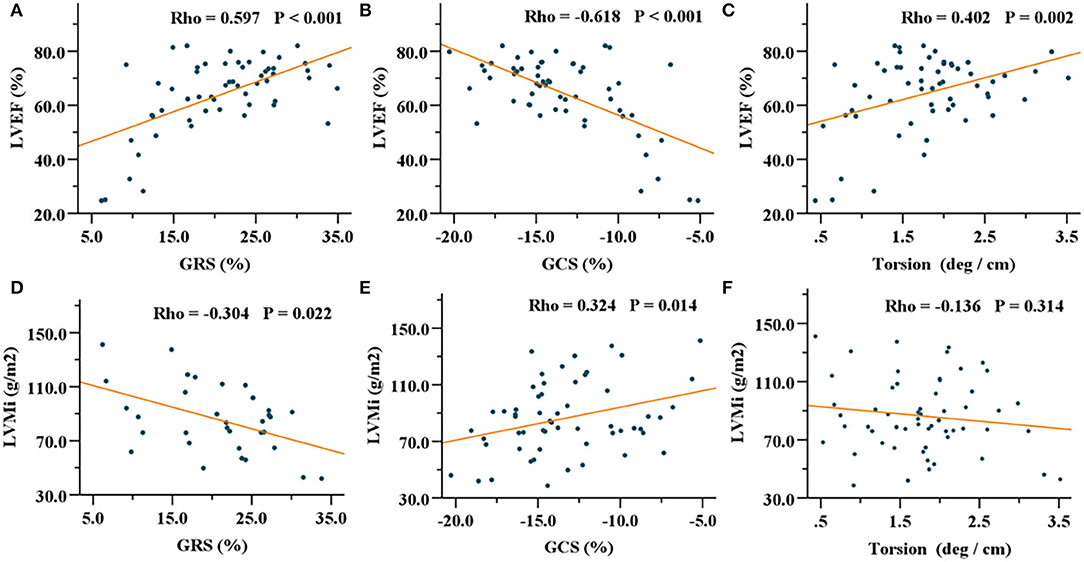
Figure 3. Correlations of GRS, GCS, and torsion with LVEF (A–C) and LVMi (D–F) in the HCM group. GRS, global radial strain; GCS, global circumferential strain; LVEF, left ventricular ejection fraction; LVMi, left ventricular mass index; HCM, hypertrophic cardiomyopathy.
Differentiation Between Hypertrophic Cardiomyopathy and Hypertensive Heart Disease
The results of the ROC analysis to discriminate between HCM and HHD are shown in Table 4. GRS (AUC: 0.734, sensitivity: 62.22%, specificity: 75.44%, P < 0.001), GCS (AUC: 0.718, sensitivity: 64.44%, specificity: 71.93%, P < 0.001), and corresponding strain rate could distinguish HCM from HHD. For segmental strain parameters, the AUCs of BRS, BCS, BLS, MRS, and MCS for discriminating between HCM and HHD were 0.781, 0.776, 0.644, 0.711, and 0.697, respectively (all P < 0.001). The torsion cutoff value of > 1.40 differentiated HCM from HHD with a sensitivity of 71.11% and a specificity of 75.44%. The AUC of LGE (mIVS) was 0.735, which was the highest in the global and other segmental LGE analyses (all P < 0.05). Overall, BRS showed the highest diagnostic performance and the value of the AUC was 0.781 (all P < 0.05). On the multivariate regression analysis, the combination model of LGE (mIVS) with GRS obtained the highest AUC value, which was 0.835 with 88.89% sensitivity and 70.18% specificity, respectively (Table 5). DeLong's test showed that the AUC of a combined model of GRS with LGE (mIVS) was higher than those of GRS, GCS, torsion, and LGE (mIVS) (all P < 0.05) (Figure 4).
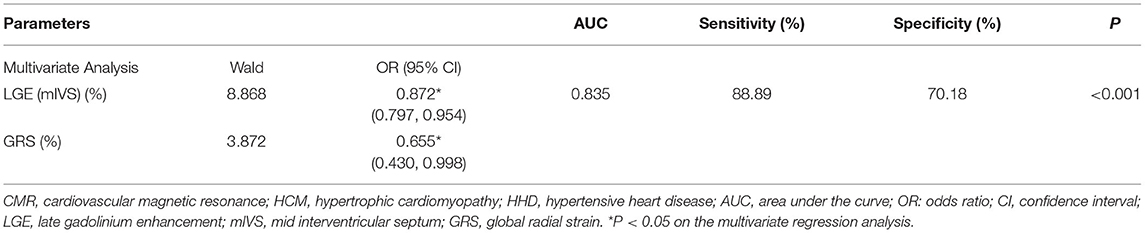
Table 5. The multivariate logistic regression analysis of CMR parameters for discrimination of patients with HCM and HHD.
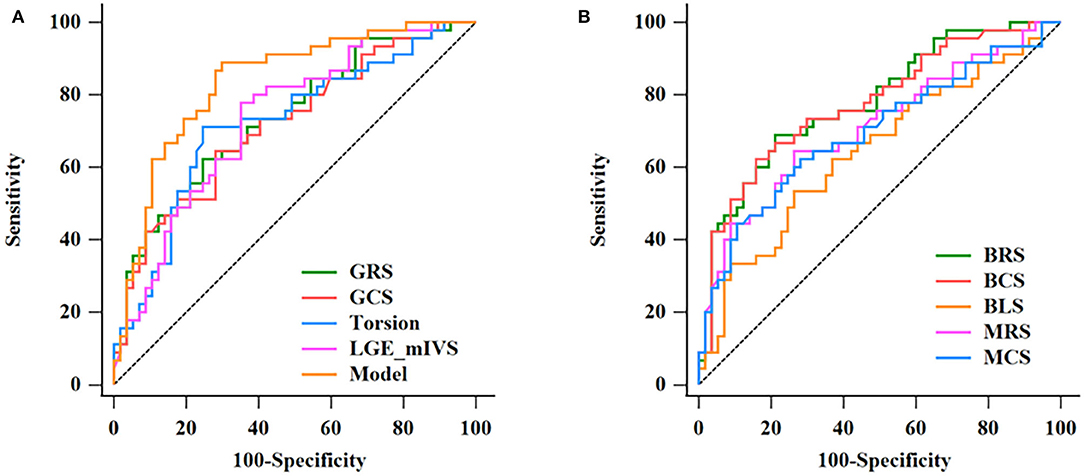
Figure 4. Discrimination of HCM and HHD by CMR parameters. The ROC curves in discrimination between HCM and HHD for single CMR parameters and the multivariate regression model are given in Table 5 (A). The AUC of combination model was the highest (0.835). The ROC curves of BRS, BCS, BLS, MRS, MCS in discrimination between HCM and HHD group (B). HCM, hypertrophic cardiomyopathy; HHD, hypertensive heart disease; ROC, receiver operating characteristic; CMR, cardiovascular magnetic resonance; AUC, area under the curve; GRS, global radial strain; GCS, global circumferential strain; LGE, late gadolinium enhancement; mIVS, mid interventricular septum; BRS, basal radial strain; BCS, basal circumferential strain; BLS, basal longitudinal strain; MRS, mid radial strain; MCS, mid circumferential strain.
Discussion
Several important outcomes were found in evaluating patients with HCM and HHD using FT parameters and LGE data. The main findings of this study were: (1) LV GRS, GCS, and torsion were significantly higher in patients with HCM compared with patients with HHD. The differences of radial and circumferential strain mainly existed in LV basal and midsegments; (2) There was no significant difference in GLS between the two groups, but it was distinguishable in the LV basal segment (BLS); (3) The difference of %LGE can be used to distinguish between the two diseases, especially in the mIVS; and (4) In the multivariate regression model, the combination of GRS and LGE (mIVS) achieved the highest diagnostic value in differentiation between HCM and HHD diseases.
Cardiovascular magnetic resonance feature tracking has been used in the assessment of HCM and HHD, respectively, due to its ability to quantify cardiac movement in different directions during the cardiac cycle (17, 18). This study found that radial strain in the HHD group was decreased when compared with the HCM group and alterations of the two diseases mainly concentrated in the basal and midsegments. There were no obvious differences in the apical segment. Moreover, segmental analysis of radial strain offered excellent precision for the differentiation between the two clinical cohorts. In another study, GRS was further validated to show significant discriminatory power for identifying LV outflow tract obstruction in patients with HCM (19). Based on the study from Niu et al., GRS improved obviously after treatment, which suggested that GRS might be an index for evaluating treatment (20). We also provided evidence that the variation and distribution of circumferential strain were similar to those of the radial orientation. The alteration in circumferential direction in this study was consistent with previous investigation (21). This may indicate that motion abilities in radial and circumferential directions of the two diseases were different during the cardiac cycle. Studies have shown that left ventricular myocardium is stratified; fibers in the mid-wall were arranged circumferentially, while such fibers near the apex were sparse (22). This may be the main reason for the significant differences of radial and circumferential strains in basal and midregions. GCS was further expected to be a potential predictor in identifying adverse prognosis, such as malignant arrhythmia (23). LV global systolic functional parameter was correlated the best to circumferential shortening in our data, followed with radial thickening. Extent of hypertrophy (LVMi) revealed an independent role in impacting GCS and GRS in both the HCM and HHD subjects. Another hypertrophy indicator (IVST max) was also proven to be associated with circumferential and radial strains merely in HCM subjects. Previous studies have also shown that myocardial strain values are significantly impaired in hypertensive patients, which are consistent with this study and may offer a preventative strategy before LVEF abnormalities (18).
Longitudinal strain refers to systolic shortening of LV wall relative to its length and it was proven to be a surrogate of subendocardial fibrotic changes in the late stage of HHD disease (24). Based on a previous study, more impaired GLS was measured in patients with HHD compared with patients with HCM (8, 25). But, we merely found a significant difference of longitudinal strain between the two groups in the LV basal segment; no obvious differences were detected in the mid and apical segments. This may be explained by us considering the basal, mid, and apical segments together, which may have diluted the regional variation. Another study also explained the discrepancy above. They reported that longitudinal strain attenuation was unable to provide significant distinguishable accuracy for patients with HCM and HHD with maximal LVWT ≥ 15 mm (7), which was the same condition in this study. Thus, impaired myocardial deformation in the longitudinal direction indicated impaired muscle fibers within the subendocardial region (24).
Left ventricular torsion provides the difference in rotation angle between the base and apex (13). Investigations examining the diagnostic potential of LV torsion were scarce in differentiating between HCM and HHD previously (26). According to this study, LV torsion was higher in the HCM group than that in the HHD group and, therefore, the assessment of LV torsion was shown to provide discriminative ability between patients with HCM and HHD. Increased LVEF was also found in patients with HCM, which was consistent with a previous study (27). The deformation parameters (GLS, GRS, GCS, and torsion) showed underlying effects on contractile function for both the cardiac hypertrophied entities. LVEF alone has previously been insufficient to represent normal deformation ability (28), as most forms of LVH show preserved LVEF until late stages of the disease progression (29). Decreased strain and LV torsion may be able to characterize cardiac pump function in the early phase of disease progression.
Cardiovascular magnetic resonance has the advantage of tissue characterization, which provides valuable diagnostic information. LGE identifies areas with myocyte necrosis or myocardial fibrosis in clinical practice, which has been shown to be closely associated with segmental dysfunction (30–32). Previous publications have demonstrated more prevalent and extensive LGE in patients with HCM than patients with HHD (33); likewise, our data resonated similarly with this. Indeed, the degree of interstitial fibrosis and scar formation in HCM was higher (5). Further comparison regarding LGE quantification (% LGE) in mIVS facilitated its significant differential value, showing that mid-wall fibrosis was a significant discriminator (7). The presence of LGE has also been included in risk prediction modeling for sudden cardiac death in patients with HCM (31). Furthermore, the combination of myocardial strain and LGE provided the highest accuracy rate for differentiation of HHD and HCM. Thus, CMR-derived LGE and myocardial strain measurements offer novel diagnostic significance in differentiating HCM and HHD. As treatment and prognosis of the two hypertrophied cardiac diseases are totally different, CMR has the potential to improve the diagnostic accuracy and facilitate treatment guidance in HCM and HHD.
Limitations
There were several limitations in this study. First, as only a small cohort of patients was included in this study, larger studies are required to validate our findings. Second, subgroup evaluation may be a promising direction to analyze the differences among diverse phenotypes of HCM and HHD, such as the classification of patients with HCM according to LV outflow tract gradients. Third, we only analyzed systolic strain parameters, which already offered diagnostic value. Diastolic dysfunction was not considered in this study and it might offer new insights to characterize the two diseases. Fourth, a prospective study with the inclusion of genetic findings may help us to better understand the interplay between the genotype and phenotype in these cohorts. Last, the predictive value of myocardial strain for adverse cardiovascular events was not studied due to lack of follow-up.
Conclusion
Cardiovascular magnetic resonance feature tracking-derived myocardial strain parameters could provide valuable methods for differentiating HCM from HHD. LV strain and torsion were closely related to LVEF, IVST max, and LVMi. LGE (mIVS) has shown the ability of discriminating between HCM and HHD. The combination of LV GRS and LGE (mIVS) achieved the highest diagnostic value. CMR strain analysis may contribute to improve the diagnostic accuracy and facilitate treatment guidance in hypertrophied cardiac diseases.
Data Availability Statement
The raw data supporting the conclusions of this article will be made available by the authors, without undue reservation.
Ethics Statement
The studies involving human participants were reviewed and approved by KY2021-132. The patients/participants provided their written informed consent to participate in this study.
Author Contributions
SL and GW designed the study and wrote the manuscript. XW, YeL, and YZ postprocessed the images. XG, JC, and BX collected the data. ZW and YuL analyzed the data. JT and BY supervised the study. All the authors have read and approved the final version of the manuscript.
Funding
This study was supported by the National Natural Science Foundation of China (Grant No. 82100529/XG) and the Fund of Key Laboratory of Myocardial Ischemia, Ministry of Education (KF201910/YL).
Conflict of Interest
The authors declare that the research was conducted in the absence of any commercial or financial relationships that could be construed as a potential conflict of interest.
Publisher's Note
All claims expressed in this article are solely those of the authors and do not necessarily represent those of their affiliated organizations, or those of the publisher, the editors and the reviewers. Any product that may be evaluated in this article, or claim that may be made by its manufacturer, is not guaranteed or endorsed by the publisher.
Acknowledgments
The authors appreciate Jianxiu Lian and Ke Jiang for the contributions of study concept and Haixia Li for the assistance of statistics analysis, as well as Xiaofang Xu for the support in acquiring CMR images.
References
1. Maron BJ. Clinical course and management of hypertrophic cardiomyopathy. N Engl J Med. (2018) 379:1977. doi: 10.1056/NEJMra1710575
2. Elliott P, Anastasakis A, Borger M, Borggrefe M, Cecchi F, Charron P, et al. 2014 ESC Guidelines on diagnosis and management of hypertrophic cardiomyopathy: the task force for the diagnosis and management of hypertrophic cardiomyopathy of the European Society of Cardiology (ESC). Eur Heart J. (2014) 35:2733–79. doi: 10.1093/eurheartj/ehu284
3. Chobanian AV, Bakris GL, Black HR, Cushman WC, Green LA, Izzo JL Jr, et al. Seventh report of the joint national committee on prevention, detection, evaluation, and treatment of high blood pressure. Hypertension. (2003) 42:1206–52. doi: 10.1161/01.HYP.0000107251.49515.c2
4. Rodrigues J, Amadu A, Dastidar A, Szantho G, Lyen S, Godsave C, et al. Comprehensive characterisation of hypertensive heart disease left ventricular phenotypes. Heart. (2016) 102:1671–9. doi: 10.1136/heartjnl-2016-309576
5. Giusca S, Steen H, Montenbruck M, Patel AR, Pieske B, Erley J, et al. Multi-parametric assessment of left ventricular hypertrophy using late gadolinium enhancement, T1 mapping and strain-encoded cardiovascular magnetic resonance. J Cardiovasc Magn Reson. (2021) 23:92. doi: 10.1186/s12968-021-00775-8
6. Xu J, Zhuang B, Sirajuddin A, Li S, Huang J, Yin G, et al. MRI T1 mapping in hypertrophic cardiomyopathy: evaluation in patients without late gadolinium enhancement and hemodynamic obstruction. Radiology. (2020) 294:275–86. doi: 10.1148/radiol.2019190651
7. Rodrigues J, Rohan S, Ghosh Dastidar A, Harries I, Lawton C, Ratcliffe L, et al. Hypertensive heart disease versus hypertrophic cardiomyopathy: multi-parametric cardiovascular magnetic resonance discriminators when end-diastolic wall thickness ≥ 15 mm. Eur Radiol. (2017) 27:1125–35. doi: 10.1007/s00330-016-4468-2
8. Neisius U, Myerson L, Fahmy AS, Nakamori S, El-Rewaidy H, Joshi G, et al. Cardiovascular magnetic resonance feature tracking strain analysis for discrimination between hypertensive heart disease and hypertrophic cardiomyopathy. PLoS ONE. (2019) 14:e0221061. doi: 10.1371/journal.pone.0221061
9. Schuster A, Paul M, Bettencourt N, Morton G, Chiribiri A, Ishida M, et al. Cardiovascular magnetic resonance myocardial feature tracking for quantitative viability assessment in ischemic cardiomyopathy. Int J Cardiol. (2013) 166:413–20. doi: 10.1016/j.ijcard.2011.10.137
10. Nazir S, Shetye A, Khan J, Singh A, Arnold J, Squire I, et al. Inter-study repeatability of circumferential strain and diastolic strain rate by CMR tagging, feature tracking and tissue tracking in ST-segment elevation myocardial infarction. Int J Cardiovasc Imaging. (2020) 36:1133–46. doi: 10.1007/s10554-020-01806-8
11. Overhoff D, Ansari U, Hohneck A, Tülümen E, Rudic B, Kuschyk J, et al. Prediction of cardiac events with non-contrast magnetic resonance feature tracking in patients with ischaemic cardiomyopathy. ESC Heart Failure. (2021) 9:574–84. doi: 10.1002/ehf2.13712
12. Vigneault DM, Yang E, Jensen PJ, Tee MW, Farhad H, Chu L, et al. Left ventricular strain is abnormal in preclinical and overt hypertrophic cardiomyopathy: cardiac MR feature tracking. Radiology. (2019) 290:640–8. doi: 10.1148/radiol.2018180339
13. Ochs A, Riffel J, Ochs MM, Arenja N, Fritz T, Galuschky C, et al. Myocardial mechanics in dilated cardiomyopathy: prognostic value of left ventricular torsion and strain. J Cardiovasc Magn Reson. (2021) 23:136. doi: 10.1186/s12968-021-00829-x
14. Kanzaki H, Nakatani S, Yamada N, Urayama S, Miyatake K, Kitakaze M. Impaired systolic torsion in dilated cardiomyopathy: reversal of apical rotation at mid-systole characterized with magnetic resonance tagging method. Basic Res Cardiol. (2006) 101:465–70. doi: 10.1007/s00395-006-0603-6
15. Gan Y, Chen Q, Zhang S, Ju S, Li Z. MRI-based strain and strain rate analysis of left ventricle: a modified hierarchical transformation model. Biomed Eng Online. (2015) 14 Suppl 1(Suppl 1):S9. doi: 10.1186/1475-925X-14-S1-S9
16. Fattori R, Biagini E, Lorenzini M, Buttazzi K, Lovato L, Rapezzi C. Significance of magnetic resonance imaging in apical hypertrophic cardiomyopathy. Am J Cardiol. (2010) 105:1592–6. doi: 10.1016/j.amjcard.2010.01.020
17. She J, Guo J, Yu Y, Zhao S, Chen Y, Ge M, et al. Left ventricular outflow tract obstruction in hypertrophic cardiomyopathy: the utility of myocardial strain based on cardiac MR tissue tracking. J Magn Reson Imaging. (2021) 53:51–60. doi: 10.1002/jmri.27307
18. Liu H, Wang J, Pan Y, Ge Y, Guo Z, Zhao S. Early and quantitative assessment of myocardial deformation in essential hypertension patients by using cardiovascular magnetic resonance feature tracking. Sci Rep. (2020) 10:3582. doi: 10.1038/s41598-020-60537-x
19. Huang L, Que L, Xi Y, Zhuang J, Yuan H, Liu H, et al. Myocardial mechanics parameters that predict left ventricular outflow tract obstruction in patients with hypertrophic cardiomyopathy: a cardiovascular magnetic resonance feature tracking analysis. J Comput Assist Tomogr. (2021) 45:65–72. doi: 10.1097/RCT.0000000000000977
20. Niu J, Zeng M, Wang Y, Liu J, Li H, Wang S, et al. Sensitive marker for evaluation of hypertensive heart disease: extracellular volume and myocardial strain. BMC Cardiovasc Disord. (2020) 20:292. doi: 10.1186/s12872-020-01553-7
21. Weise Valdés E, Barth P, Piran M, Laser K, Burchert W, Körperich H. Left-ventricular reference myocardial strain assessed by cardiovascular magnetic resonance feature tracking and fSENC-impact of temporal resolution and cardiac muscle mass. Front Cardiovasc Med. (2021) 8:764496. doi: 10.3389/fcvm.2021.764496
22. Greenbaum R, Ho S, Gibson D, Becker A, Anderson R. Left ventricular fibre architecture in man. Br Heart J. (1981) 45:248–63. doi: 10.1136/hrt.45.3.248
23. Pu C, Fei J, Lv S, Wu Y, He C, Guo D, et al. Global circumferential strain by cardiac magnetic resonance tissue tracking associated with ventricular arrhythmias in hypertrophic cardiomyopathy patients. Front Cardiov Med. (2021) 8:670361. doi: 10.3389/fcvm.2021.670361
24. Ishizu T, Seo Y, Kameda Y, Kawamura R, Kimura T, Shimojo N, et al. Left ventricular strain and transmural distribution of structural remodeling in hypertensive heart disease. Hypertension. (2014) 63:500–6. doi: 10.1161/HYPERTENSIONAHA.113.02149
25. Shi R, Wu R, An D, Chen B, Wu C, Du L, et al. Texture analysis applied in T1 maps and extracellular volume obtained using cardiac MRI in the diagnosis of hypertrophic cardiomyopathy and hypertensive heart disease compared with normal controls. Clin Radiol. (2021) 76:236.e9–e19. doi: 10.1016/j.crad.2020.11.001
26. Esch B, Warburton D. Left ventricular torsion and recoil: implications for exercise performance and cardiovascular disease. J Appl Physiol. (2009) 106:362–9. doi: 10.1152/japplphysiol.00144.2008
27. Dorobantu D, Wadey C, Amir N, Stuart A, Williams C, Pieles G. The role of speckle tracking echocardiography in the evaluation of common inherited cardiomyopathies in children and adolescents: a systematic review. Diagnostics (Basel). (2021) 11:635. doi: 10.3390/diagnostics11040635
28. Gersh B, Maron B, Bonow R, Dearani J, Fifer M, Link M, et al. 2011 ACCF/AHA guideline for the diagnosis and treatment of hypertrophic cardiomyopathy: a report of the American College of Cardiology Foundation/American Heart Association task force on practice guidelines. Developed in collaboration with the American Association for Thoracic Surgery, American Society of Echocardiography, American Society of Nuclear Cardiology, Heart Failure Society of America, Heart Rhythm Society, Society for Cardiovascular Angiography and Interventions, and Society of Thoracic Surgeons. J Am Coll Cardiol. (2011) 58:e212–60. doi: 10.1016/j.jacc.2011.06.011
29. Cikes M, Sutherland G, Anderson L, Bijnens B. The role of echocardiographic deformation imaging in hypertrophic myopathies. Nat Rev Cardiol. (2010) 7:384–96. doi: 10.1038/nrcardio.2010.56
30. Vöhringer M, Mahrholdt H, Yilmaz A, Sechtem U. Significance of late gadolinium enhancement in cardiovascular magnetic resonance imaging (CMR). Herz. (2007) 32:129–37. doi: 10.1007/s00059-007-2972-5
31. Pascoe S. Clinical course and management of hypertrophic cardiomyopathy. N Engl J Med. (2018) 379:1976–7. doi: 10.1056/NEJMc1812159
32. Urbano-Moral J, Rowin E, Maron M, Crean A, Pandian N. Investigation of global and regional myocardial mechanics with 3-dimensional speckle tracking echocardiography and relations to hypertrophy and fibrosis in hypertrophic cardiomyopathy. Circ Cardiovasc Imaging. (2014) 7:11–9. doi: 10.1161/CIRCIMAGING.113.000842
33. Rudolph A, Abdel-Aty H, Bohl S, Boyé P, Zagrosek A, Dietz R, et al. Noninvasive detection of fibrosis applying contrast-enhanced cardiac magnetic resonance in different forms of left ventricular hypertrophy relation to remodeling. J Am Coll Cardiol. (2009) 53:284–91. doi: 10.1016/j.jacc.2008.08.064
Keywords: hypertrophic cardiomyopathy, hypertensive heart disease, cardiovascular resonance magnetic, feature tracking, late gadolinium enhancement
Citation: Liu S, Li Y, Zhao Y, Wang X, Wu Z, Gu X, Xu B, Li Y, Tian J, Cui J, Wang G and Yu B (2022) The Combination of Feature Tracking and Late Gadolinium Enhancement for Identification Between Hypertrophic Cardiomyopathy and Hypertensive Heart Disease. Front. Cardiovasc. Med. 9:865615. doi: 10.3389/fcvm.2022.865615
Received: 30 January 2022; Accepted: 14 April 2022;
Published: 11 May 2022.
Edited by:
Christos Bourantas, University College London, United KingdomReviewed by:
Eleanor Wicks, Oxford University Hospitals NHS Trust, United KingdomLaura Stefani, University of Florence, Italy
Copyright © 2022 Liu, Li, Zhao, Wang, Wu, Gu, Xu, Li, Tian, Cui, Wang and Yu. This is an open-access article distributed under the terms of the Creative Commons Attribution License (CC BY). The use, distribution or reproduction in other forums is permitted, provided the original author(s) and the copyright owner(s) are credited and that the original publication in this journal is cited, in accordance with accepted academic practice. No use, distribution or reproduction is permitted which does not comply with these terms.
*Correspondence: Guokun Wang, wangguokun1986@163.com; Bo Yu, dryu_hmu@163.com
†These authors have contributed equally to this work and share first authorship