- 1Beijing Key Laboratory for Precision Medicine of Chronic Heart Failure, Key Laboratory of Ministry of Industry and Information Technology of Biomedical Engineering and Translational Medicine, Translational Medicine Research Center, Medical Artificial Intelligence Research Center, Chinese PLA General Hospital, Beijing, China
- 2BioMind Technology, Zhongguancun Medical Engineering Center, Beijing, China
- 3Department of Ultrasound Diagnosis, The Seventh Medical Center of Chinese PLA General Hospital, Beijing, China
- 4Cardiovascular Research Foundation, New York, NY, United States
Objective: Exposure to high altitudes represents physiological stress that leads to significant changes in cardiovascular properties. However, long-term cardiovascular adaptions to high altitude migration of lowlanders have not been described. Accordingly, we measured changes in cardiovascular properties following prolonged hypoxic exposure in acclimatized Han migrants and Tibetans.
Methods: Echocardiographic features of recently adapted Han migrant (3–12 months, n = 64) and highly adapted Han migrant (5–10 years, n = 71) residence in Tibet (4,300 m) using speckle tracking echocardiography were compared to those of age-matched native Tibetans (n = 75) and Han lowlanders living at 1,400 m (n = 60).
Results: Short-term acclimatized migrants showed increased estimated pulmonary artery systolic pressure (PASP) (32.6 ± 5.1 mmHg vs. 21.1 ± 4.2 mmHg, p < 0.05), enlarged right ventricles (RVs), and decreased fractional area change (FAC) with decreased RV longitudinal strain (−20 ± 2.8% vs. −25.5 ± 3.9%, p < 0.05). While left ventricular ejection fraction (LVEF) was preserved, LV diameter (41.7 ± 3.1 mm vs. 49.7 ± 4.8 mm, p < 0.05) and LV longitudinal strain (−18.8 ± 3.2% vs. −22.9 ± 3.3%, p < 0.05) decreased. Compared with recent migrants, longer-term migrants had recovered RV structure and functions with slightly improved RV and LV longitudinal strain, though still lower than lowlander controls; LV size remained small with increased mass index (68.3 ± 12.7 vs. 59.3 ± 9.6, p < 0.05). In contrast, native Tibetans had slightly increased PASP (26.1 ± 3.4 mmHg vs. 21.1 ± 4.2 mmHg, p < 0.05) with minimally altered cardiac deformation compared to lowlanders.
Conclusion: Right ventricular systolic function is impaired in recent (<1 year) migrants to high altitudes but improved during the long-term dwelling. LV remodeling persists in long-term migrants (>5 years) but without impairment of LV systolic or diastolic function. In contrast, cardiac size, structure, and function of native Tibetans are more similar to those of lowland dwelling Hans.
Introduction
High altitude exposure is associated with pulmonary hypertension and pulmonary vasoconstriction resulting in significant changes in cardiovascular properties (1). There are many factors that affect cardiac adaptation to high altitude dwelling, including altitude level, exposure time, and racial differences. Cardiac responses to acute hypoxic exposure in people normally living at low altitudes (lowlanders) have been studied extensively (2, 3). Conventional echocardiographic studies show that in healthy subjects exposed to acute hypoxic conditions of high altitude, the systolic function of both ventricles is preserved, but there is impaired diastolic function (4). More recently, speckle tracking echocardiography (STE) has been introduced, which has better reproducibility and sensitivity than conventional echocardiography for quantifying cardiac functions through analysis of deformation mechanics (5). The use of STE is more sensitive for exploring subclinical changes in cardiac function to high altitude dwellings. Maufrais et al. (6) noted that after several days (<6 days) at high altitude, there are no significant changes in left ventricular (LV) or right ventricular (RV) longitudinal strain, but there is increased LV twist. In contrast, Stembridge et al. (7) reported that RV longitudinal strain is decreased, which is a consequence of high pulmonary artery systolic pressure (PASP) following longer duration trekking (>10 days).
However, there is limited information concerning how healthy lowlanders (i.e., individuals who themselves and their ancestors are born and living below 2,500 m) adapt to longer-term (months-to-years) exposure to high altitudes (greater than 2,500 m); in particular, there are no data concerning changes in cardiac mechanics under such conditions. An example is when healthy Han individuals (the most common race in China) born and living at low altitudes (<2,500 m) migrate to the Tibet plateau (>4,000 m). It is not uncommon for young adults to live and work at high altitudes over periods of months to years, which provides the opportunity to study long-term cardiovascular adaptations to chronic hypoxia in these extreme conditions.
Accordingly, the purpose of this study was to provide a comprehensive characterization of changes in cardiac structure, function, and longitudinal strain during prolonged (month-to-years) exposure to high altitude (4,300 m) in Han migrants and compare them to characteristics of native Tibetans. We hypothesized that (1) cardiac structure (indexed by LV and RV size and wall thicknesses) and function [indexed by LV ejection fraction (EF), RV fractional area change (FAC), and global longitudinal strain (GLS)] of long-term (>5 years) Han migrants are more highly adapted to high altitude than recent acclimatized Hans (<1 year) and (2) despite long-term dwelling (>5 years), these same indexes of cardiac structure and function of Han migrants are not as highly adapted to high altitude as native Tibetans.
Methods
Study Population
Han lowlanders were recruited in Yecheng, Xinjiang (1,400 m); Han migrants and Tibetans were recruited in the Ali district of Tibet (4,300 m). A total of 328 volunteers completed a questionnaire and were given free physical and echocardiographic examinations. The questionnaire included age, ethnicity, duration of residence, family medical history, questions related to physical and psychological discomfort, and medications. As the aim of the study was to investigate the normal process of cardiac adaption to chronic hypoxia of young and healthy migrants from lowland, the criteria for being included in this study were as follows: age between 18 and 32 years, male, and a normal physical examination. Exclusion criteria included any history of smoking, self-reported history of any cardiorespiratory disease, obesity, or intake of any medication within a month before participation in this study. After excluding 36 subjects due to insufficient image quality, 292 participants were included in this study, including 217 Hans and 75 Tibetans. Participants enrolled were grouped according to ethnicity, altitude, and adaptation periods into one of 4 groups: (1) Han lowlanders (n = 82); (2) short-term acclimatized Hans (n = 64), who were defined as Han migrants with residence time in Tibet of more than 3 months but less than 1 year; (3) long-term acclimatized Hans (n = 71), who were defined as Han migrants with residence time in Tibet for more than 5 years; and (4) native Tibetans (n = 75). The protocol was approved by the Chinese PLA General Hospital Ethics Committee, and each participant provided written informed consent.
Basic and Clinical Data Acquisition
Data were collected by two physicians and an experienced sonographer who traveled between Yecheng and Ali. Measurement included height, weight, blood pressure (BP), heart rate (HR), arterial O2 saturation (SpO2), and blood samples. BP and HR were measured (HEM-7211; OMRON, Japan) in the supine position after 10 min of rest. SpO2 was measured using finger-pulse oximetry (YX303, Yuwell, Jiangsu, China) after finger warming and signal stabilization. Hemoglobin concentration (HB) and hematocrit (HCT) measurements (BC-3000Plus, Mindray, Shenzhen, China) were performed by peripheral venous sampling. From these data, body surface area (BSA) and body mass index (BMI) were calculated.
Echocardiographic Assessments
Echocardiographic image acquisition included 2D and Doppler echocardiography and was saved in the DICOM format. The procedure was performed by a certified sonographer using a portable ultrasound machine (M9, probe SP5-1; Mindray, Shenzhen, China) in the left lateral decubitus position according to society guideline recommendations (8, 9). Measurements were performed offline by an experienced physician blinded to the subjects' conditions and ethnicity. LV end-diastolic volume (EDV) and end-systolic volume (ESV) were measured from planar tracings of the endocardial border in the apical 4- and 2-chamber (4C and 2C, respectively) views using the Simpson's biplane method. LV stroke volume (SV) and EF were calculated as EDV-ESV and (EDV-ESV)/EDV, respectively. LV mass (in g) was calculated according to the cube formula using end-diastolic values of septal and posterior wall thickness (LVST and LVPWT) and the LV diastolic dimension (LVDD) from M mode tracings:
Right ventricular end-diastolic and end-systolic areas (EDA and ESA) were measured from planar tracings of the endocardial border in the apical 4C view, and RV FAC was calculated by (EDA-ESA)/EDA. Right and left atrial EDVs and ESVs were determined from planar tracings of the endocardial border in the apical 4C view by using the Simpson's method; atrial emptying fractions were calculated by (EDV-ESV)/EDV. Finally, volumes and dimensions were indexed to BSA to balance differences in body sizes. Early (E) and late (A) diastolic filling velocities were assessed using pulsed-wave Doppler recordings, and free wall velocities (s′, e′, and a′) were measured using tissue Doppler imaging at both mitral and tricuspid annular levels. Isovolumic relaxation time (IVRT) and deceleration time (DT) were measured at the level of the mitral annulus. Tricuspid annular plane systolic excursion (TAPSE) was measured from M-mode tracings. Systolic tricuspid regurgitation (TR) flow velocity was used to estimate pressure gradient (PTR, in mmHg) from the modified Bernoulli equation (10): PTR = 4·Vmax2. Right atrial pressure (RAP) was estimated by the diameter of the inferior vena cava and its variation during inspiration as detailed previously (11). PASP was calculated as PASP = PTR + RAP. Mean PAP (PAMP) was calculated from PASP as PAMP = 0.61 PASP + 2 mmHg (12). The TAPSE/PASP ratio, a surrogate of the gold standard ratio of end-systolic to arterial elastance (Ees/ Ea), was calculated to evaluate RV-arterial coupling (13).
The longitudinal strain of each chamber was analyzed offline using two-dimensional STE software (Tomtec, REF-Version 4.6 software, Munich, Germany) by an experienced physician. LV GLS was assessed from apical 2-, 3- and 4-chamber views according to recommendations (14). RV GLS, RA, and LA longitudinal strains were assessed from apical 4C views (15).
Statistical Analysis
Statistical analyses were performed using SPSS (version 17.0). Data were expressed as mean ± SD, and a test for normality was performed using the Kolmogorov–Smirnov test. The effects of hypoxic exposure on conventional echocardiographic parameters and cardiac mechanics were assessed by analysis of variance among the Han population and Tibetans. When a significant main effect was found, we used modified t-tests (i.e., t-tests with the residual variance of the analysis of variance) as a post hoc test to compare recent Han migrants with Han lowlanders and with long-term acclimatized Han migrants and to compare Native Tibetans with long-term Hans. p-values were adjusted for multiple comparisons using Bonferroni corrections. Statistical significance was declared when p < 0.05.
Results
Clinical Characteristics of the Subjects
Characteristics of the study population are summarized in Table 1. We found that Han migrants with 3–12 months of exposure to hypoxia had a moderately increased HR and BP. Elevations of HR and BP persisted in Hans during long-term (5–10 years) exposure. Also, hemoglobin concentrations and hematocrits continued to increase with persistently lower SpO2. Native Tibetans exhibited unique characteristics compared with to Han population, including relatively smaller BSAs and BMIs. They also had relatively lower BP, Hb, and hematocrits compared to highly acclimatized Hans.
Right Heart Characteristics Under Chronic Hypoxia
Figure 1 summarizes the main findings for right heart echocardiographic measurements; other echocardiographic parameters are provided in Table 2. Short-term acclimatized Hans exhibited enlarged RAs with preserved emptying fraction. RV FAC decreased significantly as a result of enlarged RV areas, especially the end-systolic area. Other parameters associated with RV systolic function such as se′ and TAPSE also decreased. Estimated PASP increased and the TAPSE/PASP ratio decreased significantly, indicating impaired RV-arterial coupling. As for cardiac mechanics, there were significant decreases in RV GLS with slightly decreased RA GLS.
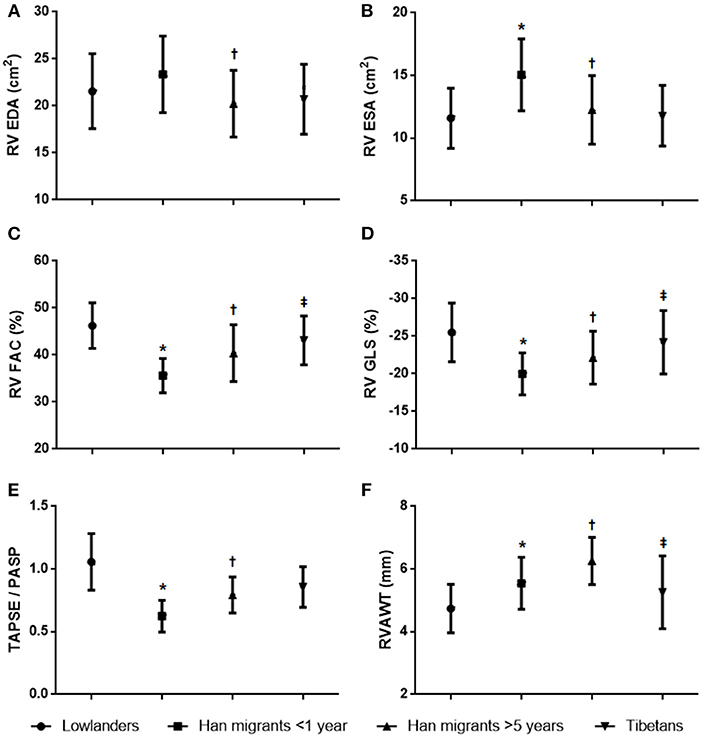
Figure 1. Between-group comparisons of right ventricular characteristics. (A) Right ventricular end-diastolic area. (B) Right ventricular end-systolic area. (C) Right ventricular fractional area change. (D) Right ventricular global longitudinal strain. (E) Tricuspid annular plane systolic excursion/pulmonary artery systolic pressure. (F) Right ventricular anterior wall sickness. *p < 0.05 Han migrants <1 year vs. lowlanders; †p < 0.05 Han migrants >5 years vs. Han migrants <1 year; ‡p < 0.05 Tibetans vs. Han migrants >5 years.
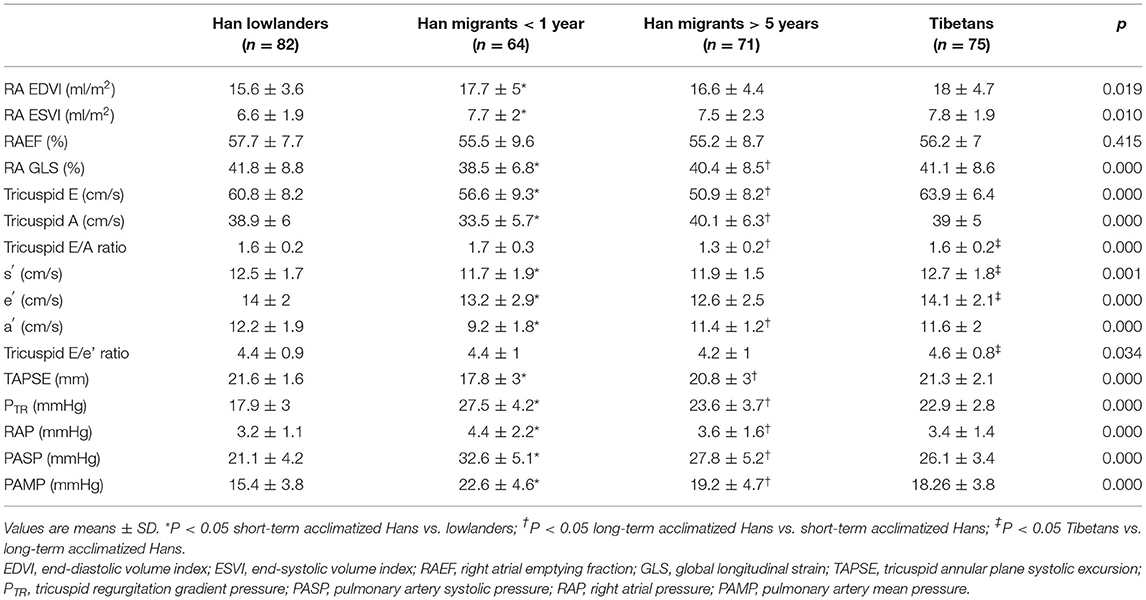
Table 2. Echocardiographic parameters of right heart in Han lowlanders, Han migrants and native Tibetans.
Compared with short-term Han migrants (3–12 months), RV FAC and TAPSE improved during a longer period of acclimatization (>5 years), and changes were also accompanied by reductions of RV end-diastolic and end-systolic area, almost back to the normal values as lowlanders. There was also evidence of RV anterior wall thickening. Importantly, RV GLS of longer migrants was also higher with improvements in RV-PA coupling (i.e., improved values of TAPSE/PASP).
Tibetans had higher RV FACs without thickening of the RV wall compared with long-term Han migrants. PASP in Tibetans was lower than in Han migrants and showed better RV-arterial coupling. Notably, Tibetans had nearly unchanged RV GLS compared to lowlanders, demonstrating more favorable adaptation to chronic hypoxia than highly acclimatized Han migrants.
Left Heart Characteristics Under Chronic Hypoxia
Left heart echocardiographic parameters are summarized in Figure 2 and Table 3. Significant changes in left heart structure under short-term exposure were characterized by reduced volumes of both LA and LV. LVEF was preserved, and there was a significant decrease in peak E and a mildly decreased E/e′. IVRT increased slightly with enhanced DT. As for cardiac mechanics, LV GLS was decreased, whereas LA GLS was preserved. Overall, these findings suggest that short-term exposure to chronic hypoxia resulted in decreased LV fillings but preserved systolic and diastolic functions.
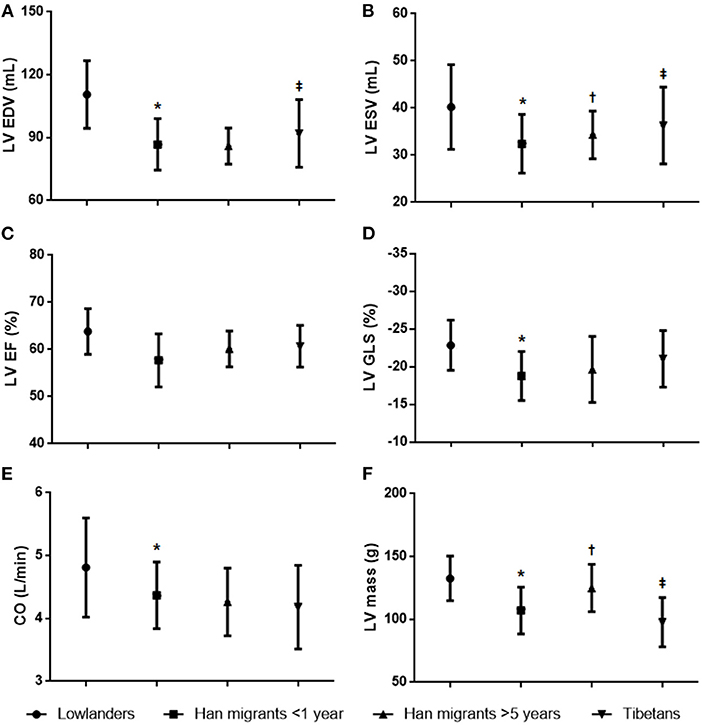
Figure 2. Between-group comparisons of left ventricular characteristics. (A) Left ventricular end-diastolic volume. (B) Left ventricular end-systolic volume. (C) Left ventricular ejection fraction. (D) Left ventricular global longitudinal strain. (E) Cardiac output. (F) Left ventricular mass. *p < 0.05 Han migrants <1 year vs. lowlanders; p < 0.05 Han migrants >5 years vs. Han migrants <1 year; ‡p < 0.05 Tibetans vs. Han migrants >5 years.
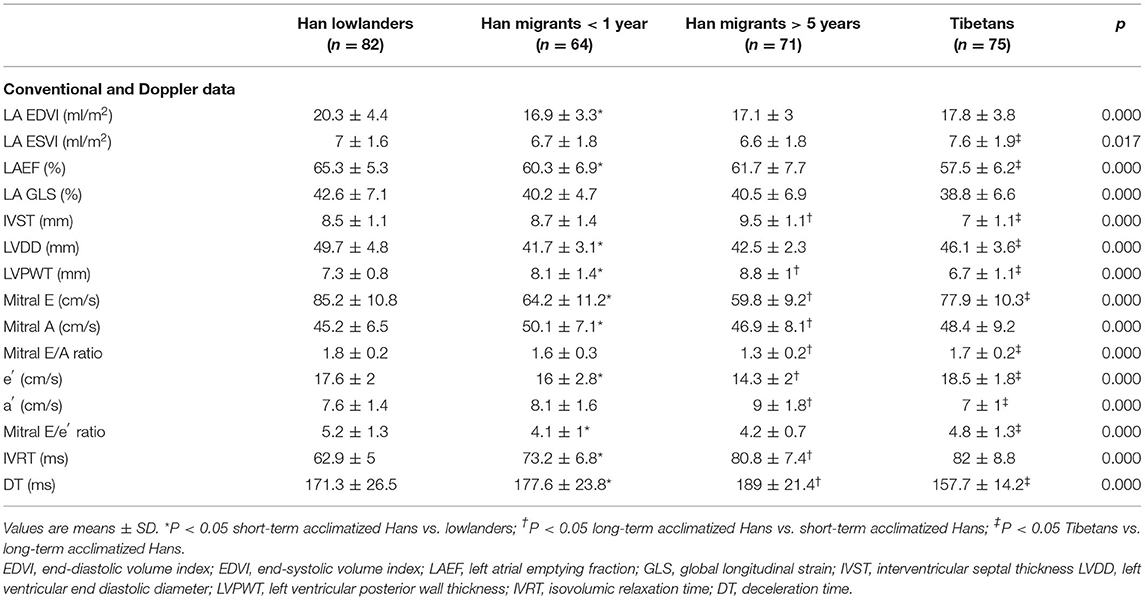
Table 3. Echocardiographic parameters of left heart in Han lowlanders, Han migrants and native Tibetans.
Compared with short-term Han migrants, LA and LV volumes of longer migrants (>5 years) remained small, and LVEF was also preserved. Notably, LV mass index was higher due to increased interventricular septum and posterior wall thicknesses. Mitral E/A and E/e′ remained low. IVRT and DT increased with preserved LA and LV GLS. This suggested that longer exposure to hypoxia caused significant LV thickening but no evidence of diastolic dysfunction or depression of cardiac mechanics.
In contrast, Tibetans had relatively larger LAs and LVs compared to highly acclimatized Hans, but no differences were noted in LVEF. Also, the LV mass index was smaller in Tibetans as they had no significant LV thickening. Additionally, LV GLS was also maintained within a normal range. Thus, overall, Tibetans demonstrated a more highly adapted pattern of cardiac characteristics than long-term acclimatized Han migrants.
Discussion
Although previous studies evaluated cardiovascular alterations in response to acute hypoxia at high altitudes, this study is the first to investigate the effects of different stages of chronic exposure on biventricular structure, function, and mechanics. By comparing clinic and echocardiographic measures among recent (3–12 months) Han migrants, long-term (>5 years) Han migrants, and native Tibetans, we observed that (1) recent Han migrants showed a significant response to hypoxia that was characterized by increased RV size, decreased RV FAC, and decreased longitudinal strain of both ventricles; (2) all of these factors improved during long-term (>5 years) dwelling, and while there was improved right ventricular-pulmonary arterial coupling, there was persistent ventricular thickening; (3) even after long-term exposure, significant differences in cardiac structure, function, and mechanics existed compared to the more highly adapted native Tibetans. The major findings of this study, along with proposed links between high altitude dwelling, cardiac structure and function, are summarized in Figure 3 and are discussed further below.
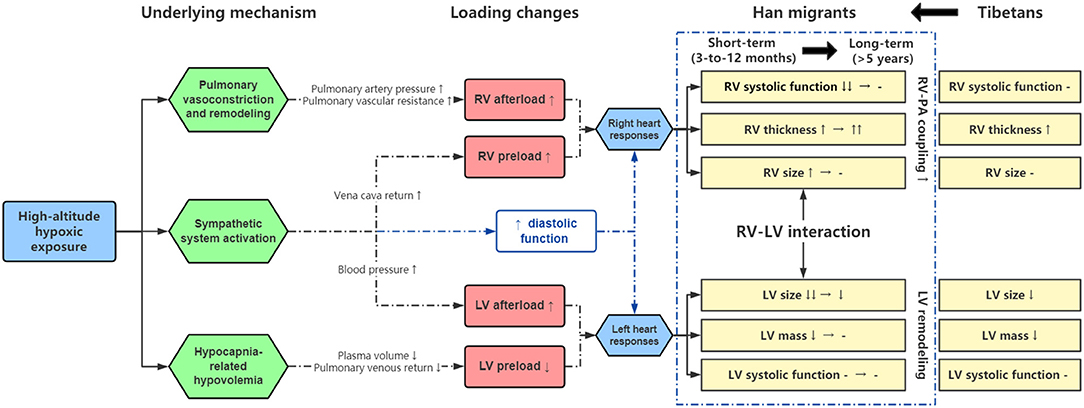
Figure 3. Summary of cardiac adaptations to prolonged exposure to chronic hypoxia. Refer to text for details.
RV Systolic Function Is Impaired in Recent (<1 Year) Migrants to High Altitudes but Improved During Long-Term (>5 Years) Dwelling
One of the important findings of our study was the adaptability of the RV and pulmonary vasculature of Han migrants to prolonged high altitude dwelling. Prior studies have shown that elevated PASP is the most immediate and prominent hemodynamic response to high altitude (16, 17). A recent survey showed that high altitude pulmonary hypertension (HAPH) could be detected in 6–35% of highlanders in the Asian population (18). Specifically, we observed a PASP of 33 ± 5 mmHg in recently adapted Han migrants, which is at the normal upper limit of healthy lowlanders; furthermore, 10% of Han migrants fulfilled the 2016 ERS/ESC guideline criteria for pulmonary hypertension (PH) with a PAMP >25 mmHg (19). Despite the increased afterload and noted changes of right ventricular properties measured in Han migrants (i.e., decreased RV FAC, TAPSE, and GLS), subjects were asymptomatic, and according to a recent expert consensus document, such responses may not have clinical significance. Specifically, it was noted that in high-altitude dwellers, the criteria for defining HAPH were PAMP > 30 mmHg and PASP > 50 mmHg in asymptomatic high-altitude dwellers (20); none of the subjects studied met these criteria. As reported previously, normal RV contractile reserve was observed in healthy high-altitude dwellers and even in patients with chronic mountain sickness (CMS), despite lower resting values of RV function (21). Also, a prior study showed that acute HA exposure impairs RA function in normal individuals (22), which has been shown to be a sensitive and valuable metric in assessing RV reserve and predicting exercise capacity (23, 24). In contrast, our study demonstrated that during prolonged adaption to high altitude, there was no significant depression of RA emptying fraction or RA GLS in Han migrants. This indicates that chronic exposure to hypoxia may not prevent adequate adaption to exercise of these healthy migrants despite decreased RV systolic function at rest.
Interestingly, the RV demonstrated significant adaptability, as we observed near normalization of RV size and functions in migrants with more than 5 years of adaption. The reasons for improvement may be partly explained by the concomitant decreases in PASP, increase in pulmonary oxygenation, and improved RV-PA coupling. Based on prior literature, mechanisms contributing to these improvements may include hypoxia-related activation of sympathetic tone, increased ventilation, and hypocapnia-induced hypovolemia (25). Evidence for increased sympathetic tone was provided by our observation that Hans always maintained a faster heart rate and smaller stroke volume during long-term high altitude dwelling. Furthermore, several studies demonstrated that acute hypoxia and HA exposure lead to a decline in heart rate variability (HRV), which indicates a marked increase in sympathetic tone (26, 27).
LV Remodeling Persists in Long-Term (>5 Years) Migrants but Was Not Associated With Impairment of LV Systolic or Diastolic Function
Han migrants showed significant LV remodeling characterized by decreased chamber size and increased wall thickness during the high-altitude dwelling. RV–LV interactions may have played an important role in the altered LV structure during 3–12 time frames. As shown in Figures 4A,B, the LV size was visibly reduced due to the leftward displacement of the ventricular septum. Moreover, decreased LV GLS was also accompanied by the decreased RV GLS we observed in recent Han migrants. However, the LVEF (overall systolic function) was preserved.
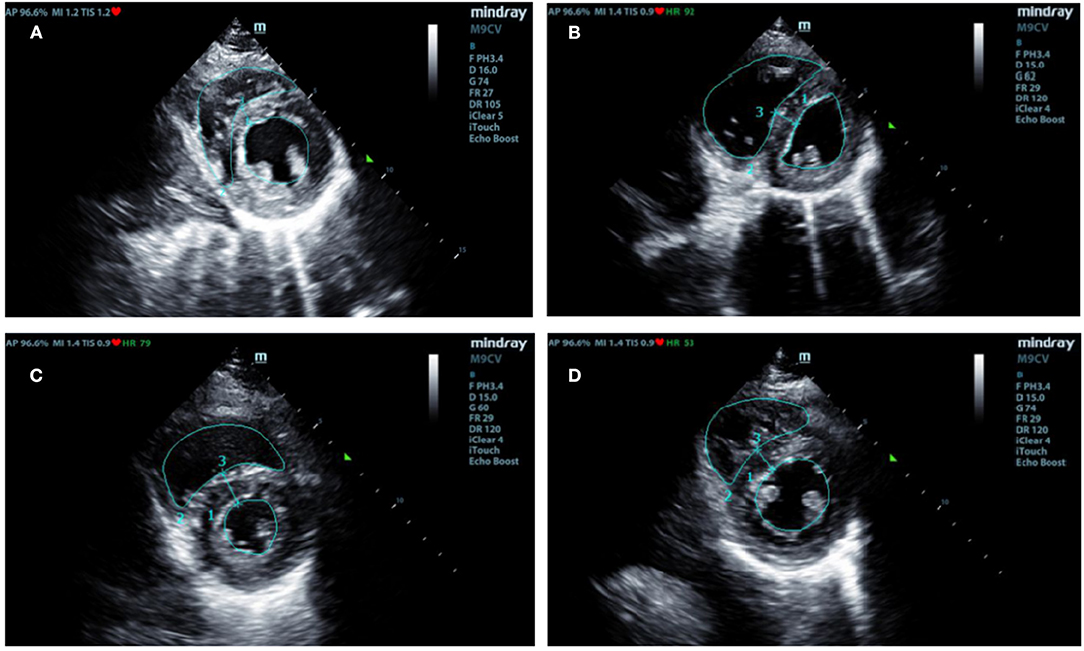
Figure 4. Typical changes of cardiac morphology during various durations of exposure to high altitude. End-systolic short-axis images. (A) Han lowlanders (controls) with normal volume and thickness. (B) Short-term Hans migrants (<1 year) had enlarged RV with the leftward shifted interventricular septum. (C) Long-term Hans migrants (>5 years) had significantly thickened LV wall with recovered RV size. (D) Native Tibetans had no or minimal differences in LV structure compared with Han lowlanders. RV, right ventricular; LV, left ventricular.
Moreover, despite the RV structure of Han migrants improving nearly back to normal during >5 years of adaption, the LV remained small with the increasingly thickened wall (Figure 4C). The small and thickened LV in long-term Han migrants may be due to persistent under-filling conditions and increased afterload as indexed by the persistently decreased mitral E/A and E/e′ and increased blood pressure, respectively. Several factors may contribute to such conditions. On the one hand, an increase in PASP reduced blood return to the left atrium. On the other hand, hypoxia and hypothermia at high altitude activated the sympathetic nervous system, which resulted in compensatory increases in ventilation, heat production, and peripheral vasoconstriction (28). In turn, these may lead to hypocapnia-related hypovolemia, an increase in LV afterload, and persistently increased LV wall thickness during the prolonged high-altitude dwelling.
Notably, such LV remodeling may not cause a significant decrease in diastolic function in these young healthy migrants. Previous studies reported that age was the most significant risk factor for the occurrence of left ventricular diastolic dysfunction among high-altitude dwellers (29). However, no evidence of diastolic dysfunction was observed in our subjects according to the definition of recent guidelines (30). We only observed slight changes in early myocardium relaxation (i.e., increased IVRT and DT) but without increased LV filling pressure (i.e., normal E/e′). In this regard, age should be taken into account as the subjects we enrolled were almost young migrants who may not be prone to develop LV diastolic dysfunction. Furthermore, as noted above, significant increases in sympathetic tone may contribute to preserving LV diastolic functions.
Tibetans Show Different Patterns of Adaptation to Chronic Hypoxia Compared With Highly Acclimatized Hans
Another finding of our study was that cardiac properties of highly acclimatized Hans do not fully return to normal. In contrast, native Tibetans show minimal differences in cardiac properties compared to lowlanders. In terms of cardiovascular properties, Tibetans have minimally altered cardiac structure, function, or mechanics. First, Tibetans showed a slight increase in PASP and a more favorable RV-PA coupling which did not cause a significant decrease in RV functions compared to Han migrants. In addition, relatively lower HR and BP in Tibetans suggest lower sympathetic tone and, therefore, would be less prone to LV thickening during long-term hypoxia exposure (Figure 4D). In terms of physiology, it was known that hypoxia can stimulate the increase of erythropoietin (EPO) and increase the number of red blood cells and hemoglobin concentration to maintain the normal arterial oxygen content (31, 32). Notably, lowlander migrants showed persistently increased hemoglobin and hematocrit along with prolonged dwellings at high altitudes. However, the excessive increase of hematocrit (> 50%), we observed, in most long-term migrants (>5 years) may increase blood viscosity, slow blood flow, and increase cardiac afterload, which can accelerate cardiac remodeling and increase the risk to develop premature cardiovascular disease (33). In contrast, there was a moderate increase of Hb and hematocrit in Tibetans that may not lead to the significant side effects of increased blood viscosity. Such a more favorable pattern of adaptation may reflect the long-term process of natural selection and generational evolution (34). Genetic differences between the Han population and Tibetans may provide important clues to mechanisms of cardiac and physiological adaption to high altitude, which should be given further consideration.
Limitations
This study has several limitations. First, this was a cross-sectional study comparing changes in cardiovascular properties in response to hypoxia in short vs. long-term migrants and long-term Hans vs. Tibetans; this cannot rule out the impact of individual variability on the results compared with a longitudinal study. Second, we did not analyze subjects of migrants with 1–5 years of adaptation due to the small number of subjects falling within this timeframe. Third, we did not enroll patients with high altitude pulmonary edema (HAPE) or CMS whose cardiovascular features have been reported in previous studies. In this regard, the results of this study provide important information concerning cardiovascular adaptations of healthy migrants to high altitudes.
Conclusion
Han migrants show improved RV structure and function during the long-term dwelling. However, LV remodeling persists without impairment of LV systolic or diastolic function. Despite after >5 years of acclimatization, cardiac properties of Han migrants do not fully return to normal. In contrast, native Tibetans show minimal differences in cardiac properties compared to lowlanders.
Data Availability Statement
The original contributions presented in the study are included in the article/supplementary material, further inquiries can be directed to the corresponding author/s.
Ethics Statement
The studies involving human participants were reviewed and approved by Chinese PLA General Hospital Ethics Committee. The patients/participants provided their written informed consent to participate in this study.
Author Contributions
XC, DB, and KH contributed to conception and design of the study. BL, QJ, and WC organized the database. FY and WW performed the statistical analysis. XC wrote the first draft of the manuscript. LY, ZL, QZ, and YL wrote sections of the manuscript. PZ and HP made charts and figures. All authors contributed to manuscript revision, read, and approved the submitted version.
Funding
This study was supported by the Science and Technology Innovation Special Zone: No. 19-163-12-ZD-037-003-02.
Conflict of Interest
HP and PZ were employed by BioMind Technology Company.
The remaining authors declare that the research was conducted in the absence of any commercial or financial relationships that could be construed as a potential conflict of interest.
Publisher's Note
All claims expressed in this article are solely those of the authors and do not necessarily represent those of their affiliated organizations, or those of the publisher, the editors and the reviewers. Any product that may be evaluated in this article, or claim that may be made by its manufacturer, is not guaranteed or endorsed by the publisher.
Acknowledgments
We sincerely appreciate the volunteers who participated in this study and the expedition team who collected data at high altitudes.
References
1. Penaloza D, Arias-Stella J. The heart and pulmonary circulation at high altitudes: healthy highlanders and chronic mountain sickness. Circulation. (2007) 115:1132–46. doi: 10.1161/circulationaha.106.624544
2. Falla M, Papagno C, Dal Cappello T, Vögele A, Hüfner K, Kim J, et al. A prospective evaluation of the acute effects of high altitude on cognitive and physiological functions in lowlanders. Front Physiol. (2021) 12:670278. doi: 10.3389/fphys.2021.670278
3. Champigneulle B, Doutreleau S, Baillieul S, Brugniaux JV, Robach P, Bouzat P, et al. Changes in cardiac function following a speed ascent to the top of Europe at 4808 m. Eur J Appl Physiol. (2022) 122:889–902. doi: 10.1007/s00421-022-04895-6
4. Naeije R. Physiological adaptation of the cardiovascular system to high altitude. Prog Cardiovasc Dis. (2010) 52:456–66. doi: 10.1016/j.pcad.2010.03.004
5. Mor-Avi V, Lang RM, Badano LP, Belohlavek M, Cardim NM, Derumeaux G, et al. Current and evolving echocardiographic techniques for the quantitative evaluation of cardiac mechanics: ase/eae consensus statement on methodology and indications endorsed by the Japanese society of echocardiography. Eur J Echocardiogr. (2011) 12:167–205. doi: 10.1093/ejechocard/jer021
6. Maufrais C, Rupp T, Bouzat P, Doucende G, Verges S, Nottin S, et al. Heart mechanics at high altitude: 6 days on the top of Europe. Eur Heart J Cardiovasc Imaging. (2017) 18:1369–77. doi: 10.1093/ehjci/jew286
7. Stembridge M, Ainslie PN, Hughes MG, Stöhr EJ, Cotter JD, Nio AQ, et al. Ventricular structure, function, and mechanics at high altitude: chronic remodeling in sherpa vs. short-term lowlander adaptation. J Appl Physiol. (2014) 117:334–43. doi: 10.1152/japplphysiol.00233.2014
8. Lang RM, Badano LP, Mor-Avi V, Afilalo J, Armstrong A, Ernande L, et al. Recommendations for cardiac chamber quantification by echocardiography in adults: an update from the american society of echocardiography and the european association of cardiovascular imaging. J Am Soc Echocardiogr. (2015) 28:1–39.e14. doi: 10.1016/j.echo.2014.10.003
9. Rudski LG, Lai WW, Afilalo J, Hua L, Handschumacher MD, Chandrasekaran K, et al. Guidelines for the echocardiographic assessment of the right heart in adults: a report from the american society of echocardiography endorsed by the european association of echocardiography, a registered branch of the european society of cardiology, and the canadian society of echocardiography. J Am Soc Echocardiogr. (2010) 23:685–713; quiz 86-8. doi: 10.1016/j.echo.2010.05.010
10. Naeije R, Torbicki A. More on the noninvasive diagnosis of pulmonary hypertension: doppler echocardiography revisited. Eur Respir J. (1995) 8:1445–9.
11. Brennan JM, Blair JE, Goonewardena S, Ronan A, Shah D, Vasaiwala S, et al. Reappraisal of the use of inferior vena cava for estimating right atrial pressure. J Am Soc Echocardiogr. (2007) 20:857–61. doi: 10.1016/j.echo.2007.01.005
12. Chemla D, Castelain V, Humbert M, Hébert JL, Simonneau G, Lecarpentier Y, et al. New formula for predicting mean pulmonary artery pressure using systolic pulmonary artery pressure. Chest. (2004) 126:1313–7. doi: 10.1378/chest.126.4.1313
13. Tello K, Wan J, Dalmer A, Vanderpool R, Ghofrani HA, Naeije R, et al. Validation of the tricuspid annular plane systolic excursion/systolic pulmonary artery pressure ratio for the assessment of right ventricular-arterial coupling in severe pulmonary hypertension. Circul Cardiovasc Imag. (2019) 12:e009047. doi: 10.1161/circimaging.119.009047
14. Voigt JU, Pedrizzetti G, Lysyansky P, Marwick TH, Houle H, Baumann R, et al. Definitions for a common standard for 2d speckle tracking echocardiography: consensus document of the eacvi/ase/industry task force to standardize deformation imaging. J Am Soc Echocardiogr. (2015) 28:183–93. doi: 10.1016/j.echo.2014.11.003
15. Badano LP, Kolias TJ, Muraru D, Abraham TP, Aurigemma G, Edvardsen T, et al. Standardization of left atrial, right ventricular, and right atrial deformation imaging using two-dimensional speckle tracking echocardiography: a consensus document of the eacvi/ase/industry task force to standardize deformation imaging. Eur Heart J Cardiovasc Imaging. (2018) 19:591–600. doi: 10.1093/ehjci/jey042
16. Bärtsch P, Gibbs JS. Effect of altitude on the heart and the lungs. Circulation. (2007) 116:2191–202. doi: 10.1161/circulationaha.106.650796
17. Soria R, Egger M, Scherrer U, Bender N, Rimoldi SF. Pulmonary artery pressure and arterial oxygen saturation in people living at high or low altitude: systematic review and meta-analysis. J Appl Physiol. (2016) 121:1151–9. doi: 10.1152/japplphysiol.00394.2016
18. Lichtblau M, Saxer S, Furian M, Mayer L, Bader PR, Scheiwiller PM, et al. Cardiac function and pulmonary hypertension in central Asian highlanders at 3250 M. Eur Resp J. (2020) 56:1902474. doi: 10.1183/13993003.02474-2019
19. Galiè N, Humbert M, Vachiery JL, Gibbs S, Lang I, Torbicki A, et al. 2015 Esc/Ers guidelines for the diagnosis and treatment of pulmonary hypertension: the joint task force for the diagnosis and treatment of pulmonary hypertension of the European Society of Cardiology (Esc) and the European Respiratory Society (Ers): Endorsed By: Association for European Paediatric and Congenital Cardiology (Aepc), International Society for Heart and Lung Transplantation (Ishlt). Eur Heart J. (2016) 37:67–119. doi: 10.1093/eurheartj/ehv317
20. León-Velarde F, Maggiorini M, Reeves JT, Aldashev A, Asmus I, Bernardi L, et al. Consensus statement on chronic and subacute high altitude diseases. High Alt Med Biol. (2005) 6:147–57. doi: 10.1089/ham.2005.6.147
21. Pratali L, Allemann Y, Rimoldi SF, Faita F, Hutter D, Rexhaj E, et al. Rv contractility and exercise-induced pulmonary hypertension in chronic mountain sickness: a stress echocardiographic and tissue doppler imaging study. JACC Cardiovasc Imag. (2013) 6:1287–97. doi: 10.1016/j.jcmg.2013.08.007
22. He C, Liu C, Yu S, Yang J, Ding X, Bian S, et al. Atrial performance in healthy subjects following high altitude exposure at 4100 m: 2d speckle-tracking strain analysis. Int J Cardiovasc Imaging. (2021) 37:1891–902. doi: 10.1007/s10554-021-02173-8
23. Alenezi F, Mandawat A. Il'Giovine ZJ, Shaw LK, Siddiqui I, Tapson VF, et al. Clinical utility and prognostic value of right atrial function in pulmonary hypertension. Circul Cardiovasc Imag. (2018) 11:e006984. doi: 10.1161/circimaging.117.006984
24. Liu W, Wang Y, Zhou J, Bai H, Wang F, Wang J. The association of functional capacity with right atrial deformation in patients with pulmonary arterial hypertension: a study with two-dimensional speckle tracking. Heart Lung Circ. (2018) 27:350–8. doi: 10.1016/j.hlc.2017.02.029
25. Stembridge M, Ainslie PN, Boulet LM, Anholm J, Subedi P, Tymko MM, et al. The independent effects of hypovolaemia and pulmonary vasoconstriction on ventricular function and exercise capacity during acclimatisation to 3800 m. J Physiol. (2019) 597:1059–72. doi: 10.1113/jp275278
26. Buchheit M, Richard R, Doutreleau S, Lonsdorfer-Wolf E, Brandenberger G, Simon C. Effect of acute hypoxia on heart rate variability at rest and during exercise. Int J Sports Med. (2004) 25:264–9. doi: 10.1055/s-2004-819938
27. Mellor A, Bakker-Dyos J, O'Hara J, Woods DR, Holdsworth DA, Boos CJ. Smartphone-enabled heart rate variability and acute mountain sickness. Clin J Sport Med. (2018) 28:76–81. doi: 10.1097/jsm.0000000000000427
28. Greaney JL, Kenney WL, Alexander LM. Neurovascular mechanisms underlying augmented cold-induced reflex cutaneous vasoconstriction in human hypertension. J Physiol. (2017) 595:1687–98. doi: 10.1113/jp273487
29. Han S, Zhao L, Ma S, Chen Z, Wu S, Shen M, et al. Alterations to cardiac morphology and function among high-altitude workers: a retrospective cohort study. Occup Environ Med. (2020) 77:447–53. doi: 10.1136/oemed-2019-106108
30. Nagueh SF, Smiseth OA, Appleton CP, Byrd BF 3rd, Dokainish H, Edvardsen T, et al. Recommendations for the evaluation of left ventricular diastolic function by echocardiography: an update from the american society of echocardiography and the european association of cardiovascular imaging. J Am Soc Echocardiogr. (2016) 29:277–314. doi: 10.1016/j.echo.2016.01.011
31. Burtscher M, Gatterer H, Burtscher J, Mairbäurl H. Extreme terrestrial environments: life in thermal stress and hypoxia. a narrative review. Front Physiol. (2018) 9:572. doi: 10.3389/fphys.2018.00572
32. Akunov A, Sydykov A, Toktash T, Doolotova A, Sarybaev A. Hemoglobin changes after long-term intermittent work at high altitude. Front Physiol. (2018) 9:1552. doi: 10.3389/fphys.2018.01552
33. Rivera-Ch M, León-Velarde F, Huicho L. Treatment of chronic mountain sickness: critical reappraisal of an old problem. Respir Physiol Neurobiol. (2007) 158:251–65. doi: 10.1016/j.resp.2007.05.003
Keywords: high altitude acclimatization, cardiac responses, cardiac physiology, echocardiography, strain
Citation: Chen X, Liu B, Deng Y, Yang F, Wang W, Lin X, Yu L, Pu H, Zhang P, Li Z, Zhong Q, Jia Q, Li Y, Wang X, Chen W, Burkhoff D and He K (2022) Cardiac Adaptation to Prolonged High Altitude Migration Assessed by Speckle Tracking Echocardiography. Front. Cardiovasc. Med. 9:856749. doi: 10.3389/fcvm.2022.856749
Received: 17 January 2022; Accepted: 06 April 2022;
Published: 23 May 2022.
Edited by:
Lan Huang, Xinqiao Hospital, ChinaReviewed by:
Robert Naeije, Université libre de Bruxelles, BelgiumNazareno Paolocci, Johns Hopkins University, United States
Copyright © 2022 Chen, Liu, Deng, Yang, Wang, Lin, Yu, Pu, Zhang, Li, Zhong, Jia, Li, Wang, Chen, Burkhoff and He. This is an open-access article distributed under the terms of the Creative Commons Attribution License (CC BY). The use, distribution or reproduction in other forums is permitted, provided the original author(s) and the copyright owner(s) are credited and that the original publication in this journal is cited, in accordance with accepted academic practice. No use, distribution or reproduction is permitted which does not comply with these terms.
*Correspondence: Kunlun He, a3VubHVuaGUmI3gwMDA0MDtwbGFnaC5vcmc=
†These authors have contributed equally to this work