- 1Division of Cardiology, Mazankowski Alberta Heart Institute, University of Alberta, Edmonton, AB, Canada
- 2Faculty of Kinesiology, University of Toronto, Toronto, ON, Canada
- 3Faculty of Nursing, College of Health Sciences, University of Alberta, Edmonton, AB, Canada
- 4Department of Agricultural, Food and Nutritional Science, University of Alberta, Edmonton, AB, Canada
- 5Department of Biomedical Engineering, University of Alberta, Edmonton, AB, Canada
Exercise is a commonly prescribed therapy for patients with established cardiovascular disease or those at high risk for de novo disease. Exercise-based, multidisciplinary programs have been associated with improved clinical outcomes post myocardial infarction and is now recommended for patients with cancer at elevated risk for cardiovascular complications. Imaging studies have documented numerous beneficial effects of exercise on cardiac structure and function, vascular function and more recently on the cardiovascular risk profile. In this contemporary review, we will discuss the effects of exercise training on imaging-derived cardiovascular outcomes. For cardiac imaging via echocardiography or magnetic resonance, we will review the effects of exercise on left ventricular function and remodeling in patients with established or at risk for cardiac disease (myocardial infarction, heart failure, cancer survivors), and the potential utility of exercise stress to assess cardiac reserve. Exercise training also has salient effects on vascular function and health including the attenuation of age-associated arterial stiffness and thickening as assessed by Doppler ultrasound. Finally, we will review recent data on the relationship between exercise training and regional adipose tissue deposition, an emerging marker of cardiovascular risk. Imaging provides comprehensive and accurate quantification of cardiac, vascular and cardiometabolic health, and may allow refinement of risk stratification in select patient populations. Future studies are needed to evaluate the clinical utility of novel imaging metrics following exercise training.
Introduction
Regular physical exercise provides many benefits to the cardiovascular system and overall health at all stages of life. As such, aerobic exercise (e.g., walking, cycling) and more recently resistance exercise (e.g., weightlifting), have been an integral component of clinical guidelines, and the cornerstone of cardiac rehabilitation for patients with or at risk for cardiovascular disease including coronary artery disease (CAD), heart failure, and cancer (1–4).
The most common form of exercise for primary and secondary prevention of cardiovascular disease is aerobic training, which improves oxygen delivery (i.e. cardiac output) during physical effort, and resistance training, which increases skeletal muscle mass and strength. Patients with established cardiovascular disease are commonly referred to cardiac rehabilitation, which typically involves a 6–24-week program of supervised, moderate-intensity (50–75% of maximal heart rate or 40–60% of heart rate reserve) continuous aerobic exercise, supplemental resistance training and other health interventions. High-intensity interval training (brief periods of 85–100% maximal heart rate or 80–90% heart rate reserve alternated with rest or low intensity) is a time efficient aerobic exercise alternative for lower risk patients and appears to offer similar health benefits (5). Exercise training interventions have been shown to exert direct and indirect beneficial effects on the cardiovascular risk profile. Aerobic and resistance training prevent or reduce insulin resistance and diabetes mellitus type II (6), and have favorable effects on blood pressure, lipid profile, vascular inflammation, body composition, and overall cardiac function in patients with established cardiac disease as well as healthy individuals (7–9). Dietary counseling for optimal nutritional study also plays an integral role in most rehabilitation programs and targeted nutrition interventions can improve body composition, metabolic and cardiovascular health (10).
Imaging provides a valuable modality to quantify the effects of exercise training and multimodal rehabilitation as it enables an evaluation of the physiological and morphological adaptations of the heart and vasculature. Imaging is frequently used to characterize the impact of exercise training interventions on cardiac function and remodeling, two prognostic measures that help to guide the therapeutic management of patients with cardiac disease. Echocardiography and magnetic resonance imaging (MRI) are the primary imaging modalities used to evaluate the cardiovascular effects of exercise based on their accuracy, versatility, and safety profile for repeat testing. In assessing the heart, these modalities are principally used to provide information on ventricular size, mass, and function as well as hemodynamic and flow quantification. Cardiac MRI also allows the evaluation of myocardial tissue characterization, including macro- and microscopic fibrosis using quantitative mapping sequences and contrast enhanced imaging (Figure 1). In assessing the vasculature, these modalities are primarily used to evaluate vascular function, stiffness, and structure. These imaging modalities are increasingly applied in real time to evaluate dynamic changes in cardiovascular function during exercise to unmask occult dysfunction that may not be identified at rest (11, 12). Furthermore, our group has also used MRI to evaluate relevant extra-cardiac sequelae of cardiac disease such as lung water content in patients with potential heart failure, regional adipose tissue deposition as a metric of cardiovascular risk, and skeletal muscle volume and function impairments (13–15).
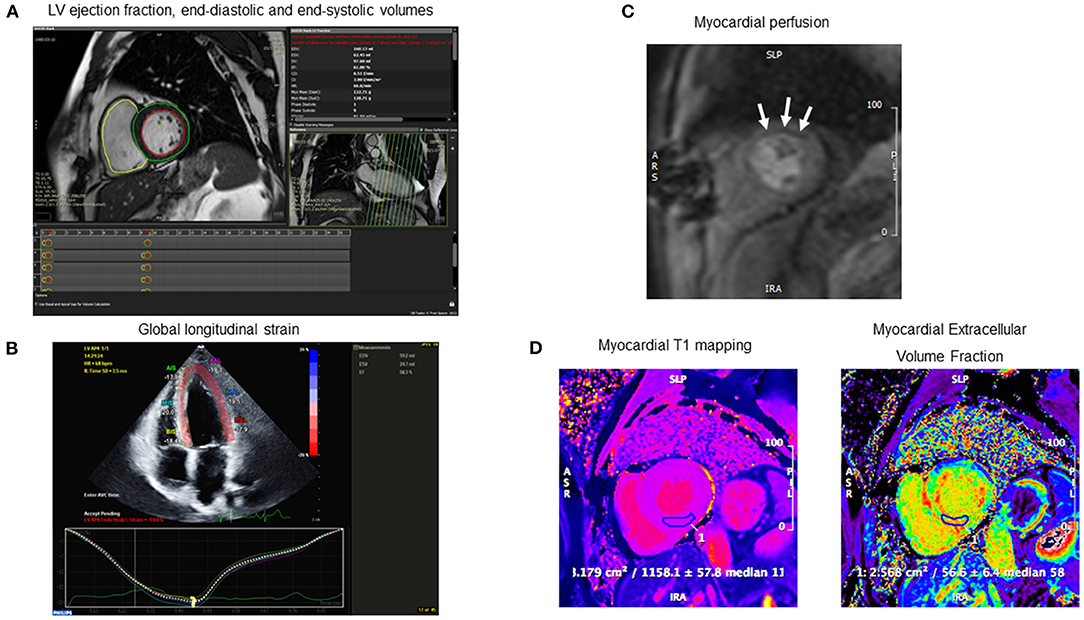
Figure 1. Examples of cardiac imaging metrics to assess the effects of exercise training in patients with or at risk for cardiovascular disease. (A) Left ventricular volumetric analysis of short-axis steady state free precession (SSFP) images acquired from cardiac MRI. (B) Global longitudinal strain acquired transthoracic echocardiogram. (C) Myocardial perfusion acquired from first-pass gadolinium enhanced imaging on vasodilator stress cardiac MRI. Note anterolateral perfusion defect (white arrows). (D) Myocardial tissue characterization on cardiac MRI with native T1 mapping (left) and extracellular volume fraction (ECV) (right). Note the elevated myocardial T1 (1,158 ms) and ECV (57%) consistent with cardiac amyloidosis.
In this review article, we will review the potential benefits of exercise on left ventricular (LV) function and remodeling, vascular function and structure, and body composition in adult patients with or at risk for cardiovascular disease and discuss the application and utility of imaging to characterize these changes.
Exercise Training Effects on Cardiac Structure and Function in Healthy Individuals
Before examining the role of exercise training in patients with cardiovascular disease, it is important to understand its effects in healthy individuals. Public health recommendations for the general population are to include 150 min per week of moderate-intensity aerobic exercise or 75 min if the intensity is classified as vigorous (16). Sustained (e.g., several hours) endurance exercise has been linked to increased circulating biomarkers of myocardial stress including cardiac troponin T and N-terminal-pro-B-type natriuretic peptide (NT-pro-BNP) (17). However, it has been postulated that BNP and NT-pro-BNP elevation in this setting may have cytoprotective and growth regulatory effects and thus be indicative of physiological stress rather myocardial damage (17).
Soluble suppression of tumorigenicity 2 (sST2) is an emerging prognostic biomarker that has been associated with myocardial fibrosis, inflammation, and function and is an independent predictor of long-term mortality (18). High-intensity and duration exercise such as a marathon race has been linked to increased levels of sST2 (19). This association might explain the increased incidence of myocardial scar on cardiac MRI late gadolinium enhancement imaging among athletes who practice intense endurance training compared to the general population (20). Although, the clinical significance of this finding in athletes is unclear and further studies are needed. Cardiac imaging, in particular echocardiography and MRI, have a vital role in examining geometric changes that may occur in athlete's hearts. Aerobic exercise training has been associated with LV dilation and hypertrophy, right ventricular and/or biatrial dilatation (21, 22). Therefore, to differentiate between exercise-induced physiological cardiac remodeling and cardiomyopathy, a recent meta-analysis of 27 studies defined normal reference values of biventricular size and function estimated by cardiac MRI in competitive athletes. Compared to the general population, competitive athletes generally have higher resting biventricular end-diastolic and end-systolic volumes, and stroke volume (22, 23). However, an important caveat is that most patients with cardiovascular disease will not complete the volume of aerobic exercise necessary to induce physiological remodeling.
Influence of Sex and Age on the Effects of Exercise Training on Cardiac Function
Sex
Ventricular volumes and mass are lower in females compared to males however cardiac function is comparable between sexes (23). Compared to men, women of comparable training status and age, typically have 5–15% lower peak VO2 after adjustment for body weight and lean body mass (24). A primary determinant of lower peak VO2 among women is lower peak exercise cardiac output relative to men (25). Given that women demonstrate a similar heart rate during exercise as men (26, 27), lower stroke volume is the primary contributor to the attenuated cardiac output response during exercise (28, 29). Additionally, sex differences exist for myocardial remodeling after injury and cardiovascular overload (e.g., training responses) (30). A recent meta-analysis of 26 studies (n = 468 healthy individuals) found similar LV hypertrophy adaptations to endurance training among men and women, but that LV end-diastolic, end-systolic and stroke volumes increased more in men (31). In addition, women appear to experience greater improvements in arteriovenous oxygen difference with endurance training compared to men (32–34). The sex differences literature on exercise training responses is primarily limited to healthy adults, as the studies of patients with heart failure have been predominantly performed in men (35). Several research gaps also exist regarding potential sex differences in the vascular adaptations to endurance training (36).
Estrogen levels have been shown to play an important role in attenuation of pathologic pressure-overload LV hypertrophy but may not affect exercise-related physiological hypertrophy in rodents (37, 38). Therefore, while menopausal status may be a determinant of pathological hypertrophy between the sexes, other factors may explain differences in cardiac adaptations to exercise training (30, 39). In younger, premenopausal women, the adaptations to endurance training are more likely related to peripheral determinants as opposed to LV adaptations, as seen in men (27, 36, 40, 41). Older, post-menopausal women experience similar peripheral adaptations to endurance training but appear to have a smaller increase in peak VO2 and blood volume compared to premenopausal women (32). Spina et al. demonstrated that among older (60–70 years) women, 9–12 months of endurance training increased peak VO2 and arteriovenous oxygen difference, but LV end-diastolic and stroke volumes did not change (40). Nio et al. compared training responses to 12 weeks of endurance training among pre- vs. post-menopausal women and found that post-menopausal women experienced a smaller increase in peak VO2 and blood volume, but no differences in cardiac output, heart rate, or LV volumes compared to premenopausal women (32).
Age
Ventricular volumes decrease with age but systolic function is unchanged with aging (23). Cardiovascular function among older adults tends to be impaired relative to younger adults owing to a number of age-related changes including worsening cardiac mechanics, decreased responsiveness to ß-adrenergic stimulation and increased vascular and aortic stiffness (36, 42–45). Among healthy, sedentary adults, there is a rate of decline in peak VO2 of an average of 5–10% per decade due to both structural and functional changes in central and peripheral determinants (27, 36, 40, 46). However, endurance training can attenuate the rate of age-related decline in peak VO2. While endurance training increases peak VO2 in older men and women, the magnitude of adaptation is less in older women relative younger controls (32, 47). The attenuated training adaptations appear to be related to lower improvements to cardiac output, stroke volume, and LV function among older vs. younger adults (27, 46). Older women experience similar improvements to peak VO2 in response to endurance training as older men, but there appears to be a sex difference in the responsible mechanisms. Prospective studies have demonstrated that older men are more likely to experience central adaptations such as improvements in cardiac output, stroke volume, and LV systolic function after 9–12 months of endurance training (48, 49). Conversely, older women are more likely to experience peripheral adaptations such as increased arteriovenous oxygen difference with minimal concurrent improvements in stroke volume (32, 33, 40, 49). Regular aerobic exercise appears to attenuate the age-related aortic stiffening among healthy men and women (36, 46) (Table 1).
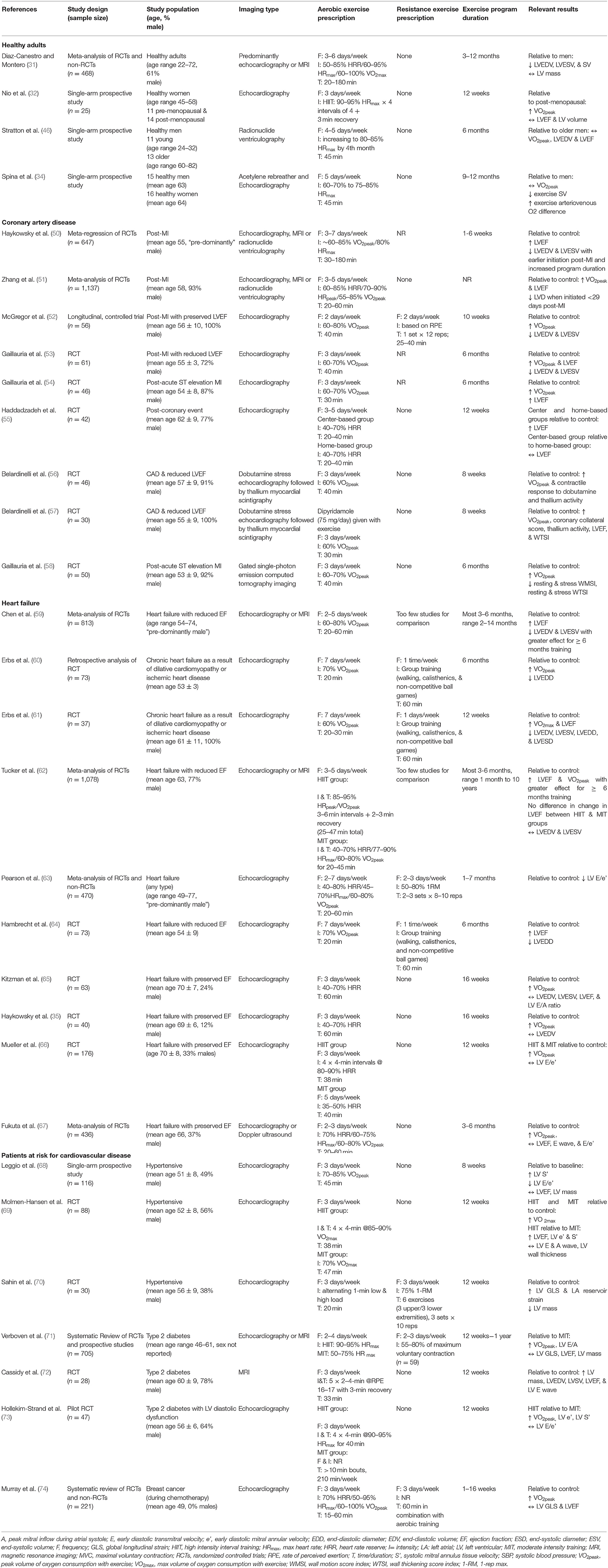
Table 1. Characteristics of the imaging studies reporting on the cardiac effects of exercise training.
Exercise Training Effects on Cardiac Structure and Function in Patients With Established Cardiovascular Disease
Cardiac Remodeling in Cardiovascular Disease States: Clinical Significance
Left ventricular remodeling after myocardial injury is defined as a structural adaptation in chamber size and shape, arising from complex biochemical and cellular changes. This deleterious cascade leads to varying degrees of LV dilatation, hypertrophy and extra-cellular collagen deposition, manifesting clinically as myocardial stiffness, and/or LV dysfunction (75). The extent of remodeling is often used as a surrogate for cardiac disease progression and is an emerging therapeutic target in heart failure. Therapeutic interventions, including cardiac rehabilitation, have been used to attenuate cardiac remodeling, typically defined as a ≥ 10% increase in LV end-diastolic or end-systolic volume in the post-myocardial infarction (MI) or heart failure setting (76). Cardiac imaging routinely provides data on remodeling-specific markers, such as LV volumes and ejection fraction (EF), which elucidate prognosis and guide the potential need for further intervention (76).
Studies of structured, exercise-based cardiac rehabilitation for patients with heart failure or post-MI have been associated with improved cardiac function and attenuation of ventricular remodeling in addition to a reduction in cardiovascular mortality (77, 78). Thus, these programs have been implemented as a class I recommendation in contemporary guidelines for patients with cardiac diseases (1–4). Cardiac imaging is commonly used to evaluate the effects of exercise training on LV size and function in conjunction with other therapeutic interventions such as pharmacotherapy, coronary revascularization and cardiac resynchronization.
Exercise Training Effects in Patients With Coronary Artery Disease
Echocardiography has been the predominant modality used to study the effects of exercise training on cardiac remodeling in patients with CAD. Pooled analyses of clinical trial data suggest that the timing and duration of the exercise intervention is important. For example, these studies have shown that the attenuation of cardiac remodeling occurs when exercise is initiated early, within 1 week of hospitalization in clinically stable, post-MI patients and is continued for at least 6 months (50, 51). These salient effects on LV remodeling post-MI have been described in patients with preserved LV function (52) as well as moderate systolic dysfunction (53). Randomized controlled trials of exercise training have demonstrated improvements in LVEF ranging from 5 to 15% (51, 54, 55). There is also preliminary evidence to suggest that exercise may improve diastolic function. A randomized controlled trial evaluating the effects of exercise on diastolic function post MI found that E wave and E/A ratio by Doppler echocardiography increased by 0.2 in the exercise group, suggesting enhanced LV filling and reduced LV wall stress (53).
Exercise training and cardiac rehabilitation are effective strategies for limiting morbidity and mortality inpatients with coronary artery disease (77, 78). The mechanisms underlying this reduction in mortality are likely multifactorial but include reductions in cardiovascular risk factors as well as improved myocardial perfusion, which can be attributed to increased coronary artery collateralization and vasorelaxation as well as reduced vascular oxidative stress (79, 80). Improved coronary artery collateralization secondary to exercise training has been demonstrate using Thallium scintigraphy and gated SPECT with technetium-99 m sestamibi (56–58, 81). Coronary autoregulation is influenced by the interplay between nitric oxide production by the endothelium and inactivation by reactive oxygen species. In patients with CAD, coronary vasomotor tone is disturbed due to an imbalance in nitric oxide metabolism, however, exercise training restores nitric oxide availability and is largely responsible for the improvement in myocardial perfusion (81).
In summary, imaging studies demonstrate that exercise training has favorable effects on LV remodeling, systolic and diastolic function as well as myocardial perfusion in patients with CAD (Table 1).
Exercise Training Effects in Patients With Heart Failure
In patients with heart failure and reduced ejection fraction (HFrEF) (LVEF < 40%), exercise training has been shown to reverse LV remodeling, with favorable effects on ejection fraction, stroke volume, and end-diastolic and end-systolic diameters (59–62).
However, similar to patients with CAD, the length of training program needed to have measurable positive effects was 6 months or longer (59, 60, 62). In one recent meta-analysis, LVEF was observed to improve with a mean difference of 6.3% in trials of ≥ 6 months duration vs. 2.3% for studies of shorter duration (62). Similarly, clinical trials of patients with reduced ejection fraction (LVEF < 35%) found that exercise training of 3 months duration or less did not impact relevant circulating cardiac biomarkers: NT-pro-BNP, high sensitivity C-reactive protein, and cardiac troponin (82). Regarding the type of exercise, the meta-analysis reported that high intensity interval training (HIIT) or combined aerobic and resistance training was not superior to moderate intensity continuous training for improvement in LVEF (62). Diastolic function also likely improves with exercise training. A meta-analysis of patients with HFrEF found that aerobic training induced a mean difference of −2.85 in E/E' ratio (early diastolic filling over diastolic tissue velocity) relative to controls, suggesting improved myocardial relaxation (63). However, this exercise-induced improvement in cardiac function is possibly explained by changes to vascular tone rather than direct cardiac effects. Using echocardiography and right-heart catheterization, Hambrecht et al. showed that 6 months of training in patients with HFrEF lowered total peripheral resistance, which strongly correlated to concurrent increased stroke volume (64). In studies of patients with heart failure and preserved ejection fraction (HFpEF) (LVEF ≥ 50%), exercise training did not result in any significant changes in LV volume, systolic function and diastolic function but did lead to a mild improvement in cardiorespiratory fitness [improves peak oxygen uptake (VO2) by ~2 ml/kg/min] (65). Furthermore, measurements of carotid artery distensibility and brachial artery flow mediated dilatation were also unaffected by aerobic training in this study (65). However, exercise trained patients with HFpEF exhibit increased arterial-venous oxygen difference after 4 months, consistent with improved skeletal muscle oxygen uptake and/or extraction by active muscles (35). A recent randomized controlled trial of HIIT, moderate intensity intermittent training or guideline-based control in 180 patients with HFpEF confirmed no improvement in echo-derived diastolic function (E/E') and left atrial volume or cardiorespiratory fitness (peak VO2) after 12 months of training (66). An earlier meta-analysis also reported no change in cardiac imaging parameters and minor improvement in exercise performance in patients with HFpEF (67) (Table 1).
Exercise Training Effects on Cardiac Structure and Function in Patients at Risk for Cardiovascular Disease
Patients With Hypertension
Uncontrolled systemic hypertension may lead to LV hypertrophy and ultimately to heart failure (83). Moderate-intensity aerobic exercise training is recommended in patients with hypertension has been associated with 2-3 mmHg reductions in both systolic and diastolic blood pressure (84). Single-site imaging studies of exercise training in hypertensive patients also suggest associated improvement in cardiac function. An observational study of 116 patients undergoing 8 weeks of moderate-to-high intensity aerobic training (70–85% of peak heart rate) found no change in LVEF or LV wall thickness however tissue Doppler echocardiography and strain imaging suggested a post-intervention improvement in systolic and diastolic function (68). In a randomized study of 88 patients with hypertension, Molmen-Hansen et al. showed that high-intensity interval training (>90% maximal heart rate) improved 24-h ambulatory blood pressure, increased LVEF from 58 to 65%, increased E' from 8.06 to 9.26 cm/s, but with no change in LV wall thickness (69). A recent study of 30 hypertensive patients participating in 10 weeks of cardiac rehabilitation improved blood pressure control, decreased LV mass from 104 to 97 g/m2 and improved LV global longitudinal strain from 19.8 to 20.7% (70). Interestingly, a meta-analysis of 16 studies of normotensive and hypertensive patients showed that isometric resistance training lowered systolic blood pressure in both groups, however potential associated effects on cardiac structure and function have not been elucidated (85) (Table 1).
Patients With Diabetes Mellitus
Diabetes mellitus is an independent risk factor for the development of atherosclerosis and heart failure. Diabetic cardiomyopathy is characterized by two distinct phenotypes, LV dilatation with systolic dysfunction or concentric LV hypertrophy with diastolic dysfunction (86). Glycemic control has been shown to influence cardiac function in individuals with diabetes (87), however, even individuals with well-controlled diabetes (defined by HbA1c < 7.5%) have subclinical myocardial dysfunction demonstrated by a significantly reduced longitudinal strain reserve on stress echocardiography (88). A systematic review of exercise training in individuals with diabetes suggested an improvement in diastolic function (E/A) but no consistent effect on systolic function or LV remodeling on echocardiography or MRI (71). Nevertheless, this apparent effect of exercise training is likely important because diastolic dysfunction with preserved LVEF is a common phenotype in patients with diabetic cardiomyopathy (86).
Two studies have suggested that high-intensity intermittent exercise may be superior to moderate-intensity exercise in reversing diabetes-associated myocardial impairment, with demonstrated improvement in systolic and diastolic function (72, 73). However, high-intensity training also increases LV wall mass and end-diastolic volume, presumably due to changes in loading conditions (72) (Table 1).
Patients With Cancer
Cancer therapy-related cardiac dysfunction (CTRCD) is an important clinical consideration for patients receiving anthracycline and/or trastuzumab-based adjuvant treatment. Early recognition and management of CTRCD is important as it has the potential to delay cancer treatment and directly impact cardiovascular morbidity and mortality. Cardiac imaging plays a critical role in the early detection and monitoring of CTRCD. This adverse complication is most commonly assessed by measuring LVEF by echocardiography, multi-gated blood pool imaging or cardiac MRI (89). Left ventricular global longitudinal strain is also recommended as a sensitive metric to. detect early LV dysfunction (89).
While pre-clinical models suggest that aerobic exercise training prevents cancer therapy-related cardiotoxicity, this has not translated to the clinical setting in the available research to-date. A recent meta-analysis of 8 studies (221 patients) of exercise training in patients with breast cancer receiving trastuzumab and/or anthracyclines showed no consistent benefit in the prevention of LV dilatation or worsening systolic function as assessed by echocardiography or cardiac MRI (74). However, exercise did improve cardio-respiratory fitness as measured by peak VO2 (Table 1).
Cardiac Imaging With Exercise Stress
Cardiac reserve function is defined as the difference in cardiac function from rest to peak exercise captured by echocardiography or cardiac MRI. Its potential importance stems from its ability to detect stress-induced myocardial dysfunction and evaluate exercise-related changes to chronotropy, inotropy, lusitropy, and vasodilation in patients with normal resting parameters (90).
In patients with HFpEF, a number of metrics have been studied with exercise imaging including LVEF, LV global longitudinal strain, E/E' ratio, mitral annular systolic velocity, and tricuspid regurgitation maximal velocity (91–93). Resting echocardiography, electrocardiogram, and serum biomarkers such as NT-pro-BNP often fail to identify patients with HFpEF, whereas a stress echocardiogram has been shown to be more sensitive for this diagnosis (93). Exercise echocardiography can be performed using a treadmill or a cycle ergometer. The latter having the advantage of imaging being performed in a semi-supine position and therefore does not require patient transfer and allows for image acquisition at incremental workloads.
Exercise stress imaging with cardiac MRI offers the advance of enhanced accuracy and reproducible quantification of cardiac volumes and biventricular function relative to other imaging modalities (94, 95). The exercise modes employed with cardiac MRI include a supine cycle ergometer or stepper device attached to the scan table to allowing real-time image acquisition while the patient is exercising, instantaneous with achievement of peak exercise or by exercise performed outside of the bore with quick transfer of patients to the MRI table for image acquisition (96, 97). Exercise-related increased respiratory rates and cardiac translation introduce both patient-related difficulties in breath-holds and ECG signal, which are required for the standard cardiac MRI acquisition methods (97). Our group recently validated a real-time, free-breathing approach that remedies these exercise-related issues in patients with cardiovascular risk factors and/or cancer (97) (Figure 2).
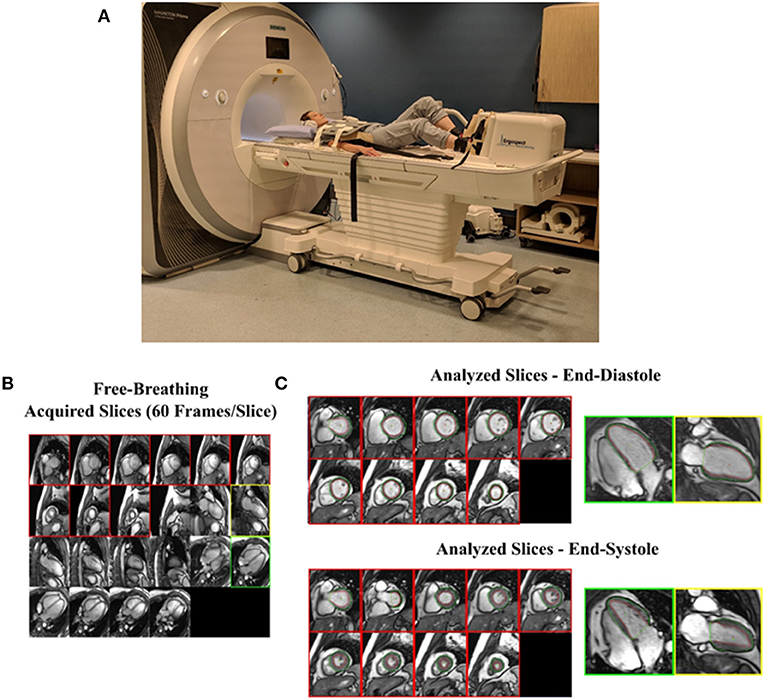
Figure 2. Real-time imaging of cardiac function during exercise using cardiac MRI. (A) Healthy volunteer in supine position outside of magnet bore using MRI conditional stepper device to achieve maximal aerobic activity. (B) All short-axis and long-axis slices are viewed simultaneously to select those for volumetric analysis. Short-axis slices with myocardium and a single 2- and 4-chamber view are chosen. (C) A full cardiac cycle for each selected slice is extracted from which end-diastolic and end-systolic images are identified and endocardial (red) and epicardial (green) borders are traced. Modified from Kirkham et al. (97).
Exercise Training Effects on Vascular Health
Imaging Metrics of Vascular Structure and Function
Age, cardiometabolic disease and toxic exposures (e.g., smoking, chemotherapy) each affect the arterial wall matrix, reduce elasticity and thus increase the potential for arterial stiffening and impaired blood flow (98). Endothelial dysfunction is also common in patients with and at risk for atherosclerotic disease and can increase arterial tone and stiffness due to impaired vascular smooth muscle function (98). Arterial stiffness is typically derived from the assessment of pulse wave velocity (PWV) in a vessel segment and is measured non-invasively using tonometry or cuff-based technologies as well as by imaging on Doppler ultrasound or MRI-derived phase velocity imaging (99). These imaging modalities also allow for complementary information on arterial wall thickness (B-mode or M-mode ultrasound) (100) and aortic distensibility (MRI cine imaging) (101) (Figure 3). Measures of arterial stiffness are clinically relevant as they predict future cardiovascular events (102–104) incident hypertension (105) and heart failure (106). Hence, therapies directed at attenuating vascular dysfunction are desirable. Aerobic exercise training is known to improve cardiovascular risk factors modulating arterial stiffness (4) and some studies also suggest direct effects on improving vascular function (107, 108).
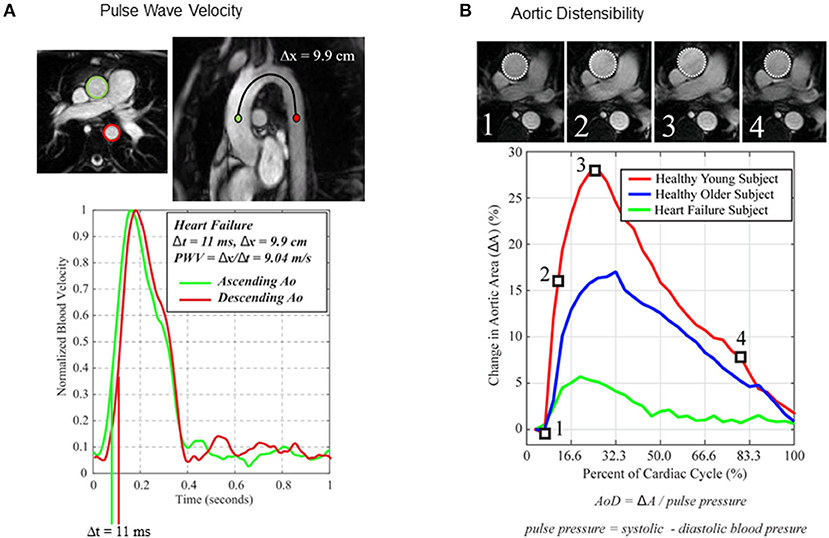
Figure 3. Imaging techniques used to assess the effects of exercise training in patients with or at risk for cardiovascular disease. (A) MRI derived arterial stiffness. Pulse wave velocity (PWV) estimated from MRI derived phase velocity imaging of the thoracic aorta in an older patient with heart failure. Adapted from Thompson et al. (101). (B) Thoracic aorta distensibility (AoD) on SSFP cines. Comparison of aortic distensibility between a young healthy individual, an older healthy individual and an older patient with heart failure.
Exercise Training Effects on Vascular Function in Patients With Established Cardiovascular Disease
Patients With Coronary Artery Disease
Aerobic exercise training has been linked to reduced arterial stiffness in patients with CAD. A systematic review of 5 studies of arterial stiffness in patients with CAD found that aerobic training was associated with lower PWV measures on Doppler ultrasound (109). One study suggested that the magnitude of benefit was greater for participants who trained for 20 weeks compared to 12 weeks, change in PWV −1.0 vs. −0.6 m/s, respectively (110). However, these studies were non-randomized, small (range 20–119 participants) and did not control for potential confounders. In contrast, a randomized-controlled trial of 12 months of combined aerobic and resistance training in 137 patients with CAD and diabetes mellitus found no effect of exercise on IMT measures (111). Although, subgroup analysis suggested improved IMT measures in patients without carotid plaques at baseline (Table 2).
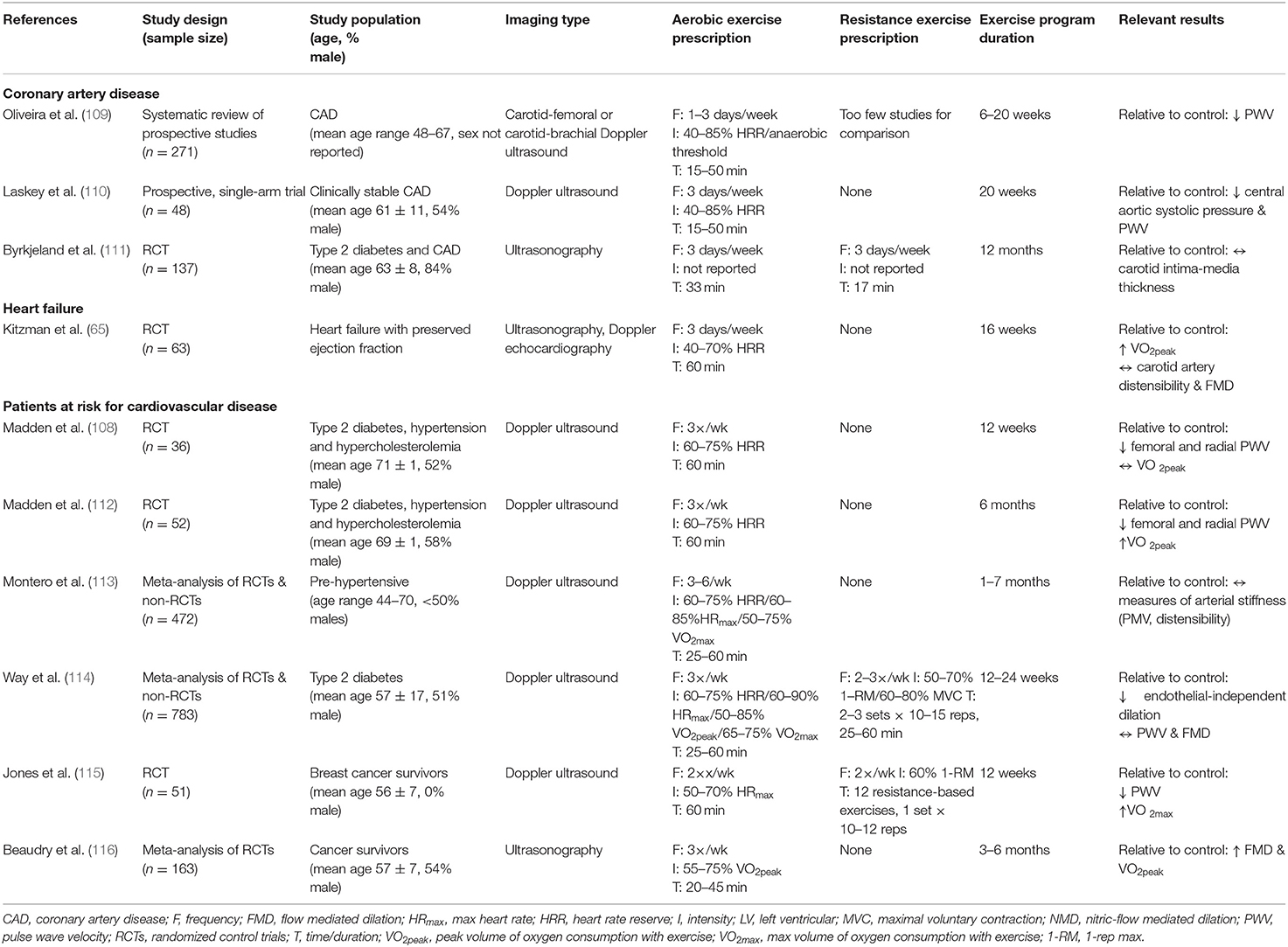
Table 2. Characteristics of the imaging studies reporting on the vascular effects of exercise training.
Patients With Heart Failure
Vascular function is believed to play an integral role in the development and progression of heart failure, particularly with preserved ejection fraction (117). Indeed, carotid artery distensibility derived from B-mode ultrasound is significantly reduced in patients with HFpEF compared to healthy controls and is also directly related to cardiorespiratory fitness (peak VO2) (118). In a cross-sectional study of 143 patients attending cardiac rehabilitation, a significant correlation between indices of arterial stiffness and cardiorespiratory exercise tolerance was found in patients with preserved ejection fraction but not in patients with reduced ejection fraction (119). However, a randomized, controlled trial of 16 weeks of combined aerobic and resistance training for patients with HFpEF found no improvement in carotid artery distensibility or in brachial artery flow mediated dilatation despite improvement in cardiorespiratory fitness (65). While vascular function appears to be an important determinant of exercise tolerance in patients with HFpEF, it does not appear to be improved by exercising training (Table 2).
Exercise Training Effects on Vascular Function in Patients at Risk for Cardiovascular Disease
Early studies suggested that 3 months of aerobic exercise training in patients with cardiovascular risk factors (hypertension, diabetes mellitus and dyslipidemia) reduced arterial PWV (108). However, these salient effects on arterial stiffness were an early response to exercise as they were not sustained at 6 months of aerobic training (112). Similarly, meta-analyses of predominantly aerobic exercise training in individuals with hypertension or diabetes found no improvement in the non-invasive assessment of arterial stiffness (113, 114). However, subgroup analysis in hypertensive patients suggested improvement with interventions of longer duration (113). Data on exercise training and arterial stiffness in patients with cancer is lacking however one recent study of 12 weeks of circuit resistance training reported significant improvement in cardiorespiratory fitness (VO2 max + 4.3 ml/kg/min) and PWV (−0.9 m/s) in 51 patients (115). A recent meta-analysis of exercise training in patients with breast or prostate cancer (163 total patients) reported that aerobic exercise improved vascular endothelial function on ultrasound (116) (Table 2).
Exercise Training Effects on Cardiac and Extra-Cardiac Tissue Structure and Composition
Late gadolinium enhancement sequences on cardiac MRI are frequently utilized to identify and quantify myocardial scar (replacement fibrosis) arising from acute ischemic injury (120). Follow-up studies of patients with acute MI, have shown that infarct size decreases by 16% on late gadolinium enhancement imaging (121). However, the effect of exercise training on infarct size has not been well-elucidated. Quantitative mapping MRI sequences also allow the characterization of both myocardial and extra-cardiac tissues to provide valuable insight into whole-body cardiovascular health profile. Elevated myocardial T1 times and extracellular volume metrics have been validated as indicators of edema in acute disease and interstitial reactive fibrosis in chronic conditions and are increasingly used as risk markers in patients at risk for or with established cardiovascular disease (122, 123). For example, Reinstadler et al. showed that in patients with ST elevation myocardial infarction, native T1 values in remote, non-infarcted myocardium independently predicted adverse cardiovascular outcomes at 6 months (124). Similarly, Vita et al. showed that in patients with non-ischemic dilated cardiomyopathy, elevated myocardial extracellular volume predicts long-term heart failure outcomes (125). Increased myocardial T1 and extracellular volume have also been reported in cancer survivors with previous exposure to anthracycline-based chemotherapy (126, 127). Kirkham et al., reported that native myocardial T1 was elevated compared to controls and correlated with cardiorespiratory fitness among anthracycline-treated breast cancer survivors, suggesting that this metric of myocardial microarchitecture has important functional implications (128). A recent non-randomized study of 27 patients with breast cancer undergoing 4 months of exercise training during anthracycline-based chemotherapy found no effect of exercise on native myocardial T1 but not report on extracellular volume fraction (129). To our knowledge, there have been no reports on the effects of exercise training on myocardial T1 and extracellular volume fraction (i.e., myocardial reactive fibrosis ± edema) in patients with established cardiovascular disease or with cardiovascular risk factors.
It is now well-established that the location of fat deposition is much more closely linked to cardiovascular disease risk than the total quantity of fat (130). For instance, subcutaneous fat (located under the skin) accounts for 80–95% of total body fat but has relatively benign cardiometabolic consequences (130). When the finite limit of the expansion capacity of subcutaneous fat is reached, or in the presence of toxic exposures (e.g., smoking or chemotherapy), fat deposition occurs in ectopic “overflow” sites instead, including around the visceral organs (visceral), in skeletal muscle (intermuscular), in liver cells, or around the heart (epicardial) (130). Ectopic fat only accounts for 5–15% of total body fat but has much worse cardiometabolic consequences. For example, visceral and intermuscular fat deposition are independent predictors for cardiometabolic disease including hypertension, dyslipidemia, insulin resistance and atherogenesis and are predictive of cardiovascular mortality (131, 132). Furthermore, our group has shown that thigh intermuscular fat is a strong independent predictor of cardiorespiratory fitness level in male and female patients with HFpEF, breast cancer survivors, and females with cardiovascular risk factors (15, 133, 134). We have shown that visceral and intermuscular fat volume at the time of a breast cancer diagnosis predicts later cardiac events (135) and also significantly and rapidly increase with trastuzumab-based chemotherapy treatment (14).
Imaging modalities used to quantify body composition in clinical practice and research include computed tomography, dual energy X-ray absorptiometry and MRI (Figure 4). MRI is uniquely suited to accurately and safely (without ionizing radiation) quantify fat in all of these locations as well as complementary information on cardiac structure and function in a single assessment.
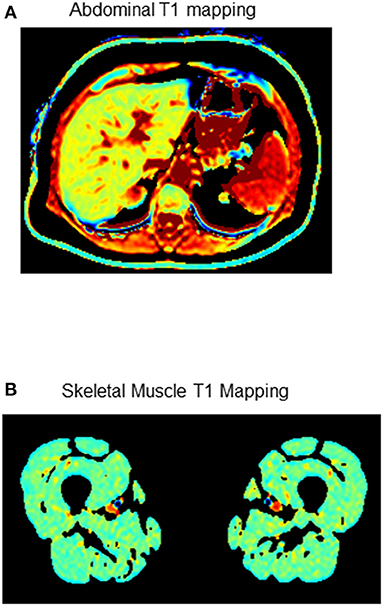
Figure 4. Examples of MRI T1 mapping sequences to assess the extra-cardiac effects of exercise training in patients with or at risk for cardiovascular disease. (A) Abdominal fat density (B) Skeletal muscle fat compartments. Dark signal = fat.
Exercise training and caloric restriction (i.e., reduced daily calorie intake) are the primary interventions used to improve body composition. A meta-analysis comparing the effects of exercise training to caloric restriction in patients with obesity found that both interventions independently reduce visceral fat with a potential trend toward greater reduction with exercise (136). In patients with CAD undergoing cardiac rehabilitation, bioelectrical impedance-derived measures of visceral fat have been shown to improve, but with a greater magnitude of benefit with a greater volume of exercise training (137). Similarly, in a small study of patients with chronic heart failure, 5 months of cardiac rehabilitation also appears to reduce visceral adipose tissue (138). Data on the effects of exercise training in patients with hypertension is limited. However, one study of 156 hypertensive patients randomized to 12 months of exercise training or usual care found 30% reduction in visceral fat area on B-mode ultrasound compared to no effect with antihypertensive pharmacotherapy alone (139). Meta-analyses of the effect of exercise training on visceral fat in both overweight adults and patients with type 2 diabetes mellitus suggest that aerobic exercise training (but not resistance training) appears to provide the greatest benefits (140) (Table 3).
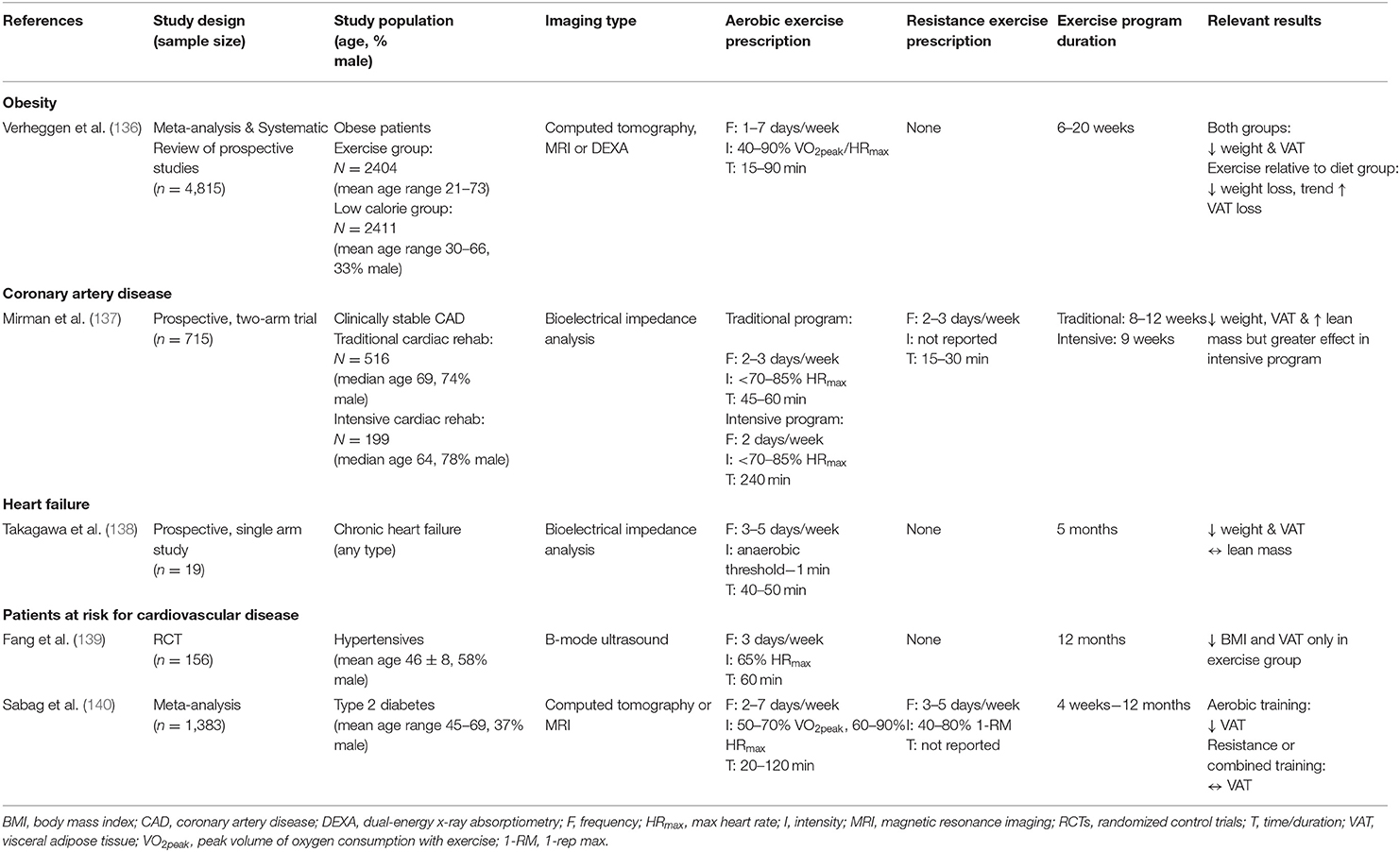
Table 3. Characteristics of the studies reporting on the extra-cardiac effects of exercise training.
Our group is conducting a randomized-controlled trial of a multi-modal intervention including combined aerobic and resistance exercise training and dietary counseling (with no caloric restriction) on LV function in patients receiving cardiotoxic breast cancer treatment and includes quantification of ectopic fat as a secondary outcome measure (141). In a recent randomized controlled trial where exercise was performed shortly after the completion of breast cancer treatment, whole-body, and visceral fat volume were significantly reduced by 16-weeks of combined aerobic and resistance training (142). Therefore, another strategy to reduce cardiovascular risk in this population may be to perform an exercise intervention after treatment completion.
Summary and Future Directions
Exercise training is a cornerstone treatment for patients with cardiovascular disease due its demonstrated impact on risk profile and clinical outcomes. Ultrasound or MR imaging for quantification of changes to the cardiac and extra-cardiac phenotype of patients, provide valuable whole-body information about the cardiovascular risk profile and have an excellent safety profile to allow for repeat testing.
The choice of imaging modality to evaluate changes in cardiovascular structure and function is an important consideration. In general, echocardiography is the most readily available modality and offers a comprehensive assessment of ventricular volumes, mass and function. It can also more easily be used to evaluate cardiac function in real time during exercise on a semi-recumbent bicycle. Cardiac MRI is considered the gold standard imaging test for ventricular volumes, mass and function due to high reproducibility. As mentioned previously, it also allows assessment of cardiac and extra cardiac tissue characterization which has increasingly been linked to prognosis in patients with or at risk for cardiovascular disease. Therefore, cardiac MRI should likely be used to study the effects of exercise training in higher risk patient groups when available.
There is strong evidence supporting a beneficial effect of exercise training, particularly >6 months duration, on cardiac function and remodeling in patients with CAD or heart failure (Table 4). Imaging studies on the effects of exercise training for vascular function in patients with or at risk for cardiovascular disease have shown either modest or no improvement in arterial stiffness. Furthermore, these studies are limited by the small number of participants, non-randomized design and lack of controlling for confounders.
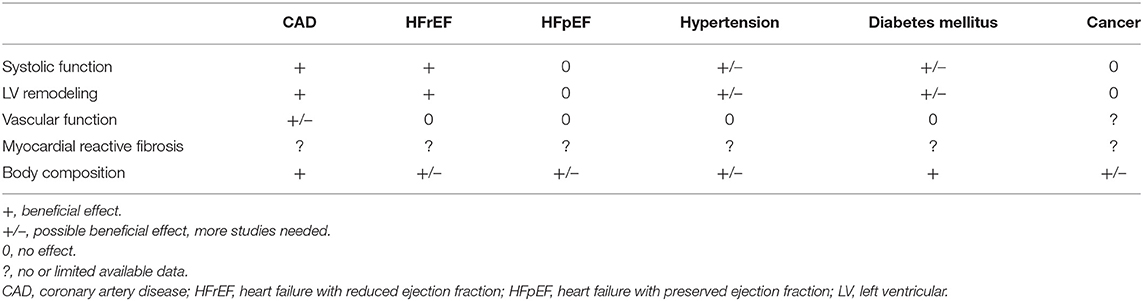
Table 4. Overview of the exercise training effects of on cardiovascular imaging metrics in patients with or at risk for cardiovascular disease.
Ectopic fat deposition, particularly, visceral and intermuscular fat are important indicators of cardiovascular risk that can be reduced by exercise training in numerous patient populations. MRI-acquired myocardial tissue characterization using T1 mapping sequences also provide incremental prognostic information however, the effect of exercise training on this parameter has not well-studied. MRI and/or ultrasound is well-suited for multiparametric evaluation of exercise training interventions and is easily translatable to the clinical setting to help guide patient management. Incorporation of MRI-derived lung water quantification could also help to identify cardiogenic causes of exercise intolerance and evaluate exercise training in patients with subclinical pulmonary edema (13, 143). Exercise cardiac reserve is another attractive approach to evaluating cardiogenic causes of exercise intolerance and detecting subclinical disease, and related studies are underway in the cancer setting (144, 145).
Targeted nutrition interventions can also positively impact cardiovascular health and should be further explored in combination with exercise interventions (146). Dietary patterns and specific nutrients can have independent, synergistic, or additive effects on reducing cardiovascular disease risk (147). Furthermore, nutrition interventions also have a therapeutic role for certain cardiovascular diseases (148). In addition to providing calories and macronutrients, specific nutrients can reduce oxidative damage, increase pro-inflammatory mediators and decrease anti-inflammatory mediators, while also promoting optimal body composition (e.g., decreasing ectopic fat, increase protein synthesis and reducing degradation) (148, 149).
Preclinical models of cardiovascular disease have been used extensively to study the cardioprotective effects of exercise training. Aerobic training has been shown to attenuate cardiac injury in animal models of myocardial infarction (150), ischemic heart failure (151), and preclinical disease (71, 152, 153). These models provide significant insight on the salient effects of exercise in cardiovascular disease and allow extensive histological and biochemical characterization of cardiovascular structure and function in a controlled setting. By comparison, resistance training protocols have been understudied in animal models of cardiovascular disease, in part due to a lack of standardized protocols. Nevertheless, beneficial cardiac and vascular adaptations with resistance training have been reported in small animal models of cardiovascular disease (154). Future preclinical work in exercise training requires refinement and standardization of training protocols to improve study comparisons and to better simulate exercise programs in the clinical setting (155). In conclusion, ultrasound and magnetic resonance-based imaging provides detailed information on the cardiac and extra-cardiac effects of exercise training. Imaging studies provide moderate or good evidence for exercise training to mitigate or improve cardiac remodeling and function in patients with or at risk for cardiovascular disease. Similar work on vascular function suggests a benefit in patients with CAD although these studies were non-randomized and included small numbers of patients. There is no clear benefit of exercise training in vascular function in patients with heart failure, hypertension or diabetes mellitus. There is little data on the effects of exercise training on replacement or reactive myocardial fibrosis. Future work on imaging studies of exercise training should also evaluate the complementary role of nutrition interventions given their importance in cardiac rehabilitation.
Author Contributions
WA and DIP proposed the review subject matter and drafted the manuscript. All authors made critical revisions to the manuscript, contributed to the article, and approved the submitted version.
Funding
MH was supported by the Faculty of Nursing Research Chair in aging and quality of life at the University of Alberta, EP was supported by a Tier 2 Canada Research Chair, and CP was supported by a Campus Alberta Innovates Program Chair in Nutrition, Food, and Health as well as a Canadian Institutes of Health Research new investigator award.
Conflict of Interest
DIP reports consultant fees from Alnylam and Pfizer.
The remaining authors declare that the research was conducted in the absence of any commercial or financial relationships that could be construed as a potential conflict of interest.
Publisher's Note
All claims expressed in this article are solely those of the authors and do not necessarily represent those of their affiliated organizations, or those of the publisher, the editors and the reviewers. Any product that may be evaluated in this article, or claim that may be made by its manufacturer, is not guaranteed or endorsed by the publisher.
References
1. Collet JP, Thiele H, Barbato E, Barthélémy O, Bauersachs J, Bhatt DL, et al 2020 ESC guidelines for the management of acute coronary syndromes in patients presenting without persistent ST-segment elevation: the task force for the management of acute coronary syndromes in patients presenting without persistent ST-segment elevation of the European Society of Cardiology (ESC). Eur Heart J. (2021) 42:1289–367. doi: 10.15829/1560-4071-2021-4418
2. Bozkurt B, Fonarow GC, Goldberg LR, Guglin M, Josephson RA, Forman DE, et al. ACC's heart failure and transplant section and leadership council. Cardiac rehabilitation for patients with heart failure: JACC expert panel. J Am Coll Cardiol. (2021) 77:1454–69. doi: 10.1016/j.jacc.2021.01.030
3. Gilchrist SC, Barac A, Ades PA, Alfano CM, Franklin BA, Jones LW, et al. Cardio-Oncology rehabilitation to manage cardiovascular outcomes in cancer patients and survivors: a scientific statement from the American heart association. Circulation. (2019) 139:e997–1012. doi: 10.1161/CIR.0000000000000679
4. Balady GJ, Williams MA, Ades PA, Bittner V, Comoss P, Foody JM, et al. Core components of cardiac rehabilitation/secondary prevention programs: 2007 update: a scientific statement from the American heart association exercise, cardiac rehabilitation, and prevention committee, the council on clinical cardiology; the councils on cardiovascular nursing, epidemiology and prevention, and nutrition, physical activity, and metabolism; and the American association of cardiovascular and pulmonary rehabilitation. Circulation. (2007) 115:2675–82. doi: 10.1161/CIRCULATIONAHA.106.180945
5. Garber CE, Blissmer B, Deschenes MR, Franklin BA, Lamonte MJ, Lee IM, et al. American college of sports medicine. American college of sports medicine position stand. Quantity and quality of exercise for developing and maintaining cardiorespiratory, musculoskeletal, and neuromotor fitness in apparently healthy adults: guidance for prescribing exercise. Med Sci Sports Exerc. (2011) 43:1334–59. doi: 10.1249/MSS.0b013e318213fefb
6. Kriska AM, Saremi A, Hanson RL, Bennett PH, Kobes S, Williams DE, et al. Physical activity, obesity, and the incidence of type 2 diabetes in a high-risk population. Am J Epidemiol. (2003) 158:669–75. doi: 10.1093/aje/kwg191
7. Sarzynski MA, Burton J, Rankinen T, Blair SN, Church TS, Després JP, et al. The effects of exercise on the lipoprotein subclass profile: A meta-analysis of 10 interventions. Atherosclerosis. (2015) 243:364–72. doi: 10.1016/j.atherosclerosis.2015.10.018
8. Kokkinos P, Myers J. Exercise and physical activity: clinical outcomes and applications. Circulation. (2010) 122:1637–48. doi: 10.1161/CIRCULATIONAHA.110.948349
9. Mathieu P, Poirier P, Pibarot P, Lemieux I, Despres JP. Visceral obesity: the link among inflammation, hypertension, and cardiovascular disease. Hypertension. (2009) 53:577–84. doi: 10.1161/HYPERTENSIONAHA.108.110320
10. Estruch R, Martínez-González MA, Corella D, Salas-Salvadó J, Ruiz-Gutiérrez V, Covas MI, et al. Effects of a mediterranean-style diet on cardiovascular risk factors: a randomized trial. Ann Intern Med. (2006) 145:1–11. doi: 10.7326/0003-4819-145-1-200607040-00004
11. Foulkes S, Claessen G, Howden EJ, Daly RM, Fraser SF, La Gerche A. The utility of cardiac reserve for the early detection of cancer treatment-related cardiac dysfunction: a comprehensive overview. Front Cardiovasc Med. (2020) 7:32. doi: 10.3389/fcvm.2020.00032
12. Pandey A, Khera R, Park B, Haykowsky M, Borlaug BA, Lewis GD, et al. Relative impairments in hemodynamic exercise reserve parameters in heart failure with preserved ejection fraction: a study-level pooled analysis. JACC Heart Fail. (2018) 6:117–126. doi: 10.1016/j.jchf.2017.10.014
13. Thompson RB, Chow K, Pagano JJ, Sekowski V, Michelakis ED, Tymchak W, et al. Quantification of lung water in heart failure using cardiovascular magnetic resonance imaging. J Cardiovasc Magn Reson. (2019) 21:58. doi: 10.1186/s12968-019-0567-y
14. Kirkham AA, Pituskin E, Thompson RB, Mackey JR, Koshman SL, Jassal D, et al. Cardiac and cardiometabolic phenotyping of trastuzumab-mediated cardiotoxicity: a secondary analysis of the MANTICORE trial. Eur Heart J Cardiovasc Pharmacother. (2021) pvab016. doi: 10.1093/ehjcvp/pvab016. [Epub ahead of print].
15. Beaudry RI, Kirkham AA, Thompson RB, Grenier JG, Mackey JR, Haykowsky MJ. Exercise intolerance in anthracycline-treated breast cancer survivors: the role of skeletal muscle bioenergetics, oxygenation, and composition. Oncologist. (2020) 25:e852–60. doi: 10.1634/theoncologist.2019-0777
16. American Heart Association editorial staff,. American Heart Association Recommendations for Physical Activity in Adults Kids. (2018). Available online at: https://www.heart.org/en/healthy-living/fitness/fitness-basics/aha-recs-for-physical-activity-in-adults (accessed August 2, 2021).
17. Scharhag J, Herrmann M, Urhausen A, Haschke M, Herrmann W, Kindermann W. Independent elevations of N-terminal pro–brain natriuretic peptide and cardiac troponins in endurance athletes after prolonged strenuous exercise. Am Heart J. (2005) 150:1128–34. doi: 10.1016/j.ahj.2005.01.051
18. Dieplinger B, Egger M, Haltmayer M, Kleber ME, Scharnagl H, Silbernagel G, et al. Increased soluble ST2 predicts long-term mortality in patients with stable coronary artery disease: results from the ludwigshafen risk and cardiovascular health study. Clin Chem. (2014) 60:530–40. doi: 10.1373/clinchem.2013.209858
19. Aengevaeren VL, VAN Kimmenade RRJ, Hopman MTE, VAN Royen N, Snider JV, Januzzi JL, et al. Exercise-induced changes in soluble ST2 concentrations in marathon runners. Med Sci Sports Exerc. (2019) 51:405–10. doi: 10.1249/MSS.0000000000001806
20. Zhang CD, Xu SL, Wang XY, Tao LY, Zhao W, Gao W. Prevalence of myocardial fibrosis in intensive endurance training athletes: a systematic review and meta-analysis. Front Cardiovasc Med. (2020) 7:585692. doi: 10.3389/fcvm.2020.585692
21. La Gerche A, Taylor AJ, Prior DL. Athlete's heart: the potential for multimodality imaging to address the critical remaining questions. JACC Cardiovasc Imaging. (2009) 2:350–63. doi: 10.1016/j.jcmg.2008.12.011
22. D'Ascenzi F, Anselmi F, Piu P, Fiorentini C, Carbone SF, Volterrani L, et al. Cardiac magnetic resonance normal reference values of biventricular size and function in male athlete's heart. JACC Cardiovasc Imaging. (2019) 12:1755–65. doi: 10.1016/j.jcmg.2018.09.021
23. Kawel-Boehm N, Hetzel SJ, Ambale-Venkatesh B, Captur G, Francois CJ, Jerosch-Herold M, et al. Reference ranges (“normal values”) for cardiovascular magnetic resonance (CMR) in adults and children: 2020 update. J Cardiovasc Magn Reson. (2020) 22:87. doi: 10.1186/s12968-020-00683-3
24. Charkoudian N, Joyner MJ. Physiologic considerations for exercise performance in women. Clin Chest Med. (2004) 25:247–55. doi: 10.1016/j.ccm.2004.01.001
25. Wiebe CG, Gledhill N, Warburton DE, Jamnik VK, Ferguson S. Exercise cardiac function in endurance-trained males versus females. Clin J Sport Med. (1998) 8:272–9. doi: 10.1097/00042752-199810000-00004
26. Fu Q, Levine BD. Cardiovascular response to exercise in women. Med Sci Sports Exerc. (2005) 37:1433–5. doi: 10.1249/01.mss.0000174886.08219.85
27. Ogawa T, Spina RJ, Martin WH III, Kohrt WM, Schechtman KB, Holloszy JO, et al. Effects of aging, sex, and physical training on cardiovascular responses to exercise. Circulation. (1992) 86:494–503. doi: 10.1161/01.CIR.86.2.494
28. Best SA, Okada Y, Galbreath MM, Jarvis SS, Bivens TB, Adams-Huet B, et al. Age and sex differences in muscle sympathetic nerve activity in relation to haemodynamics, blood volume and left ventricular size. Exp Physiol. (2014) 99:839–48. doi: 10.1113/expphysiol.2013.077248
29. Hutchinson PL, Cureton KJ, Outz H, Wilson G. Relationship of cardiac size to maximal oxygen uptake and body size in men and women. Int J Sports Med. (1991) 12:369–73. doi: 10.1055/s-2007-1024696
30. Piro M, della Bona R, Abbate A, Biasucci LM, Crea F. Sex-related differences in myocardial remodeling. J Am Coll Cardiol. (2010) 55:1057–65. doi: 10.1016/j.jacc.2009.09.065
31. Diaz-Canestro C, Montero D. The impact of sex on left ventricular cardiac adaptations to endurance training: a systematic review and meta-analysis. Sports Med. (2020) 50:1501–13. doi: 10.1007/s40279-020-01294-9
32. Nio AQX, Rogers S, Mynors-Wallis R, Meah VL, Black JM, Stembridge M, et al. The menopause alters aerobic adaptations to high-intensity interval training. Med Sci Sports Exerc. (2020) 52:2096–106. doi: 10.1249/MSS.0000000000002372
33. Murias JM, Kowalchuk JM, Paterson DH. Mechanisms for increases in VO2max with endurance training in older and young women. Med Sci Sports Exerc. (2010) 42:1891–8. doi: 10.1249/MSS.0b013e3181dd0bba
34. Spina RJ, Ogawa T, Kohrt WM, Martin WH, Holloszy JO, Ehsani AA. Differences in cardiovascular adaptations to endurance exercise training between older men and women. J Appl Physiol. (1993) 75:849–55. doi: 10.1152/jappl.1993.75.2.849
35. Haykowsky MJ, Brubaker PH, Stewart KP, Morgan TM, Eggebeen J, Kitzman DW. Effect of endurance training on the determinants of peak exercise oxygen consumption in elderly patients with stable compensated heart failure and preserved ejection fraction. J Am Coll Cardiol. (2012) 60:120–8. doi: 10.1016/j.jacc.2012.02.055
36. Seals DR, Nagy EE, Moreau KL. Aerobic exercise training and vascular function with ageing in healthy men and women. J Physiol. (2019) 597:4901–14. doi: 10.1113/JP277764
37. van Eickels M, Grohé C, Cleutjens JP, Janssen BJ, Wellens HJ, Doevendans PA. 17beta-estradiol attenuates the development of pressure-overload hypertrophy. Circulation. (2001) 104:1419–23. doi: 10.1161/hc3601.095577
38. Haines CD, Harvey PA, Leinwand LA. Estrogens mediate cardiac hypertrophy in a stimulus-dependent manner. Endocrinology. (2012) 153:4480–90. doi: 10.1210/en.2012-1353
39. Babiker FA, De Windt LJ, van Eickels M, Thijssen V, Bronsaer RJ, Grohé C, et al. 17beta-estradiol antagonizes cardiomyocyte hypertrophy by autocrine/paracrine stimulation of a guanylyl cyclase A receptor-cyclic guanosine monophosphate-dependent protein kinase pathway. Circulation. (2004) 109:269–76. doi: 10.1161/01.CIR.0000105682.85732.BD
40. Spina RJ, Ogawa T, Miller TR, Kohrt WM, Ehsani AA. Effect of exercise training on left ventricular performance in older women free of cardiopulmonary disease. Am J Cardiol. (1993) 71:99–104. doi: 10.1016/0002-9149(93)90718-R
41. Barnes JN, Fu Q. Sex-Specific ventricular and vascular adaptations to exercise. Adv Exp Med Biol. (2018) 1065:329–46. doi: 10.1007/978-3-319-77932-4_21
42. Spina RJ, Miller TR, Bogenhagen WH, Schechtman KB, Ehsani AA. Gender-related differences in left ventricular filling dynamics in older subjects after endurance exercise training. J Gerontol A Biol Sci Med Sci. (1996) 51:B232–7. doi: 10.1093/gerona/51A.3.B232
43. Lakatta EG. Cardiovascular regulatory mechanisms in advanced age. Physiol Rev. (1993) 73:413–67. doi: 10.1152/physrev.1993.73.2.413
44. Lakatta EG, Gerstenblith G, Angell CS, Shock NW, Weisfeldt ML. Diminished inotropic response of aged myocardium to catecholamines. Circ Res. (1975) 36:262–9. doi: 10.1161/01.RES.36.2.262
45. Lakatta EG, Yin FC. Myocardial aging: functional alterations and related cellular mechanisms. Am J Physiol. (1982) 242:H927–41. doi: 10.1152/ajpheart.1982.242.6.H927
46. Stratton JR, Levy WC, Schwartz RS, Abrass IB, Cerqueira MD. Beta-adrenergic effects on left ventricular filling: influence of aging and exercise training. J Appl Physiol. (1994) 77:2522–9. doi: 10.1152/jappl.1994.77.6.2522
47. Spina RJ. Cardiovascular adaptations to endurance exercise training in older men and women. Exerc Sport Sci Rev. (1999) 27:317–32. doi: 10.1249/00003677-199900270-00012
48. Levy WC, Cerqueira MD, Abrass IB, Schwartz RS, Stratton JR. Endurance exercise training augments diastolic filling at rest and during exercise in healthy young and older men. Circulation. (1993) 88:116–26. doi: 10.1161/01.CIR.88.1.116
49. Ehsani AA, Ogawa T, Miller TR, Spina RJ, Jilka SM. Exercise training improves left ventricular systolic function in older men. Circulation. (1991) 83:96–103. doi: 10.1161/01.CIR.83.1.96
50. Haykowsky M, Scott J, Esch B, Schopflocher D, Myers J, Paterson I, et al. A meta-analysis of the effects of exercise training on left ventricular remodeling following myocardial infarction: start early and go longer for greatest exercise benefits on remodeling. Trials. (2011) 12:92. doi: 10.1186/1745-6215-12-92
51. Zhang YM, Lu Y, Tang Y, Yang D, Wu HF, Bian ZP, et al. The effects of different initiation time of exercise training on left ventricular remodeling and cardiopulmonary rehabilitation in patients with left ventricular dysfunction after myocardial infarction. Disabil Rehabil. (2016) 38:268–76. doi: 10.3109/09638288.2015.1036174
52. McGregor G, Gaze D, Oxborough D, O'Driscol J Shave R. Reverse left ventricular remodelling: effect of cardiac rehabilitation exercise training in myocardial infarction patients with preserved ejection fraction. Eur J Phys Rehabil Med. (2016) 52:370–8.
53. Giallauria F, Cirillo P, Lucci R, Pacileo M, De Lorenzo A, D'Agostino M, et al. Left ventricular remodelling in patients with moderate systolic dysfunction after myocardial infarction: favourable effects of exercise training and predictive role of N-terminal pro-brain natriuretic peptide. Eur J Cardiovasc Prev Rehabil. (2008) 15:113–8. doi: 10.1097/HJR.0b013e3282f00990
54. Giallauria F, Acampa W, Ricci F, Vitelli A, Torella G, Lucci R, et al. Exercise training early after acute myocardial infarction reduces stress-induced hypoperfusion and improves left ventricular function. Eur J Nucl Med Mol Imaging. (2013) 40:315–24. doi: 10.1007/s00259-012-2302-x
55. Haddadzadeh MH, Maiya AG, Padmakumar R, Shad B, Mirbolouk F. Effect of exercise-based cardiac rehabilitation on ejection fraction in coronary artery disease patients: a randomized controlled trial. Heart Views. (2011) 12:51–7. doi: 10.4103/1995-705X.86013
56. Belardinelli R, Georgiou D, Ginzton L, Cianci G, Purcaro A. Effects of moderate exercise training on thallium uptake and contractile response to low-dose dobutamine of dysfunctional myocardium in patients with ischemic cardiomyopathy. Circulation. (1998) 97:553–61. doi: 10.1161/01.CIR.97.6.553
57. Belardinelli R, Belardinelli L, Shryock J. Effects of dipyridamole on coronary collateralization and myocardial perfusion in patients with ischaemic cardiomyopathy. Eur Heart J. (2001) 22:1205–13. doi: 10.1053/euhj.2000.2446
58. Giallauria F, Acampa W, Ricci F, Vitelli A, Maresca L, Mancini M, et al. Effects of exercise training started within 2 weeks after acute myocardial infarction on myocardial perfusion and left ventricular function: a gated SPECT imaging study. Eur J Prev Cardiol. (2012) 19:1410–9. doi: 10.1177/1741826711425427
59. Chen YM, Li ZB, Zhu M, Cao YM. Effects of exercise training on left ventricular remodelling in heart failure patients: an updated meta-analysis of randomised controlled trials. Int J Clin Pract. (2012) 66:782–91. doi: 10.1111/j.1742-1241.2012.02942.x
60. Erbs S, Linke A, Gielen S, Fiehn E, Walther C, Yu J, et al. Exercise training in patients with severe chronic heart failure: impact on left ventricular performance and cardiac size. A retrospective analysis of the leipzig heart failure training trial. Eur J Cardiovasc Prev Rehabil. (2003) 10:336–44. doi: 10.1097/01.hjr.0000099031.38268.27
61. Erbs S, Höllriegel R, Linke A, Beck EB, Adams V, Gielen S, et al. Exercise training in patients with advanced chronic heart failure (NYHA IIIb) promotes restoration of peripheral vasomotor function, induction of endogenous regeneration, and improvement of left ventricular function. Circ Heart Fail. (2010) 3:486–94. doi: 10.1161/CIRCHEARTFAILURE.109.868992
62. Tucker WJ, Beaudry RI, Liang Y, Clark AM, Tomczak CR, Nelson MD, et al. Meta-analysis of exercise training on left ventricular ejection fraction in heart failure with reduced ejection fraction: a 10-year update. Prog Cardiovasc Dis. (2019) 62:163–71. doi: 10.1016/j.pcad.2018.08.006
63. Pearson MJ, Mungovan SF, Smart NA. Effect of exercise on diastolic function in heart failure patients: a systematic review and meta-analysis. Heart Fail Rev. (2017) 22:229–42. doi: 10.1007/s10741-017-9600-0
64. Hambrecht R, Gielen S, Linke A, Fiehn E, Yu J, Walther C, et al. Effects of exercise training on left ventricular function and peripheral resistance in patients with chronic heart failure: a randomized trial. JAMA. (2000) 283:3095–101. doi: 10.1001/jama.283.23.3095
65. Kitzman DW, Brubaker PH, Herrington DM, Morgan TM, Stewart KP, Hundley WG, et al. Effect of endurance exercise training on endothelial function and arterial stiffness in older patients with heart failure and preserved ejection fraction. J Am Coll Cardiol. (2013) 62:584–92. doi: 10.1016/j.jacc.2013.04.033
66. Mueller S, Winzer EB, Duvinage A, Gevaert AB, Edelmann F, Haller B, et al. Effect of high-intensity interval training, moderate continuous training, or guideline-based physical activity advice on peak oxygen consumption in patients with heart failure with preserved ejection fraction: a randomized clinical trial. JAMA. (2021) 325:542–51. doi: 10.1001/jama.2020.26812
67. Fukuta H, Goto T, Wakami K, Kamiya T, Ohte N. Effects of exercise training on cardiac function, exercise capacity, and quality of life in heart failure with preserved ejection fraction: a meta-analysis of randomized controlled trials. Heart Fail Rev. (2019) 24:535–47. doi: 10.1007/s10741-019-09774-5
68. Leggio M, Mazza A, Cruciani G, Sgorbini L, Pugliese M, Bendini MG, et al. Effects of exercise training on systo-diastolic ventricular dysfunction in patients with hypertension: an echocardiographic study with tissue velocity and strain imaging evaluation. Hypertens Res. (2014) 37:649–54. doi: 10.1038/hr.2014.44
69. Molmen-Hansen HE, Stolen T, Tjonna AE, Aamot IL, Ekeberg IS, Tyldum GA, et al. Aerobic interval training reduces blood pressure and improves myocardial function in hypertensive patients. Eur J Prev Cardiol. (2012) 19:151–60. doi: 10.1177/1741826711400512
70. Sahin AA, Ozben B, Sunbul M, Yagci I, Sayar N, Cincin A, et al. The effect of cardiac rehabilitation on blood pressure, and on left atrial and ventricular functions in hypertensive patients. J Clin Ultrasound. (2021) 49:456–65. doi: 10.1002/jcu.22956
71. Verboven M, Van Ryckeghem L, Belkhouribchia J, Dendale P, Eijnde BO, Hansen D, et al. Effect of exercise intervention on cardiac function in type 2 diabetes mellitus: a systematic review. Sports Med. (2019) 49:255–68. doi: 10.1007/s40279-018-1003-4
72. Cassidy S, Thoma C, Hallsworth K, Parikh J, Hollingsworth KG, Taylor R, et al. High intensity intermittent exercise improves cardiac structure and function and reduces liver fat in patients with type 2 diabetes: a randomised controlled trial. Diabetologia. (2016) 59:56–66. doi: 10.1007/s00125-015-3741-2
73. Hollekim-Strand SM, Bjørgaas MR, Albrektsen G, Tjønna AE, Wisløff U, Ingul CB. High-intensity interval exercise effectively improves cardiac function in patients with type 2 diabetes mellitus and diastolic dysfunction: a randomized controlled trial. J Am Coll Cardiol. (2014) 64:1758–60. doi: 10.1016/j.jacc.2014.07.971
74. Murray J, Bennett H, Bezak E, Perry R. The role of exercise in the prevention of cancer therapy-related cardiac dysfunction in breast cancer patients undergoing chemotherapy: systematic review. Eur J Prev Cardiol. (2021) 9:zwab006. doi: 10.1093/eurjpc/zwab006
75. St John Sutton MG, Sharpe N. Left ventricular remodeling after myocardial infarction pathophysiology and therapy clinical cardiology: new frontiers. Circulation. (2000) 101:2981–8. doi: 10.1161/01.CIR.101.25.2981
76. Aimo A, Gaggin HK, Barison A, Emdin M, Januzzi JL Jr. Imaging, biomarker, and clinical predictors of cardiac remodeling in heart failure with reduced ejection fraction. JACC Heart Fail. (2019) 7:782–794. doi: 10.1016/j.jchf.2019.06.004
77. Taylor RS, Brown A, Ebrahim S, Jolliffe J, Noorani H, Rees K, et al. Exercise-based rehabilitation for patients with coronary heart disease: systematic review and meta-analysis of randomized controlled trials. Am J Med. (2004) 116:682–92. doi: 10.1016/j.amjmed.2004.01.009
78. Anderson L, Thompson DR, Oldridge N, Zwisler AD, Rees K, Martin N, et al. Exercise-based cardiac rehabilitation for coronary heart disease: cochrane systematic review and meta-analysis. J Am Coll Cardiol. (2016) 67:1–12. doi: 10.1016/j.jacc.2015.10.044
79. Prior BM, Yang HT, Terjung RL. What makes vessels grow with exercise training? J Appl Physiol. (2004) 97:1119–28. doi: 10.1152/japplphysiol.00035.2004
80. Adams V, Linke A, Krankel N, Erbs S, Gielen S, Möbius-Winkler S, et al. Impact of regular physical activity on the NAD(P)H oxidase and angiotensin receptor system in patients with coronary artery disease. Circulation. (2005) 111:555–62. doi: 10.1161/01.CIR.0000154560.88933.7E
81. Linke A, Erbs S, Hambrecht R. Exercise and the coronary circulation—alterations and adaptations in coronary artery disease. Prog Cardiovasc Dis. (2006) 48:270–84. doi: 10.1016/j.pcad.2005.10.001
82. Ahmad T, Fiuzat M, Mark DB, Neely B, Neely M, Kraus WE, et al. The effects of exercise on cardiovascular biomarkers in patients with chronic heart failure. Am Heart J. (2014) 167:193–202.e1. doi: 10.1016/j.ahj.2013.10.018
83. Kenchaiah S, Pfeffer MA. Cardiac remodeling in systemic hypertension. Med Clin North Am. (2004) 88:115–30. doi: 10.1016/S0025-7125(03)00168-8
84. Brook RD, Appel LJ, Rubenfire M, Ogedegbe G, Bisognano JD, Elliott WJ, et al. Beyond medications and diet: alternative approaches to lowering blood pressure: a scientific statement from the American heart association. Hypertension. (2013) 61:1360–80. doi: 10.1161/HYP.0b013e318293645f
85. López-Valenciano A, Ruiz-Pérez I, Ayala F, Sánchez-Meca J; Vera-Garcia FJ. Updated systematic review and meta-analysis on the role of isometric resistance training for resting blood pressure management in adults. J Hypertens. (2019) 37:1320–33. doi: 10.1097/HJH.0000000000002022
86. Seferović PM, Paulus WJ. Diabetic cardiomyopathy—fact or fiction? Clinical diabetic cardiomyopathy: a two-faced disease with restrictive and dilated phenotypes. Eur Heart J. (2015) 36:1718–27. doi: 10.1093/eurheartj/ehv134
87. Leung M, Wong VW, Hudson M, Yeung DY. Impact of improved glycemic control on cardiac function in type 2 diabetes mellitus. Circ Cardiovasc Imaging. (2016) 9:e003643. doi: 10.1161/CIRCIMAGING.115.003643
88. Van Ryckeghem L, Keytsman C, Verbaanderd E, Frederix I, Bakelants E, Petit T, et al. Asymptomatic type 2 diabetes mellitus display a reduced myocardial deformation but adequate response during exercise. Eur J Appl Physiol. (2021) 121:929–40. doi: 10.1007/s00421-020-04557-5
89. Plana JC, Galderisi M, Barac A, Ewer MS, Ky B, Scherrer-Crosbie M, et al. Expert consensus for multimodality imaging evaluation of adult patients during and after cancer therapy: a report from the American society of echocardiography and the European association of cardiovascular imaging. J Am Soc Echocardiogr. (2014) 27:911–39. doi: 10.1016/j.echo.2014.07.012
90. Holland DJ, Prasad SB, Marwick TH. Contribution of exercise echocardiography to the diagnosis of heart failure with preserved ejection fraction (HFpEF). Heart. (2010) 96:1024–8. doi: 10.1136/hrt.2009.183947
91. Donal E, Lund LH, Oger E, Reynaud A, Schnell F, Persson H, et al. Value of exercise echocardiography in heart failure with preserved ejection fraction: a substudy from the KaRen study. Eur Heart J Cardiovasc Imaging. (2016) 17:106–13. doi: 10.1093/ehjci/jev144
92. Borlaug BA, Olson TP, Lam CS, Flood KS, Lerman A, Johnson BD, et al. Global cardiovascular reserve dysfunction in heart failure with preserved ejection fraction. J Am Coll Cardiol. (2010) 56:845–54. doi: 10.1016/j.jacc.2010.03.077
93. Meluzín J, Sitar J, Krístek J, Prosecky R, Pesl M, Podrouzková H, et al. The role of exercise echocardiography in the diagnostics of heart failure with normal left ventricular ejection fraction. Eur J Echocardiogr. (2011) 12:591–602. doi: 10.1093/ejechocard/jer082
94. La Gerche A, Claessen G, Van de Bruaene A, Pattyn N, Van Cleemput J, Gewillig M, et al. Cardiac MRI: a new gold standard for ventricular volume quantification during high-intensity exercise. Circ Cardiovasc Imaging. (2013) 6:329–38. doi: 10.1161/CIRCIMAGING.112.980037
95. Jekic M, Foster EL, Ballinger MR, Raman SV, Simonetti OP. Cardiac function and myocardial perfusion immediately following maximal treadmill exercise inside the MRI room. J Cardiovasc Magn Reson. (2008) 10:3. doi: 10.1186/1532-429X-10-3
96. Le TT, Bryant JA, Ting AE, Ho PY, Su B, Teo RC, et al. Assessing exercise cardiac reserve using real-time cardiovascular magnetic resonance. J Cardiovasc Magn Reson. (2017) 19:7. doi: 10.1186/s12968-017-0322-1
97. Kirkham AA, Goonasekera MV, Mattiello BC, Grenier JG, Haykowsky MJ, Thompson RB. Reliability and reproducibility of cardiac MRI quantification of peak exercise function with long-axis views. PLoS ONE. (2021) 16:e0245912. doi: 10.1371/journal.pone.0245912
98. Zieman SJ, Melenovsky V, Kass DA. Mechanisms, pathophysiology, and therapy of arterial stiffness. Arterioscler Thromb Vasc Biol. (2005) 25:932–43. doi: 10.1161/01.ATV.0000160548.78317.29
99. Townsend RR, Wilkinson IB, Schiffrin EL, Avolio AP, Chirinos JA, Cockcroft JR, et al. American heart association council on hypertension. Recommendations for improving and standardizing vascular research on arterial stiffness: a scientific statement from the American heart association. Hypertension. (2015) 66:698–722. doi: 10.1161/HYP.0000000000000033
100. Roman MJ, Naqvi TZ, Gardin JM, Gerhard-Herman M, Jaff M, Mohler E, et al. American society of echocardiography report. Clinical application of noninvasive vascular ultrasound in cardiovascular risk stratification: a report from the American society of echocardiography and the society for vascular medicine and biology. Vasc Med. (2006) 11:201–11. doi: 10.1177/1358863x06070511
101. Thompson RB, Tomczak CR, Haykowsky MJ. Evaluation of cardiac, vascular, and skeletal muscle function with MRI: novel physiological end points in cardiac rehabilitation research. Can J Cardiol. (2016) 32:S388–96. doi: 10.1016/j.cjca.2016.07.006
102. Vlachopoulos C, Aznaouridis K, Stefanadis C. Prediction of cardiovascular events and all-cause mortality with arterial stiffness: a systematic review and meta-analysis. J Am Coll Cardiol. (2010) 55:1318–27. doi: 10.1016/j.jacc.2009.10.061
103. Lorenz MW, Polak JF, Kavousi M, Mathiesen EB, Völzke H, Tuomainen TP, et al. Carotid intima-media thickness progression to predict cardiovascular events in the general population (the PROG-IMT collaborative project): a meta-analysis of individual participant data. Lancet. (2012) 379:2053–62. doi: 10.1016/S0140-6736(12)60441-3
104. Willeit P, Tschiderer L, Allara E, Reuber K, Seekircher L, Gao L, et al. Carotid intima-media thickness progression as surrogate marker for cardiovascular risk: meta-analysis of 119 clinical trials involving 100 667 patients. Circulation. (2020) 142:621–42. doi: 10.1161/CIRCULATIONAHA.120.046361
105. Kaess BM, Rong J, Larson MG, Hamburg NM, Vita JA, Levy D, et al. Aortic stiffness, blood pressure progression, and incident hypertension. JAMA. (2012) 308:875–81. doi: 10.1001/2012.jama.10503
106. Chae CU, Pfeffer MA, Glynn RJ, Mitchell GF, Taylor JO, Hennekens CH. Increased pulse pressure and risk of heart failure in the elderly. JAMA. (1999) 281:634–9. doi: 10.1001/jama.281.7.634
107. Tanaka H, Dinenno FA, Monahan KD, Clevenger CM, DeSouza CA, Seals DR. Aging, habitual exercise, and dynamic arterial compliance. Circulation. (2000) 102:1270–5. doi: 10.1161/01.CIR.102.11.1270
108. Madden KM, Lockhart C, Cuff D, Potter TF, Meneilly GS. Short-term aerobic exercise reduces arterial stiffness in older adults with type 2 diabetes, hypertension, and hypercholesterolemia. Diabetes Care. (2009) 32:1531–5. doi: 10.2337/dc09-0149
109. Oliveira NL, Ribeiro F, Alves AJ, Campos L, Oliveira J. The effects of exercise training on arterial stiffness in coronary artery disease patients: a state-of-the-art review. Clin Physiol Funct Imaging. (2014) 34:254–62. doi: 10.1111/cpf.12093
110. Laskey W, Siddiqi S, Wells C, Lueker R. Improvement in arterial stiffness following cardiac rehabilitation. Int J Cardiol. (2013) 167:2734–8. doi: 10.1016/j.ijcard.2012.06.104
111. Byrkjeland R, Stensæth KH, Anderssen S, Njerve IU, Arnesen H, Seljeflot I, et al. Effects of exercise training on carotid intima-media thickness in patients with type 2 diabetes and coronary artery disease. Influence of carotid plaques. Cardiovasc Diabetol. (2016) 15:13. doi: 10.1186/s12933-016-0336-2
112. Madden KM, Lockhart C, Cuff D, Potter TF, Meneilly GS. Aerobic training-induced improvements in arterial stiffness are not sustained in older adults with multiple cardiovascular risk factors. Hum Hypertens. (2013) 27:335–9. doi: 10.1038/jhh.2012.38
113. Montero D, Roche E, Martinez-Rodriguez A. The impact of aerobic exercise training on arterial stiffness in pre- and hypertensive subjects: a systematic review and meta-analysis. Int J Cardiol. (2014) 13:361–8. doi: 10.1016/j.ijcard.2014.03.072
114. Way KL, Keating SE, Baker MK, Chuter VH, Johnson NA. The effect of exercise on vascular function and stiffness in type 2 diabetes: a systematic review and meta-analysis. Curr Diabetes Rev. (2016) 12:369–83. doi: 10.2174/1573399811666150817124601
115. Jones LM, Stoner L, Baldi JC, McLaren B. Circuit resistance training and cardiovascular health in breast cancer survivors. Eur J Cancer Care. (2020) 29:e13231. doi: 10.1111/ecc.13231
116. Beaudry RI, Liang Y, Boyton ST, Tucker WJ, Brothers RM, Daniel KM, et al. Meta-analysis of exercise training on vascular endothelial function in cancer survivors. Integr Cancer Ther. (2018) 17:192–9. doi: 10.1177/1534735418756193
117. Marti CN, Gheorghiade M, Kalogeropoulos AP, Georgiopoulou VV, Quyyumi AA, Butler J. Endothelial dysfunction, arterial stiffness, and heart failure. J Am Coll Cardiol. (2012) 60:1455–69. doi: 10.1016/j.jacc.2011.11.082
118. Kitzman DW, Herrington DM, Brubaker PH, Moore JB, Eggebeen J, Haykowsky MJ. Carotid arterial stiffness and its relationship to exercise intolerance in older patients with heart failure and preserved ejection fraction. Hypertension. (2013) 61:112–9. doi: 10.1161/HYPERTENSIONAHA.111.00163
119. Fujiwara K, Shimada K, Nishitani-Yokoyama M, Kunimoto M, Matsubara T, Matsumori R, et al. Arterial stiffness index and exercise tolerance in patients undergoing cardiac rehabilitation. Int Heart J. (2021) 62:230–7. doi: 10.1536/ihj.20-418
120. Bulluck H, Hammond-Haley M, Weinmann S, Martinez-Macias R, Hausenloy DJ. Myocardial infarct size by CMR in clinical cardioprotection studies: insights from randomized controlled trials. JACC Cardiovasc Imaging. (2017) 10:230–40. doi: 10.1016/j.jcmg.2017.01.008
121. Ingkanisorn WP, Rhoads KL, Aletras AH, Kellman P, Arai AE. Gadolinium delayed enhancement cardiovascular magnetic resonance correlates with clinical measures of myocardial infarction. J Am Coll Cardiol. (2004) 43:2253–9. doi: 10.1016/j.jacc.2004.02.046
122. Wong TC, Piehler K, Meier CG, Testa SM, Klock AM, Aneizi AA, et al. Association between extracellular matrix expansion quantified by cardiovascular magnetic resonance and short-term mortality. Circulation. (2012) 126:1206–16. doi: 10.1161/CIRCULATIONAHA.111.089409
123. Treibel TA, Fridman Y, Bering P, Sayeed A, Maanja M, Frojdh F, et al. Extracellular volume associates with outcomes more strongly than native or post-contrast myocardial T1. JACC Cardiovasc Imaging. (2020) 13:44–54. doi: 10.1016/j.jcmg.2019.03.017
124. Reinstadler SJ, Stiermaier T, Liebetrau J, Fuernau G, Eitel C, de Waha S, et al. Prognostic significance of remote myocardium alterations assessed by quantitative noncontrast T1 mapping in ST-segment elevation myocardial infarction. JACC Cardiovasc Imaging. (2018) 11:411–9. doi: 10.1016/j.jcmg.2017.03.015
125. Vita T, Gräni C, Abbasi SA, Neilan TG, Rowin E, Kaneko K, et al. Comparing CMR mapping methods and myocardial patterns toward heart failure outcomes in nonischemic dilated cardiomyopathy. JACC Cardiovasc Imaging. (2019) 12:1659–69. doi: 10.1016/j.jcmg.2018.08.021
126. Tham EB, Haykowsky MJ, Chow K, Spavor M, Kaneko S, Khoo NS, et al. Diffuse myocardial fibrosis by T 1-mapping in children with subclinical anthracycline cardiotoxicity: relationship to exercise capacity, cumulative dose and remodeling. J Cardiovasc Magn Reson. (2013) 15:48. doi: 10.1186/1532-429X-15-48
127. Jordan JH, Vasu S, Morgan TM, D'Agostino RB Jr, Meléndez GC, Hamilton CA, et al. Anthracycline-associated T1 mapping characteristics are elevated independent of the presence of cardiovascular comorbidities in cancer survivors. Circ Cardiovasc Imaging. (2016) 9:e004325. doi: 10.1161/CIRCIMAGING.115.004325
128. Kirkham AA, Goonasekera MV, Mattiello BC, Grenier JG, Haykowsky MJ, Thompson RB. Aerobic fitness is related to myocardial fibrosis post-anthracycline therapy. Med Sci Sports Exerc. (2021) 53:267–74. doi: 10.1249/MSS.0000000000002469
129. Costello BT, Roberts TJ, Howden EJ, Bigaran A, Foulkes SJ, Beaudry RI, et al. Exercise attenuates cardiotoxicity of anthracycline chemotherapy measured by global longitudinal strain. JACC CardioOncol. (2019) 1:298–301. doi: 10.1016/j.jaccao.2019.09.002
130. Despres JP. Body fat distribution and risk of cardiovascular disease: an update. Circulation. (2012) 126:1301–13. doi: 10.1161/CIRCULATIONAHA.111.067264
131. Hausman GJ, Basu U, Du M, Fernyhough-Culver M, Dodson MV. Intermuscular and intramuscular adipose tissues: bad vs. good adipose tissues. Adipocyte. (2014) 3:242–55. doi: 10.4161/adip.28546
132. Neeland IJ, Ross R, Després JP, Matsuzawa Y, Yamashita S, Shai I, et al. Visceral and ectopic fat, atherosclerosis, and cardiometabolic disease: a position statement. Lancet Diabetes Endocrinol. (2019) 7:715–25. doi: 10.1016/S2213-8587(19)30084-1
133. Haykowsky MJ, Nicklas BJ, Brubaker PH, Hundley WG, Brinkley TE, Upadhya B, et al. Regional adipose distribution and its relationship to exercise intolerance in older obese patients who have heart failure with preserved ejection fraction. JACC Heart Fail. (2018) 6:640–9. doi: 10.1016/j.jchf.2018.06.002
134. Ying W, Sharma K, Yanek LR, Vaidya D, Schär M, Markl M, et al. Visceral adiposity, muscle composition, and exercise tolerance in heart failure with preserved ejection fraction. ESC Heart Failure. (2021) 8:2535–45. doi: 10.1002/ehf2.13382
135. Cespedes Feliciano EM, Chen WY, Bradshaw PT, Prado CM, Alexeeff S, Albers KB, et al. Adipose tissue distribution and cardiovascular disease risk among breast cancer survivors. J Clin Oncol. (2019) 37:2528–36. doi: 10.1200/JCO.19.00286
136. Verheggen RJ, Maessen MF, Green DJ, Hermus AR, Hopman MT, Thijssen DH. A systematic review and meta-analysis on the effects of exercise training versus hypocaloric diet: distinct effects on body weight and visceral adipose tissue. Obes Rev. (2016) 17:664–90. doi: 10.1111/obr.12406
137. Mirman AM, Nardoni NR, Chen AY, Horwich TB. Body composition changes during traditional versus intensive cardiac rehabilitation in coronary artery disease. J Cardiopulm Rehabil Prev. (2020) 40:388–93. doi: 10.1097/HCR.0000000000000497
138. Takagawa Y, Yagi S, Ise T, Ishii A, Nishikawa K, Fukuda D, et al. Improved exercise capacity after cardiac rehabilitation is associated with reduced visceral fat in patients with chronic heart failure. Int Heart J. (2017) 58:746–54. doi: 10.1536/ihj.16-454
139. Fang H, Liu C, Cavdar O. The relation between submaximal aerobic exercise improving vascular elasticity through loss of visceral fat and antihypertensive. Clin Exp Hypertens. (2021) 43:203–10. doi: 10.1080/10641963.2020.1847127
140. Sabag A, Way KL, Keating SE, Sultana RN, O'Connor HT, Baker MK, et al. Exercise and ectopic fat in type 2 diabetes: a systematic review and meta-analysis. Diabetes Metab. (2017) 43:195–210. doi: 10.1016/j.diabet.2016.12.006
141. Pituskin E, Haykowsky M, McNeely M, Mackey J, Chua N, Paterson I. Rationale and design of the multidisciplinary team IntervenTion in cArdio-oNcology study (TITAN). BMC Cancer. (2016) 16:733. doi: 10.1186/s12885-016-2761-8
142. Dieli-Conwright CM, Courneya KS, Demark-Wahnefried W, Sami N, Lee K, Buchanan TA, et al. Effects of aerobic and resistance exercise on metabolic syndrome, sarcopenic obesity, and circulating biomarkers in overweight or obese survivors of breast cancer: a randomized controlled trial. J Clin Oncol. (2018) 36:875–83. doi: 10.1200/JCO.2017.75.7526
143. Thompson RB, Pagano JJ, Chow K, Sekowski V, Paterson I, Ezekowitz J, et al. Sub-clinical pulmonary edema is associated with reduced exercise capacity in heart failure with reduced or preserved ejection fraction. J Am Coll Cardiol. (2017) 70:1827–8. doi: 10.1016/j.jacc.2017.07.787
144. Foulkes SJ, Howden EJ, Antill Y, Loi S, Salim A, Haykowsky MJ, et al. Exercise as a diagnostic and therapeutic tool for preventing cardiovascular morbidity in breast cancer patients–the BReast cancer EXercise InTervention (BREXIT) trial protocol. BMC Cancer. (2020) 20:655. doi: 10.1186/s12885-020-07123-6
145. Kirkham AA, Paterson DI, Prado CM, Mackey JR, Courneya KS, Pituskin E, et al. Rationale and design of the caloric restriction and exercise protection from anthracycline toxic effects (CREATE) study: a 3-arm parallel group phase II randomized controlled trial in early breast cancer. BMC Cancer. (2018) 18:864. doi: 10.1186/s12885-018-4778-7
146. Butler T, Kerley CP, Altieri N, Alvarez J, Green J, Hinchliffe J, et al. Optimum nutritional strategies for cardiovascular disease prevention and rehabilitation (BACPR). Heart. (2020) 106:724–31. doi: 10.1136/heartjnl-2019-315499
147. Ignarro LJ, Balestrieri ML, Napoli C. Nutrition, physical activity, and cardiovascular disease: an update. Cardiovasc Res. (2007) 73:326–40. doi: 10.1016/j.cardiores.2006.06.030
148. Brandhorst S, Longo VD. Dietary restrictions and nutrition in the prevention and treatment of cardiovascular disease. Circ Res. (2019) 124:952–65. doi: 10.1161/CIRCRESAHA.118.313352
149. Prado CM, Anker SD, Coats AJS, Laviano A, von Haehling S. Nutrition in the spotlight in cachexia, sarcopenia and muscle: avoiding the wildfire. J Cachexia Sarcopenia Muscle. (2021) 12:3–8. doi: 10.1002/jcsm.12673
150. Freimann S, Scheinowitz M, Yekutieli D, Feinberg MS, Eldar M, Kessler-Icekson G. Prior exercise training improves the outcome of acute myocardial infarction in the rat. Heart structure, function, and gene expression. J Am Coll Cardiol. (2005) 45:931–8. doi: 10.1016/j.jacc.2004.11.052
151. Aït Mou Y, Reboul C, Andre L, Lacampagne A, Cazorla O. Late exercise training improves non-uniformity of transmural myocardial function in rats with ischaemic heart failure. Cardiovasc Res. (2009) 81:555–64. doi: 10.1093/cvr/cvn229
152. Lee J, Cho HS, Park S, Kim WK. Regular exercise produced cardioprotective effects on rat's heart with hypertension induced by L-NAME administration. Clin Exp Hypertens. (2009) 31:364–75. doi: 10.1080/10641960902977924
153. Ghignatti PVC, Nogueira LJ, Lehnen AM, Leguisamo NM. Cardioprotective effects of exercise training on doxorubicin-induced cardiomyopathy: a systematic review with meta-analysis of preclinical studies. Sci Rep. (2021) 11:6330. doi: 10.1038/s41598-021-83877-8
154. Melo SFS, da Silva Júnior ND, Barauna VG, Oliveira EM. Cardiovascular adaptations induced by resistance training in animal models. Int J Med Sci. (2018) 15:403–10. doi: 10.7150/ijms.23150
Keywords: cardiovascular disease, exercise training, imaging, left ventricular function, vascular function, body composition
Citation: Alhumaid W, Small SD, Kirkham AA, Becher H, Pituskin E, Prado CM, Thompson RB, Haykowsky MJ and Paterson DI (2022) A Contemporary Review of the Effects of Exercise Training on Cardiac Structure and Function and Cardiovascular Risk Profile: Insights From Imaging. Front. Cardiovasc. Med. 9:753652. doi: 10.3389/fcvm.2022.753652
Received: 05 August 2021; Accepted: 17 January 2022;
Published: 21 February 2022.
Edited by:
Jian Yang, Fudan University, ChinaReviewed by:
Owais Bhat, Virginia Commonwealth University, United StatesSebastian Kelle, Deutsches Herzzentrum Berlin, Germany
Copyright © 2022 Alhumaid, Small, Kirkham, Becher, Pituskin, Prado, Thompson, Haykowsky and Paterson. This is an open-access article distributed under the terms of the Creative Commons Attribution License (CC BY). The use, distribution or reproduction in other forums is permitted, provided the original author(s) and the copyright owner(s) are credited and that the original publication in this journal is cited, in accordance with accepted academic practice. No use, distribution or reproduction is permitted which does not comply with these terms.
*Correspondence: D. Ian Paterson, ip3@ualberta.ca