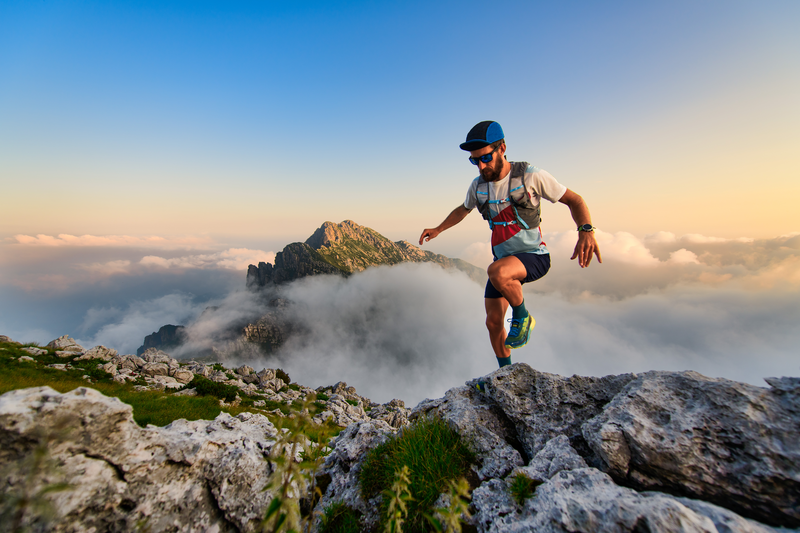
94% of researchers rate our articles as excellent or good
Learn more about the work of our research integrity team to safeguard the quality of each article we publish.
Find out more
HYPOTHESIS AND THEORY article
Front. Cardiovasc. Med. , 11 July 2022
Sec. General Cardiovascular Medicine
Volume 9 - 2022 | https://doi.org/10.3389/fcvm.2022.818202
This article is part of the Research Topic Pregnancy and Cardiovascular Diseases View all 15 articles
Maternal hypercholesterolemia during pregnancy is associated with an increased risk of preterm birth which is defined as <37 weeks of complete gestation. However, the underlying mechanism for the association between hypercholesterolemia and preterm birth is not fully understood. Macrophage, as one of the largest cell types in the placenta, plays a very critical role in mediating inflammation and triggers labor initiation. Here, we hypothesize that macrophages can uptake maternal excessive cholesterol leading to its accumulation, resulting in a breach of the immune tolerance and precipitating labor.
The hydrophobic lipid, cholesterol (C27H46O), first isolated from human gallstones more than two centuries ago, plays a critical role in maintaining normal human physiology. Disruption in cholesterol metabolism can cause congenital human diseases (such as Familial hypercholesterolemia, Tangier disease, Schnyder corneal dystrophy) and acquired diseases (such as atherosclerosis, cardiovascular disease, and Alzheimer's disease) (1).
Cholesterol is a structural component and presents in every cellular membrane, which is also essential for embryonic and fetal development. Burgeoning evidence supports the role of cholesterol in parturition with very high level of cholesterol in pregnant women being associated with an increased risk of preterm birth (PTB) in comparison to women with moderate cholesterol level (2, 3). A meta-analysis involving 13,025 pregnant women found that maternal dyslipidemia during pregnancy, either the elevated total cholesterol or triglycerides, was associated with an increased risk of PTB (4). Besides, the cholesterol transporters such as ATP-binding cassette (ABC)-transporters, ABCA1 and ABCG1 are involved in parturition (5, 6) as evidenced by the study showing the association between abnormal expression of ABCA1 to the dysregulation of placental lipid metabolism and development of spontaneous PTB (7).
PTB is defined as delivery prior to 37 weeks gestational age. With an estimated global incidence of ~15 million per year, PTB constitutes the leading cause of neonatal morbidity and mortality worldwide (8), thereby putting a tremendous emotional and economic burden on society. Premature infants are also at risk of developing immediate complications like respiratory distress syndrome, sepsis, intraventricular hemorrhage, necrotizing enterocolitis, hypothermia, hypoglycemia, hyperbilirubinemia and long-term morbidity like retinopathy of prematurity, neurodevelopmental impairment, and cerebral palsy (8). However, pathophysiology of initiation of labor is poorly understood, the therapeutic strategies of prevention and treatment of PTB is limited. Cholesterol from pregnant woman with hypercholesteremia may get transported into placenta and accumulate, which triggers an inflammation response and results in PTB. Therefore, it is important to illustrate underlying mechanism of maternal dyslipidemia that can lead to premature delivery, with an aim of providing novel therapeutic target.
Massive amounts of cholesterol are needed for growth. Fetuses have two sources of cholesterol, de novo synthesized cholesterol and exogenous cholesterol from maternal circulation via the placenta. Well-documented evidence has shown that fetus procure cholesterol from their mother (9). Newborns have a large amount of plant sterols, the content of which is about 40–50% of that in the mother (10). Since plant sterols are only obtained from the diet, their presence in fetal serum indicates their vertical transmission. Substantial cholesterol is also present in fetus who are unable to synthesize cholesterol due to genetic abnormalities (11, 12). A recent study also found that there was substantial uptake of cholesterol from mother (measured as difference in the arterial-venous concentrations) by the fetus using a 4-vessel sampling method (13). Transfer of maternal cholesterol to the embryo as well as the fetus was also confirmed in mice (14). Maternal cholesterol must cross trophoblast barrier of placenta to reach the fetal circulation (15), suggesting entry of maternal cholesterol through placenta.
The various cell types in the placental disc include trophoblasts, macrophages, connective tissue fibroblasts and vascular cells. It is unclear which cell type in the placenta contributes to uptake of cholesterol. In maternal circulation, the majority of cholesterol is in the form of LDL-cholesterol or HDL-cholesterol, which can be uptake by trophoblast of placenta via its low-density lipoprotein receptor (LDLR) and scavenger receptor class B type I (SR-B1), respectively (9). Macrophages take up native and modified (for example, oxidized) LDL-cholesterol via micropinocytosis, phagocytosis, or scavenger receptor-mediated pathways (including via SR-B1, lectin-like oxidized LDL receptor 1, and CD36) (16). Macrophages were reported to express multiple scavenger receptors for uptake of LDL, which promotes the cellular accumulation of cholesterol (17). Scavenger receptors constitute a heterogeneous family of receptors including CD36 and SR-B1. CD36 is a membrane glycoprotein that is expressed on various types of cells, which can bind to multiple ligands and mediate the endocytosis of LDL (18). SR-B1 can mediate cholesteryl esters selective uptake and the bi-directional flux of free cholesterol (19). Peroxisome proliferator–activated receptors (PPARs) and liver X receptors (LXRs) are members of the nuclear receptor superfamily of transcription factors that play a key role in regulating the expression of scavenger receptors of macrophages (20). PPARγ is required for placental development and regulates essential placental functions (21), which may also be a drug target for complicated pregnancy (22). LXR was also reported to be an important factor in early-pregnancy lipogenesis which is necessary to protect against abnormalities in fetoplacental lipid homeostasis (23). However, whether PPARγ and LXRs involve in the uptake of cholesterol by macrophages in the placenta needs more studies.
Besides, cholesterol can also transfer from one cell type to adjacent different cell types (24), raising a possibility that the excess cholesterol of trophoblast may be a result of cholesterol accumulation by adjacent macrophage. Down-regulation of lipids associated receptors of placenta such as LDLR, SR-B1 results in decreased uptake of cholesterol by placental from maternal circulation (5). The mRNA expression of lipoprotein receptors, including LDLR and very low-density lipoprotein receptor (VLDLR) was significantly increased in placenta from hypercholesterolemic women as comparison to the control group (25). A plausible explanation can be the uptake of LDL-cholesterol by placenta may lead to upregulation of lipoprotein receptors, which in turn, results in uptake of LDL-cholesterol in placenta macrophages.
A large body of literature shows the accumulated LDL-cholesterol in tissues can be modified to function as a ligand for macrophage pattern recognition receptors, including Toll-like receptors (TLRs), thereby directly triggering pro-inflammatory signaling pathways (26). Besides, accumulation of LDL-cholesterol in macrophages through endocytosis can also trigger TLR signaling (27, 28). Production of cytokines and chemokines may be amplified by increased TLR, amplifying the inflammatory process (27, 28).
Macrophages are a major type of leukocytes in the placenta and play a critical role throughout pregnancy (29). These placental macrophages support a variety of processes essential for successful pregnancy such as remodeling of the uterine connective tissues and blood vessels, regulation of trophoblast implantation, immune-tolerance toward fetal antigens, immunomodulation of neighboring leukocytes and initiation of parturition (30). Besides, placental macrophages are an important component for suppression of maternal immunologic response to the allogenic placenta and fetus due to its decreased ability to present antigens to T cells during pregnancy. Near term, placental macrophage activity switched to inflammation state contributes to parturition through production of pro-inflammatory cytokines and prostaglandin E2 (31), which in turn break the immune tolerance and initiate parturition. Accumulation of cholesterol in macrophages can trigger pro-inflammatory signaling pathways (26). It is well-validated that pregnant women with hypercholesterolemia are associated with increased risk of PTB (2, 3, 32). Therefore, we hypothesize excessive cholesterol from the mother with hypercholesterolemia will be transported into the placenta and accumulate in macrophages, which will subsequently increase the inflammation, triggering PTB (Figure 1).
Figure 1. Potential mechanism of maternal hypercholesterolemia contributing to preterm birth. Excessive cholesterol from mother with hypercholesterolemia will be transported into placenta and accumulates in macrophages, which will increase inflammation response and consequently trigger preterm birth.
We will design some experiments to test this hypothesis. ①C57BL/6 male and female mice will be randomly given normal chow diet or 5% high cholesterol diet for 2–4 months (need to be optimized). Set up timed breeding and monitor the timing of parturition. At the endpoint of the experiment, mice will be sacrificed and blood, placenta will be collected. We will measure cholesterol levels in blood and placenta. ②We will isolate macrophage in placenta and measure lipids levels and the expression of cholesterol-uptake associated receptors including VLDLR, LDLR, CD36, and SR-B1. We will isolate macrophages from placenta by magnetic-activated cell sorting (MACS), then detect the cytokine, lipids and inflammation related gene expression in the absence or presence of the cultured macrophages with high LDL-cholesterol and oxidized LDL-cholesterol. ③To investigate the cholesterol uptake from the mother to macrophages in the placenta, the pregnant female mice will be injected with APOB-labeled particle ([125I]-LDL) or LDL-cholesterol ([3H]-CE-LDL) via tail veins at 17.5 days post coitum (dpc). Macrophages in the placenta will be isolated at 30 min, 1, 2, and 4 h after injection. Radioactivity in the homogenate of macrophages will be measured by γ-counting.
The original contributions presented in the study are included in the article/supplementary material, further inquiries can be directed to the corresponding author/s.
JinC and JiaC conceived the idea. JinC and FL wrote the manuscript. JiaC, LH, and JinC collected and read the literature. All authors read and approved the final manuscript. All authors contributed to the article and approved the submitted version.
This work was supported by the grants from Hunan Provincial Natural Science Foundation of China (2022JJ40675 to JinC and 2021JJ40852 to FL), the National Natural Science Foundation of China (82100495 to FL), and Scientific Research Project of Hunan Provincial Health Commission (202203014009 to FL).
The authors declare that the research was conducted in the absence of any commercial or financial relationships that could be construed as a potential conflict of interest.
All claims expressed in this article are solely those of the authors and do not necessarily represent those of their affiliated organizations, or those of the publisher, the editors and the reviewers. Any product that may be evaluated in this article, or claim that may be made by its manufacturer, is not guaranteed or endorsed by the publisher.
1. Luo J, Yang H, Song BL. Mechanisms and regulation of cholesterol homeostasis. Nat Rev Mol Cell Biol. (2020) 21:225–45. doi: 10.1038/s41580-019-0190-7
2. Nasioudis D, Doulaveris G, Kanninen TT. Dyslipidemia in pregnancy and maternal-fetal outcome. Minerva Ginecol. (2019) 71:155–62. doi: 10.23736/S0026-4784.18.04330-7
3. Smith CJ, Baer RJ, Oltman SP, Breheny PJ, Bao W, Robinson JG, et al. Maternal dyslipidemia and risk for preterm birth. PLoS ONE. (2018) 13:e0209579. doi: 10.1371/journal.pone.0209579
4. Jiang S, Jiang J, Xu H, Wang S, Liu Z, Li M, et al. Maternal dyslipidemia during pregnancy may increase the risk of preterm birth: a meta-analysis. Taiwan J Obstet Gynecol. (2017) 56:9–15. doi: 10.1016/j.tjog.2016.07.012
5. Chatuphonprasert W, Jarukamjorn K, Ellinger I. Physiology and pathophysiology of steroid biosynthesis, transport and metabolism in the human placenta. Front Pharmacol. (2018) 9:1027. doi: 10.3389/fphar.2018.01027
6. Christiansen-Weber TA, Voland JR, Wu Y, Ngo K, Roland BL, Nguyen S, et al. Functional loss of ABCA1 in mice causes severe placental malformation, aberrant lipid distribution, and kidney glomerulonephritis as well as high-density lipoprotein cholesterol deficiency. Am J Pathol. (2000) 157:1017–29. doi: 10.1016/S0002-9440(10)64614-7
7. Cheng-Mao X, Yan L, Li L, Hua J, Xiao-Ju W, Jie-Wen Z. Placental ABCA1 expression is increased in spontaneous preterm deliveries compared with iatrogenic preterm deliveries and term deliveries. Biomed Res Int. (2017) 2017:8248094. doi: 10.1155/2017/8248094
8. Purisch SE, Gyamfi-Bannerman C. Epidemiology of preterm birth. Semin Perinatol. (2017) 41:387–91. doi: 10.1053/j.semperi.2017.07.009
9. Woollett LA. Review: transport of maternal cholesterol to the fetal circulation. Placenta. (2011) 32(Suppl. 2):S218–21. doi: 10.1016/j.placenta.2011.01.011
10. Vuorio AF, Miettinen TA, Turtola H, Oksanen H, Gylling H. Cholesterol metabolism in normal and heterozygous familial hypercholesterolemic newborns. J Lab Clin Med. (2002) 140:35–42. doi: 10.1067/mlc.2002.125214
11. Linck LM, Hayflick SJ, Lin DS, Battaile KP, Ginat S, Burlingame T, et al. Fetal demise with Smith-Lemli-Opitz syndrome confirmed by tissue sterol analysis and the absence of measurable 7-dehydrocholesterol Delta(7)-reductase activity in chorionic villi. Prenat Diagn. (2000) 20:238–40. doi: 10.1002/(SICI)1097-0223(200003)20:3<238::AID-PD792>3.0.CO;2-W
12. Nowaczyk MJ, Farrell SA, Sirkin WL, Velsher L, Krakowiak PA, Waye JS, et al. Smith-Lemli-Opitz (RHS) syndrome: holoprosencephaly and homozygous IVS8-1G–>C genotype. Am J Med Genet. (2001) 103:75–80. doi: 10.1002/1096-8628(20010915)103:1<75::AID-AJMG1502>3.0.CO; 2-R
13. Horne H, Holme AM, Roland MCP, Holm MB, Haugen G, Henriksen T, et al. Maternal-fetal cholesterol transfer in human term pregnancies. Placenta. (2019) 87:23–9. doi: 10.1016/j.placenta.2019.09.001
14. Yoshida S, Wada Y. Transfer of maternal cholesterol to embryo and fetus in pregnant mice. J Lipid Res. (2005) 46:2168–74. doi: 10.1194/jlr.M500096-JLR200
15. Palinski W. Maternal-fetal cholesterol transport in the placenta: good, bad, and target for modulation. Circ Res. (2009) 104:569–71. doi: 10.1161/CIRCRESAHA.109.194191
16. Moore KJ, Sheedy FJ, Fisher EA. Macrophages in atherosclerosis: a dynamic balance. Nat Rev Immunol. (2013) 13:709–21. doi: 10.1038/nri3520
17. Moore KJ, Freeman MW. Scavenger receptors in atherosclerosis: beyond lipid uptake. Arterioscler Thromb Vasc Biol. (2006) 26:1702–11. doi: 10.1161/01.ATV.0000229218.97976.43
18. Collot-Teixeira S, Martin J, McDermott-Roe C, Poston R, McGregor JL. CD36 and macrophages in atherosclerosis. Cardiovasc Res. (2007) 75:468–77. doi: 10.1016/j.cardiores.2007.03.010
19. Vazquez MM, Gutierrez MV, Salvatore SR, Puiatti M, Dato VA, Chiabrando GA, et al. Nitro-oleic acid, a ligand of CD36, reduces cholesterol accumulation by modulating oxidized-LDL uptake and cholesterol efflux in RAW2647 macrophages. Redox Biol. (2020) 36:101591. doi: 10.1016/j.redox.2020.101591
20. Ricote M, Valledor AF, Glass CK. Decoding transcriptional programs regulated by PPARs and LXRs in the macrophage: effects on lipid homeostasis, inflammation, and atherosclerosis. Arterioscler Thromb Vasc Biol. (2004) 24:230–9. doi: 10.1161/01.ATV.0000103951.67680.B1
21. Barak Y, Nelson MC, Ong ES, Jones YZ, Ruiz-Lozano P, Chien KR, et al. PPAR gamma is required for placental, cardiac, and adipose tissue development. Mol Cell. (1999) 4:585–95. doi: 10.1016/S1097-2765(00)80209-9
22. McCarthy FP, Delany AC, Kenny LC, Walsh SK. PPAR-gamma – a possible drug target for complicated pregnancies. Br J Pharmacol. (2013) 168:1074–85. doi: 10.1111/bph.12069
23. Nikolova V, Papacleovoulou G, Bellafante E, Borges Manna L, Jansen E, Baron S, et al. Changes in LXR signaling influence early-pregnancy lipogenesis and protect against dysregulated fetoplacental lipid homeostasis. Am J Physiol Endocrinol Metab. (2017) 313:E463–72. doi: 10.1152/ajpendo.00449.2016
24. He C, Jiang H, Song W, Riezman H, Tontonoz P, Weston TA, et al. Cultured macrophages transfer surplus cholesterol into adjacent cells in the absence of serum or high-density lipoproteins. Proc Natl Acad Sci USA. (2020) 117:10476–83. doi: 10.1073/pnas.1922879117
25. Zhang R, Dong S, Ma WW, Cai XP, Le ZY, Xiao R, et al. Modulation of cholesterol transport by maternal hypercholesterolemia in human full-term placenta. PLoS ONE. (2017) 12:e0171934. doi: 10.1371/journal.pone.0171934
26. Tall AR, Yvan-Charvet L. Cholesterol, inflammation and innate immunity. Nat Rev Immunol. (2015) 15:104–16. doi: 10.1038/nri3793
27. Moore KJ, Tabas I. Macrophages in the pathogenesis of atherosclerosis. Cell. (2011) 145:341–55. doi: 10.1016/j.cell.2011.04.005
28. Fessler MB, Parks JS. Intracellular lipid flux and membrane microdomains as organizing principles in inflammatory cell signaling. J Immunol. (2011) 187:1529–35. doi: 10.4049/jimmunol.1100253
29. Mezouar S, Benammar I, Boumaza A, Diallo AB, Chartier C, Buffat C, et al. Full-term human placental macrophages eliminate Coxiella burnetii through an IFN-gamma autocrine loop. Front Microbiol. (2019) 10:2434. doi: 10.3389/fmicb.2019.02434
30. Brown MB, von Chamier M, Allam AB, Reyes L. M1/M2 macrophage polarity in normal and complicated pregnancy. Front Immunol. (2014) 5:606. doi: 10.3389/fimmu.2014.00606
31. Liu S, Diao L, Huang C, Li Y, Zeng Y, Kwak-Kim JYH. The role of decidual immune cells on human pregnancy. J Reprod Immunol. (2017) 124:44–53. doi: 10.1016/j.jri.2017.10.045
Keywords: preterm birth, macrophage, cholesterol, low-density lipoprotein receptor, inflammation
Citation: Chen J, Hua L, Luo F and Chen J (2022) Maternal Hypercholesterolemia May Involve in Preterm Birth. Front. Cardiovasc. Med. 9:818202. doi: 10.3389/fcvm.2022.818202
Received: 19 November 2021; Accepted: 21 June 2022;
Published: 11 July 2022.
Edited by:
Avash Das, University of Texas Southwestern Medical Center, United StatesReviewed by:
Owais Bhat, Virginia Commonwealth University, United StatesCopyright © 2022 Chen, Hua, Luo and Chen. This is an open-access article distributed under the terms of the Creative Commons Attribution License (CC BY). The use, distribution or reproduction in other forums is permitted, provided the original author(s) and the copyright owner(s) are credited and that the original publication in this journal is cited, in accordance with accepted academic practice. No use, distribution or reproduction is permitted which does not comply with these terms.
*Correspondence: Fei Luo, bHVvZmVpMDA1OEBjc3UuZWR1LmNu; Jianlin Chen, SmlhbmxpbmNoZW5AY3N1LmVkdS5jbg==
Disclaimer: All claims expressed in this article are solely those of the authors and do not necessarily represent those of their affiliated organizations, or those of the publisher, the editors and the reviewers. Any product that may be evaluated in this article or claim that may be made by its manufacturer is not guaranteed or endorsed by the publisher.
Research integrity at Frontiers
Learn more about the work of our research integrity team to safeguard the quality of each article we publish.