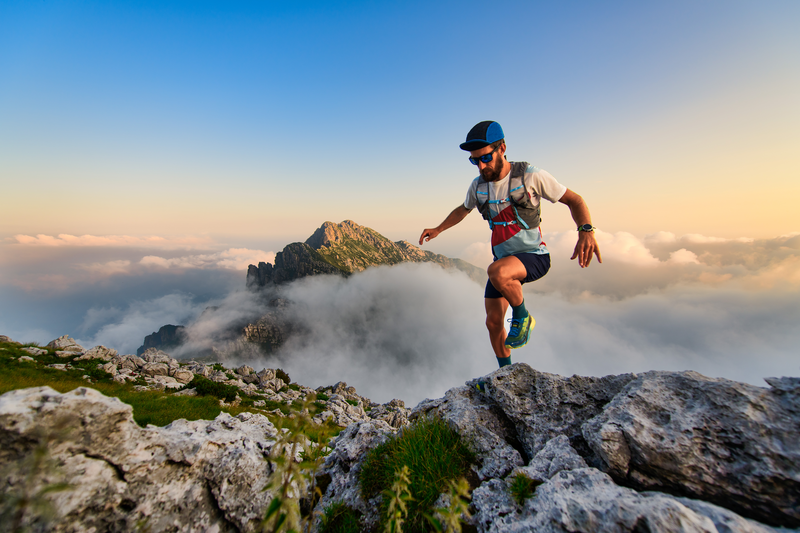
94% of researchers rate our articles as excellent or good
Learn more about the work of our research integrity team to safeguard the quality of each article we publish.
Find out more
REVIEW article
Front. Cardiovasc. Med. , 24 March 2022
Sec. Heart Valve Disease
Volume 9 - 2022 | https://doi.org/10.3389/fcvm.2022.849540
This article is part of the Research Topic Advances in the Imaging and Treatment of Valvular Heart Disease View all 22 articles
The incidence and prevalence of valvular heart disease (VHD) is increasing and has been described as the next cardiac epidemic. Advances in imaging and therapeutics have revolutionized how we assess and treat patients with VHD. Although echocardiography continues to be the first-line imaging modality to assess the severity and the effects of VHD, advances in cardiac computed tomography (CT) now provide novel insights into VHD. Transcatheter valvular interventions rely heavily on CT guidance for procedural planning, predicting and detecting complications, and monitoring prosthesis. This review focuses on the current role and future prospects of CT in the assessment of aortic and mitral valves for transcatheter interventions, prosthetic valve complications such as thrombosis and endocarditis, and assessment of the myocardium.
Interest in valvular heart disease (VHD) has been invigorated with the advancement in new imaging modalities and pathological insights, and most importantly the advent of transcatheter valve interventions. Transcatheter aortic valve replacement (TAVR) has now overtaken surgical aortic valve replacement (SAVR) in volume in Germany (and the United States) (1) and is driving innovation in transcatheter interventions on other valves (2). Cardiac computed tomography (CT) has become an essential tool for the heart valve team to supplement the assessment by echocardiography, and decision making for suitability and mode of intervention. Technical developments in CT technology have made this possible by providing high temporal, spatial and contrast resolution for imaging one of the most challenging imaging targets of the body. The use of CT is now recommended in guidelines for the pre-procedural work-up for TAVR (3) and is an important tool for the diagnosis of valvular thrombosis (4) and infective endocarditis (5). This has led to its widespread use in the assessment and management of patients with VHD providing additional novel insights into remodeling, pathophysiology, and prognosis. This review article explores its current role, limitations and future prospects in the assessment and management of patients with VHD. It does not cover the technical aspects of CT data acquisition and reconstruction, which can be found elsewhere (3, 4, 6).
Aortic stenosis (AS) is the commonest type of VHD in the developed world (7). Treatment using either SAVR or TAVR is considered for severe AS (8). Determining severity is largely done using echocardiography, with aortic valve area (AVA) being the most commonly used marker of severity. It is calculated using the continuity equation with the incorrect assumption of a circular left ventricular outflow tract (LVOT) (9). Although using a CT derived LVOT area for the continuity equation is more accurate than a 2D echo-based LVOT area, this has not translated into better diagnostic performance (correlation with transvalvular gradients) or mortality prediction (10). However, discordant echocardiographic parameters (discordant AVA and gradient) occur in up to a third of patients, making the quantification of AS severity difficult (11, 12). CT has an important role in determining severity among these patients, especially those with paradoxical low-flow, low-gradient AS (8, 13). Calcification is the cornerstone underlying the pathophysiology of AS in most patients. A sequence of pathological changes involving lipid infiltration of the valve, inflammation, fibrosis and mineralization, leads to AS (14). Using a non-contrast CT, calcification is identified as areas of increased radio-opacity. The commonly used Agatston score method defines calcification where the density is greater than 130 Hounsfield units (HU) (15). CT derived aortic valve calcium score (AVCS) demonstrates high inter- and intra-observer reproducibility (16), correlates well with the severity of AS determined by echocardiography (17, 18) and calcium weight on explanted valves (16), thus making it a very useful marker of AS severity. AVCS is also prognostically important (13, 19) and determines progression of AS, with higher AVCS at baseline correlating with faster progression of AS (20). Compared to men, women have less calcification, but more fibrosis for the same severity of stenosis (21), leading to different recommended thresholds for the definition of severe AS; 1,200 Agatston units (AU) in women and ∼2,000AU in men (22). However, these thresholds may not be applicable in patients with bicuspid AS or rheumatic valve disease due to differences in pathophysiological mechanisms (12).
An alternative method utilizes planimetry of the orifice during systole. This anatomical, rather than functional measurement, correlates poorly with other measurements of AVA and with transvalvular gradients (10). Consequently this is seldom used clinically.
Outcomes in patients with moderate AS are known to be poor, especially if systolic function is compromised (23, 24). An ongoing trial is evaluating whether TAVR has a role in such patients (25). CT may play a role in the future for identifying patients for intervention with less than severe valvular disease as calcification has been shown to correlate with the rate of progression and mortality in patients with less than severe AS (26).
The utility of TAVR has seen a dramatic increase over the last decade, with CT being routinely used to facilitate its use, improve efficacy and reduce complications.
Cardiac computed tomography angiography of the aorta and peripheral vasculature provides a quick and complete dataset for TAVR planning (Figure 1). In addition to illustrating the dimensions of the aortic annulus and root, and degree and distribution of aortic valve calcification, CT can demonstrate the degree of iliac vessel wall calcification, tortuosity, ilio-femoral stenosis, presence of aorto-iliac aneurysms, foci of dissection, large penetrating ulcers, and potentially thrombi, as well as previous vascular procedures with grafting/stent implantation—useful considerations for procedural planning (27–29). When a transfemoral access site is unfavorable, CT can provide valuable information regarding alternative sites, such as subclavian, carotid, apical, trans-aortic, and trans-caval (crossing from the inferior vena cava into the abdominal aorta and using a closure device to plug the aortic wall after implantation of the valve) (30). Trans-caval access is increasingly being used and greatly benefits from pre-procedural planning using CT. Using electrocautery, a puncture is made from the inferior vena cava (IVC) to the adjacent descending aorta between the aortic bifurcation and renal arteries. A calcium-free window on the aorta adjacent to the IVC needs to be located using CT and defined by the vertebral level. Additional measurements such as the distance between aorta and IVC, lumen diameters and identification of bail-out access (in case endograft therapy is required) are useful and can be performed using CT (31, 32).
Figure 1. Peripheral access planning for TAVR, requires assessment of the size, tortuosity, calcification (both severity and distribution) and any prosthetic material such as stents or pathologies such as aneurysms. (A) Multiplanar reconstruction of the vascular tree, (B) Sagittal view, (C) Axial view.
First, using multiplanar reconstructions (MPR), CT can be used to determine the optimum fluoroscopic projection for valve implantation-orthogonal to the aortic valve (29). This has been shown to reduce additional aortograms, procedural time and contrast use (33). Second, CT provides an accurate guide for sizing an aortic bioprosthesis based on aortic valve (AV) annular dimensions, with a resulting reduction in post-TAVR aortic regurgitation (34, 35). Annulus diameters, area and perimeter are typically used to derive the most appropriate transcatheter valve diameter, applying recommendations provided in manufacturers’ charts. Third, additional measurements are typically taken at levels of the sinus of Valsalva, sino-tubular junction, ascending aorta and the heights of the coronary ostia from the AV annulus- guiding the procedure and enabling the prediction of complications (Figure 2). Low coronary ostial heights and narrow sinuses of Valsalva are associated with a higher risk of coronary obstruction and difficulty in coronary artery engagement for angiography or intervention (36, 37). Correct valve sizing to prevent oversizing is essential to prevent annular rupture, which often results in fatal outcomes (38).
Figure 2. Measurements of the aortic root and ascending aorta. (A) aortic valve (AV) annulus, (B) sinus of Valsalva, (C) sino-tubular junction, (D) ascending aorta, (E) left coronary ostial height from AV annulus, (F) right coronary ostial height.
Aortic valve calcification is important to ensure the anchorage of the bioprosthesis and prevent valve migration (aortic root dilatation and the lack of calcification commonly preclude the use of TAVR for aortic regurgitation) (39). However, both an increased burden and bulky eccentric calcification can result in inadequate valve apposition, leading to paravalvular regurgitation, which is poorly tolerated post-TAVR and associated with poor outcomes (40–42).
Conduction abnormalities and permanent pacemaker implantation rates remain high (43). Pacing in TAVR patients is associated with less recovery of left ventricular ejection fraction and a higher rate of heart failure hospitalization (44). Therefore attempts to avoid conduction abnormalities and subsequently pacemaker implantation are important. Device landing zone calcification can predict post-TAVR pacemaker requirement, especially if calcification is located around the LVOT, the basal septum (45, 46) or the mitral annulus (47). Conduction abnormalities can also arise due to a short membranous septum. The bundle of His runs close to the membranous septum and is susceptible to compression by the implanted bioprosthesis. Membranous septal depth is measured from the AV annulus to the start of the muscular interventricular septum. Depths of <7.8 mm are predictive of high degree atrioventricular block (46).
Calcification in the LVOT, especially below the non-coronary cusp, is associated with annular rupture (area under the ROC curve 0.81), a complication that often leads to death (48). Other factors associated with annular rupture include device oversizing and post-dilatation (38).
The height of the coronary ostia from the aortic annulus is an important parameter to measure as short heights can result in coronary obstruction from the newly implanted prosthesis (29). Coronary ostial heights are considered low if <12 mm. The sinuses of Valsalva that house the coronary ostia are also important when considering coronary occlusion. A mean diameter <30 mm is associated with increased risk as the space between the bioprosthetic valve and coronary ostia is reduced (3, 37). However, these cut-offs have low specificity and are not prohibitive for a TAVR. Additionally, CT allows evaluation of the extent and severity of coronary artery disease, which dictates the need for further assessment and management (3, 49).
The mitral valve, annulus and associated apparatus form a complex 3D structure. Although echocardiography remains the primary imaging modality for mitral assessment, CT can highlight valve pathology, provide clues to its etiology and importantly, assist in planning for valve repair/replacement.
Mitral valve prolapse is a common cause of primary MR, and CT can reliably detect this (50). In these cases, two- and three-chamber views can be used to identify leaflet thickening (>5 mm) and a flail leaflet, both seen in the context of mitral prolapse. Using retrospective ECG gating, multiple phases of the cardiac cycle can be reconstructed, facilitating moving cine images, which is important in recognizing prolapse.
In patients with secondary MR, evaluation of the leaflets, ventricle and coronary arteries will enable both the diagnosis and etiology of the MR. In a study of 151 patients with heart failure and functional mitral regurgitation (FMR), CT was able to identify that those with moderate to severe FMR had significantly increased posterior leaflet angles and mitral valve tenting heights at central and postero-medial levels. These were described as the strongest determinants of FMR severity (51). CT can provide accurate left ventricular dimensions enabling an understanding of left ventricular dilatation (52). Other cardiomyopathies can also result in MR. Systolic anterior motion of the mitral valve can lead to MR and has been described with hypertrophic cardiomyopathy and cardiac amyloidosis.
Cardiac computed tomography may also play a role in quantifying MR. When measuring regurgitant volumes in 49 patients with isolated MR, the severity of regurgitation correlated well with echocardiography findings (53). This can be done by calculating total stroke volume of the left and right ventricles (end-diastolic volume minus end-systolic volume), with the regurgitant volume being the difference between the stroke volumes of the left and right ventricle. However, this is rarely used clinically, but could have a role in patients with poor echo windows and if cardiac magnetic resonance imaging is contraindicated.
Echocardiography remains the gold standard for the diagnosis and grading the severity of mitral stenosis (MS). However, CT can confirm the presence of related features such as left atrial enlargement, as well as certain appearances, which point to specific causes of mitral stenosis, such as thickening of the mitral valve leaflets with commissural fusion and calcification, commonly seen in rheumatic mitral stenosis (so-called fish mouth appearance) (54).
Transcatheter mitral valve interventions include technology for both repair and replacement, each requiring different approaches and techniques. An in-depth review of the various technologies available can be found here (55). Once a decision to intervene has been made, CT plays a vital role in procedural planning.
Sizing the annulus is important for selecting a transcatheter mitral valve prosthesis. The mitral annulus is saddle-shaped. However, for the purposes of certain transcatheter prostheses, a D-shaped annulus can be assumed; the medial and lateral fibrous trigones are connected via a virtual straight line, and the diameter and area then calculated by “tracing” its perimeter border (56). 3D software packages are then able to recreate the annulus, allowing further measurements to be made. Specifically, the landing zone is an important consideration when choosing a transcatheter MV prosthesis; each prosthesis has a different anchoring mechanism and requires certain anatomical characteristics. For this reason, leaflet length, chordal anatomy, the presence of a myocardial shelf and left ventricular cavity dimensions need to be assessed on CT (57).
The mitral valve has an anterior and a posterior leaflet, both of which have three scallops. These are identifiable via CT, and seen in both reconstructed short-axis and long-axis views. Although echocardiography remains the primary imaging modality to evaluate the mitral leaflets, numerous geometric measurements can also be estimated from CT, including leaflet length, area, tenting height, and coaptation angle. These measurements are important for understanding the mechanism of MR and guiding intervention. Indeed, comparisons of three-dimensional (3D) transesophageal echocardiography and cardiac CT have shown that both imaging modalities provide good detailing of mitral leaflet morphology (58). In addition, calcification and clefts of the leaflets are important to note as these can preclude adequate transcatheter edge to edge repair (TEER) (59).
Left ventricular outflow tract (LVOT) obstruction is a known complication of TMVR carrying a significant risk of mortality. A new LVOT (termed the “neo-LVOT”) is formed from the interventricular septum anteriorly and the native anterior mitral valve leaflet. Pre-procedural CT planning simulating a neo-LVOT can help predict the risk of LVOT obstruction (60). Extrapolation from hypertrophic cardiomyopathy studies initially identified a LVOT area of 2 cm2 as a safe cut-off for TMVR (61). Further studies specific to TMVR suggested a neo-LVOT < 1.7 cm2 at end-systole as high risk (60), but more recent evidence has suggested that even smaller areas are safe (62).
Several factors predict LVOT obstruction; device related, remodeling related and native anatomical factors. Of these, the aorto-mitral angulation is important and can be calculated using CT. Defined as the angle between the mitral annular trajectory and LVOT long axis; the smaller the angle, the lower the risk of LVOT obstruction. Coupled with this, the size of the mitral annulus, annulus-to-interventricular septal distance and LVOT and septal shape should also be taken into consideration (57, 60).
Mitral annular calcification (MAC) has a reported incidence of between 10 and 42% (63, 64) and in patients with co-existent aortic stenosis is found in 50% of patients (47). MAC can be easily identified and its distribution mapped out using CT. MAC increases the technical complexity of surgical intervention, specifically increasing the risk of AV groove disruption, paravalvular leak and increasing pump and clamp times (65, 66). It also increases the risk for percutaneous intervention; performing TMVR in patients with MAC carries substantially more risk of LVOT obstruction than performing it in valve-in-valve or valve-in-ring (60). MAC can make it challenging to recognize the boundary between annulus and blood pool and therefore accurately measure annular dimensions.
Mitral annular calcification itself can serve as a bed on which the new valve can anchor. In procedures involving the implantation of a TAVR prosthesis in the mitral valve position, non-circumferential or thin MAC can result in poor device sealing (67). MAC can also make it difficult to determine the correct position for valve deployment, with 17% of valve-in-MAC cases requiring a second valve deployed in an early study. The population in this study was at high surgical risk (STS score 14.4 ± 9.5%) and had a 30-day all-cause mortality of 30% (68). In order to plan a TMVR, 3D reconstructions can be created and valve implantation simulated using dedicated off-line software (Figure 3).
Figure 3. Steps in planning for mitral intervention in a patient with a heavily calcified mitral annulus. (A) 2D ECG-gated CT scan. Pre-existing TAVR valve in aortic position, with dense calcification of the mitral annulus. (B) Coronal view. 3D volume-rendered image of pre-existing TAVR valve in situ in aortic position. Cylindrical valve simulated in mitral position, thereby allowing for anatomical and geometrical calculations to be made prior to implantation. (C) 2D CT- En-face view of calcification surrounding mitral annulus. Also visible is the TAVR valve in the aortic position. (D) 3D volume-rendered en-face image of mitral annulus down through the left atrium. MAC highlighted in yellow. Panels (B,D) created courtesy of post-acquisition processing with Mimics Enlight TMVR planner, Beta version, Materialise NV Inc.
The trans-septal approach is increasingly being used to access the mitral valve. With CT, operators are able to determine the precise anatomy of the left atrium and plan the site of trans-septal puncture in order to minimize the risk of complications, including aortic puncture or myocardial perforation (69). Anomalies within the left atrium that can be seen via CT include aneurysms of the inter-atrial septum, patent foramen ovale and atrial septal defects, all requiring a tailored approach for the trans-septal puncture (70).
With respect to trans-apical approach, access requires an intimate knowledge of the position of the apex and its relation to the chest wall. Valve deployment using this approach requires a perpendicular deployment at the level of the mitral annulus. Once the apex is located, CT can identify the position of the papillary muscles, coronary arteries and chords, so as to plan the approach and prevent complications (61, 71).
As with TAVR planning, CT for TMVR planning can guide vascular access by defining the anatomy of peripheral vessels, including vessel dimension, tortuosity, location and extent of calcification and any prosthetic material such as stents.
Similar to percutaneous mitral valve interventions, minimal-invasive mitral valve surgery (MIMVS) is being performed with increasing frequency. MIMVS commonly refers to procedures involving mini-thoracotomy, port access and robotic-assisted techniques. Certain differences exist between MIMVS and standard open-heart surgery, including access (various locations and lengths of incisions), vision (direct, video-assisted or endoscopic), and cardio-pulmonary bypass strategies (antegrade vs retrograde), as well as between individual centers and operators.
Cardiac computed tomographic angiography and post-processing 3D reconstructions allow assessment for suitability [suitability for retrograde cannulation, presence of a heavily-calcified abdominal aorta, vessel tortuosity and pericardial calcification (72)] and access planning for MIMVS (location of mini-thoracotomy to access the left atrium). CT also allows for evaluation of the aortic dimensions. In MIMVS, one popular technique involves the use of an endo-aortic balloon; its safe use and efficacy dependent on aortic dimensions (73). The CT scan protocol can also include a CT coronary angiogram, which allows for accurate assessment of co-existent coronary disease. This is particularly sensitive in MIMVS patients, many of whom are young with few coronary risk factors and thus low risk profiles for coronary disease (74).
Cardiac computed tomography also plays a role in the evaluation of tricuspid valve pathology and the planning of related interventions. As with all right-sided lesions, comprehensive assessment via transthoracic echocardiography can be limited by suboptimal cardiac windows, especially when trying to accurately evaluate the three leaflets of the tricuspid valve (anterior, posterior, and septal) and associated structures. Like MR, tricuspid regurgitation (TR) can be primary, but is more often secondary, related to distortion of the right atrial or ventricular anatomy, and consequent annular dilatation.
Cardiac computed tomography facilitates accurate measurement of dimensions for the tricuspid annulus, right ventricular size and distance from the annulus to right ventricular apex, and thus allows deductions to be made as to the likely etiology of regurgitation.
As annular dilatation is often a key pathological process in TR, the majority of devices focus around annuloplasty (including Trialign, Tricinch, and Cardioband), edge-to-edge repair (Triclip and Forma) or the placement of valves in the vena cava to reduce the damage of the tricuspid regurgitant jet on hepatic and renal vasculature (TricValve).
For procedure planning, determining access to the right heart is key. Vascular access can be clearly defined by CT, including vessel dimensions and tortuosity. For the edge-to-edge repair systems, transfemoral venous access is routinely used, whilst for annuloplasty devices a trans-jugular approach is preferred. Accurate assessment of subclavian and axillary veins can also be done, aiding sheath and device delivery (75).
Annuloplasty-based treatments require delineation of landing zones, be that the tricuspid valve annulus, the inferior vena cava or the commissures. The relation of the landing zone with adjacent structures is also important. The right coronary artery runs along the posterior aspect of the tricuspid annulus along the heart’s epicardial surface. Its compression needs to be avoided when securing devices to the tricuspid annulus (76). Calcification along the annulus can also impair percutaneous valve deployment, which can be detected pre-procedurally via CT (77).
Structural valve degeneration (SVD) is defined as acquired abnormalities affecting the bioprosthetic valve leaflets and/or its supporting structures that eventually results in valve dysfunction (78). One study assessing patients with an assortment of surgical bioprostheses demonstrated at a median follow-up of 10 years, a rate of clinical SVD of 6.6% and subclinical SVD of 30.1% (79). Data on TAVR prostheses have demonstrated a rate of SVD at 1 year of 2.5% (80) and at a median of 5.8 years of 9% with severe SVD affecting <1% (81). CT provides a valuable tool for assessing the structure and mobility of prosthetic valves, but cannot determine transvalvular hemodynamics (78). Therefore the diagnosis and quantification of stenosis or regurgitation is best achieved using echocardiography with CT providing supplementary information.
Multi-slice CT angiography has provided important insights into the natural history of prosthetic valves with a particular focus on valve thrombosis. Hypo-attenuated leaflet thickening (HALT) can be found in 10–38% of prosthetic valves (82, 83), with the prevalence possibly higher in TAVR valves (84). Although lacking histological confirmation, this is highly suspected to be thrombus, based on its resolution with anticoagulation (85). HALT usually involves the periphery and bases of a leaflet and extends to a varying degree toward the center of the bioprosthesis (Figure 4) (4). HALT can develop as early as 5 days post-TAVR and has been shown to either progress, stabilize or regress over time (82, 83). Progression of HALT can lead to valve dysfunction described as restricted leaflet motion. This causes an increase in echocardiographically defined transvalvular gradients and eventually leads to symptoms of valve dysfunction (83). CT provides a reliable and potentially more sensitive methodology compared to transthoracic echocardiography for identifying and monitoring HALT (86, 84). It can also help determine management; the composition of acute thrombus has a low attenuation <90 HU, whereas chronic thrombus has values of 90–145 HU. Small acute thrombi are amenable to thrombolysis making this differentiation between types of thrombi important (87). 2D MPR provides an axial cross-sectional assessment to identify leaflet abnormalities. 3D volume rendered CT acquired through multiple phases provides confirmation of the leaflet abnormalities. When reconstructed into a movie (4D virtual reality CT), this can reliably illustrate restricted leaflet motion (4, 88, 89). Prophylaxis and treatment of thromboembolic disease associated with prosthetic valves have important implications for anti-thrombotic therapy, which is discussed elsewhere (90).
Figure 4. Hypo-attenuated leaflet thickening seen in three views of the same patient (A) at the level of the sinus of Valsalva, (B) left ventricular outflow tract view, (C) three chamber view.
Additionally, a common and late complication of prosthetic valves is pannus formation, which commonly coexists with thrombus. Differentiation of the two pathologies is important for management. Pannus has a high attenuation >145 HU and the degree to which it is obstructing the valve orifice can be calculated, making CT a useful modality for its detection (87).
Although echocardiography is the main imaging modality used to diagnose and monitor infective endocarditis, CT can play a valuable role and its use is advocated by international guidelines (91). CT can provide confirmation of a diagnosis with high accuracy if echocardiography is equivocal. Additionally, CT provides supplementary information such as extra-cardiac foci of infection, abscesses and pseudoaneursyms (5, 92). When combined with positron emission tomography (PET), CT provides diagnostic and prognostic value in prosthetic valve endocarditis for future cardiovascular events (93, 94) (Figure 5).
Figure 5. Aortic root abscess seen in a patient with a previous metallic surgical aortic valve implanted in 2010. An axial slice from 2013 without an abscess (A), and a similar slice from 2017 showing a root abscess indicated by a white arrow (B). A coronal view (C) and 3D reconstructions (D,E) illustrate the abscess indicated by the white arrow.
VHD causes myocardial remodeling affecting ECV and its composition (95). ECV quantification using cardiac magnetic resonance imaging has been shown to track myocardial fibrosis and provide prognostic value in AS patients (96, 97). Based on similar concepts to cardiac magnetic resonance imaging, ECV can be calculated using CT (98). Certain pathologies such as cardiac amyloidosis result in very high ECV, enabling CT to act as a screening tool (Figure 6) (99).
Figure 6. Extracellular volume quantification in two patients: (A) severe AS and (B) severe AS and cardiac transthyretin amyloidosis. Each panel illustrates a short-axis, 4 and 2 chamber views and a bull’s eye plot. High extracellular volume seen in panel (B) is identified by the yellow/orange coloration compared to lower extracellular volume in panel (A) identified by the green/blue areas.
Valvular heart disease directly affects the myocardial structure, function, and perfusion. Therefore, assessing these facets guides both, prognosis and management. CT myocardial perfusion imaging (CT MPI) provides prognostic information that can influence management strategies (100). Additionally the quantification of extracellular volume (ECV) has been shown to provide unique insights into diffuse myocardial fibrosis and cardiac amyloid (101). Fusion imaging using a CT overlay on live fluoroscopy imaging may provide a useful tool for transcatheter interventions enabling more complex and safer procedures (102). Photon counting CT potentially heralds a new era in cardiac CT, improving signal to noise ratio, reducing artifacts and radiation. Its integration into clinical use may improve the utility of CT for valvular heart disease (103).
Cardiac CT has become an irreplaceable adjunct to echocardiography in the clinical assessment of significant aortic stenosis and with the expansion of transcatheter valve intervention, the indications and utility of CT are continually growing. With high spatial resolution, CT allows evaluation of valve anatomy and coronary artery status, aortic pathology and vascular access planning, identification of the risk of likely complications and significant incidental extracardiac findings that influence treatment decisions, prognosis or trigger additional investigations.
KP and TT were involved in the genesis and planning of this manuscript. All authors contributed to the literature review, figures, text and editing of the final manuscript.
KP was funded by a University College London-held British Heart Foundation clinical research training fellowship grant (FS/19/48/34523) and has an unrestricted research grant from Edwards Lifesciences. FP had received research support from Siemens Healthineers. TT was supported by British Heart Foundation Intermediate fellowship (FS/19/35/3434). JM and TT were directly and indirectly supported by the UCLH and Barts NIHR Biomedical Research Units.
The authors declare that the research was conducted in the absence of any commercial or financial relationships that could be construed as a potential conflict of interest.
All claims expressed in this article are solely those of the authors and do not necessarily represent those of their affiliated organizations, or those of the publisher, the editors and the reviewers. Any product that may be evaluated in this article, or claim that may be made by its manufacturer, is not guaranteed or endorsed by the publisher.
AS, aortic stenosis; AVA, aortic valve area; AVCS, aortic valve calcium score; CAD, coronary artery disease; CT, cardiac computed tomography; CTCA, computed tomography coronary angiogram; LVOT, left ventricular outflow tract; MPR, multi-planar reconstruction; MR, mitral regurgitation; SAVR, surgical aortic valve replacement; TAVR, transcatheter aortic valve replacement; TMVP, transcatheter mitral valve prosthesis; TMVR, transcatheter mitral valve replacement; VHD, valvular heart disease.
1. Gaede L, Blumenstein J, Liebetrau C, Dörr O, Kim WK, Nef H, et al. Outcome after transvascular transcatheter aortic valve implantation in 2016. Eur Heart J. (2018) 39:667–75. doi: 10.1093/eurheartj/ehx688
2. Regueiro A, Granada JF, Dagenais F, Rodés-Cabau J. Transcatheter mitral valve replacement: insights from early clinical experience and future challenges. J Am Coll Cardiol. (2017) 2:2175–92. doi: 10.1016/j.jacc.2017.02.045
3. Blanke P, Weir-McCall JR, Achenbach S, Delgado V, Hausleiter J, Jilaihawi H, et al. Computed tomography imaging in the context of transcatheter aortic valve implantation (TAVI)/transcatheter aortic valve replacement (TAVR): an expert consensus document of the society of cardiovascular computed tomography. JACC Cardiovasc Imaging. (2019) 12:1–24. doi: 10.1016/j.jcmg.2018.12.003
4. Jilaihawi H, Asch FM, Manasse E, Ruiz CE, Jelnin V, Kashif M, et al. Systematic CT methodology for the evaluation of subclinical leaflet thrombosis. JACC Cardiovasc Imaging. (2017) 10:461–70. doi: 10.1016/j.jcmg.2017.02.005
5. Habets J, Tanis W, Van Herwerden LA, Van Den Brink RBA, Mali WPTM, De Mol BAJM, et al. Cardiac computed tomography angiography results in diagnostic and therapeutic change in prosthetic heart valve endocarditis. Int J Cardiovasc Imaging. (2014) 30:377–87. doi: 10.1007/s10554-013-0335-2
6. Faggioni L, Gabelloni M, Accogli S, Angelillis M, Costa G, Spontoni P, et al. Preprocedural planning of transcatheter mitral valve interventions by multidetector CT: what the radiologist needs to know. Eur J Radiol Open. (2018) 31:131–40. doi: 10.1016/j.ejro.2018.08.005
7. Nkomo VT, Gardin JM, Skelton TN, Gottdiener JS, Scott CG, Enriquez-Sarano M. Burden of valvular heart diseases: a population-based study. Lancet. (2006) 368:1005–11. doi: 10.1016/S0140-6736(06)69208-8
8. Baumgartner H, Falk V, Bax JJ, De Bonis M, Hamm C, Holm PJ, et al. 2017 ESC/EACTS guidelines for the management of valvular heart disease. Eur Heart J. (2017) 38:2739–86. doi: 10.1093/eurheartj/ehx391
9. Messika-Zeitoun D, Serfaty JM, Brochet E, Ducrocq G, Lepage L, Detaint D, et al. Multimodal assessment of the aortic annulus diameter. Implications for transcatheter aortic valve implantation. J Am Coll Cardiol. (2010) 55:186–94. doi: 10.1016/j.jacc.2009.06.063
10. Clavel MA, Malouf J, Messika-Zeitoun D, Araoz PA, Michelena HI, Enriquez-Sarano M. Aortic valve area calculation in aortic stenosis by CT and doppler echocardiography. JACC Cardiovasc Imaging. (2015) 8:248–57. doi: 10.1016/j.jcmg.2015.01.009
11. Pibarot P, Dumesnil JG. Low-flow, low-gradient aortic stenosis with normal and depressed left ventricular ejection fraction. J Am Coll Cardiol. (2012) 60:1845–53. doi: 10.1016/j.jacc.2012.06.051
12. David M-Z, Guy L. Aortic valve stenosis: evaluation and management of patients with discordant grading. E J Cardiol Pract. (2018) 15:N26.
13. Pawade T, Clavel MA, Tribouilloy C, Dreyfus J, Mathieu T, Tastet L, et al. Computed tomography aortic valve calcium scoring in patients with aortic stenosis. Circ Cardiovasc Imaging. (2018) 11:e007146. doi: 10.1161/CIRCIMAGING.117.007146
14. Pawade TA, Newby DE, Dweck MR. Calcification in aortic stenosis: the skeleton key. J Am Coll Cardiol. (2015) 66:561–77. doi: 10.1016/j.jacc.2015.05.066
15. Agatston AS, Janowitz WR, Hildner FJ, Zusmer NR, Viamonte M, Detrano R. Quantification of coronary artery calcium using ultrafast computed tomography. J Am Coll Cardiol. (1990) 15:827. doi: 10.1016/0735-1097(90)90282-T
16. Messika-Zeitoun D, Aubry MC, Detaint D, Bielak LF, Peyser PA, Sheedy PF, et al. Evaluation and clinical implications of aortic valve calcification measured by electron-beam computed tomography. Circulation. (2004) 110:356–62. doi: 10.1161/01.CIR.0000135469.82545.D0
17. Cueff C, Serfaty JM, Cimadevilla C, Laissy JP, Himbert D, Tubach F, et al. Measurement of aortic valve calcification using multislice computed tomography: correlation with haemodynamic severity of aortic stenosis and clinical implication for patients with low ejection fraction. Heart. (2011) 97:721–6. doi: 10.1136/hrt.2010.198853
18. Clavel MA, Messika-Zeitoun D, Pibarot P, Aggarwal SR, Malouf J, Araoz PA, et al. The complex nature of discordant severe calcified aortic valve disease grading: new insights from combined Doppler echocardiographic and computed tomographic study. J Am Coll Cardiol. (2013) 62:2329–38. doi: 10.1016/j.jacc.2013.08.1621
19. Clavel MA, Pibarot P, Messika-Zeitoun D, Capoulade R, Malouf J, Aggarval S, et al. Impact of aortic valve calcification, as measured by MDCT, on survival in patients with aortic stenosis: results of an international registry study. J Am Coll Cardiol. (2014) 64:1202–13. doi: 10.1016/j.jacc.2014.05.066
20. Messika-Zeitoun D, Bielak LF, Peyser PA, Sheedy PF, Turner ST, Nkomo VT, et al. Aortic valve calcification: determinants and progression in the population. Arterioscler Thromb Vasc Biol. (2007) 27:642–8. doi: 10.1161/01.ATV.0000255952.47980.c2
21. Aggarwal SR, Clavel MA, Messika-Zeitoun D, Cueff C, Malouf J, Araoz PA, et al. Sex differences in aortic valve calcification measured by multidetector computed tomography in aortic stenosis. Circ Cardiovasc Imaging. (2013) 6:40–7. doi: 10.1161/CIRCIMAGING.112.980052
22. Falk V, Baumgartner H, Bax JJ, De Bonis M, Hamm C, Holm PJ, et al. 2017 ESC/EACTS guidelines for the management of valvular heart disease. Eur J Cardiothorac Surg. (2017) 52:616–64. doi: 10.1093/ejcts/ezx324
23. Jean G, Van Mieghem NM, Gegenava T, van Gils L, Bernard J, Geleijnse ML, et al. Moderate aortic stenosis in patients with heart failure and reduced ejection fraction. J Am Coll Cardiol. (2021) 77:2796–803. doi: 10.1016/j.jacc.2021.04.014
24. Strange G, Stewart S, Celermajer D, Prior D, Scalia GM, Marwick T, et al. Poor long-term survival in patients with moderate aortic stenosis. J Am Coll Cardiol. (2019) 74:1851–63. doi: 10.1016/j.jacc.2019.08.004
25. Clinicaltrials.gov Transcatheter Aortic Valve Replacement to UNload the Left Ventricle in Patients with Advanced Heart Failure: A Randomized Trial (TAVR UNLOAD). Available online at: https://clinicaltrials.gov/ct2/show/NCT02661451 (accessed June 12, 2021).
26. Rosenhek R, Klaar U, Schemper M, Scholten C, Heger M, Gabriel H, et al. Mild and moderate aortic stenosis: natural history and risk stratification by echocardiography. Eur Heart J. (2004) 25:199–205. doi: 10.1016/j.ehj.2003.12.002
27. Hayashida K, Lefvre T, Chevalier B, Hovasse T, Romano M, Garot P, et al. Transfemoral aortic valve implantation: new criteria to predict vascular complications. JACC Cardiovasc Interv. (2011) 4:851–8. doi: 10.1016/j.jcin.2011.03.019
28. Toggweiler S, Gurvitch R, Leipsic J, Wood DA, Willson AB, Binder RK, et al. Percutaneous aortic valve replacement: vascular outcomes with a fully percutaneous procedure. J Am Coll Cardiol. (2012) 59:113–8. doi: 10.1016/j.jacc.2011.08.069
29. Achenbach S, Delgado V, Hausleiter J, Schoenhagen P, Min JK, Leipsic JA. SCCT expert consensus document on computed tomography imaging before transcatheter aortic valve implantation (TAVI)/transcatheter aortic valve replacement (TAVR). J Cardiovasc Comput Tomogr. (2012) 6:366–80. doi: 10.1016/j.jcct.2012.11.002
30. Biasco L, Ferrari E, Pedrazzini G, Faletra F, Moccetti T, Petracca F, et al. Access sites for TAVI: patient selection criteria, technical aspects, and outcomes. Front Cardiovasc Med. (2018) 5:88. doi: 10.3389/fcvm.2018.00088
31. Lederman RJ, Chen MY, Rogers T, Wang DD, Paone G, Guerrero M, et al. Planning transcaval access using CT for large transcatheter implants. JACC Cardiovasc Imaging. (2014) 7:1167–71. doi: 10.1016/j.jcmg.2014.05.015
32. Lederman RJ, Greenbaum AB, Rogers T, Khan JM, Fusari M, Chen MY. Anatomic suitability for transcaval access based on computed tomography. JACC Cardiovasc Interv. (2017) 10:1–10. doi: 10.1016/j.jcin.2016.09.002
33. Hell MM, Biburger L, Marwan M, Schuhbaeck A, Achenbach S, Lell M, et al. Prediction of fluoroscopic angulations for transcatheter aortic valve implantation by CT angiography: influence on procedural parameters. Eur Heart J Cardiovasc Imaging. (2016) 18:906–14. doi: 10.1093/ehjci/jew144
34. Schwarz F, Lange P, Zinsser D, Greif M, Boekstegers P, Schmitz C, et al. CT-angiography based evaluation of the aortic annulus for prosthesis sizing in transcatheter aortic valve implantation (TAVI)-predictive value and optimal thresholds for major anatomic parameters. PLoS One. (2014) 9:e103481. doi: 10.1371/journal.pone.0103481
35. Hayashida K, Bouvier E, Lefèvre T, Hovasse T, Morice MC, Chevalier B, et al. Impact of CT-guided valve sizing on post-procedural aortic regurgitation in transcatheter aortic valve implantation. EuroIntervention. (2012) 8:546–55. doi: 10.4244/EIJV8I5A85
36. Dvir D, Leipsic J, Blanke P, Ribeiro HB, Kornowski R, Pichard A, et al. Coronary obstruction in transcatheter aortic valve-in-valve implantation. Circ Cardiovasc Interv. (2015) 8:e002079. doi: 10.1161/circinterventions.114.002079
37. Ribeiro HB, Webb JG, Makkar RR, Cohen MG, Kapadia SR, Kodali S, et al. Predictive factors, management, and clinical outcomes of coronary obstruction following transcatheter aortic valve implantation: insights from a large multicenter registry. J Am Coll Cardiol. (2013) 62:1552–62. doi: 10.1016/j.jacc.2013.07.040
38. Barbanti M, Yang T-H, Cabau JR, Tamburino C, Wood DA, Jilaihawi H, et al. Anatomical and procedural features associated with aortic root rupture during balloon-expandable transcatheter aortic valve replacement. Circulation. (2013) 128:244–53. doi: 10.1161/CIRCULATIONAHA.113.002947
39. Van Mieghem NM, Schultz CJ, Van Der Boon RMA, Nuis RJ, Tzikas A, Geleijnse ML, et al. Incidence, timing, and predictors of valve dislodgment during TAVI with the medtronic corevalve system. Catheter Cardiovasc Interv. (2012) 79:726–32. doi: 10.1002/ccd.23275
40. Takagi H, Umemoto T. Impact of paravalvular aortic regurgitation after transcatheter aortic valve implantation on survival. Int J Cardiol. (2016) 221:46–51. doi: 10.1016/j.ijcard.2016.07.006
41. Delgado V, Ng ACT, Van De Veire NR, Van Der Kley F, Schuijf JD, Tops LF, et al. Transcatheter aortic valve implantation: role of multi-detector row computed tomography to evaluate prosthesis positioning and deployment in relation to valve function. Eur Heart J. (2010) 31:1114–23. doi: 10.1093/eurheartj/ehq018
42. Pollari F, Dell’Aquila AM, Söhn C, Marianowicz J, Wiehofsky P, Schwab J, et al. Risk factors for paravalvular leak after transcatheter aortic valve replacement. J Thorac Cardiovasc Surg. (2019) 157:1406.e–15.e. doi: 10.1016/j.jtcvs.2018.08.085
43. Kawsara A, Sulaiman S, Alqahtani F, Eleid MF, Deshmukh AJ, Cha Y, et al. Temporal trends in the incidence and outcomes of pacemaker implantation after transcatheter aortic valve replacement in the United States (2012\&\#x2013;2017). J Am Heart Assoc. (2020) 9:e016685. doi: 10.1161/JAHA.120.016685
44. Chamandi C, Barbanti M, Munoz-Garcia A, Latib A, Nombela-Franco L, Gutiérrez-Ibanez E, et al. Long-term outcomes in patients with new permanent pacemaker implantation following transcatheter aortic valve replacement. JACC Cardiovasc Interv. (2018) 11:301–10. doi: 10.1016/j.jcin.2017.10.032
45. Latsios G, Gerckens U, Buellesfeld L, Mueller R, John D, Yuecel S, et al. “Device landing zone” calcification, assessed by MSCT, as a predictive factor for pacemaker implantation after TAVI. Catheter Cardiovasc Interv. (2010) 76:431–9. doi: 10.1002/ccd.22563
46. Hamdan A, Guetta V, Klempfner R, Konen E, Raanani E, Glikson M, et al. Inverse relationship between membranous septal length and the risk of atrioventricular block in patients undergoing transcatheter aortic valve implantation. JACC Cardiovasc Interv. (2015) 8:1218–28. doi: 10.1016/j.jcin.2015.05.010
47. Abramowitz Y, Kazuno Y, Chakravarty T, Kawamori H, Maeno Y, Anderson D, et al. Concomitant mitral annular calcification and severe aortic stenosis: prevalence, characteristics and outcome following transcatheter aortic valve replacement. Eur Heart J. (2017) 38:1194–203. doi: 10.1093/eurheartj/ehw594
48. Hansson NC, Nørgaard BL, Barbanti M, Nielsen NE, Yang T-H, Tamburino C, et al. The impact of calcium volume and distribution in aortic root injury related to balloon-expandable transcatheter aortic valve replacement. J Cardiovasc Comput Tomogr. (2015) 9:382–92. doi: 10.1016/j.jcct.2015.04.002
49. Chieffo A, Giustino G, Spagnolo P, Panoulas VF, Montorfano M, Latib A, et al. Routine screening of coronary artery disease with computed tomographic coronary angiography in place of invasive coronary angiography in patients undergoing transcatheter aortic valve replacement. Circ Cardiovasc Interv. (2015) 8:e002025. doi: 10.1161/CIRCINTERVENTIONS.114.002025
50. Feuchtner GM, Alkadhi H, Karlo C, Sarwar A, Meier A, Dichtl W, et al. Cardiac CT angiography for the diagnosis of mitral valve prolapse: comparison with echocardiography. Radiology. (2010) 254:374–83. doi: 10.1148/radiol.2541090393
51. Delgado V, Tops LF, Schuijf JD, de Roos A, Brugada J, Schalij MJ, et al. Assessment of mitral valve anatomy and geometry with multislice computed tomography. JACC Cardiovasc Imaging. (2009) 2:556–65. doi: 10.1016/j.jcmg.2008.12.025
52. Palazzuoli A, Cademartiri F, Geleijnse ML, Meijboom B, Pugliese F, Soliman O, et al. Left ventricular remodelling and systolic function measurement with 64 multi-slice computed tomography versus second harmonic echocardiography in patients with coronary artery disease: a double blind study. Eur J Radiol. (2010) 73:82–8. doi: 10.1016/j.ejrad.2008.09.022
53. Guo YK, Yang ZG, Ning G, Rao L, Dong L, Pen Y, et al. Isolated mitral regurgitation: quantitative assessment with 64-section multidetector CT – comparison with MR imaging and echocardiography. Radiology. (2009) 252:369–76. doi: 10.1148/radiol.2522081714
54. Morris MF, Maleszewski JJ, Suri RM, Burkhart HM, Foley TA, Bonnichsen CR, et al. CT and MR imaging of the mitral valve: radiologic-pathologic correlation. Radiographics. (2010) 30:1603–20. doi: 10.1148/rg.306105518
55. Shah M, Jorde UP. Percutaneous mitral valve interventions (Repair): current indications and future perspectives. Front Cardiovasc Med. (2019) 6:88. doi: 10.3389/fcvm.2019.00088
56. Blanke P, Dvir D, Cheung A, Ye J, Levine RA, Precious B, et al. A simplified D-shaped model of the mitral annulus to facilitate CT-based sizing before transcatheter mitral valve implantation. J Cardiovasc Comput Tomogr. (2014) 8:459–67. doi: 10.1016/j.jcct.2014.09.009
57. Blanke P, Naoum C, Webb J, Dvir D, Hahn RT, Grayburn P, et al. Multimodality imaging in the context of transcatheter mitral valve replacement establishing consensus among modalities and disciplines. JACC Cardiovasc Imaging. (2015) 8:1191–208. doi: 10.1016/j.jcmg.2015.08.004
58. Shanks M, Delgado V, Ng ACT, Van Der Kley F, Schuijf JD, Boersma E, et al. Mitral valve morphology assessment: three-dimensional transesophageal echocardiography versus computed tomography. Ann Thorac Surg. (2010) 90:1922–9. doi: 10.1016/j.athoracsur.2010.06.116
59. Gössl M, Sorajja P. MitraClip patient selection: inclusion and exclusion criteria for optimal outcomes. Ann Cardiothorac Surg. (2018) 7:771–5. doi: 10.21037/acs.2018.08.04
60. Yoon SH, Bleiziffer S, Latib A, Eschenbach L, Ancona M, Vincent F, et al. Predictors of left ventricular outflow tract obstruction after transcatheter mitral valve replacement. JACC Cardiovasc Interv. (2019) 12:182–93. doi: 10.1016/j.jcin.2018.12.001
61. Naoum C, Blanke P, Cavalcante JL, Leipsic J. Cardiac computed tomography and magnetic resonance imaging in the evaluation of mitral and tricuspid valve disease. Circ Cardiovasc Imaging. (2017) 10:e005331. doi: 10.1161/CIRCIMAGING.116.005331
62. Meduri CU, Reardon MJ, Lim DS, Howard E, Dunnington G, Lee DP, et al. Novel multiphase assessment for predicting left ventricular outflow tract obstruction before transcatheter mitral valve replacement. JACC Cardiovasc Interv. (2019) 12:2402–12. doi: 10.1016/j.jcin.2019.06.015
63. Allison MA, Cheung P, Criqui MH, Langer RD, Wright CM. Mitral and aortic annular calcification are highly associated with systemic calcified atherosclerosis. Circulation. (2006) 113:861–6. doi: 10.1161/CIRCULATIONAHA.105.552844
64. Barasch E, Gottdiener JS, Marino Larsen EK, Chaves PHM, Newman AB, Manolio TA. Clinical significance of calcification of the fibrous skeleton of the heart and aortosclerosis in community dwelling elderly. the cardiovascular health study (CHS). Am Heart J. (2006) 151:39–47. doi: 10.1016/j.ahj.2005.03.052
65. Guerrero M, Urena M, Himbert D, Wang DD, Eleid M, Kodali S, et al. 1-Year outcomes of transcatheter mitral valve replacement in patients with severe mitral annular calcification. J Am Coll Cardiol. (2018) 71:1841–53. doi: 10.1016/j.jacc.2018.02.054
66. Okada Y. Surgical management of mitral annular calcification. Gen Thorac Cardiovasc Surg. (2013) 61:619–25. doi: 10.1007/s11748-013-0207-7
67. Weir-McCall JR, Blanke P, Naoum C, Delgado V, Bax JJ, Leipsic J. Mitral valve imaging with CT: relationship with transcatheter mitral valve interventions. Radiology. (2018) 288:638–55. doi: 10.1148/radiol.2018172758
68. Guerrero M, Dvir D, Himbert D, Urena M, Eleid M, Wang DD, et al. Transcatheter mitral valve replacement in native mitral valve disease with severe mitral annular calcification: results from the first multicenter global registry. JACC Cardiovasc Interv. (2016) 9:1361–71. doi: 10.1016/j.jcin.2016.04.022
69. O’Brien B, Zafar H, De Freitas S, Sharif F. Transseptal puncture — review of anatomy, techniques, complications and challenges. Int J Cardiol. (2017) 233:12–22. doi: 10.1016/j.ijcard.2017.02.009
70. Yasunaga D, Hamon M. MDCT of interatrial septum. Diagn Interv Imaging. (2015) 96:891–9. doi: 10.1016/j.diii.2015.02.011
71. Yu W-L, Omid-Fard N, Arepalli C, Shao M, Hart SL., Weir McCall J, et al. Role of computed tomography in pre-procedural planning of transcatheter mitral valve replacement. Struct Heart. (2018) 2:23–9. doi: 10.1080/24748706.2017.1407503
72. Heuts S, Maessen JG, Sardari Nia P. Preoperative planning of left-sided valve surgery with 3D computed tomography reconstruction models: sternotomy or a minimally invasive approach? Interact Cardiovasc Thorac Surg. (2016) 22:587–93. doi: 10.1093/icvts/ivv408
73. Czesla M, Mogilansky C, Balan R, Kattner S, van Ingen G, Massoudy P. Evolution of a minimally invasive mitral valve program. J Vis Surg. (2016) 2:169. doi: 10.21037/jovs.2016.11.04
74. Morris MF, Suri RM, Akhtar NJ, Young PM, Gruden JF, Burkhart HM, et al. Computed tomography as an alternative to catheter angiography prior to robotic mitral valve repair. Ann Thorac Surg. (2013) 95:1354–9. doi: 10.1016/j.athoracsur.2012.12.010
75. Van Praet KM, Kofler M, Montagner M, Heck R, Eggert-Doktor D, Stamm C, et al. Minimally invasive mitral valve repair using external clamping—pearls and pitfalls. J Vis Surg. (2020) 6:45.
76. Latib A, Agricola E, Pozzoli A, Denti P, Taramasso M, Spagnolo P, et al. First-in-man implantation of a tricuspid annular remodeling device for functional tricuspid regurgitation. JACC Cardiovasc Interv. (2015) 8:e211–4. doi: 10.1016/j.jcin.2015.06.028
77. Winkel MG, Brugger N, Khalique OK, Gräni C, Huber A, Pilgrim T, et al. Imaging and patient selection for transcatheter tricuspid valve interventions. Front Cardiovasc Med. (2020) 7:60. doi: 10.3389/fcvm.2020.00060
78. Dvir D, Bourguignon T, Otto CM, Hahn RT, Rosenhek R, Webb JG, et al. Standardized definition of structural valve degeneration for surgical and transcatheter bioprosthetic aortic valves. Circulation. (2018) 137:388–99. doi: 10.1161/CIRCULATIONAHA.117.030729
79. Rodriguez-Gabella T, Voisine P, Dagenais F, Mohammadi S, Perron J, Dumont E, et al. Long-term outcomes following surgical aortic bioprosthesis implantation. J Am Coll Cardiol. (2018) 71:1401–12. doi: 10.1016/j.jacc.2018.01.059
80. Vemulapalli S, Holmes DR, Dai D, Matsouaka R, Mack MJ, Grover FL, et al. Valve hemodynamic deterioration and cardiovascular outcomes in TAVR: a report from the STS/ACC TVT registry. Am Heart J. (2018) 195:1–13. doi: 10.1016/j.ahj.2017.09.005
81. Blackman DJ, Saraf S, MacCarthy PA, Myat A, Anderson SG, Malkin CJ, et al. Long-term durability of transcatheter aortic valve prostheses. J Am Coll Cardiol. (2019) 73:537–45. doi: 10.1016/j.jacc.2018.10.078
82. Pache G, Schoechlin S, Blanke P, Dorfs S, Jander N, Arepalli CD, et al. Early hypo-attenuated leaflet thickening in balloon-expandable transcatheter aortic heart valves. Eur Heart J. (2016) 37:2263–71. doi: 10.1093/eurheartj/ehv526
83. Sondergaard L, De Backer O, Kofoed KF, Jilaihawi H, Fuchs A, Chakravarty T, et al. Natural history of subclinical leaflet thrombosis affectingmotion in bioprosthetic aortic valves. Eur Heart J. (2017) 38:2201–7. doi: 10.1093/eurheartj/ehx369
84. Chakravarty T, Søndergaard L, Friedman J, De Backer O, Berman D, Kofoed KF, et al. Subclinical leaflet thrombosis in surgical and transcatheter bioprosthetic aortic valves: an observational study. Lancet. (2017) 17:2383–92. doi: 10.1016/S0140-6736(17)30757-2
85. Ruile P, Neumann F-J. Valve thrombosis after TAVI. Eur Heart J. (2017) 38:2700–1. doi: 10.1093/eurheartj/ehx472
86. Leetmaa T, Hansson NC, Leipsic J, Jensen K, Poulsen SH, Andersen HR, et al. Early aortic transcatheter heart valve thrombosis: diagnostic value of contrast-enhanced multidetector computed tomography. Circ Cardiovasc Interv. (2015) 8:e001596. doi: 10.1161/CIRCINTERVENTIONS.114.001596
87. Aladmawi MA, Pragliola C, Vriz O, Galzerano D. Use of multidetector-row computed tomography scan to detect pannus formation in prosthetic mechanical aortic valves. J Thorac Dis. (2017) 9:s343–8. doi: 10.21037/jtd.2017.04.14
88. Pache G, Blanke P, Zeh W, Jander N. Cusp thrombosis after transcatheter aortic valve replacement detected by computed tomography and echocardiography. Eur Heart J. (2013) 34:3546. doi: 10.1093/eurheartj/eht316
89. Makkar RR, Fontana G, Jilaihawi H, Chakravarty T, Kofoed KF, De Backer O, et al. Possible subclinical leaflet thrombosis in bioprosthetic aortic valves. N Engl J Med. (2015) 373:2015–24. doi: 10.1056/NEJMoa1509233
90. Calabrò P, Gragnano F, Niccoli G, Marcucci R, Zimarino M, Spaccarotella C, et al. Antithrombotic therapy in patients undergoing transcatheter interventions for structural heart disease. Circulation. (2021) 144:1323–43. doi: 10.1161/CIRCULATIONAHA.121.054305
91. Habib G, Lancellotti P, Antunes MJ, Bongiorni MG, Casalta JP, Del Zotti F, et al. 2015 ESC infective endocarditis. Eur Heart J. (2015) 36:3075–128. doi: 10.1093/eurheartj/ehv319
92. Feuchtner GM, Stolzmann P, Dichtl W, Schertler T, Bonatti J, Scheffel H, et al. Multislice computed tomography in infective endocarditis. Comparison with transesophageal echocardiography and intraoperative findings. J Am Coll Cardiol. (2009) 53:436–44. doi: 10.1016/j.jacc.2008.01.077
93. San S, Ravis E, Tessonier L, Philip M, Cammilleri S, Lavagna F, et al. Prognostic value of 18F-fluorodeoxyglucose positron emission tomography/computed tomography in infective endocarditis. J Am Coll Cardiol. (2019) 74:1031–1040. doi: 10.1016/j.jacc.2019.06.050
94. Pizzi MN, Roque A, Fernández-Hidalgo N, Cuéllar-Calabria H, Ferreira-González I, Gonzàlez-Alujas MT, et al. Improving the diagnosis of infective endocarditis in prosthetic valves and intracardiac devices with 18F-fluordeoxyglucose positron emission tomography/computed tomography angiography: initial results at an infective endocarditis referral center. Circulation. (2015) 132:1113–26. doi: 10.1161/CIRCULATIONAHA.115.015316
95. Treibel TA, López B, González A, Menacho K, Schofield RS, Ravassa S, et al. Reappraising myocardial fibrosis in severe aortic stenosis: an invasive and non-invasive study in 133 patients. Eur Heart J. (2018) 39:699–709. doi: 10.1093/eurheartj/ehx353
96. Treibel TA, Kozor R, Schofield R, Benedetti G, Fontana M, Bhuva AN, et al. Reverse myocardial remodeling following valve replacement in patients with aortic stenosis. J Am Coll Cardiol. (2018) 71:860–71. doi: 10.1016/j.jacc.2017.12.035
97. Chin CWL, Everett RJ, Kwiecinski J, Vesey AT, Yeung E, Esson G, et al. Myocardial fibrosis and cardiac decompensation in aortic stenosis. JACC Cardiovasc Imaging. (2017) 10:1320–33. doi: 10.1016/j.jcmg.2016.10.007
98. Treibel TA, Bandula S, Fontana M, White SK, Gilbertson JA, Herrey AS, et al. Extracellular volume quantification by dynamic equilibrium cardiac computed tomography in cardiac amyloidosis. J Cardiovasc Comput Tomogr. (2015) 9:585–92. doi: 10.1016/j.jcct.2015.07.001
99. Treibel T, Bandula S, Fontana M, White SK, Gilbertson JA, Punwani SA, et al. Quantification cardiac amyloid by cardiac computed tomography. Eur Heart J Cardiovasc Imaging. (2015) 9:585–92.
100. Takx RAP, Celeng C, Schoepf UJ. CT myocardial perfusion imaging: ready for prime time? Eur Radiol. (2018) 28:1253–6. doi: 10.1007/s00330-017-5057-8
101. Scully PR, Bastarrika G, Moon JC, Treibel TA. Myocardial extracellular volume quantification by cardiovascular magnetic resonance and computed tomography. Curr Cardiol Rep. (2018) 20:15. doi: 10.1007/s11886-018-0961-3
102. Mousavi N, Spaziano M. The Expanding Role of CT in Pre-procedural Planning of Percutaneous Structural Heart Disease Interventions. Washington, DC: American College of Cardiology (2018).
Keywords: valvular heart disease, aortic stenosis, TAVR, TMVR, cardiac computed tomography
Citation: Patel KP, Vandermolen S, Herrey AS, Cheasty E, Menezes L, Moon JC, Pugliese F and Treibel TA (2022) Cardiac Computed Tomography: Application in Valvular Heart Disease. Front. Cardiovasc. Med. 9:849540. doi: 10.3389/fcvm.2022.849540
Received: 06 January 2022; Accepted: 22 February 2022;
Published: 24 March 2022.
Edited by:
Ronak Rajani, Guy’s and St Thomas’ NHS Foundation Trust, United KingdomReviewed by:
Felice Gragnano, University of Campania Luigi Vanvitelli, ItalyCopyright © 2022 Patel, Vandermolen, Herrey, Cheasty, Menezes, Moon, Pugliese and Treibel. This is an open-access article distributed under the terms of the Creative Commons Attribution License (CC BY). The use, distribution or reproduction in other forums is permitted, provided the original author(s) and the copyright owner(s) are credited and that the original publication in this journal is cited, in accordance with accepted academic practice. No use, distribution or reproduction is permitted which does not comply with these terms.
*Correspondence: Thomas A. Treibel, VGhvbWFzLlRyZWliZWwuMTJAdWNsLmFjLnVr
Disclaimer: All claims expressed in this article are solely those of the authors and do not necessarily represent those of their affiliated organizations, or those of the publisher, the editors and the reviewers. Any product that may be evaluated in this article or claim that may be made by its manufacturer is not guaranteed or endorsed by the publisher.
Research integrity at Frontiers
Learn more about the work of our research integrity team to safeguard the quality of each article we publish.