- 1Department of Emergency, The First Affiliated Hospital of Wannan Medical College, Wuhu, China
- 2Department of Cardiology, The First Affiliated Hospital of Wannan Medical College, Wuhu, China
Significant reductions in the incidence of cardiac arrhythmia (CA) and sudden cardiac death (SCD), along with amelioration of heart failure, have been reported for treatment with Sacubitril/valsartan (SV). However, its anti-arrhythmic mechanism remains unclear. The current study aims to explore the anti-arrhythmic molecular mechanism of SV. The direct protein targets (DPT) of SV were extracted from DrugBank. The protein-protein interaction (PPI) network of SV DPTs was constructed using STRING, and the indirect protein targets (IPTs) were also identified. A search for arrhythmia-related genes was conducted using GeneCards and the Comparative Toxicogenomics Database (CTD). The DTPs, ITPs, and arrhythmia-related genes from the two datasets were combined in a Venn diagram, and the overlapping genes were identified as core target genes. The Gene Ontology (GO) and Kyoto Encyclopedia of Genes and Genomes (KEGG) enrichment analyses identified the top 20 biological processes and signaling pathways related to disease and the therapeutic effects of SV. The renin-angiotensin system, adrenergic signaling in cardiomyocytes, and gap junction pathways are strongly implicated in the effects of SV on CA. In conclusion, our bioinformatics analyses provided evidence pertaining to the possible antiarrhythmic mechanisms of SV and may contribute to the development of novel drugs for CA.
Introduction
Cardiac arrhythmia (CA) is a group of heterogeneous diseases that often leads to abnormal pulse formation and conduction due to the mutation of potassium and sodium channels (1). Almost all antiarrhythmic drugs that are used to treat CA have arrhythmogenic effects. Amiodarone, a broad-spectrum antiarrhythmic drug, has limited long-term suitability in patients with heart failure (HF) due to its extracardiac toxicity (2). Ideal antiarrhythmic drugs can prevent serious arrhythmias, effectively control the ectopic rhythm, restore or maintain sinus rhythm, and have no toxic effects on hemodynamics and vital organs (3). The search continues for better antiarrhythmic drugs.
Sacubitril/valsartan (SV), as an angiotensin receptor-neprilysin inhibitor (ARNI), positively improves the imbalance between the rein-angiotensin-aldosterone-system (RAAS) and natriuretic peptide systems (4), and decreases rehospitalization rates and cardiovascular mortality of patients with HF compared with enalapril (5). More surprisingly, SV decreases the incidence of ventricular tachycardia, ventricular fibrillation, implantable cardioverter-defibrillator discharge, and SCD in patients with HF (6–8). Recent studies have shown that inflammation, fibrosis, and autoimmune mechanisms may also lead to electrical remodeling or ion channel dysfunction and promote arrhythmia (9–11). Drugs that inhibit myocardial remodeling (12), such as ARNI, may play antiarrhythmic roles by reducing the formation of ectopic impulses and reentry from the upstream mechanism (13). However, the controversy around SV remains: it increases the incidence of ventricular arrhythmias, especially in patients with ischemic heart disease (14, 15), although after adjusting the outcomes, the cardiovascular death caused by etiologies of HF such as ischemic, non-ischemic, and hypertensive cardiomyopathies are similar (16, 17). But, in men with ejection fraction of <35%, patients who recently took SV may be more prone to ventricular arrhythmias (18). The data between the antiarrhythmic effect of SV and the etiology, gender differences, and age categories of HF are still limited (4). Therefore, the relationship between SV and CA deserves further study. The question of whether SV has a direct or indirect antiarrhythmic effect or has a pro-arrhythmic effect, as with other antiarrhythmic drugs, remains to be answered.
Network pharmacology is a vital tool to indicate interactions between drugs and organisms. Drug pleiotropy may be considered in the context of interactions between the regulatory network of the drug target and the disease’ gene product. Thus, network analyses give insights into multiple actions of drugs and their mechanisms (19). This approach has been used successfully to clarify the multi-targeted regulation of Traditional Chinese Medicine for treating disease (20).
The current study investigates the molecular mechanisms by which SV ameliorates CA using the approach of network pharmacology. The resulting bioinformatics data may assist with basic research and enable the development of new drugs for CA.
Materials and Methods
Recognition of Direct Protein Targets of Sacubitril/Valsartan
Drugbank is a network database containing comprehensive molecular information about drugs, their mechanisms, interactions, and targets. Since it was first released in 2006, Drugbank has been widely used to promote drug target discovery, design, docking, and screening and to predict metabolism and interactions in addition to general pharmaceutical education. A large number of new data have been added to DrugBank 5.0, including information on pharmacometabolomics, pharmacotranscriptomics, and pharmacoprotoemics, as well as hundreds of new drug clinical trials (21). The direct protein targets (DPTs) of SV were derived from DrugBank 5.0.
Protein-Protein Interaction Network and Signaling Pathways for the Direct Protein Targets of Sacubitril/Valsartan
Search Tool for the Retrieval of Interacting Genes (STRING)1 is an online database for searching known protein-protein interaction (PPI) networks. Cytoscape is an open-source software project, which integrates biomolecular interaction networks with high-throughput expression data and other molecular states, producing a unified conceptual framework (22). The PPI network of the DPTs of SV was constructed using STRING. No more than 50 interactors on the first and second shells were set as the cut-off criteria with the highest confidence (score = 0.9). Highly relevant genes obtained were used as IPTs, and then, IPTs and DPTs were visualized by Cytoscape (v.3.8.2).
Human Cardiac Arrhythmia-Related Genomic Data Sources
GeneCards2 is a searchable database that provides comprehensive and user-friendly information on all annotated and predicted human genes. Arrhythmia-related genes were searched within GeneCards and 4,317 results were obtained. The top 400 genes were selected as the research object according to the ‘‘correlation score’’ ranking. The Comparative Toxicogenomics Database (CTD)3 is a powerful and open database designed to promote an understanding of how environmental exposure affects human health. It provides artificially curated information on chemical–gene/protein interactions, chemical–disease, and gene-disease relationships. A total of 383,061 results were obtained by searching “disease and arrhythmia” within the CTD database. Repeated genes were removed according to the descending order of “inference score” and the top 400 genes were selected as research objects.
Obtaining Potential Therapeutic Target Genes for Sacubitril/Valsartan Concerning Human Arrhythmia
The DPTs and IPTs of SV and CA-related genes from the GeneCards and CTD databases were introduced into a Venn diagram.4 Through integration and intersection, potential therapeutic target genes of SV for arrhythmia were obtained.
Establishing Protein-Protein Interaction Network, Gene Ontology, and Kyoto Encyclopedia of Genes and Genomes Enrichment Analyses of Target Genes and Network Construction for Drug-Target Genes-Disease
Overlapping genes for the drug and disease were imported into the STRING database and a PPI network was constructed. The screening conditions were the species, “Homo sapiens” and a combined score of >0.4. A PPI diagram was constructed in which each node represented a gene and the nodes were connected by lines. The GO and KEGG were used to enrich and analyze overlapping genes using Omicshare tools,5 a free online data analysis platform.
Network relationships of core target-related GO/KEGG enrichment of SV against CA were generated via Cytoscape software (v.3.8.2). The study design is shown in Figure 1.
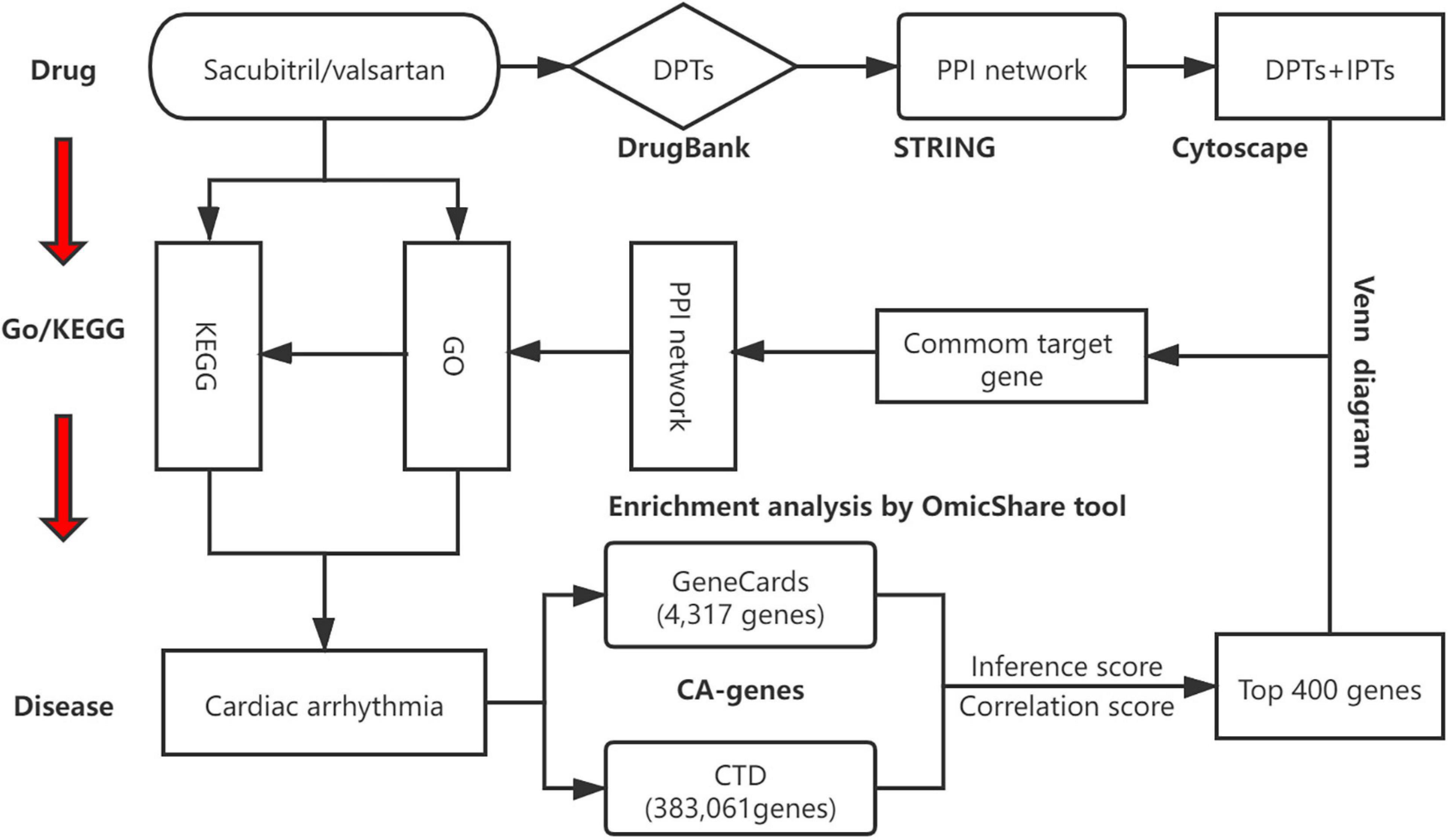
Figure 1. A schematic diagram showing the network pharmacological method for identifying targets, PPI network, biological processes, and key pathways of SV acting on cardiac arrhythmia (CA). All known targets of Sacubitril/valsartan (SV) and CA were obtained through online databases. Anti-arrhythmia targets of SV were identified. After constructing a protein-protein interaction (PPI) network and determining the potential targets of SV concerning CA, gene ontology (GO) and Kyoto Encyclopedia of Genes and Genomes (KEGG) enrichment analyses were carried out. Finally, the SV-GO-KEGG-CA network was generated. CTD: comparative toxicology genomics database.
Results
Identification of Direct Protein Targets of Sacubitril/Valsartan
The SV was described as a neprilysin inhibitor and anti-hypertensive agent in output DB09292 and DB00177 from DrugBank5.0. We identified 6 DPTs of SV (Table 1). These were MME (Neprilysin), SLCO1B3 (Solute carrier organic anion transporter family member 1B3), SLCO1B1 (Solute carrier organic anion transporter family member 1B1), AGTR1 (Type-1 angiotensin II receptor), CYP2C9 (Cytochrome P450 family 2 subfamily C member 9), and ABCC2 (Canalicular multispecific organic anion transporter 1).
Construction of a Protein-Protein Interaction Network for the Direct Protein Targets of Sacubitril/Valsartan and Further Searches for Closely Related Indirect Target Proteins
Six target genes for SV were input into the STRING database. A PPI network was obtained after selecting “Homo sapiens” and a medium confidence of >0.4, as shown in Figure 2. There were 6 nodes and 6 edges in this network with a local clustering coefficient of 0.889. Conditions of not more than 50 interactors at 1st and 2nd shell, with the highest confidence of >0.9, were imposed and 105 DPTs and IPTs identified with 105 nodes and 493 edges (local clustering coefficient: 0.65; PPI enrichment p < 1 e-16) was entered into Cytoscape (3.8.2) to rebuild a PPI network of SV’s target genes, as shown in Figure 3.
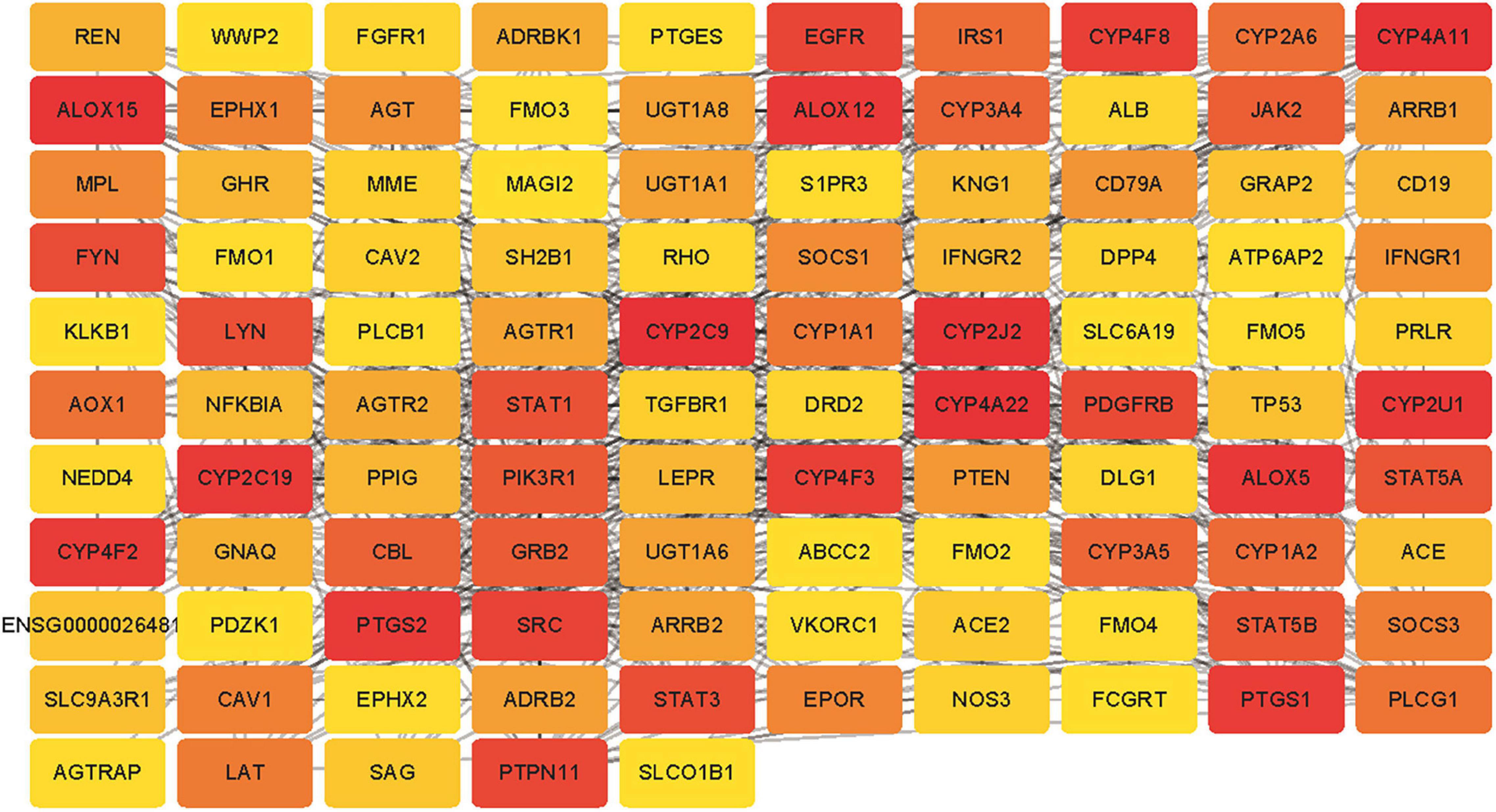
Figure 3. The PPI network for the DPTs and indirect protein targets (IPTs) of SV was constructed using STRING and no more than 50 interactors on the first and second shell were set as the cut-off criteria with the highest confidence (score = 0.9). The IPTs and DPTs were visualized by Cytoscape (v.3.8.2). The red to yellow rectangles represent scores from high to low.
Identification of Target Genes Related to Cardiac Arrhythmia and Identification of Potential Therapeutic Target Genes for Sacubitril/Valsartan in Human Arrhythmia
GeneCards were searched for CA-related genes and 4,317 results were obtained. The top 400 genes were selected as the research object according to the “correlation score” ranking. A search of “disease and arrhythmia” within the CTD database yielded 383,061 results. Repeated genes were removed according to the descending order of “inference score” and the top 400 genes were selected as the research object and analyzed using an online Venn diagram along with 105 DPTs and IPTs for SV. Seven core targets, ACE (Angiotensin I converting enzyme), CAV1 (Caveolin1), AGT (Angiotensinogen), REN (Renin), ADRB2 (Adrenoreceptor β2), TP53 (Tumor Protein P53), and ALB (Albumin) were identified from the intersections (Figure 4A).
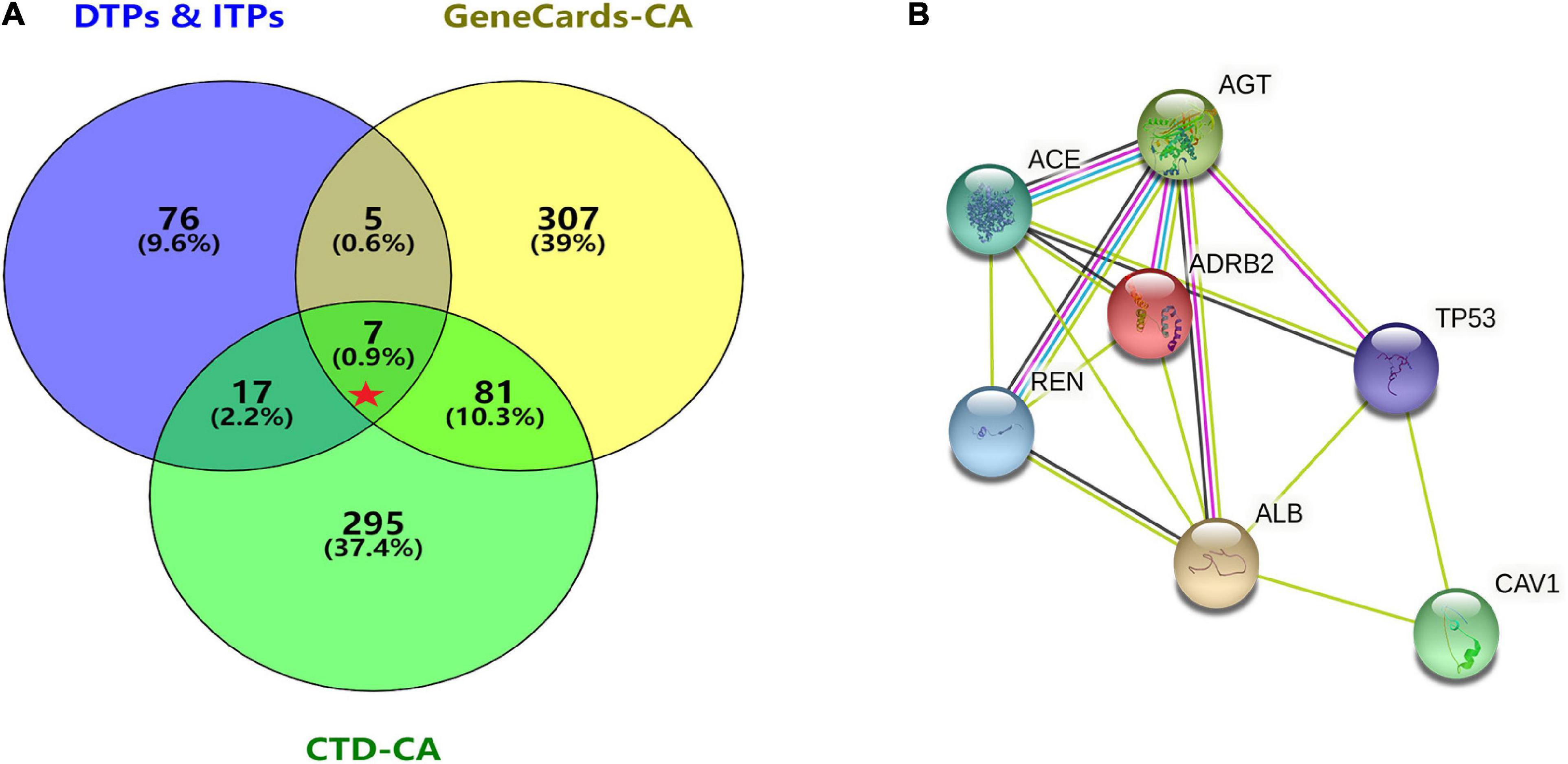
Figure 4. Acquisition of core target proteins and protein-protein interaction network. (A) SV-related drug target proteins [DTPs and indirect target proteins (ITPs)] were obtained from Drugbank Data and STRING. The top 400 CA-related genes were selected from GeneCards and CTD. The intersection of drug target protein genes (DTPs and ITPs) and the top two CA-related genes were selected by the Venn diagram. There were seven core target proteins (Red Pentagram). (B) A PPI network of the antiarrhythmic targets of SV was generated by the STRING database with medium confidence (0.400).
Establishing a Protein-Protein Interaction Network, Gene Ontology, and Kyoto Encyclopedia of Genes and Genomes Enrichment Analyses of Core Target Genes and Network of Drug-Target Genes-Disease
The seven core target genes identified by the above method were imported into the STRING database and a PPI network was constructed (medium confidence: 0.400; Figure 4B). Overlapping genes were enriched and analyzed using GO and KEGG via OmicShare tools. Enriched molecular functions included signaling receptor binding, potassium channel regulator activity, copper ion binding, β2-adrenergic receptor activity, type 2 angiotensin receptor binding, and inward rectifier potassium channel inhibitor activity, among others (Figure 5A); Enriched biological processes included tissue remodeling, regulation of blood volume by renin-angiotensin, regulation of systemic arterial blood pressure mediated by a chemical signal, gap junction assembly, regulation of gap junction assembly, and regulation of systemic arterial blood pressure, among others (Figures 5B,C). The enriched molecular signaling pathways of the core targets were involved in renin secretion, renin-angiotensin system, hypertrophic cardiomyopathy, fluid shear stress, and atherosclerosis adrenergic signaling in cardiomyocytes, in addition to others (Figure 6). The network of the core targets for SV with therapeutic potential for CA and interaction diagrams of the core target related pathways were constructed (Figure 7).
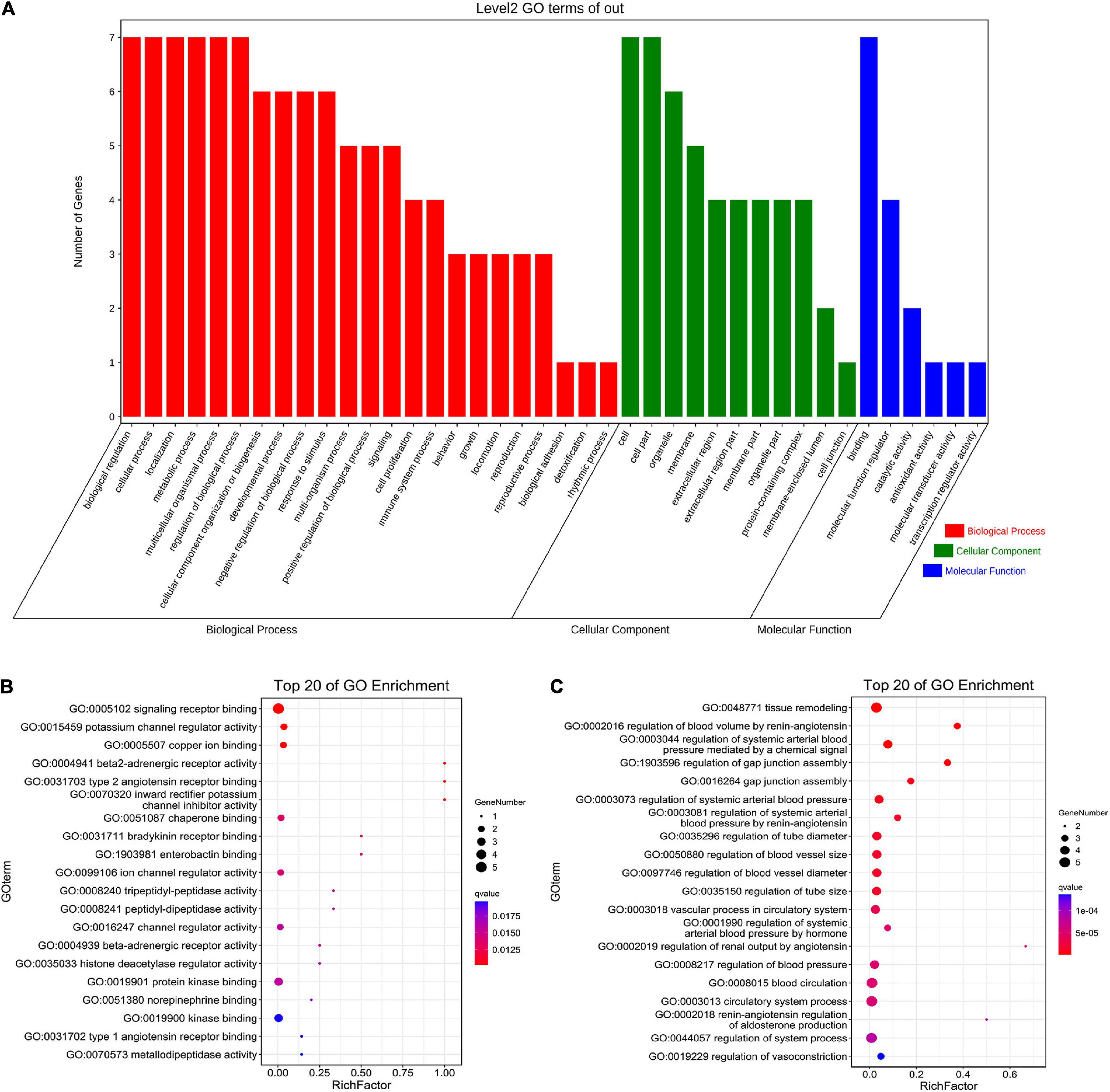
Figure 5. GO enrichment analysis of seven target genes (ACE, CAV1, AGT, REN, ADRB2, TP53, and ALB) for anti-arrhythmias with SV. (A) Biological process, cellular component and molecular function of Go enrichment of the target genes. (B,C) The bubble diagram shows the top 20 enriched molecular function and biological process respectively.
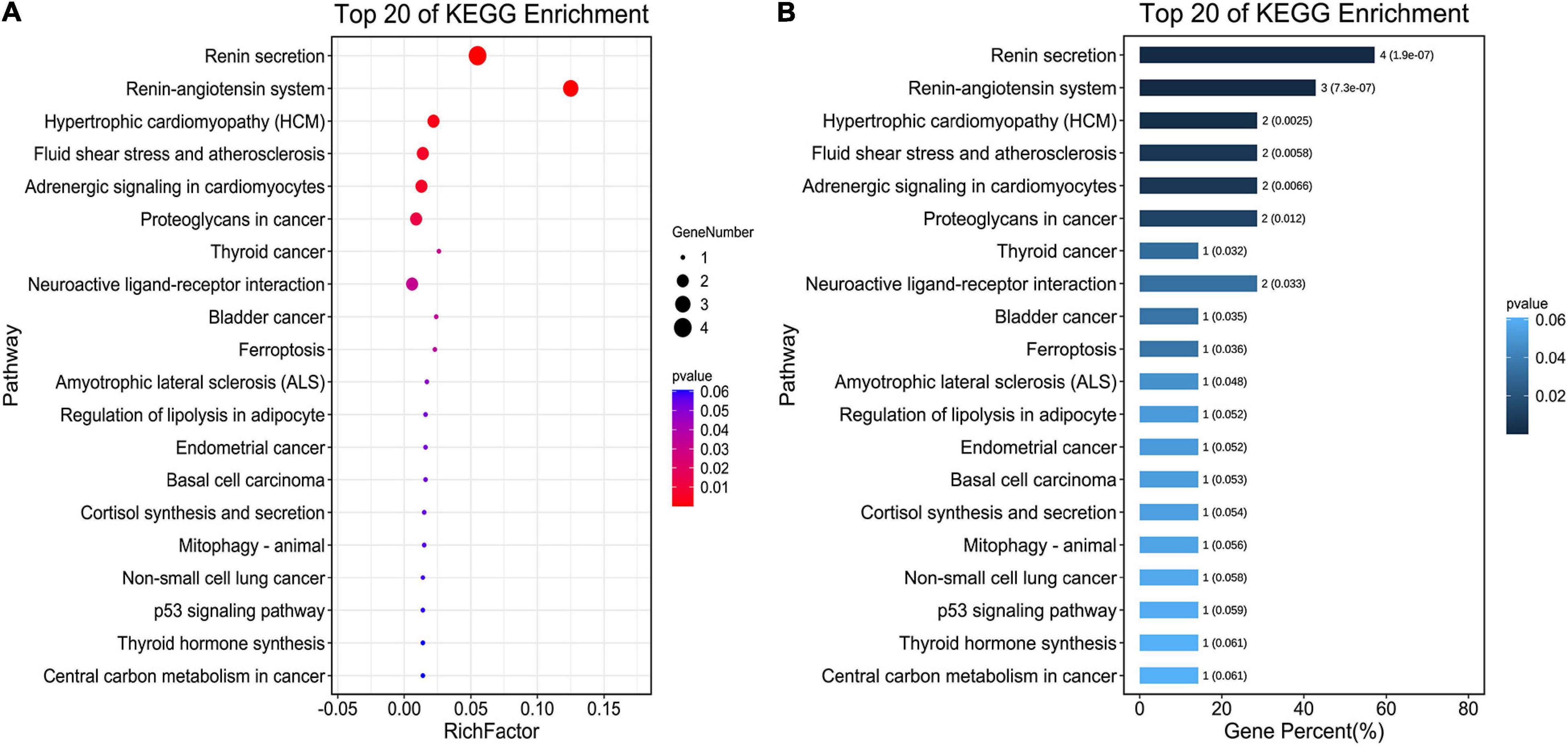
Figure 6. Pathway enrichment analyses of seven target genes (ACE, CAV1, AGT, REN, ADRB2, TP53, and ALB) for the treatment of CA with SV. (A) The bubble chart shows the top 20 enriched KEGG pathways. The x-axis indicates the Enrichment Factor and the intensity of different colors indicates the adjusted p-value. (B) The histogram shows the top 20 enriched KEGG pathways. The x-axis represents the enriched Gene Percentage (%) and the intensity of different colors indicates the adjusted p-value.
Discussion
The question addressed by the present study was whether SV has an antiarrhythmic or proarrhythmic effect in patients with HF. Using a network pharmacology approach, the current study reveals that SV, acting on core target genes (ACE, CAV1, AGT, REN, TP53, ADRB2, and ALB), has both direct and indirect effects during the treatment of CA.
The occurrence and maintenance of CA are usually mediated by ectopic activity and re-entry. The prolongation of the action potential/shortening of the effective refractory period and structural remodeling leads to slow and heterogeneous conduction, which provides the basis for reentrant arrhythmia. It was found that inhibiting the over-activation of RAAS reversed myocardial remodeling (23), reduced myocardial fibrosis and myocardial heterogeneous conduction. The formation of reentry and the occurrence of arrhythmia were both reduced (6, 7, 24), indicating an antiarrhythmic role. The current study showed that SV regulates ACE, CAV1, AGT, REN, and ADRB2 expression, inhibits tissue remodeling, and regulates vascular tension and volume by inhibiting the pathway of RAAS. In addition to myocardial remodeling, the remodeling of gap junctions is central to the generation and maintenance of arrhythmias. Gap junction remodeling leads to uncoupling of the myocardium, affects the conduction of the myocardial current, and generates arrhythmia. The SV may reduce the ventricular arrhythmias induced by myocardial hypertrophy by acting on the gap junction pathway (25, 26). Therefore, SV plays an indirect antiarrhythmic role by reversing myocardial and gap junction remodeling. Interestingly, angiotensin-converting enzyme inhibitor (ACEI) also reversed myocardial and gap remodeling, but did not reduce the incidence of SCD (27), suggesting that the antiarrhythmic effect of SV maybe also be related to neprilysin inhibition.
A decompensated state of HF is also represented by the over-activation of the SNS and is associated with poor prognosis, contributing to increased mortality risk. Neprilysin inhibition has been shown to activate the neuropeptide system, including that of atrial natriuretic peptide and brain natriuretic peptide, and synergistically inhibit SNS activity with valsartan (28). One pilot study showed that after taking SV for 2 months, the burst frequency and income of muscle sympathetic nerve activity decreased significantly, while heart rate and blood pressure did not change (29). It is by this strong antagonistic effect on the SNS that SV can reduce the incidence of SCD in patients with HF with reduced ejection fraction, an action that ACEI are unable to achieve (8). The β-adrenergic receptor antagonists are effective in reducing the damaging effects of increased SNS activity and produce significant mortality benefits for patients with HF. The ADRB2 gene encodes the β2-adrenergic receptor. Researchers have found that ADRB2 strongly increases localized RyR2-related cAMP levels during the over-activation of the SNS associated with cardiac hypertrophy or HF and increases ventricular arrhythmias (30). The ADRB2 also increases infiltration by pro-inflammatory macrophages, induces the production of IL-18, and sequentially stimulates the expression of connexin43 (Cx43) in fibroblasts in a paracrine manner, resulting in gap junction remodeling, myocardial fibrosis, and deterioration of cardiac function (31). The current findings that SV regulates ADRB2 expression suggest that the drug plays a direct antiarrhythmic role through pathways of hypertrophic cardiology and adrenergic signaling in cardiomyocytes.
Furthermore, the current study also found that SV regulates the levels of cav1 and TP53 and plays an antiarrhythmic role through the fluid shear stress and atherosclerosis pathway. Atrial fibrillation was associated with decreased CAV1 in right and left atria (p = 0.03); Loss of CAV1 leads to cSrc tyrosine kinase activation, gap junction remodeling, and ventricular arrhythmia, slowing left ventricular conduction velocity, and increasing ventricular arrhythmia inducibility (32). Further studies have shown that, when cardiac RAAS activity is enhanced, the dissociation of CAV1 from cSrc leads to cSrc activation, impaired gap junction function, and increased tendency toward ventricular arrhythmia and sudden cardiac death (33). These findings indicate that SV targets the regulation of CAV1 and reduces arrhythmia risk in heart disease associated with the activation of RAAS. Increased fibrosis and activated TP53 signaling were demonstrated in heart tissue from patients with dilated cardiomyopathy disease with ventricular tachycardia (34).
Any molecular mechanisms, where SV promotes arrhythmia, were not found in the current study. Clinical reports have indicated that SV can increase the incidence of ventricular arrhythmias in patients with HF (8, 14), but with short follow-up times and small sample sizes. Etiology, gender differences, and inclusion of high-risk patients may also affect the results (4). Further basic and clinical research into SV is needed to clarify whether the drug has proarrhythmic effects.
Conclusion
In summary, a network pharmacological approach was used to demonstrate that SV acted on seven core target genes (ACE, CAV1, AGT, REN, TP53, ADRB2, and ALB) and reversed myocardial and gap junction remodeling improved the imbalance of RAAS, SNS, and neuropeptide system and had an indirect and direct antiarrhythmic effect. The most enriched pathways were renin secretion, renin-angiotensin system, hypertrophic cardiomyopathy, fluid shear stress and atherosclerosis, and adrenergic signaling in cardiomyocytes. The current study may further guide the basic and clinical investigations on the antiarrhythmic or proarrhythmic effects of SV on CA and contribute to the discovery of ideal antiarrhythmic drugs.
Limitations
We acknowledge some limitations of the current study. Firstly, SV was decomposed into two separate components, Sacubitril and Valsartan, and the combination was not studied leading to some potential experimental deviations by comparison with the clinically administered formula. Secondly, targets for SV with potential antiarrhythmic effects were collected using public databases, which may lead to some inaccuracies. Thirdly, the core targets identified in this study require further validation via both basic scientific and clinical studies.
Data Availability Statement
The datasets presented in this study can be found in online repositories. The names of the repository/repositories and accession number(s) can be found in the article/supplementary material.
Author Contributions
CJ designed and performed the research. YZ wrote and revised the manuscript. SR and ST analyzed the public data and revised the manuscript. All authors read and approved the final manuscript.
Funding
This study was supported by the key specialty project of Anhui Province Medical and Health.
Conflict of Interest
The authors declare that the research was conducted in the absence of any commercial or financial relationships that could be construed as a potential conflict of interest.
Publisher’s Note
All claims expressed in this article are solely those of the authors and do not necessarily represent those of their affiliated organizations, or those of the publisher, the editors and the reviewers. Any product that may be evaluated in this article, or claim that may be made by its manufacturer, is not guaranteed or endorsed by the publisher.
Acknowledgments
We would like to express their gratitude to EditSprings (https://www.editsprings.cn/) for the expert linguistic services provided.
Footnotes
- ^ https://string-db.org/cgi/input.pl
- ^ https://www.genecards.org/
- ^ http://ctdbase.org/
- ^ https://bioinfogp.cnb.csic.es/tools/venny/
- ^ www.omicshare.com/tools
References
1. Ng GA. Feasibility of selection of antiarrhythmic drug treatment on the basis of arrhythmogenic mechanism – relevance of electrical restitution, wavebreak and rotors. Pharmacol Ther. (2017) 176:1–12. doi: 10.1016/j.pharmthera.2016.10.002
2. Opie LHSP. Dronedarone in high-risk permanent atrial fibrillation. N Engl J Med. (2012) 366:1159. doi: 10.1056/NEJMc1200742
3. Muhiddin KATP. Is there an ideal antiarrhythmic drug? A review – with particular reference to class I antiarrhythmic agents. Postgrad Med J. (1985) 61:665–78. doi: 10.1136/pgmj.61.718.665
4. Abumayyaleh M, El-Battrawy I, Behnes M, Borggrefe M, Akin I. Current evidence of sacubitril/valsartan in the treatment of heart failure with reduced ejection fraction. Future Cardiol. (2020) 16:227–36. doi: 10.2217/fca-2020-0002
5. Docherty KF, Vaduganathan M, Solomon SD, McMurray JJV. Sacubitril/valsartan: neprilysin inhibition 5 years after PARADIGM-HF. JACC Heart Fail. (2020) 8:800–10. doi: 10.1016/j.jchf.2020.06.020
6. Li LY, Lou Q, Liu GZ, Lv JC, Yun FX, Li TK, et al. Sacubitril/valsartan attenuates atrial electrical and structural remodelling in a rabbit model of atrial fibrillation. Eur J Pharmacol. (2020) 881:173120. doi: 10.1016/j.ejphar.2020.173120
7. Martens P, Nuyens D, Rivero-Ayerza M, Van Herendael H, Vercammen J, Ceyssens W, et al. Sacubitril/valsartan reduces ventricular arrhythmias in parallel with left ventricular reverse remodeling in heart failure with reduced ejection fraction. Clin Res Cardiol. (2019) 108:1074–82. doi: 10.1007/s00392-019-01440-y
8. Vicent L, Méndez-Zurita F, Viñolas X, Alonso-Martín C, Arbòs CM, Pamies J, et al. Clinical characteristics of patients with sustained ventricular arrhythmias after sacubitril/valsartan initiation. Heart Vessels. (2019) 35:136–42. doi: 10.1007/s00380-019-01454-6
9. Mascolo A, Urbanek K, De Angelis A, Sessa M, Scavone C, Berrino L, et al. Angiotensin II and angiotensin 1-7: which is their role in atrial fibrillation? Heart Fail Rev. (2020) 25:367–80. doi: 10.1007/s10741-019-09837-7
10. Tanaka H, Matsuyama TA, Takamatsu T. Towards an integrated understanding of cardiac arrhythmogenesis – growing roles of experimental pathology. Pathol Int. (2017) 67:8–16. doi: 10.1111/pin.12487
11. Widiapradja A, Chunduri P, Levick SP. The role of neuropeptides in adverse myocardial remodeling and heart failure. Cell Mol Life Sci. (2017) 74:2019–38. doi: 10.1007/s00018-017-2452-x
12. Donahue JK. Current state of the art for cardiac arrhythmia gene therapy. Pharmacol Ther. (2017) 176:60–5. doi: 10.1016/j.pharmthera.2017.06.005
13. Williams EA, Russo V, Ceraso S, Gupta D, Barrett-Jolley R. Anti-arrhythmic properties of non-antiarrhythmic medications. Pharmacol Res. (2020) 156:104762. doi: 10.1016/j.phrs.2020.104762
14. Abumayyaleh M, Pilsinger C, El-Battrawy I, Kummer M, Kuschyk J, Borggrefe M, et al. Clinical outcomes in patients with ischemic versus non-ischemic cardiomyopathy after angiotensin-neprilysin inhibition therapy. J Clin Med. (2021) 10:4989. doi: 10.3390/jcm10214989
15. El-Battrawy I, Pilsinger C, Liebe V, Lang S, Kuschyk J, Zhou X, et al. Impact of sacubitril/valsartan on the long-term incidence of ventricular arrhythmias in chronic heart failure patients. J Clin Med. (2019) 8:1582. doi: 10.3390/jcm8101582
16. Balmforth C, Simpson J, Shen L, Jhund PS, Lefkowitz M, Rizkala AR, et al. Outcomes and effect of treatment according to etiology in HFrEF: an analysis of PARADIGM-HF. JACC Heart Fail. (2019) 7:457–65. doi: 10.1016/j.jchf.2019.02.015
17. El-Battrawy I, Borggrefe M, Akin I. The risk for sudden cardiac death and effect of treatment with sacubitril/valsartan in heart failure. JACC Heart Fail. (2019) 7:999. doi: 10.1016/j.jchf.2019.05.010
18. Vicent L, Juarez M, Bruna V, Devesa C, Sousa-Casasnovas I, Fernandez-Aviles F, et al. Clinical profile and ventricular arrhythmias after sacubitril/valsartan initiation. Cardiology. (2019) 142:26–7. doi: 10.1159/000497190
19. Berger SI, Iyengar R. Network analyses in systems pharmacology. Bioinformatics. (2009) 25:2466–72. doi: 10.1093/bioinformatics/btp465
20. Luo T-T, Lu Y, Yan S-K, Xiao X, Rong X-l, Guo J. Network pharmacology in research of Chinese medicine formula: methodology, application and prospective. Chin J Integr Med. (2019) 26:72–80. doi: 10.1007/s11655-019-3064-0
21. Wishart DS, Feunang YD, Guo AC, Lo EJ, Marcu A, Grant JR, et al. DrugBank 5.0: a major update to the DrugBank database for 2018. Nucleic Acids Res. (2018) 46:D1074–82. doi: 10.1093/nar/gkx1037
22. Doncheva NT, Morris JH, Gorodkin J, Jensen LJ. Cytoscape StringApp: network analysis and visualization of proteomics data. J Proteome Res. (2019) 18:623–32. doi: 10.1021/acs.jproteome.8b00702
23. Alvarez CK, Cronin E, Baker WL, Kluger J. Heart failure as a substrate and trigger for ventricular tachycardia. J Interv Card Electrophysiol. (2019) 56:229–47. doi: 10.1007/s10840-019-00623-x
24. Cheng S, Zhang N, Hua W. Sacubitril/valsartan in the management of heart failure patients with cardiac implantable electronic devices. Am J Cardiovasc Drugs. (2021) 21:383–93. doi: 10.1007/s40256-020-00448-4
25. De Mello WC. Chemical communication between heart cells is disrupted by intracellular renin and angiotensin II: implications for heart development and disease. Front Endocrinol (Lausanne). (2015) 6:72. doi: 10.3389/fendo.2015.00072
26. Nadarajah R, Patel PA, Tayebjee MH. Is hypertensive left ventricular hypertrophy a cause of sustained ventricular arrhythmias in humans? J Hum Hypertens. (2021) 35:492–8. doi: 10.1038/s41371-021-00503-w
27. Valentim Goncalves A, Pereira-da-Silva T, Galrinho A, Rio P, Moura Branco L, Soares R, et al. Antiarrhythmic effect of sacubitril-valsartan: cause or consequence of clinical improvement? J Clin Med. (2019) 8:869. doi: 10.3390/jcm8060869
28. Kobalava Z, Kotovskaya Y, Averkov O, Pavlikova E, Moiseev V, Albrecht D, et al. Pharmacodynamic and pharmacokinetic profiles of sacubitril/valsartan (LCZ696) in patients with heart failure and reduced ejection fraction. Cardiovasc Ther. (2016) 34:191–8. doi: 10.1111/1755-5922.12183
29. Bunsawat K, Ratchford SM, Alpenglow JK, Stehlik J, Smith AS, Richardson RS, et al. Sympathoinhibitory effect of sacubitril-valsartan in heart failure with reduced ejection fraction: a pilot study. Auton Neurosci. (2021) 235:102834. doi: 10.1016/j.autneu.2021.102834
30. Berisha F, Gotz KR, Wegener JW, Brandenburg S, Subramanian H, Molina CE, et al. cAMP imaging at ryanodine receptors reveals beta2-adrenoceptor driven arrhythmias. Circ Res. (2021) 129:81–94. doi: 10.1161/CIRCRESAHA.120.318234
31. Zhang Y, Hou MC, Li JJ, Qi Y, Zhang Y, She G, et al. Cardiac beta-adrenergic receptor activation mediates distinct and cell type-dependent changes in the expression and distribution of connexin 43. J Cell Mol Med. (2020) 24:8505–17. doi: 10.1111/jcmm.15469
32. Martin RI, Babaei MS, Choy MK, Owens WA, Chico TJ, Keenan D, et al. Genetic variants associated with risk of atrial fibrillation regulate expression of PITX2, CAV1, MYOZ1, C9orf3 and FANCC. J Mol Cell Cardiol. (2015) 85:207–14. doi: 10.1016/j.yjmcc.2015.06.005
33. Yang KC, Rutledge CA, Mao M, Bakhshi FR, Xie A, Liu H, et al. Caveolin-1 modulates cardiac gap junction homeostasis and arrhythmogenecity by regulating cSrc tyrosine kinase. Circ Arrhythm Electrophysiol. (2014) 7:701–10. doi: 10.1161/CIRCEP.113.001394
Keywords: sacubitril/valsartan, cardiac arrhythmia, network pharmacology, molecular mechanism, heart failure
Citation: Zhou Y, Rui S, Tang S and Ju C (2022) Exploration of Mechanisms of Sacubitril/Valsartan in the Treatment of Cardiac Arrhythmias Using a Network Pharmacology Approach. Front. Cardiovasc. Med. 9:829484. doi: 10.3389/fcvm.2022.829484
Received: 05 December 2021; Accepted: 10 March 2022;
Published: 13 April 2022.
Edited by:
Mads Kjolby, Aarhus University, DenmarkReviewed by:
Maria Lucia Narducci, Catholic University of the Sacred Heart, ItalyIbrahim El-Battrawy, Ruhr University Bochum, Germany
Copyright © 2022 Zhou, Rui, Tang and Ju. This is an open-access article distributed under the terms of the Creative Commons Attribution License (CC BY). The use, distribution or reproduction in other forums is permitted, provided the original author(s) and the copyright owner(s) are credited and that the original publication in this journal is cited, in accordance with accepted academic practice. No use, distribution or reproduction is permitted which does not comply with these terms.
*Correspondence: Changlin Ju, SmNsenkyMDE5QDE2My5jb20=