- 1Department of Pediatrics, School of Medicine, Saint Louis University, St. Louis, MO, United States
- 2School of Medicine, Saint Louis University, St. Louis, MO, United States
- 3Department of Biochemistry and Molecular Biology, School of Medicine, Saint Louis University, St. Louis, MO, United States
Cardiovascular disease (CVD) in Mucopolysaccharidosis Type IVA (Morquio A), signified by valvular disease and cardiac hypertrophy, is the second leading cause of death and remains untouched by current therapies. Enzyme replacement therapy (ERT) is the gold-standard treatment for MPS disorders including Morquio A. Early administration of ERT improves outcomes of patients from childhood to adulthood while posing new challenges including prognosis of CVD and ERT’s negligible effect on cardiovascular health. Thus, having accurate biomarkers for CVD could be critical. Here we show that cathepsin S (CTSS) and elastin (ELN) can be used as biomarkers of extracellular matrix remodeling in Morquio A disease. We found in a cohort of 54 treatment naïve Morquio A patients and 74 normal controls that CTSS shows promising attributes as a biomarker in young Morquio A children. On the other hand, ELN shows promising attributes as a biomarker in adolescent and adult Morquio A. Plasma/urine keratan sulfate (KS), and urinary glycosaminoglycan (GAG) levels were significantly higher in Morquio A patients (p < 0.001) which decreased with age of patients. CTSS levels did not correlate with patients’ phenotypic severity but differed significantly between patients (median range 5.45–8.52 ng/mL) and normal controls (median range 9.61–15.9 ng/mL; p < 0.001). We also studied α -2-macroglobulin (A2M), C-reactive protein (CRP), and circulating vascular cell adhesion molecule-1 (sVCAM-1) in a subset of samples to understand the relation between ECM biomarkers and the severity of CVD in Morquio A patients. Our experiments revealed that CRP and sVCAM-1 levels were lower in Morquio A patients compared to normal controls. We also observed a strong inverse correlation between urine/plasma KS and CRP (p = 0.013 and p = 0.022, respectively) in Morquio A patients as well as a moderate correlation between sVCAM-1 and CTSS in Morquio A patients at all ages (p = 0.03). As the first study to date investigating CTSS and ELN levels in Morquio A patients and in the normal population, our results establish a starting point for more elaborate studies in larger populations to understand how CTSS and ELN levels correlate with Morquio A severity.
Introduction
Mucopolysaccharidosis IVA (MPS IVA; Morquio A syndrome) is an inherited, autosomal recessive disease caused by a deficiency of N-acetylgalactosamine-6-sulfate sulfatase (GALNS), which results in excessive lysosomal storage of keratan sulfate (KS) and chondroitin 6-sulfate (C6S) in many tissues and organs. This accumulation leads to chronic and progressive clinical findings including but not limited to systemic skeletal dysplasia, joint abnormalities, short stature, hearing and vision loss, coarse facial features, valvular heart disease, and pulmonary compromise (1–3). The extent of GALNS deficiency is highly variable thus resulting in a heterogenous clinical presentation (2, 3). Early diagnosis of Morquio A is important to employ disease modifying treatments to slow progression as no cure exists (4).
Currently available therapies for Morquio A include enzyme replacement therapy (ERT) with recombinant human GALNS enzyme, hematopoietic stem cell therapy (HSCT), and orthopedic surgeries. ERT is the most widely used therapeutic option in many lysosomal storage disorders including Morquio A. The enzyme, Elosulfase alfa, works within lysosomes to catabolize the glycosaminoglycans (GAGs) that have accumulated within. ERT has shown clinical improvement in somatic manifestations, activities of daily living, and quality of life (5–9). It has a low mortality risk with no limitations on use, however, the enzymes have a short half-life with rapid clearance from circulation resulting in poor delivery to bone and avascular tissues such as cartilage and cornea even when started at a young age. Other limitations include the need for weekly treatments, hypersensitivity reactions, and high cost (1). Another treatment option is HSCT which is a one-time permanent treatment allowing continuous production of the enzyme. HSCT has shown improvement in pulmonary function, activities of daily living, bone mineral density, cardiovascular involvement, and reduction in number of surgical interventions (10–12). However, it has many health and age limitations, requires a matched donor, carries a high risk of mortality and complications, and has limited effect on bone and cartilage (10, 11, 13). Morquio A patients often develop severe skeletal dysplasia requiring multiple orthopedic surgical interventions in the upper cervical spine and lower extremities as well as tracheal obstruction surgery. ERT and HSCT have limited to no impact on skeletal dysplasia (1, 3, 14).
Although these therapeutic treatments have extended life expectancy for Morquio A patients by attenuating disease progression, they have shown only a limited effect on heart disease which is the second leading cause of death in this population (1, 3, 15, 16). Cardiovascular disease (CVD) in these patients emerges silently and contributes significantly to early mortality (17). Cardiovascular involvement has been reported in Morquio patients as early as 3.6 months of age with subsequent progression of the disease (18). A wide range of cardiac findings have been associated with Morquio A including mitral and/or aortic insufficiency, aortic stenosis, tricuspid regurgitation, thickened interventricular septum, hypertrophic cardiomyopathy, and aortic root dilatation (15, 17–20). Furthermore, post-mortem histopathology on a 20-year-old patient has shown valvular infiltration by foam cells and macrophages along with GAG deposition (particularly C6S), and fragmented, attenuated elastic fibers in the aortic intima and media. These findings imply aortic intima thickening, atherosclerotic plaques, and aortic valve hypertrophy (21). A recent study of 12 Morquio A patients has revealed increased carotid intima media thickness and carotid hyperelasticity. These findings may be explained by alteration of arterial elasticity by GAG bearing proteoglycans (16). Limited therapeutic treatments exist for Morquio A that can address the CVD. ERT has poor delivery of the enzyme to cardiac valves and the aorta because of its short half-life in circulation, effect on cartilaginous tissues, and low density of mannose 6 phosphate receptors (1, 22). Another explanation of the slight therapeutic efficacy of ERT in heart has been attributed historically to the “avascular” nature of heart valves, which remains to be a debatable topic that needs further investigation in Morquio A patients (23). ERT studies with short term echocardiographic follow up have shown only a negligible therapeutic effect on cardiovascular findings (1, 15, 16, 20, 22, 24). The incidence of cardiac surgery in Morquio A patients is low (2%) compared to other surgeries. However, the most common cardiac surgery in adult Morquio A patients is aortic valve replacement (3, 25–27). Thus, development of a therapy to ameliorate CVD could be lifesaving in Morquio A patients.
Attenuated and fragmented elastin fibrils have been found not only in the aortic intima of a Morquio A patient (21) but also in patients and animal models of other MPS types, such as MPS I, VI, VII (28–30), and IVA (unpublished). Elastin fragmentation is the result of proteolysis by cathepsins and the matrix metalloproteinases secreted by GAG-activated macrophages (28, 31). It results in acquired CVD including atherosclerosis, calcification, and aneurysms. Elastic fiber degradation allows infiltration of lipids and immune cells into the aortic wall for plaque formation and rupture. The products from degradation further enhance atherogenesis. Calcification in the arterial medial wall and aneurysmal formation have also been linked to elastic fiber degradation and fragmentation. Fragmented elastic fibers may be more susceptible to protease digestion leading to aneurysmal dilation and stimulation and signaling of cytokine and inflammatory cell infiltration (32).
Elastin degradation leads to an irreversible and difficult to halt cascade of arterial remodeling, and cytokine and inflammatory signaling pathway activation. Damaged and degraded elastic fibers are generally not repaired due to the limited timeframe of elastin synthesis (32). Elastin is a highly abundant hydrophobic extracellular matrix (ECM) protein within the arterial media, and it is arranged in tightly coiled laminae. It is responsible for the resilience and elasticity of large arteries allowing them to withstand blood pressure oscillations throughout the cardiac cycle (32, 33). Elastic fibers can be easily degraded by specific proteases called elastases. One of these elastases, cathepsin S, is a lysosomal cysteine protease highly expressed in GAG-bearing macrophages (32, 34). Secreted cathepsin S at the basal membrane of blood vessels cleaves several ECM proteins including laminin, collagen, and preferentially elastin which generates bioactive elastin peptides (35). This potent elastolytic and collagenolytic activity has been linked to vascular inflammation and calcification resulting in the acquired CVD atherosclerosis and aneurysmal formation (32, 34, 36). Although protein levels and/or gene expression of elastin and cathepsin S have not been explored in Morquio A patients, we have observed dysregulation with age (unpublished) in Morquio A mice. Thus elastin and cathepsin S may be potential biomarkers to predict the severity of heart disease and phenotype of this patient population.
Materials and Methods
Study Populations
Plasma and urine samples of Morquio A patients were obtained from a de-identified repository located at Saint Louis University. The Institutional Review Board (IRB) at Saint Louis University determined that our human subjects research was exempt from a formal IRB submission due to the lack of patient identifiers or protected health information (PHI). Morquio A patients from the de-identified repository were previously diagnosed based on clinical phenotype, elevated KS in blood, elevated GAG levels in urine, and by having reduced GALNS enzyme activity below 5% of the normal level in leukocytes and/or by having two or more pathogenic mutations in the GALNS gene. Morquio A patients were classified by phenotype as mild or severe based on gene mutation effects and by patient height (severe phenotype with a lower 90th centile height isopleth in Morquio A growth charts) (2). Mutation effects were determined by in vitro assays (37–39) and by using the PolyPhen-2 program (40). Age-matched normal controls were obtained from a de-identified repository from Saint Louis University and Cardinal Glennon Children’s Hospital.
Measurement of Glycosaminoglycans
Concentrations of urinary and plasma GAGs and KS were measured by tandem mass spectroscopy as described elsewhere (16). Briefly, the chromatographic system consists of an HP1100 system (Agilent Technologies) and a Hypercarb column (Thermo Electron). The API-4000 mass spectrometer (Applied Biosystems) was equipped with a turbo ionspray ion source. Disaccharides of keratan, heparan, dermatan sulfate were used as standards [Galß1,4GlcNAc(6S), ΔDiHS-0S, ΔDiHS-NS, ΔDi-4S, ΔDi-6S (Seikagaku). Chondrosine was used as internal standard (Glycosyn). Urine or plasma was centrifuged, and the supernatants were digested overnight with 1 mU of chondroitinase b, 1mU heparitinase, and 1mU keratanase II (Seikagaku). Recovered samples were analyzed by the LC-MS/MS system and normalized by creatinine concentration in urine. Total GAGs refer to total glycosaminoglycans or the additive sum of all the GAGs measured (HS, DS and KS). Disaccharide GAG concentrations were calculated by Analyst 1.5.1 software (AB SCIEX). Each sample was measured in triplicate with three injections for each sample.
Investigational Biomarkers
Plasma concentrations of cathepsin S were measured in triplicate by enzyme-linked immunosorbent assay (ELISA) (R&D systems) after diluting the samples 1:100. Plasma concentrations of elastin were determined in duplicate by ELISA (Abcam) after diluting the samples 1:4 following the manufacturer’s instructions. C-reactive protein (CRP), α-2-macroglobulin (A2M), and circulating vascular cell adhesion molecule-1 (sVCAM-1) were detected in triplicates from plasma samples by LUMINEX technology, using a Millipore Milliplex kit according to the manufacturer’s instructions.
Statistical Analysis
Data was expressed as the median values and interquartile range. P-values were determined by Kruskal-Wallis test, Mann–Whitney U-test, Chi-square test, or One-Way ANOVA where appropriate. Multiple comparisons were corrected using Šidák’s-test. Pearson correlations coefficients were used to assess linear correlations between age, KS, GAG, cathepsin S, elastin, CRP, A2M, and sVCAM-1 levels. Receiver operating characteristic (ROC) analyses of plasma cathepsin S and elastin levels were performed to examine the sensitivity and specificity of plasma cathepsin S and elastin levels as biomarkers for CVD in all age groups. P-values <0.05 were considered statistically significant. Statistical analyses were performed on Prism 9.0 (GraphPad, San Diego, CA, United States).
Results
Subject Characteristics and Comparisons of Extracellular Matrix Biomarkers
Fifty-four Morquio A patients and 74 age-matched controls were included in this study. Morquio A patients were identified as mild (n = 19) or severe (n = 35) phenotype (Supplementary Table 1). Forty-three different pathogenic mutations were identified, 95% causing mild or severe phenotype. The median age of Morquio A patients is similar amongst severity and consistent with pediatric age range (median [IQR] mild, 9 [7–14] years and severe, 8 [5.5–13] years) (Table 1). Present height and weight were significantly different among Morquio A patient severity (p < 0.001), which has been previously described (2).
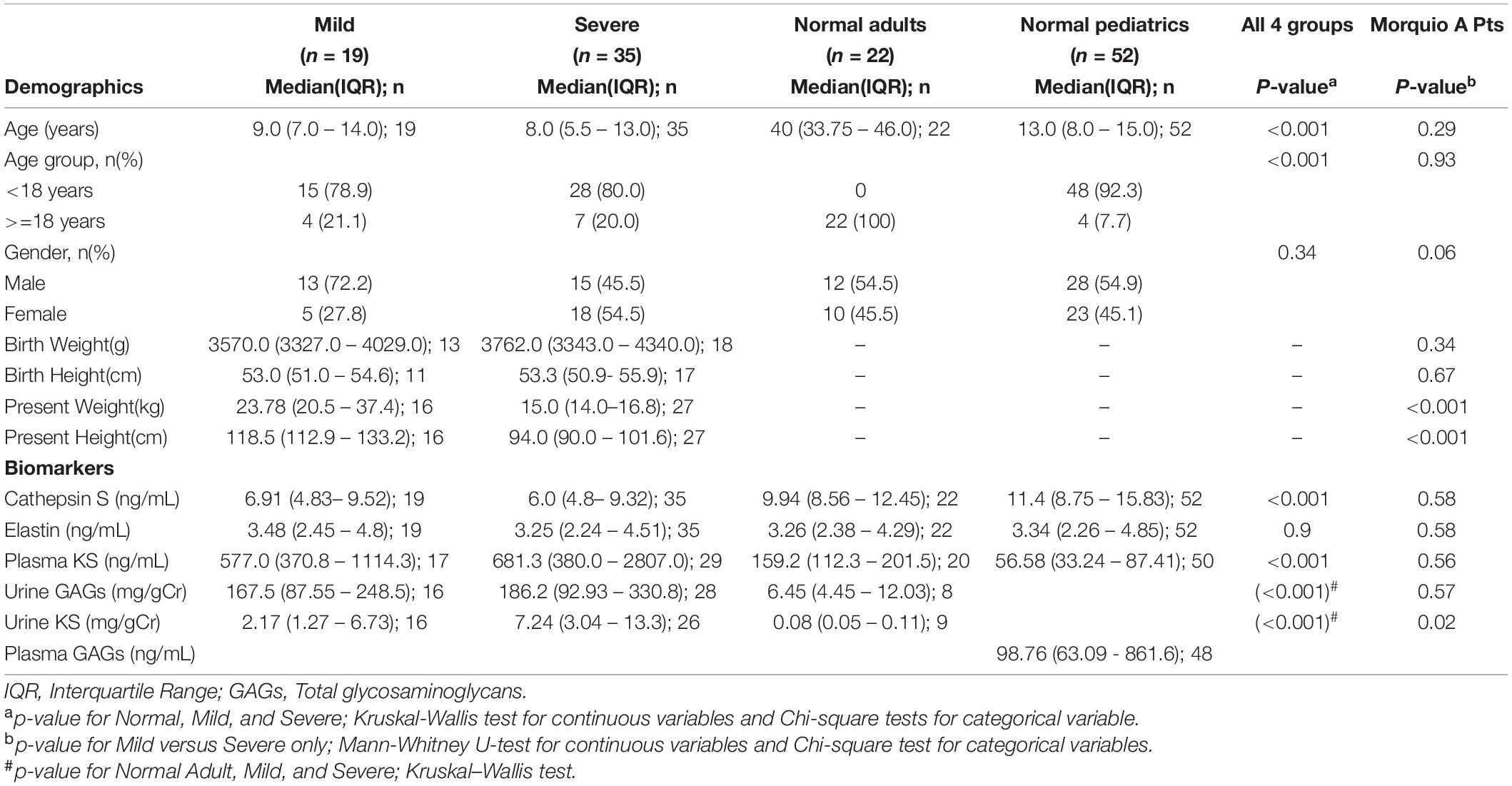
Table 1. Demographic and biomarker comparisons of Morquio A patients by severity and normal controls.
Cathepsin S levels differed significantly between patients and normal controls (p < 0.001). In contrast, there was no significant difference of elastin levels between Morquio A patients and controls. Plasma KS, urine GAGs, and urine KS levels were significantly higher in Morquio A patients (p < 0.001), consistent with previous literature (41–44), however, displayed no significant difference amongst severity except for urine KS levels (median [IQR] mild, 2.17 [1.27–6.73] mg/gCr and severe, 7.24 [3.04–13.3] mg/gCr; p = 0.02).
Age Group Dependent Analysis of Extracellular Matrix Biomarkers
To understand if there was an age dependent effect of cathepsin S and elastin, Morquio A patients and normal controls were stratified by age groups. Cathepsin S levels were similar amongst Morquio A patient age groups (median range 5.45–8.52 ng/mL; Table 2), however, differed from normal controls (p < 0.001) with higher median levels (median range 9.61–15.9 ng/mL). Normal controls 0 to 5 years old had the highest level (median [IQR] 15.9 [12.3–19.98] ng/mL). Elastin levels differed amongst Morquio A patients and normal controls (p = 0.02) with highest median levels in Morquio A patients >40 years old (median [IQR] 5.19 [4.19– 5.87] ng/mL) and normal controls >5 to 10 years old (median [IQR] 4.99 [3.42 – 8.29] ng/mL). As expected, and previously described, urinary GAGs and KS levels as well as plasma KS levels were the highest in Morquio A childhood (41). Urinary GAGs and KS differed between all age groups (p < 0.001); however, we did not obtain urine samples in the normal pediatric population which limits the available data for comparison. Plasma KS was significantly different between all age groups (p < 0.001). Plasma KS levels of Morquio A 0–5 years old group was twenty-two times higher than that of the normal control group of the same age. Similarly, plasma KS levels of Morquio A >5–10 years old were ten times higher than the plasma KS levels of normal controls of the same age. Morquio A group >10–15 years old showed 6.5 times higher plasma KS levels compared to the normal age-matched controls. Plasma GAG data was only available for normal age groups 0–20 years with concentrations the highest in early childhood, specifically 0 to 5 years old (median [IQR] 1139.0 [86.97 – 1374.0] ng/mL). These findings confirm that plasma GAGs are elevated in childhood and decreased with age (45).
Identical Age Group Analysis of Extracellular Matrix Biomarkers
We observed differences of cathepsin S and elastin levels when the patients were grouped by age. Cathepsin S levels differed in early childhood (0–5 years old) and early adolescence (>10–15 years old, p < 0.001; Table 3) between Morquio A patients and normal controls. In addition, elastin levels were significantly different amongst >5–10 years old (p = 0.02) Morquio A patients and normal controls. Urinary GAG and KS levels were significantly different between Morquio A and normal controls >20–40 years old (p = 0.03). Plasma KS levels differed significantly amongst all age groups from 0 to 20 years old.
Association Between Extracellular Matrix Biomarkers and Glycosaminoglycans
To determine the association of GAGs, elastin, cathepsin S, and age in normal population, we measured their correlation with each other. A strong correlation existed among all age groups for plasma GAGs and KS (p < 0.001) and a moderate correlation was identified for plasma GAGs and cathepsin S (p = 0.02) (not shown). A strong inverse correlation was present between various biomarkers and age groups including cathepsin S and elastin in >15–20 years old (p = 0.015), plasma KS and cathepsin S in >40 years old (p = 0.026). In addition, there was a strong inverse correlation between age and plasma KS in 0–5 years old (p < 0.005), and a moderate inverse correlation between age and cathepsin S for >10–15 years old (p = 0.031) (Figure 1).
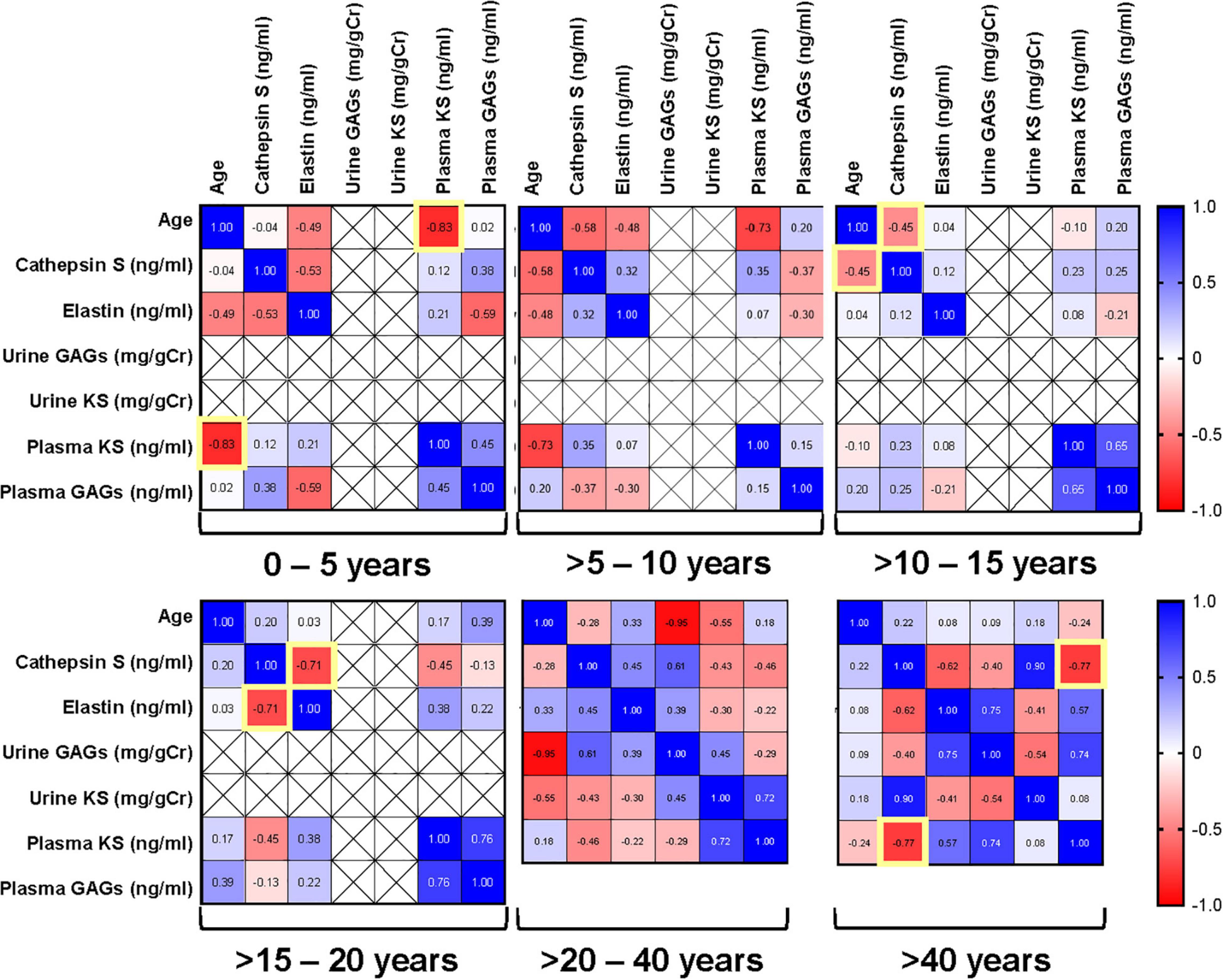
Figure 1. Pairwise Pearson correlation matrix of age, keratan sulfate (KS), glycosaminoglycans (GAGs), cathepsin S and elastin in normal samples stratified by age groups. Blue indicates positive correlation, and red indicates negative correlation. Darker colors are associated with stronger correlation coefficients. Yellow boxes indicate significant correlations.
To understand the relationship of cathepsin S, elastin, and GAG production in Morquio A patients, we determined correlation between them at different age groups. There was a strong direct correlation between urine GAGs and urine KS in all age groups of Morquio A patients (p < 0.001; Figure 2), consistent with previous studies (46, 47). A strong correlation was also present between urine GAGs and elastin in patients >20–40 years old (p = 0.046), and elastin and cathepsin S in >40 years old (p = 0.029). A strong inverse correlation between urine KS and elastin was displayed in >10–15 years old (p = 0.035).
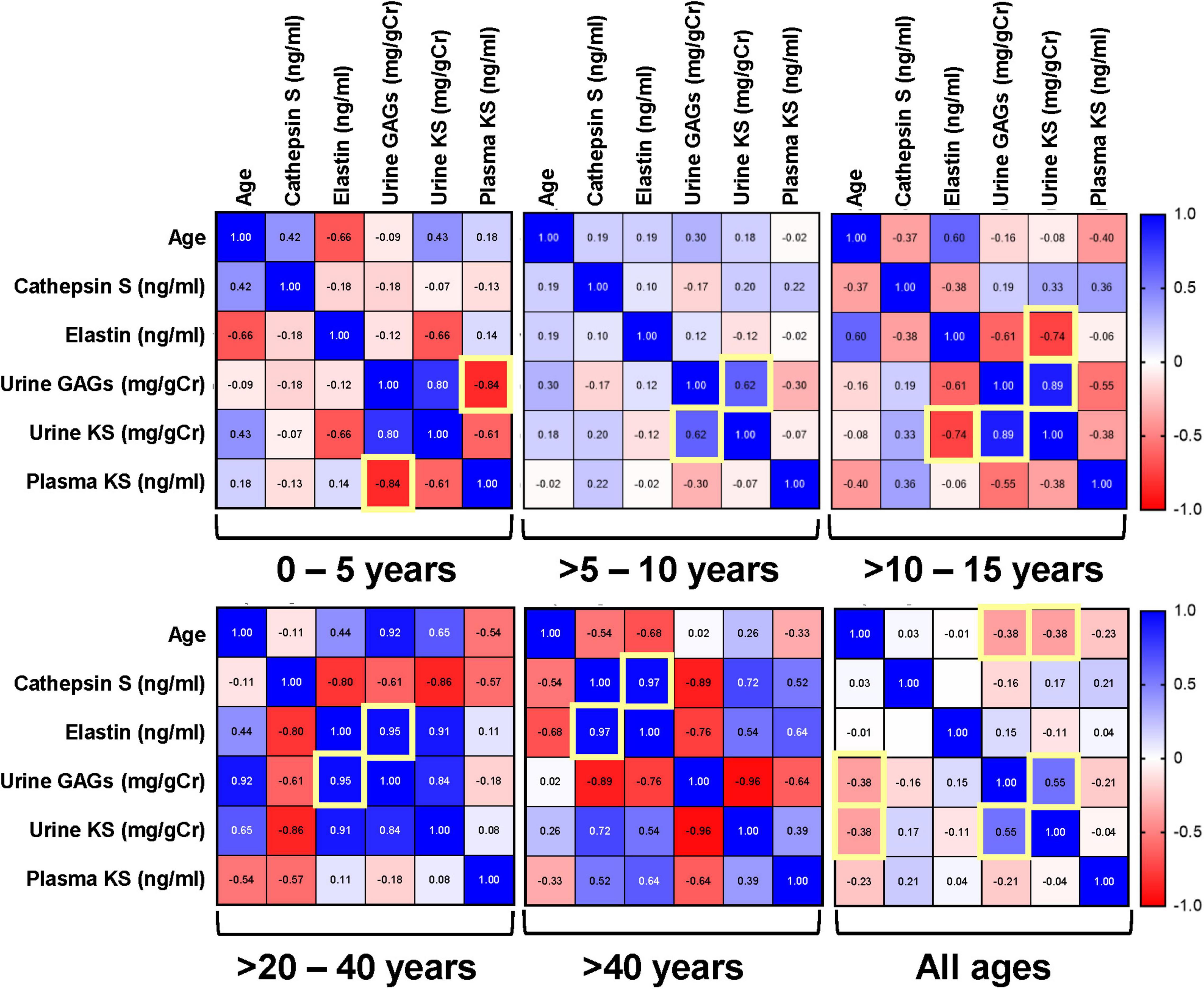
Figure 2. Pairwise Pearson correlation matrix of age, keratan sulfate (KS), glycosaminoglycans (GAGs), cathepsin S and elastin in Morquio A samples stratified by age groups. Blue indicates positive correlation, and red indicates negative correlation. Darker colors are associated with stronger correlation coefficients. Yellow boxes indicate significant correlations. The term “all ages” refers to the compound group of different ages that are part of this study.
Discrimination of Cathepsin S and Elastin as Extracellular Matrix Biomarkers for Morquio A Disease
To evaluate the diagnostic power of elastin and cathepsin S as potential biomarkers to discriminate between Morquio A and normal controls at different age groups, we performed receiver operating characteristic (ROC) curves. The ROC curves help to evaluate the discriminating ability of a biomarker by providing information between its sensitivity (true positive rate) and specificity (1- false positive rate). Values of the area under the ROC curve close to 1 indicate that the biomarker has high diagnostic accuracy, while a value of 0.5 has no predictive value (48, 49). ROC curve profiles suggest that cathepsin S levels can be used to discriminate Morquio A patients of any age from controls 0–5 years old (AUC >0.9 (p = 0.0003); Figures 3A–F). Similarly, ROC curve profiles suggest that Cathepsin S levels can be used to discriminate Morquio A children of various ages [0–15 years old] from normal controls of all ages (AUC >0.8). ROC curve profiles reflect that elastin levels can be used to discriminate Morquio A patients (>15 years old) from normal controls (AUC >0.8; Table 4). In addition, elastin levels can be clearly distinguished between adult Morquio A patients >40 years old and adult normal controls >20–40 years old (AUC 1.0; (p = 0.003); Figures 4A–D).
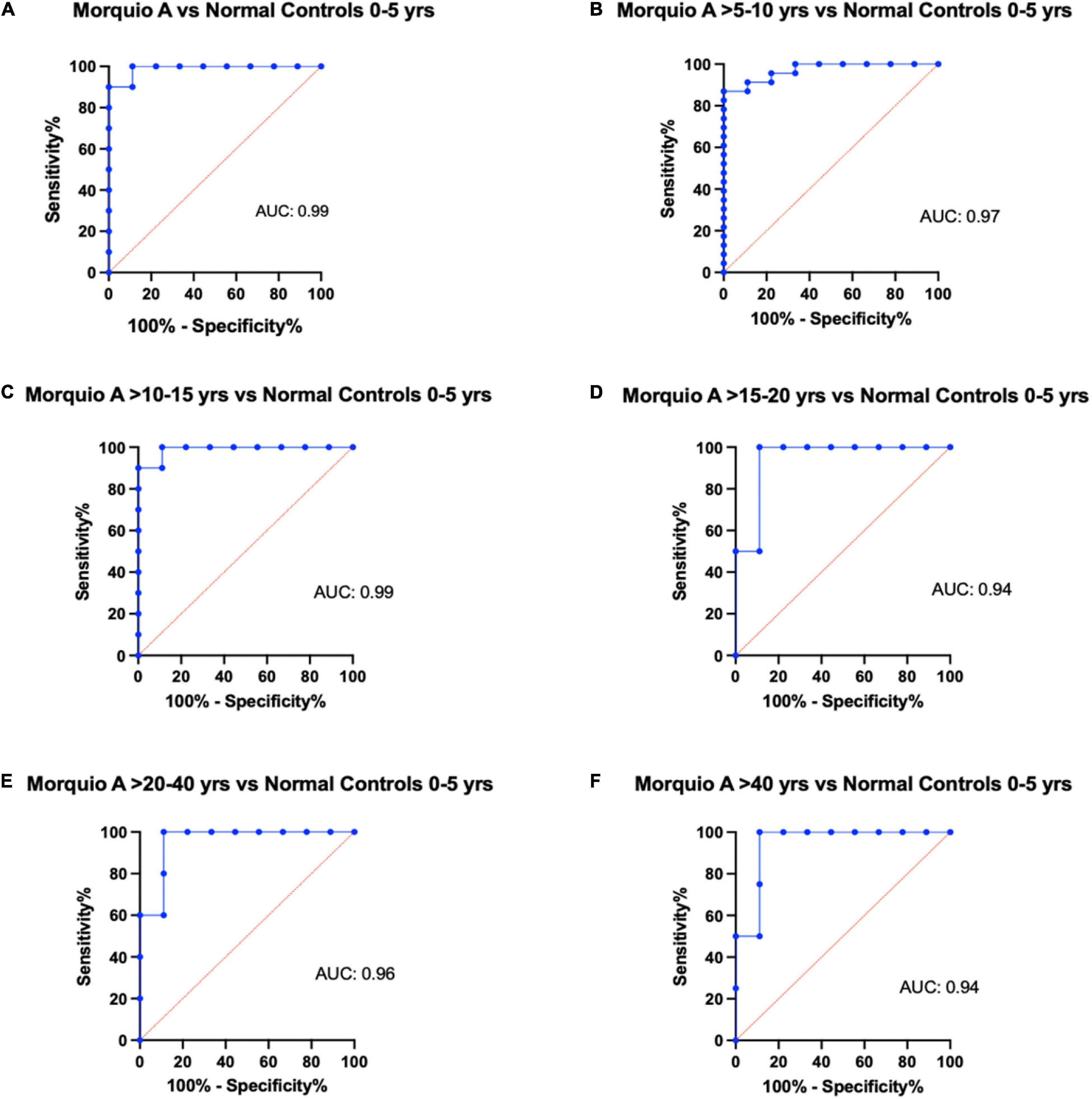
Figure 3. Representative figures of receiver operating characteristic (ROC) curves for cathepsin S comparing different age groups. Comparison of the diagnostic ability of Cathepsin S in the Morquio A cohort and the normal cohort stratified by age. Comparison of normal controls 0 to 5 years of age with: (A) Morquio A patients 0 to 5 years of age, (B) Morquio A patients >5 to 10 years of age, (C) Morquio A patients >10 to 15 years of age, (D) Morquio A patients >15–20 years of age, (E) Morquio A patients >20 to 40 years of age, and (F) Morquio A patients >40 years of age. AUC, Area under the curve.
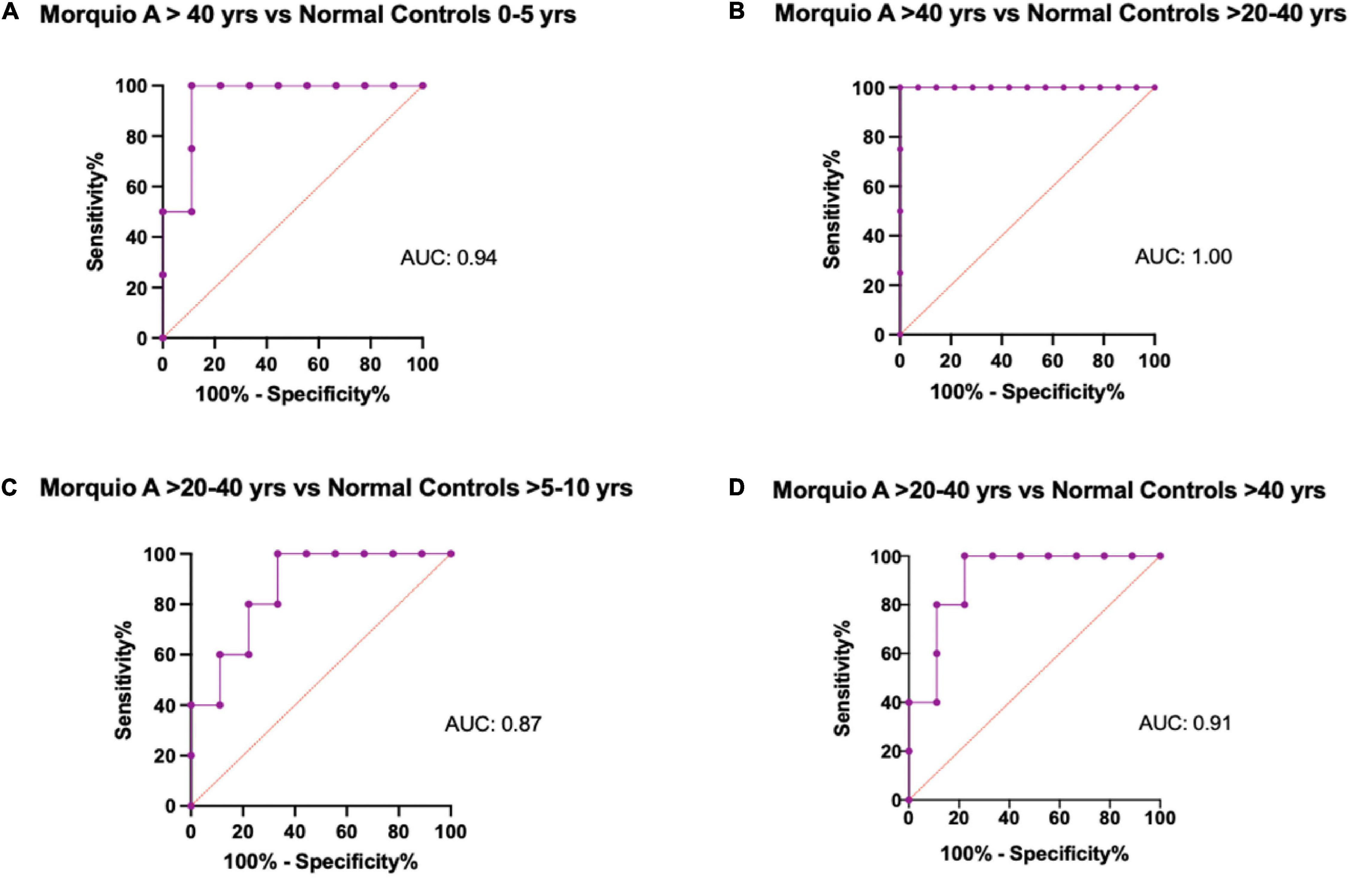
Figure 4. Representative figures of receiver operating characteristic (ROC) curves for Elastin comparing different age groups. Comparison of the diagnostic ability of Elastin in the Morquio A cohort and the normal cohort stratified by age. (A) Morquio A patients >40 years of age vs. Normal controls 0 to 5 years of age, (B) Morquio A patients >40 years of age vs. Normal controls >20 to 40 years of age, (C) Morquio A patients >20 to 40 years of age vs. Normal controls 5 to 10 years of age, (D) Morquio A patients >20 to 40 years of age vs. Normal controls >40 years of age. AUC, Area under the curve.
Extracellular Matrix Biomarker Comparisons Between Morquio A Patients and Normal Controls
To compare cathepsin S and elastin levels among different age groups, we performed scatter plot analyses in Morquio A patients and normal controls. Cathepsin S levels were significantly different between Morquio A patients and normal controls (p < 0.001). Cathepsin S levels in young Morquio A patients 0–5 years old and early adolescence >10–15 years old were lower than those in normal controls of the same age groups (p < 0.001 and p = 0.005; Figure 5A). Cathepsin S levels in normal controls 0–5 years old were very different than all others. Elastin levels in Morquio A disease seem to increase with age (Figure 5B). On the other hand, elastin levels in normal controls increased with age until 10 years of age and then showed a steady decrease until 40 years of age. Elastin levels in normal controls >5 to 10 years old were significantly higher than elastin levels in i) Morquio A patients in the same age group (p = 0.017), ii) Morquio A patients >10–15 years old (p = 0.02), and iii) Morquio A patients >20–40 years old (p = 0.02).
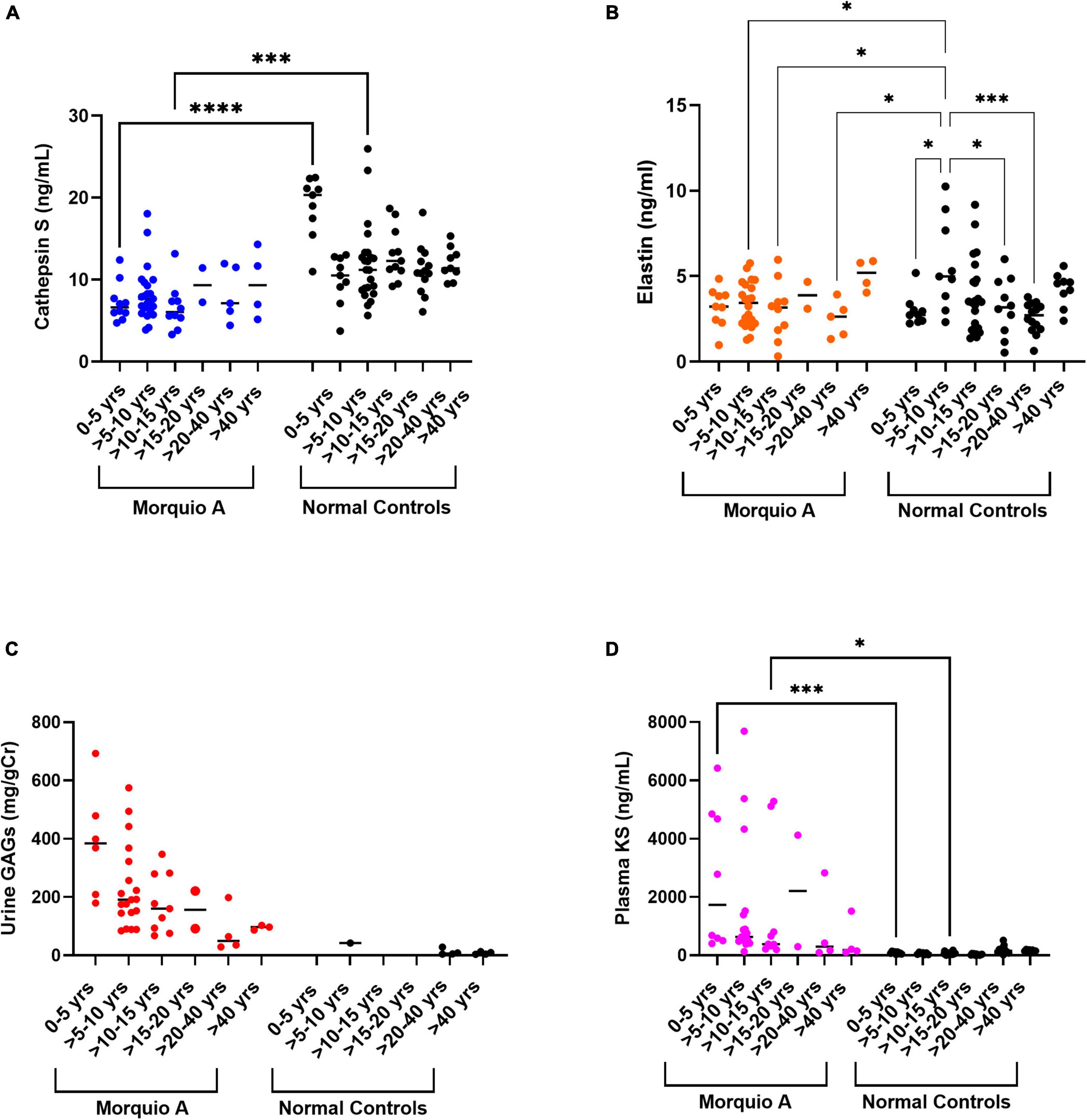
Figure 5. Biomarkers comparisons between Morquio A and normal controls. Comparison of (A) Cathepsin S, (B) Elastin, (C) Urine GAGs or (D) Plasma KS, between Morquio A and normal controls stratified by age groups. Significant differences are denoted with asterisks (*p ≤ 0.05, ***p ≤ 0.001, and ****p ≤ 0.0001). P-values were determined by one-way ANOVA followed by ordinary one-way ANOVA multiple comparisons test.
To confirm previous findings that showed a steady decrease of GAG levels with age (45, 47), we made a comparison of urine GAGs and plasma KS in Morquio A patients and normal controls. Urine GAG levels contrasted between Morquio A patients and normal controls (p = 0.0001; Figure 5C). There was a dramatic reduction of urine GAG levels in Morquio A patients from 5 years of age until having values close to the ones of normal controls after 20 years of age. Plasma KS levels differed between Morquio A patients and normal controls (p < 0.0001; Figure 5D). Significant difference of plasma KS levels was displayed between 0–5 years old and >10–15 years old age groups (p < 0.001 and p = 0.03) of Morquio A patients and normal controls.
Comparison of Cardiovascular Disease Biomarkers and Extracellular Matrix Biomarkers
To investigate whether changes in ECM biomarkers were related to the severity of CVD in Morquio A patients, we looked at three different CVD biomarkers in a subset of samples (Table 5). We measured levels of α-2-macroglubulin (A2M) and C-reactive protein (CRP) which are associated with chronic inflammation causing plaque development ending with clinical ischemic complications. We found that although there was difference in A2M levels at different ages in Morquio A and normal controls (p = 0.0034) (Figure 6A), there was no significant difference overall between those two populations (Figure 6B). In contrast, levels of CRP increased with age in both Morquio A and normal controls (Figure 6C), and the overall levels of CRP were significantly higher in normal controls (p = 0.018) when compared to Morquio A patients (Figure 6D). We also explored levels of circulating vascular cell adhesion molecule-1 (sVCAM-1) in Morquio A patients, which is indicative of endothelial dysfunction. We found that the sVCAM-1 levels are higher in Morquio A patients between 0 and 5 years of age (Figure 6E) and the overall levels in Morquio A patients were significantly lower than those in the normal controls (Figure 6F).
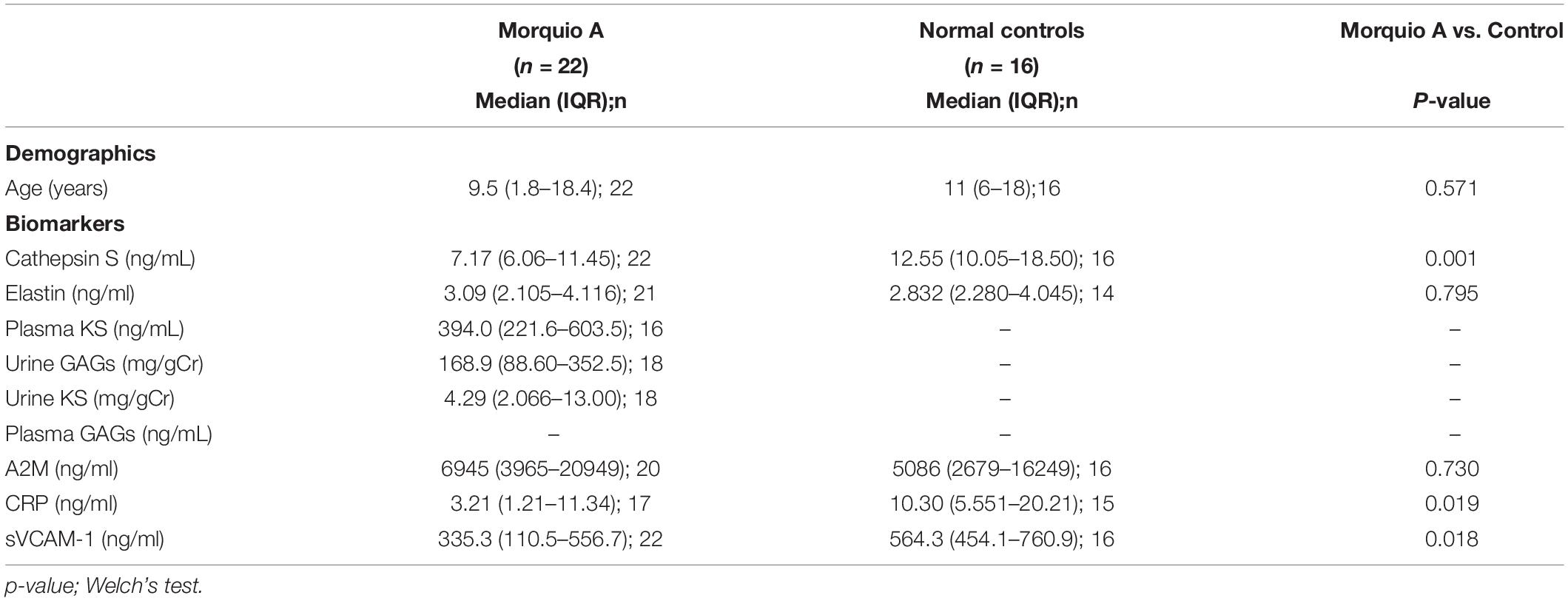
Table 5. Demographic and cardiovascular disease biomarker comparisons of Morquio A patients and normal controls.
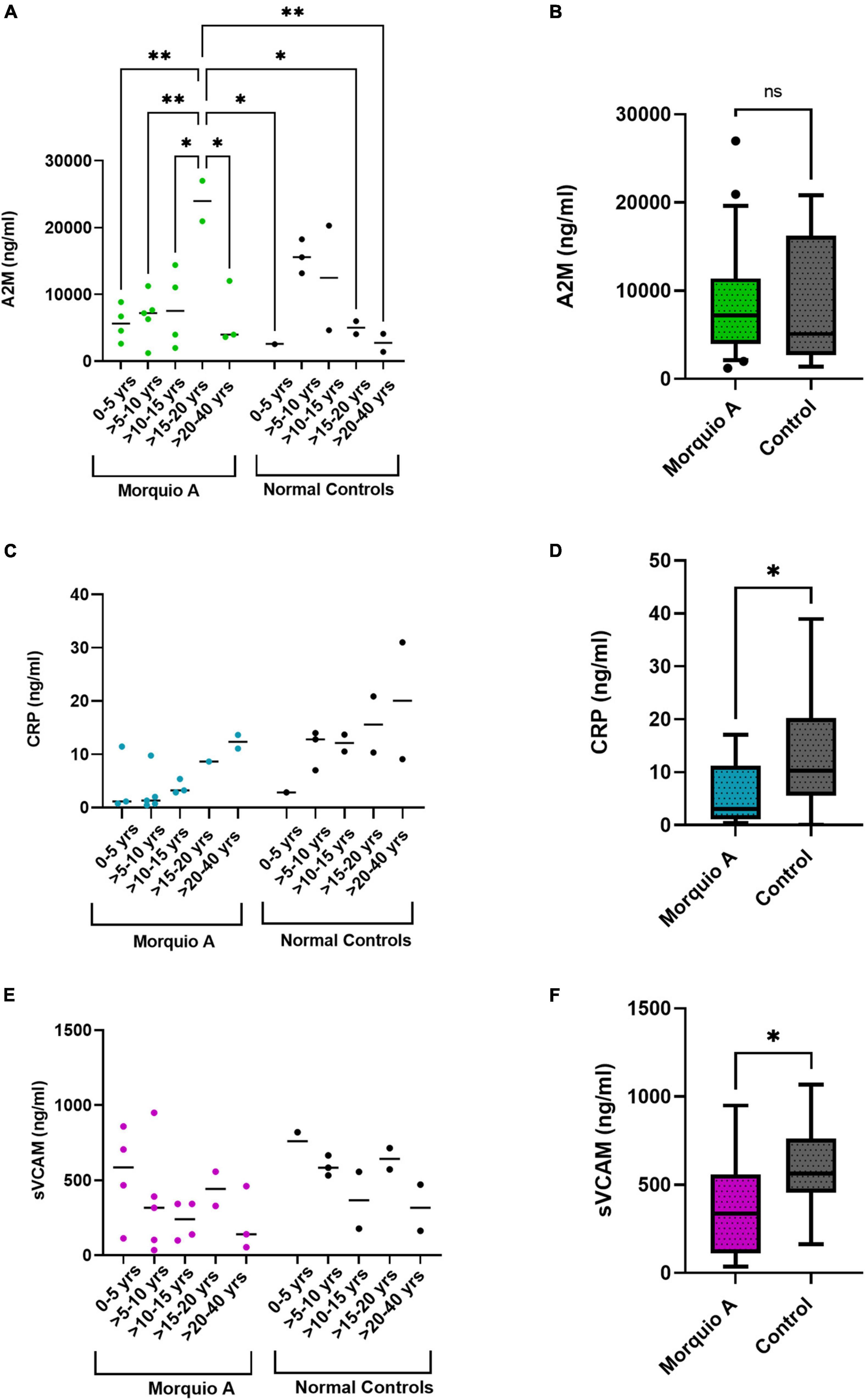
Figure 6. Comparison of cardiovascular biomarkers between Morquio A and normal controls. Scatter plot and comparison of (A,B) α-2-macroglobulin [A2M], (C,D) C-reactive protein [CRP], and (E,F) circulating vascular cell adhesion molecule-1 [sVCAM-1], between Morquio A and normal controls stratified (A,C,E) or non-stratified (B,D,F) by age groups. Significant differences are denoted with asterisks (*p ≤ 0.05, **p ≤ 0.01). p-values were determined by one-way ANOVA followed by ordinary one-way ANOVA multiple comparisons test.
To further investigate the relationship of these three CVD biomarkers and the ECM biomarkers, we measured their correlation. A moderate to strong inverse correlation existed among all age groups for urine KS and CRP (p = 0.013) as well as plasma KS and CRP (p = 0.022) in Morquio A patients. In addition, we found a moderate correlation between sVCAM-1 and Cathepsin S in Morquio A patients at all ages (p = 0.03) (Figure 7A). In normal controls, we found a strong inverse correlation between Cathepsin S and A2M (p = 0.001) and a moderate to strong correlation between elastin and A2M (p = 0.01) (Figure 7B).
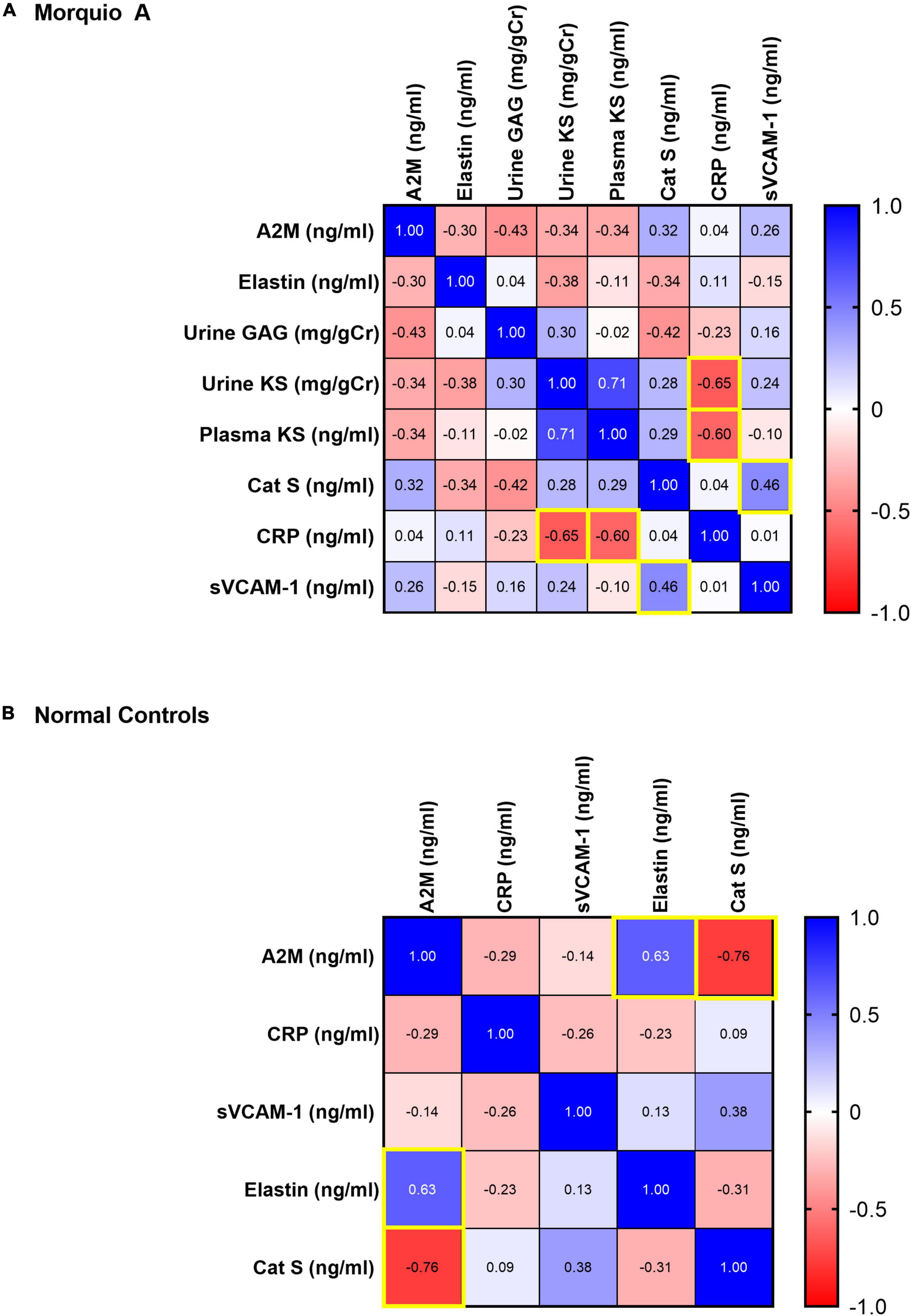
Figure 7. Pairwise Pearson correlation matrix of (A) α-2-macroglobulin (A2M), C-reactive protein (CRP), circulating vascular cell adhesion molecule-1 (sVCAM-1), elastin, cathepsin S, keratan sulfate (KS), and glycosaminoglycans (GAGs) in Morquio A samples, and (B) A2M, CRP, sVCAM-1, elastin, and cathepsin S in normal controls. Blue indicates positive correlation, and red indicates negative correlation. Darker colors are associated with stronger correlation coefficients. Yellow boxes indicate significant correlations.
Discussion
Cardiovascular disease in Morquio A remains the second leading cause of death. It has been documented as early as infancy and it progresses with age. Current therapeutic options have shown limited to no impact on CVD progression. This study aimed to discover novel lifesaving biomarkers for the diagnosis, prognosis, and treatment of CVD in this patient population.
This study is the first to investigate cathepsin S and elastin levels as biomarkers to assess the severity of CVD, genotype, and phenotype in Morquio A Syndrome. We found that cathepsin S levels in Morquio A patients were significantly lower when compared to normal controls independent of patient’s severity. This reflects that cathepsin S activity may be impeded in Morquio A. Cathepsin S must be activated and secreted from the lysosome into the ECM to cleave its substrates and its remodeling (50). It has been previously shown that large amounts of GAGs, in particular C4S and C6S, within the lysosome can alter and affect the maturation of cathepsin S (51). Stability and enhanced activation of cathepsin S into its functioning form occurs in the presence of physiologic levels of GAGs (50). This may explain our findings of increased cathepsin S levels in normal controls, especially in the young pediatric age range. GAG levels in pediatric controls are the highest in early childhood and decrease with cessation of growth into adulthood (45, 46, 52). Our experiments displayed similar findings in our pediatric normal control population. Cathepsin S activity may be enhanced by the increased presence of GAGs in early normal control childhood resulting in higher cathepsin S levels during this time frame. This contrasts to young Morquio A children who tend to display large, excessive amounts of GAGs during childhood which may be altering cathepsin S activity with subsequent reflection in decreased levels.
Furthermore, our findings suggest that cathepsin S levels can be used to discriminate Morquio A children from normal controls of all ages, especially early childhood. To date, there is no published reference range for circulating cathepsin S in human plasma. Previous studies looking at cathepsin S in various human disease processes report serum and/or plasma levels in age matched healthy controls >20 (53–58). Cathepsin S levels in the pediatric age range have yet to be reported. Our study is the first to investigate cathepsin S levels in the pediatric population and in Morquio A disease. To the best of our knowledge, there exists no study on cathepsin S in serum and plasma of MPS patients. We note the existence of cathepsin studies in animal models of MPS I (59), II (60), III (59), or VII (28, 31, 61).
Cathepsin S has been associated with the pathogenesis of many conditions including but not limited to lung diseases (62), autoimmune diseases (56, 57, 63, 64), CVD (36, 65), type 2 diabetes (66, 67), obesity (58, 68), metabolic syndrome (55), neurodegenerative disease (69), and cancer (70, 71). The majority of studies report elevated cathepsin S levels in association with the specific disease process, however, a recent study has reported decreased cathepsin S levels in patients with systemic sclerosis associated interstitial lung disease (72).
In normal controls, we discovered that elastin increased throughout childhood then decreased until middle adulthood before elevating again. These findings may be explained by normal elastin fiber formation. Elastogenesis is a complex hierarchal process compromised of many distinct phases that begins with tropoelasin synthesis. Tropoelastin synthesis mainly occurs perinatally with elastin fiber formation and deposition occurring prior to birth and throughout childhood (73). De novo elastin synthesis normally terminates leading up to and following adolescence (32). While the majority of tropoelastin synthesis occurs perinatally, synthesis has also been shown to occur in response to tissue damage (73). Tissue damage occurs with the normal aging process (74). Our findings of increased elastin levels after age 40 could be explained by increasing tropoelastin synthesis in response to the normal tissue damage that occurs with aging.
In contrast to the normal control findings, elastin levels in Morquio A patients tended to increase with age with highest levels after 40 years of age. This too may be explained by the presence of large amounts of GAGs in Morquio A childhood. Elastogenesis or the formation of elastic fibers occurs very early in life and disappears by the onset of puberty (75). The presence of high amounts of GAGs in the ECM in early Morquio A childhood could prevent the proper assembly of elastic fibers or cause irreversible damage. For example, it has been speculated that marked accumulated GAGs in arteries cause swelling and separation of elastic lamella (76). As GAG levels decrease into adolescence and adulthood, the remaining elastic fibers may assemble properly reflecting the increase in elastin levels. Cell culture studies have shown that GAGs induce production of tropoelastin in cells and upregulate microfibrillar associated genes leading to the formation of elastic fibers (77). Thus, the presence of accumulated GAGs in early Morquio childhood could be leading to induction of tropoelastin, a soluble precursor of elastin essential to elastin fiber assembly. Tropoelastin then must be chaperoned outside of the cell to form elastin in the ECM (73). These same intracellular GAGs that induce tropoleastin production may affect the actual elastin fiber synthesis pathway. This has been shown in vitro in MPS I in which increased dermatan sulfate leads to a deficiency of elastic binding protein which is a key chaperone for elastin binding synthesis (78). As GAGs decrease throughout childhood into adulthood, proper elastin fiber assembly can be achieved from the remaining intracellular tropoelastin which would explain gradual increase in elastin levels observed in our study.
Our study suggests that elastin levels can be used to discriminate adolescent and adult Morquio patients from normal controls. Like cathepsin S, there is no published reference ranges for circulating plasma and/or serum elastin. Previous studies have mainly explored levels of elastin derived peptides or anti-elastin antibodies in pathological disease processes (79–83). The relationship of elastin protein levels to elastin derived peptides and anti-elastin antibodies has yet to be explored. Our study is the first to explore plasma elastin protein levels in the normal population and in a pathological disease. In addition, our findings confirm that urinary KS is associated with clinical severity of Morquio A (52, 84).
Our experiments show that sVCAM-1 levels in Morquio A patients are lower than in normal controls, which can be explained by the dysregulation of the vascular endothelial glycocalyx in MPS patients. In CVD, inflammatory activation of endothelial cells triggers the expression of some leukocyte adhesion molecules including sVCAM-1 followed by the migration of monocytes in the tunica intima of the arterial wall thus amplifying the inflammatory response (85, 86). Chondroitin sulfate (CS) represents the largest population of arterial GAGs, therefore it actively participates in the development of arterial disease (87). Patients with Morquio A disease showed increased cIMT (16) and their endothelial dysfunction/dysregulation may be primed by the accumulation of C6S developing atherosclerotic lesions. Since CS proteoglycans play a key role in the organization and assembly of ECM, the continuous accumulation of CS and KS substrate cause widespread dysregulation including inhibition of elastic fiber assembly and cell adhesion molecules (88).
C-reactive protein (CRP) and α2M are biomarkers widely used to predict severity of CVD. CRP is known to modulate innate immune response, promote platelet activation, vascular remodeling, and angiogenesis (89). Recent studies showed that CS intake is associated with a reduction in CRP concentration in blood, indicating decrease in inflammation (90–92). These findings, along with the significantly lower CRP levels in Morquio A patients found in this study, open a new direction in this field to gain insights in the understanding of vascular endothelial glycocalyx dysregulation in this patient population.
Identification of life-saving novel biomarkers remains the main therapeutic target in Morquio A CVD. This novel study is the first to explore the relationship of cathepsin S and elastin in Morquio A and the normal population. We discovered that cathepsin S has promising attributes as a biomarker in Morquio A children. Elastin, on the other hand, has promising attributes as a biomarker in Morquio A adolescents and adults. Further studies are needed to understand how cathepsin S and elastin correlate with Morquio A severity and treatment outcomes.
Limitations
Our study also had several limitations. First, our study was underpowered in several age groups which may have impacted results of age group dependent analyses. Second, urine was not collected from the normal pediatric patients limiting comparisons of urinary GAGs and urinary KS in age dependent analyses. Similarly, plasma GAGs were not analyzed on Morquio A patients or normal adults further limiting comparisons. Importantly, patients’ database was de-identified so we could not reach out to patients to obtain echocardiograms or ultrasounds to have additional clinical information on the CVD status in patients without reported known cardiovascular symptoms. Lastly, we were unable to compare cathepsin S and elastin levels found in this study to previous studies as this is the first study to explore pediatric and adult aged Morquio A patients and healthy normal controls.
Data Availability Statement
The original contributions presented in the study are included in the article/Supplementary Material, further inquiries can be directed to the corresponding author.
Ethics Statement
The studies involving human participants were reviewed and approved by the Institutional Review Board (IRB) at Saint Louis University. Written informed consent for participation was not required for this study in accordance with the national legislation and the institutional requirements.
Author Contributions
AM conceived and supervised the study. BM and AM designed the study and wrote the manuscript. BM, LW, and QG performed laboratory analysis. AA contributed to the analysis of CVD biomarkers. BM, AA, and AM performed statistical analysis. BM, LW, QG, AA, and AM critically reviewed the manuscript. All authors contributed to the article and approved the submitted version.
Conflict of Interest
The authors declare that the research was conducted in the absence of any commercial or financial relationships that could be construed as a potential conflict of interest.
Publisher’s Note
All claims expressed in this article are solely those of the authors and do not necessarily represent those of their affiliated organizations, or those of the publisher, the editors and the reviewers. Any product that may be evaluated in this article, or claim that may be made by its manufacturer, is not guaranteed or endorsed by the publisher.
Acknowledgments
We would like to thank Paula Buchanan for statistical support.
Supplementary Material
The Supplementary Material for this article can be found online at: https://www.frontiersin.org/articles/10.3389/fcvm.2022.829111/full#supplementary-material
References
1. Puckett Y, Mulinder H, Montaño AM. Enzyme replacement therapy with elosulfase alfa for mucopolysaccharidosis IVA (Morquio A syndrome): milestones and challenges. Expert Opin Orphan Drugs. (2017) 5:741–52. doi: 10.1080/21678707.2017.1366900
2. Montaño AM, Tomatsu S, Brusius A, Smith M, Orii T. Growth charts for patients affected with Morquio A disease. Am J Med Genet A. (2008) 146A:1286–95. doi: 10.1002/ajmg.a.32281
3. Montaño AM, Tomatsu S, Gottesman GS, Smith M, Orii T. International Morquio A registry: clinical manifestation and natural course of Morquio A disease. J Inherit Metab Dis. (2007) 30:165–74. doi: 10.1007/s10545-007-0529-7
4. Sawamoto K, Suzuki Y, Mackenzie WG, Theroux MC, Pizarro C, Yabe H, et al. Current therapies for Morquio A syndrome and their clinical outcomes. Expert Opin Orphan Drugs. (2016) 4:941–51. doi: 10.1080/21678707.2016.1214572
5. Hendriksz CJ, Parini R, AlSayed MD, Raiman J, Giugliani R, Mitchell JJ, et al. Impact of long-term elosulfase alfa on activities of daily living in patients with Morquio A syndrome in an open-label, multi-center, phase 3 extension study. Mol Genet Metab. (2018) 123:127–34. doi: 10.1016/j.ymgme.2017.11.015
6. Lin HY, Lee CL, Chang CY, Chiu PC, Chien YH, Niu DM, et al. Survival and diagnostic age of 175 Taiwanese patients with mucopolysaccharidoses (1985-2019). Orphanet J Rare Dis. (2020) 15:314. doi: 10.1186/s13023-020-01598-z
7. Solano M, Fainboim A, Politei J, Porras-Hurtado GL, Martins AM, Souza CFM, et al. Enzyme replacement therapy interruption in patients with mucopolysaccharidoses: recommendations for distinct scenarios in Latin America. Mol Genet Metab Rep. (2020) 23:100572. doi: 10.1016/j.ymgmr.2020.100572
8. Politei J, Porras-Hurtado GL, Guelbert N, Fainboim A, Horovitz DDG, Satizabal JM. Enzyme replacement therapy interruption in mucopolysaccharidosis type IVA patients and its impact in different clinical outcomes. JIMD Rep. (2021) 58:104–13. doi: 10.1002/jmd2.12192
9. Kilavuz S, Basaran S, Kor D, Bulut FD, Erdem S, Balli HT, et al. Morquio A syndrome and effect of enzyme replacement therapy in different age groups of Turkish patients: a case series. Orphanet J Rare Dis. (2021) 16:144. doi: 10.1186/s13023-021-01761-0
10. Yabe H, Tanaka A, Chinen Y, Kato S, Sawamoto K, Yasuda E, et al. Hematopoietic stem cell transplantation for Morquio A syndrome. Mol Genet Metab. (2016) 117:84–94.
11. Taylor M, Khan S, Stapleton M, Wang J, Chen J, Wynn R, et al. Hematopoietic stem cell transplantation for mucopolysaccharidoses: past, present, and future. Biol Blood Marrow Transplant. (2019) 25:e226–46. doi: 10.1016/j.bbmt.2019.02.012
12. Sawamoto K, Stapleton M, Almeciga-Diaz CJ, Espejo-Mojica AJ, Losada JC, Suarez DA, et al. Therapeutic options for mucopolysaccharidoses: current and emerging treatments. Drugs. (2019) 79:1103–34. doi: 10.1007/s40265-019-01147-4
13. Sawamoto K, Alvarez Gonzalez JV, Piechnik M, Otero FJ, Couce ML, Suzuki Y, et al. Mucopolysaccharidosis IVA: diagnosis. Treatment, and management. Int J Mol Sci. (2020) 1517:21. doi: 10.3390/ijms21041517
14. Tomatsu S, Mackenzie WG, Theroux MC, Mason RW, Thacker MM, Shaffer TH, et al. Current and emerging treatments and surgical interventions for Morquio A syndrome: a review. Res Rep Endocr Disord. (2012) 2012:65–77. doi: 10.2147/RRED.S37278
15. Kampmann C, Abu-Tair T, Gokce S, Lampe C, Reinke J, Mengel E, et al. Heart and cardiovascular involvement in patients with mucopolysaccharidosis type IVA (Morquio-A syndrome). PLoS One. (2016) 11:e0162612. doi: 10.1371/journal.pone.0162612
16. Wang RY, Rudser KD, Dengel DR, Evanoff N, Steinberger J, Movsesyan N, et al. Abnormally increased carotid intima media-thickness and elasticity in patients with Morquio A disease. Orphanet J Rare Dis. (2020) 15:73. doi: 10.1186/s13023-020-1331-y
17. Braunlin EA, Harmatz PR, Scarpa M, Furlanetto B, Kampmann C, Loehr JP, et al. Cardiac disease in patients with mucopolysaccharidosis: presentation, diagnosis and management. J Inherit Metab Dis. (2011) 34:1183–97. doi: 10.1007/s10545-011-9359-8
18. Fesslova V, Corti P, Sersale G, Rovelli A, Russo P, Mannarino S, et al. The natural course and the impact of therapies of cardiac involvement in the mucopolysaccharidoses. Cardiol Young. (2009) 19:170–8. doi: 10.1017/S1047951109003576
19. John RM, Hunter D, Swanton RH. Echocardiographic abnormalities in type IV mucopolysaccharidosis. Arch Dis Child. (1990) 65:746–9. doi: 10.1136/adc.65.7.746
20. Lin HY, Chuang CK, Chen MR, Lin SM, Hung CL, Chang CY, et al. Cardiac structure and function and effects of enzyme replacement therapy in patients with mucopolysaccharidoses I, II, IVA and VI. Mol Genet Metab. (2016) 117:431–7. doi: 10.1016/j.ymgme.2016.02.003
21. Yasuda E, Fushimi K, Suzuki Y, Shimizu K, Takami T, Zustin J, et al. Pathogenesis of Morquio A syndrome: an autopsied case reveals systemic storage disorder. Mol Genet Metab. (2013) 109:301–11. doi: 10.1016/j.ymgme.2013.04.009
22. Takahashi K, Donovan MJ, Rogers RA, Ezekowitz RA. Distribution of murine mannose receptor expression from early embryogenesis through to adulthood. Cell Tissue Res. (1998) 292:311–23. doi: 10.1007/s004410051062
23. Saini N, Saikia UN, Sahni D, Singh RS. Vascularity of human atrioventricular valves: a myth or fact? J Thorac Cardiovasc Surg. (2014) 147:517–21. doi: 10.1016/j.jtcvs.2013.03.031
24. Lin HY, Chen MR, Lin SM, Hung CL, Niu DM, Chuang CK, et al. Cardiac features and effects of enzyme replacement therapy in Taiwanese patients with mucopolysaccharidosis IVA. Orphanet J Rare Dis. (2018) 13:148.
25. Pagel PS, Almassi GH. Perioperative implications of Morquio syndrome in a 31-year-old woman undergoing aortic valve replacement. J Cardiothorac Vasc Anesth. (2009) 23:855–7. doi: 10.1053/j.jvca.2008.12.009
26. Barry MO, Beardslee MA, Braverman AC. Morquio’s syndrome: severe aortic regurgitation and late pulmonary autograft failure. J Heart Valve Dis. (2006) 15:839–42.
27. Nicolini F, Corradi D, Bosio S, Gherli T. Aortic valve replacement in a patient with morquio syndrome. Heart Surg Forum. (2008) 11:E96–8. doi: 10.1532/HSF98.20071197
28. Metcalf JA, Linders B, Wu S, Bigg P, O’Donnell P, Sleeper MM, et al. Upregulation of elastase activity in aorta in mucopolysaccharidosis I and VII dogs may be due to increased cytokine expression. Mol Genet Metab. (2010) 99:396–407. doi: 10.1016/j.ymgme.2009.12.003
29. Wang RY, Braunlin EA, Rudser KD, Dengel DR, Metzig AM, Covault KK, et al. Carotid intima-media thickness is increased in patients with treated mucopolysaccharidosis types I and II, and correlates with arterial stiffness. Mol Genet Metab. (2014) 111:128–32. doi: 10.1016/j.ymgme.2013.11.001
30. Lew V, Pena L, Edwards R, Wang RY. Cardiovascular histopathology of a 11-year old with mucopolysaccharidosis VII demonstrates fibrosis, macrophage infiltration, and arterial luminal stenosis. JIMD Rep. (2018) 39:31–7. doi: 10.1007/8904_2017_43
31. Baldo G, Wu S, Howe RA, Ramamoothy M, Knutsen RH, Fang J, et al. Pathogenesis of aortic dilatation in mucopolysaccharidosis VII mice may involve complement activation. Mol Genet Metab. (2011) 104:608–19. doi: 10.1016/j.ymgme.2011.08.018
32. Cocciolone AJ, Hawes JZ, Staiculescu MC, Johnson EO, Murshed M, Wagenseil JE. Elastin, arterial mechanics, and cardiovascular disease. Am J Physiol Heart Circ Physiol. (2018) 315:H189–205. doi: 10.1152/ajpheart.00087.2018
33. Maurice P, Blaise S, Gayral S, Debelle L, Laffargue M, Hornebeck W, et al. Elastin fragmentation and atherosclerosis progression: the elastokine concept. Trends Cardiovasc Med. (2013) 23:211–21. doi: 10.1016/j.tcm.2012.12.004
35. Figueiredo JL, Aikawa M, Zheng C, Aaron J, Lax L, Libby P, et al. Selective cathepsin S inhibition attenuates atherosclerosis in apolipoprotein E-deficient mice with chronic renal disease. Am J Pathol. (2015) 185:1156–66. doi: 10.1016/j.ajpath.2014.11.026
36. Wu H, Du Q, Dai Q, Ge J, Cheng X. Cysteine protease cathepsins in atherosclerotic cardiovascular diseases. J Atheroscler Thromb. (2018) 25:111–23. doi: 10.5551/jat.RV17016
37. Montaño AM, Sukegawa K, Kato Z, Carrozzo R, Di Natale P, Christensen E, et al. Effect of ‘attenuated’ mutations in mucopolysaccharidosis IVA on molecular phenotypes of N-acetylgalactosamine-6-sulfate sulfatase. J Inherit Metab Dis. (2007) 30:758–67. doi: 10.1007/s10545-007-0702-z
38. Sukegawa K, Nakamura H, Kato Z, Tomatsu S, Montaño AM, Fukao T, et al. Biochemical and structural analysis of missense mutations in N-acetylgalactosamine-6-sulfate sulfatase causing mucopolysaccharidosis IVA phenotypes. Hum Mol Genet. (2000) 9:1283–90. doi: 10.1093/hmg/9.9.1283
39. Tomatsu S, Nishioka T, Montaño AM, Gutierrez M, Pena O, Orii K, et al. Mucopolysaccharidosis IVA: identification of mutations and methylation study in GALNS gene. J Med Genet. (2004) 41:e98. doi: 10.1136/jmg.2003.018010
40. Adzhubei IA, Schmidt S, Peshkin L, Ramensky VE, Gerasimova A, Bork P, et al. A method and server for predicting damaging missense mutations. Nat Methods. (2010) 7:248–9. doi: 10.1038/nmeth0410-248
41. Khan S, Almeciga-Diaz CJ, Sawamoto K, Mackenzie WG, Theroux MC, Pizarro C, et al. Mucopolysaccharidosis IVA and glycosaminoglycans. Mol Genet Metab. (2017) 120:78–95. doi: 10.1016/j.ymgme.2016.11.007
42. Tomatsu S, Shimada T, Mason RW, Montaño AM, Kelly J, LaMarr WA, et al. Establishment of glycosaminoglycan assays for mucopolysaccharidoses. Metabolites. (2014) 4:655–79. doi: 10.3390/metabo4030655
43. Hintze JP, Tomatsu S, Fujii T, Montaño AM, Yamaguchi S, Suzuki Y, et al. Comparison of liquid chromatography-tandem mass spectrometry and sandwich ELISA for determination of keratan sulfate in plasma and urine. Biomark Insights. (2011) 6:69–78. doi: 10.4137/BMI.S7451
44. Tomatsu S, Montaño AM, Oguma T, Dung VC, Oikawa H, de Carvalho TG, et al. Validation of keratan sulfate level in mucopolysaccharidosis type IVA by liquid chromatography-tandem mass spectrometry. J Inherit Metab Dis. (2010) 33(Suppl. 3):S35–42. doi: 10.1007/s10545-009-9013-x
45. Komosinska-Vassev KB, Winsz-Szczotka K, Kuznik-Trocha K, Olczyk P, Olczyk K. Age-related changes of plasma glycosaminoglycans. Clin Chem Lab Med. (2008) 46:219–24.
46. Ellsworth KA, Pollard LM, Cathey S, Wood T. Measurement of elevated concentrations of urine keratan sulfate by UPLC-MSMS in lysosomal storage disorders (LSDs): comparison of urine keratan sulfate levels in MPS IVA versus other LSDs. JIMD Rep. (2017) 34:11–8. doi: 10.1007/8904_2016_1
47. Wood TC, Harvey K, Beck M, Burin MG, Chien YH, Church HJ, et al. Diagnosing mucopolysaccharidosis IVA. J Inherit Metab Dis. (2013) 36:293–307.
48. Grund B, Sabin C. Analysis of biomarker data: logs, odds ratios, and receiver operating characteristic curves. Curr Opin HIV AIDS. (2010) 5:473–9. doi: 10.1097/COH.0b013e32833ed742
49. Vexler A, Schisterman EF, Liu A. Estimation of ROC curves based on stably distributed biomarkers subject to measurement error and pooling mixtures. Stat Med. (2008) 27:280–96. doi: 10.1002/sim.3035
50. Vizovisek M, Fonovic M, Turk B. Cysteine cathepsins in extracellular matrix remodeling: extracellular matrix degradation and beyond. Matrix Biol. (2019) 75-76:141–59. doi: 10.1016/j.matbio.2018.01.024
51. Novinec M, Lenarcic B, Turk B. Cysteine cathepsin activity regulation by glycosaminoglycans. Biomed Res Int. (2014) 2014:309718. doi: 10.1155/2014/309718
52. Tomatsu S, Yasuda E, Patel P, Ruhnke K, Shimada T, Mackenzie WG, et al. Morquio A syndrome: diagnosis and current and future therapies. Pediatr Endocrinol Rev. (2014) 12(Suppl. 1):141–51.
53. Jobs E, Adamsson V, Larsson A, Jobs M, Nerpin E, Ingelsson E, et al. Influence of a prudent diet on circulating cathepsin S in humans. Nutr J. (2014) 13:84. doi: 10.1186/1475-2891-13-84
54. Tanaka H, Yamaguchi E, Asai N, Yokoi T, Nishimura M, Nakao H, et al. Cathepsin S, a new serum biomarker of sarcoidosis discovered by transcriptome analysis of alveolar macrophages. Sarcoidosis Vasc Diffuse Lung Dis. (2019) 36:141–7. doi: 10.36141/svdld.v36i2.7620
55. Chen RP, Ren A, Ye SD. Correlation between serum cathepsin S and insulin resistance in type 2 diabetes. Exp Ther Med. (2013) 6:1237–42. doi: 10.3892/etm.2013.1290
56. Ruge T, Sodergren A, Wallberg-Jonsson S, Larsson A, Arnlov J. Circulating plasma levels of cathepsin S and L are not associated with disease severity in patients with rheumatoid arthritis. Scand J Rheumatol. (2014) 43:371–3. doi: 10.3109/03009742.2014.882979
57. Haves-Zburof D, Paperna T, Gour-Lavie A, Mandel I, Glass-Marmor L, Miller A. Cathepsins and their endogenous inhibitors cystatins: expression and modulation in multiple sclerosis. J Cell Mol Med. (2011) 15:2421–9. doi: 10.1111/j.1582-4934.2010.01229.x
58. Naour N, Rouault C, Fellahi S, Lavoie ME, Poitou C, Keophiphath M, et al. Cathepsins in human obesity: changes in energy balance predominantly affect cathepsin s in adipose tissue and in circulation. J Clin Endocrinol Metab. (2010) 95:1861–8. doi: 10.1210/jc.2009-1894
59. Ohmi K, Greenberg DS, Rajavel KS, Ryazantsev S, Li HH, Neufeld EF. Activated microglia in cortex of mouse models of mucopolysaccharidoses I and IIIB. Proc Natl Acad Sci USA. (2003) 100:1902–7. doi: 10.1073/pnas.252784899
60. Salvalaio M, D’Avanzo F, Rigon L, Zanetti A, D’Angelo M, Valle G, et al. Brain RNA-seq profiling of the mucopolysaccharidosis type II mouse model. Int J Mol Sci. (2017) 18:1072. doi: 10.3390/ijms18051072
61. Parente MK, Rozen R, Seeholzer SH, Wolfe JH. Integrated analysis of proteome and transcriptome changes in the mucopolysaccharidosis type VII mouse hippocampus. Mol Genet Metab. (2016) 118:41–54. doi: 10.1016/j.ymgme.2016.03.003
62. Brown R, Nath S, Lora A, Samaha G, Elgamal Z, Kaiser R, et al. Cathepsin S: investigating an old player in lung disease pathogenesis, comorbidities, and potential therapeutics. Respir Res. (2020) 21:111. doi: 10.1186/s12931-020-01381-5
63. Rupanagudi KV, Kulkarni OP, Lichtnekert J, Darisipudi MN, Mulay SR, Schott B, et al. Cathepsin S inhibition suppresses systemic lupus erythematosus and lupus nephritis because cathepsin S is essential for MHC class II-mediated CD4 T cell and B cell priming. Ann Rheum Dis. (2015) 74:452–63. doi: 10.1136/annrheumdis-2013-203717
64. Edman MC, Janga SR, Meng Z, Bechtold M, Chen AF, Kim C, et al. Increased cathepsin S activity associated with decreased protease inhibitory capacity contributes to altered tear proteins in Sjogren’s syndrome patients. Sci Rep. (2018) 8:11044. doi: 10.1038/s41598-018-29411-9
65. Liu CL, Guo J, Zhang X, Sukhova GK, Libby P, Shi GP. Cysteine protease cathepsins in cardiovascular disease: from basic research to clinical trials. Nat Rev Cardiol. (2018) 15:351–70. doi: 10.1038/s41569-018-0002-3
66. Li X, Wu K, Edman M, Schenke-Layland K, MacVeigh-Aloni M, Janga SR, et al. Increased expression of cathepsins and obesity-induced proinflammatory cytokines in lacrimal glands of male NOD mouse. Invest Ophthalmol Vis Sci. (2010) 51:5019–29. doi: 10.1167/iovs.09-4523
67. Hsing LC, Kirk EA, McMillen TS, Hsiao SH, Caldwell M, Houston B, et al. Roles for cathepsins S, L, and B in insulitis and diabetes in the NOD mouse. J Autoimmun. (2010) 34:96–104. doi: 10.1016/j.jaut.2009.07.003
68. Taleb S, Lacasa D, Bastard JP, Poitou C, Cancello R, Pelloux V, et al. Cathepsin S, a novel biomarker of adiposity: relevance to atherogenesis. FASEB J. (2005) 19:1540–2. doi: 10.1096/fj.05-3673fje
69. Lowry JR, Klegeris A. Emerging roles of microglial cathepsins in neurodegenerative disease. Brain Res Bull. (2018) 139:144–56. doi: 10.1016/j.brainresbull.2018.02.014
70. Zhang L, Wang H, Xu J. Cathepsin S as a cancer target. Neoplasma. (2015) 62:16–26. doi: 10.4149/neo_2015_003
71. McDowell SH, Gallaher SA, Burden RE, Scott CJ. Leading the invasion: the role of Cathepsin S in the tumour microenvironment. Biochim Biophys Acta Mol Cell Res. (2020) 1867:118781. doi: 10.1016/j.bbamcr.2020.118781
72. Toyama S, Yamashita T, Saigusa R, Miura S, Nakamura K, Hirabayashi M, et al. Decreased serum cathepsin S levels in patients with systemic sclerosis-associated interstitial lung disease. J Dermatol. (2020) 47:1027–32. doi: 10.1111/1346-8138.15458
73. Ozsvar J, Yang C, Cain SA, Baldock C, Tarakanova A, Weiss AS. Tropoelastin and elastin assembly. Front Bioeng Biotechnol. (2021) 9:643110. doi: 10.3389/fbioe.2021.643110
74. Poulose N, Raju R. Aging and injury: alterations in cellular energetics and organ function. Aging Dis. (2014) 5:101–8. doi: 10.14336/AD.2014.0500101
75. Wahart A, Hocine T, Albrecht C, Henry A, Sarazin T, Martiny L, et al. Role of elastin peptides and elastin receptor complex in metabolic and cardiovascular diseases. FEBS J. (2019) 286:2980–93. doi: 10.1111/febs.14836
76. Humphrey JD. Possible mechanical roles of glycosaminoglycans in thoracic aortic dissection and associations with dysregulated transforming growth factor-beta. Author reply. J Vasc Res. (2013) 50:198–9.
77. Almeida-Gonzalez FR, Gonzalez-Vazquez A, Mithieux SM, O’Brien FJ, Weiss AS, Brougham CM. A step closer to elastogenesis on demand; Inducing mature elastic fibre deposition in a natural biomaterial scaffold. Mater Sci Eng C Mater Biol Appl. (2021) 120:111788. doi: 10.1016/j.msec.2020.111788
78. Clarke LA. Pathogenesis of skeletal and connective tissue involvement in the mucopolysaccharidoses: glycosaminoglycan storage is merely the instigator. Rheumatology (Oxford). (2011) 50(Suppl. 5):v13–8. doi: 10.1093/rheumatology/ker395
79. Baud S, Duca L, Bochicchio B, Brassart B, Belloy N, Pepe A, et al. Elastin peptides in aging and pathological conditions. Biomol Concepts. (2013) 4:65–76. doi: 10.1515/bmc-2011-0062
80. Hong YJ, Kim J, Oh BR, Lee YJ, Lee EY, Lee EB, et al. Serum elastin-derived peptides and anti-elastin antibody in patients with systemic sclerosis. J Korean Med Sci. (2012) 27:484–8. doi: 10.3346/jkms.2012.27.5.484
81. Lee SH, Shin K, Park S, Kang SM, Choi D, Lee SH, et al. Circulating anti-elastin antibody levels and arterial disease characteristics: associations with arterial stiffness and atherosclerosis. Yonsei Med J. (2015) 56:1545–51. doi: 10.3349/ymj.2015.56.6.1545
82. Gminski J, Mykala-Ciesla J, Machalski M, Drozdz M. Anti-elastin antibodies in patients with lung cancer. Immunol Lett. (1992) 33:211–5. doi: 10.1016/0165-2478(92)90049-t
83. Baydanoff S, Nicoloff G, Alexiev C. Age-related changes in anti-elastin antibodies in serum from normal and atherosclerotic subjects. Atherosclerosis. (1987) 63:267–71. doi: 10.1016/0021-9150(87)90131-6
84. Tomatsu S, Okamura K, Taketani T, Orii KO, Nishioka T, Gutierrez MA, et al. Development and testing of new screening method for keratan sulfate in mucopolysaccharidosis IVA. Pediatr Res. (2004) 55:592–7. doi: 10.1203/01.PDR.0000113767.60140.E9
85. Karangelis DE, Kanakis I, Asimakopoulou AP, Karousou E, Passi A, Theocharis AD, et al. Glycosaminoglycans as key molecules in atherosclerosis: the role of versican and hyaluronan. Curr Med Chem. (2010) 17:4018–26. doi: 10.2174/092986710793205354
86. Mozaffarian D, Katan MB, Ascherio A, Stampfer MJ, Willett WC. Trans fatty acids and cardiovascular disease. N Engl J Med. (2006) 354:1601–13.
87. Theocharis AD, Theocharis DA, De Luca G, Hjerpe A, Karamanos NK. Compositional and structural alterations of chondroitin and dermatan sulfates during the progression of atherosclerosis and aneurysmal dilatation of the human abdominal aorta. Biochimie. (2002) 84:667–74.
88. Ernst H, Zanin MK, Everman D, Hoffman S. Receptor-mediated adhesive and anti-adhesive functions of chondroitin sulfate proteoglycan preparations from embryonic chicken brain. J Cell Sci. (1995) 108(Pt 12):3807–16. doi: 10.1242/jcs.108.12.3807
89. Badimon L, Pena E, Arderiu G, Padro T, Slevin M, Vilahur G, et al. C-Reactive protein in atherothrombosis and angiogenesis. Front Immunol. (2018) 9:430. doi: 10.3389/fimmu.2018.00430
90. Rondanelli M, Miraglia N, Putignano P, Peroni G, Faliva MA, Naso M, et al. Short- and long-term effectiveness of supplementation with non-animal chondroitin sulphate on inflammation, oxidative stress and functional status in obese subjects with moderate knee osteoarthritis before and after physical stress: a randomized, double-blind, placebo-controlled trial. Antioxidants (Basel). (2020) 9:1241. doi: 10.3390/antiox9121241
91. Kantor ED, O’Connell K, Du M, Cao C, Zhang X, Lee DH, et al. Glucosamine and chondroitin use in relation to C-reactive protein concentration: results by supplement form, formulation, and dose. J Altern Complement Med. (2021) 27:150–9. doi: 10.1089/acm.2020.0283
Keywords: Morquio A, MPS IVA, cardiovascular disease, biomarkers, cathepsin S, elastin
Citation: Montavon B, Winter LE, Gan Q, Arasteh A and Montaño AM (2022) Mucopolysaccharidosis Type IVA: Extracellular Matrix Biomarkers in Cardiovascular Disease. Front. Cardiovasc. Med. 9:829111. doi: 10.3389/fcvm.2022.829111
Received: 04 December 2021; Accepted: 06 April 2022;
Published: 10 May 2022.
Edited by:
Karolina M. Stepien, Salford Royal NHS Foundation Trust, United KingdomReviewed by:
Raymond Wang, Children’s Hospital of Orange County, United StatesShunji Tomatsu, Alfred I. duPont Hospital for Children, United States
Gregory M. Pastores, Mater Misericordiae University Hospital, Ireland
Copyright © 2022 Montavon, Winter, Gan, Arasteh and Montaño. This is an open-access article distributed under the terms of the Creative Commons Attribution License (CC BY). The use, distribution or reproduction in other forums is permitted, provided the original author(s) and the copyright owner(s) are credited and that the original publication in this journal is cited, in accordance with accepted academic practice. No use, distribution or reproduction is permitted which does not comply with these terms.
*Correspondence: Adriana M. Montaño, YWRyaWFuYS5tb250YW5vQGhlYWx0aC5zbHUuZWR1