- 1Department of Cardiology, Renmin Hospital of Wuhan University, Wuhan, China
- 2Cardiac Autonomic Nervous System Research Centre of Wuhan University, Wuhan, China
- 3Cardiovascular Research Institute, Wuhan University, Wuhan, China
- 4Hubei Key Laboratory of Cardiology, Wuhan, China
Background: Both coronary physiology and deceleration capacity (DC) showed prognostic efficacy for patients with acute coronary syndrome (ACS). This retrospective cohort study was performed to evaluate the prognostic implication of DC combined with the relative increase and final coronary physiology as detected by quantitative flow ratio (QFR) for patients with non-ST-elevation ACS (NSTE-ACS) who underwent complete and successful percutaneous coronary intervention (PCI).
Methods: Patients with NSTE-ACS who underwent PCI with pre- and post-procedural QFR in our department between January 2018 and November 2019 were included. The 24-hour deceleration capacity (DC 24h) was obtained via Holter monitoring. The incidence of major adverse cardiac and cerebrovascular events (MACCEs) during follow up was defined as the primary outcome. The optimal cutoffs of the relative increase, final QFR, and DC 24h for prediction of MACCEs were determined via receiver operating characteristic (ROC) analysis and the predictive efficacies were evaluated with multivariate Cox regression analysis.
Results: Overall, 240 patients were included. During a mean follow up of 21.3 months, 31 patients had MACCEs. Results of multivariate Cox regression analyses showed that a higher post-PCI QFR [adjusted hazard ratio (HR): 0.318; 95% confidence interval (CI): 0.129–0.780], a higher relative QFR increase (HR: 0.161; 95% CI: 0.066–0.391], and a higher DC (HR: 0.306; 95% CI: 0.134–0.701) were all independent predictors of lower risk of MACCEs. Subsequently, incorporating low DC (≤2.42) into the risk predicting model with clinical variables, the predictive efficacies of low relative QRS increase (≤23%) and low post-PCI QFR (≤0.88) for MACCEs were both significantly improved.
Conclusions: The DC combined with relative increase and final coronary physiology may improve the predictive efficacy of existing models based on clinical variables for MACCEs in NSTE-ACS patients who underwent complete and successful PCI.
Introduction
Currently, physiological assessment of coronary artery stenosis has become an important standard for decision making in percutaneous coronary intervention (PCI) (1–3). Indeed, it has been shown that up to 20% of patients with successful revascularization as evidenced by angiographic findings still suffer from subsequent adverse coronary events (4–6). Recently studies showed that these patients were likely to have residual or diffuse disease and/or stented segment, due to condition of anatomical revascularization, but not functional revascularization (4–6). Previous studies have shown that the relative increase and final fractional flow reserve (FFR) were reliable parameters for evaluating coronary functional revascularization and may confer prognostic efficacy for patients after PCI (4). Furthermore, recent studies suggest that quantitative flow ratio (QFR), a highly consistent parameter, with FFR indicating functional stenosis of coronary arteries, may also be a validated prognostic index after PCI (5–7). However, the current understanding of coronary artery disease (CAD) indicates that the progression of disease is not only determined by the anatomy or physiology of the coronary lesion alone but is also influenced by systemic factors, such as inflammation and autonomic dysfunction (8–10). In this regard, an integrated approach incorporating parameters of functional revascularization, such as QFR, may confer better prognostic implications in patients with non-ST-elevation acute coronary syndrome (NSTE-ACS) after PCI.
Our previous study revealed a significant association between parameters of heart rate variability (HRV), inflammation, and coronary artery physiology based on QFR (11). Specifically, the 24-hour deceleration capacity (DC 24h), a Holter-derived indicator of parasympathetic activity, has also been suggested as a strong predictor of mortality for patients with myocardial infarction (12). Physiologically, automatic nervous system (ANS) carries the essential function in the formation of the heart and critical regulator of vascular development during cardiovascular development (13). Moreover, pathologically, autonomic function, particularly the activity of the vagus nerve, has been correlated with systemic inflammation (13, 14) and vascular tension (15). Therefore, we hypothesized that an integrated approach incorporating the relative increase and final coronary physiology with DC 24h may improve the prognostic efficacy of current models based on clinical variables in NSTE-ACS patients who underwent complete and successful PCI.
Methods
Patient Population
Patients with NSTE-ACS who underwent complete and successful PCI with adequate information of pre- and post-PCI QFR computation in the Department of Cardiology of Renmin Hospital of Wuhan University between January 2018 and November 2019 were retrospectively included. The diagnosis of NSTE-ACS was in accordance with the criteria of current guidelines (16), which include unstable angina pectoris (UA) and non-ST segment elevation myocardial infarction (NSTEMI). Complete and successful PCI was defined as the achievement of residual stenosis <20% and final thrombolysis in myocardial infarction (TIMI) flow grade 3. Patients with the following clinical conditions were excluded from the study: atrioventricular block, bundle branch blocks, pacemaker implantation, atrial fibrillation, atrial flutter, chronic coronary syndrome, acute ST-segment elevation myocardial infarction, hyperthyroidism, excessive alcohol intake, any malignancies, any systemic acute or chronic inflammation, use of any medications affecting autonomic function, scarcity of 24h Holter monitoring data, and nonstandard dual-antiplatelet therapy. Patients with the following coronary lesion characteristics which prevented QRS analysis were also excluded: prolonged occluded lesion, coronary bypass graft, left main coronary artery disease, coronary slow flow, unqualified coronary angiographic images including ostial lesion, myocardial bridge, severe vessel overlap or tortuosity at the stenotic segments, and poor coronary image quality where measurement of QFR was not applicable. The study was approved by the Ethics Committee of Renmin Hospital of Wuhan University (No. WDRY2021-K078) before the performance. The flowchart of patient enrollment is shown in Figure 1.
Holter Monitoring
Before the PCI procedure, all patients received Holter monitor examination for HRV analysis and derivation of DC 24h. Holter monitor data was analyzed as previously described (12, 17–20) to obtain the standard time-domain and frequency-domain parameters. Briefly, R peak detection was used to identify normal sinus RR intervals and then the standard deviation of all normal sinus RR intervals (SDNN), root mean square successive difference (RMSSD), and standard deviation average of NN intervals (SDANN) were calculated. PNN50 represents the percentage of the number of times that the difference between adjacent normal RR intervals is >50 ms over the total number of NN intervals. The high-frequency power (HF) was defined as high frequency spectra 0.15–0.4 Hz; low-frequency power (LF) as frequency spectra 0.04–0.15 Hz; and very low-frequency (VLF) as frequency spectra 0.003–0.04 Hz. Low-frequency/high-frequency (LF/HF) denotes the ratio of the parameters. Normalized LF and HF powers were calculated with the following equations: LFn = 100*LF/ (total power-VLF) and HFn = 100*HF/ (total power-VLF). DC 24h was calculated, following transformation of the RR intervals using phase-rectified signal averaging, by introducing anchor points (RR0) into the tachygram and generating a plot of all RR intervals recorded. Four-beat segments were defined as two beats prior to and two beats after the anchor points. The preceding RR-intervals, defined as RR-1 and RR-2, and the RR-interval following RR0 (RR + 1) were used in the analysis. The mean values of RR-2, RR-1, RR0 and RR + 1 were used in the equation DC 24h = [X (0) + X (1) – X (−1) – X (−2)] /4 to calculate DC 24h (12, 19, 20).
QFR Computation
For pre-PCI QFR and post-PCI QFR, analysis of all participants was performed offline and analyzed with the AngioPlus system (Pulse Medical Imaging Technology, Shanghai, China). Two selected views of the same coronary artery greater than 25°were transferred to the QFR system and the QFR was calculated by establishing the contrast flow model. Using a modified TIMI frame count method, the contrast flow rate was estimated from coronary angiography images and the contrast flow model was calculated.
In our study, the contrast-flow quantitative flow ratio (cQFR) was derived from routine coronary angiography of the target vessel that was most clinically relevant or with the most severe stenosis. The relative increase of QFR was calculated by %QFR increase with PCI [(post-PCI QFR–pre-PCI QFR) / pre-PCI QFR* 100]. Representative examples are shown in Figure 2.
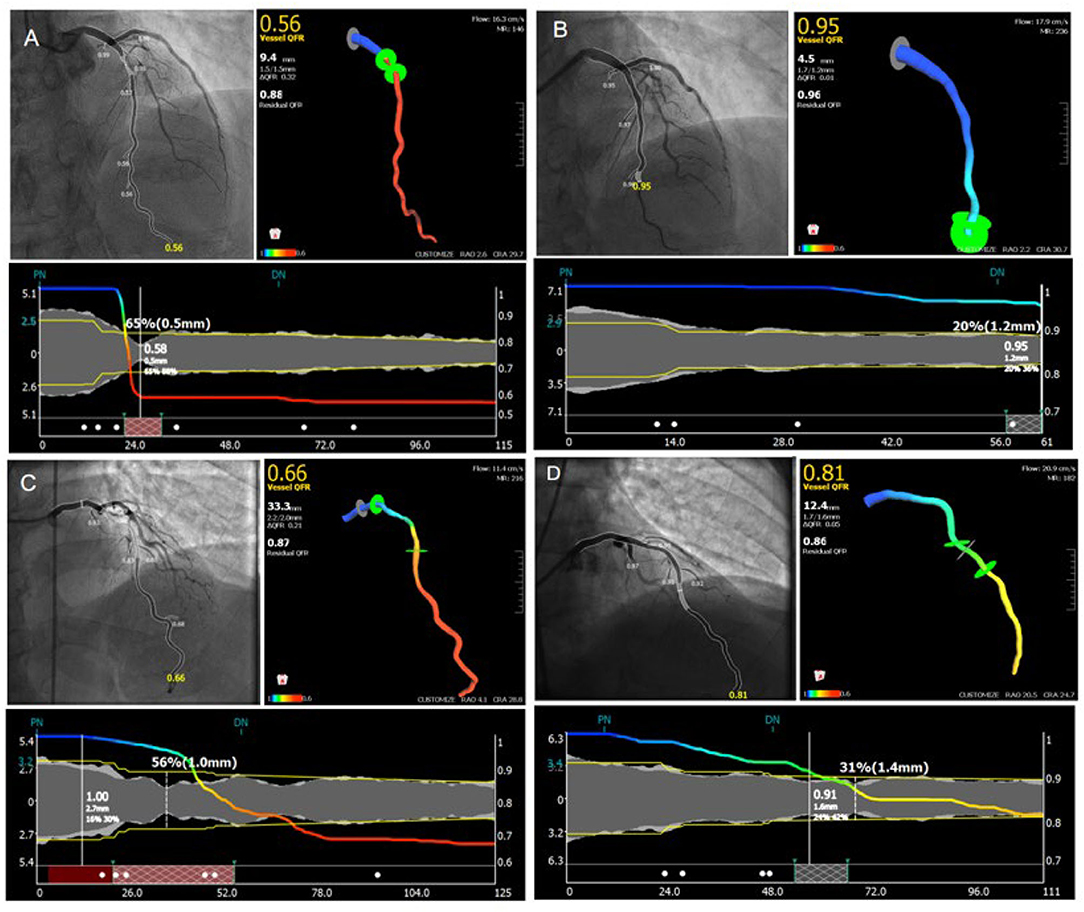
Figure 2. Representative images for cQFR analysis. (A,C) Representative images for the measuring of pre-percutaneous coronary intervention quantitative flow ratio. (B,D) Representative images for the measuring of post-percutaneous coronary intervention quantitative flow ratio.
Follow Up
Outcome data were obtained either by phone or by clinical visit after discharge. The incidence of major adverse cardiac and cerebrovascular events (MACCEs) during follow up was selected as the primary outcome, which was defined as a composite of cardiac mortality, stroke, revascularization and re-admission for UA. Cardiac mortality was defined as overall mortality from cardiac causes. Stroke was defined as fatal or non-fatal ischemic stroke. Revascularization was defined as revascularization on target or non-target vessels. Re-admission for UA was defined as a new admission for UA following discharge from the index hospitalization for successful PCI.
PCI was performed by an experienced senior interventional cardiologist according to standard procedures with a second-generation drug eluting stent (21). Each patient received a loading dose of either aspirin and clopidogrel prior to PCI. All patients were instructed to take aspirin indefinitely plus a P2Y12 inhibitor for at least 1 year after PCI in conformity with current guidelines with respect to recommended duration of drug therapy (21). After PCI, patients continued with optimized medical treatments and were followed up at clinics regularly after discharge.
Statistical Analysis
Continuous variables were presented as “mean ± SD” for normal distribution, and medians and interquartile ranges (IQRs) for skewed distribution. A chi-square (χ2) test was used to analyze the differences among categorical variables, and comparisons of means among multiple groups were performed with ANOVA. A Mann-Whitney U test or Kruskal-Wallis variance analysis was used for analyzing non-normal distribution. The Kaplan-Meier survival curve was used to analyze the potential associations between DC 24h, post-PCI QFR, and % QFR increase at baseline with the incidence of MACCEs in NSTE-ACS patients with stent placement. Univariate analysis was carried out first, followed by multivariate Cox regression analysis incorporating variables with significant findings in univariate analysis. The predictability of MACCEs using DC 24h, post PCI QFR, % QFR increase and LF/HF by ROC curve analysis. Moreover, comparisons were also performed to evaluate whether adding DC 24h, post PCI QFR and % QFR increase to the classic risk factors for cardiovascular disease could improve the predictive ability of the models. SPSS 23 was applied for the statistical analysis, with p < 0.05 indicating statistical significance.
Results
Patient Characteristics
A total of 240 patients with NSTE-ACS who underwent PCI were retrospectively included. The mean age of the patients was 62.8 years and 75.4% of them were male. Most (88.3%) of the patients had UA. During a mean follow-up of 21.3 months, 31 patients had MACCEs.
ROC Analyses for MACCEs
As shown in Figure 3, ROC analyses showed that post-PCI QFR, percent QFR increase, DC 24h and LF/HF were all potential predictors for MACCEs, with the optimized cutoff values of 0.88, 23%, 2.42, and 1.08 and the area under the ROC (AUC) of 0.784, 0.724, 0.703, and 0.676, respectively.
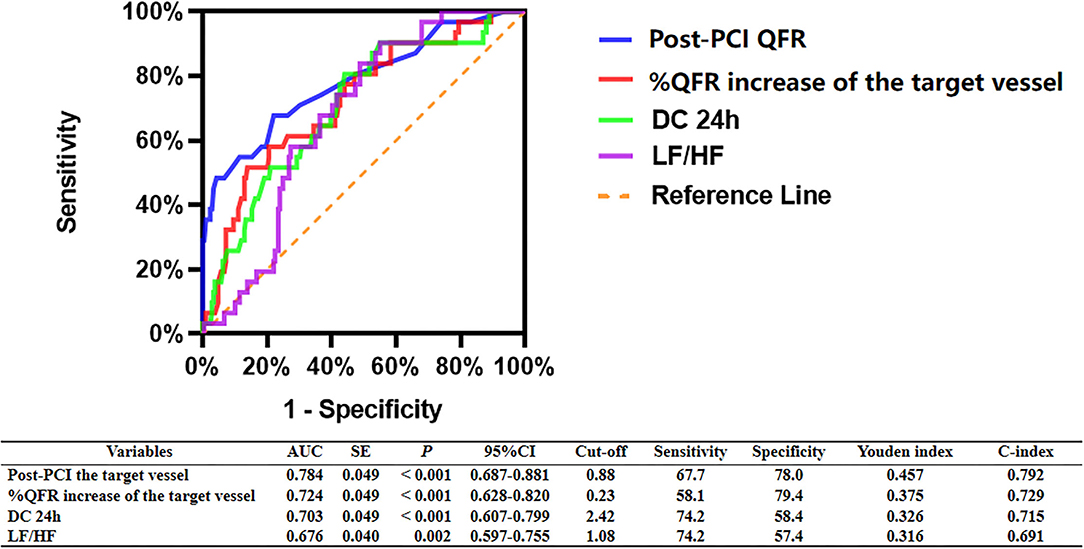
Figure 3. ROC analysis comparing the predictive efficacies of related variables for the incidence of MACCEs during follow up.
Patient and Target Vessel Characteristics According to DC 24h
As shown in Table 1, patients with lower DC 24h were more likely to be older (p < 0.05). Moreover, patients with low DC 24h had higher incidence of MACCEs, revascularization, and re-admission for UA compared to patients with high DC 24h (all p < 0.05; Table 2).
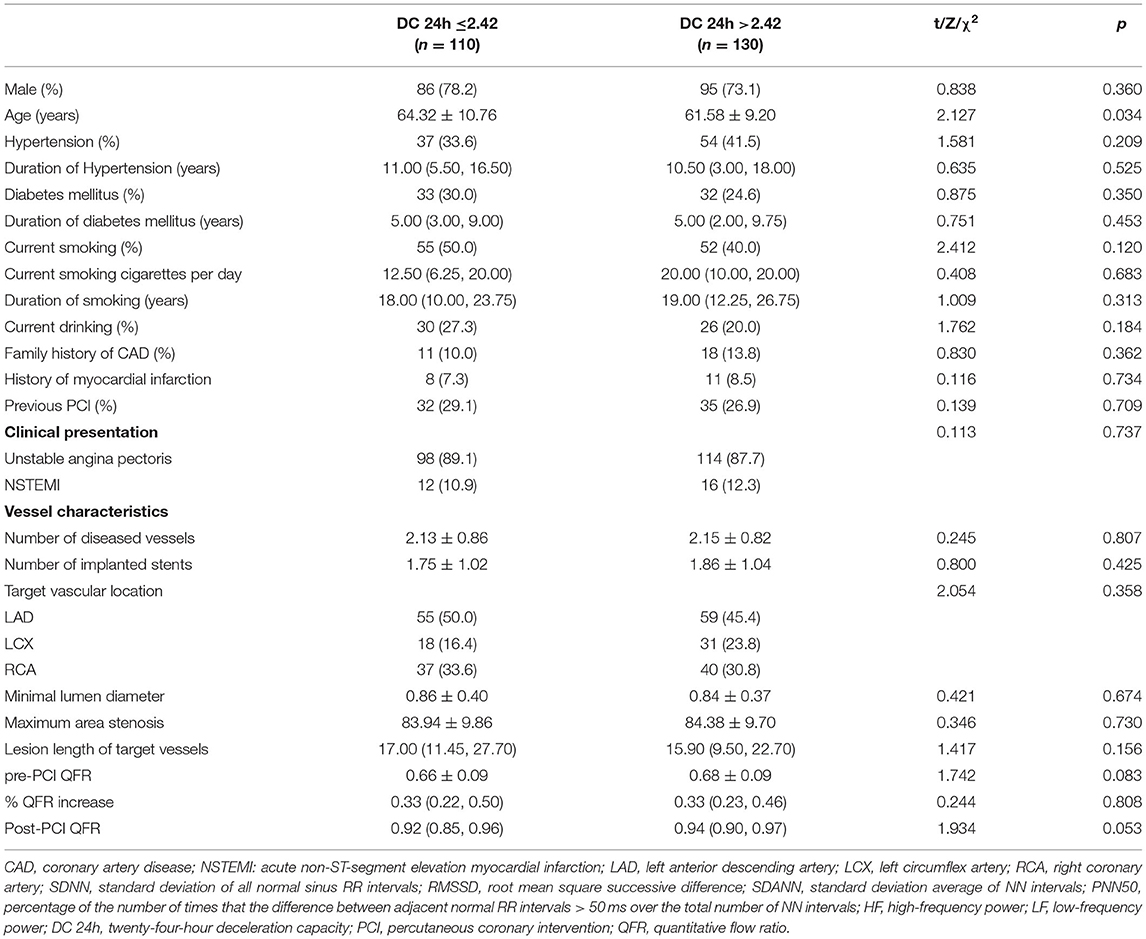
Table 1. Comparison of patient information and target vessel characteristics according to 24-hour deceleration capacity.
Patient and Target Vessel Characteristics According to Post-PCI QFR
As shown in Table 3, patients with a post-PCI QFR ≤ 0.88 were more likely to have target lesions of the left anterior descending coronary artery, lower LFn, LF/HF, DC 24h, and pre-PCI QFR, and a smaller relative QFR increase compared to patients with post-PCI QFR > 0.88 of the target vessels (all p < 0.05). Besides, patients with post-PCI QFR ≤ 0.88 had higher incidence of MACCEs, revascularization and re-admission for UA compared to those with post-PCI QFR > 0.88 of the target vessels (all p < 0.05; Table 4).
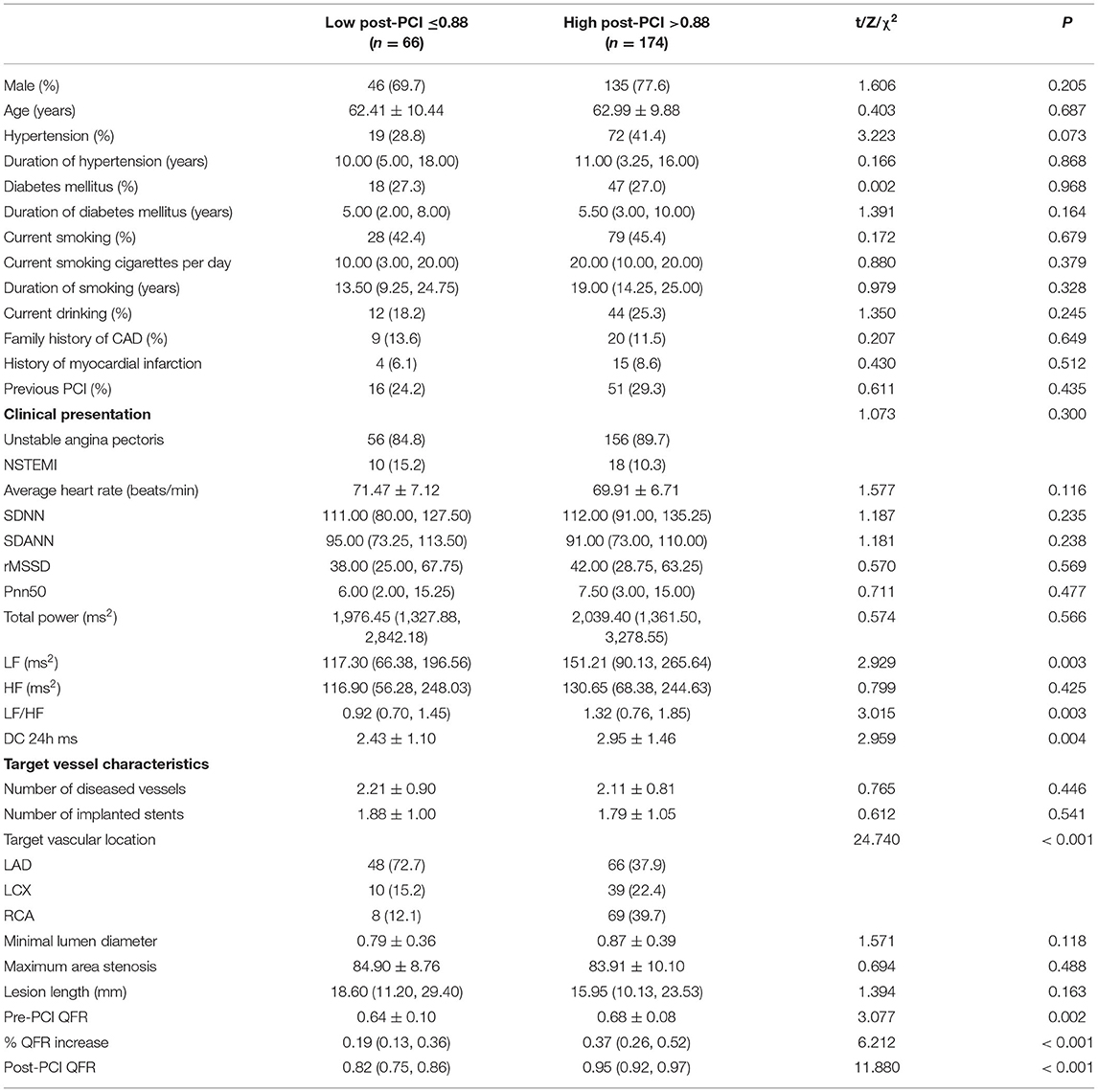
Table 3. Comparison of patient information and characteristics of target vessels in patients with NSTE-ACS according to post-PCI QFR of the target vessel.
Patient and Target Vessel Characteristics According to Relative QFR Increase
As shown in Table 5, patients with smaller %QFR increase were more likely to have lower LFn, LF/HF, maximum area stenosis of the target vessel, post-PCI QFR and higher pre-PCI QFR (all p < 0.05). Moreover, those with smaller %QFR increase had a higher incidence of MACCEs and re-admission for UA compared to patients with larger %QFR increase (all p < 0.05; Table 6).
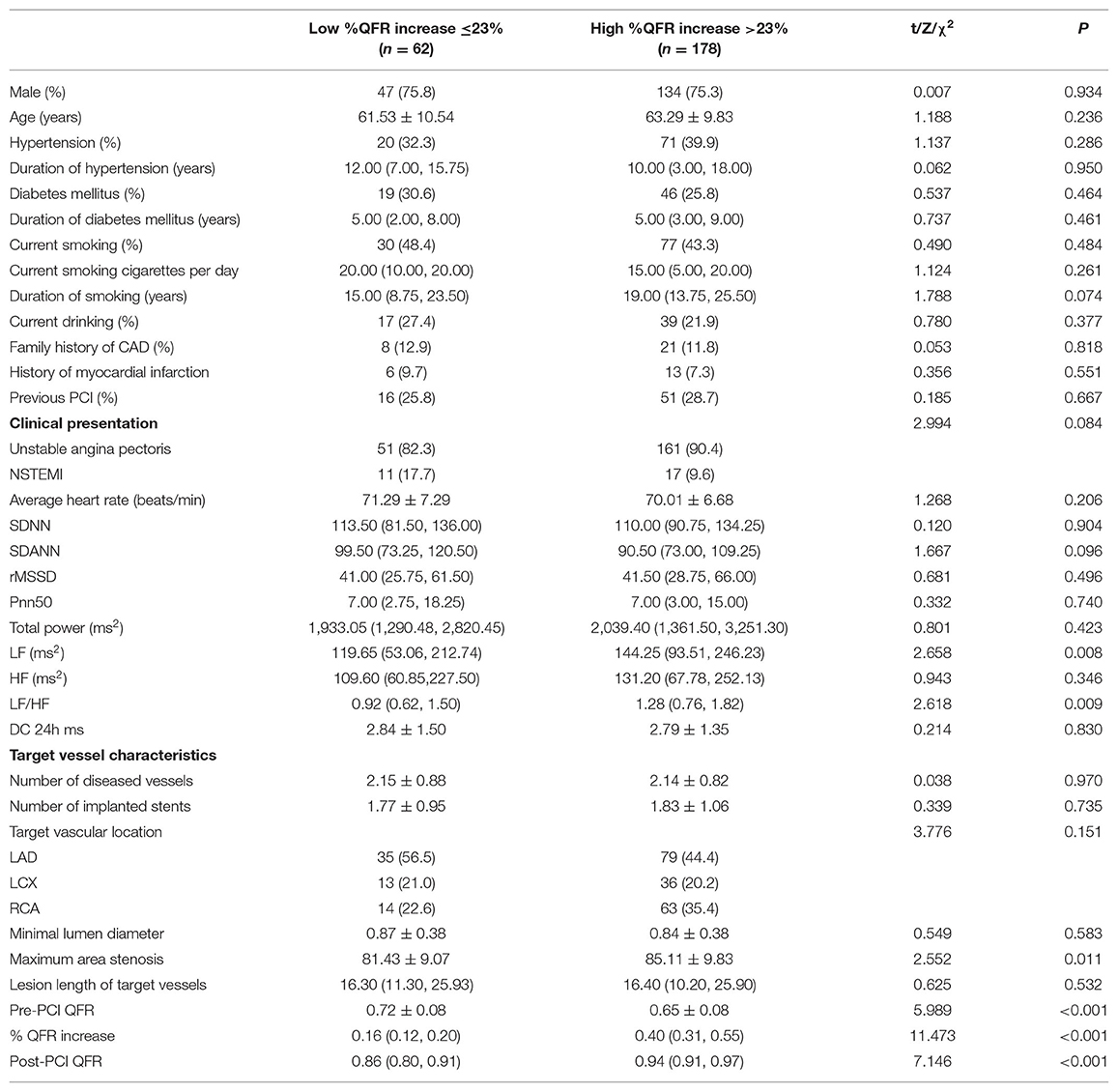
Table 5. Comparison of patient information and target vessel characteristics according to percent QFR increase of the target vessel.
Association Between Relative Increase and Final QFR, and DC for MACCEs
The Kaplan-Meier analyses showed that the incidence of MACCEs was significantly different between patients with lower and higher of DC 24h (cutoff: 2.42, χ2 = 11.531, p = 0.001, Figure 4), post-PCI QFR (cutoff: 0.88, χ2 = 31.159, p < 0.001, Figure 5), and %QFR increase of the target vessel (cutoff: 23%, χ2 = 20.420, p < 0.001, Figure 6). Results of multivariate Cox regression analyses suggested that hypertension (HR: 6.816; 95% CI: 2.986–15.559), LF/HF >1.08 (HR: 0.335; 95% CI: 0.144–0.779), DC 24h >2.42 ms (HR: 0.306; 95% CI: 0.134–0.701), pre-PCI QFR (HR: 0.527; 95% CI: 0.346–0.802), post-PCI QFR > 0.88 (HR: 0.318; 95% CI: 0.129–0.780) and relative increase percentage of QFR >23% (HR: 0.161; 95% CI: 0.066–0.391) were all independent predictors of MACCEs (Table 7, all p < 0.05).
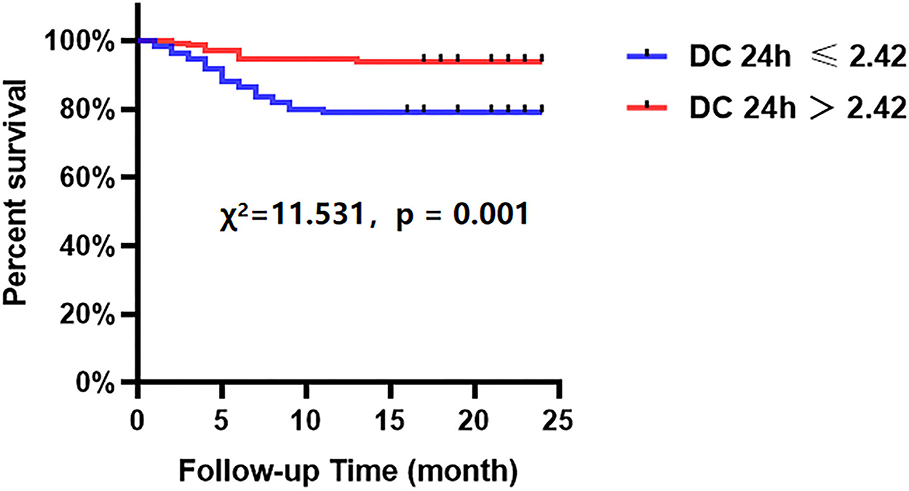
Figure 4. Cumulative event-free survival probability of MACCEs in patients with NSTE-ACS who underwent PCI according to the DC 24 h.
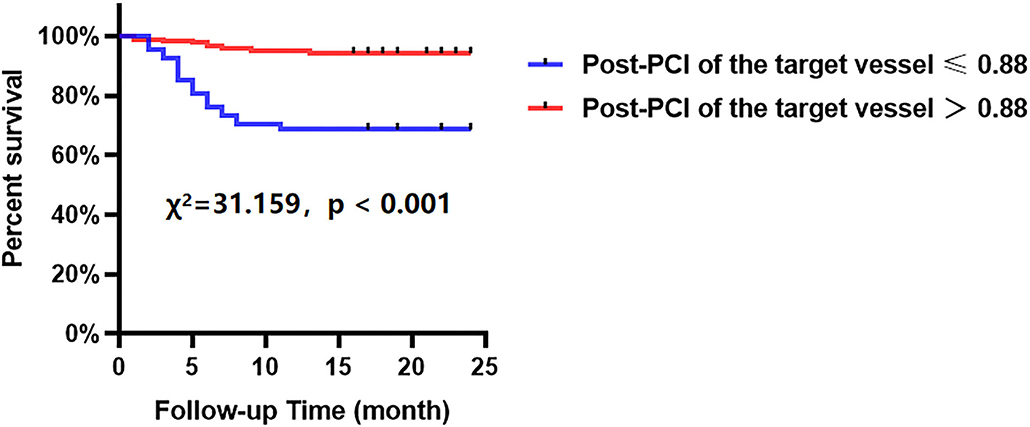
Figure 5. Cumulative event-free survival probability of MACCEs in patients with NSTE-ACS who underwent PCI according to post-PCI of the target vessel.
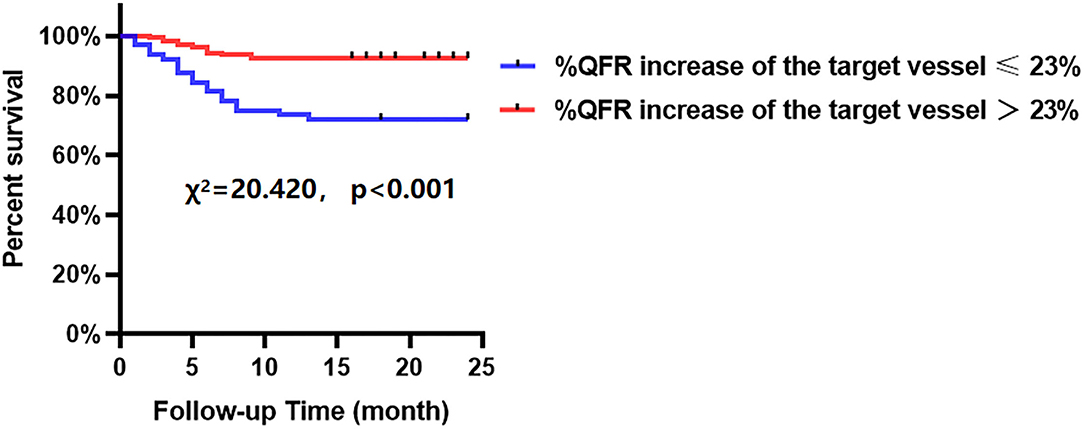
Figure 6. Cumulative event-free survival probability of MACCEs in patients with NSTE-ACS who underwent PCI according to %QFR increase of the target vessel.
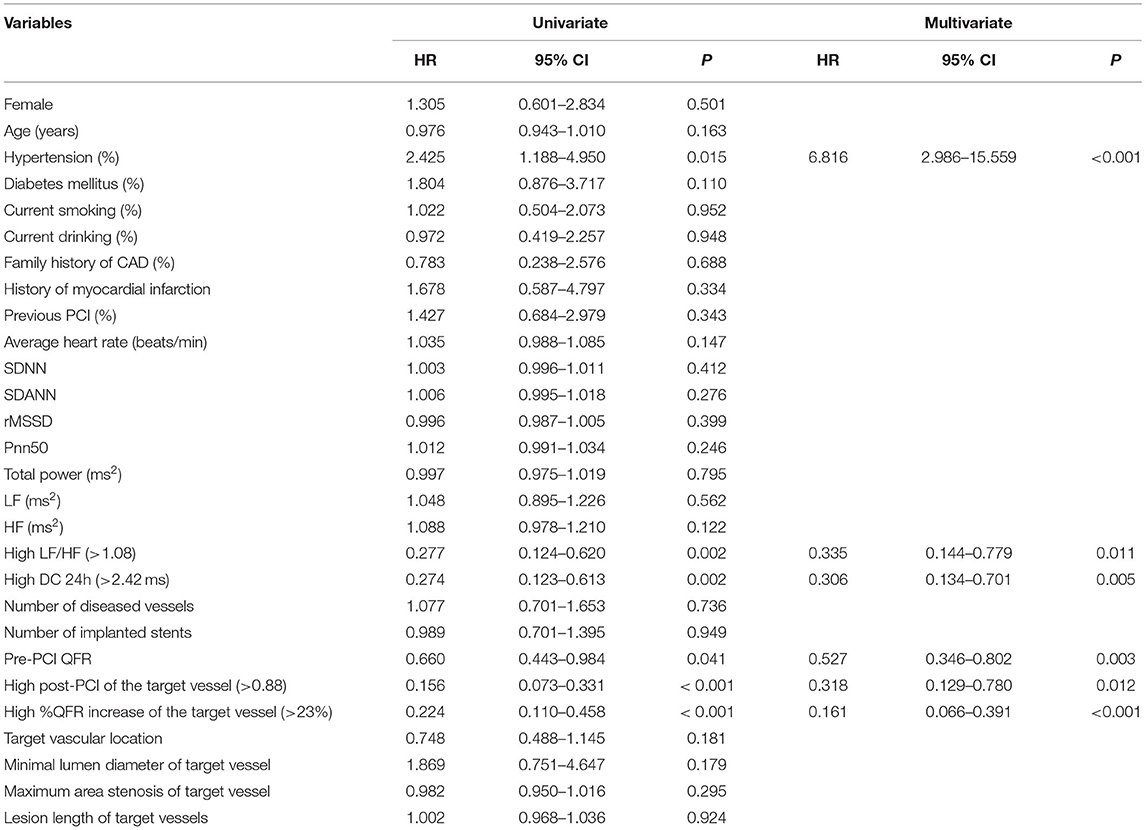
Table 7. Potential predictors for the incidence of MACCEs in patients with NSTE-ACS who underwent PCI.
Prognostic Implication of Relative Increase and Final QFR Combined With DC
As shown in Figure 7, incorporating post-PCI QFR (Model 2) significantly enhanced the ability to predict accurately the MACCEs compared with Model 1 which included traditional cardiovascular risk factors only (AUC: 0.858 versus 0.685). The predictive ability further increased in Model 3, which incorporated % QFR increase (AUC: 0.867; C-index: 0.881; Youden index: 0.665; sensitivity: 87.1%; specificity: 79.4%; p < 0.001). Moreover, Adding DC 24h ≤2.42 into Model 4 further improved the predictive efficacy of the model for MACCEs (AUC: 0.888; C-index: 0.903; Youden index: 0.684; sensitivity: 87.1%; specificity: 81.3%; CI: 0.829–0.947; p < 0.001, Figure 8).
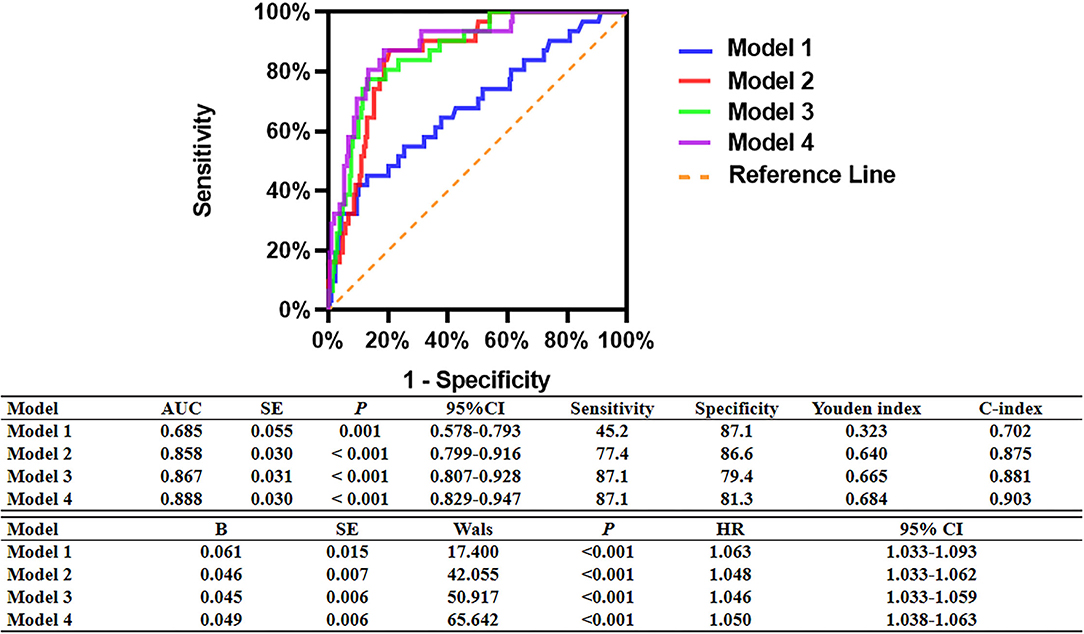
Figure 7. Comparison of the predictive capacity and accuracy of predictive models for MACCEs. Model 1: Age + Sex+ Hypertension + Diabetes mellitus + Current smoking + Family history of CAD + History of myocardial infarction. Model 2: Model 1 + Post-PCI QFR of the target vessel ≤0.88. Model 3: Model 2 + %QFR increase of the target vessel ≤23%. Model 4: Model 3 + DC 24 h ≤2.42 ms.
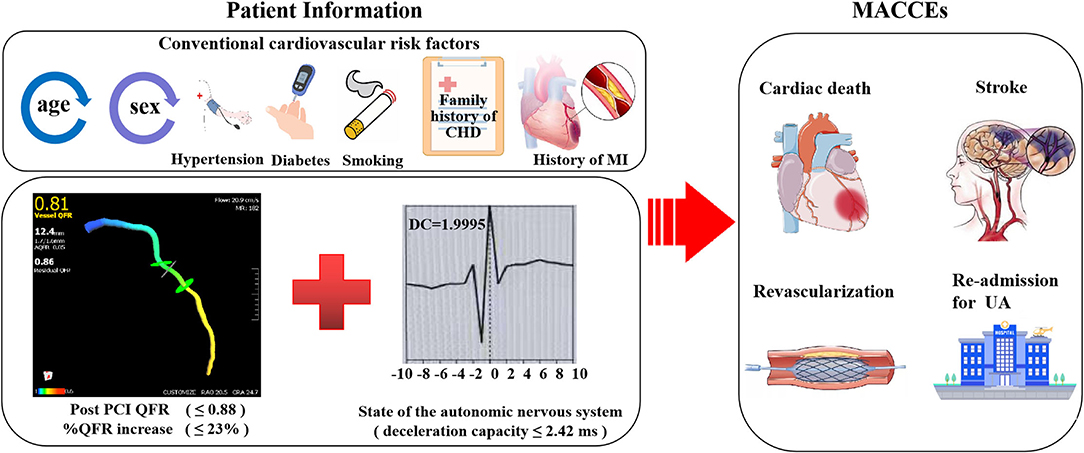
Figure 8. Combined efficacies of post-PCI QFR, %QFR increase and deceleration capacity for risk stratification in patients with NSTE-ACS.
As shown in Supplementary Figure 1, the C-index was 0.875 (95% CI: 0.799–0.916, p < 0.001) for prognostic model 5, containing model 1 plus post-PCI QFR of the target vessel ≤ 0.88, and 0.802 (95% CI: 0.695–0.877, p < 0.001) for model 6, containing model 1 plus %QFR increase of the target vessel ≤ 23%. For model 7, containing model 1 plus DC 24h ≤2.42 ms, the C-index was 0.793 (95% CI: 0.689–0.865, p < 0.001). These results suggest that model 5 is a more powerful predictor of MACCEs than %QFR increase of the target vessel ≤23% or DC 24h ≤2.42 ms.
Discussion
In this retrospective cohort study, we included patients with NSTEMI who underwent complete revascularization with PCI and who had adequate information on pre- and post-procedural QFR and Holter-derived HRV data. We found that lower post-PCI QFR (≤0.88), smaller % QFR increase (≤23%), and lower DC 24h (≤2.42) at baseline were all independent predictors for the risk of MACCEs during follow up. Moreover, incorporation of relative increase and final QFR combined with DC may improve the predictive efficacy of existing models based on clinical variables for MACCEs in patients with NSTE-ACS.
Risk stratification remains challenging in patients with NSTE-ACS, particularly for those after PCI (22, 23). Although multiple large observational studies have suggested a potential role of post-PCI FFR as a predictor for adverse events in the future, the optimal cutoff remains unknown and may be variable according to the different patient populations included (24–26). More importantly, FFR can only be obtained via invasive procedures with the assistance of an additional pressure guidewire and the use of adenosine, which significantly limits its use in clinical practice (27). In comparison, QFR, as a noninvasive angiographically-derived FFR measurement is more applicable in clinical settings. Previous studies have confirmed that QFR is highly consistent with FFR and could be used as a validated indicator of functional coronary stenosis (28, 29). A previous study that included 602 patients who underwent complete and successful revascularization with a mean follow-up of 629 days showed that lower values of QFR might be a risk factor for the increased incidence of vessel-oriented adverse events (5). Alternatively, a retrospective study that included 771 vessels with post-PCI QFR suggested a predictive efficacy and independent correlation between post-PCI QFR and long-term vessel-related clinical outcomes in state of the PCI practice (6). Our results, which showed a possible prognostic role of post-PCI QFR for MACCEs in NSTE-ACS patients after PCI, is consistent with the findings of these studies. Nevertheless, neither pre-PCI FFR nor post-PCI QFR alone could fully discriminate the degree of relative contribution of stented and non-stented segment disease burden. Therefore, in order to discriminate the relative contribution of each component of coronary artery lesions, previous studies showed that the percentage of increase of the FFR value before and after PCI has also been independently and significantly correlated with poor long-term prognosis (4), and integration of the concept of percent FFR increase and post-PCI FFR value allowed a better discrimination of high-risk patients after complete and successful PCI (4). In our study, significant associations were observed between percent QFR increase and post-PCI QFR with MACCEs after PCI. However, all these findings are focused on the local interaction between stents and targeted lesions. It could be hypothesized that incorporating parameters that indicated the systematic burden of CAD, such as systematic inflammation and autonomic dysregulation, may further improve the prognostic efficacy of models based on current clinical variables.
Previous studies showed that the ANS is directly involved in cardiovascular development (30–32). Previous ex vivo studies showed that the imbalance of ANS may be an early marker of acute cardiovascular disease events (33–36). In addition, ANS is shown to have key effects on vasoconstriction and vasodilation, which influence vessel physiology (13, 37). Alternatively, the mechanism underlying persistently lowered shear stress appears to be the formation of vulnerable plaque (38). However, there remains scant evidence from literature to indicate the potential association between ANS, hydrodynamic shear forces and the vulnerability of coronary plaque. Our results therefore support the hypothesis that imbalance in cardiac ANS may affect local hydrodynamic shear forces and lead to vulnerability of coronary lesions, and may therefore play a key role in the pathogenesis of acute coronary events. Interestingly, our previous studies showed a significant interaction between ANS and immune inflammation on coronary physiology evaluated by QFR (11, 39). These findings support the incorporation of ANS imbalance for risk stratification of patients with CAD. Notably, measurement of DC is refractory to external factors and premature beat, which could therefore objectively reflect the modulation of heart rate by the ANS and quantitatively analyze vagal nerve activity compared with HRV (12, 19, 20).
Our overall findings are in line with a previous prospective study including 2,111 patients with acute myocardial infarction, which demonstrated that impaired DC was a strong predictor of mortality after myocardial infarction, and the predictive efficacy of DC was even stronger than the conventional measures of HRV (12). In this study, we found that incorporation of relative increase and final QFR combined with DC is associated with stronger predictive efficacy of existing models based on clinical variables for MACCEs in patients with NSTE-ACS. Briefly, acute myocardial ischemia might impair cardiac vagal nerve and induce the production of inflammatory cytokines (14), and subsequently lead to endothelial dysfunction, lipid dysregulation, vascular smooth muscle cell activation, macrophage infiltration, thus accelerating coronary artery plaque rupture of residual lesions and effects on the burden of coronary heart disease (40, 41). Although the exact molecular pathways remain to be determined, the results of our study support the incorporation of relative increase and final QFR combined with DC to improve the predictive efficacy of existing models based on clinical variables for MACCEs in patients with NSTE-ACS.
Study Limitations
Due to the retrospective nature of the study, possible selection bias may overestimate the predictive value of DC 24h, post-PCI QFR or %QFR increase for MACCEs, particularly with the small sample size. In order to validate the predictive value of these parameters, prospective cohort studies with larger samples sizes will be required. Also, we evaluated the DC only once, on patient admission. It is unclear whether DC monitored for multiple times would be more valid as a predictor of MACCEs. In addition, it should be mentioned that the post-PCI QFR or %QFR increase has not yet been validated against post-PCI FFR or %FFR increase. Studies are warranted for further confirmation and optimization of the software and protocols for QFR measurement. Moreover, our findings might not be generalizable to other institutions due to the differences between offline and online analyses. Nevertheless, the concept regarding that “higher is better” for DC 24hr, post-PCI QFR or %QFR increase when undergoing complete revascularization with PCI is beyond doubt. In addition, intravascular ultrasound or optical coherence tomography may be considered to provide detailed assessment of culprit lesions and non-culprit lesions of the patients, and it might also improve the prognostic efficacy if parameters related to these examinations are incorporated. Due to methodological reasons, DC could not be applied to ACS patients with atrial fibrillation or other non-sinus rhythms. Finally, no inflammatory markers were analyzed because we did not measure these markers in the included patients.
Conclusions
In conclusion, the results of our study showed that the relative increase and final coronary physiology combined with DC may improve the predictive efficacy of existing models based on clinical variables for MACCEs in NSTE-ACS patients who underwent complete and successful PCI. These results support the incorporation of relative increase and final coronary physiology combined with DC for risk stratification in NSTE-ACS patients, although validation will require larger prospective studies.
Data Availability Statement
The datasets used and/or analyzed during this study are available from the corresponding author on reasonable request. Requests to access these datasets should be directed to LY, bGlsZWl5dSYjeDAwMDQwO3dodS5lZHU=.cn or HJ, aG9uZy1qaWFuZyYjeDAwMDQwO3dodS5lZHU=.cn.
Ethics Statement
The studies involving human participants were reviewed and approved by the Ethics Committee of Renmin Hospital of Wuhan University (No. WDRY2021-K078). The Ethics Committee waived the requirement of written informed consent for participation.
Author Contributions
LY and HJ: substantial contributions to conception and design, data acquisition, and data analysis and interpretation. JW, CL, FG, ZZ, HC, LZ, YW, HZ, ZL, SD, JS, QD, and SX: drafting the article or critically revising it for important intellectual content. JW, CL, and FG: final approval of the version to be published and agreement to be accountable for all aspects of the work in ensuring that questions related to the accuracy or integrity of the work are appropriately investigated and resolved. All authors contributed to the article and approved the submitted version.
Funding
This work was supported by the National Natural Science Foundation of China (81871486, 81970287, and 82100530).
Conflict of Interest
The authors declare that the research was conducted in the absence of any commercial or financial relationships that could be construed as a potential conflict of interest.
Publisher's Note
All claims expressed in this article are solely those of the authors and do not necessarily represent those of their affiliated organizations, or those of the publisher, the editors and the reviewers. Any product that may be evaluated in this article, or claim that may be made by its manufacturer, is not guaranteed or endorsed by the publisher.
Supplementary Material
The Supplementary Material for this article can be found online at: https://www.frontiersin.org/articles/10.3389/fcvm.2022.848499/full#supplementary-material
Supplementary Figure 1. Comparison of the predictive capacity and accuracy of relative increase and final QFR and DC for MACCEs. Model 5: Model 1 + Post-PCI QFR of the target vessel ≤0.88. Model 6: Model 1 + %QFR increase of the target vessel ≤23%. Model 7: Model 1 + DC 24h ≤2.42 ms.
Abbreviations
DC, deceleration capacity; DC 24h, 24-hour deceleration capacity; QFR, quantitative flow ratio; ACS, acute coronary syndrome; CAD, coronary artery disease; NSTE-ACS, non-ST-elevation ACS; NSTEMI, acute non-ST-segment elevation myocardial infarction; LAD, left anterior descending artery; LCX, left circumflex artery; RCA, right coronary artery; PCI, percutaneous coronary intervention; MACCEs, major adverse cardiac and cerebrovascular events; ROC, receiver operating characteristic; HR, hazard ratio; CI, confidence interval; ANS, automatic nervous system; SDNN, standard deviation of all normal sinus RR intervals; RMSSD, root mean square successive difference; SDANN, standard deviation average of NN intervals; PNN50, percentage of the number of times that the difference between adjacent normal RR intervals >50 ms over the total number of NN intervals; HF, high-frequency power; LF, low-frequency power.
References
1. Tonino PA, De Bruyne B, Pijls NH, Siebert U, Ikeno F, van' t Veer M, et al. Fractional flow reserve versus angiography for guiding percutaneous coronary intervention. N Engl J Med. (2009) 360:213–24. doi: 10.1056/NEJMoa0807611
2. De Bruyne B, Fearon WF, Pijls NH, Barbato E, Tonino P, Piroth Z, et al. Fractional flow reserve-guided PCI for stable coronary artery disease. N Engl J Med. (2014) 371:1208–17. doi: 10.1056/NEJMoa1408758
3. Xaplanteris P, Fournier S, Pijls NHJ, Fearon WF, Barbato E, Tonino PAL, et al. Five-year outcomes with PCI guided by fractional flow reserve. N Engl J Med. (2018) 379:250–9. doi: 10.1056/NEJMoa1803538
4. Lee JM, Hwang D, Choi KH, Rhee TM, Park J, Kim HY, et al. Prognostic implications of relative increase and final fractional flow reserve in patients with stent implantation. JACC Cardiovasc Interv. (2018) 11:2099–109. doi: 10.1016/j.jcin.2018.07.031
5. Biscaglia S, Tebaldi M, Brugaletta S, Cerrato E, Erriquez A, Passarini G, et al. Prognostic value of QFR measured immediately after successful stent implantation: the international multicenter prospective HAWKEYE study. JACC Cardiovasc Interv. (2019) 12:2079–88. doi: 10.1016/j.jcin.2019.06.003
6. Kogame N, Takahashi K, Tomaniak M, Chichareon P, Modolo R, Chang CC, et al. Clinical implication of quantitative flow ratio after percutaneous coronary intervention for 3-vessel disease. JACC Cardiovasc Interv. (2019) 12: 2064–75. doi: 10.1016/j.jcin.2019.08.009
7. Kogame N, Ono M, Kawashima H, Tomaniak M, Hara H, Leipsic J, et al. The impact of coronary physiology on contemporary clinical decision making. JACC Cardiovasc Interv. (2020) 13:1617–38. doi: 10.1016/j.jcin.2020.04.040
8. Ridker PM, Everett BM, Thuren T, MacFadyen JG, Chang WH, Ballantyne C, et al. Antiinflammatory therapy with canakinumab for atherosclerotic disease. N Engl J Med. (2017) 377:1119–31. doi: 10.1056/NEJMoa1707914
9. Goldfine AB, Shoelson SE. Therapeutic approaches targeting inflammation for diabetes and associated cardiovascular risk. J Clin Invest. (2017) 127:83–93. doi: 10.1172/JCI88884
10. Hinterdobler J, Schott S, Jin H, Meesmann A, Steinsiek AL, Zimmermann AS, et al. Acute mental stress drives vascular inflammation and promotes plaque destabilization in mouse atherosclerosis. Eur Heart J. (2021) 19:ehab371. doi: 10.1093/eurheartj/ehab371
11. Wang J, Liu W, Chen H, Liu C, Wang M, Chen H, et al. Novel insights into the interaction between the autonomic nervous system and inflammation on coronary physiology: a quantitative flow ratio study. Front Cardiovasc Med. (2021) 8:700943. doi: 10.3389/fcvm.2021.700943
12. Bauer A, Kantelhardt JW, Barthel P, Schneider R, Mäkikallio T, Ulm K, et al. Deceleration capacity of heart rate as a predictor of mortality after myocardial infarction: cohort study. Lancet. (2006) 367:1674–81. doi: 10.1016/S0140-6736(06)68735-7
13. Raab S, Plate KH. Different networks, common growth factors: shared growth factors and receptors of the vascular and the nervous system. Acta Neuropathol. (2007) 113:607–26. doi: 10.1007/s00401-007-0228-3
14. Borovikova LV, Ivanova S, Zhang M, Yang H, Botchkina GI, Watkins LR, et al. Vagus nerve stimulation attenuates the systemic inflammatory response to endotoxin. Nature. (2000) 405:458–62. doi: 10.1038/35013070
15. James JM, Mukouyama YS. Neuronal action on the developing blood vessel pattern. Semin Cell Dev Biol. (2011) 9:1019–27. doi: 10.1016/j.semcdb.2011.09.010
16. Mendis S, Thygesen K, Kuulasmaa K, Giampaoli S, Mähönen M, Ngu Blackett K, et al. World Health Organization definition of myocardial infarction:2008-09 revision. Int J Epidemiol. (2011) 40:139–46. doi: 10.1093/ije/dyq165
17. El Aarbaoui T, Méline J, Brondeel R, Chaix B. Short-term association between personal exposure to noise and heart ratevariability: the RECORD MultiSensor Study. Environ Pollut. (2017) 231:703–11. doi: 10.1016/j.envpol.2017.08.031
18. Xhyheri B, Manfrini O, Mazzolini M, Pizzi C, Bugiardini R. Heart rate variability today. Prog Cardiovasc Dis. (2012) 55:321–31. doi: 10.1016/j.pcad.2012.09.001
19. Kantelhardt JW, Bauer A, Schumann AY, Barthel P, Schneider R, Malik M, et al. Phase-rectified signal averaging for the detection of quasi-periodicities and the prediction of cardiovascular risk. Chaos. (2007) 17:015112. doi: 10.1063/1.2430636
20. Liu Q, Chen YF, Fan SZ, Abbod MF, Shieh JS. Quasi-periodicities detection using phase-rectified signal averaging in EEG signals as a depth of anesthesia monitor. IEEE Trans Neural Syst Rehabil Eng. (2017) 25:1773–84. doi: 10.1109/TNSRE.2017.2690449
21. Authors/Task Force members., Windecker S, Kolh P, Alfonso F, Collet JP, Cremer J, et al. 2014 ESC/EACTS Guidelines on myocardial revascularization: the Task Force on Myocardial Revascularization of the European Society of Cardiology (ESC) and the European Association for Cardio-Thoracic Surgery (EACTS) developed with the special contribution of the European Association of Percutaneous Cardiovascular Interventions (EAPCI). Eur Heart J. (2014) 35:2541–619. doi: 10.1093/eurheartj/ehu278
22. Amsterdam EA, Wenger NK, Brindis RG, Casey DE Jr, Ganiats TG, Holmes DR Jr, et al. 2014 AHA/ACC guideline for the management of patients with non-ST-elevation acute coronary syndromes: a report of the American College of Cardiology/American Heart Association Task Force on Practice Guidelines. J Am Coll Cardiol. (2014) 64:e139–228. doi: 10.1016/j.jacc.2014.09.016
23. Tziakas D, Chalikias G, Al-Lamee R, Kaski JC. Total coronary occlusion in non ST elevation myocardial infarction: Time to change our practice? Int J Cardiol. (2021) 329:1–8. doi: 10.1016/j.ijcard.2020.12.082
24. Johnson NP, Tóth GG, Lai D, Zhu H, Açar G, Agostoni P, et al. Prognostic value of fractional flow reserve: linking physiologic severity to clinical outcomes. J Am Coll Cardiol. (2014) 64:1641–54. doi: 10.1016/j.jacc.2014.07.973
25. Li SJ, Ge Z, Kan J, Zhang JJ, Ye F, Kwan TW, et al. Cutoff value and longterm prediction of clinical events by FFR measured immediately after implantation of a drug-eluting stent in patients with coronary artery disease: 1-to 3-year results from the DKCRUSH VII registry study. J Am Coll Cardiol Intv. (2017) 10:986–95. doi: 10.1016/j.jacc.2017.09.197
26. Piroth Z, Toth GG, Tonino PAL, Barbato E, Aghlmandi S, Curzen N, et al. Prognostic value of fractional flow reserve measured immediately after drug-eluting stent implantation. Circ Cardiovasc Interv. (2017) 10:e005233. doi: 10.1161/CIRCINTERVENTIONS.116.005233
27. De Maria GL, Garcia-Garcia HM, Scarsini R, Hideo-Kajita A, Gonzalo López N, Leone AM, et al. Novel Indices of Coronary Physiology: Do We Need Alternatives to Fractional Flow Reserve? Circ Cardiovasc Interv. (2020) 13:e008487. doi: 10.1161/circinterventions.119.008487
28. Xu B, Tu S, Song L, Jin Z, Yu B, Fu G, et al. Angiographic quantitative flow ratio-guided coronary intervention (FAVOR III China): a multicentre, randomised, sham-controlled trial. Lancet. (2021) 3:S0140-6736(21)02248-0. doi: 10.1016/S0140-6736(21)02248-0
29. Xu B, Tu S, Qiao S, Qu X, Chen Y, Yang J, et al. Diagnostic accuracy of angiography-based quantitative flow ratio measurements for online assessment of coronary stenosis. J Am Coll Cardiol. (2017) 70:3077–87. doi: 10.1016/j.jacc.2017.10.035
30. Honma Y, Araki T, Gianino S, Bruce A, Heuckeroth R, Johnson E, et al. Artemin is a vascular-derived neurotropic factor for developing sympathetic neurons. Neuron. (2002) 35:267–82. doi: 10.1016/S0896-6273(02)00774-2
31. Zukowska Z, Grant DS, Lee EW. Neuropeptide Y: a novel mechanism for ischemic angiogenesis. Trends Cardiovasc Med. (2003) 13:86–92. doi: 10.1016/S1050-1738(02)00232-3
32. Pan L, Tang J, Liu H, Cheng B. Sympathetic nerves: how do they affect angiogenesis, particularly during wound healing of soft tissues?. Clin Hemorheol Microcirc. (2016) 2:181–91. doi: 10.3233/CH-152019
33. Meng G, Zhou X, Wang M, Zhou L, Wang Z, Wang M, et al. Gut microbe-derived metabolite trimethylamine N-oxide activates the cardiac autonomic nervous system and facilitates ischemia-induced ventricular arrhythmia via two different pathways. EBioMedicine. (2019) 44:656–64. doi: 10.1016/j.ebiom.2019.03.066
34. Yu L, Wang Y, Zhou X, Huang B, Wang M, Li X, et al. Leptin injection into the left stellate ganglion augments ischemia-related ventricular arrhythmias via sympathetic nerve activation. Heart Rhythm. (2018) 15:597–606. doi: 10.1016/j.hrthm.2017.12.003
35. Yu L, Huang B, Zhou X, Wang S, Wang Z, Wang M, et al. Renal sympathetic stimulation and ablation affect ventricular arrhythmia by modulating autonomic activity in a cesium-induced long QT canine model. Heart Rhythm. (2017) 14:912–9. doi: 10.1016/j.hrthm.2017.02.010
36. Yu L, Huang B, Po SS, Tan T, Wang M, Zhou L, et al. Low-level tragus stimulation for the treatment of ischemia and reperfusion injury in patients with ST-segment elevation myocardial infarction: a proof-of-concept study. JACC Cardiovasc Interv. (2017) 10:1511–20. doi: 10.1016/j.jcin.2017.04.036
37. Florea VG, Cohn JN. The autonomic nervous system and heart failure. Circ Res. (2014) 114:1815–26. doi: 10.1161/CIRCRESAHA.114.302589
38. Pedrigi RM, Poulsen CB, Mehta VV, Ramsing Holm N, Pareek N, Post AL, et al. Inducing persistent flow disturbances accelerates atherogenesis and promotes thin cap fibroatheroma development in D374Y-PCSK9 hypercholesterolemic minipigs. Circulation. (2015) 132:1003–12. doi: 10.1161/CIRCULATIONAHA.115.016270
39. Liu C, Yu Z, Chen H, Wang J, Liu W, Zhou L, et al. Relationship between immunoinflammation and coronary physiology evaluated by quantitative flow ratio in patients with coronary artery disease. Front Cardiovasc Med. (2021) 2021:1223. doi: 10.3389/fcvm.2021.714276
Keywords: deceleration capacity, non-ST-elevation acute coronary syndrome (NSTE-ACS), coronary physiology, major adverse cardiac and cerebrovascular events (MACCEs), quantitative flow ratio (QFR)
Citation: Wang J, Liu C, Guo F, Zhou Z, Zhou L, Wang Y, Chen H, Zhou H, Liu Z, Duan S, Sun J, Deng Q, Xu S, Jiang H and Yu L (2022) Deceleration Capacity Improves Prognostic Accuracy of Relative Increase and Final Coronary Physiology in Patients With Non-ST-Elevation Acute Coronary Syndrome. Front. Cardiovasc. Med. 9:848499. doi: 10.3389/fcvm.2022.848499
Received: 04 January 2022; Accepted: 04 February 2022;
Published: 22 March 2022.
Edited by:
Jinwei Tian, The Second Affiliated Hospital of Harbin Medical University, ChinaReviewed by:
Xiaoxiang Yan, Shanghai Jiao Tong University, ChinaTong Liu, Tianjin Medical University, China
Copyright © 2022 Wang, Liu, Guo, Zhou, Zhou, Wang, Chen, Zhou, Liu, Duan, Sun, Deng, Xu, Jiang and Yu. This is an open-access article distributed under the terms of the Creative Commons Attribution License (CC BY). The use, distribution or reproduction in other forums is permitted, provided the original author(s) and the copyright owner(s) are credited and that the original publication in this journal is cited, in accordance with accepted academic practice. No use, distribution or reproduction is permitted which does not comply with these terms.
*Correspondence: Hong Jiang, aG9uZy1qaWFuZyYjeDAwMDQwO3dodS5lZHUuY24=; Lilei Yu, bGlsZWl5dSYjeDAwMDQwO3dodS5lZHUuY24=
†These authors have contributed equally to this work