- 1Department of Nephrology, Zhongshan Hospital, Fudan University, Shanghai, China
- 2Shanghai Medical Center of Kidney, Shanghai, China
- 3Shanghai Key Laboratory of Kidney and Blood Purification, Shanghai, China
Introduction: The question of whether the increased burden of chronic kidney disease (CKD) is caused by the interaction of hyperuricemia and cardiovascular disease (CVD) risk factors or is accelerated by aging remains unresolved. The purpose of this study is to better understand the effect modification of hyperuricemia, cardiovascular risk, and age on CKD among the Chinese population.
Methods: This cross-sectional study of 8243 participants was derived from the China Health and Nutrition Survey (CHNS) in 2009. Inclusion criteria included age ≥18 years, non-pregnancy, and no history of high-protein diet prior to blood test. Demographics, comorbidities, health-related behaviors, and serum biomarkers were collected. Interaction association of hyperuricemia, CVD risk and age with CKD were analyzed using Logistic regression.
Results: CKD was detected in 359 (27.2%, 95% CI 24.8∼29.7%) of 1321 participants with hyperuricemia and 680 (9.8%, 95% CI 9.1∼10.5%) of 6,922 participants without hyperuricemia, and these patterns remained significant after controlling for age, gender, and Framingham risk score (adjusted odds ratio [aOR] 3.82, 95% CI 3.20∼4.57). We found a negative multiplicative interaction between hyperuricemia and CVD risk on CKD. The aOR in low-CVD risk groups was 5.51 (95% CI 4.03∼7.52), followed by medium-CVD risk groups (aOR: 3.64, 95% CI 2.61∼5.09) and high-CVD risk groups (aOR: 2.89, 95% CI 2.12∼3.96). CVD risk was less associated with CKD in hyperuricemia group (aOR: 0.92, 95% CI 0.68∼1.22) than in non-hyperuricemia group (aOR: 1.43, 95% CI 1.21∼1.70). Furthermore, hyperuricemia and age had a significant additive effect on CKD, with a synergy index of 2.26 (95% CI 1.45∼3.52). Coexisting with older age and hyperuricemia, the likelihood of developing CKD was higher than the sum of the two alone.
Conclusion: The link between hyperuricemia and CKD begins at a young age and becomes stronger in the low CVD risk group. For young adults, early detection of hyperuricemia, routine CVD risk assessment, and timely intervention of modifiable factors are warranted.
Introduction
Chronic kidney disease (CKD) is a growing public health concern around the world (1). According to the Global Burden of Disease Study, there were 697⋅5 million CKD cases in 2017, which was considerably higher than those individuals with diabetes, asthma, osteoarthritis and chronic obstructive pulmonary disease (COPD) (2, 3). China has the most CKD patients (132.3 million), accounting for one-fifth of all cases worldwide. If left untreated, CKD can progress to end-stage renal disease (ESRD), which necessitates renal replacement therapy and hurts the quality of life (4). The global all-age mortality rate from CKD has increased 41⋅5% in the last three decades (2). Impaired kidney functions also contributed to 7⋅6% of cardiovascular disease (CVD) deaths (2). Therefore, the importance of CKD prevention and early detection should pose the same priority as the management of diabetes and hypertension.
The kidney is the major excretory and endocrine organ of the body and plays an important role in maintaining homeostasis. Cardiovascular function and metabolic status are closely related to kidney function (5–7). Previous evidence suggests that CVD risk factors (hypertension, diabetes, smoking, and alcohol drinking) and elevated serum uric acid are associated with an increased incidence of CKD (8, 9). Kidney dysfunction, in turn, accelerates the onset of CVD and impairs urate excretion (10, 11). However, the relative importance of CVD risk and hyperuricemia to CKD has not been well established. Furthermore, as an aging-related disease, CKD was more common in older adults (12). This complicates determining whether the increased CKD burden is due to the interaction of hyperuricemia and CVD risk or is accelerated by aging itself. Most of the previous studies were limited by insufficient sample size and a lack of age-stratified analysis to quantify the effect modification of hyperuricemia, CVD risk, and age on CKD.
We hypothesized that hyperuricemia and CVD risk factors had complex interactions with CKD and this process is modified by age as well. To this end, we conducted a cross-sectional analysis derived from the China Health and Nutrition Survey (CHNS) cohort to better understand the association and interaction effects of hyperuricemia, CVD risk, and age on CKD.
Materials and Methods
Study Design and Patients
China health and nutrition survey is a China-wide prospective cohort study that is currently being conducted in fifteen provinces and autonomous cities/districts (Beijing, Chongqing, Guangxi, Guizhou, Heilongjiang, Henan, Hubei, Hunan, Jiangsu, Liaoning, Shaanxi, Shandong, Shanghai, Yunnan, and Zhejiang). For each study field, community-based participants were recruited through multistage, random cluster sampling. The survey began in 1989 and follow-up data collection has occurred every 2–4 years since then. CHNS has completed ten waves by 2015. An in-depth description of CHNS was can be found at https://www.cpc.unc.edu/projects/china/. In 2009, fasting blood was collected for the first time, covering twenty-six measures. Major CVD biomarkers (lipids, HbA1c, glucose, insulin, triglycerides), renal biomarkers (urea, creatinine), metabolic biomarkers (uric acid, cholesterol, and triglyceride), and important nutrition biomarkers (transferrin, hemoglobin, albumin, and ferritin) were included. Our study was designed to be cross-sectional. A total of 9,516 participants who provided blood samples and anthropometry data were recruited as the study population. After exclusion of 787 adolescents (<18 years old), 62 pregnant women, 31 individuals failing in blood test, 336 individuals on a high protein-rich diet (>110g/day) (13), and 12 individuals with implausible data, 8,243 participants were eligible for the formal analysis (Figure 1). When using the sample size formula for () and setting the CKD prevalence (π) to 10.8% (12) and the limit error (δ) to 3%, a minimum of 411 subjects would have been needed to achieve 90% power and 5% significance level. The present sample size provides adequate statistical capacity. The institutional review boards of the University of North Carolina (United States) and the National Institute of Nutrition and Food Safety (China) approved the ethics of CHNS (14). All participants signed written informed consent forms and their identities were withheld from the public datasets.
Data Collection and Measurements
Standardized questionnaires were used to collect demographic information (age, gender, education, residence, nationality and family income), comorbidity (hypertension, diabetes and myocardial infarction), and health-related behaviors (smoking, alcohol consumption and physical exercise). Age was grouped into 15-year age categories: 18∼29 years, 30∼44 years, 45∼59 years, 60∼74 years, and 75∼ years. Education was divided into never, primary school, junior high school, senior high school, and post-secondary education. Health-related behaviors included smoking status (never, former, or current smoker), alcohol use (never, former, or current drinker), and physical activity. We applied metabolic equivalents (MET) per week to quantify physical activity and classified it into three groups: <57.17 MET/week (low tertile T1), 57.17–148.06 MET/week (medium tertile T2), and ≥148.06 MET/week (high tertile T3) (15). Additionally, anthropomorphic and blood pressure (BP) measurements were taken from the participants. Body mass index (BMI) was calculated as weight in kilograms divided by height in meters squared. Waist-hip ratio was calculated by dividing the waist circumference (cm) by the hip circumference (cm). BP was measured at rest with a mercury sphygmomanometer and collected in triplicate to obtain the mean value. Hypertension was defined as a systolic BP ≥140 mmHg or diastolic BP ≥90 mmHg, or self-reported diagnosis by questionnaire. Diabetes was defined by self-reported or diabetes treatment records. About 12 ml of fasting blood was drawn by venipuncture. The serum was separated and stored in the ultra-low-temperature freezer. All samples were analyzed in Beijing at a national central lab under strict quality control (16). Serum uric acid was detected using enzymatic colorimetric method (Hitachi 7600 automated analyzer). Serum creatinine was detected using picric acid method. Total cholesterol (TC) was detected by CHOD-PAP method, and high-density lipoprotein cholesterol (HDL-C) was detected by enzymatic method. Hemoglobin A1c (HbA1c) was detected in high-performance liquid chromatography system (HLC-723 G7).
Definition of Hyperuricemia, Cardiovascular Disease Risk, and Chronic Kidney Disease
Hyperuricemia was defined as a fasting serum uric acid level >420 μmol/L in males and >360 μmol/L in females, consistent with other studies (17, 18). CVD risk was assessed using the Framingham risk score (FRS), which can predict 10-year CVD risk based on factors including age, gender, TC, HDL-C, smoking status, BP, and diabetes (19, 20). CVD risk was graded as low (FRS <10%), medium (FRS 10–20%), or high (FRS >20%) (21). We applied the CKD-EPI 2009 creatinine equation to calculate the estimated glomerular filtration rate (eGFR) (22). The outcome variable of CKD was defined as eGFR <60 mL/min/1.73 m2 according to Kidney Disease: Improving Global Outcomes (KDIGO) 2012 guideline (4).
Statistical Analysis
Continuous data were expressed as mean ± standard deviation or median (interquartile range), and categorical data were expressed as frequency (percentage). Group comparisons between continuous variables were assessed using Student’ t-test and Mann–Whitney test when appropriate. Pearson chi-square test was used for binary and unordered categorical variables, whereas Cochran-mantel-haenszel test was used for ordinal categorical variables. Logistic regression was done to analyze the association of hyperuricemia and CVD risk with CKD. We further introduced a multiplicative interaction term (hyperuricemia × CVD-risk × age) into the model to investigate effect modification. Subsequently, subgroup analyses were performed by adjusting for age, gender, and FRS (or uric acid) depending on which association was examined. In addition, we did analyses to examine the additive interaction of age+hyperuricemia and age+CVD-risk. The synergistic effect was quantified using relative excess risk due to interaction (RERI), attributable proportion due to interaction (AP), and synergy index (SI). Sensitivity analyses were done to assess the robustness of the results by treating eGFR as a continuous variable. When the 95% confidence interval (CI) for RERI, AP, and SI did not overlap 0, 0 and 1, respectively, significant additive interaction was observed. A two-tailed p < 0.05 was considered the statistical significance threshold. Analyses were performed using the package of “ggplot2,” “gmodels” and “epiR” in the R 3.6.1 software (R core team).
Results
Baseline Characteristics
In total, 8,243 participants were recruited in the final analysis (Figure 1). The mean age was 51.59 ± 15.28 years, and the gender ratio was 0.85 (male 3790/female 4453). Approximately 16.0% of participants had hyperuricemia and 12.6% had CKD. Men and well-educated participants had a higher prevalence of hyperuricemia, whereas women and participants with illiteracy or primary education had a higher prevalence of CKD (Table 1). Older age, urban residence, and higher family income were all associated with both hyperuricemia and CKD. Compared to healthy controls, participants with hyperuricemia and CKD had more CVD risk factors, including former smoking, hypertension, diabetes, overweight, and lower physical activities. These resulted in an overall higher FRS value (p < 0.001) and a greater proportion of medium- and high-CVD risk among participants (p < 0.001) with hyperuricemia and CKD. Notably, current smokers and drinkers were more susceptible to hyperuricemia but had the lowest prevalence of CKD. The sensitivity analysis between analytic participants and excluded participants found that the proportions of illiteracy, suffering from comorbidities and never smoker/drinkers were lower in the excluded group (Supplementary Table 1), which was primarily attributed to the exclusion criteria for adolescents (<18 years old).
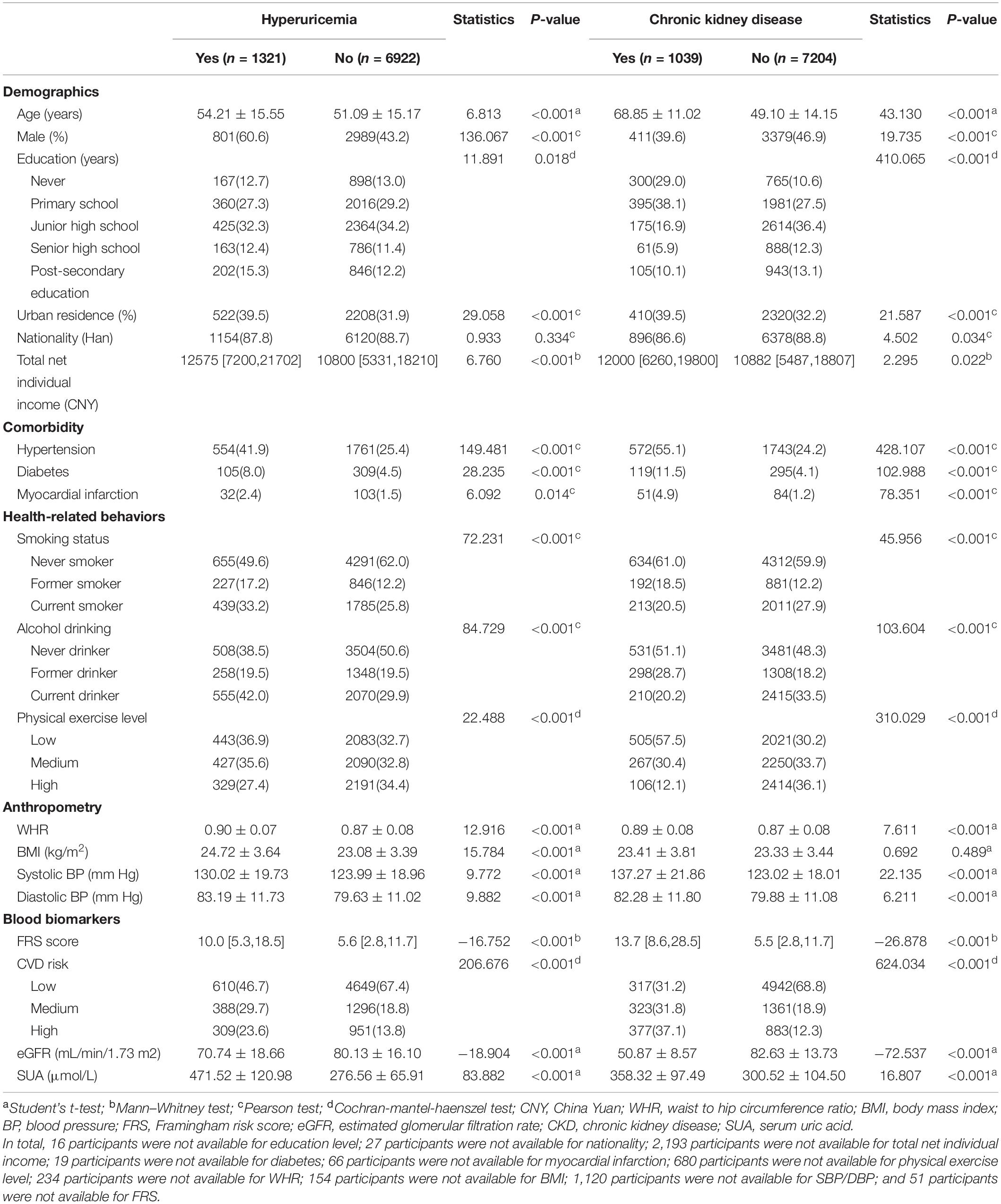
Table 1. Baseline characteristics of the participants stratified by hyperuricemia and chronic kidney disease (n = 8243).
Age-Specific Association Analysis of Hyperuricemia and Cardiovascular Disease Risk With Chronic Kidney Disease
Chronic kidney disease (eGFR <60 mL/min/1.73 m2) was found in 359 (27.2%, 95% CI 24.8∼29.7%) of 1321 participants with hyperuricemia and 680 (9.8%, 95% CI 9.1∼10.5%) of 6922 participants without hyperuricemia. Patterns remained significant after adjusting for age, gender, and FRS (adjusted odds ratio [aOR] 3.82, 95% CI 3.20∼4.57). The likelihood of CKD increased along with the progression of CVD risk strata (6.0% vs. 19.2% vs. 29.9%, p < 0.001), and this positive significant association was maintained in the adjusted model (aOR 1.37, 95% CI 1.20∼1.56). Similarly, eGFR values were lower in participants with hyperuricemia and a high-CVD risk than healthy controls, with the differences of −8.04 (95% CI −8.79∼−7.30) and −1.33 (95% CI −1.85∼−0.81), respectively.
Participants with hyperuricemia had higher FRS values than participants without hyperuricemia in the 18∼29, 30∼44, 45∼59, and 60∼74 age groups, but the difference was no longer significant in the 75∼ age group (Figure 2). In contrast, significant higher proportions of CKD were observed in participants with hyperuricemia in age groups 30∼44 years (4.3% vs. 0.7% [p < 0.001]), 45∼59 years (15.9% vs. 4.6% [p < 0.001]), 60∼74 years (46.8% vs. 16.8% [p < 0.001]) and 75∼ years (77.4% vs. 54.0% [p < 0.001]), but not at 18∼29 years (1.0% vs. 0.2% [p = 0.266]), implying larger differences in older age. A comparison of eGFR estimates across age groups in participants with and without hyperuricemia revealed similar patterns, as did the correlation analysis among FRS, eGFR, and uric acid.
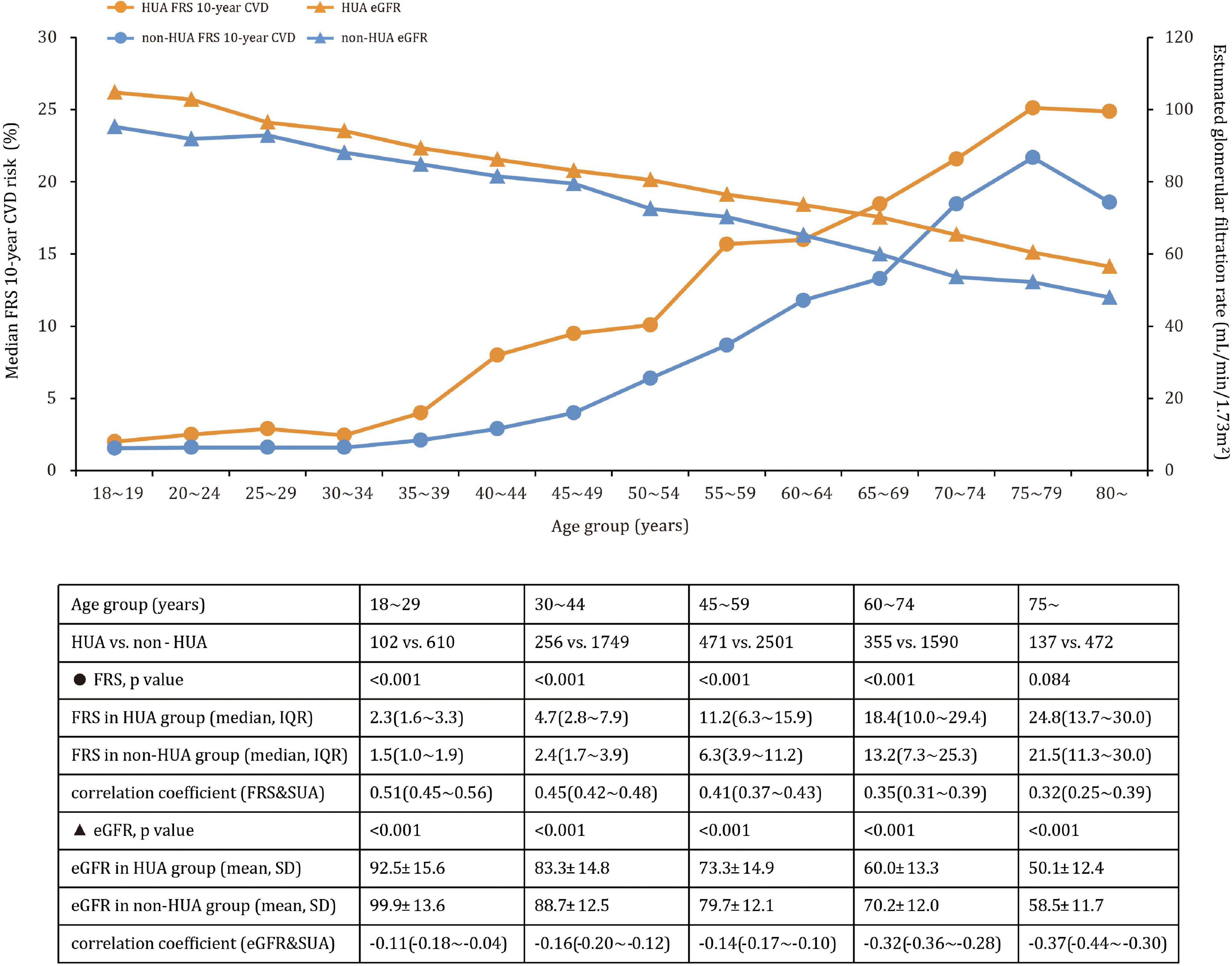
Figure 2. Mean eGFR (SD) and median CVD risk (interquartile range) across age groups in participants with and without hyperuricemia.
Multiplicative Interaction Models of Hyperuricemia, Cardiovascular Disease Risk, and Age on Chronic Kidney Disease
A significant negative interaction between hyperuricemia and CVD risk was observed on the likelihood of CKD in the multivariable model (βhyperuricemi*CVD–risk = −0.343, p = 0.001), showing that the effect of hyperuricemia on CKD attenuated with increased CVD risk (Figure 3A). In particular, hyperuricemia was associated with CKD, independent of age, gender and FRS, in low-CVD risk groups (aOR: 5.51, 95% CI 4.03∼7.52), medium-CVD risk groups (aOR: 3.64, 95% CI 2.61∼5.09), and high-CVD risk groups (aOR: 2.89, 95% CI 2.12∼3.96). The aOR curve was an inverted parabola in the medium- and high-CVD groups and participants aged 60∼74 years had the highest CKD prevalence (aOR 4.64 and 3.44 [all p < 0.001]). In a sensitivity analysis using eGFR as a continuous variable, the interaction between hyperuricemia and CVD risk was positively related to eGFR value (βhyperuricemia*CVD risk = 1.89, p = 0.002). Furthermore, there was an interaction between hyperuricemia and age, negatively influencing eGFR (βhyperuricemia*age = −1.57, p = 0.002 in Figure 3B).
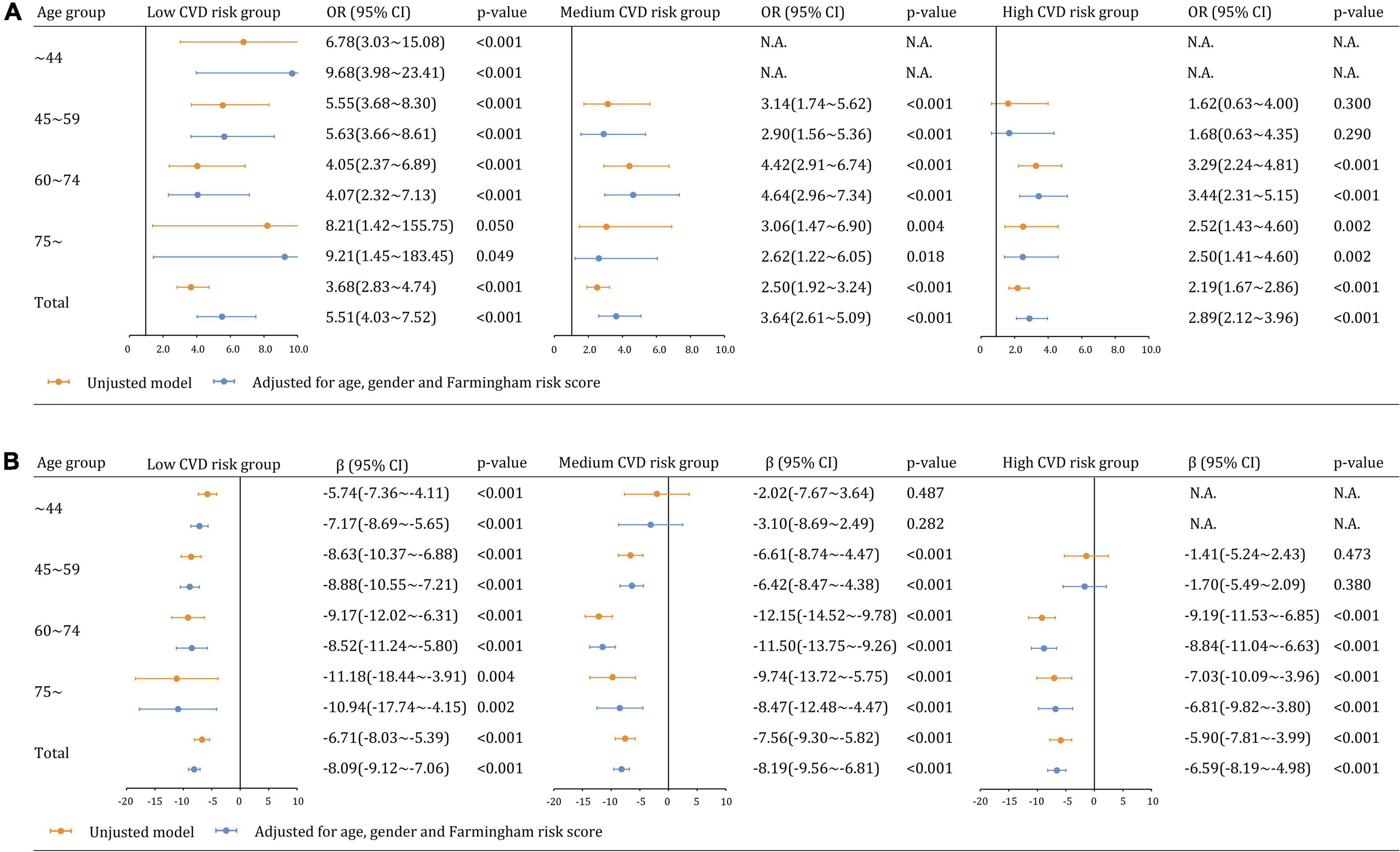
Figure 3. Multiplicative interaction models examining the relationships between hyperuricemia and kidney dysfunction across CVD risk and age groups (A) CKD (eGFR <60 mL/min/1.73 m2). (B) eGFR as a continuous variable.
We further analyzed the relationship between CVD risk and CKD across age groups. CVD risk was less likely to be significantly associated with CKD and eGFR estimates in participants with hyperuricemia than those without hyperuricemia (Figure 4A). The adjusted associations between CVD risk and CKD in participants with hyperuricemia were not significant overall (aOR: 0.92, 95% CI 0.68∼1.22 [p = 0.559]) and in all age groups. In contrast, the associations became significant among participants without hyperuricemia overall (aOR: 1.43, 95% CI 1.21∼1.70), as well as in the age groups of 45∼59 years (aOR: 1.58, 95% CI 1.05∼2.38), 60∼74 years (aOR: 1.54, 95% CI 1.22∼1.94), but not in the age groups of 75∼ years (aOR: 1.33, 95% CI 0.94∼1.89 [p = 0.111]). Similar findings were observed in the sensitivity analysis of eGFR as a continuous variable (Figure 4B).
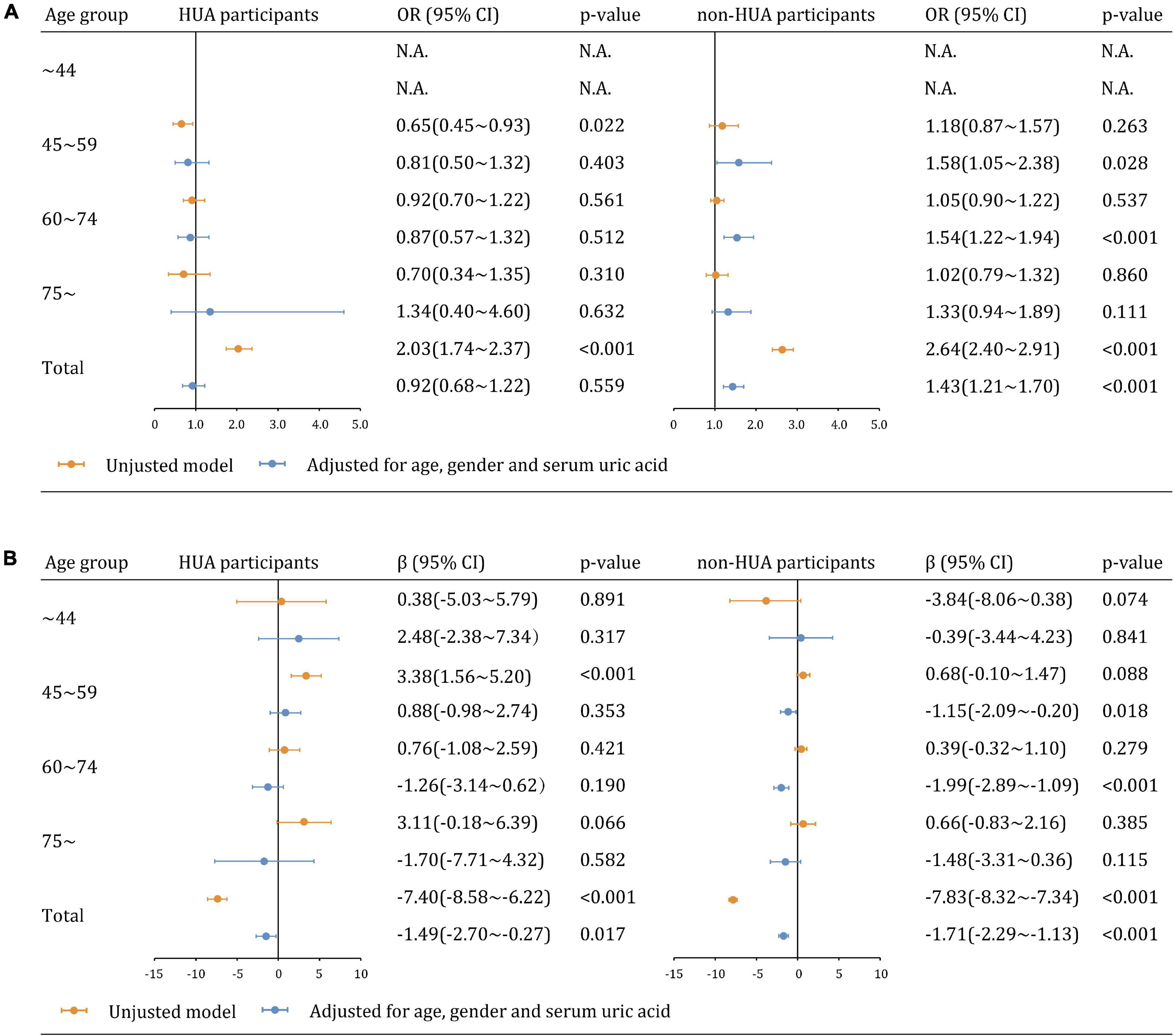
Figure 4. Multiplicative interaction models examining the relationships between CVD risk and kidney dysfunction across hyperuricemia and age groups (A) CKD (eGFR <60 mL/min/1.73 m2). (B) eGFR as a continuous variable.
Additive Interaction Models of Hyperuricemia, Cardiovascular Disease Risk and Age on Chronic Kidney Disease
Figure 5 depicted the additive interaction of hyperuricemia, CVD risk, and age group in relation to CKD. After adjusting for age and gender, participants with hyperuricemia in 75∼ age group had the highest likelihood of CKD when compared to those who aged ∼44 years and without hyperuricemia. The values of RERI, AP, and SI were 18.38 (95% CI 4.40∼32.36), 0.54 (95% CI 0.35∼0.73), and 2.26 (95% CI 1.45∼3.52), respectively. It indicated that coexisting with older age and hyperuricemia, the likelihood of CKD was significantly higher than the sum of the two alone. According to the estimate of AP, 54% of the likelihood of CKD could be attributed to the synergistic effect of hyperuricemia+age. However, no such additive interaction between hyperuricemia and CVD risk was not discovered. In a sensitivity analysis using eGFR as a continuous variable, both hyperuricemia+age and hyperuricemia+CVD risks were found to have additive interactive effects (all p < 0.001, Supplementary Figure 1).
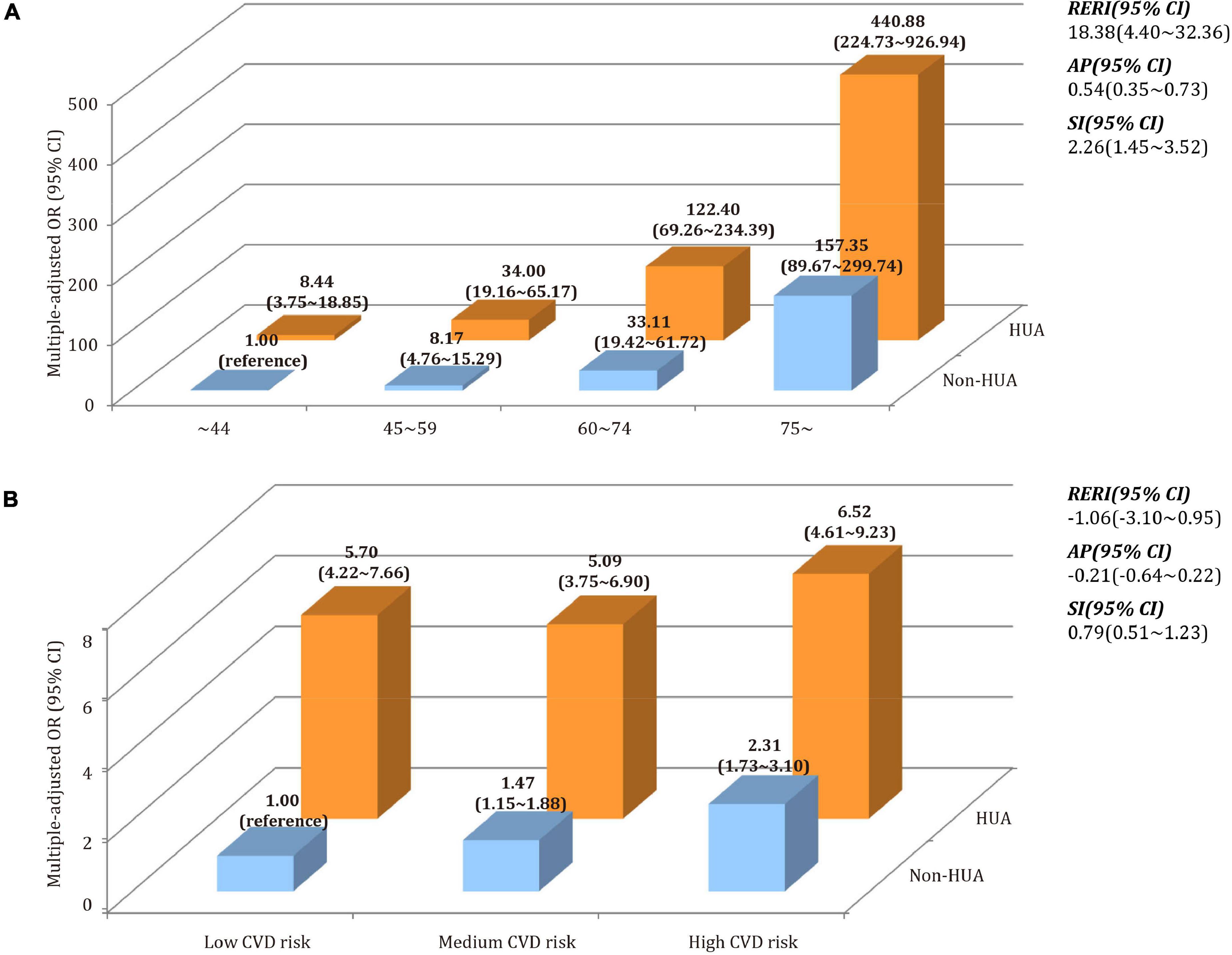
Figure 5. Synergistic effect of the interaction between hyperuricemia, CVD risk, and age on CKD. (A) Additive model of hyperuricemia (HUA) + age on CKD. (B) Additive model of hyperuricemia (HUA) + CVD risk on CKD.
Discussion
In this cross-sectional study, we discovered a negative multiplicative interaction between hyperuricemia and CVD risk, and a positive additive interaction between hyperuricemia and age, both of which have a complex impact on CKD. It was observed that hyperuricemia was more strongly associated with CKD in the low CVD-risk subgroup compared with those in the medium- and high-groups. The significant association between CVD risk and CKD was found only in participants without hyperuricemia. Furthermore, when older age and hyperuricemia coexisted, the combined effect was greater than the sum of their independent effects. To the best of our knowledge, this is the first study in China that identified the age-specific effect modification of metabolic disease and traditional cardiovascular risk on the prevalence of CKD in the general population.
Consistent with previous research (23–25), hyperuricemia was found to be associated with CKD independent of other covariates. Daniel et al. (23) found there was a 7% increase in the risk of CKD for every serum uric acid increase (aOR: 1.07, 95% CI 1.01 to 1.14). Notably, in patients with increased SUA levels, the risk of developing new-onset CKD increased as the duration of follow-up increased, suggesting that hyperuricemia may play an important role in the long-term progression of chronic renal dysfunction (24, 25). Other studies, however, have not found such a positive association. Yasuaki et al. (26) found that, in patients with diabetes, the baseline uric acid levels were not significantly associated with the development of nephropathy. Another cohort study in patients with diabetes found the decline rate of eGFR was not correlated with uric acid (27). To obtain robust results, we established multivariate models by taking CKD and eGFR as outcomes. It was observed that the associations between hyperuricemia and CKD remained significant in all CVD risk groups and were stronger in the low-CVD risk. Furthermore, participants with hyperuricemia had a higher FRS value and lower kidney function since the age group of 18∼29 years. These findings suggest that young participants with hyperuricemia are at risk of early onset of CVD events and kidney dysfunction, despite the absence of clinical hyperuricemia and gout symptoms. Since early-stage of hyperuricemia is often asymptomatic, it has traditionally been overlooked as a simple bystander effect of decreased eGFR (28). Once the kidneys are impaired, urate-lowering treatments are ineffective in slowing the progression of CKD (29). Two negative clinical trials (30, 31), published in the New England Journal of Medicine, suggested that lowering uric acid is unlikely to be beneficial in CKD patients. Moreover, our study found a synergistic effect between age and hyperuricemia on CKD. It is well known that the morphology of the kidney changes with age, and its physiological function continues to deteriorate. Prolonged hyperuricemia exposure to the elderly increases the cumulative risk of kidney damage. The disappearance of the association in the age group of 75∼ years is due to the effect of hyperuricemia on CKD being masked by the complicated comorbidities and smaller sample size.
The specific mechanisms by which hyperuricemia may be associated with CKD have primarily been reported in experimental studies. Firstly, crystallized monosodium urate is suspected of causing urolithiasis, and the subsequent hydronephrosis causes increased intratubular pressure, resulting in decreased renal blood flow and glomerular filtration (32, 33). Secondly, elevated uric acid levels can promote preglomerular arterioles medial thickening and are directly correlated with glomerular capillary pressure (34). Such arteriolar changes in the kidney can result in ischemia and hypoxia, two of the most powerful triggers for tubulointerstitial fibrosis (35). Thirdly, hyperuricemia can hasten renal function deterioration indirectly through hypertension, cyclooxygenase-mediated thromboxane-induced vascular disease, and insulin resistance (36, 37).
Traditional CVD risk factors, such as hypertension, diabetes, and former smoking, were found to be associated with a high prevalence of CKD. The cardiovascular and renal systems have numerous pathophysiological connections. Disease in one organ may cause dysfunction in the other, leading to the failure of both organs (38). On one hand, cardiac dysfunction can increase intrarenal resistance and decrease renal blood perfusion, which further aggravates kidney damage by activating the renin-angiotensin system, increasing inflammatory responses and oxidative stress (39). On the other hand, CKD patients often encountered anemia, sodium-water retention, calcium-phosphorus metabolism disorders and emerging uremic toxin. These pathological changes increase the risk of CVD events and mortality in CKD patients (10). Our data showed that the association between CVD risk on CKD was significant only in participants without hyperuricemia in the stratified analysis. It indicated that hyperuricemia had a stronger link to CKD, which could overshadow the effects of other factors. Previous studies also found that traditional CVD risk factors do not account for the high cardiovascular risk in CKD patients, and that managing CVD in CKD patients was ineffective (5). Therefore, timely intervention in the early stages of hyperuricemia may be more beneficial than in the later stages, when CVD risks have accumulated and kidney damages have occurred.
Our study is unique in that it is a large-scale study of CKD in the Chinese population using standardized sampling, and it uses stratified analyses to evaluate the interactive effects of hyperuricemia, CVD risk and age on CKD. However, some limitations must be mentioned. Firstly, the cross-sectional design does not allow us to examine the effect of hyperuricemia and CVD risk on the progression of CKD. The present associations are not considered causal. Secondly, the diagnosis of CKD was on serum creatinine without taking albuminuria into consideration. It may lead to misclassification for estimating the true prevalence of CKD. Thirdly, selection bias might still exist in this study, although the difference between excluded and included people was mainly due to the exclusion of participants aged below 18 years. Fourthly, high purine diets and concomitant medications (urate-lowering therapy and antihypertensive agents) may affect the estimate of hyperuricemia and CVD risk (40, 41). However, treatment information was not collected for all participants in the CHNS study, which could lead to potential confounding biases.
Conclusion
Our findings provide evidence that hyperuricemia is associated with CKD independently of CVD risk and age. Interaction analyses revealed that such an association begins at a young age and becomes stronger in the low-CVD risk group, implying a risk of early onset of CKD. Further studies should be conducted to clarify the causal effect modifications and identify novel biomarkers for CKD prediction in various disease contexts. For young adults, early detection of hyperuricemia, routine CVD risk assessment, and timely intervention of modifiable factors are warranted.
Data Availability Statement
Publicly available datasets were analyzed in this study. This data can be found here: https://www.cpc.unc.edu/projects/china/news.
Ethics Statement
The studies involving human participants were reviewed and approved by Boards of the University of North Carolina (United States) and the National Institute of Nutrition and Food Safety (China) approved the ethics of CHNS. The patients/participants provided their written informed consent to participate in this study.
Author Contributions
YL, YF, and XD contributed to the conception and design of the work. YL, BZ, and YX contributed to the acquisition, analysis, and interpretation of data for the work. SJ and WZ participated in data management. YL and BZ drafted the manuscript. XD and YF critically revised the manuscript. All authors gave final approval and agreed to be accountable for all aspects of work ensuring integrity and accuracy.
Funding
This analytic study was sponsored by the National Natural Science Foundation of China (82103911), Natural Science Foundation of Shanghai (21ZR1412400), Shanghai Key Laboratory of Kidney and Blood Purification (20DZ2271600), and Shanghai Clinical Medical Center (2017ZZ01015). This research uses data from China Health and Nutrition Survey (CHNS). We are grateful to research grant funding from the National Institute for Health (NIH), the Eunice Kennedy Shriver National Institute of Child Health and Human Development (NICHD) for R01 HD30880, National Institute on Aging (NIA) for R01 AG065357, National Institute of Diabetes and Digestive and Kidney Diseases (NIDDK) for R01DK104371 and R01HL108427, the NIH Fogarty grant D43 TW009077 since 1989, and the China-Japan Friendship Hospital, Ministry of Health for support for CHNS 2009, Chinese National Human Genome Center at Shanghai since 2009, and Beijing Municipal Center for Disease Prevention and Control since 2011.
Conflict of Interest
The authors declare that the research was conducted in the absence of any commercial or financial relationships that could be construed as a potential conflict of interest.
Publisher’s Note
All claims expressed in this article are solely those of the authors and do not necessarily represent those of their affiliated organizations, or those of the publisher, the editors and the reviewers. Any product that may be evaluated in this article, or claim that may be made by its manufacturer, is not guaranteed or endorsed by the publisher.
Supplementary Material
The Supplementary Material for this article can be found online at: https://www.frontiersin.org/articles/10.3389/fcvm.2022.853917/full#supplementary-material
Abbreviations
AP, attributable proportion due to interaction; BMI, body mass index; BP, blood pressure; CHNS, China health and nutrition survey; CNY, China Yuan; CI, confidence interval; CKD, chronic kidney disease; COPD, chronic obstructive pulmonary disease; CVD, cardiovascular disease; eGFR, estimated glomerular filtration rate; ESRD, end-stage kidney disease; FRS, Framingham risk score; HbA1c, Hemoglobin A1c; HDL-C, high-density lipoprotein cholesterol; IQR, interquartile range; KDIGO, kidney disease: improving global outcomes; MET, metabolic equivalents; RERI, relative excess risk due to interaction; SI, synergy index; SUA, serum uric acid; TC, total cholesterol; WHR, waist to hip circumference ratio; OR, odds ratio.
References
1. Drawz P, Rahman M. Chronic kidney disease. Ann Intern Med. (2015) 162:ITC1–16. doi: 10.7326/aitc201506020
2. Bikbov B, Purcell CA, Levey AS, Smith M, Abdoli A, Abebe M, et al. Global, regional, and national burden of chronic kidney disease, 1990–2017: a systematic analysis for the global burden of disease study 2017. Lancet. (2020) 395:709–33. doi: 10.1016/S0140-6736(20)30045-3
3. James SL, Abate D, Abate KH, Abay SM, Abbafati C, Abbasi N, et al. Global, regional, and national incidence, prevalence, and years lived with disability for 354 diseases and injuries for 195 countries and territories, 1990–2017: a systematic analysis for the global burden of disease study 2017. Lancet. (2018) 392:1789–858. doi: 10.1016/S0140-6736(18)32279-7
4. Levin A, Stevens P, Bilous RW, Coresh J, Francisco A, Jong PE, et al. Kidney disease: improving global outcomes (KDIGO) CKD work group. KDIGO 2012 clinical practice guideline for the evaluation and management of chronic kidney disease. Kidney Int Suppl. (2013) 3:1–150. doi: 10.1038/kisup.2012.73
5. Liu M, Li XC, Lu L, Cao Y, Sun RR, Chen S, et al. Cardiovascular disease and its relationship with chronic kidney disease. Eur Rev Med Pharmacol Sci. (2014) 18:2918–26.
6. Weaver DJ Jr. Uric acid and progression of chronic kidney disease. Pediatr Nephrol. (2019) 34:801–9. doi: 10.1007/s00467-018-3979-2
7. Johnson RJ, Nakagawa T, Jalal D, Sánchez-Lozada LG, Kang DH, Ritz E. Uric acid and chronic kidney disease: which is chasing which? Nephrol Dial Transplant. (2013) 28:2221–8. doi: 10.1093/ndt/gft029
8. Kelly JT, Su G, Zhang L, Qin X, Marshall S, González-Ortiz A, et al. Modifiable lifestyle factors for primary prevention of CKD: a systematic review and meta-analysis. J Am Soc Nephrol. (2021) 32:239–53. doi: 10.1681/asn.2020030384
9. Zhu P, Liu Y, Han L, Xu G, Ran JM. Serum uric acid is associated with incident chronic kidney disease in middle-aged populations: a meta-analysis of 15 cohort studies. PLoS One. (2014) 9:e100801. doi: 10.1371/journal.pone.0100801
10. Gansevoort RT, Correa-Rotter R, Hemmelgarn BR, Jafar TH, Heerspink HJ, Mann JF, et al. Chronic kidney disease and cardiovascular risk: epidemiology, mechanisms, and prevention. Lancet. (2013) 382:339–52. doi: 10.1016/s0140-6736(13)60595-4
11. Zoccali C, Mallamaci F. Uric acid in chronic kidney disease: the quest for causality continues. Nephrol Dial Transplant. (2018) 33:193–5. doi: 10.1093/ndt/gfx341
12. Zhang L, Wang F, Wang L, Wang W, Liu B, Liu J, et al. Prevalence of chronic kidney disease in China: a cross-sectional survey. Lancet. (2012) 379:815–22. doi: 10.1016/s0140-6736(12)60033-6
13. Chinese Nutrition Society. Chinese Dietary Reference Intakes Handbook (2013 version). Beijing: Standards Press of China (2014).
14. Zhang B, Zhai FY, Du SF, Popkin BM. The China health and nutrition survey, 1989-2011. Obes Rev. (2014) 15(Suppl. 1):2–7. doi: 10.1111/obr.12119
15. Ainsworth BE, Haskell WL, Herrmann SD, Meckes N, Bassett DR Jr, Tudor-Locke C, et al. 2011 compendium of physical activities: a second update of codes and MET values. Med Sci Sports Exerc. (2011) 43:1575–81. doi: 10.1249/MSS.0b013e31821ece12
16. Yan S, Li J, Li S, Zhang B, Du S, Gordon-Larsen P, et al. The expanding burden of cardiometabolic risk in China: the China health and nutrition survey. Obes Rev. (2012) 13:810–21. doi: 10.1111/j.1467-789X.2012.01016.x
17. Zhu Y, Pandya BJ, Choi HK. Prevalence of gout and hyperuricemia in the US general population: the national health and nutrition examination survey 2007-2008. Arthritis Rheum. (2011) 63:3136–41. doi: 10.1002/art.30520
18. Baker JF, Krishnan E, Chen L, Schumacher HR. Serum uric acid and cardiovascular disease: recent developments, and where do they leave us? Am J Med. (2005) 118:816–26. doi: 10.1016/j.amjmed.2005.03.043
19. D’Agostino RB Sr, Vasan RS, Pencina MJ, Wolf PA, Cobain M, Massaro JM, et al. General cardiovascular risk profile for use in primary care: the Framingham heart study. Circulation. (2008) 117:743–53. doi: 10.1161/circulationaha.107.699579
20. Wilson PW, D’Agostino RB, Levy D, Belanger AM, Silbershatz H, Kannel WB. Prediction of coronary heart disease using risk factor categories. Circulation. (1998) 97:1837–47. doi: 10.1161/01.cir.97.18.1837
21. Lin H, Ding Y, Ning C, Qiao X, Chen X, Chen X, et al. Age-specific associations between HIV infection and carotid artery intima-media thickness in China: a cross-sectional evaluation of baseline data from the CHART cohort. Lancet HIV. (2019) 6:e860–8. doi: 10.1016/s2352-3018(19)30263-2
22. Levey AS, Stevens LA, Schmid CH, Zhang Y, Castro AF, Feldman HI, et al. A new equation to estimate glomerular filtration rate. Ann Intern Med. (2009) 150:604–12. doi: 10.7326/0003-4819-150-9-200905050-00006
23. Weiner DE, Tighiouart H, Elsayed EF, Griffith JL, Salem DN, Levey AS. Uric acid and incident kidney disease in the community. J Am Soc Nephrol. (2008) 19:1204–11. doi: 10.1681/asn.2007101075
24. Li L, Yang C, Zhao Y, Zeng X, Liu F, Fu P. Is hyperuricemia an independent risk factor for new-onset chronic kidney disease?: a systematic review and meta-analysis based on observational cohort studies. BMC Nephrol. (2014) 15:122. doi: 10.1186/1471-2369-15-122
25. Mende C. Management of chronic kidney disease: the relationship between serum uric acid and development of nephropathy. Adv Ther. (2015) 32:1177–91. doi: 10.1007/s12325-015-0272-7
26. Hayashino Y, Okamura S, Tsujii S, Ishii H. Association of serum uric acid levels with the risk of development or progression of albuminuria among Japanese patients with type 2 diabetes: a prospective cohort study [diabetes distress and care registry at Tenri (DDCRT 10)]. Acta Diabetol. (2016) 53:599–607. doi: 10.1007/s00592-015-0825-x
27. Gu L, Huang L, Wu H, Lou Q, Bian R. Serum uric acid to creatinine ratio: a predictor of incident chronic kidney disease in type 2 diabetes mellitus patients with preserved kidney function. Diab Vasc Dis Res. (2017) 14:221–5. doi: 10.1177/1479164116680318
28. Ponticelli C, Podestà MA, Moroni G. Hyperuricemia as a trigger of immune response in hypertension and chronic kidney disease. Kidney Int. (2020) 98:1149–59. doi: 10.1016/j.kint.2020.05.056
29. Floege J, Johnson RJ. Hyperuricemia and progression of chronic kidney disease: to treat or not to treat? Kidney Int. (2021) 99:14–6. doi: 10.1016/j.kint.2020.10.022
30. Badve SV, Pascoe EM, Tiku A, Boudville N, Brown FG, Cass A, et al. Effects of allopurinol on the progression of chronic kidney disease. N Engl J Med. (2020) 382:2504–13. doi: 10.1056/NEJMoa1915833
31. Doria A, Galecki AT, Spino C, Pop-Busui R, Cherney DZ, Lingvay I, et al. Serum urate lowering with allopurinol and kidney function in type 1 diabetes. N Engl J Med. (2020) 382:2493–503. doi: 10.1056/NEJMoa1916624
32. Li CC, Chien TM, Wu WJ, Huang CN, Chou YH. Uric acid stones increase the risk of chronic kidney disease. Urolithiasis. (2018) 46:543–7. doi: 10.1007/s00240-018-1050-1
33. Worcester E, Parks JH, Josephson MA, Thisted RA, Coe FL. Causes and consequences of kidney loss in patients with nephrolithiasis. Kidney Int. (2003) 64:2204–13. doi: 10.1046/j.1523-1755.2003.00317.x
34. Sánchez-Lozada LG, Tapia E, Avila-Casado C, Soto V, Franco M, Santamaría J, et al. Mild hyperuricemia induces glomerular hypertension in normal rats. Am J Physiol Renal Physiol. (2002) 283:F1105–10. doi: 10.1152/ajprenal.00170.2002
35. Liu M, Ning X, Li R, Yang Z, Yang X, Sun S, et al. Signalling pathways involved in hypoxia-induced renal fibrosis. J Cell Mol Med. (2017) 21:1248–59. doi: 10.1111/jcmm.13060
36. Kang DH, Nakagawa T, Feng L, Watanabe S, Han L, Mazzali M, et al. A role for uric acid in the progression of renal disease. J Am Soc Nephrol. (2002) 13:2888–97. doi: 10.1097/01.asn.0000034910.58454.fd
37. Zhu Y, Hu Y, Huang T, Zhang Y, Li Z, Luo C, et al. High uric acid directly inhibits insulin signalling and induces insulin resistance. Biochem Biophys Res Commun. (2014) 447:707–14. doi: 10.1016/j.bbrc.2014.04.080
38. Ronco C, Bellasi A, Di Lullo L. Cardiorenal syndrome: an overview. Adv Chronic Kidney Dis. (2018) 25:382–90. doi: 10.1053/j.ackd.2018.08.004
39. Kaesler N, Babler A, Floege J, Kramann R. Cardiac remodeling in chronic kidney disease. Toxins. (2020) 12:161. doi: 10.3390/toxins12030161
40. Lee JS, Oh JS, Kim YG, Lee CK, Yoo B, Hong S. Rapid reduction in uric acid by a urate-lowering agent is associated with recurrent cardiovascular events. Med Hypothes. (2020) 141:109740. doi: 10.1016/j.mehy.2020.109740
Keywords: hyperuricemia, cardiovascular disease risk, chronic kidney disease, interaction analysis, China health and nutrition survey
Citation: Li Y, Zhu B, Xie Y, Jin S, Zhou W, Fang Y and Ding X (2022) Effect Modification of Hyperuricemia, Cardiovascular Risk, and Age on Chronic Kidney Disease in China: A Cross-Sectional Study Based on the China Health and Nutrition Survey Cohort. Front. Cardiovasc. Med. 9:853917. doi: 10.3389/fcvm.2022.853917
Received: 13 January 2022; Accepted: 16 February 2022;
Published: 07 March 2022.
Edited by:
Gen-Min Lin, Hualien Armed Forces General Hospital, TaiwanReviewed by:
Abebe Bereda Timerga, Independent Researcher, Kulubi, EthiopiaEdward Zimbudzi, Monash University, Australia
Copyright © 2022 Li, Zhu, Xie, Jin, Zhou, Fang and Ding. This is an open-access article distributed under the terms of the Creative Commons Attribution License (CC BY). The use, distribution or reproduction in other forums is permitted, provided the original author(s) and the copyright owner(s) are credited and that the original publication in this journal is cited, in accordance with accepted academic practice. No use, distribution or reproduction is permitted which does not comply with these terms.
*Correspondence: Yi Fang, fang.yi@zs-hospital.sh.cn; Xiaoqiang Ding, ding.xiaoqiang@zs-hospital.sh.cn
†These authors have contributed equally to this work