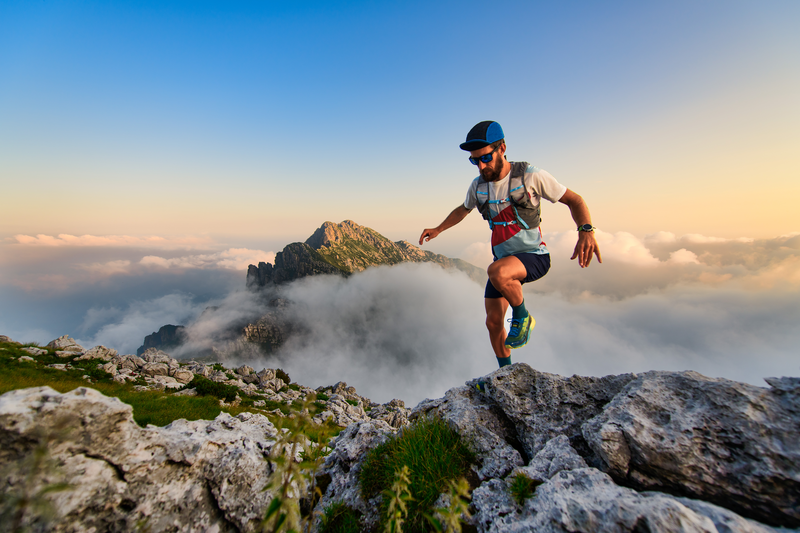
94% of researchers rate our articles as excellent or good
Learn more about the work of our research integrity team to safeguard the quality of each article we publish.
Find out more
REVIEW article
Front. Cardiovasc. Med. , 12 January 2023
Sec. General Cardiovascular Medicine
Volume 9 - 2022 | https://doi.org/10.3389/fcvm.2022.1049143
Leukocyte differentiation antigen 74 (CD74), also known as invariant chain, is a molecular chaperone of major histocompatibility complex class II (MHC II) molecules involved in antigen presentation. CD74 has recently been shown to be a receptor for the macrophage migration inhibitory factor family proteins (MIF/MIF2). Many studies have revealed that CD74 plays an important role in cardiovascular disease. In this review, we summarize the structure and main functions of CD74 and then focus on the recent research progress on the role of CD74 in cardiovascular diseases. In addition, we also discuss potential treatment strategies that target CD74. Our systematic review of the role of CD74 in cardiovascular disease will fill some knowledge gaps in the field.
Leukocyte differentiation antigen 74 (CD74), also known as invariant chain (Ii), is a transmembrane glycoprotein that is not polymorphic. CD74 was initially identified only as a molecular chaperone of major histocompatibility complex class II (MHC II) molecules involved in exogenous antigen presentation. Subsequent studies found that CD74 also participates in the endogenous presentation of antigen through MHC I molecules (1, 2). Additionally, CD74 is also a high affinity membrane receptor of macrophage migration inhibitory factor (MIF) and d-dopachrome tautomerase (D-DT/MIF2). CD74 is also a functional receptor for tissue inhibitor of metalloproteinases-1 (TIMP-1) (3) and regulates the transport of angiotensin II type I receptor (AT1) (4). It activates a number of pathophysiological pathways when a complex is formed by CD44 and MIF/MIF2. For instance, phosphatidylinositol 3-kinase/protein kinase B (PI3K/Akt), adenosine monophosphate-activated protein kinase (AMPK), nuclear factor-κB (NF-κB), and extracellular signal-regulated kinase (ERK) pathways, that are involved in B cells differentiation and maturation, cell proliferation, and energy metabolism, etc. CD74 through these signaling pathways plays regulatory roles in the development and progress of various diseases, such as ischemic heart disease (5), atherosclerosis (6), hepatic fibrosis (7), immunological disorders, and cancers (1).
The molecular structure of CD74 is comprised of the N-terminal cytoplasmic region, the transmembrane region, and the C-terminal extracellular region. Structural models of CD74 are shown in Figure 1 (8). In human, CD74 is encoded by chromosome 5 with a gene length of roughly 9 kb and consisted of nine exons (9). There are four isoforms of human CD74, which can be divided into p33, p35, p41, and p43 according to their molecular weight (Figure 2), of which p33 (33 kDa) is the most common isoform and has a 216 amino acid sequence (10). There are two isoforms of CD74 in the mouse, p31 and p41, corresponding to human p33 and p41, respectively (11). In human, the start codons of p33 and p41 can be switched, allowing for an N-terminal extension of 16 amino acids to form other two isoforms, p35 and p43. Phosphorylation of this N-terminal extension domain is thought to be important for CD74-MHC II complex exiting from the endoplasmic reticulum and transporting to the endosomal compartment (12, 13). Additionally, in the gene sequence encoding the two longer isoforms (p41 and p43), an additional exon 6b (located between exon 6 and exon 7) can encode a cysteine rich domain containing 64 amino acids (209–273 amino acid residues refer to human p43 variant). This domain is called thyroglobulin type I domain, and it has high homology with thyroglobulin (9). This domain binds to cathepsin L and prevents it from degradation, allowing cathepsin L to remain active in the extracellular environment and regulate extracellular matrix degradation during inflammation (14). In addition, the class II-associated invariant chain peptides (CLIP), which contain the 98–119 amino acid residues (human p43 variant) of CD74 molecule, is a site that binds to MHC II and plays an important role in antigen presentation (15, 16).
Figure 1. Pictures depicting the secondary, tertiary, and quaternary structures of leukocyte differentiation antigen 74 (CD74). The picture on the left shows the structural view from the top. The right picture shows a side view of the same homotrimer. Pictures adopted from Meza-Romero et al. with permission (No. 5392361035630) (8).
Figure 2. The structure of mouse and human leukocyte differentiation antigen 74 (CD74). Two isoforms (p31 and p41) in mice and four isoforms (p33, p35, p41, and p43) in humans. CLIP, the class II-associated invariant chain peptides; ICD, intracellular domains. Figure adopted from Schroder et al. with permission (No. 5390740929053) (19).
After synthesis in the endoplasmic reticulum, CD74 is first assembled into a homotrimer and then bound with three MHC II heterodimers (αβ) to form a nonamer (αβIi)3. CD74 occupies the binding groove of MHC II, which prevents the binding of endogenous peptide molecules to MHC II in the endoplasmic reticulum. The formation of CD74 homotrimer is related to transmembrane region and amino acid residues 134–208 of trimerization domain (human p43 variant) in C-terminal extracellular region, which is a protease resistant domain (10, 17–19). A recent study found that both the transmembrane region and the C-terminal trimerization domain can independently induce CD74 homotrimer formation, which is in the absence of either the transmembrane region or the C-terminal trimerization domain, but with less efficiency (18). Further, The study also found that no domain except the transmembrane region and the C-terminal trimerization domain could polymerize CD74 to form a trimer (18). Once the nonamers (αβIi)3 formed, it immediately leaves the endoplasmic reticulum and enters the Golgi apparatus. Subsequently, CD74 is hydrolyzed by proteases, leaving only the CLIP fragment to protect the binding groove of MHC II (19, 20). Inhibition of these proteases would prevent the antigen presentation and immune response in which MHC II is involved, while cell surface expression of MHC II is also inhibited (21, 22). Finally, the Class II accessory molecule DM (HLA-DM in human; H2-M in mouse) catalyzes the substitution of CLIP fragment by the antigenic peptide results in the formation of a mature MHC II-peptide complex that is released to the cell surface for recognition by CD4+T cells and initiates an immune response (Figure 3) (19, 23, 24). CD74 has also been shown to be involved in MHC I presenting antigens, which in turn activates CD8+T cells, causing MHC I-mediated cytotoxic T-cells responses (2). In addition, it was found that the number of CD4+ T cells in thymus and peripheral blood was greatly reduced in CD74–/– mice but had a more CD8+ T cells compared to wild-type (WT) control mice (6).
Figure 3. Primary functions of leukocyte differentiation antigen 74 (CD74). The CD74 is released to the cell surface following synthesis, folding, and trimerization (2–5%). Together with CD44, CXCR2 and CXCR4, CD74 serves as a receptor for migration inhibitory factor (MIF) and MIF2. As most of CD74 occupies the binding groove of major histocompatibility complex class II (MHC II), it prevents the binding of endogenous polypeptide molecules to MHC II in the endoplasmic reticulum. Afterwards, CD74 is partially cleaved, leaving the CLIP fragment that protects the binding groove of MHC II. Once the CLIP fragment is released, the exogenous peptide can be loaded into the binding groove of the MHC II and form the MHC II-peptide. Finally, CD4+ T cells recognize the MHC II-peptide on the cell membrane. CLIP, the class II-associated invariant chain peptides; TCR, T-cell receptor.
MIF is a multifunctional inflammatory cytokine, composing of 115 amino acid residues. It is a highly conserved protein with a molecular weight of about 12.5 kDa. MIF has many functions, such as pro-inflammation, chemotaxis and regulating cell proliferation, angiogenesis, and fibrosis (25). MIF also possesses oxidoreductase and tautomerase activities, but the role of MIF enzyme activity is still not very clear, and some studies have found that it may be related to immune function (26). MIF2 shares similar amino acid sequence, structure and biological activity with MIF and MIF2 also has tautomerase activity similar to MIF. MIF2 consists of 117 amino acid residues with a molecular weight of 13 kDa (27). The genes of MIF and MIF2 are within 80 kb from each other in mouse and human genomes. The amino acid sequence homology of human MIF and MIF2 is 34%, and that of mouse MIF and MIF2 is 27% (27). Like MIF, MIF2 also forms a homotrimer structure, and its overall topology and trimeric structure are similar to MIF (28).
Leng et al. first found that CD74 is a high affinity membrane receptor for MIF. The extracellular domain between 109 and 149 amino acid residues (human p43 variant) of CD74 binds to MIF (Figure 2), and one MIF homotrimer binds three CD74 homotrimers to form a dodecamer (29). Merk et al. found that MIF2 is also a high affinity ligand for CD74 through competitive binding experiments, and MIF2 diminishes the binding of MIF to the extracellular domain of CD74 in a dose-dependent manner. The binding rate of MIF2 to CD74 is approximately three times higher than MIF, but the dissociation rate is 11 times faster than MIF (27). Different from MIF and CD74 binding to form a dodecamer, one CD74 homotrimer binds to only one MIF2 homotrimer to form a hexamer (30).
CD44 is a transmembrane glycoprotein with well-known kinase-activating properties, it participates in MIF and MIF2 signaling together with CD74. CD44 consists of three components: the N-terminal extracellular region (amino acid residues 1–270), the transmembrane region (amino acid residues 271–291), and the C-terminal intracellular region (amino acid residues 292–361) (31). A recent study documented the formation of CD74/CD44 complexes by immunoprecipitation, and further observed that neither CD74+CD44– nor CD74–CD44+ cells could activate the MIF signaling pathway. These findings suggest that CD74 is required to work together with CD44 to mediate the MIF/MIF2 signaling pathway (30). Due to alternative splicing, CD44 has subtypes with different molecular weight and size, but only the full length of CD44 can assist CD74 in MIF signaling (32). Similarly, CD74/CD44 is also involved in MIF2 signaling (27). Besides, CD74 can form complexes with CXCR2, CXCR4 or CXCR7 to participate in MIF signaling (Figure 3) (30).
MIF binds to the CD74/CD44 complex to activate Src-family protein kinases, and then activate extracellular signal-regulated kinase-1/2 (ERK1/2), mitogen-activated protein kinase (MAPK), PI3K-Akt cascade signaling, and inhibit p53. These signaling pathways are involved in regulation of inflammation, cell proliferation, apoptosis and survival, prostaglandin E2 production and tumorigenesis (29, 30, 33–35). MIF-CD74/CD44 can also activate the NF-κB pathway. It has been found that CD74 lacking the extracellular region can still activate the NF-κB pathway and induce differentiation and maturation of B lymphocytes (36, 37), suggesting that NF-κB activation may be mediated by the CD74 intracellular domains (CD74-ICD) (38). CD74-ICD is formed through the regulated intramembrane proteolysis (RIP), which then enters the nucleus to activate NF-κB (39). In addition, soluble CD74 (sCD74) is a membrane truncated protein that has been shown to be associated with a variety of diseases, such as autoimmune liver disease, acute respiratory distress syndrome, burn injury, myocardial ischemia-reperfusion (I/R) injury, and myocardial fibrosis (40–44). sCD74 has been shown to bind MIF and regulate its activity (29, 45). There is a 6 kDa difference in molecular weight between full-length CD74 and truncated sCD74. Interestingly, the molecular weight of CD74-ICD is also known to be 6 kDa. Therefore, it is speculated that the two truncated short segments of CD74, sCD74, and CD74-ICD, may be generated simultaneously by the RIP process (40).
It appears that those cells expressing MHC II also express CD74, including B-cells, monocytes, Langerhans cells, dendritic cells, and thymic epithelial cells (46). Whereas, some cells such as macrophages, epithelial and endothelial cells, podocytes, vascular smooth muscle cell, cardiac fibroblasts, and cardiomyocytes, even without MHC II, also express CD74, which imply the existence of a mechanism for translocation of CD74 to the cell surface during development independent of MHC II (1, 47–52). However, some cell types do not express CD74, such as neutrophils or the cell lines HEK293 and HeLa (53). CD74 predominantly exists in cells, and only 2–5% of CD74 is expressed on the cell surface independent of MHC II (46). Due to rapid endocytosis, the residence time of CD74 on the cell surface is very short, and the half-life is less than 10 min, with 3 × 103 CD74 molecules were endocytosed every minute. However, owing to its rapidly renewal, about 4 × 106 CD74 molecule can be expressed cumulatively on the cell surface daily (54).
In the context of CD74 expressed in a verity of cardiac cell types and immune cells, manifestation, pathophysiological impact and potential clinical significance of CD74 and MIF family proteins and potential mechanisms or signaling pathways involved will be discussed under different pathological settings.
The pathologic basis of coronary atherosclerotic heart disease is the chronically long-term growth of atherosclerotic plaques in the coronary vessel wall, which leads to the lumen stenosis, blood flow limitation and plaque rupture with acute thrombotic occlusion. Chronic inflammation of the vessel wall, characterized by the infiltration of circulating immune cells, is a key factor in the development of atherosclerotic lesions (55). Cytokines are released at the site of tissue injury or infection and are regulators of adaptive immune response and inflammatory response. MIF is a pro-inflammatory regulator of many acute and chronic inflammatory diseases and can modulate the development of atherosclerotic lesions (56). The role of MIF in atherosclerosis has been intensively investigated. MIF is expressed in atherosclerotic plaques, which is highly expressed in unstable plates (56–58). Blocking MIF can help vulnerable plaques transition to a more stable and less prone to rupture state (55). MIF has a chemokine-like function that activates CXCR2 and CXCR4, and thus can recruit monocytes and T lymphocytes to promote atherosclerosis (57, 59, 60).
Bernhagen et al. observed that atherogenic or inflammatory monocyte recruitment induced by MIF is not only CXCR2 dependent but also involves the its receptor, CD74. The formation of CXCR2/CD74 complexes was discovered by co-immunoprecipitation, indicating that MIF transmits signals through functional CXCR2/CD74 complexes (Figure 4) (60). CD74 lacks a domain that transduces intracellular signals. However, CD74 can signal by recruiting CD44 and Src family protein kinases, thereby generating chemotaxis and recruiting monocytes. MIF-induced leukocyte chemotaxis via CXCR2/CD74 was further confirmed by blocking both CXCR2 and CD74 that significantly impaired the ability of MIF to induce monocyte infiltration (60).
Figure 4. The role of leukocyte differentiation antigen 74 (CD74) in atherosclerosis. Migration inhibitory factor (MIF) promotes monocyte chemotaxis via CXCR2/CD74/CD44 complex. The secretion of monocyte chemoattractant protein-1 (MCP-1) is promoted by inhibits atherosclerosis by reducing interferon-gamma (IFNγ) inducing CD74/NF-κB. The MIF/CXCR4/CD74/CD44 signaling pathway promotes B-cell and T-cell chemotaxis, which releases antibodies that neutralize ox-LDL and promote the clearance of dead and apoptotic cells in atherosclerotic lesions. Additionally, MIF promotes CXCL8 expression through CXCR4/CD74/CD44, which plays an important role in atherosclerosis. As of yet, it is unclear whether MIF2 contributes to atherosclerotic lesions. SMC, smooth muscle cell; ox-LDL, oxidized low density lipoprotein.
In T-cells, MIF can also quickly trigger the activation of the classical JNK/c-Jun/AP-1 pathway through the CXCR4/CD74/Src/PI3K axis, which induces the activation of cytokine CXCL8 or IL-8 mediated signaling pathway and promoting inflammatory processes. CXCL8 is an inflammatory factor that has been linked to the progression of atherosclerosis (61). Whether MIF2 is involved in the regulation of the atherosclerotic process remains unclear as MIF2 does not have an pseudo-(E)LR motif like MIF and cannot activate CXCR2 (45, 58, 62). Deficiency of T-cells that mediate adaptive immune responses can reduce atherosclerotic plaques by 80%, and CD4+T cells are the major subtype of T-cells engaged in atherosclerosis. Their activation requires antigen presentation by antigen presenting cell (APC), and MHC II mediate this process (63). CD74, as a molecular chaperone of MHC II molecules, is involved in the folding of MHC II, intracellular transport and loading of antigen peptides (10). Sun et al. observed that atherosclerosis was aggravated in LDLR–/– mice after high-fat diet feeding while the degree of atherosclerosis was lower in hybrid mice with both LDLR and CD74 deficiency, which was associated with an impaired adaptive immune response to disease-specific antigens (6). In term of mechanism, CD74 deficiency impairs APC antigen presentation and T-cell activation, which reduces atherosclerosis (6).
In addition, MIF also promotes B lymphocyte survival by activating the CD74-CD44 receptor complex (64). Primary B-cells from WT mice incubated with anti-CD74 antibody or AMD3100 to block CD74 or CXCR4, respectively, both inhibited the chemotactic response of B-cells to MIF, suggesting that MIF exerts a chemotactic effect on B-cells via both CD74 and CXCR4 (65). Circulating B-cell numbers are reduced in MIF-deficient atherosclerotic mice accompanied by defective B-cell development (66). Notably, MIF/CD74 signaling pathway induces B-cell maturation and survival independently of its chaperone activity (38, 67, 68). Oxidized low density lipoprotein (ox-LDL) can be phagocytized rapidly by macrophages and smooth muscle cells and exhibits the property of monocyte chemotaxis. It is a major factor in the formation of foam cells. B-cells play a protective role against atherosclerosis by producing natural IgM antibodies, which are used to neutralize ox-LDL and to promote the clearance of apoptotic and dead cells in atherosclerotic lesions (6, 69).
There is evidence for a role of CD74 in atherosclerosis. CD74 expression was elevated in carotid plaques and peripheral blood mononuclear cells (PBMCs) of patients with carotid stenosis, and correlated with carotid intima-media thickness in subjects without clinical cardiovascular disease (49). A very recent study also found that in human carotid plaques, CD74 expression is lesion-dependently increased and is associated with necrotic core formation and plaque rupture, clinical symptoms, macrophage apoptosis, ferritin, and thrombin receptors (70). Moreover, CD74 levels were inversely correlated to high-density lipoproteins and statin treatment, and positively correlated to triglycerides (70). This study suggests that CD74 in apoptotic macrophages is linked to inflammation and thrombosis in progression of human atherosclerotic plaques, lipid metabolism, and clinical manifestation in atherosclerosis. Surface CD74 in apoptotic macrophages and ferritin production induced by oxidized lipids may contribute to inflammation and plaque vulnerability in atherosclerosis. Thus, CD74 may be a potential therapeutic target for atherosclerosis by reducing inflammatory response, which require further preclinical study to validate.
Many experimental studies have revealed protective effect of MIF family proteins and its receptor in the animal model of ischemic heart disease. Qi et al. observed a larger infarct size in CD74–/– compared with MIF–/– mice after 15 min ischemia followed by 30 min reperfusion, which was approximately about twice that of MIF–/– mice (71). This observation may imply a converged/central role of CD74 in mediating cardio-protection. In other word, there may be more factors rather than only MIF through CD74 involving in the downstream signaling transduction. Notably, the expression levels of MIF, CD74 and CD44 in the infarct zone were significantly upregulated in both the acute (1 day) and subacute (5 days) phases of the MI mouse model, further supporting a key role of MIF via CD74 in this disease condition (5).
Both MIF and MIF2 are highly expressed in murine cardiomyocytes and secreted by the heart under ischemic stress (52, 71, 72). MIF released from cardiomyocytes activated the CD74/CD44/AMPK signaling pathway, which leads to an increased glucose uptake by cardiomyocytes and thus protects the heart (52, 73–75). Likewise, MIF2 was also able to protect ischemic heart from dysfunction and necrosis by activation of the metabolic stress enzyme AMPK, which was mediated by a CD74/CaMKK II-dependent mechanism (71). In addition, MIF also exerts cardio-protection by activating ERK1/2 and inhibiting c-Jun N-terminal kinase (JNK) induced apoptosis in cardiomyocytes (76), anti-oxidative stress (44, 77, 78) or elevating oxidoreductase activity (Figure 5). It is worth to note that all these protective effects were achieved under a short ischemic duration.
Figure 5. Signaling cascades in cardiovascular diseases involving migration inhibitory factor/leukocyte differentiation antigen 74 (MIF/CD74) and MIF2/CD74. MIF exerts myocardial protection by inhibiting c-Jun N-terminal kinase (JNK) and activating extracellular signal-regulated kinase (ERK) 1/2-mediated cardiomyocyte apoptosis. MIF/CD74 and MIF2/CD74 reinforce glucose uptake and utilization by activating adenosine monophosphate-activated protein kinase (AMPK) to promote GLUT4 expression, and inhibit fatty acid oxidation by inhibiting ACC. MIF/CD74 promotes sarcoplasmic reticulum (SR) Ca2+ ATPase and Na+/Ca2+ exchanger (NCX) expression and activation via CaMKK II/RyR2 to regulate atrial arrhythmia. MIF/CD74 inhibits myocardial fibrosis through inhibition of Smad 2/Smad 3 and promotes fibroblast necroptosis through regulated intramembrane proteolysis 1 (RIP 1)/RIP 3/MLKL. Oxidoreductase activity of MIF also attenuates myocardial hypertrophy and I/R injury oxidative stress, while sCD74 appears to enhance this activity.
However, open-heart surgery with cardiopulmonary bypass (CPB) causes ischemia-reperfusion of the heart, which triggers inflammatory reactions and leads to organ dysfunction in patients. There is an early study reported that MIF levels after CPB correlated with multiple organ dysfunction including hematological, circulatory and pulmonary global organ dysfunction (79). Thereafter, several clinical studies further investigated dynamic changes and clinical significance of MIF family proteins during cardiac surgery with CPB and reported opposite results. They found that serum levels of MIF and MIF2 significantly increased intraoperatively, while sCD74 decreased correspondingly (44). Interestingly, the time-change curves of circulating MIF and MIF2 levels are similar, but the serum levels of MIF are approximately five times higher than MIF2 (44). The peak value of MIF release is negatively related to post-operative atrial fibrillation and acute kidney injury, whereas the level of MIF2 is opposite of MIF and intraoperative MIF2 levels are related to the increased incidence of post-operative atrial fibrillation and pneumonia (44, 77). Moreover, decreased sCD74 levels during myocardial reperfusion were negatively correlated with MIF levels and circulating sCD74/MIF complexes can be detected in 50% of patients subjected to cardiac surgery with CPB, which acts as a protective agent against ischemic injury by enhancing the oxidoreductase activity of MIF (44).
The sCD74 was recently identified in autoimmune liver disease and played a role in regulating MIF signaling activity (40). There are other studies reported that sCD74/MIF complex was able to enhance the oxidoreductase activity of MIF, thereby protecting the heart and reducing the risk of acute kidney injury induced by oxidative stress after cardiac surgery (44, 77, 78). These findings emphasize the anti-oxidative action of MIF owing to its oxidoreductase activity. However, in comparison to MIF, MIF2 is deficient in oxidoreductase activity (44). Besides AMPK activation, MIF improves ischemic myocardial protection also via CXCR2/CD74 in cardiac resident cells, while it adversely affects CXCR2-expressing inflammatory cells (53). Recombinant MIF induced rapid LV systolic dysfunction, an adverse effect not associated with CD74 (80). Surprisingly, recombinant MIF2 did not adversely affect cardiac systolic function or heart rate (71). These findings suggest that MIF2 lacks the pseudo-(E)LR motif and fails to induce inflammatory cell infiltration and it is therefore more selectively protective in ischemic myocardium.
In summary, albeit MIF and MIF2 shares partial sequence and structural homology, interaction between MIF, MIF2, and CD74 may be independent. Especially, a verity of cardiac cells and immune cells express MIF family proteins and their receptor, under certain diseased settings, MIF, MIF2, CD74, and sCD74 may exert diverse pathophysiological effect owing to serval reasons. First, CD74 participates in T-cell antigen presentation independently of MIF and MIF2, and T-cells play an important role in atherosclerosis. It has been reported that the number of CD4+ T-cells in thymus and peripheral circulation is greatly reduced in CD74-deficient mice, further confirming the role CD74 plays in T-cell development. Second, CD74 is also a functional receptor for TIMP-1 and regulates the transport of AT1. Third, although CD74 is involved in some of the roles of MIF together with CXCR2/4, MIF can act through CXCR2/4 alone. Fourth, MIF is also able to bind with CXCR7 to activate PI3K-Akt pathway independent of CD74 (81). In addition, MIF also possesses oxidoreductase and tautomerase activities, of which their functions still need to be further explored. Finally, a truncated fragment of CD74, sCD74, is released extracellularly to neutralize MIF and regulate MIF oxidoreductase activity.
Hypertension increases cardiac afterload and the heart enhances contractility to overcome peripheral resistance and maintains effective cardiac output, which results in left ventricular hypertrophy. Under pressure-overload conditions, MIF–/– mice showed more severe myocardial hypertrophy, systolic dysfunction, and myocardial fibrosis than WT mice (82, 83). This may be attributed to the fact that MIF can activate autophagy through AMPK-mTOR signaling (Figure 5), thereby alleviating the degree of cardiac hypertrophy in mice under stress loading conditions (82). Moreover, the inherent oxidoreductase activity of MIF also reduces the degree of myocardial hypertrophy under pressure-overload by limiting the oxidative stress of cardiomyocytes (84). MIF2 myocardial-specific knockout mice undergone transverse aortic constriction (TAC) developed cardiac systolic dysfunction faster than WT mice, along with more severe cardiac dilatation and pulmonary edema (84). It is worth noting that CD74 expression was significantly increased in both WT and MIF–/– mice under pressure-overload conditions, suggesting that activation of CD74 may engage in the cardio-protection of MIF and MIF2 (83), but there is still a lack of relevant direct evidence.
Diabetic cardiomyopathy is featured by ventricular dilatation, cardiac remodeling (hypertrophy, interstitial fibrosis), loss of ventricular compliance, diastolic dysfunction, and finally systolic dysfunction. Chen et al. reported elevated plasma MIF levels in patients with diabetes mellitus (type 2 diabetes mellitus, T2D) (85). They then established a T2D model in WT and CD74–/– mice by injection of streptozotocin (STZ) together with high-fat diet feeding and found that T2D mice developed obvious global metabolic disorder, cardiac remodeling, contractile dysfunction, myocyte apoptosis, pyroptosis and ferroptosis, and mitochondrial dysfunction. Knockdown of CD74 attenuated the above-mentioned adverse reactions induced by T2D without affecting the overall metabolic disorder (85). Moreover, acute ethanol injury and lipopolysaccharide (LPS) injury can lead to contractile dysfunction, cellular ultrastructural changes, inflammation, and apoptosis in the mouse heart. CD74 knockout can attenuate acute ethanol- and LPS-induced injury through AMPK-mTOR-mediated autophagy regulation (51, 86).
In a hyperoxic lung injury model, MIF- or CD74-deficiency and CD74 inhibition treatment results in worsen lung injury and poor survival, and inhibition of CD74 in primary murine lung endothelial cells (MLECs) abrogated the protective effect of MIF, suggesting endothelial CD74 mediates MIF protection in lung injury (87). In addition, interaction between rMIF and CD74 expressed on the surface of alveolar macrophages induces p44/p42 MAPK activation and inflammatory chemokine release, resulting in the accumulation of neutrophils in the alveolar space. This result suggests that alveolar macrophage-derived CD74 may mediate neutrophilic lung inflammation and acute lung injury (88).
Increased CD74 expression levels in circulating PBMCs mainly in CD4+ T cells, monocytes and dendric cells has been reported in human ischemic stroke patients (89). The number of CD74+ cells in blood correlated strongly with infarct size and neurological outcomes. However, the manifestation, exact action and clinical significance of CD74 expressed in a verity of different cell types under cardiac pathological settings is still required for further explorations.
Heinrichs et al. found in liver fibrosis disease that MIF exerted an anti-fibrotic effect by inhibiting PDGF-induced hepatic stellate cell migration and proliferation, which was promoted by CD74 through phosphorylation of AMPK (7). In mouse pressure-overload (TAC) model, the degree of fibrosis was four times higher in MIFKO mice than in WT mice (83). The combination of exogenous sCD74 and recombinant MIF could inhibit the MIF/CXCR4 axis, and induce a molecular switch from MIF-mediated transduction through pro-fibrotic CXCR4/Akt to anti-fibrotic CD74-mediated cell death, phosphorylation of protein kinases RIP 1 and RIP 3 (Figure 5), and finally necroptosis in the fibroblast (43). Endogenous CD74 also stimulates the antifibrotic effect of sCD74/MIF complex. Unlike the influence of sCD74/MIF in fibroblasts, combination of sCD74/MIF did not induce cardiomyocyte death (43). By measuring serum levels of sCD74 and MIF in patients with heart failure or MI and in healthy subjects, researchers found that the ratio of serum sCD74/MIF was only one-tenth that of normal controls in heart failure patients, and one-fifth that of normal controls in MI patients, and that the increased sCD74/MIF ratio would enhance the depletion of myofibroblasts in infarcted scars and alleviate reactive fibrosis (43). Ma et al. reported that rMIF2 treatment for mouse cardiac fibroblasts reduced TGF-β-induced Smad-2 phosphorylation and formation of extracellular matrix, thereby attenuating myocardial fibrosis. They then isolated cardiac fibroblasts from CD74–/– mice and stimulated with rMIF2 and found that rMIF2 failed to inhibit TGF-β-induced Smad-2 phosphorylation, indicating that CD74 receptor is required for mediating antifibrotic effects of MIF2 in fibroblasts (84).
A higher circulating level of MIF was reported in patients with atrial fibrillation (90). MIF may be a key factor in atrial arrhythmogenesis by regulating T-type and L-type calcium channels in atrial myocytes (91, 92). Additional evidence suggests that intraoperative serum MIF levels during myocardial reperfusion are independently associated with a lower risk of post-operative atrial fibrillation (44). MIF-treated atrial myocytes presented a higher calcium transient, increased sarcoplasmic reticulum calcium content, Ca2+ leakage, and Na+/Ca2+ exchanger (NCX) efflux rate. Whereas blocking CD74 abolished these effects of MIF (93). Likewise, MIF-treated atrial myocytes had augmented potassium currents, and blocking CD74 diminished such effect. In whole animals, MIF treatment in mice exhibited increased spontaneous atrial ectopic beats, but treatment with both MIF and anti-CD74 antibodies tended to have less atrial ectopic beats and atrial fibrosis (93). Moreover, treatment with anti-CD74 antibody only partially reversed MIF-induced atrial arrhythmias in mice (93). The potential underlying mechanism is related to expression of ATPase, NCX, ryanodine receptor 2 (RyR2) phosphorylation and CaMKII-RyR2 signaling activation triggered by MIF, which can be attenuated by blocking CD74 (Figure 5) (93). In additional, bioinformatic analysis of the myocardium of patients with arrhythmogenic right ventricular cardiomyopathy (ARVC) versus normal controls revealed an immune-related hub module, which Protein-Protein Interaction Network analysis identified CD74 as one of the most important hub genes (94). This information suggests that MIF/CD74 signaling is a potential therapeutic intervention for arrhythmia.
Cathepsin S is a cysteine protease of the papain family which modulates the antigen presentation of MHC II and is active in secreted form (95). It is reported that cathepsin S is mainly expressed in APCs such as B-cells, macrophages, and dendritic cells (96). MHC II molecules are loaded with antigenic peptides after degradation of CD74 and release of CLIP. In APCs, cathepsin S gene knockout or inhibition by small molecule compounds reduces the degradation of CD74 and prevents the binding of MHC II to antigen peptides, thus reducing antigen presentation to CD4+T cells (96). Studies have found that the increase of serum cathepsin S level was related to the aggravation of coronary artery stenosis, carotid artery thickening, blood pressure increase, and abnormal vascular endothelial function (97). However, it is not related to the increased risk of cardiovascular events in patients with stable coronary heart disease (98). Plasma cathepsin S is significantly elevated in patients with unstable angina, and the higher level of cathepsin S suggests the existence of vulnerable plaques (99). Endogenous cathepsin S plays an important role in atherosclerotic plaque instability and plaque rupture (100). Cathepsin S may participate in the progression of atherosclerosis through macrophage mediated phagosomes (101). Treatment with cathepsin S inhibitors reduced the size of atherosclerotic plaques by 36% in male and 68% in female mice (102). Moreover, cathepsin S selective inhibitors LY3000328 or MIV-247 attenuated myocardial I/R injury by inhibiting inflammation and apoptosis and improved survival at 21 days after ischemic insult, suggesting the potential value of clinical application of cathepsin S inhibitors for cardioprotection (103). However, some studies also reported that cathepsin S deficiency exacerbated LV dilatation and dysfunction after MI, and administration of the non-selective histone inhibitor E64d at 7 and 28 days after MI enhanced inflammatory response and worsened LV dysfunction. Meanwhile, it also inhibited phosphorylation of Smad 2/3, the key molecules of fibrosis signaling pathway, resulting in impaired cardiac remodeling (104). Therefore, whether cathepsin S is involved in pathophysiological alterations in cardiovascular disease by affecting CD74 remains to be further investigated.
Small interfering RNAs (siRNAs) are short double-stranded RNAs that can be dissociated into single strands and bound specifically to target messenger RNA (mRNA) sequences. Their conjugation induces target mRNA cleavage and degradation, thus preventing translation and protein expression (105, 106). In recent years, siRNA-based therapeutics have been a popular topic, with numerous medications approved to access the pharmaceutical market (105, 107). CD74 siRNA, which silences the CD74 gene in dendritic cells, may improve dendritic cell-based antitumor immunity (108, 109). CD74 siRNA has been proved to inhibit a variety of tumors, such as breast cancer (110), acute myeloid leukemia (111), head and neck squamous cell carcinoma (112), and brain tumorigenesis (113). Interestingly, CD74 siRNA inhibits atherosclerosis by reducing interferon-gamma (IFNγ)-induced NF-κB activation and monocyte chemoattractant protein-1 (MCP-1) production in human vascular smooth muscle cells, and preventing vascular smooth muscle cells activation (49). Stem/progenitor cell-based treatments are effective therapies for a variety of heart diseases, including ischemic heart disease and heart failure. These cells stimulate angiogenesis and endogenous stem cell activation, thereby improving cardiac function (114, 115). MIF promotes cardiac stem cell survival and proliferation through CD74 to activate the PI3K/Akt/mTOR and AMPK signaling pathways. However, siRNA for CD74 reduced the proliferation of MIF-treated cardiac stem cell, and cell cycle analysis also showed that CD74 knockdown significantly reduced the number of S-phase cells. The activation of AMPK by MIF was significantly inhibited after knockdown of CD74 (115). As a result, since CD74 plays different roles in various cardiovascular diseases, CD74 siRNA may have clinical applications in certain cardiovascular diseases.
Leukocyte differentiation antigen 74 is highly expressed in a variety of tumor cells, such as breast cancer (116), lymphoma (117), multiple myeloma (118), and chronic lymphocytic leukemia (119, 120). The profile of CD74 expression in tumor cells and its rapid endocytosis properties make it a promising therapeutic target. Monoclonal antibodies are highly uniform antibodies produced by B-cells clones, which only target a specific antigen epitope. They can specifically recognize cell surface markers to achieve precise targeted therapy. Milatuzuma is a synthetic monoclonal antibody that targets CD74 and has been used in the therapy of several tumors, such as chronic granulocytic leukemia (119) and various lymphomas (120). Milatuzuma reduces expression of adhesion molecules β7-integrin and CD62L and inhibits B-cells proliferation (121). In addition, Milatuzumab is also used in the treatment of autoimmune diseases such as SLE (122). Current clinical information on utilization of Milatuzumab in a variety of oncological as well as autoimmune diseases will facilitate the clinical transition of targeted CD74 therapy to cardiovascular disease.
Leukocyte differentiation antigen 74 is involved in antigen presentation as a molecular chaperone of MHC II and has also been recently identified to be involved in antigen presentation of MHC I. CD74 binds to the two main ligands MIF/MIF2 and activates several downstream signaling pathways. Activation of NF-κB pathway by MIF/MIF2-CD74 promoted B-cells maturation and activation of AMPK pathway promoted glucose uptake and utilization in the myocardium under ischemic and hypoxic conditions as well as pressure overload, which played a protective role for the heart. CD74 also regulates cytosolic calcium homeostasis that plays a regulatory role in cardiac arrhythmias. MIF-CD74 works with CXCR2 to act as a chemotactic agent, promoting atherosclerosis progression. sCD74, a truncated form of the extracellular domain of CD74, was first identified in autoimmune liver disease and subsequently in patients with heart failure and MI, yet its specific role in disease progression remains to be further explored.
Although MIF/MIF2 both play important roles in various diseases through CD74, interaction between MIF/MIF2 and CD74 may be independent. Therapeutic strategies targeting CD74 may provide additional options for the clinical treatment of multiple cardiovascular diseases. The characteristics of high expression of CD74 in atherosclerosis and acute MI and the rapid endocytosis of CD74 provide an essential basis for the precise targeting of CD74 for treatment. Currently, Milatuzumab, an anti-CD74 monoclonal antibody, has been tested in numerous phase I-II clinical trials in a variety of tumors as well as autoimmune diseases. Cathepsin S inhibitors and siRNA targeting the regulation of CD74 function have also made progress in animal models of atherosclerosis or cardiac I/R injury. Nevertheless, the role of CD74 in cardiovascular disease remains obscure and needs to be further studied. Targeted drug therapies for CD74 in cardiovascular disease remain in urgent need of exploration.
Q-LL drafted the manuscript. JT and LZ generated the images. AR and X-FS edited the manuscript. X-MG revised the manuscript. All authors have read and approved the content of the manuscript.
This work was supported by grants of the Natural Science Foundation of China (Nos. U1903212 and 81870272), Xinjiang University Scientific Research Project (XJEDU2019I018), Opening Project of the Xinjiang Key Laboratory (2021D04020), and the State Key Laboratory of Pathogenesis, Prevention and Treatment of Central Asia High Incidence Diseases fund (SKL-HIDCA-2021-XXG1).
The authors declare that the research was conducted in the absence of any commercial or financial relationships that could be construed as a potential conflict of interest.
All claims expressed in this article are solely those of the authors and do not necessarily represent those of their affiliated organizations, or those of the publisher, the editors and the reviewers. Any product that may be evaluated in this article, or claim that may be made by its manufacturer, is not guaranteed or endorsed by the publisher.
1. Su H, Na N, Zhang X, Zhao Y. The biological function and significance of CD74 in immune diseases. Inflamm Res. (2017) 66:209–16. doi: 10.1007/s00011-016-0995-1
2. Basha G, Omilusik K, Chavez-Steenbock A, Reinicke A, Lack N, Choi K, et al. A CD74-dependent MHC class I endolysosomal cross-presentation pathway. Nat Immunol. (2012) 13:237–45. doi: 10.1038/ni.2225
3. Schoeps B, Eckfeld C, Flüter L, Keppler S, Mishra R, Knolle P, et al. Identification of invariant chain CD74 as a functional receptor of tissue inhibitor of metalloproteinases-1 (TIMP-1). J Biol Chem. (2021) 297:101072. doi: 10.1016/j.jbc.2021.101072
4. Szaszák M, Chen H, Chen H, Baukal A, Hunyady L, Catt K. Identification of the invariant chain (CD74) as an angiotensin AGTR1-interacting protein. J Endocrinol. (2008) 199:165–76. doi: 10.1677/joe-08-0190
5. Voss S, Krüger S, Scherschel K, Warnke S, Schwarzl M, Schrage B, et al. Macrophage Migration Inhibitory Factor (MIF) Expression Increases during Myocardial Infarction and Supports Pro-Inflammatory Signaling in Cardiac Fibroblasts. Biomolecules. (2019) 9:38. doi: 10.3390/biom9020038
6. Sun J, Hartvigsen K, Chou M, Zhang Y, Sukhova G, Zhang J, et al. Deficiency of antigen-presenting cell invariant chain reduces atherosclerosis in mice. Circulation. (2010) 122:808–20. doi: 10.1161/circulationaha.109.891887
7. Heinrichs D, Knauel M, Offermanns C, Berres M, Nellen A, Leng L, et al. Macrophage migration inhibitory factor (MIF) exerts antifibrotic effects in experimental liver fibrosis via CD74. Proc Natl Acad Sci USA. (2011) 108:17444–9. doi: 10.1073/pnas.1107023108
8. Meza-Romero R, Benedek G, Jordan K, Leng L, Pantouris G, Lolis E, et al. Modeling of both shared and distinct interactions between MIF and its homologue MIF2 with their common receptor CD74. Cytokine. (2016) 88:62–70. doi: 10.1016/j.cyto.2016.08.024
9. Koch N, Lauer W, Habicht J, Dobberstein B. Primary structure of the gene for the murine Ia antigen-associated invariant chains (Ii). An alternatively spliced exon encodes a cysteine-rich domain highly homologous to a repetitive sequence of thyroglobulin. EMBO J. (1987) 6:1677–83.
10. Stumptner-Cuvelette P, Benaroch P. Multiple roles of the invariant chain in MHC class II function. Biochim Biophys Acta. (2002) 1542:1–13. doi: 10.1016/s0167-4889(01)00166-5
11. Shachar I, Elliott E, Chasnoff B, Grewal I, Flavell R. Reconstitution of invariant chain function in transgenic mice in vivo by individual p31 and p41 isoforms. Immunity. (1995) 3:373–83. doi: 10.1016/1074-7613(95)90121-3
12. Kuwana T, Peterson P, Karlsson L. Exit of major histocompatibility complex class II-invariant chain p35 complexes from the endoplasmic reticulum is modulated by phosphorylation. Proc Natl Acad Sci USA. (1998) 95:1056–61. doi: 10.1073/pnas.95.3.1056
13. Anderson H, Roche P. Phosphorylation regulates the delivery of MHC class II invariant chain complexes to antigen processing compartments. J Immunol. (1998) 160:4850–8.
14. Fiebiger E, Maehr R, Villadangos J, Weber E, Erickson A, Bikoff E, et al. Invariant chain controls the activity of extracellular cathepsin L. J Exp Med. (2002) 196:1263–9. doi: 10.1084/jem.20020762
15. Bangia N, Watts T. Evidence for invariant chain 85-101 (CLIP) binding in the antigen binding site of MHC class II molecules. Int Immunol. (1995) 7:1585–91. doi: 10.1093/intimm/7.10.1585
16. Bijlmakers M, Benaroch P, Ploegh H. Mapping functional regions in the lumenal domain of the class II-associated invariant chain. J Exp Med. (1994) 180:623–9. doi: 10.1084/jem.180.2.623
17. Ashman J, Miller J. A role for the transmembrane domain in the trimerization of the MHC class II-associated invariant chain. J Immunol. (1999) 163:2704–12.
18. Cloutier M, Fortin J, Thibodeau J. The transmembrane domain and luminal C-terminal region independently support invariant chain trimerization and assembly with MHCII into nonamers. BMC Immunol. (2021) 22:56. doi: 10.1186/s12865-021-00444-6
19. Schroder B. The multifaceted roles of the invariant chain CD74–More than just a chaperone. Biochim Biophys Acta. (2016) 1863:1269–81. doi: 10.1016/j.bbamcr.2016.03.026
20. Roche P, Furuta K. The ins and outs of MHC class II-mediated antigen processing and presentation. Nat Rev Immunol. (2015) 15:203–16. doi: 10.1038/nri3818
21. Riese R, Chapman H. Cathepsins and compartmentalization in antigen presentation. Curr Opin Immunol. (2000) 12:107–13. doi: 10.1016/s0952-7915(99)00058-8
22. Neefjes J, Ploegh H. Inhibition of endosomal proteolytic activity by leupeptin blocks surface expression of MHC class II molecules and their conversion to SDS resistance alpha beta heterodimers in endosomes. EMBO J. (1992) 11:411–6. doi: 10.1002/j.1460-2075.1992.tb05069.x
23. Mosyak L, Zaller D, Wiley D. The structure of HLA-DM, the peptide exchange catalyst that loads antigen onto class II MHC molecules during antigen presentation. Immunity. (1998) 9:377–83. doi: 10.1016/s1074-7613(00)80620-2
24. Welsh R, Sadegh-Nasseri S. The love and hate relationship of HLA-DM/DO in the selection of immunodominant epitopes. Curr Opin Immunol. (2020) 64:117–23. doi: 10.1016/j.coi.2020.05.007
25. Weiser W, Temple P, Witek-Giannotti J, Remold H, Clark S, David J. Molecular cloning of a cDNA encoding a human macrophage migration inhibitory factor. Proc Natl Acad Sci USA. (1989) 86:7522–6. doi: 10.1073/pnas.86.19.7522
26. Bendrat K, Al-Abed Y, Callaway D, Peng T, Calandra T, Metz C, et al. Biochemical and mutational investigations of the enzymatic activity of macrophage migration inhibitory factor. Biochemistry. (1997) 36:15356–62. doi: 10.1021/bi971153a
27. Merk M, Zierow S, Leng L, Das R, Du X, Schulte W, et al. The D-dopachrome tautomerase (DDT) gene product is a cytokine and functional homolog of macrophage migration inhibitory factor (MIF). Proc Natl Acad Sci USA. (2011) 108:E577–85. doi: 10.1073/pnas.1102941108
28. Sugimoto H, Taniguchi M, Nakagawa A, Tanaka I, Suzuki M, Nishihira J. Crystal structure of human D-dopachrome tautomerase, a homologue of macrophage migration inhibitory factor, at 1.54 A resolution. Biochemistry. (1999) 38:3268–79. doi: 10.1021/bi982184o
29. Leng L, Metz C, Fang Y, Xu J, Donnelly S, Baugh J, et al. MIF signal transduction initiated by binding to CD74. J Exp Med. (2003) 197:1467–76. doi: 10.1084/jem.20030286
30. Jankauskas S, Wong D, Bucala R, Djudjaj S, Boor P. Evolving complexity of MIF signaling. Cell Signal. (2019) 57:76–88. doi: 10.1016/j.cellsig.2019.01.006
31. Senbanjo L, Chellaiah M. CD44: a multifunctional cell surface adhesion receptor is a regulator of progression and metastasis of cancer cells. Front Cell Dev Biol. (2017) 5:18. doi: 10.3389/fcell.2017.00018
32. Shi X, Leng L, Wang T, Wang W, Du X, Li J, et al. CD44 is the signaling component of the macrophage migration inhibitory factor-CD74 receptor complex. Immunity. (2006) 25:595–606. doi: 10.1016/j.immuni.2006.08.020
33. Meza-Romero R, Benedek G, Leng L, Bucala R, Vandenbark A. Predicted structure of MIF/CD74 and RTL1000/CD74 complexes. Metab Brain Dis. (2016) 31:249–55. doi: 10.1007/s11011-016-9798-x
34. Lue H, Thiele M, Franz J, Dahl E, Speckgens S, Leng L, et al. Macrophage migration inhibitory factor (MIF) promotes cell survival by activation of the Akt pathway and role for CSN5/JAB1 in the control of autocrine MIF activity. Oncogene. (2007) 26:5046–59. doi: 10.1038/sj.onc.1210318
35. Osaki M, Oshimura M, Ito H. PI3K-Akt pathway: its functions and alterations in human cancer. Apoptosis. (2004) 9:667–76. doi: 10.1023/B:APPT.0000045801.15585.dd
36. Matza D, Kerem A, Medvedovsky H, Lantner F, Shachar I. Invariant chain-induced B cell differentiation requires intramembrane proteolytic release of the cytosolic domain. Immunity. (2002) 17:549–60. doi: 10.1016/s1074-7613(02)00455-7
37. Matza D, Wolstein O, Dikstein R, Shachar I. Invariant chain induces B cell maturation by activating a TAF(II)105-NF-kappaB-dependent transcription program. J Biol Chem. (2001) 276:27203–6. doi: 10.1074/jbc.M104684200
38. Gore Y, Starlets D, Maharshak N, Becker-Herman S, Kaneyuki U, Leng L, et al. Macrophage migration inhibitory factor induces B cell survival by activation of a CD74-CD44 receptor complex. J Biol Chem. (2008) 283:2784–92. doi: 10.1074/jbc.M703265200
39. Becker-Herman S, Arie G, Medvedovsky H, Kerem A, Shachar I. CD74 is a member of the regulated intramembrane proteolysis-processed protein family. Mol Biol Cell. (2005) 16:5061–9. doi: 10.1091/mbc.e05-04-0327
40. Assis D, Leng L, Du X, Zhang C, Grieb G, Merk M, et al. The role of macrophage migration inhibitory factor in autoimmune liver disease. Hepatology. (2014) 59:580–91. doi: 10.1002/hep.26664
41. Kim B, Stoppe C, Grieb G, Leng L, Sauler M, Assis D, et al. The clinical significance of the MIF homolog d-dopachrome tautomerase (MIF-2) and its circulating receptor (sCD74) in burn. Burns. (2016) 42:1265–76. doi: 10.1016/j.burns.2016.02.005
42. Wu G, Sun Y, Wang K, Chen Z, Wang X, Chang F, et al. Relationship between elevated soluble CD74 and severity of experimental and clinical ALI/ARDS. Sci Rep. (2016) 6:30067. doi: 10.1038/srep30067
43. Soppert J, Kraemer S, Beckers C, Averdunk L, Möllmann J, Denecke B, et al. Soluble CD74 reroutes MIF/CXCR4/AKT-mediated survival of cardiac myofibroblasts to necroptosis. J Am Heart Assoc. (2018) 7:e009384. doi: 10.1161/jaha.118.009384
44. Stoppe C, Rex S, Goetzenich A, Kraemer S, Emontzpohl C, Soppert J, et al. Interaction of MIF family proteins in myocardial ischemia/reperfusion damage and their influence on clinical outcome of cardiac surgery patients. Antioxid Redox Signal. (2015) 23:865–79. doi: 10.1089/ars.2014.6243
45. Tilstam P, Qi D, Leng L, Young L, Bucala R. MIF family cytokines in cardiovascular diseases and prospects for precision-based therapeutics. Expert Opin Ther Targets. (2017) 21:671–83. doi: 10.1080/14728222.2017.1336227
46. Sanchez-Nino M, Sanz A, Ruiz-Andres O, Poveda J, Izquierdo M, Selgas R, et al. MIF, CD74 and other partners in kidney disease: tales of a promiscuous couple. Cytokine Growth Factor Rev. (2013) 24:23–40. doi: 10.1016/j.cytogfr.2012.08.001
47. Momburg F, Koch N, Möller P, Moldenhauer G, Butcher G, Hämmerling G. Differential expression of Ia and Ia-associated invariant chain in mouse tissues after in vivo treatment with IFN-gamma. J Immunol. (1986) 136:940–8.
48. Badve S, Deshpande C, Hua Z, Lögdberg L. Expression of invariant chain (CD 74) and major histocompatibility complex (MHC) class II antigens in the human fetus. J Histochem Cytochem. (2002) 50:473–82. doi: 10.1177/002215540205000404
49. Martín-Ventura J, Madrigal-Matute J, Muñoz-Garcia B, Blanco-Colio L, Van Oostrom M, Zalba G, et al. Increased CD74 expression in human atherosclerotic plaques: contribution to inflammatory responses in vascular cells. Cardiovasc Res. (2009) 83:586–94. doi: 10.1093/cvr/cvp141
50. Sanchez-Niño M, Sanz A, Ihalmo P, Lassila M, Holthofer H, Mezzano S, et al. The MIF receptor CD74 in diabetic podocyte injury. J Am Soc Nephrol. (2009) 20:353–62. doi: 10.1681/asn.2008020194
51. Luo Y, Fan C, Yang M, Dong M, Bucala R, Pei Z, et al. CD74 knockout protects against LPS-induced myocardial contractile dysfunction through AMPK-Skp2-SUV39H1-mediated demethylation of BCLB. Br J Pharmacol. (2020) 177:1881–97. doi: 10.1111/bph.14959
52. Miller E, Li J, Leng L, McDonald C, Atsumi T, Bucala R, et al. Macrophage migration inhibitory factor stimulates AMP-activated protein kinase in the ischaemic heart. Nature. (2008) 451:578–82. doi: 10.1038/nature06504
53. Liehn E, Kanzler I, Konschalla S, Kroh A, Simsekyilmaz S, Sönmez T, et al. Compartmentalized protective and detrimental effects of endogenous macrophage migration-inhibitory factor mediated by CXCR2 in a mouse model of myocardial ischemia/reperfusion. Arterioscler Thromb Vasc Biol. (2013) 33:2180–6. doi: 10.1161/atvbaha.113.301633
54. Roche P, Teletski C, Stang E, Bakke O, Long E. Cell surface HLA-DR-invariant chain complexes are targeted to endosomes by rapid internalization. Proc Natl Acad Sci USA. (1993) 90:8581–5. doi: 10.1073/pnas.90.18.8581
55. Schober A, Bernhagen J, Weber C. Chemokine-like functions of MIF in atherosclerosis. J Mol Med. (2008) 86:761–70. doi: 10.1007/s00109-008-0334-2
56. Pan J, Sukhova G, Yang J, Wang B, Xie T, Fu H, et al. Macrophage migration inhibitory factor deficiency impairs atherosclerosis in low-density lipoprotein receptor-deficient mice. Circulation. (2004) 109:3149–53. doi: 10.1161/01.Cir.0000134704.84454.D2
57. Lin S, Yu X, Chen Y, Huang X, Metz C, Bucala R, et al. De novo expression of macrophage migration inhibitory factor in atherogenesis in rabbits. Circ Res. (2000) 87:1202–8. doi: 10.1161/01.res.87.12.1202
58. Sinitski D, Kontos C, Krammer C, Asare Y, Kapurniotu A, Bernhagen J. Macrophage migration inhibitory factor (MIF)-based therapeutic concepts in atherosclerosis and inflammation. Thromb Haemost. (2019) 119:553–66. doi: 10.1055/s-0039-1677803
59. Degryse B, de Virgilio M. The nuclear protein HMGB1, a new kind of chemokine? FEBS Lett. (2003) 553:11–7. doi: 10.1016/s0014-5793(03)01027-5
60. Bernhagen J, Krohn R, Lue H, Gregory J, Zernecke A, Koenen R, et al. MIF is a noncognate ligand of CXC chemokine receptors in inflammatory and atherogenic cell recruitment. Nat Med. (2007) 13:587–96. doi: 10.1038/nm1567
61. Lue H, Dewor M, Leng L, Bucala R, Bernhagen J. Activation of the JNK signalling pathway by macrophage migration inhibitory factor (MIF) and dependence on CXCR4 and CD74. Cell Signal. (2011) 23:135–44. doi: 10.1016/j.cellsig.2010.08.013
62. Weber C, Kraemer S, Drechsler M, Lue H, Koenen R, Kapurniotu A, et al. Structural determinants of MIF functions in CXCR2-mediated inflammatory and atherogenic leukocyte recruitment. Proc Natl Acad Sci USA. (2008) 105:16278–83. doi: 10.1073/pnas.0804017105
63. Mallat Z, Gojova A, Brun V, Esposito B, Fournier N, Cottrez F, et al. Induction of a regulatory T cell type 1 response reduces the development of atherosclerosis in apolipoprotein E-knockout mice. Circulation. (2003) 108:1232–7. doi: 10.1161/01.Cir.0000089083.61317.A1
64. Bucala R, Shachar I. The integral role of CD74 in antigen presentation, MIF signal transduction, and B cell survival and homeostasis. Mini Rev Med Chem. (2014) 14:1132–8. doi: 10.2174/1389557515666150203144111
65. Klasen C, Ohl K, Sternkopf M, Shachar I, Schmitz C, Heussen N, et al. MIF promotes B cell chemotaxis through the receptors CXCR4 and CD74 and ZAP-70 signaling. J Immunol. (2014) 192:5273–84. doi: 10.4049/jimmunol.1302209
66. Schmitz C, Noels H, El Bounkari O, Straussfeld E, Megens R, Sternkopf M, et al. Mif-deficiency favors an atheroprotective autoantibody phenotype in atherosclerosis. FASEB J. (2018) 32:4428–43. doi: 10.1096/fj.201800058R
67. Shachar I, Flavell R. Requirement for invariant chain in B cell maturation and function. Science. (1996) 274:106–8. doi: 10.1126/science.274.5284.106
68. Matza D, Lantner F, Bogoch Y, Flaishon L, Hershkoviz R, Shachar I. Invariant chain induces B cell maturation in a process that is independent of its chaperonic activity. Proc Natl Acad Sci USA. (2002) 99:3018–23. doi: 10.1073/pnas.052703299
69. Ait-Oufella H, Herbin O, Bouaziz J, Binder C, Uyttenhove C, Laurans L, et al. B cell depletion reduces the development of atherosclerosis in mice. J Exp Med. (2010) 207:1579–87. doi: 10.1084/jem.20100155
70. Li W, Sultana N, Yuan L, Forssell C, Yuan X. CD74 in Apoptotic Macrophages Is Associated with Inflammation, Plaque Progression and Clinical Manifestations in Human Atherosclerotic Lesions. Metabolites. (2022) 12:54. doi: 10.3390/metabo12010054
71. Qi D, Atsina K, Qu L, Hu X, Wu X, Xu B, et al. The vestigial enzyme D-dopachrome tautomerase protects the heart against ischemic injury. J Clin Invest. (2014) 124:3540–50. doi: 10.1172/jci73061
72. Chan W, White D, Wang X, Bai R, Liu Y, Yu H, et al. Macrophage migration inhibitory factor for the early prediction of infarct size. J Am Heart Assoc. (2013) 2:e000226. doi: 10.1161/jaha.113.000226
73. Ma H, Wang J, Thomas D, Tong C, Leng L, Wang W, et al. Impaired macrophage migration inhibitory factor-AMP-activated protein kinase activation and ischemic recovery in the senescent heart. Circulation. (2010) 122:282–92. doi: 10.1161/circulationaha.110.953208
74. Ruze A, Chen B, Liu F, Chen X, Gai M, Li X, et al. Macrophage migration inhibitory factor plays an essential role in ischemic preconditioning-mediated cardioprotection. Clin Sci. (2019) 133:665–80. doi: 10.1042/cs20181013
75. Young L, Li J, Baron S, Russell RR. AMP-activated protein kinase: a key stress signaling pathway in the heart. Trends Cardiovasc Med. (2005) 15:110–8. doi: 10.1016/j.tcm.2005.04.005
76. Qi D, Hu X, Wu X, Merk M, Leng L, Bucala R, et al. Cardiac macrophage migration inhibitory factor inhibits JNK pathway activation and injury during ischemia/reperfusion. J Clin Invest. (2009) 119:3807–16. doi: 10.1172/jci39738
77. Stoppe C, Werker T, Rossaint R, Dollo F, Lue H, Wonisch W, et al. What is the significance of perioperative release of macrophage migration inhibitory factor in cardiac surgery? Antioxid Redox Signal. (2013) 19:231–9. doi: 10.1089/ars.2012.5015
78. Koga K, Kenessey A, Powell S, Sison C, Miller E, Ojamaa K. Macrophage migration inhibitory factor provides cardioprotection during ischemia/reperfusion by reducing oxidative stress. Antioxid Redox Signal. (2011) 14:1191–202. doi: 10.1089/ars.2010.3163
79. de Mendonça-Filho H, Pereira K, Fontes M, Vieira D, de Mendonça M, Campos L, et al. Circulating inflammatory mediators and organ dysfunction after cardiovascular surgery with cardiopulmonary bypass: a prospective observational study. Crit Care. (2006) 10:R46. doi: 10.1186/cc4857
80. Garner L, Willis M, Carlson D, DiMaio J, White M, White D, et al. Macrophage migration inhibitory factor is a cardiac-derived myocardial depressant factor. Am J Physiol Heart Circ Physiol. (2003) 285:H2500–9. doi: 10.1152/ajpheart.00432.2003
81. Chatterjee M, Borst O, Walker B, Fotinos A, Vogel S, Seizer P, et al. Macrophage migration inhibitory factor limits activation-induced apoptosis of platelets via CXCR7-dependent Akt signaling. Circ Res. (2014) 115:939–49. doi: 10.1161/circresaha.115.305171
82. Xu X, Hua Y, Nair S, Bucala R, Ren J. Macrophage migration inhibitory factor deletion exacerbates pressure overload-induced cardiac hypertrophy through mitigating autophagy. Hypertension. (2014) 63:490–9. doi: 10.1161/hypertensionaha.113.02219
83. Koga K, Kenessey A, Ojamaa K. Macrophage migration inhibitory factor antagonizes pressure overload-induced cardiac hypertrophy. Am J Physiol Heart Circ Physiol. (2013) 304:H282–93. doi: 10.1152/ajpheart.00595.2012
84. Ma Y, Su K, Pfau D, Rao V, Wu X, Hu X, et al. Cardiomyocyte d-dopachrome tautomerase protects against heart failure. JCI Insight. (2019) 4:e128900. doi: 10.1172/jci.insight.128900
85. Chen L, Yin Z, Qin X, Zhu X, Chen X, Ding G, et al. CD74 ablation rescues type 2 diabetes mellitus-induced cardiac remodeling and contractile dysfunction through pyroptosis-evoked regulation of ferroptosis. Pharmacol Res. (2022) 176:106086. doi: 10.1016/j.phrs.2022.106086
86. Yang L, Wang S, Ma J, Li J, Yang J, Bucala R, et al. CD74 knockout attenuates alcohol intake-induced cardiac dysfunction through AMPK-Skp2-mediated regulation of autophagy. Biochim Biophys Acta Mol Basis Dis. (2019) 1865:2368–78. doi: 10.1016/j.bbadis.2019.05.020
87. Sauler M, Zhang Y, Min J, Leng L, Shan P, Roberts S, et al. Endothelial CD74 mediates macrophage migration inhibitory factor protection in hyperoxic lung injury. FASEB J. (2015) 29:1940–9. doi: 10.1096/fj.14-260299
88. Takahashi K, Koga K, Linge H, Zhang Y, Lin X, Metz C, et al. Macrophage CD74 contributes to MIF-induced pulmonary inflammation. Respir Res. (2009) 10:33. doi: 10.1186/1465-9921-10-33
89. Yang L, Kong Y, Ren H, Li M, Wei C, Shi E, et al. Upregulation of CD74 and its potential association with disease severity in subjects with ischemic stroke. Neurochem Int. (2017) 107:148–55. doi: 10.1016/j.neuint.2016.11.007
90. Wan C, Li Z. Serum macrophage migration inhibitory factor is correlated with atrial fibrillation. J Clin Lab Anal. (2018) 32:e22225. doi: 10.1002/jcla.22225
91. Rao F, Deng C, Wu S, Xiao D, Huang W, Deng H, et al. Mechanism of macrophage migration inhibitory factor-induced decrease of T-type Ca(2+) channel current in atrium-derived cells. Exp Physiol. (2013) 98:172–82. doi: 10.1113/expphysiol.2012.066761
92. Rao F, Deng C, Wu S, Xiao D, Yu X, Kuang S, et al. Involvement of Src in L-type Ca2+ channel depression induced by macrophage migration inhibitory factor in atrial myocytes. J Mol Cell Cardiol. (2009) 47:586–94. doi: 10.1016/j.yjmcc.2009.08.030
93. Cheng W, Kao Y, Chen Y, Lin Y, Chen S, Chen Y. Macrophage migration inhibitory factor increases atrial arrhythmogenesis through CD74 signaling. Transl Res. (2020) 216:43–56. doi: 10.1016/j.trsl.2019.10.002
94. Lu W, Li Y, Dai Y, Chen K. Dominant myocardial fibrosis and complex immune microenvironment jointly shape the pathogenesis of arrhythmogenic right ventricular cardiomyopathy. Front Cardiovasc Med. (2022) 9:900810. doi: 10.3389/fcvm.2022.900810
95. Lee-Dutra A, Wiener D, Sun S. Cathepsin S inhibitors: 2004-2010. Expert Opin Ther Pat. (2011) 21:311–37. doi: 10.1517/13543776.2011.553800
96. Shi G, Villadangos J, Dranoff G, Small C, Gu L, Haley K, et al. Cathepsin S required for normal MHC class II peptide loading and germinal center development. Immunity. (1999) 10:197–206. doi: 10.1016/s1074-7613(00)80020-5
97. Huang S, Cao Y. Correlation of cathepsin S with coronary stenosis degree, carotid thickness, blood pressure, glucose and lipid metabolism and vascular endothelial function in atherosclerosis. Exp Ther Med. (2020) 19:61–6. doi: 10.3892/etm.2019.8222
98. Wuopio J, Hilden J, Bring C, Kastrup J, Sajadieh A, Jensen G, et al. Cathepsin B and S as markers for cardiovascular risk and all-cause mortality in patients with stable coronary heart disease during 10 years: a CLARICOR trial sub-study. Atherosclerosis. (2018) 278:97–102. doi: 10.1016/j.atherosclerosis.2018.09.006
99. Gu F, Lü S, Chen Y, Zhou Y, Song X, Jin Z, et al. Relationship between plasma cathepsin S and cystatin C levels and coronary plaque morphology of mild to moderate lesions: an in vivo study using intravascular ultrasound. Chin Med J. (2009) 122:2820–6.
100. Rodgers K, Watkins D, Miller A, Chan P, Karanam S, Brissette W, et al. Destabilizing role of cathepsin S in murine atherosclerotic plaques. Arterioscler Thromb Vasc Biol. (2006) 26:851–6. doi: 10.1161/01.ATV.0000203526.75772.4b
101. Wang H, Jiang H, Cheng X. Cathepsin S are involved in human carotid atherosclerotic disease progression, mainly by mediating phagosomes: bioinformatics and in vivo and vitro experiments. PeerJ. (2022) 10:e12846. doi: 10.7717/peerj.12846
102. Samokhin A, Lythgo P, Gauthier J, Percival M, Brömme D. Pharmacological inhibition of cathepsin S decreases atherosclerotic lesions in Apoe-/- mice. J Cardiovasc Pharmacol. (2010) 56:98–105. doi: 10.1097/FJC.0b013e3181e23e10
103. Chen H, Wang J, Xiang M, Lin Y, He A, Jin C, et al. Cathepsin S-mediated fibroblast trans-differentiation contributes to left ventricular remodelling after myocardial infarction. Cardiovasc Res. (2013) 100:84–94. doi: 10.1093/cvr/cvt158
104. Peng K, Liu H, Yan B, Meng X, Song S, Ji F, et al. Inhibition of cathepsin S attenuates myocardial ischemia/reperfusion injury by suppressing inflammation and apoptosis. J Cell Physiol. (2021) 236:1309–20. doi: 10.1002/jcp.29938
105. Zhang M, Bahal R, Rasmussen T, Manautou J, Zhong X. The growth of siRNA-based therapeutics: updated clinical studies. Biochem Pharmacol. (2021) 189:114432. doi: 10.1016/j.bcp.2021.114432
106. Alshaer W, Zureigat H, Al Karaki A, Al-Kadash A, Gharaibeh L, Hatmal M, et al. siRNA: mechanism of action, challenges, and therapeutic approaches. Eur J Pharmacol. (2021) 905:174178. doi: 10.1016/j.ejphar.2021.174178
107. Saw P, Song E. siRNA therapeutics: a clinical reality. Sci China Life Sci. (2020) 63:485–500. doi: 10.1007/s11427-018-9438-y
108. Ke S, Chen X, Zhu Z, Li J, Yu B, Gu Q, et al. Silencing invariant chains of dendritic cells enhances anti-tumor immunity using small-interfering RNA. Chin Med J. (2010) 123:3193–9.
109. Ke S, Chen X, Li H, Li J, Gu Q, Liu B, et al. Silencing invariant chain of DCs enhances Th1 response using small interfering RNA. Cell Biol Int. (2007) 31:663–71. doi: 10.1016/j.cellbi.2006.12.004
110. Ssadh H, Abdulmonem W, Rasheed Z, Madar I, Alhoderi J, Eldeen S, et al. Knockdown of CD-74 in the proliferative and apoptotic activity of breast cancer cells. Open Access Maced J Med Sci. (2019) 7:3169–76. doi: 10.3889/oamjms.2019.354
111. van Luijn M, Chamuleau M, Thompson J, Ostrand-Rosenberg S, Westers T, Souwer Y, et al. Class II-associated invariant chain peptide down-modulation enhances the immunogenicity of myeloid leukemic blasts resulting in increased CD4+ T-cell responses. Haematologica. (2010) 95:485–93. doi: 10.3324/haematol.2009.010595
112. Kindt N, Lechien J, Nonclercq D, Laurent G, Saussez S. Involvement of CD74 in head and neck squamous cell carcinomas. J Cancer Res Clin Oncol. (2014) 140:937–47. doi: 10.1007/s00432-014-1648-9
113. Ghoochani A, Schwarz M, Yakubov E, Engelhorn T, Doerfler A, Buchfelder M, et al. MIF-CD74 signaling impedes microglial M1 polarization and facilitates brain tumorigenesis. Oncogene. (2016) 35:6246–61. doi: 10.1038/onc.2016.160
114. Tang X, Rokosh G, Sanganalmath S, Yuan F, Sato H, Mu J, et al. Intracoronary administration of cardiac progenitor cells alleviates left ventricular dysfunction in rats with a 30-day-old infarction. Circulation. (2010) 121:293–305. doi: 10.1161/circulationaha.109.871905
115. Cui J, Zhang F, Wang Y, Liu J, Ming X, Hou J, et al. Macrophage migration inhibitory factor promotes cardiac stem cell proliferation and endothelial differentiation through the activation of the PI3K/Akt/mTOR and AMPK pathways. Int J Mol Med. (2016) 37:1299–309. doi: 10.3892/ijmm.2016.2542
116. Noer J, Talman M, Moreira J. HLA class II histocompatibility antigen γ chain (CD74) expression is associated with immune cell infiltration and favorable outcome in breast cancer. Cancers. (2021) 13:6179. doi: 10.3390/cancers13246179
117. Zhao S, Molina A, Yu A, Hanson J, Cheung H, Li X, et al. High frequency of CD74 expression in lymphomas: implications for targeted therapy using a novel anti-CD74-drug conjugate. J Pathol Clin Res. (2019) 5:12–24. doi: 10.1002/cjp2.114
118. Burton J, Ely S, Reddy P, Stein R, Gold D, Cardillo T, et al. CD74 is expressed by multiple myeloma and is a promising target for therapy. Clin Cancer Res. (2004) 10:6606–11. doi: 10.1158/1078-0432.Ccr-04-0182
119. Hertlein E, Triantafillou G, Sass E, Hessler J, Zhang X, Jarjoura D, et al. Milatuzumab immunoliposomes induce cell death in CLL by promoting accumulation of CD74 on the surface of B cells. Blood. (2010) 116:2554–8. doi: 10.1182/blood-2009-11-253203
120. Berkova Z, Tao R, Samaniego F. Milatuzumab - a promising new immunotherapeutic agent. Expert Opin Investig Drugs. (2010) 19:141–9. doi: 10.1517/13543780903463854
121. Frölich D, Blassfeld D, Reiter K, Giesecke C, Daridon C, Mei H, et al. The anti-CD74 humanized monoclonal antibody, milatuzumab, which targets the invariant chain of MHC II complexes, alters B-cell proliferation, migration, and adhesion molecule expression. Arthritis Res Ther. (2012) 14:R54. doi: 10.1186/ar3767
Keywords: CD74, MIF, D-DT/MIF2, cardiovascular diseases, invariant chain
Citation: Li Q-L, Tang J, Zhao L, Ruze A, Shan X-F and Gao X-M (2023) The role of CD74 in cardiovascular disease. Front. Cardiovasc. Med. 9:1049143. doi: 10.3389/fcvm.2022.1049143
Received: 20 September 2022; Accepted: 21 December 2022;
Published: 12 January 2023.
Edited by:
Yajing Wang, The University of Alabama at Birmingham, United StatesReviewed by:
Fujie Zhao, The University of Alabama at Birmingham, United StatesCopyright © 2023 Li, Tang, Zhao, Ruze, Shan and Gao. This is an open-access article distributed under the terms of the Creative Commons Attribution License (CC BY). The use, distribution or reproduction in other forums is permitted, provided the original author(s) and the copyright owner(s) are credited and that the original publication in this journal is cited, in accordance with accepted academic practice. No use, distribution or reproduction is permitted which does not comply with these terms.
*Correspondence: Xiao-Ming Gao, eGlhb21pbmdnYW8yMDE3QDE2My5jb20=
Disclaimer: All claims expressed in this article are solely those of the authors and do not necessarily represent those of their affiliated organizations, or those of the publisher, the editors and the reviewers. Any product that may be evaluated in this article or claim that may be made by its manufacturer is not guaranteed or endorsed by the publisher.
Research integrity at Frontiers
Learn more about the work of our research integrity team to safeguard the quality of each article we publish.