- 1Third-Grade Pharmacological Laboratory on Traditional Chinese Medicine China Three Gorges University, Yichang, China
- 2Medical College, China Three Gorges University, Yichang, China
- 3The Second People’s Hospital of Yichang, Yichang, China
- 4The First People’s Hospital of Yichang, Yichang, China
Objective: Polyamines mainly contain spermine (SPM), spermidine (SPD), and putrescine (PUT). Many research results suggest that polyamines participate in cell proliferation, differentiation, and the regulation of gene expression, and have a close relationship with the occurrence and development of many diseases. However, the role and possible mechanisms of action of polyamines from cardiac mast cells in myocardial remodeling induced by pressure overload remain to be elucidated.
Methods: Pressure overload was induced by abdominal aortic constriction (AAC). Toluidine blue staining was used to visualize mast cells in cardiac tissue. The polyamine content of cardiac tissue was analyzed using high-performance liquid chromatography. Opening of the mitochondrial permeability transition pore (MPTP) was determined by the Ca2+-induced swelling of isolated cardiac mitochondria, measured as a reduction in A520.
Results: Compared with sham rats, the cardiac mast cell density, the polyamine content (PUT, SPB, and SPM), and myocardial MPTP opening in rats with AAC were significantly increased (P < 0.05), and were accompanied by increased myocardial fibrosis and heart weight/body weight ratio. Intraperitoneal injection of polyamines mimicked these results, and these effects were reversed by cromolyn sodium, a mast cell stabilizer (P < 0.05). Myocardial MPTP opening increased in rats with AAC (P < 0.05), and the three polyamines also increased myocardial MPTP opening (P < 0.05).
Conclusion: Mast cell-derived polyamines are involved in pressure overload-induced myocardial remodeling by increasing opening of the MPTP.
Introduction
A large number of studies have shown that cardiac mast cells play an important role in cardiac remodeling (1). Experimental studies have found that inhibition of mast cell degranulation significantly reduces cardiac remodeling in several heart diseases including hypertension, ischemia/reperfusion injury, and transplantation/rejection disease (1–6). Studies in mast cell-deficient animals have shown that cardiac weight is significantly reduced after hypertension and ischemia-reperfusion (7). The process of mast cell involvement in cardiac remodeling is closely associated with the material released by mast cell degranulation after activation, which include a variety of active substances including histamine, cytokines, phospholipase, proteases, and fatty-acid metabolites (8–10). Although studies have suggested that some of these components such as proteases and cytokines activate collagenases and matrix metalloproteinases that are involved in cardiac remodeling (11, 12), the specific substances that induce remodeling after degranulation of cardiac mast cells still need further investigation. A recent study has established for the first time that, in addition to the above known substances, polyamines are also released by mast cells after activation (13).
Interestingly, in cases of cardiac ischemia and increased pressure overload, there is an increase in the polyamine content of heart tissue in addition to an increase in mast cell density (13–15). And many research results suggest that polyamines are involved in the cardiac remodeling process induced by stress (16, 17).
Polyamines mainly contain spermine (SPM), spermidine (SPD), and putrescine (PUT), which are small organic molecules widely found in eukaryotic cells. In the organism, ornithine is decarboxylated to form PUT under the action of ornithine decarboxylase, PUT produces SPD under the action of SPD synthase, and SPD produces SPM under the action of SPM synthase. Polyamines not only participate in many important physiological processes such as cell proliferation, differentiation, and the regulation of gene expression, but also have a close relationship with the occurrence and development of many diseases (18–20). It has been reported that the cardiac polyamine content is higher in spontaneously hypertensive rats, and the administration of SPM to such rats significantly increases their blood pressure (21). Studies on vascular polyamines in hypertensive rats have also shown similar changes in cardiac polyamines in rats with spontaneous hypertension, and this change is accompanied by changes in vascular function (22). These results suggest that polyamines are closely associated with hypertension, a stress-loading heart disease, and have important research value. Studies have shown that polyamines are involved in the cardiac hypertrophy induced by the stimulation of norepinephrine and its receptor system (23), suggesting that polyamines play an important role in cardiac remodeling. Research has shown that the respiratory amine concentration increases in tracheal cystic fibrosis (24), suggesting that polyamines participate in the process of tissue fibrosis. However, the exact role and mechanisms of action of polyamines in cardiac remodeling induced by pressure overload remain unclear.
Research on cardiac remodeling mechanisms indicates that the mitochondrial permeability transition pore (MPTP) is involved in the process of cardiac remodeling: in mice: with knockout of the MPTP opening regulatory protein SIRT3, the MPTP opens easily, and this is accompanied by cardiac hypertrophy and fibrosis (25). In mice with gene knockout of the MPTP regulatory protein cyclophilin D, stress overload results in more severe cardiac hypertrophy and fibrosis (26, 27). These findings suggest that abnormal opening of the MPTP leads to more severe cardiac hypertrophy and fibrosis. However, these experimental data were all obtained under a condition of artificially changing the opening of the MPTP. Whether the cardiac remodeling induced by pressure overload is accompanied by an abnormal change of the MPTP, and if so, what the underlying possible mechanism is, remain unclear.
Based on the above, we hypothesized that pressure overload induces cardiac mast cell aggregation in cardiac tissue and polyamines released from these cells may be involved in the cardiac remodeling induced by pressure overload through the regulation of MPTP opening. Here, we tested this hypothesis using an animal model of pressure overload induced by abdominal aortic stenosis and mitochondria isolated from the rat heart.
Materials and Methods
Animals
Male and female Sprague–Dawley rats (160–170 g) were obtained from the Animal Center of China Three Gorges University, housed under standard conditions, and maintained on commercial rat chow and tap water ad libitum. All experimental protocols were approved by the Institutional Animal Care and Use Committee of China Three Gorges University and adhered to the National Institutes of Health guidelines for the use of experimental animals.
Pressure-Overload Rat Model
The rat model of pressure overload was induced by abdominal aortic constriction (AAC) (28). Briefly, rats were anesthetized with 10% chloral hydrate (350 mg/kg, i.p.), and the aorta was dissected above the two renal arteries. A silver clip (0.70 mm internal diameter) was placed on the abdominal aorta above the level of the left renal artery.
Study Design
Rats were randomly divided into the following groups: the AAC group, in which the rats were subjected to AAC (half male and half female); the sham group, in which the rats underwent the same surgery as in the AAC group but without placement of the abdominal aortic clip; the CS group, in which the rats were treated with cromolyn sodium (CS, 25 mg/kg/day, i.p., half male and half female) (29) after AAC surgery; and the polyamine groups, in which the rats were treated with polyamines (PUT, SPD, or SPM, 10 mg/kg/day, i.p., half male and half female) (30–32). All hearts were then carefully removed after 4 or 8 weeks of treatment. The middle section of the left ventricle was immediately divided into 2-mm thick transverse sections and immersed in 10% neutral buffered paraformaldehyde for histological analysis. Other tissue samples were flash-frozen in liquid nitrogen and stored at -80°C until Assays were performed.
Chemicals
Putrescine, SPD, SPM, and 1,6 hexamethylene diamine were from Sigma Chemical Co.; CS, chymase, and histamine were from Macklin; Masson’s trichromatic staining solution and toluidine blue color solution were from Google Biology; and hematoxylin-eosin staining solution was prepared in the laboratory.
Measurement of Rat Myocardium Polyamine Levels Using High-Performance Liquid Chromatography
After euthanasia, hearts were excised and weighed. In each group, ∼150 mg of the left ventricle was taken, and cold perchloric acid (0.3 mol/L) was added. Then a tissue homogenate was prepared in an ice water bath, and centrifuged before collecting the supernatant. The perchloric supernatant was alkalinized by 2 mol/L NaOH, followed by the addition of benzoyl chloride to form derivatives. The derivatives were separated and determined on a Waters 2,690 reverse-phase high-performance liquid chromatography system equipped with an auto-sampler, column oven, ultraviolet (UV) detector and a reversed-phase Luna C18, analytical column (150 × 4.6 mm id, 5 μm particle size). Data and spectra were recorded and analyzed by the Millennium 32 Chromatography Workstation (Waters, United States). Chromatographic separation was achieved with isocratic elution using a mobile phase composed of water (60%) and acetonitrile (40%) at 1.0 ml/min.
Histological Analysis
Transverse sections at the mid-ventricular level (2 mm) were fixed in 10% neutral buffer. Then, histological analyses were done using standard protocols. Masson’s trichrome staining (33) was used to assess the area of fibrotic infiltration in the wall of the left ventricle. The collagen fibers stained blue, in contrast to red-stained myocardium. In each specimen, the degree of fibrosis was quantified independently by two pathologists in a blinded fashion. Each section was imaged using an Olympus BX53 camera (Tokyo, Japan). The area of myocardial fibrosis was quantified using Image-Pro Plus 6.0 (Media Cybernetics, Rockville, MD, United States). The area fraction of myocardial fibrosis was expressed by the fractional area (percentage of total surface area) of the section occupied by collagen deposition. The mast cells in the myocardium were stained with toluidine blue in paraffin sections. Mast cells were counted in a high-power field of view (400× magnification). Mast cell density was quantified by counting the number of toluidine blue-positive mast cells in five consecutive fields.
Mitochondria Preparation
Mitochondria were isolated from the hearts of all groups with the same protocol as before (34). In brief, heart tissue was homogenized in ice-cold buffer containing 160 mmol/L KCl, 10 mmol/L EGTA (pH 7.4), and 0.5% fatty-acid-free bovine serum albumen. The homogenate was centrifuged at 1,000 g for 10 min at 2°C, and the supernatant was centrifuged at 8,000 g for 10 min at 2°C. The pellet was re-suspended in buffer containing 320 mmol/L sucrose and 10 mmol/L Tris–HCl (pH 7.4), and centrifuged at 8,000 g for 10 min at 2°C. Then we acquired the purified mitochondria. The protein concentration in samples was determined using the Lowry method. Each sample used 150 μl of protein solution (i.e., mitochondria). All experiments were repeated at least five times and done in triplicate.
Mitochondrial Permeability Transition Pore Opening Assay
Mitochondrial permeability transition pore (MPTP) opening was detected by assessing mitochondrial swelling, which was determined by a decrease in light absorbance at 520 nm (A520). A UV-2100 UV-Vis spectrophotometer (Unocal Instruments) was used to measure OD changes at 520 nm for 15 min. To induce mitochondrial swelling, CaCl2 (200 μM) was applied to microplates containing mitochondria. The effects of PUT (10 μM), SPD (10 μM), and SPM (10 μM) on MPTP opening were recorded.
Statistical Analysis
All values are expressed as the mean ± SD. Statistical comparisons were performed by one-way analysis of variance and followed by the Newman–Keuls test. Differences were considered significant when P < 0.05.
Results
Pressure Overload Increases Mast Cell Density in Cardiac Tissue
Compared with sham rats, the cardiac mast cell density was significantly increased in rats 4 or 8 weeks after AAC surgery, and this effect was reversed by treatment with CS, a mast cell stabilizer (Figure 1).
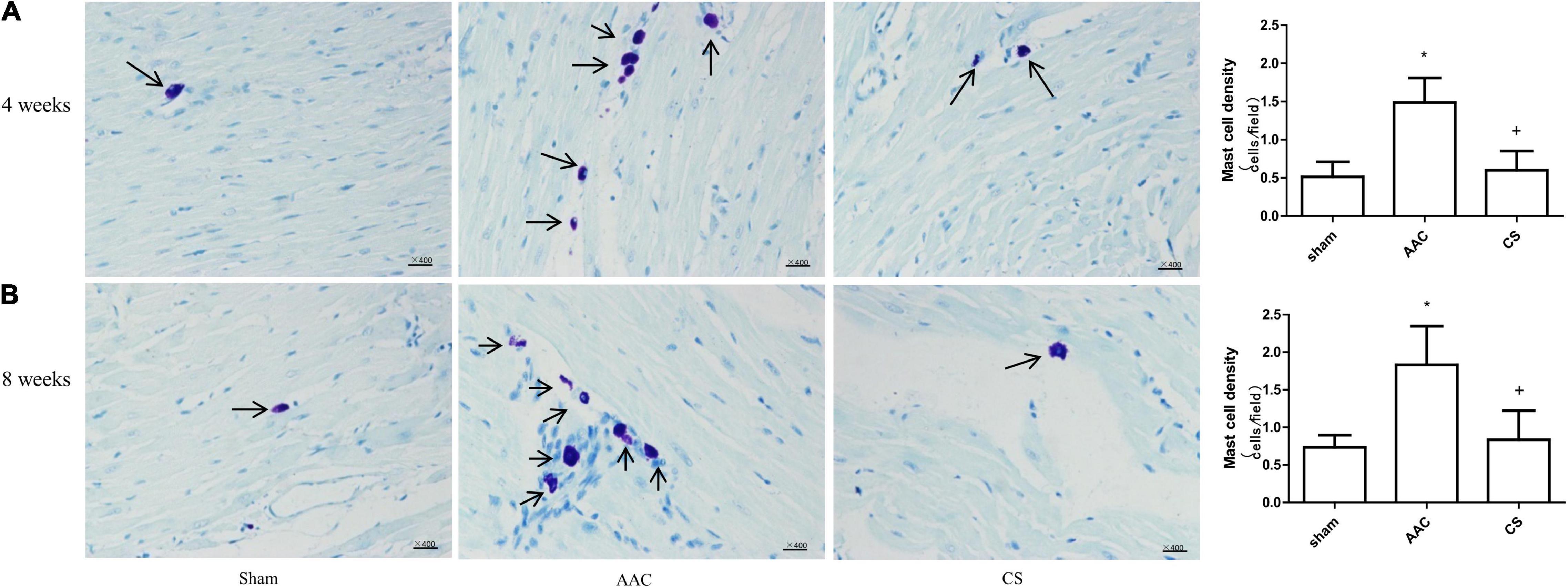
Figure 1. Mast cell density changes in cardiac tissue. (A,B) Mast cell density 4 weeks (A) and 8 weeks (B) after abdominal aortic constriction; mast cells indicated by arrows stained dark purple with toluidine blue (400× magnification). AAC, abdominal aortic constriction; CS, cromolyn sodium; n = 7 per group. *P < 0.05 vs sham group; +P < 0.05 vs AAC group; Scale bars, 100 μm.
The Increased Polyamine Content in Cardiac Tissue Induced by Abdominal Aortic Constriction Is Attenuated by Inhibition of Mast Cells
Compared with sham rats, 4 or 8 weeks after AAC surgery, the polyamine content of cardiac tissue increased significantly, and this increase was attenuated by inhibition of mast cells with its stabilizer, indicating that the increase of polyamines is dependent on mast cell activation (Figure 2).
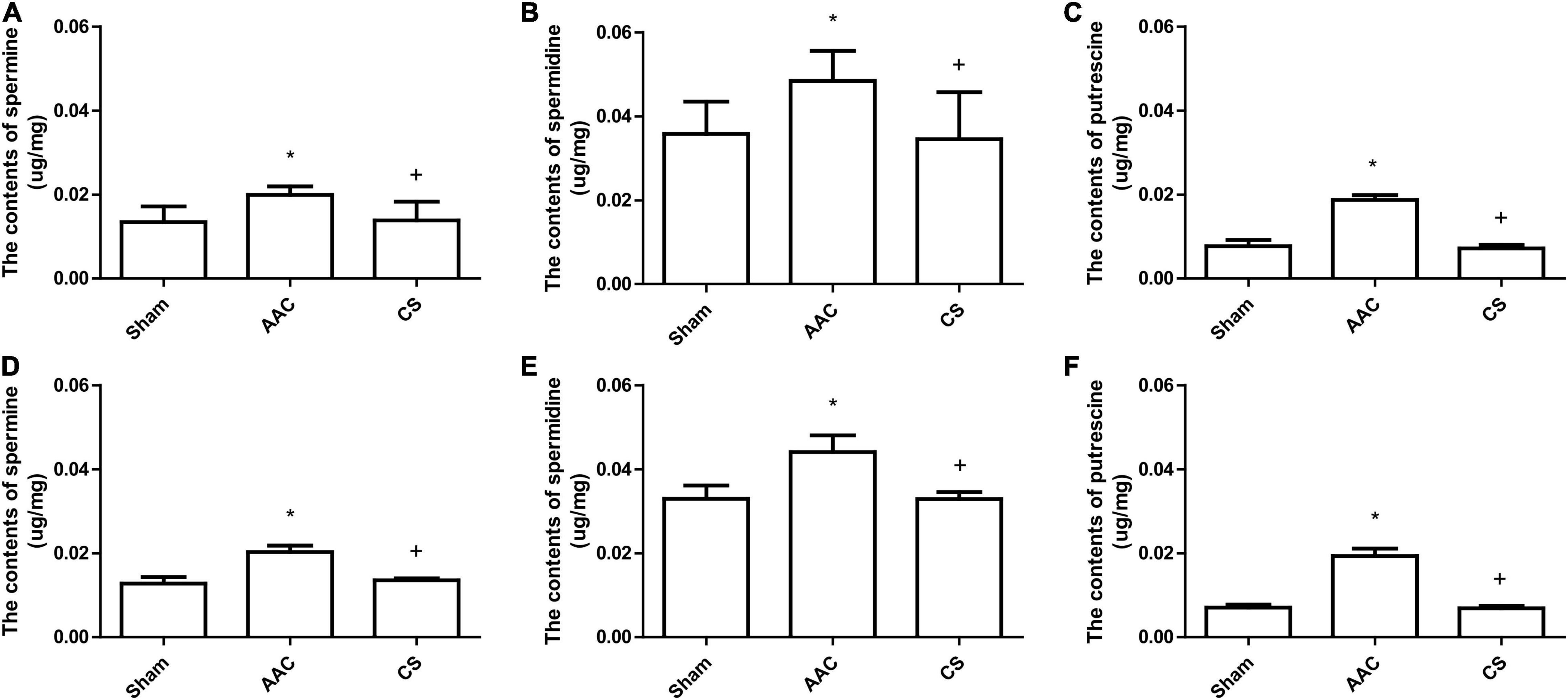
Figure 2. Polyamine content in cardiac tissue from rats 4 weeks (A–C) or 8 weeks (D–F) after AAC surgery. AAC, abdominal aortic constriction; CS, cromolyn sodium; *P < 0.05 vs sham group; +P < 0.05 vs AAC group. n = 9 per group.
Exogenous Administration of Polyamines Mimics the Cardiac Remodeling Induced by Pressure Overload
Compared with sham rats, the heart-weight to body-weight (HW/BW) ratio in rats with AAC increased significantly, and this was attenuated by administration of a mast cell stabilizer (Table 1). However, exogenous administration of polyamines for 4 or 8 weeks not only caused an increase of the HW/BW ratio similar to that in AAC rats, but also showed a significant increase of polyamine content in cardiac tissue (Figure 3).
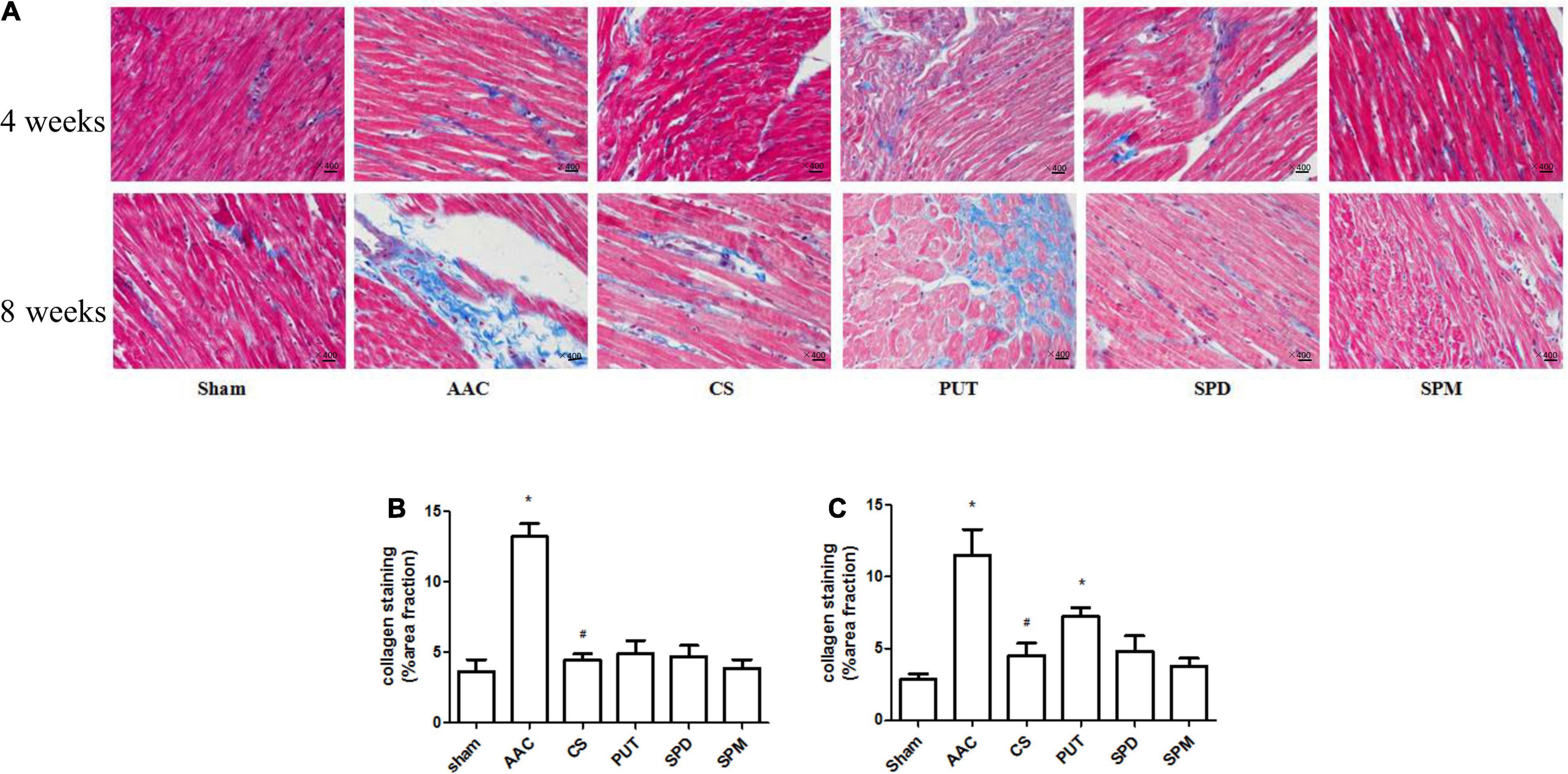
Figure 3. Collagen deposition in cardiac tissue. (A) Representative images of collagen staining (400× magnification). Heart sections stained with Masson’s trichrome; collagen is stained blue. AAC, abdominal aortic constriction; CS, cromolyn sodium; PUT, putrescine; SPM, spermine, SPD, spermidine. (B,C) Statistical analysis of collagen deposition after 4 weeks (B) and 8 weeks (C) of treatment with polyamines. *P < 0.05 vs sham group; #P < 0.05 vs AAC group. n = 8 per group. Scale bars, 100 μm.
Collagen deposition, a marker of interstitial fibrosis, was also significantly enhanced in cardiac tissue in AAC rats in comparison with that in sham rats, and this increase was attenuated by the mast cell stabilizer. Exogenous administration of PUT caused an increase of collagen deposition in cardiac tissue similar to that in AAC rats, but SPM and SPD did not (Figure 3).
Polyamines From Mast Cells Increase Cardiac Mitochondrial Permeability Transition Pore Opening
To assess the effects of polyamines on MPTP opening, we used the Ca2+-induced mitochondrial swelling method, and the results showed that opening of the MPTP was significantly increased in cardiac mitochondria from rats 4 or 8 weeks after AAC; however, this phenomenon was absent in cardiac mitochondria from rats treated with CS, the mast cell stabilizer (Figures 4A–D). To determine the role of polyamines in this process, we further assessed the effects of exogenous administration of polyamines on MPTP opening, and the results showed that the MPTP opening after continuous administration of PUT, SPM, or SPD was also significantly increased compared with sham rats (Figures 4E,F). Among the three polyamines, PUT made the greatest contribution to MPTP opening (Figures 4G,H).
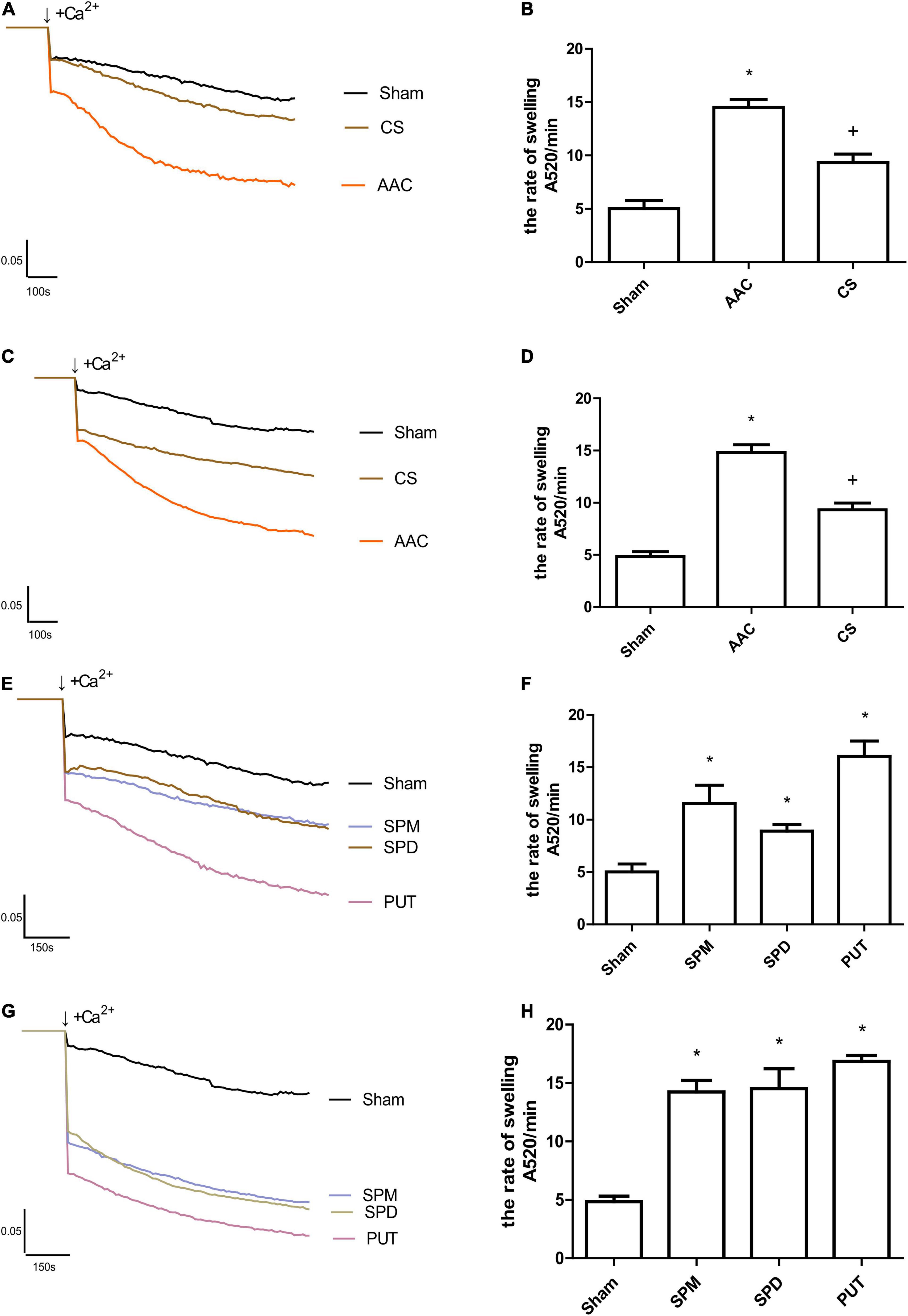
Figure 4. Changes in Ca2+-induced mitochondrial swelling rate. (A,B) Tracings of cardiac mitochondrial swelling (A), and statistics of swelling rates (B) 4 weeks after different treatments; (C,D) Tracings (C) and statistics (D) of cardiac mitochondrial swelling 8 weeks after different treatments; (E,F) Tracings (E) and statistics (F) of cardiac mitochondrial swelling 4 weeks after administration of polyamines; (G,H) Tracings (G) and statistics (H) of cardiac mitochondrial swelling 8 weeks after administration of polyamines. AAC, abdominal aortic constriction; CS, cromolyn sodium; PUT, putrescine; SPM, spermine; SPD, spermidine; n = 7 per group.; *P < 0.05 vs Sham group; +P < 0.05 vs AAC group.
Discussion
Our present results show that pressure overload can induce the recruitment of mast cells in cardiac tissue and the release of polyamines, which then participate in the process of myocardial remodeling by regulating opening of the MPTP.
In the present study, the recruitment of mast cells in cardiac tissue was demonstrated by the increased cardiac mast cell density 4 or 8 weeks after AAC in rats, and this was accompanied by increases in the HW/BW ratio and cardiac fibrosis. These results are similar to those in other reports (28, 35, 36). Interestingly, in our study, these effects were attenuated by inhibition of mast cell activation, suggesting that the activation and degranulation of mast cells are involved in the cardiac remodeling induced by pressure overload.
Although the activation of mast cells releases a variety of components including histamine, cytokines, phospholipase, proteases, and fatty-acid metabolites (37), we found an increase of polyamines in cardiac tissue after pressure overload. To confirm that these polyamines were released from mast cells, we inhibited mast cells using a stabilizer, and the results showed that the increase of polyamines was significantly attenuated, indicating that the increased polyamine content was from cardiac mast cells.
Research data has shown that the myocardial polyamine content is increased in hypertensive patients (21, 22), and that polyamines might be involved in the cardiac hypertrophy induced by norepinephrine and its receptor system (23), as well as being involved in fibrosis (24). To further investigate whether the increased polyamines participate in the cardiac remodeling induced by pressure overload, we assessed the effects of exogenous polyamines on heart remodeling, and the results showed that after 4 weeks of continuous administration of PUT, SPM, or SPD, not only the polyamine content in cardiac tissue but also the HW/BW ratio increased significantly, and after 8 weeks of continuous administration of PUT, myocardial fibrosis and the number of myocardial mast cells increased as much as that in rats with pressure overload. In order to further investigate whether inhibiting the release of polyamines from mast cells can decrease the myocardial fibrosis, we inhibited degranulation of mast cells by using mast cell stabilizers. The results showed a great decrease of polyamines, which was associated with a decrease of myocardial fibrosis. All the above experimental results indicated that polyamines from mast cells are involved in the cardiac remodeling induced by pressure overload.
Studies have shown that MPTP opening is involved in the process of cardiac remodeling: in mice with knockout of an MPTP opening regulatory protein, the MPTP opens more easily, and this is accompanied by cardiac hypertrophy and fibrosis (25). In mice with gene knockout of cyclophilin D, an MPTP regulatory protein, more severe stress overload-induced cardiac hypertrophy and increases in fibrosis also occur (26, 27). These findings suggested that abnormal opening of the MPTP is closely associated with cardiac hypertrophy and fibrosis. However, these experimental data were all collected under conditions in which the MPTP opening was artificially altered. In the situation of pressure overload, whether the cardiac MPTP is in an abnormal open state, and particularly whether cardiac mast cells participate in the regulation of MPTP opening is still unclear. We found that opening of the cardiac MPTP in AAC rats increased significantly, and inhibition of mast cells attenuated the MPTP opening, suggesting that mast cell recruitment and activation can induce opening of the MPTP. Since we detected a significant increase in the polyamines released from cardiac mast cells after pressure overload, we further investigated whether polyamines released by mast cells participate in regulating the MPTP. We did experiments to measure the effects of PUT, SPD, and SPM on MPTP opening in mitochondria from normal rats, and the results showed that these polyamines significantly enhanced opening of the MPTP, indicating that polyamines from mast cells mediate myocardial remodeling through increasing abnormal opening of the MPTP. Although we did not further investigate the possible underlying mechanisms of polyamine-induced MPTP opening, according to the published reports, the corresponding underlying mechanisms may be complex: endoplasmic reticulum stress, oxidative stress, intracellular Ca2+ homeostasis, and even autophagy may all participate in the regulation of MPTP opening (38–45). Thus the exact mechanisms need to be further examined.
Activation of mast cells also releases other substances, mainly 5-HT, chymase, and histamine, and whether these substances participate in the abnormal opening of the cardiac MPTP need to be further examined.
Although we did not perform cardiac function tests, in another study on myocardial remodeling (the results have not yet been published), our group examined the cardiac function of rats with AAC, and found that 8 weeks after surgery, the cardiac function did not decline, although fibrosis had occurred.
In summary, although we cannot exclude the possibility that other substances from mast cells also participate in the regulation of cardiac MPTP opening, our results do suggest that polyamines derived from cardiac mast cells are involved in the adverse ventricular remodeling induced by pressure overload via increasing cardiac MPTP opening.
Data Availability Statement
The original contributions presented in the study are included in the article/supplementary material, further inquiries can be directed to the corresponding author.
Ethics Statement
The animal study was reviewed and approved by the Institutional Animal Care and Use Committee of China Three Gorges University.
Author Contributions
XX, XJ, and CX collected and analyzed the data. JL helped to do some measurements. XX and SZ wrote the manuscript. All authors contributed to the article and approved the submitted version.
Funding
This study was supported by the Natural Science Foundation in Hubei (2015CFB288) and the National Natural Science Foundation of China (30872716 and 81873499).
Conflict of Interest
The authors declare that the research was conducted in the absence of any commercial or financial relationships that could be construed as a potential conflict of interest.
Publisher’s Note
All claims expressed in this article are solely those of the authors and do not necessarily represent those of their affiliated organizations, or those of the publisher, the editors and the reviewers. Any product that may be evaluated in this article, or claim that may be made by its manufacturer, is not guaranteed or endorsed by the publisher.
References
1. Levick SP, Melendez GC, Plante E, McLarty JL, Brower GL, Janicki JS. Cardiac mast cells: the centrepiece in adverse myocardial remodelling. Cardiovasc Res. (2011) 89:12–9. doi: 10.1093/cvr/cvq272
2. Janicki JS, Spinale FG, Levick SP. Gender differences in non-ischemic myocardial remodeling: are they due to estrogen modulation of cardiac mast cells and/or membrane type 1 matrix metalloproteinase. Pflugers Arch. (2013) 465:687–97. doi: 10.1007/s00424-013-1229-9
3. Murray DB, Gardner JD, Brower GL, Janicki JS. Endothelin-1 mediates cardiac mast cell degranulation, matrix metalloproteinase activation, and myocardial remodeling in rats. Am J Physiol Heart Circ Physiol. (2004) 287:H2295–9. doi: 10.1152/ajpheart.00048.2004
4. Palaniyandi SS, Inagaki K, Mochly-Rosen D. Mast cells and epsilonPKC: a role in cardiac remodeling in hypertension-induced heart failure. J Mol Cell Cardiol. (2008) 45:779–86. doi: 10.1016/j.yjmcc.2008.08.009
5. Li J, Jubair S, Janicki JS. Estrogen inhibits mast cell chymase release to prevent pressure overload-induced adverse cardiac remodeling. Hypertension. (2015) 65:328–34. doi: 10.1161/HYPERTENSIONAHA.114.04238
6. Brower GL, Janicki JS. Pharmacologic inhibition of mast cell degranulation prevents left ventricular remodeling induced by chronic volume overload in rats. J Card Fail. (2005) 11:548–56. doi: 10.1016/j.cardfail.2005.05.005
7. Kennedy RH, Hauer-Jensen M, Joseph J. Cardiac function in hearts isolated from a rat model deficient in mast cells. Am J Physiol Heart Circ Physiol. (2005) 288:H632–7. doi: 10.1152/ajpheart.00803.2004
8. Theoharides TC, Kempuraj D, Tagen M, Conti P, Kalogeromitros D. Differential release of mast cell mediators and the pathogenesis of inflammation. Immunol Rev. (2007) 217:65–78. doi: 10.1111/j.1600-065X.2007.00519.x
9. Kanagaratham C, El AY, Sallis BF, Hollister BA, Lewis OL, Minnicozzi SC, et al. Omeprazole inhibits IgE-mediated mast cell activation and allergic inflammation induced by ingested allergen in mice. J Allergy Clin Immunol. (2020) 146:884–93. doi: 10.1016/j.jaci.2020.02.032
10. Jensen BM, Bartko EA, Baumann K, Skov PS. Measuring histamine and cytokine release from basophils and mast cells. Methods Mol Biol. (2020) 2163:247–62. doi: 10.1007/978-1-0716-0696-4_21
11. Stewart JJ, Wei CC, Brower GL, Rynders PE, Hankes GH, Dillon AR, et al. Cardiac mast cell- and chymase-mediated matrix metalloproteinase activity and left ventricular remodeling in mitral regurgitation in the dog. J Mol Cell Cardiol. (2003) 35:311–9. doi: 10.1016/s0022-2828(03)00013-0
12. de Almeida A, Mustin D, Forman MF, Brower GL, Janicki JS, Carver W. Effects of mast cells on the behavior of isolated heart fibroblasts: modulation of collagen remodeling and gene expression. J Cell Physiol. (2002) 191:51–9. doi: 10.1002/jcp.10071
13. Garcia-Faroldi G, Rodriguez CE, Urdiales JL, Perez-Pomares JM, Davila JC, Pejler G, et al. Polyamines are present in mast cell secretory granules and are important for granule homeostasis. PLoS One. (2010) 5:e15071. doi: 10.1371/journal.pone.0015071
14. Ibrahim J, Hughes AD, Schachter M, Sever PS. Enhanced tissue polyamine content in the spontaneously hypertensive rat. Clin Exp Pharmacol Physiol. (1996) 23:410–4. doi: 10.1111/j.1440-1681.1996.tb02750.x
15. Ruskoaho H, Raunio H. Altered cardiac polyamine biosynthesis in spontaneously hypertensive rats. Am J Physiol. (1987) 253(2 Pt 2):H262–9. doi: 10.1152/ajpheart.1987.253.2.H262
16. Meana C, Rubin JM, Bordallo C, Suarez L, Bordallo J, Sanchez M. Correlation between endogenous polyamines in human cardiac tissues and clinical parameters in patients with heart failure. J Cell Mol Med. (2016) 20:302–12. doi: 10.1111/jcmm.12674
17. Yang Y, Ma L, Song M, Li X, He F, Wang C, et al. The role of the complement factor B-arginase-polyamine molecular axis in uremia-induced cardiac remodeling in mice. Eur J Immunol. (2020) 50:220–33. doi: 10.1002/eji.201948227
18. Igarashi K, Kashiwagi K. The functional role of polyamines in eukaryotic cells. Int J Biochem Cell Biol. (2019) 107:104–15. doi: 10.1016/j.biocel.2018.12.012
19. Zhang H, Simon AK. Polyamines reverse immune senescence via the translational control of autophagy. Autophagy. (2020) 16:181–2. doi: 10.1080/15548627.2019.1687967
20. Bae DH, Lane D, Jansson PJ, Richardson DR. The old and new biochemistry of polyamines. Biochim Biophys Acta Gen Subj. (2018) 1862:2053–68. doi: 10.1016/j.bbagen.2018.06.004
21. Tipnis UR, Li S. Hypertension in normotensive and hypertensive rats by spermine ingestion. Cytobios. (2001) 106(Suppl. 1):85–98.
22. Soltis EE, Newman PS, Olson JW. Polyamines, vascular smooth muscle, and deoxycorticosterone acetate-salt hypertension. Hypertension. (1991) 18:85–92. doi: 10.1161/01.hyp.18.1.85
23. Lin Y, Liu JC, Zhang XJ, Li GW, Wang LN, Xi YH, et al. Downregulation of the ornithine decarboxylase/polyamine system inhibits angiotensin-induced hypertrophy of cardiomyocytes through the NO/cGMP-dependent protein kinase type-I pathway. Cell Physiol Biochem. (2010) 25:443–50. doi: 10.1159/000303049
24. Grasemann H, Shehnaz D, Enomoto M, Leadley M, Belik J, Ratjen F. L-ornithine derived polyamines in cystic fibrosis airways. PLoS One. (2012) 7:e46618. doi: 10.1371/journal.pone.0046618
25. Hafner AV, Dai J, Gomes AP, Xiao CY, Palmeira CM, Rosenzweig A, et al. Regulation of the mPTP by SIRT3-mediated deacetylation of CypD at lysine 166 suppresses age-related cardiac hypertrophy. Aging (Albany NY). (2010) 2:914–23. doi: 10.18632/aging.100252
26. Elrod JW, Wong R, Mishra S, Vagnozzi RJ, Sakthievel B, Goonasekera SA, et al. Cyclophilin D controls mitochondrial pore-dependent Ca(2+) exchange, metabolic flexibility, and propensity for heart failure in mice. J Clin Invest. (2010) 120:3680–7. doi: 10.1172/JCI43171
27. Giorgio V, Soriano ME, Basso E, Bisetto E, Lippe G, Forte MA, et al. Cyclophilin D in mitochondrial pathophysiology. Biochim Biophys Acta. (2010) 1797:1113–8. doi: 10.1016/j.bbabio.2009.12.006
28. Liu JJ, Huang N, Lu Y, Zhao M, Yu XJ, Yang Y, et al. Improving vagal activity ameliorates cardiac fibrosis induced by angiotensin II: in vivo and in vitro. Sci Rep. (2015) 5:17108. doi: 10.1038/srep17108
29. Liu DZ, Chen ZG, Ge M, Gan XL, Hei ZQ. [Effects of cromolyn sodium on intestinal ischemia-reperfusion injury by inhibiting PAR-2 expression in rats]. Zhonghua Yi Xue Za Zhi. (2012) 92:2597–600.
30. Kim GD, Kim TH, Park YS, Ahn HJ, Cho JJ, Park CS. Immune response against 2,4-dinitrofluorobenzene-induced atopic dermatitis-like clinical manifestation is suppressed by spermidine in NC/Nga mice. Scand J Immunol. (2015) 81:221–8. doi: 10.1111/sji.12274
31. Kaouass M, Sulon J, Deloyer P, Dandrifosse G. Spermine-induced precocious intestinal maturation in suckling rats: possible involvement of glucocorticoids. J Endocrinol. (1994) 141:279–83. doi: 10.1677/joe.0.1410279
32. Til HP, Falke HE, Prinsen MK, Willems MI. Acute and subacute toxicity of tyramine, spermidine, spermine, putrescine and cadaverine in rats. Food Chem Toxicol. (1997) 35:337–48. doi: 10.1016/s0278-6915(97)00121-x
33. Zhang Y, Zhang L, Fan X, Yang W, Yu B, Kou J, et al. Captopril attenuates TAC-induced heart failure via inhibiting Wnt3a/beta-catenin and Jak2/Stat3 pathways. Biomed Pharmacother. (2019) 113:108780. doi: 10.1016/j.biopha.2019.108780
34. Cao Y, Zhang SZ, Zhao SQ, Bruce IC. The mitochondrial Ca(2+)-activated K(+) channel contributes to cardioprotection by limb remote ischemic preconditioning in rat. Life Sci. (2011) 88:1026–30. doi: 10.1016/j.lfs.2011.03.011
35. Hara M, Ono K, Hwang MW, Iwasaki A, Okada M, Nakatani K, et al. Evidence for a role of mast cells in the evolution to congestive heart failure. J Exp Med. (2002) 195:375–81. doi: 10.1084/jem.20002036
36. Li J, Jubair S, Levick SP, Janicki JS. The autocrine role of tryptase in pressure overload-induced mast cell activation, chymase release and cardiac fibrosis. IJC Metab Endocr. (2016) 10:16–23. doi: 10.1016/j.ijcme.2015.11.003
37. Galli SJ, Gaudenzio N, Tsai M. Mast cells in inflammation and disease: recent progress and ongoing concerns. Annu Rev Immunol. (2020) 38:49–77. doi: 10.1146/annurev-immunol-071719-094903
38. Hunter DR, Haworth RA. The Ca2+-induced membrane transition in mitochondria. I. The protective mechanisms. Arch Biochem Biophys. (1979) 195:453–9. doi: 10.1016/0003-9861(79)90371-0
39. Wang Y, Wang Y, Li F, Zhang X, Li H, Yang G, et al. Spermine protects cardiomyocytes from high glucose-induced energy disturbance by targeting the CaSR-gp78-Ubiquitin proteasome system. Cardiovasc Drugs Ther. (2021) 35:73–85. doi: 10.1007/s10557-020-07064-z
40. He Y, Yang J, Li H, Shao H, Wei C, Wang Y, et al. Exogenous spermine ameliorates high glucose-induced cardiomyocytic apoptosis via decreasing reactive oxygen species accumulation through inhibiting p38/JNK and JAK2 pathways. Int J Clin Exp Pathol. (2015) 8:15537–49.
41. Wei C, Wang Y, Li M, Li H, Lu X, Shao H, et al. Spermine inhibits endoplasmic reticulum stress-induced apoptosis: a new strategy to prevent cardiomyocyte apoptosis. Cell Physiol Biochem. (2016) 38:531–44. doi: 10.1159/000438648
42. Wei C, Li H, Wang Y, Peng X, Shao H, Li H, et al. Exogenous spermine inhibits hypoxia/ischemia-induced myocardial apoptosis via regulation of mitochondrial permeability transition pore and associated pathways. Exp Biol Med (Maywood). (2016) 241:1505–15. doi: 10.1177/1535370216643417
43. Hu J, Lu X, Zhang X, Shao X, Wang Y, Chen J, et al. Exogenous spermine attenuates myocardial fibrosis in diabetic cardiomyopathy by inhibiting endoplasmic reticulum stress and the canonical Wnt signaling pathway. Cell Biol Int. (2020) 44:1660–70. doi: 10.1002/cbin.11360
44. Eisenberg T, Abdellatif M, Schroeder S, Primessnig U, Stekovic S, Pendl T, et al. Cardioprotection and lifespan extension by the natural polyamine spermidine. Nat Med. (2016) 22:1428–38. doi: 10.1038/nm.4222
Keywords: pressure overload, cardiac remodeling, mast cell, polyamine, MPTP
Citation: Xiong X, Li J, Zhang S, Jia X and Xiao C (2022) Involvement of Polyamines From Cardiac Mast Cells in Myocardial Remodeling Induced by Pressure Overload Through Mitochondrial Permeability Transition Pore Opening. Front. Cardiovasc. Med. 9:850688. doi: 10.3389/fcvm.2022.850688
Received: 08 January 2022; Accepted: 03 March 2022;
Published: 11 April 2022.
Edited by:
David Della-Morte, University of Miami, United StatesReviewed by:
Sarfaraz Ahmad, Wake Forest University, United StatesJun Ren, University of Washington, United States
Copyright © 2022 Xiong, Li, Zhang, Jia and Xiao. This is an open-access article distributed under the terms of the Creative Commons Attribution License (CC BY). The use, distribution or reproduction in other forums is permitted, provided the original author(s) and the copyright owner(s) are credited and that the original publication in this journal is cited, in accordance with accepted academic practice. No use, distribution or reproduction is permitted which does not comply with these terms.
*Correspondence: Shizhong Zhang, zhangsz@ctgu.edu.cn